- 1Department of Horticulture, Michigan State University, East Lansing, MI, United States
- 2Field Development Representative, Mid-West, FMC Corporation, Plainfield, IL, United States
- 3Department of Plant, Soil and Microbial Sciences, Michigan State University, East Lansing, MI, United States
Liverwort (Marchantia polymorpha) is a problematic weed in ornamental crop production. The major limitation of liverwort control is lack of herbicide options inside greenhouses and their potential to cause injury. The current research was undertaken to study the effectiveness of allelopathic properties of six different organic mulches including rice hull (RH), cocoa hull (CH), pine bark (PB), maple leaf (ML), shredded cypress (SC), and red hardwood (HW) for liverwort control. Mulch extracts were prepared and used to impregnate agar media at an increasing dose at 1X (2 mL mulch extract), 2X (4 mL), 3X (6 mL), and 4X (8 mL) rates, in a randomized complete block design. Ten liverwort gemmae were transferred to each petri dish and they were maintained inside a growth chamber. The number of gemmae germinating in each petri dish after 1 week and gemmae surviving at the end of 2 weeks was recorded. In greenhouse study, mulch extracts were applied to containers filled with substrate for assessing liverwort control. The mulch extracts or no extract (control) were applied to the containers uniformly at 1X (15 mL), 2X (30 mL), 3X (45 mL) or 4X (60 mL) rates, in a randomized complete block design. The percentage of substrate surface covered by liverwort thalli was visually estimated bi-weekly for 10 weeks. Fresh biomass of the thalli and gemmae cup counts in each pot were also recorded. After 1 week in the growth chamber, ML followed by SC, PB and RH extracts showed maximum suppression of liverwort gemmae germination. At 2 weeks, ML applied at either of the rates provided complete inhibition of liverwort growth. In greenhouse, all the mulch extracts were able to provide complete liverwort control for the first two weeks. All the mulches and rates of applications were significantly different from the control after 6, 8, and 10 weeks. PB and HW mulches showed excellent liverwort control and minimum fresh biomass of liverwort after 10 weeks as compared to other mulches. The allelopathic potential of the organic mulches can be a promising option for biopesticidal control of liverwort and a component of integrated liverwort management.
1 Introduction
Common liverwort (Marchantia polymorpha) is a nonvascular, spore-bearing bryophyte in the family Marchantiaceae (Durand, 1908); more closely related to lower plants such as algae, mosses, and ferns than to higher plants such as angiosperms and gymnosperms (Altland, 2003; Svenson et al., 1997). In the northeast and pacific northwest of the United States, it is considered one of the most problematic weeds in containerized greenhouse and nursery production (Newby et al., 2006). Liverwort propagates by both asexual (gametophytic) and sexual (sporophytic) means of reproduction. Sporophytic reproduction occurs at cooler temperatures (10 to 15°C), due to the development of sexual structures – antheridia (male) and archegonia (female). The sperm cells from antheridia fertilize the egg cells of archegonia, resulting in the formation of diploid, sexual spores. Gametophytic reproduction occurs at warmer temperatures (18 to 22°C) and results from the formation of gemma cups which contain numerous gemmae within them (Newby et al., 2006; O’Hanlon, 1926). Gemmae are small clumps of dispersible somatic cells that are able to regenerate liverwort thallus after they are scattered. Rain and overhead irrigation systems in nurseries and greenhouses can promote the spread of the spores and gemmae by splashing water which spread the infestation to surrounding areas (Svenson et al., 1997). Liverwort thrives best in environments with high humidity and/or soil moisture, low ultraviolet radiation, and high fertility (Newby et al., 2006). In containerized production, liverwort competes with ornamental crops for water, nutrients, and other resources intended for the crop, obstructs water and fertilizer movement into the root-zone and reduces market value and overall crop quality (Svenson et al., 1997). Therefore, controlling liverwort in containerized greenhouse and nursery production systems is very important.
The major limitation for liverwort control in greenhouses is that hand-weeding is highly laborious and not economical, as it forms a mat like structure on the top of the container, which is very difficult separate from the substrate. Additionally, there are limited organic or synthetic herbicides labeled for use in greenhouses as they can volatilize and cause severe injuries to sensitive ornamentals. Additionally, only a few studies have quantified the efficacy of herbicides or biopesticides on weed control in nursery and greenhouse operations due to low financial incentives for chemical companies. Therefore, allelochemicals or biopesticides can be a promising option and an effective, sustainable, and environment-friendly substitute to chemical weed control.
Many plants produce allelochemicals, which are secondary metabolites such as terpenoids, hydroxylated aromatic compounds, and phenolics that are produced through various metabolic pathways that effect the growth of other plants (Duryea et al., 1999; Singh et al., 2003; Farooq et al., 2020). These chemicals often act as germination or growth inhibitors for surrounding plants, providing a competitive advantage for the plant that produces the allelochemical (Farooq et al., 2020; Hadacek, 2002; Jabran, 2017; Jabran et al., 2015). Various organic mulches have been identified that possess allelopathic properties such as pine bark, shredded wood chips, black walnut wood chips, red maple leaves and shredded cypress (Rathinasabapathi et al., 2005; Duryea et al., 1999; Henschke and Politycka, 2016; Stein, 1988). Santos et al. (2013) identified the presence of phenolic compounds – catechin, quinic acid, gallic acid, protocatechuic acid, and chlorogenic acid; that were responsible for allelopathic nature of eucalyptus hardwood. Pine bark mulch possess allelopathic properties due to the presence of monoterpenes, pinenes, camphene, and carene that can inhibit germination and growth, of weed seeds (Harman-Ware et al., 2016). Therefore, allelochemicals have the potential to act as natural herbicides or biopesticides and can be used for weed management in ornamental crop production. Using natural products instead of synthetic chemicals could be beneficial because of their ability to readily decompose and there is no risk of volatilization or drift. In addition, many allelochemicals may have different modes of action than chemical herbicides, thus providing alternative sites of action for weed control. This can help in tackling herbicide resistance issues that are becoming ever more prevalent in weedy populations (Duke et al., 2000; Dayan et al., 1999; Marble et al., 2015). Previous studies have focused on allelopathic effects of mulch extracts for controlling broadleaves weeds or grasses but not on lower plants such as liverwort. The objective of this research was to assess the allelopathic effects of different organic mulch materials on liverwort gemmae germination and growth.
2 Materials and methods
2.1 Mulch extract preparation
During the summer and fall 2022, six different mulch materials (aged for 6–12 months) including rice hull (RH) (Nature’s Seed, Lehi, UT), cocoa hull (CH) (Hull Farm, Lake Geneva, WI), pine bark (PB) (Timberline, Marion, IA), maple leaf (ML) (collected leaves, Lansing, MI), shredded cypress woodchips (SC) (NoFloat cypress blend, Oldcastle Lawn and Garden, Atlanta, GA) and red hardwood chips (HW) (Timberline, Marion, IA) were used to obtain mulch extracts. Mulch extracts were prepared by following the modified EPA 1312 synthetic precipitation procedure (method adopted from www.epa.gov). Mulch materials were crushed and ground to <9.5 mm in size. An extraction fluid was prepared by adding a 60/40 weight percent mixture of concentrated sulfuric and nitric acids to deionized ASTM type II water maintaining a pH of 4.2 ± 0.05 using a pH meter (Accumet® Portable Laboratory, Fisher Scientific, Waltham, MA). The extraction fluid was added to extraction bottles containing mulch materials in 20:1 (extraction fluid: mulch) ratio. The extraction bottle was then allowed to rest for 18 h, and thereafter the extract was filtered out through a 20 micron, 7 cm diameter, qualitative filter paper (USA Labs, Livonia, MI). This liquid extract was collected separately for RH, HW, CH, ML, SC, and PB mulch materials.
2.2 Laboratory and growth chamber experiment
The mulch extracts obtained above were used to impregnate agar media each at increasing doses (1X, 2X, 3X, and 4X rates). The agar culture media was prepared by adding 23 g nutrient agar in 1 L of room temperature distilled water and sterilized in an autoclave at a steam pressure of 0.004-kilogram per square meter (121°C) for 20 minutes (Consolidated Sterilizer Systems autoclave, Boston, MA). As the media cooled down to ~50°C, the mulch extracts of either RH, HW, CH, ML, SC and PB mulches were added to the solution at either 1X (2 mL mulch extract in 25 mL media), 2X (4 mL mulch extract in 25 mL media), 3X (6 mL mulch extract in 25 mL media), and 4X (8 mL mulch extract in 25 mL media) rates (where X = 2 mL). According to methods outlined in Saha (2019), 2 mL of mulch extract was added to 25 mL agar media in 47 mm diameter petri dishes for studying allelopathic effects of organic mulches on broadleaf weed seed germination. Therefore, 2 to 8 mL of mulch extracts were applied to each plate. Approximately 25 mL of media containing the mulch extract was transferred to sterile petri plates under the laminar air flow (Nuaire laminar Flow Products, Plymouth, MN), to obtain mulch extract impregnated agar media.
The liverwort gemmae were collected from stock plants maintained in a greenhouse (air temperature ranging from 21 to 26.6°C, with an average daily temperature of 23.8°C). The gemmae were collected from the gemmae cups using forceps and transferred to a vial containing a few drops of distilled water. For sterilization of the gemmae, another vial was filled with 1:30 bleach (Clorox, Oakland, CA) water solution, with a small amount of detergent added in order to break the surface tension of the floating gemmae and wash them thoroughly. This solution was quickly added to the first vial, and the contents were poured back and forth a few times. It was allowed to stand nearly 5 minutes and then the bleach solution containing gemmae was poured into a funnel lined with filter paper. The gemmae collected in the filter paper were then rinsed with 100 mL of distilled water, to obtain sterile gemmae to be transferred to the culture medium (Miller, 1964). Ten gemmae were transferred to the culture medium in each petri dish containing mulch-extract impregnated agar media at an increasing dose. All petri dishes containing gemmae were maintained inside a 0.42 m2 growth chamber (Percival Scientific, Inc, Perry, IA). Cool-white fluorescent lamps (Philips model F17T8/TL741-17 watt, Cambridge, MA) mounted 20 cm above each shelf provided an average photosynthetic photon flux density (PPFD) of 72 µmol·m–2·s–1 during the 16-h photoperiod for 3 weeks. The air temperature above the petri plates was 20°C during the light/dark period. The petri plates were observed for liverwort germination and vegetative growth.
Data were recorded for number of gemmae germinating in each petri dish after 1 week and germination percentage was calculated using the following formula:
After 2 weeks, the number of gemmae surviving were counted and survival percentage was calculated using the following formula:
The experiment was conducted in 7 × 4 factorial treatment arrangement with seven treatments of mulch extracts including control, and 4 rates of application in a randomized complete block design on four shelves of a growth chamber. There were four petri plate replications per treatment. The experiment was repeated twice but there was no significant difference between data obtained from two runs. Therefore, combined data from run 1 and 2 were analyzed by PROC GLIMMIX in SAS (Ver. 9.4, SAS Institute, Cary, NC) to conduct the Analysis of Variance (ANOVA) to determine the effects of mulch extracts, and their rates of application; and the interactions of these variables on data collected for various experimental parameters. The replications were considered as random effects and mulch extracts, and their rates of application were considered fixed effects. When the results showed significant effects, mean comparisons for fixed factors were performed using Tukey’s honest significant differences (HSD) test at alpha=0.05.
2.3 Greenhouse experiment
The experiment was conducted in a greenhouse with a double-sided polyethylene roof and polycarbonate side walls in 2022 at the Michigan State University Horticulture Teaching and Research Center. The air temperature ranged from 21 to 26.6°C, with an average daily temperature of 23.8°C. The greenhouse study allowed for all conditions such as temperature, irrigation, pest pressure, other weed competition to be controlled and only the treatments to be varied (Gallina et al., 2023). The mulch extracts obtained in the laboratory experiment were utilized in the container liverwort control study. Square plastic containers (East Jordan Plastics Inc., East Jordan, MI), 10.5 cm (width) × 11.4 cm (height), were filled with commercial soilless media containing 70% peat moss, 21% perlite, and 9% vermiculite (Suremix, Michigan Grower Products Inc., Galesburg, MI) and amended with 7-5-11 controlled release fertilizer (Osmocote 8 to 9 month, ICL Specialty Fertilizers, Dublin, OH) at the manufacturer’s labeled medium rate of 7.1 g/L. RH, HW, CH, ML, SC or PB mulch extracts were sprayed uniformly over the top of the substrate using a hand-held sprayer, at 1X (15 mL of mulch extract solution), 2X (30 mL), 3X (45 mL) or 4X (60 mL) rates. These extracts were also applied bi-weekly until the end of the experiment. To simulate a greenhouse environment, liverwort plants were placed between the pots to serve as a source of inoculum to spread the gemmae by overhead irrigation. Overhead irrigation of 1.2 cm was applied daily. A control set without any mulch extract application was included as well.
The percentage of substrate surface covered by liverwort thalli in each container were visually estimated after 2, 4, 6, 8 and 10 weeks from the beginning of the experiment, based on a scale 0 to 100% (where 0% was no liverwort coverage and 100% was liverwort coverage on the substrate surface). After 10 weeks, the number of gemmae cups and fresh biomass of the liverwort thalli were recorded. Liverwort thalli from each pot were separated from the substrate and placed into individual paper bags and weighed.
The experiment was conducted in a 7 × 4 factorial treatment arrangement with seven treatments of mulch extracts including control, and 4 rates of application in a randomized complete block design. There were four single-container replications per treatment. All data were analyzed by PROC GLIMMIX in SAS (Ver. 9.4, SAS Institute, Cary, NC) to conduct the Analysis of Variance (ANOVA) to determine the effects of mulch extracts, and their rates of application; and the interactions of these variables on data collected for various experimental parameters. The replications were considered as random effects and mulch extracts, and their rates of application were considered fixed effects. When the results showed significant effects, mean comparisons for fixed factors were performed using Tukey’s honest significant differences (HSD) test at alpha=0.05.
3 Results
3.1 Effects of allelopathic properties of organic mulch extracts on liverwort gemmae germination and growth in agar-impregnated media
The effects of mulch extracts (p <0.0001) and rate of application (p < 0.0001) significantly impacted liverwort gemmae germination (Tables 1, 2). The untreated control showed 80% gemmae germination while all the mulch extract applications provided significantly lower germination. The ML extract reduced the germination of gemmae the most (25.6%) when compared to other mulch extracts and the control (Table 1). Across all mulch extracts, higher rates of application were generally more effective at reducing liverwort germination than lower rates (p < 0.0001). Liverwort germination was 46.9, 34.4, 34.4, and 30.2% for the 1X, 2X, 3X, and 4X rates of application, respectively (Table 2). After 2 weeks, significant interactions were observed between the mulch extract and the rates of application (p <0.05) at reducing the survival of liverwort gemmae (Table 3, Figure 1). All the mulch extracts applied from 1X to 4X rates were significantly influential in limiting liverwort survival below 50%, in comparison to the control that had 73.7% survival. The ML extract at all rates, SC extract at the 2X rate and RW at the 4X rate provided 100% suppression of liverwort growth. All other mulch extract applications provided a marginal control ranging from 5 to 48% (Table 3, Figure 1).
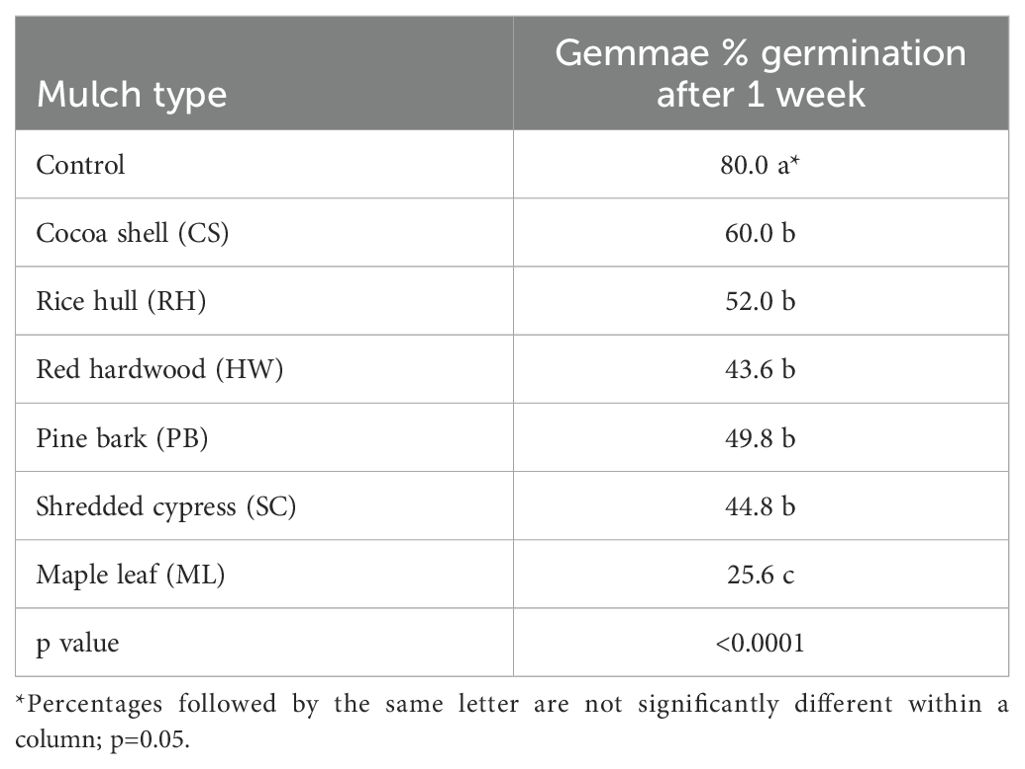
Table 1. Liverwort gemmae germination (%) in petri plates containing agar media impregnated with organic mulch extracts (cocoa shell, maple leaf, pine bark, red hardwood, rice hull, and shredded cypress) after 1 week.
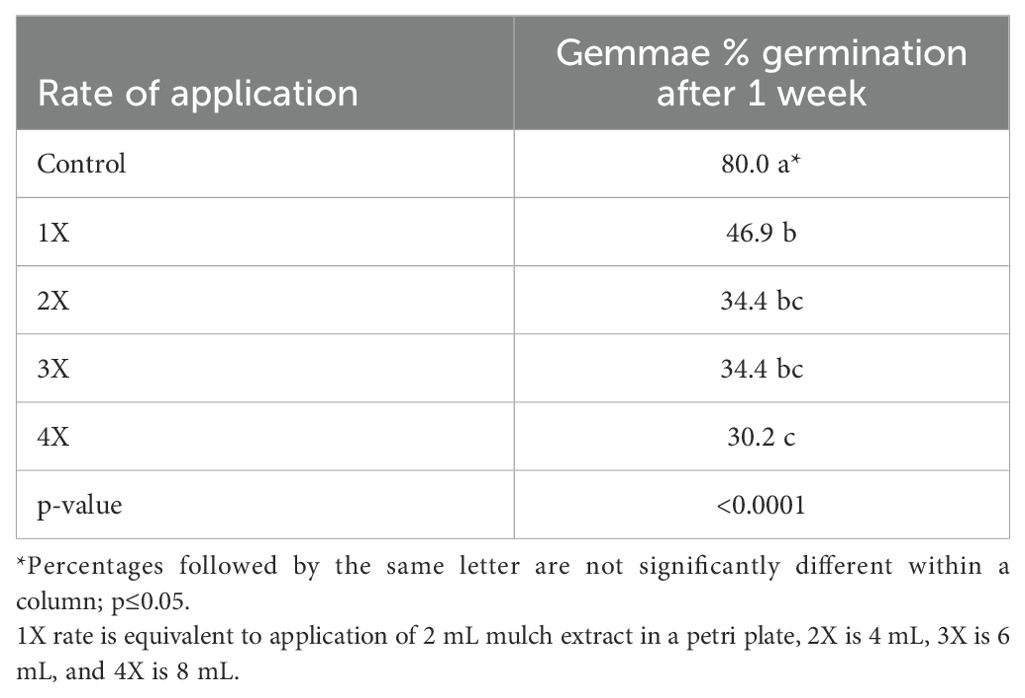
Table 2. Liverwort gemmae germination (%) in petri plates containing agar media impregnated with organic mulch extracts at four different rates (1X, 2X, 3X and 4X) after 1 week.
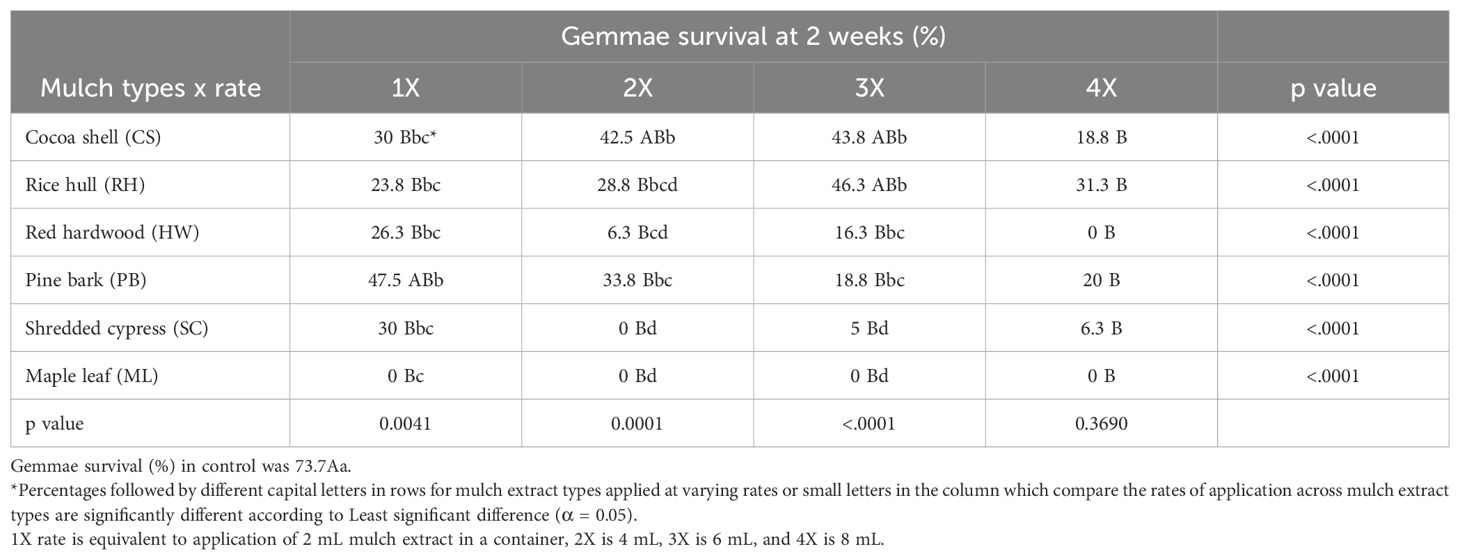
Table 3. Liverwort gemmae survival (%) in petri plates containing agar media impregnated with organic mulch extracts at four different rates at 2 weeks.
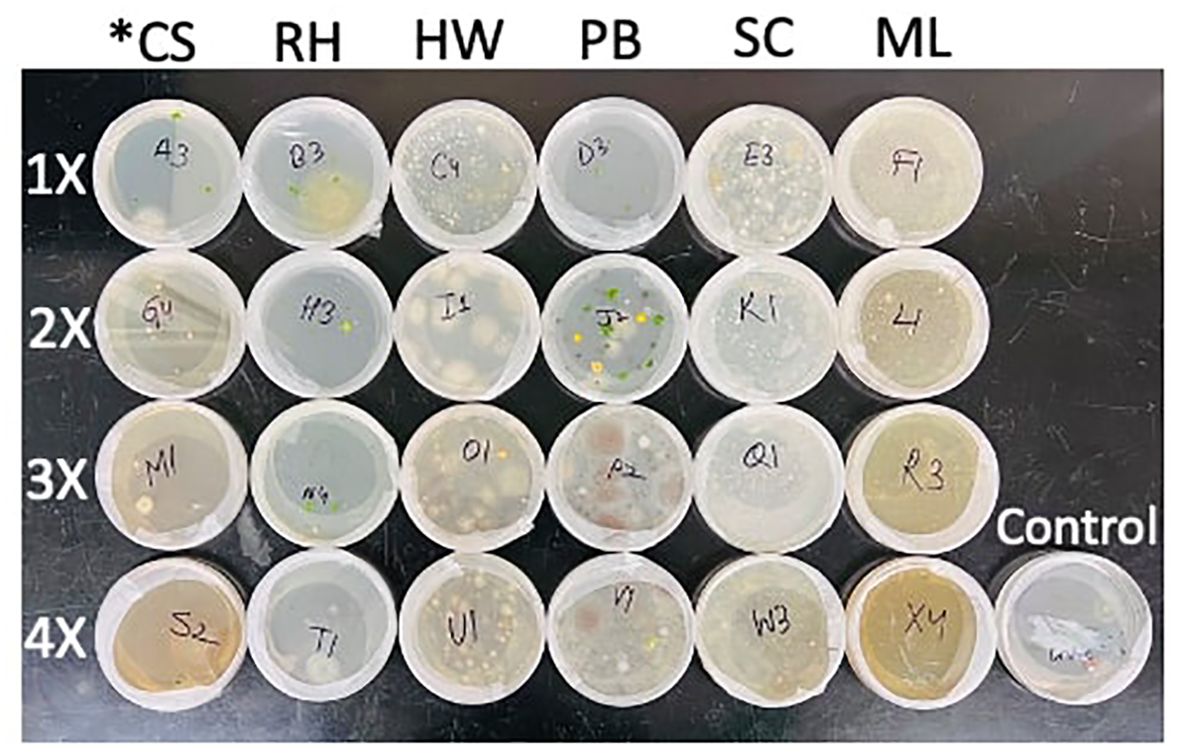
Figure 1. Liverwort gemmae germination in petri plates containing agar media impregnated with organic mulch extracts (*cocoa shell, maple leaf, pine bark, red hardwood, rice hull, and shredded cypress) after 2 weeks. 1X rate is equivalent to application of 2 mL mulch extract in a container, 2X is 4 mL, 3X is 6 mL, and 4X is 8 mL.
3.2 Effects of allelopathic properties of organic mulch extracts on growth of liverwort gemmae in containers
The interaction among the mulch extract types and rate of application were non-significant (p ≥ 0.05) for liverwort thallus coverage in containers from 4 to 10 weeks, fresh biomass of thallus, and number of gemmae cups; Therefore, results are presented based on the main effects (Tables 4, 5). All of the mulch extracts and rates tested provided 100% liverwort control for the initial two weeks. The mulch extracts were significantly different from untreated control for their effects on liverwort coverage, number of gemmae cups produced and fresh biomass recorded of liverwort thallus (p <0.05). After 6 weeks, there was 4.0% liverwort coverage on containers receiving PB mulch extract, followed by containers receiving SC, HW, ML, and RH mulch extracts, while the CS extract was the least effective (Table 4). After 8 weeks, CS and RH provided minimal control, while all other mulch extract were effective at controlling liverwort growth and limiting the percent liverwort coverage over the top of substrate to 13 to 21%. At the end of the experiment, HW and PB mulch extracts were the most effective, limiting the liverwort coverage to 26 to 31%, followed by SC (41%) and ML extracts (46%) (Table 4, Figure 2).
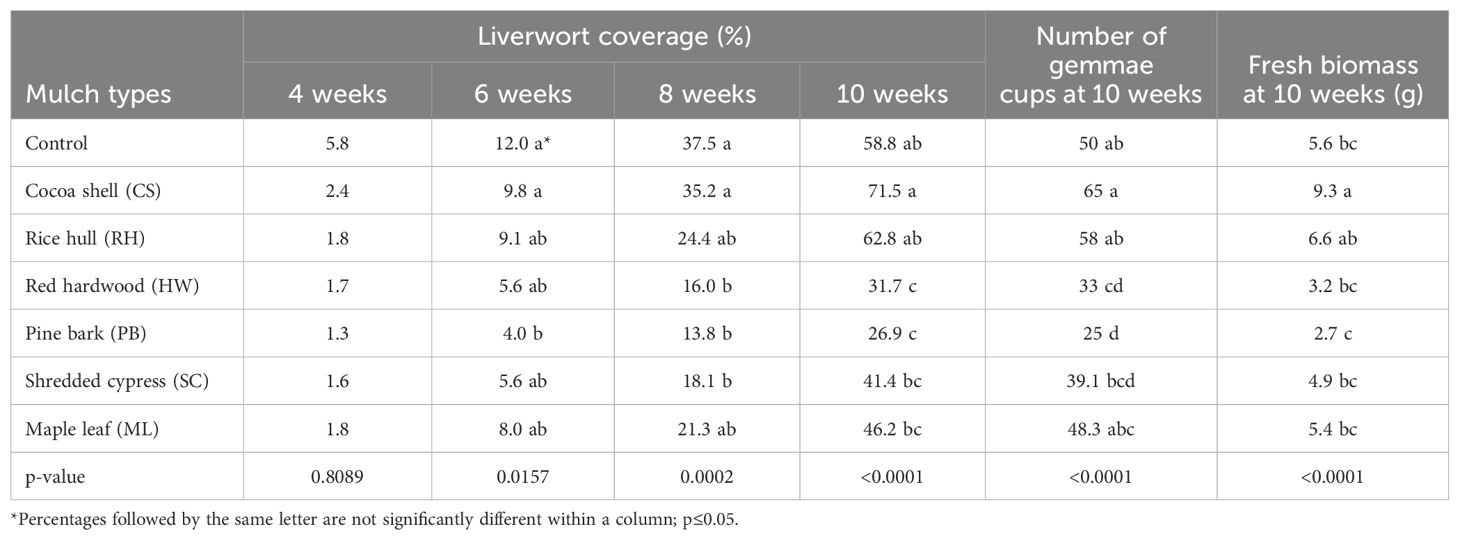
Table 4. Liverwort thallus coverage (%) after 4, 6, 8, and 10 weeks, fresh weigh of thallus (grams) at 10 weeks and number of gemmae cups at 10 weeks in containers treated with organic mulch extracts (cocoa shell, maple leaf, pine bark, red hardwood, rice hull and shredded cypress).
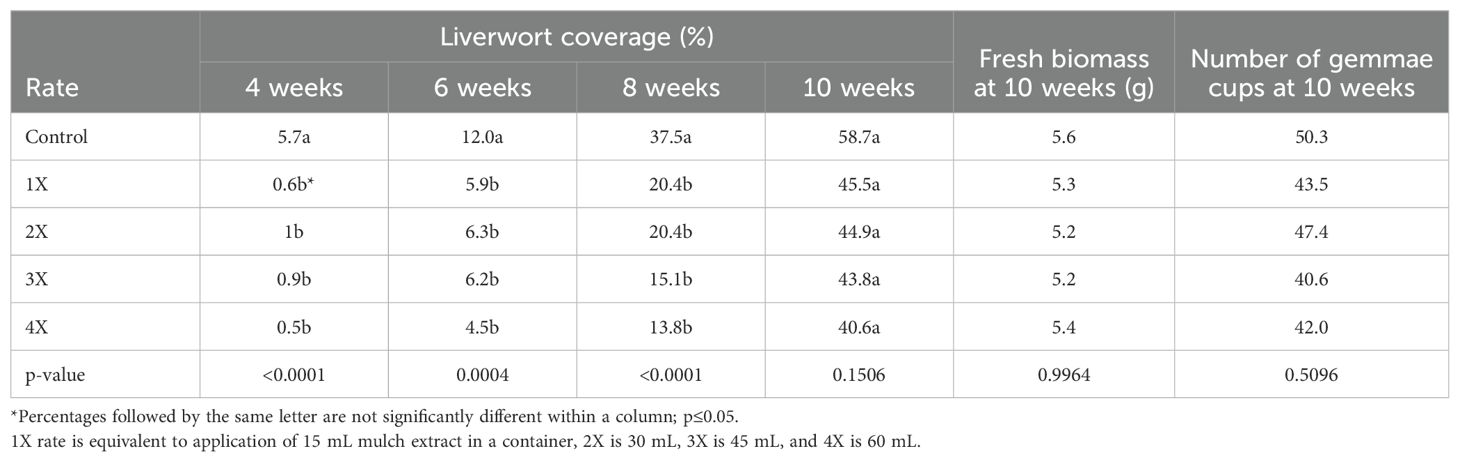
Table 5. Liverwort thallus coverage (%) at 4, 6, 8, and 10 weeks, fresh biomass of thallus (grams) at 10 weeks and number of gemmae cups at 10 weeks in containers treated with organic mulch extracts at four different rates (1X, 2X, 3X and 4X).
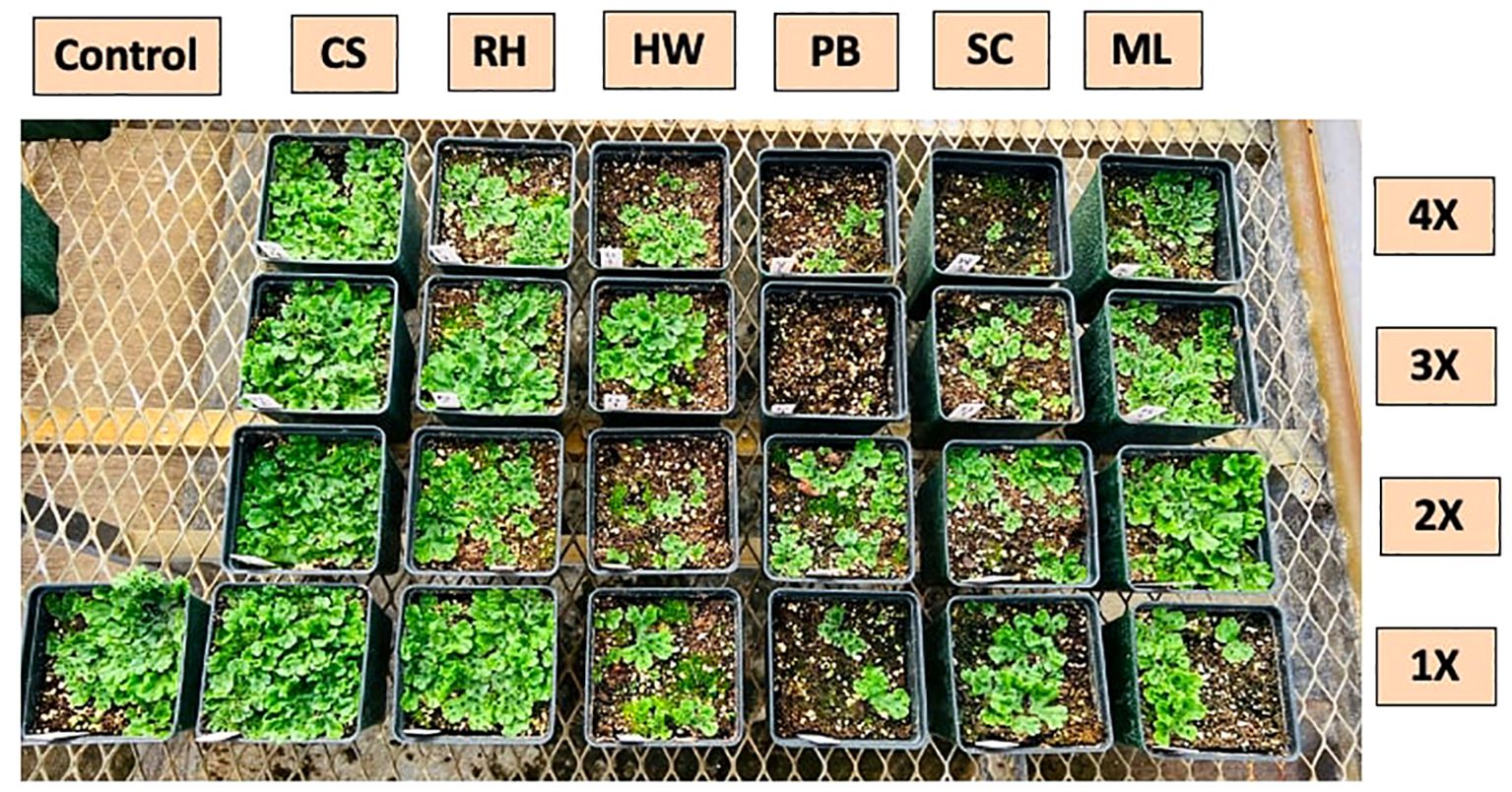
Figure 2. Liverwort thallus coverage (%) after 4, 6, 8, and 10 weeks, fresh weigh of thallus (grams) at 10 weeks and number of gemmae cups at 10 weeks in containers treated with organic mulch extracts (cocoa shell, maple leaf, pine bark, red hardwood, rice hull and shredded cypress) at different rates (1X, 2X, 3X and 4X). 1X rate is equivalent to application of 15 mL mulch extract in a container, 2X is 30 mL, 3X is 45 mL, and 4X is 60 mL.
The number of gemmae cups recorded was influenced by the type and application rate of mulch extracts. The containers that received PB (25) extract had the least number of gemmae cups, followed by the containers receiving HW (33) and SC (39) mulch extracts. The CS extract minimally affected the production of gemmae cups. Similarly, the fresh biomass of liverwort thallus was lowest (2.6 g) when containers were treated with PB extract providing the minimal fresh biomass (2.7 g), followed by HW (3.2 g) and SC (4.9 g). The fresh biomass of liverwort thallus in containers treated with CS mulch extracts was highest (9.3 g), compared to control (5.6 g) and all other mulches (Table 4).
The Liverwort percent coverage was impacted by the various rates of mulch extract applications when compared with the control, but they were not different amongst themselves after 4, 6, and 8 weeks. All the rates of application had no effect on gemmae cup production and recorded fresh biomass of liverwort thallus at the end of the experiment (p >0.05) (Table 5).
4 Discussion
4.1 Effects of allelopathic properties of organic mulch extracts on liverwort gemmae germination and growth in agar-impregnated media
Our results showed that the mulch extracts from maple leaves, shredded cypress, pine bark, red hardwood and rice hulls provided inhibition of liverwort gemmae germination and suppressed its growth over time. The mulch extract compounds derived from various organic mulches such as cypress, PB, walnut (Juglans regia) leaves and hardwood are known to be phytotoxic to weed seeds and have helped to achieve improved weed control efficacy in previous studies (Đorđević et al., 2022; Khamare et al., 2022; Farooq et al., 2020; Iqbal et al., 2020). Saha et al. (2018) observed that allelochemicals present in PB mulch inhibited germination and growth of weed seeds, due to the potential presence of β-pinene and camphene in the mulch. Rathinasabapathi et al. (2005) showed that mulch eluates from shredded wood chips of red cedar (Juniperus silicicola), red maple (Acer rubrum), neem (Azadirachta indica), swamp chestnut oak (Quercus michauxii), and magnolia (Magnolia grandiflora L.) inhibited germination and growth of seeds in a lettuce bioassay. Additionally, the inhibition of growth of hypcotyl and radicle were higher under the application of black walnut wood chips (Juglans nigra). Inhibitory allelochemicals were also found to be present in leaves of red maple (Acer rubrum) apart from their activity in the wood chips (Rathinasabapathi et al., 2005). In another study, Duryea et al. (1999) reported that the allelopathic effects of PB and cypress mulches were due to hydroxylated aromatic compounds that inhibited lettuce seed germination in a standard germination test. In a study conducted by Henschke and Politycka (2016), the phenolic compounds from PB mulch adversely affected the growth and flowering of ornamental grasses during first year of the study, but the effect diminished during the consecutive year. Another study by Stein (1988) concluded that mulch leachate from red maple leaves inhibited weed seed germination of pigweed (Amaranthus retroflexus) and morning glory (Ipomoea purpurea). Li et al. (2021) reported the allelopathic effects of Chinese mugwort (Artemisia argyi) on weed seed germination in incubator and field conditions. Further RNA-Seq analysis of the extracts indicated the suppression was due to multi-target and multi-path inhibition, in addition to the inhibition of chlorophyll synthesis, the key mechanism causing inhibition of weed seeds germination.
4.2 Effects of allelopathic properties of organic mulch extracts on growth of liverwort gemmae in containers
The application rate of mulch extracts was found to be effective for liverwort control. The higher rates of application were more effective than the lower rates for most mulch extract materials, with the exception of RH, where a lower rate was more effective. We postulate that higher application rates of some organic mulch extracts may have opposite or complimentary effects on seed growth or have no further effect on inhibition of seed germination. Another possible explanation could be that the lower rates of mulch application undertaken in the current study (1X, 2X, or 3X) caused the inhibition/suppression of liverwort. Therefore, future studies need to be conducted to validate the effects of different rates of application of specific types of organic mulch extracts and identifying the underlying chemical compounds that reduce liverwort gemmae germination and growth.
The application of organic mulch derived allelochemical extract had a quite significant impact on controlling liverwort gemmae germination and growth, specifically early in the experiment, when all the mulch extracts were equally effective in their activity. Their activity declined over time, and liverwort gemmae were able to utilize available resources and establish themselves. However, different mulch extract treatments and rates of application performed markedly better in comparison to the control. Previously, several phenolic acids and similar compounds having allelopathic properties have been identified in PB and needles and in soils from pine tree communities (Lee and Monsi, 1963; Kil and Yim, 1983; Node et al., 2003). Allelopathic extracts of red pine needles (Pinus densiflora) have been shown to inhibit the root and shoot growth of various weeds such as cress (Cardamine hirsuta), ryegrass (Lolium perenne), large crabgrass (Digitaria sanguinalis), barnyard grass (Echinichloa crus-galli) and timothy-grass (Phleum pratense). Also, increasing the doses of these extracts led to increased inhibition of root and shoot growth of weeds (Kato-Noguchi et al., 2009). Nektarios et al. (2005) reported that phenolics present in pine needles and straw of Aleppo pine (Pinus halepensis) inhibited growth of bermudagrass and tall fescue. Our results align with these previous research results which show that pine tree products (bark, straw, needles, etc.) contain allelochemicals that can control weeds. Allelopathic properties of rice have been reported to have an adverse impact on development and establishment of weeds, which could be used as a biochemical tool for integrated weed management (Serra Serra et al., 2021; Rahaman et al., 2022). Nikolai et al. (1998) reported that maple (Acer spp.) and oak (Quercus spp.) tree leaf litter could be mulched into turfgrass without any deleterious effects on turfgrass quality and color. Further, Kowalewski et al. (2009) found that the application of leaf litter from silver maple (Acer saccharinum), red maple (Acer rubrum), sugar maple (Acer saccharum), high-sugar-content sugar maple, and red oak (Quercus rubra) as mulch was effective in reducing the incidence of common dandelion (Taraxacum officinale) populations in a Kentucky bluegrass (Poa pratensis) stand. The application of these mulches resulted in an 80 and 53% reduction in common dandelion counts after one and two applications, respectively. In a study conducted by Cheema (1988), sorgholene, a water-soluble compound in sorghum has been found to inhibit the growth of cannarygrass (Phalaris canariensis), lambsquarters (Chenopodium album), bindweed (Convovulus arvensis), toothed duck (Rumex dentatus). Mulching with sorghum also resulted in significant weed control, reducing growth or purple nutsedge (Cyperus rotundus) by 38–41% (Cheema et al., 2004). Sorgholene is very effective in controlling weeds especially lower plants, by affecting their photosynthesis (Ashraf et al., 2017). Matloob et al. (2010) reported that soil incorporation of brassica, sunflower or sorghum residues suppressed sprouting and growth of horse purslane (Trianthema portulacastrum) and purple nutsedge (Cyperus rotundus) in container production system. Rathinasabapathi et al. (2005) also found an allelopathic potential of red cedar wood chips significantly suppressed Florida beggarweed (Desmodium tortuosum) in a greenhouse, as compared to gravel-mulching and non-mulch control.
In the laboratory study, the ML and SC extracts were most impactful in reducing the germination and growth of liverwort gemmae in agar-impregnated with mulch extracts. However, in the greenhouse study, PB and HW, followed by ML and SC provided good results in comparison to other mulch extract types and the control. The slight reduction in activity of ML and SC mulch extracts in the greenhouse study could be due to various reasons. Some possible explanations could be that inside the greenhouse, the overhead irrigation system may have resulted in leaching down the allelochemicals, or they may have become bound to the substrate, or decomposed by microbial activity in the substrate.
5 Conclusion
In conclusion, mulch extracts of PB, HW, ML, and SC possess allelopathic properties that can reduce or suppress liverwort growth and gemmae germination. These allelochemicals could be a promising option in the integrated liverwort management in containerized greenhouse production. This study is a novel piece of research in the field of liverwort management in containerized ornamental production and results from the current research could serve as a source of information for future investigations on the allelopathic control of liverwort. Further research is needed to study other potential organic mulches for their allelochemical properties, evaluating their phytotoxic effects on ornamental plants, to identify the specific chemicals present in these extracts which are responsible for the allelopathy, and to analyze deeply the reasons behind discrepancies between the results of lab and greenhouse studies. Future studies also need to focus on investigating the effects of allelopathic compounds under other modes of irrigation such as drip or sub-surface irrigation, in comparison to overhead sprinkler system. Upon confirmation by further studies, these allelopathic compounds could provide a clue to developing newer modes of action for prospective herbicides or could be commercialized as bioherbicides for liverwort control inside greenhouse conditions.
Data availability statement
The raw data supporting the conclusions of this article will be made available by the authors, without undue reservation.
Author contributions
MS: Formal analysis, Investigation, Methodology, Validation, Writing – original draft. SC: Writing – review & editing. RL: Writing – review & editing. EP: Writing – review & editing. DS: Conceptualization, Funding acquisition, Investigation, Methodology, Project administration, Resources, Supervision, Validation, Visualization, Writing – review & editing.
Funding
The author(s) declare financial support was received for the research, authorship, and/or publication of this article. Michigan Department of Agriculture and Rural Development Horticulture Fund 2023-24 (Grant number 230000002558) and Western Michigan Greenhouse Association (WMGA) fund 2022.
Acknowledgments
We would like to acknowledge our undergraduate research assistants, Mckinleigh Cordhal and Shriya Kethireddy, who helped in setting up the experiments in the laboratory, growth chamber, and greenhouse.
Conflict of interest
SC was employed by FMC Corporation.
The remaining authors declare that the research was conducted in the absence of any commercial or financial relationships that could be construed as a potential conflict of interest.
Publisher’s note
All claims expressed in this article are solely those of the authors and do not necessarily represent those of their affiliated organizations, or those of the publisher, the editors and the reviewers. Any product that may be evaluated in this article, or claim that may be made by its manufacturer, is not guaranteed or endorsed by the publisher.
References
Altland J. (2003). Weed control in container crops: A guide to effective weed management through preventive measures (Oregon State university Extension Service: Oregon State University Extention). Available at: https://oregonstate.edu/dept/nursery-weeds/feature_articles/EM8823.
Ashraf R., Sultana B., Yaqoob S., Iqbal M. (2017). Allelochemicals and crop management: A review. Curr. Sci. 3, 1–13.
Cheema Z. A. (1988). Weed control in where through sorghum allelochemicals. Ph.D. Thesis. Department Agronomy, University of Agriculture, Faisalabad.
Cheema Z. A., Khaliq A., Saeed S. (2004). Weed control in maize (Zea mays L.) through sorghum allelopathy. J. Sust. Agric. 23, 73–86. doi: 10.1300/J064v23n04_07
Dayan F. E., Romagni J., Tellez M., Rimando A., Duke S. (1999). Managing weeds with natural products. Pestic. Outlook. 10, 185–188.
Đorđević T., Đurović-Pejčev R., Stevanović M., Sarić-Krsmanović M., Radivojević L., Šantrić L., et al. (2022). Phytotoxicity and allelopathic potential of Juglans regia L. leaf extract. Front. Plant Sci. 13, 986740. doi: 10.3389/fpls.2022.986740
Duke S. O., Romagni J. G., Dayan F. E. (2000). Natural products as sources for new mechanisms of herbicidal action. Crop Protec. 19, 583–589. doi: 10.1016/S0261-2194(00)00076-4
Durand E. J. (1908). The development of sexual organs and sporogonium of Marchantia polymorpha. Bull. Torrey Botani. Club 35, 321–335. doi: 10.2307/2485335
Duryea M. L., English R. J., Hermansen L. A. (1999). A comparison of landscape mulches: chemical, allelopathic, and decomposition properties. Arboric. J. 25, 88–97. doi: 10.48044/jauf.1999.014
Farooq N., Abbas T., Tanveer A., Jabran K. (2020). Allelopathy for weed management. In book: Co-evolution of secondary metabolites. (New York: Springer), 505–519.
Gallina G., Cregg B., Patterson E., Hill E., Saha D. (2023). Alternative integrated weed management options for clopyralid-resistant common ragweed. Hortic 9, 985. doi: 10.3390/horticulturae9090985
Hadacek F. (2002). Secondary metabolites as plant traits: current assessment and future perspectives. Crit. Rev. Plant Sci. 21, 273–322. doi: 10.1080/0735-260291044269
Harman-Ware A. E., Sykes R., Peter G. F., Davis M. (2016). Determination of terpenoids content in pine by organic solvent extraction and fast-GC analysis. Front. Energy Res. 4. doi: 10.3389/fenrg.2016.00002
Henschke M., Politycka B. (2016). Application of wood chips for soil mulching in the cultivation of ornamental grasses. Folia Hortic. 28, 187. doi: 10.1515/fhort-2016-0022
Iqbal R., Raza M. A. S., Valipour M., Saleem M. F., Zaheer M. S., Ahmad S., et al. (2020). Potential agricultural and environmental benefits of mulches—a review. Bull. Nat. Res. Cen. 44, 1–16. doi: 10.1186/s42269-020-00290-3
Jabran K. (2017). Manipulation of allelopathic crops for weed control (Cham: Springer International Publishing), 65–75.
Jabran K., Mahajan G., Sardana V., Chauhan B. S. (2015). Allelopathy for weed control in agricultural systems. Crop Protec. 72, 57–65. doi: 10.1016/j.cropro.2015.03.004
Kato-Noguchi H., Fushimi Y., Shigemori H. (2009). An Allelopathic substance in red pine needles (Pinus densiflora). J. Plant Physiol. 166, 442–446. doi: 10.1016/j.jplph.2008.06.012
Khamare Y., Chen J., Marble S. C. (2022). Allelopathy and its application as a weed management tool: A review. Front. Plant Sci. 13, 1034649. doi: 10.3389/fpls.2022.1034649
Kil B. S., Yim Y. J. (1983). Allelopathic effects of Pinus densiflora on undergrowth of red pine forest. J. Chem. Ecol. 8, 1135–1151. doi: 10.1007/BF00982217
Kowalewski A. R., Buhler D. D., Lang N. S., Nair M. G., Rogers J. N. (2009). Mulched maple and oak leaves associated with a reduction in common dandelion populations in established Kentucky bluegrass. HortTech 19, 297–304. doi: 10.21273/HORTTECH.19.2.297
Lee I. K., Monsi M. (1963). Ecological studies on Pinus densiflora forest 1. Effects of plant substances on the floristic composition of the undergrowth. Bot. Mag. 76, 400–413. doi: 10.15281/jplantres1887.76.400
Li D., Chen S. C., Chen B. J., Miao B. J., Peng B. J., Huang B. J., et al (2021). Allelopathic effect of Artemisia argyi on the germination and growth of various weeds. Scientific reports 11 (1), 4303.
Marble S. C., Koeser A. K., Hasing G. (2015). A review of weed control practices in landscape planting beds: part II- chemical weed control methods. Hortic. Sci. 50, 857–862. doi: 10.21273/HORTSCI.50.6.857
Matloob A., Khaliq A., Farooq M., Cheema Z. A. (2010). Quantification of allelopathic potential of different crop residues for the purple nutsedge suppression. Pak. J. Weed Sci. Res. 16, 1–12.
Miller M. W. (1964). A technique for isolating and culturing gemmae of Marchantia polymorpha L. under aseptic conditions. Bryol 67, 317–320.
Nektarios P. A., Economou G., Avgoulas C. (2005). Allelopathic effects of Pinus halepensis needles on turfgrasses and biosensor plants. Hortic. Sci. 40, 246–250. doi: 10.21273/HORTSCI.40.1.246
Newby A., Altland J. E., Gilliam C. H., Wehtje G. (2006). Postemergence liverwort control in container-grown nursery crops. J. Environ. Hortic. 24, 230–236. doi: 10.24266/0738-2898-24.4.230
Nikolai T. A., Rieke P. E., McVay N. T. (1988). Leaf mulch forum “research and real-world techniques.” In 68th Ann. Michigan Turfgrass Conf. Proc 27 (Vol. 66, p. 68).
Node M., Tomita-Yokotani K., Suzuki T., Kosemura S., Hirata H., Hirata K. (2003). Allelopathy of pinecone in Japanese red pine tree (Pinus densiflora Sieb. et Zucc.). Weed Biol. Manage. 3, 111–116. doi: 10.1046/j.1445-6664.2003.00092.x
O’Hanlon M. E. (1926). Germination of spores and early stages in development of gametophyte of Marchantia polymorpha. Bot. Gaz. 82, 215–222. doi: 10.1086/333650
Rahaman F., Shukor Juraimi A., Rafii M. Y., Hassan L., Karim S. M. R., Yusuff O., et al. (2022). Allelopathic potential in rice-a biochemical tool for plant defense against weeds. Front. Plant Sci. 13, 1072723. doi: 10.3389/fpls.2022.1072723
Rathinasabapathi B., Ferguson J., Gal M. (2005). Evaluation of allelopathic potential of wood chips for weed suppression in horticultural production systems. HortSci 40, 711–713. doi: 10.21273/HORTSCI.40.3.711
Saha D., Marble S. C., Pearson B. J. (2018). Allelopathic effects of common landscape and nursery mulch materials on weed control. Front. Plant Sci. 9, 733.
Saha D. (2019). Influence of mulch chemical and physical properties on preemergence herbicide interactions and weed. (Ph.D. Dissertation, Department of Environmental Horticulture, University of Florida, United States) 101–103.
Santos S. A., Vilela C., Freire C. S., Neto C. P., Silvestre A. J. (2013). Ultra-high performance liquid chromatography coupled to mass spectrometry applied to the identification of valuable phenolic compounds from Eucalyptus wood. Journal of Chromatography B 938, 65–74.
Serra Serra N., Shanmuganathan R., Becker C. (2021). Allelopathy in rice: a story of momilactones, kin recognition, and weed management. J. Experi. Bot. 72, 4022–4037. doi: 10.1093/jxb/erab084
Singh H. P., Batish D. R., Kohli R. K. (2003). Allelopathic interactions and allelochemicals: new possibilities for sustainable weed management. Crit. Rev. Plant Sci. 22, 39–311. doi: 10.1080/713610858
Stein K. K. (1988). Allelopathic effect of landscape mulches on the germination of weed seeds and growth of ornamental plants. (Knoxville: MS thesis, University of Tennessee).
Svenson S. E., Smith B., Briggs B. (1997). Controlling liverworts and moss in nursery production. Comb. Proc. Int. Plant Prop. Soc 47, 414–422.
www.epa.gov. Available online at: https://www.epa.gov/hw-sw846/sw-846-test-method-1312-synthetic-precipitation-leaching-procedure. (Accessed February 17, 2024).
Keywords: biopesticides, greenhouse production, hardwood mulch, mulch extract, pine bark mulch, weed control
Citation: Sidhu MK, Chaudhari S, Lopez R, Patterson EL and Saha D (2024) Assessing the effects of allelopathic properties of organic mulches on liverwort control in container-grown ornamentals. Front. Agron. 6:1422292. doi: 10.3389/fagro.2024.1422292
Received: 23 April 2024; Accepted: 15 August 2024;
Published: 02 September 2024.
Edited by:
Lynn M. Sosnoskie, Cornell University, United StatesReviewed by:
Agnieszka Synowiec, University of Agriculture in Krakow, PolandIoannis Roussis, Agricultural University of Athens, Greece
Jatinder Aulakh, Connecticut Agricultural Experiment Station, United States
Copyright © 2024 Sidhu, Chaudhari, Lopez, Patterson and Saha. This is an open-access article distributed under the terms of the Creative Commons Attribution License (CC BY). The use, distribution or reproduction in other forums is permitted, provided the original author(s) and the copyright owner(s) are credited and that the original publication in this journal is cited, in accordance with accepted academic practice. No use, distribution or reproduction is permitted which does not comply with these terms.
*Correspondence: Debalina Saha, sahadeb2@msu.edu