- 1Department of Crop, Soil, and Environmental Sciences, University of Arkansas, Fayetteville, AR, United States
- 2University of Arkansas Systems Division of Agriculture, Lonoke, AR, United States
Cover crops, including cereal rye, are generally known to suppress weeds in various cropping systems; however, research on their effective use is lacking. This study investigated the effects of different cereal rye termination timings on weed control and corn performance in a two-pass herbicide program across 5 site-years (2021–2023) in Arkansas, USA. The herbicide program consisted of one application at cereal rye (Secale cereale) termination (cereal rye environment) or at corn (Zea mays) planting (conventional system) and another at the V4 corn stage. Palmer amaranth (Amaranthus palmeri) at V4 corn was better controlled in cereal rye environments regardless of its termination timing (>75%) than in the conventional corn system (<50%), and the control was generally excellent in both systems 4 weeks later, with subtly greater control in the cereal rye environments. In contrast, yellow nutsedge (Cyperus esculentus) control, in general, was slightly less in the cereal rye environments than in the conventional system. Barnyardgrass (Echinochloa crus–galli) and broadleaf signalgrass (Urochloa platyphylla) control was similar to that of Palmer amaranth, especially at the V4 corn stage, with clear benefits in its control with the later termination timings. Corn height was greater (by 8% to 10%) in cereal rye environments terminated at 1 or 2 weeks after corn planting compared to the conventional system or other cereal rye environments. Corn yield was similar between the conventional system and the cereal rye environments terminated before corn planting; however, the yield was 16% to 22% less with those terminated at or after corn planting. These findings indicate the constrained use of cereal rye in corn, with its termination time optimized for 2 weeks before corn planting. Further research could identify factors that mitigate the yield loss from delayed cereal rye termination and improve its use as a cover crop in corn.
1 Introduction
Less than adequate weed control promotes the perpetual increase in abundance and threatens successful crop production (Oerke, 2006). Herbicides offer economic, efficient, and effective weed control, often with rotational flexibility for major crops, and they have been the mainstay for much of the major crop production systems in the US. However, this approach has led to escalating concerns about selecting for weed resistance to herbicides (Norsworthy et al., 2012). In response to these challenges, the agricultural sector has been compelled to explore additional non-chemical tools to integrate into weed management programs. One longstanding strategy has been the integration of cover crops into crop production systems. Cover crops are implemented between two main crops and are known to provide various ecological services in agro-ecosystems, such as protection against soil erosion, reduction of nutrient losses, improvement of soil and water quality, and the reduction of weeds and pests (Johnson et al., 1993; Blanco–Canqui et al., 2011; Runck et al., 2020), and the integration of cover crops into the crop production systems is increasingly recognized as a sustainable practice with multifaceted benefits (Lal, 2004; Strock et al., 2004; Lin, 2011; Poeplau and Don, 2015; Seifert et al., 2018; Runck et al., 2020).
One factor that has been attracting interest in the use of cover crops in US agriculture in recent years is the increasing number of weed species resistant to herbicides (Osipitan et al., 2018; Heap, 2023). Nonetheless, the potential contribution of cover crops to integrated weed management has long been recognized (Hartwig and Ammon, 2002). Cover crops are known to contribute to weed suppression through various mechanisms. These include competition with weeds for resources while the cover crops are alive (Hartwig and Ammon, 2002), obstructing weed seedling emergence with physical barriers from cover crop residues (Teasdale and Mohler, 2002), limiting light penetration through cover crop residues (Creamer et al., 1996), and displaying selective allelopathic activities (Barnes and Putnam, 1983; Westo, 1996; Caamal-Maldonado et al., 2001). Previous research indicates that combining cover crops with conservation tillage can suppress early-season weeds as effectively as chemical and mechanical methods (Teasdale and Mohler, 1993; Johnson et al., 1993), given the level of weed suppression influenced by factors such as the durability of its residue, the extent of surface coverage, and the management practices of both the cover and main crops (Sainju et al., 2006).
Among cover crops, cereal rye (Secale cereale L.) has gained significant attention for its potential benefits and promising fit to the agroecology of a significant amount of US crop production (Huddell et al., 2024). Cereal rye is a winter grass cover crop and is known to exhibit allelopathic properties; it releases benzoxazinones [2,4-dihydroxy-1,4(2H)-benzoxazin-3-one and 2(3H)-benzoxazolinone] that inhibit the growth of weeds and, with its biomass, acts as a suppressant for small-seeded weeds (Barnes and Putnam, 1983, Barnes and Putnam, 1986; Schulz et al., 2013; Snapp et al., 2005). The potential use of cereal rye as a promising tool for controlling small-seeded weeds in large-seeded row crops, such as corn (Zea mays L.), has long been identified (Burgos and Talbert, 2000; Dhima et al., 2006). Recent research in this area continues to provide encouraging results (Aulakh et al., 2012; Hand et al., 2021; Pittman et al., 2020). However, yield reduction in row crops, especially in corn, has remained a common concern for integrating cereal rye in the cropping system (Tollenaar et al., 1993; Koehler–Cole et al., 2020; Deines et al., 2023) and has been identified as one of the reasons for the limited adoption of winter cereal cover crops in the US, especially in Midwest agriculture.
Much of the research in this regard comes from the Midwest or semiarid regions. Water-limited condition is likely a rare occurrence in the Midwest region (Daigh et al., 2014; Martinez-Feria et al., 2016). However, severe moisture depletion in the rainfed system particularly when coupled with delayed cover crop termination in general has been considered a potential factor that negatively impacts corn yield.
When terminated at an appropriate stage, cereal rye can enhance nutrient cycling by releasing organic matter and nutrients into the soil. However, if termination is delayed excessively, then nitrogen immobilization, a phenomenon where soil microbes sequester nitrogen in the soil, may occur rendering it unavailable to crops. The termination timing is expected to have a substantial impact on weed suppression and corn growth and development as well, typically in direct proportion to the termination delay (Sainju and Singh, 2001; Acharya et al., 2017). Therefore, the hypothesis of this research is that delayed termination will enhance weed suppression. However, finding the right balance in termination timing in the local and regional context is crucial for maximizing the benefits of cereal rye while minimizing its potential drawbacks. The objective of this research is to elucidate essential insights into the effects of different cereal rye termination timings on weed suppression under a conventional two-pass herbicide program and their implications for the crop yield in irrigated corn in Arkansas.
2 Materials and methods
Five–site-year field experiments were carried out in University of Arkansas Division of Agriculture research sites in Arkansas, USA (Fayetteville, Marianna, and Tillar, Figure 1A) during corn growing season in 2021, 2022, and/or 2023. The soil types were Captina silt loam, Loring silt loam, and Hebert silt loam for Fayetteville, Marianna, and Tillar sites, respectively. The experiment was established in a randomized complete block design with four replications. Cereal rye at 67 kg ha−1 was drill-planted the previous fall in October. No supplemental nitrogen was applied to enhance cover crop biomass production. Glyphosate- and glufosinate-resistant corn hybrids (DK 26–69, DEKALB® brand, Bayer Crop Sciences in Fayetteville sites, and P1222YHR, Pioneer® brand, Corteva Agrisciences in Marianna and Tillar sites) were planted 2.5- to 3-cm deep at a seeding rate of 69,000–80,000 seeds ha−1 with a 76- to 80-cm-wide row spacing, depending on the site. Each plot consisted of four corn rows 9 m in length. Corn planting for each site-year is presented in Figure 1A.
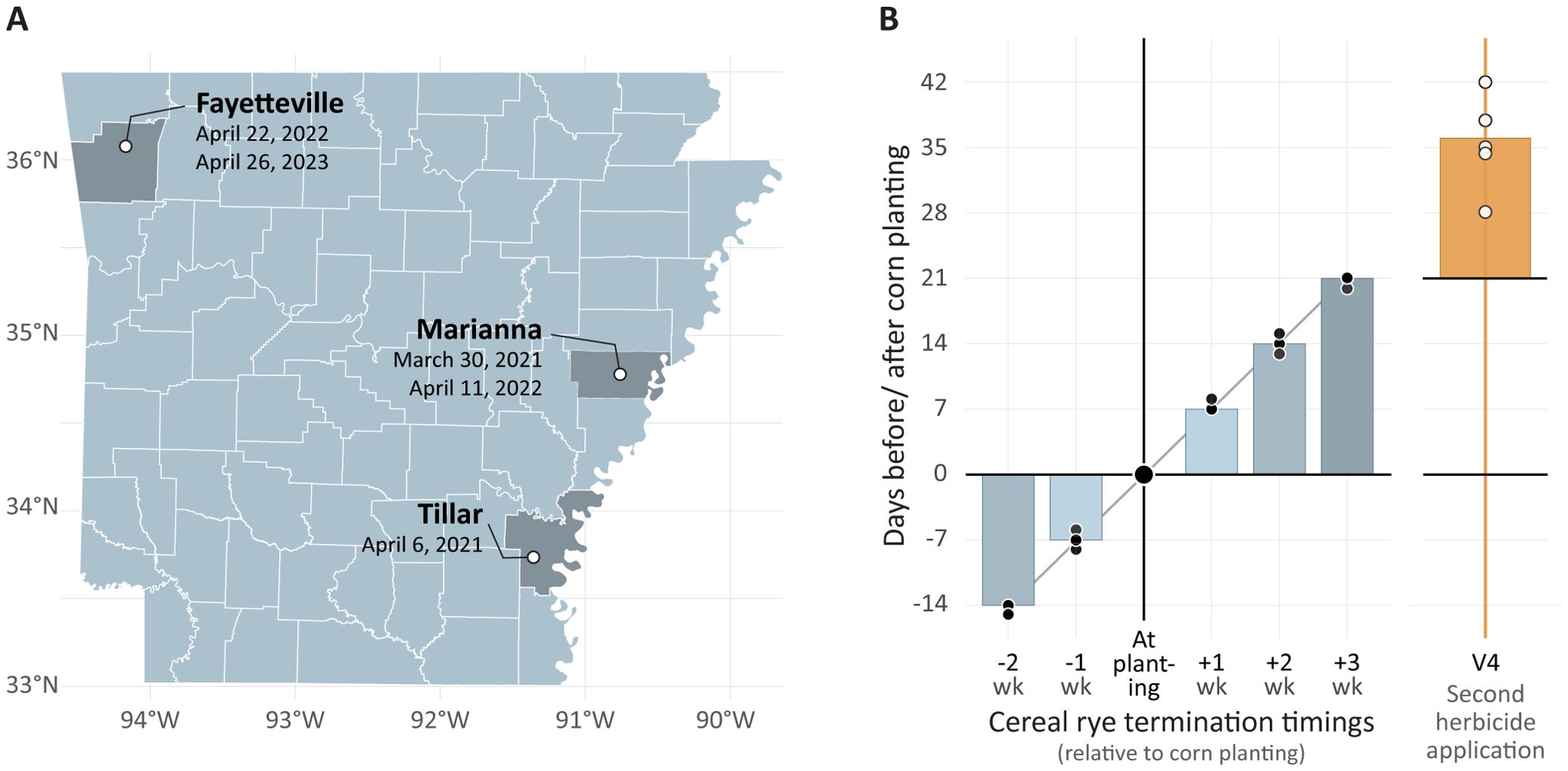
Figure 1. Map showing five experimental site-years in Arkansas, USA 2021 through 2023 with corn planting dates for each site-year (A), and cereal rye termination timings (black circles represents individual site-year) relative to corn planting along with the timing of the second herbicide application at V4 corn (B). Note some variability in the time of herbicide application at V4 corn.
Treatments consisted of two cereal rye termination timings before corn planting (hereafter occasionally referred as preplant terminated), one at corn planting, and three after corn planting (hereafter occasionally referred as post-plant–terminated), all termination timings approximately 1 week apart (Figure 1B). A control treatment was included in the study. The control treatment was a no-till system where winter vegetation was controlled with a burndown application of glyphosate 8 weeks before planting corn (hereafter referred as the conventional system). Cereal rye was terminated with glyphosate with the addition of atrazine and S-metolachlor for residual weed control (the first pass of the herbicide program). The conventional system received the same application at corn planting. The second pass of the herbicide program included the same herbicide mixture plus mesotrione. Herbicide information (trade name, active ingredient, and rate) for these herbicide programs is given in Table 1. Cereal rye aboveground biomass was harvested from two randomly placed 0.25-m2 quadrants at the time of termination from the Fayetteville sites. An additional application of glyphosate + mesotrione + S-metolachlor was applied at V4 corn. Herbicide treatments were applied using a CO2-pressurized backpack sprayer calibrated to deliver 140 L ha−1 at 166 kPa fitted with AIXR 110015 flat-fan nozzles (TeeJet®; Spraying Systems Co., Wheaton, IL). Fertility and pest management were maintained in all sites following the University of Arkansas System Division of Agriculture Cooperative Extension Service recommendations (Faske et al., 2022; Studebaker et al., 2022). Irrigation, either furrow or overhead, was employed weekly when rainfall was <2.5 cm to prevent moisture stress during the growing season in all site-years.
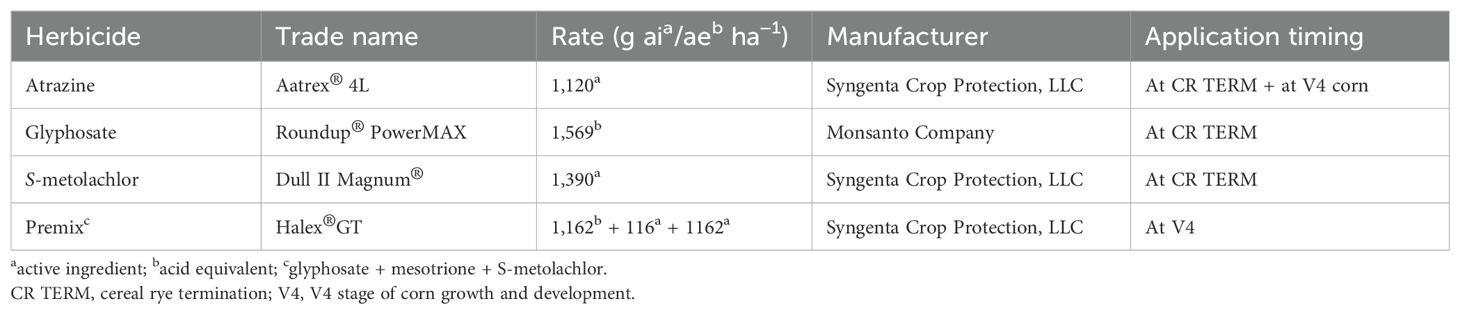
Table 1. Herbicides used in the two-pass herbicide program employed in the cereal rye termination time study.
The harvested cereal rye biomass samples were oven-dried at 65°C for 3 days and weighed. In conventional plots, densities of major weed species were recorded (two 1-m−2 quadrants) at the time of corn planting. Weed control (by species) ratings were based on visual assessment of weed density and vigor and were performed at three key stages: the time of cereal rye termination, at V4 corn, and 4 weeks after V4 corn. Weed density refers to the visually assessed number of weeds present in a specific plot. The vigor of the weed indicates their health and robustness. The ratings were assigned on a scale of 0% (no weed control) to 100% (complete weed death). This scale was not merely a measure of whether the weeds were alive or dead. Instead, it provided a more comprehensive view of weed control effectiveness by considering both the quantity (density) and quality (vigor) of the weeds. For instance, a plot with a low density of weak, unhealthy weeds would receive a high control rating, close to 100%. Conversely, a plot with a high density of vigorous, healthy weeds would receive a low control rating, closer to 0%. Crop height at all sites and stand count at Fayetteville site-year was taken from five random plants per plot 4 week after V4 corn. At crop maturity, two center rows of corn were harvested using a small plot combine, and grain yield was adjusted to 15% moisture. Yield data were converted to a percentage of the yield in the conventional system.
Statistical analysis was performed using R statistical software version 4.3.1 (R Core Team, 2024) and the GLMMTMB package (function glmmTMB; Brooks et al., 2017). Weed control (by species) and corn stand, height, and yield data were fit to generalized linear mixed-effect models (GLMM) (glmmTMB function (Stroup, 2015). Data were analyzed with cereal rye termination time as a fixed effect, whereas site-year and block (block nested within site-year) were considered random effects. Corn height and yield data were expressed as a percentage of conventional planting. Weed control data were transformed to 1/100 and analyzed with beta distribution (link = “logit”), and height and yield data with Gaussian (link = “identity”) error distributions. ANOVA was performed on the fitted model using the CAR package (Fox and Weisberg, 2019) with Type II Wald chi-square tests. Estimated marginal means (EMMs) (Searle et al., 1980) for the treatments were obtained using EMMEANS package (Lenth, 2022), and the Sidak method was used to adjust for multiple comparisons. A compact letter display was generated using MULTCOMP package (Hothorn et al., 2008) to visually represent which groups were significantly different from each other based on the results of multiple comparison tests, including contrasts.
3 Results and discussion
The study’s objective and subsequent results were approached with a broad inference perspective. In this analytical framework, site-years were treated as random effects, allowing for a more generalized interpretation of the findings. This approach was adopted to account for the inherent variability among site-years, ensuring that the conclusions hold broader relevance, encompassing a spectrum of environmental conditions and operational timings. A cooler climate and higher elevation are characteristic of Fayetteville in the northwest portion of the state. Slightly warmer temperatures are experienced in Marianna in the east and Tillar in the southeast. The timing of field operations also varied slightly across different site-years (Figure 1B). For a better perspective, the standard deviations of the random effects (block:site-year or site-year) accompany the respective figures for each variable.
3.1 Cereal rye biomass at termination
Originally, the study was conducted over 7 site-years, with 2 site-years discarded because of poor cereal rye establishment. While cereal rye biomass was not quantitatively measured at all sites due to logistical constraints, visual observations suggested that the growth of cereal rye was fairly consistent across the site-years. This observation, although not quantified, suggests a level of consistency in the cereal rye performance across different locations and years. Cereal rye biomass collected from the 2 site-years in Fayetteville (2022 and 2023) did not vary at the time of corn planting and averaged 5,600 kg ha−1 (data not shown). The biomass was 25% (P < 0.001) and 66% (P < 0.001) less 1 and 2 weeks before planting, respectively, compared to the biomass at corn planting. The biomass is much higher compared to the recently reported national average (Huddell et al., 2024). The biomass was at its maximum at the time of corn planting, and there was no increase in biomass at the later termination time points.
3.2 Weed control
The weed control effects of different cover crop termination timings were evaluated only for the major weeds present in each site-year. In cereal rye plots, weeds were undetectable at the time of cereal rye termination (data not shown), indicating a strong broad-spectrum suppression of early emerging cohorts of weeds. The first weed control assessment, conducted at V4 corn, reflects the cumulative effects of the burndown treatment and the first in-crop herbicide application at corn planting for conventional plots, whereas it reflects the effects of the cereal rye and the herbicides applied at the respective termination timing for cereal rye plots. The time gap between corn planting and the V4 corn ranged from 4 to 6 weeks among site-years (Figure 1B). The second assessment conducted 4 weeks after V4 represents the weed control achieved from the additional herbicide application at V4 corn.
3.2.1 Palmer amaranth control
Palmer amaranth (Amaranthus palmeri S. Watson) was consistently present across all site-years. The estimated density of Palmer amaranth in conventional plots, which received a burndown treatment 3 weeks before planting, varied from two to five plants m−2 across site-years at the time of corn planting. At V4, weed control in conventional corn system was less than 50% when averaged across 5 site-years, whereas the control was greater than 75% in cereal rye environments, regardless of termination timings (Figure 2A, P < 0.001). Four weeks after V4 corn, Palmer amaranth control was similar across treatments, with only the termination timings 1 or 2 weeks after corn planting providing greater control (by >5%) than conventional system (Figure 2B, P < 0.001). Cereal rye terminated at corn planting or earlier resulted in similar levels of Palmer amaranth control to the conventional system. Palmer amaranth control greatly varied across sites.
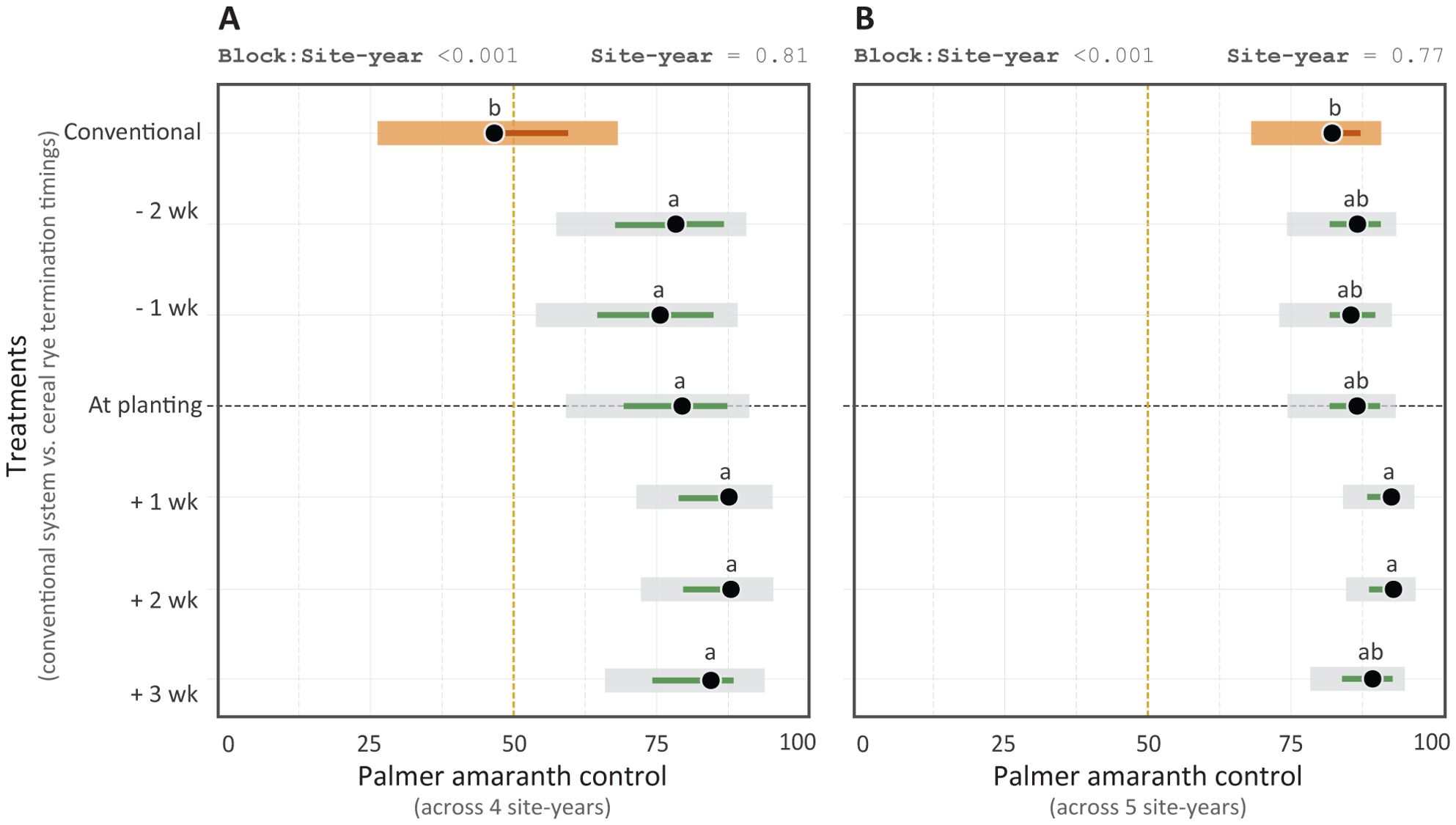
Figure 2. Palmer amaranth control at V4 corn (A) and 4 weeks after V4 corn (B) under cereal rye environments terminated at varying timings and conventional corn system. Weed control is based on a scale of 0 (no control) to 100% (complete control). Light broad bars denote the 95% confidence interval (CI) of estimated marginal means, with the circle representing the mean. Non-overlapping narrow solid bars within the respective CIs indicate significantly different means. Also, means with similar letters are not different from each other. Random effects estimated at two hierarchical levels: blocks within sites (block:site) and sites (site-year) and are shown on top of the corresponding figure.
Cover crops have been shown to have a positive effect on suppressing weed growth, including Palmer amaranth (Aulakh et al., 2012; Bunchek et al., 2020; Hand et al., 2021; Price et al., 2012; Webster et al., 2016; Wiggins et al., 2016). In this study, the two-pass herbicide program employed across both the conventional and cereal rye systems resulted in fairly similar control of Palmer amaranth. However, the key distinction between the conventional system and the cereal rye system lies in the sole versus reduced reliance on herbicides for controlling the weed. It is important to note that Palmer amaranth has evolved resistance to multiple herbicide modes of action, including ALS inhibitors, triazines, HPPD inhibitors, dinitroanilines, glufosinate, and glyphosate (Heap, 2023). Palmer amaranth, which has dioecious reproduction, forces outcrossing and genetic diversity. This gives Palmer amaranth the ability to adapt and quickly spread herbicide resistance genes when selection pressure is applied, such as when producers heavily rely on herbicides for weed management (Ward et al., 2013). The use of cover crops has been suggested as one of the proactive management practices for herbicide resistance management. It can help in reducing the selection pressure for herbicide resistance, thereby slowing down the evolution of herbicide resistance in weeds (Bunchek et al., 2020; Norsworthy et al., 2012).
3.2.2 Yellow nutsedge control
At V4 corn, yellow nutsedge (Cyperus esculentus L.) control was slightly less (< 95%) with cereal rye terminated 2 weeks before corn planting compared to the conventional corn system (98%) (Figure 3A, P < 0.001). The control with cereal rye environments that were terminated at corn planting or 1 week before/after corn planting was comparable to the conventional corn system, whereas the later termination timings were less effective than the conventional corn system. The control levels slightly decreased overall (by 2 to 5%) across all treatments 4 weeks later (Figure 3B). The control with the cereal rye termination 2 weeks before planting remained significantly less at 4 weeks after V4 (P = 0.003). All other cereal rye termination timings, when considered individually, resulted in similar control of yellow nutsedge compared to the conventional corn system. However, their average effect was significantly lower than the conventional system (P = 0.014), indicating some differentiation in the level of yellow nutsedge control between the cereal rye environment and the conventional system. Yellow nutsedge control greatly varied within site as indicated by the magnitude of block:site variability.
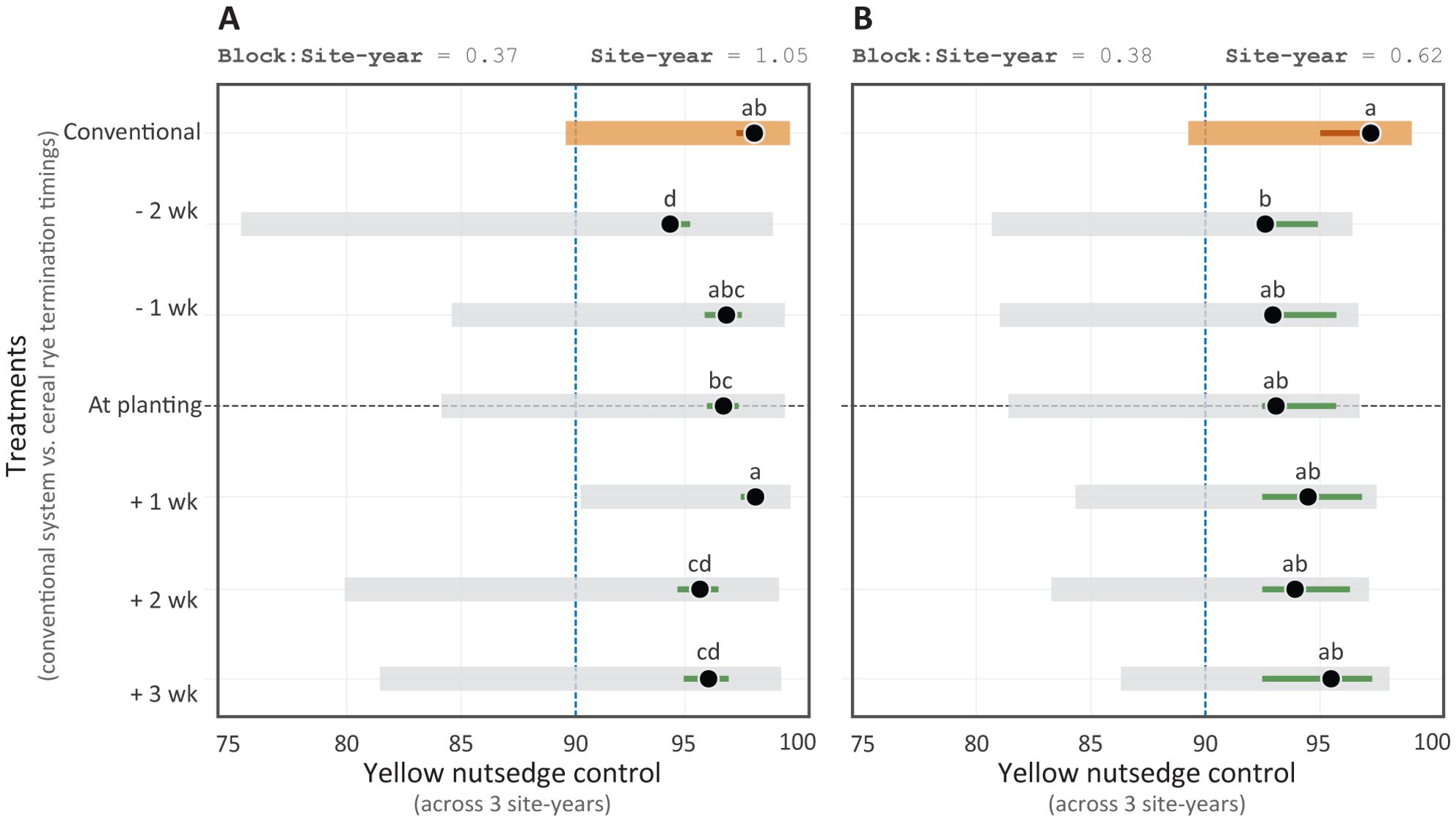
Figure 3. Yellow nutsedge control at V4 corn (A) and 4 weeks after V4 corn (B) under cereal rye environments terminated at varying timings and conventional corn system. Weed control is based on a scale of 0 (no control) to 100% (complete control). Light broad bars denote the 95% confidence interval (CI) of estimated marginal means, with the circle representing the mean. Non-overlapping narrow solid bars within the respective CIs indicate significantly different means. Also, means with similar letters are not different from each other. Random effects estimated at two hierarchical levels: blocks within sites (block:site) and sites (site-year) and are shown on top of the corresponding figure.
Initial burndown followed by two applications of herbicides at planting and at the V4 corn stage provided effective control of yellow nutsedge in conventional corn system, slightly surpassing the efficacy in cereal rye environments. Ormeño–Núñez et al. (2008) observed greater than 80% control of yellow nutsedge when a mulch of cereal rye cover crop was present. In contrast, another study reported that the cereal rye mulch or management timing did not impact yellow nutsedge emergence or development (Mirsky et al., 2011). These results lead to ambiguity regarding the relative contribution and/or interaction among residue interference of herbicide spray droplets, time between two herbicide applications, as well as the suppression from the cereal rye on yellow nutsedge.
3.2.3 Grass weed control
Barnyardgrass [Echinochloa crus–galli (L.) P. Beauv] and broadleaf signalgrass [Urochloa platyphylla (Munro ex C. Wright) R.D. Webster] were present at significant density (> 2 plants m−2) in 2 site-years (Tillar in 2021 and Marianna in 2022) and 3 site-years (Fayetteville in 2023, Tillar in 2021 and Marianna in 2021), respectively.
Barnyardgrass control at V4 corn was greater (93% to 96%) in cereal rye systems, regardless of the termination times compared to the conventional corn system (77%) (P < 0.001), except when the cereal rye was terminated 2 weeks before corn planting (Figure 4A). Overall, barnyardgrass control generally decreased 4 weeks later, and the level of control in some cereal rye environments was not differentiated from the conventional system (Figure 4B). Only the cereal rye environments that were terminated 1 or 2 weeks after corn planting had greater control of barnyardgrass (92%) than the conventional corn system (60%) (P < 0.001). When pooled and compared against the conventional system, the control with the cereal rye environments was slightly greater (P = 0.002). The magnitude of variability observed for site-year factor at both the evaluation timings indicates that the barnyardgrass control outcome may deviate in either way on site-specific context.
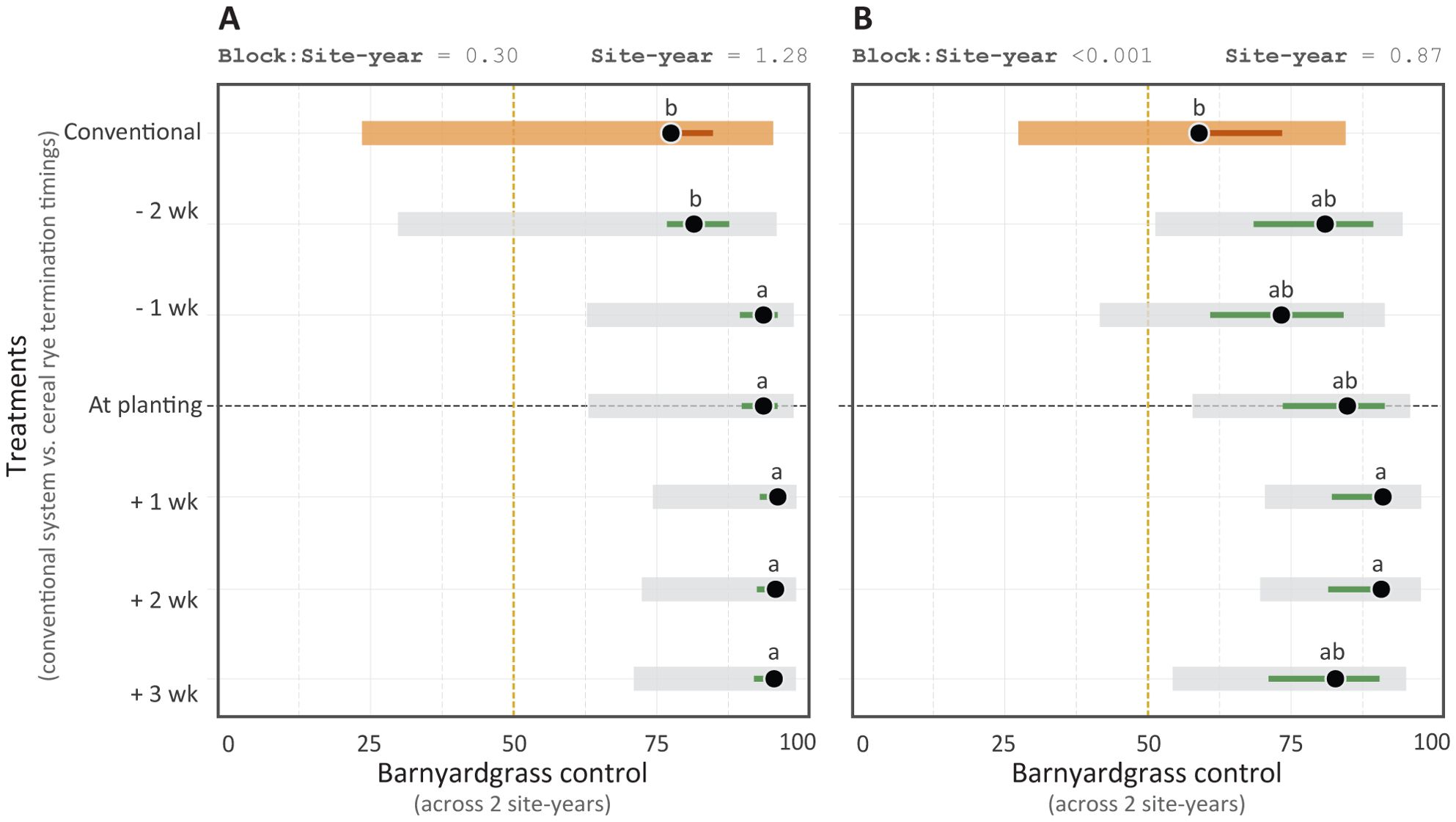
Figure 4. Barnyardgrass control at V4 corn (A) and 4 weeks after V4 corn (B) under cereal rye environments terminated at varying timings and conventional corn system. Weed control is based on a scale of 0 (no control) to 100% (complete control). Light broad bars denote the 95% confidence interval (CI) of estimated marginal means, with the circle representing the mean. Non-overlapping narrow solid bars within the respective CIs indicate significantly different means. Also, means with similar letters are not different from each other. Random effects estimated at two hierarchical levels: blocks within sites (block:site) and sites (site-year) and are shown on top of the corresponding figure.
Broadleaf signalgrass control at V4 corn was greater only in the cereal rye environments terminated after corn planting (83% to 88%) compared to the conventional system (45%) (Figure 5A, P = 0.001). The control was similar in cereal rye environments terminated at or before corn planting to the conventional system; however, their aggregated effect was slightly greater than the conventional system (P = 0.024). The site-year factor contributed a significant amount of variation in broadleaf signalgrass control. The control was greater than 98% in all the cereal rye environments as well as in conventional corn system four week later (Figure 5B), which is attributed to the excellent efficacy of the herbicide application at V4 corn.
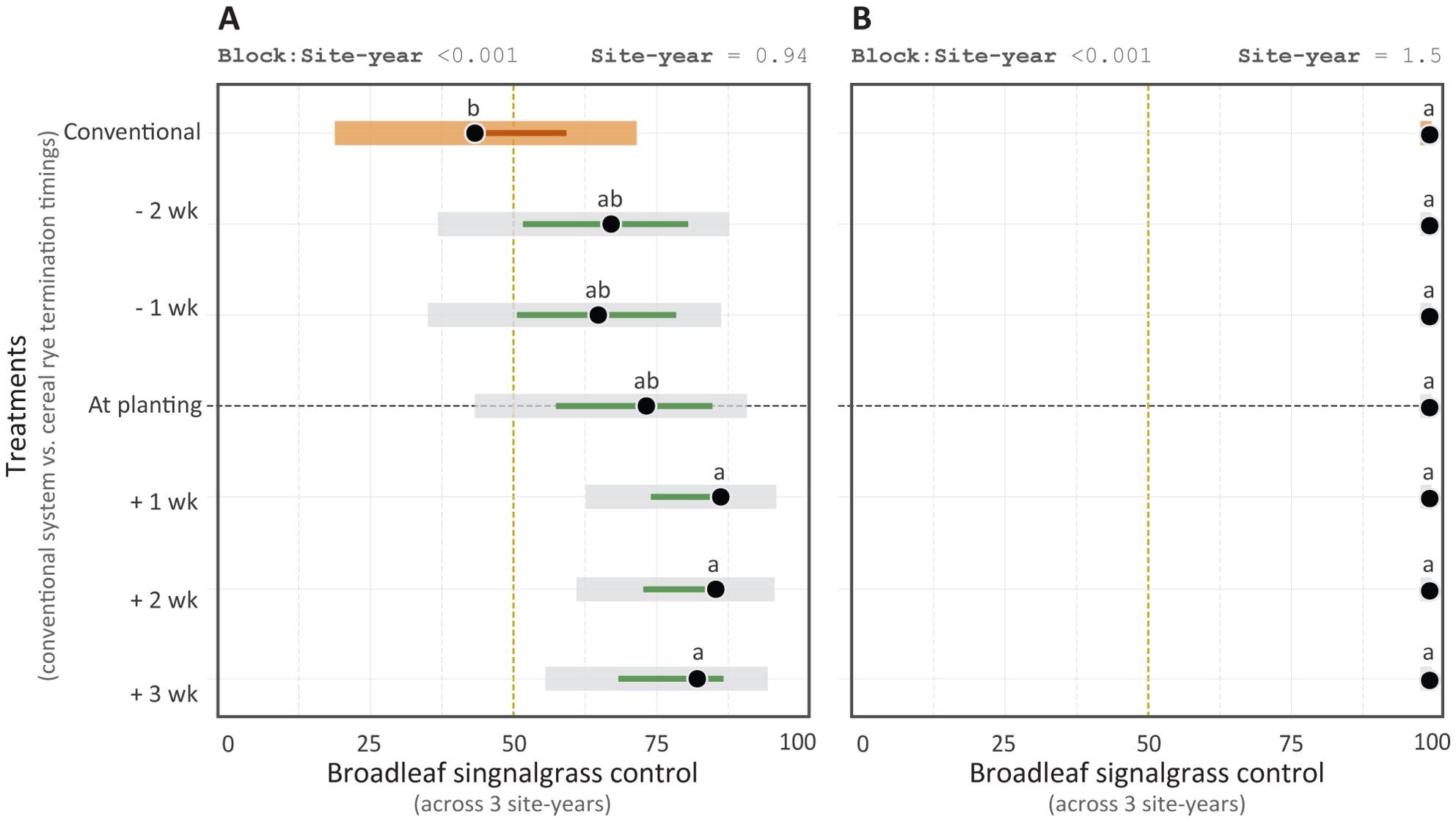
Figure 5. Broadleaf signalgrass control at V4 corn (A) and 4 weeks after V4 corn (B) under cereal rye environments terminated at varying timings and conventional corn system. Weed control is based on a scale of 0 (no control) to 100% (complete control). Light broad bars denote the 95% confidence interval (CI) of estimated marginal means, with the circle representing the mean. Non-overlapping narrow solid bars within the respective CIs indicate significantly different means. Also, means with similar letters are not different from each other. Random effects estimated at two hierarchical levels: blocks within sites (block:site) and sites (site-year) and are shown on top of the corresponding figure.
Barnyardgrass and broadleaf signalgrass are known to have differential response to herbicides. In this particular scenario, the observed difference in the control between two species, especially at 4 weeks after V4 corn, could be the emergence pattern of the two species. Neither species was present at the time of cereal rye termination. Relatively poor control of broadleaf signalgrass at V4 corn could be attributed to grass species in general being relatively less sensitive to the allelopathic effects of cereal rye (Barnes and Putnam, 1987), or the emergence time that escaped the first herbicide application, or the concert of both factors.
The weed control benefit of using cereal rye in corn slightly varied among weed species and was largely influenced by the timing of cereal rye termination. Delaying the termination of cereal rye until after corn planting—a practice commonly referred to as “planting green”—substantially enhanced weed suppression compared to its earlier termination. Notably, the impact of cover crops, inclusive of those terminated earlier, was prominent at V4 corn. However, under the two-pass herbicide program, this differentiation diminished in the final weed control outcome. Despite this, it is crucial to recognize that all the cereal rye environments, even those terminated early, reduced the reliance on herbicides for weed control. The average biomass (5,600 kg ha−1) present in this study provided excellent suppression of weeds at cover crop termination. In the conventional production system, the early weed flushes are typically controlled by tillage or burndown applications incurring environmental cost or herbicide selection pressure. In this research, the sole contribution of the cover crop afterward cannot be isolated as the residual herbicide was included in the cover crop termination treatment. Previous research has shown that similar biomass of cover crop(s) as in this study provides considerable weed suppression (Pittman et al., 2020). Overall, these findings suggest that integrating cereal rye into corn production system not only enhances weed management outcomes but also mitigates the selection exerted by herbicides on weed populations, an important consideration for sustainable weed management practice.
3.3 Crop stand, height, and yield
3.3.1 Crop stand
Averaged over 2 site-years (Fayetteville sites only), cereal rye termination timings had an effect on the crop stand (P < 0.001, data not shown). Compared to the stand of conventional corn (8.9 plants per m row), only cereal rye environments that were terminated 2 or 3 weeks after corn planting reduced the crop stand (6.8 plants per m row). The decrease in plant stand may be primarily attributed to the exhausted moisture in the soil under the living cereal rye environment. Prior research has shown greater moisture depletion (soil drying) by standing green cover crop at planting compared to preplant terminated cereal rye environments (Liebl et al, 1992; Munawar et al., 1990; Reed et al., 2019). Interference of cereal rye on crop stand establishment has been previously documented (Duiker and Curran, 2005; Liebl et al., 1992; Reed et al., 2019). While direct evidence of the allopathic effect of cereal rye in corn seedling establishment has not been reported to our knowledge, we cannot rule out this possibility. Lower crop stand under post-plant–terminated cereal rye may directly impact weed suppression, crop canopy closure, and herbicide coverage and, most importantly, may contribute to crop yield loss.
3.3.2 Crop height
Corn height did not differ among the cereal rye termination timings or from the conventional planting, except when cereal rye was terminated at planting or 1 week later (Figure 6). These termination timings resulted in slightly greater height (8 to 10%) than in the conventional corn system (P = 0.031). Grass cover crop research in corn has typically been conducted under preplant termination conditions. Galloway and Weston (1996) reported no effect on sweet corn height, whereas Burgos and Talbert (1996) observed a decrease in sweet corn height. Under preplant termination conditions, leguminous cover crops have generally been shown to positively affect corn height (Koger and Reddy, 2005; Wagger, 1989; Wiggins et al., 2015). The magnitude of block:site factor variability suggests that future research should identify factors that cause local variation in corn stand, such as seeding depth, residue distribution, soil crusting, and soil moisture. Nonlinearity in allelopathic interactions has been demonstrated (Belz et al., 2005), and it is very possible that the allelochemical from cereal rye vary locally, potentially contributing to such variation.
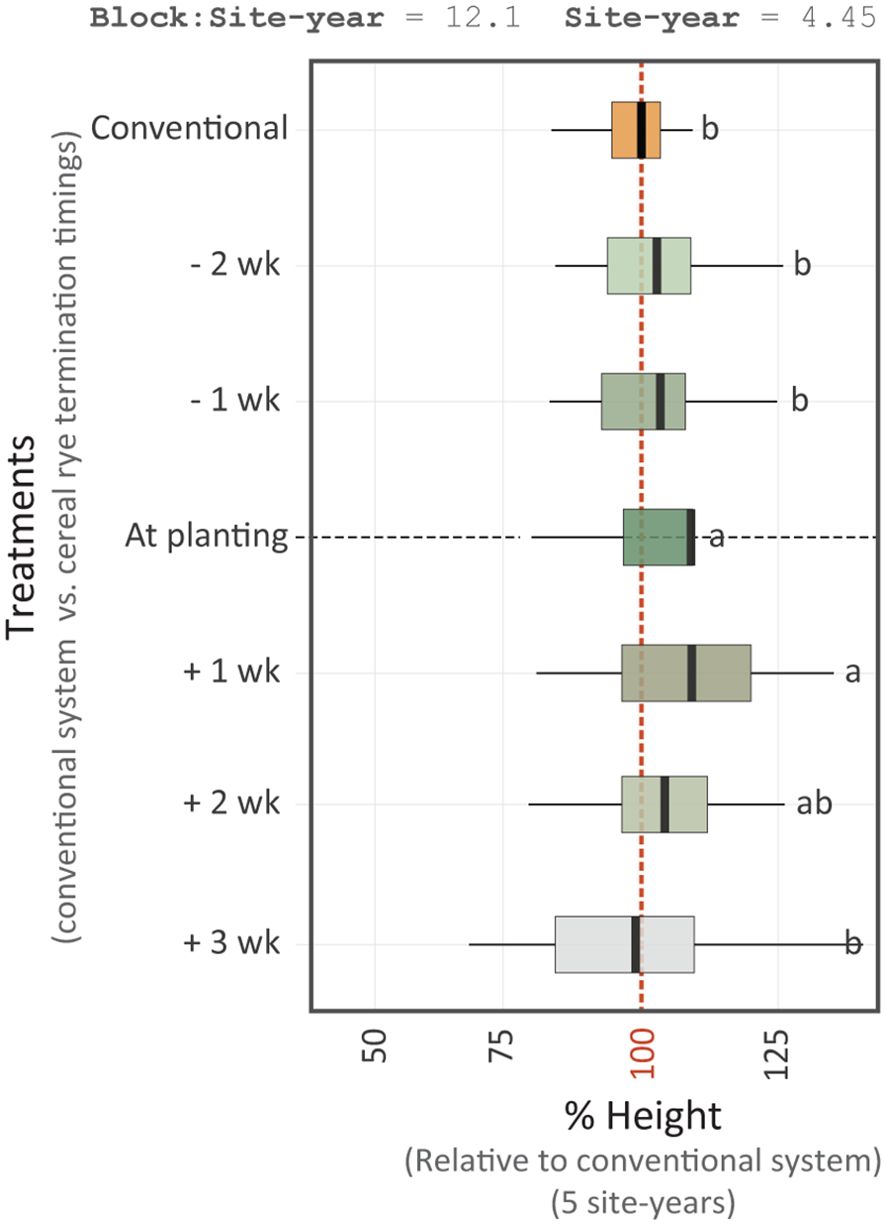
Figure 6. Box plot showing corn height under cereal rye environments terminated at different timings relative to conventional corn system. The vertical bar represents estimated marginal mean. Means with similar letters are not different from each other. Random effects estimated at two hierarchical levels: blocks within sites (block:site) and sites (site) and are shown on the top.
3.3.3 Crop yield
Cereal rye termination timings affected corn yield. Corn yield was similar to the conventional system in the preplant terminated cereal rye environments; however, it decreased significantly when cereal rye termination coincided with or followed corn planting, ranging from 16% to 22% (Figure 7, P < 0.001). The smaller variability at the block:site level than at the site level signifies a broader inference across different system environments regarding corn yield, but this also point to the need for future research to decipher the local factors that cause such site level variation. Previous research has presented a complex array of yield outcomes of cover crops within corn and soybean systems. Earlier meta-analyses by Tonitto et al. (2006), and Miguez and Bollero (2005) indicated fluctuating yield effects from non-leguminous cover crops, with slight positive or negative trends. A more recent meta-analysis shows a neutral effect of winter grass cover crops on corn yield Marcillo and Miguez (2017). Munawar et al. (1990) and Reese et al (2014) highlight moisture availability as a pivotal factor in semiarid regions. Seifert et al. (2018) points to modest yield improvements with cover crops yet emphasize the need to consider site-specific factors. Notwithstanding, the observed reduction in yield in Arkansas is most appropriately attributed to the reduced crop stands as observed in two site-years and also to the known allelopathic effects of cereal rye on corn (Raimbault et al., 1990; Kessavalou and Walters, 1997), rather than primarily to conditions in moisture-limited environments. However, the depleted soil moisture condition at crop planting in the post-plant–terminated cereal rye environments may have created an unfavorable condition for seedling emergence and establishment. The specific factors that cause yield reduction in the late-terminated cereal rye environment are not well known or are little understood. Additionally, available soil nitrogen dynamics and planting interference by cereal rye are factors that can influence corn stand and yield (Mitchell and Tell, 1977; Tollenaar et al., 1993; Duiker and Curran, 2005).
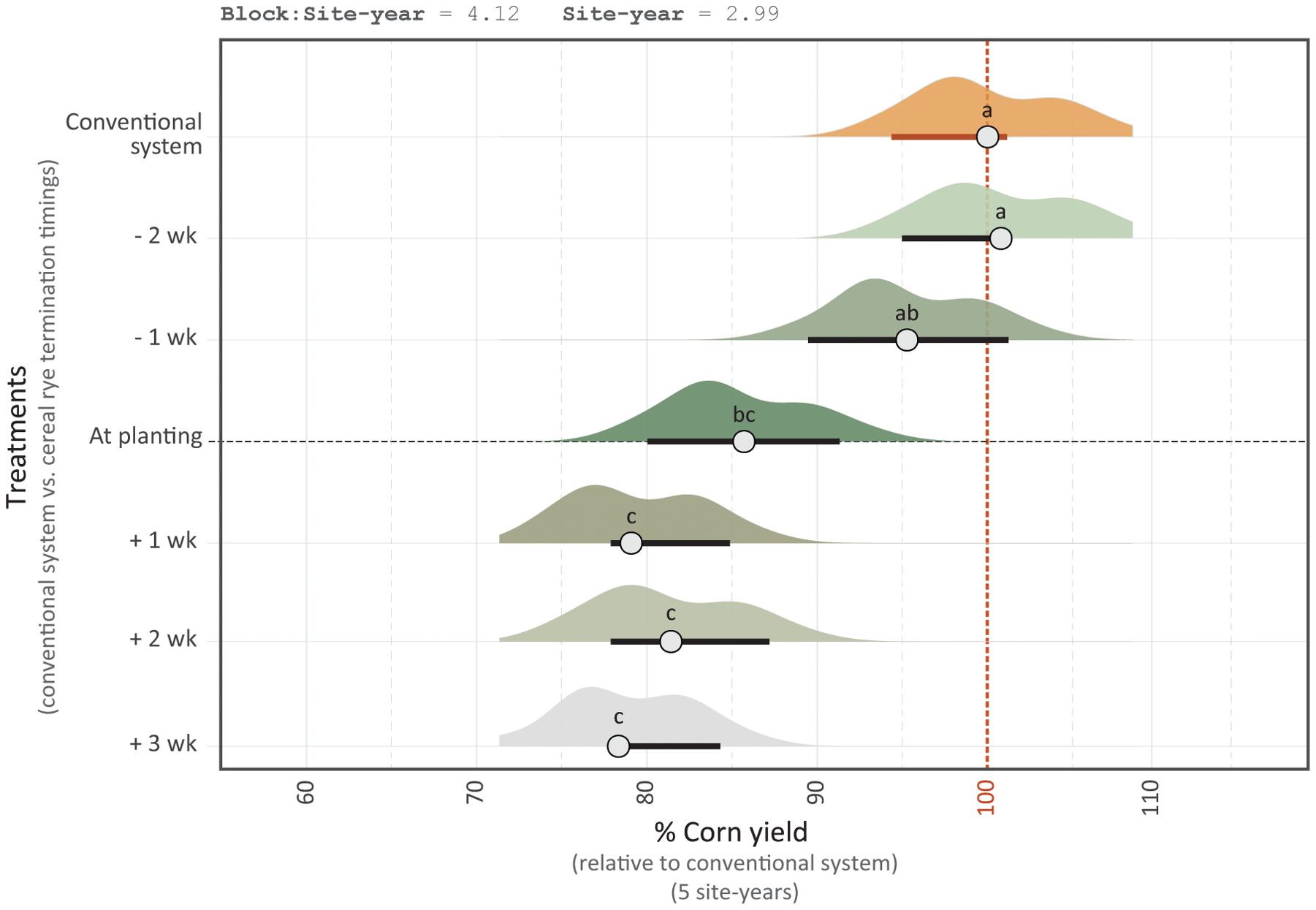
Figure 7. Corn yield under cereal rye environments terminated at different timings relative to conventional corn system. The density plot represents the predicted values of yield across 5 site-years, with the circle representing the mean. Non-overlapping bars indicate significantly different means. Also, means with similar letters are not different from each other. Random effects estimated at two hierarchical levels: blocks within sites (block:site) and sites (site) and are shown on the top.
Earlier termination of cereal rye has been suggested for several agroecological conditions (Munawar et al., 1990; Raimbault et al., 1990; Ball–Coelho et al, 2005; Acharya et al., 2017). Results from this 5–site-year study corroborate those previous reports in terms of corn yield and establish a similar threshold for cereal rye termination timing for the region: 2 weeks before planting corn was identified as the optimal timing. Cereal rye termination 1 week before planting may be considered as the latest acceptable timing to avoid yield decline. In regard to weed control, the integration of cereal rye in corn should aim at improving weed management and more importantly in reducing herbicide selection pressure to delay herbicide resistance evolution or manage existing resistance issues. As the termination before corn planting curtails the weed suppression benefits of cereal rye, its termination right at corn planting could remain a viable alternative in special scenarios where weeds are difficult to control with other available options.
The conclusions presented herein are derived from multi-year, multi-site data, providing a generalized interpretation of the study’s outcomes. The timing of cereal rye termination relative to corn planting is a key management consideration and subject to crop yield penalty if the cereal rye termination is delayed. Future research could explore strategies to mitigate the trade-offs associated with the late termination timings, which may be, to some extent, subject to site-specific contexts. There is potential for future studies to quantify and isolate these site-specific factors in order to fine-tune the threshold point. Facts that the variability among cereal rye varieties, particularly in terms of the amount of allelochemical content and the degradation rate of these chemicals in the soil, is influenced by biotic and abiotic factors (Tollenaar et al., 1993; Burgos et al., 1999; Brooks et al., 2012; Schulz et al., 2013) point to the possibility of further improvement in the use of cereal rye as cover crop in corn. Additionally, an understanding of the relative contributions of allelopathy and resource competition in the interactions between cereal rye and corn or between cereal rye and weeds, which can be challenging (Duke, 2015), could help to refine the strategic use of cereal rye and to guide its integration practice.
This study highlights the strategic incorporation of cereal rye cover crops within corn systems. Timely termination of cereal rye can yield corn comparable to conventional systems in the region while enhancing weed control and notably reducing herbicide selection for weed resistance. The conclusions drawn from this multi–site-year study furnish practical recommendations for farmers and agronomists. With the integration of cover crops being increasingly recognized as a valuable addition to sustainable agricultural practices, this research offers key insights into the impact of cereal rye on weed suppression and corn yield expectations in the region. A broad inference drawn from this research could potentially apply to regions in the Midsouth with conditions like those in Arkansas. Moreover, the noted improvement in weed control with delayed termination timings and the associated trade-off on corn yield guides future research in this regard. Broadly, the study contemplates the implications of the research findings for integrated weed management and crop production strategies.
Data availability statement
The raw data supporting the conclusions of this article will be made available by the authors, without undue reservation.
Author contributions
AG: Data curation, Formal analysis, Investigation, Software, Visualization, Writing – original draft, Writing – review & editing. JN: Conceptualization, Data curation, Funding acquisition, Investigation, Methodology, Project administration, Resources, Software, Supervision, Validation, Writing – review & editing. LTB: Conceptualization, Data curation, Funding acquisition, Investigation, Methodology, Project administration, Resources, Software, Supervision, Validation, Writing – review & editing.
Funding
The author(s) declare that financial support was received for the research, authorship, and/or publication of this article. Arkansas Corn and Grain Sorghum Promotion Board.
Acknowledgments
This publication is a contribution of the University of Arkansas System Division of Agriculture.
Conflict of interest
The authors declare that the research was conducted in the absence of any commercial or financial relationships that could be construed as a potential conflict of interest.
Publisher’s note
All claims expressed in this article are solely those of the authors and do not necessarily represent those of their affiliated organizations, or those of the publisher, the editors and the reviewers. Any product that may be evaluated in this article, or claim that may be made by its manufacturer, is not guaranteed or endorsed by the publisher.
References
Acharya J., Bakker M. G., Moorman T. B., Kaspar T. C., Lenssen A. W., Robertson A. E. (2017). Time interval between cover crop termination and planting influences corn seedling disease, plant growth, and yield. Plant Dis. 101, 591–600. doi: 10.1094/PDIS-07-16-0975-RE
Aulakh J., Price A., Enloe S., Santen E., Wehtje G., Patterson M. (2012). Integrated Palmer amaranth management in glufosinate-resistant cotton: I. Soil-inversion high-residue cover crops herbicide regimes. Agron. 2, 295–311. doi: 10.3390/agronomy2040295
Ball–Coelho B. R., Roy R. C., Bruin A. J. (2005). Long–term effects of late–summer overseeding of winter rye on corn grain yield and nitrogen balance. Can. J. Plant Sci. 85, 543–554. doi: 10.4141/p04-070
Barnes J. P., Putnam A. R. (1983). Rye residues contribute weed suppression in no–tillage cropping systems. J. Chem. Ecol. 9, 1045–1057. doi: 10.1007/BF00982210
Barnes J. P., Putnam A. R. (1986). Evidence for allelopathy by residues and aqueous extracts of rye (Secale cereale L.). Weed Sci. 34, 384–390. doi: 10.1017/S0043174500067035
Barnes J. P., Putnam A. R. (1987). Role of benzoxazinones in allelopathy by rye (Secale cereale L.). J. Chem. Ecol. 13, 889–906. doi: 10.1007/BF01020168
Belz R. G., Hurle K., Duke S. O. (2005). Dose–response–a challenge for allelopathy? Nonlinearity. Biol. Toxicol. Med. 3, 173–211. doi: 10.2201/nonlin.003.02.002
Blanco–Canqui H., Mikha M. M., Presley D. R., Claassen M. M. (2011). Addition of cover crops enhances no–till potential for improving soil physical properties. Soil Sci. Soc Am. J. 75, 1471–1482. doi: 10.2136/sssaj2010.0430
Brooks A. M., Danehower D. A., Murphy J. P., Reberg–Horton S. C., Burton J. D. (2012). Estimation of heritability of benzoxazinoid production in rye (Secale cereale) using gas chromatographic analysis. Plant Breed. 131, 104–109. doi: 10.1111/j.1439-0523.2011.01885.x
Brooks M. E., Kristensen K., van Benthem K. J., Magnusson A., Berg C. W., Nielsen A., et al. (2017). glmmTMB balances speed and flexibility among packages for zero–inflated generalized linear mixed modeling. R. J. 9, 378–400. doi: 10.32614/RJ-2017-066
Bunchek J. M., Wallace J. M., Curran W. S., Mortensen D. A., VanGessel M. J., Scott B. A. (2020). Alternative performance targets for integrating cover crops as a proactive herbicide-resistance management tool. Weed Sci. 68, 534–544. doi: 10.1017/wsc.2020.49
Burgos N. R., Talbert R. R. (1996). Weed control and sweet Corn (Zea mays var. rugosa) response in a no-till system with cover crops. Weed Sci. 44, 355–361. doi: 10.1017/S0043174500094005
Burgos N. R., Talbert R. E. (2000). Differential activity of allelochemicals from Secale cereale in seedling bioassays. Weed Sci. 48, 302–310. doi: 10.1614/0043-1745(2000)048[0302:DAOAFS]2.0.CO;2
Burgos N. R., Talbert R. E., Mattice J. D. (1999). Cultivar and age differences in the production of allelochemicals by Secale cereale. Weed Sci. 47, 481–485. doi: 10.1017/S0043174500092146
Caamal-Maldonado J., Jiménez-Osornio J. J., Torres-Barragán A., Anaya A. L. (2001). The use of allelopathic legume cover and mulch species for weed control in cropping systems. Agron. J. 93, 27–36. doi: 10.2134/agronj2001.93127x
Creamer N. G., Bennett M. A., Stinner B. R., Cardina J. (1996). A comparison of four processing tomato production systems differing in cover crop and chemical inputs. J. Amer. Soc Hortic. Sci. 121, 559–568. doi: 10.21273/JASHS.121.3.559
Daigh A. L., Helmers M. J., Kladivko E., Zhou X., Goeken R., Cavdini J., et al. (2014). Soil water during the drought of 2012 as affected by rye cover crops in fields in Iowa and Indiana. J. Soil Water Conserv. 69, 564–573. doi: 10.2489/jswc.69.6.564
Deines J. M., Guan K., Lopez B., Zhou Q., White C. S., Wang S., et al. (2023). Recent cover crop adoption is associated with small maize and soybean yield losses in the United States. Global Change Biol. 29, 794–807. doi: 10.1111/gcb.16489
Dhima K. V., Vasilakoglou I. B., Eleftherohorinos I. G., Lithourgidis A. S. (2006). Allelopathic potential of winter cereals and their cover crop mulch effect on grass weed suppression and corn development. Crop Sci. 46, 345–335. doi: 10.2135/cropsci2005-0186
Duiker S., Curran W. (2005). Rye cover crop management for corn production in the northern Mid–Atlantic region. Agron. J. 97, 1413–1418. doi: 10.2134/agronj2004.0317
Duke S. O. (2015). Proving allelopathy in crop–weed interactions. Weed Sci. 63, 121–132. doi: 10.1614/WS-D-13-00130.1
Faske T., Supurlock T., Cato A., Smith S., Urrea–Morawicki K., Wamishe Y. (2022). MP154, Arkansas plant disease control products guide (Little Rock: University of Arkansas System Division of Agriculture, Research and Extension), 158.
Fox J., Weisberg S. (2019). An R companion to applied regression (Thousand Oaks CA: Sage). Available online at: https://socialsciences.mcmaster.ca/jfox/Books/Companion/ (Accessed October 14, 2023).
Galloway B. A., Weston L. A. (1996). Influence of cover crop and herbicide treatment on weed control and yield in no-till sweet corn (Zea mays L.) and pumpkin (Cucurbita maxima Duch.). Weed Technol. 10, 341–346. doi: 10.1017/S0890037X00040069
Hand L. C., Randell T. M., Nichols R. L., Steckel L. E., Basinger N. T., Culpepper A. S. (2021). Cover crops and residual herbicides reduce selection pressure for Palmer amaranth resistance to dicamba applied postemergence in cotton. Agron. J. 113, 5373–5382. doi: 10.1002/agj2.v113.6
Hartwig N. L., Ammon H (2002). Cover crops and living mulches. U.Weed Sci. 50, 688–699. doi: 10.1614/0043-1745(2002)050[0688:AIACCA]2.0.CO;2
Heap I. (2023). The international herbicide–resistant weed database. Available online at: http://www.weedscience.org (Accessed October 23, 2023).
Hothorn T., Bretz F., Westfall P. (2008). multcomp: Simultaneous inference in general parametric models. Available online at: https://CRAN.R–project.org/package=multcomp (Accessed October 14, 2023).
Huddell A. M., Thapa R., Marcillo G. S., Abendroth L. J., Ackroyd V. J., Armstrong S. D., et al. (2024). U.S. cereal rye winter cover crop growth database. Sci. Data 11, 200. doi: 10.1038/s41597-024-02996-9
Johnson G. A., Defelice M. S., Helsel Z. R. (1993). Cover crop management and weed control in corn (Zea mays). Weed Technol. 7, 425–430. doi: 10.1017/S0890037X00027834
Kessavalou A., Walters D. T. (1997). Winter rye as a cover crop following soybean under conservation tillage. Agron. J. 89, 68–74. doi: 10.2134/agronj1997.00021962008900010011x
Koehler–Cole K., Everhart S. E., Gu Y., Proctor C. A., Marroquin–Guzman M., Redfearn D. D., et al. (2020). Is allelopathy from winter cover crops affecting row crops? Agric. Environ. Lett. 5, e20015. doi: 10.1002/ael2.20015
Koger C. H., Reddy K. N. (2005). Effects of hairy vetch (Vicia villosa) cover crop and banded herbicides on weeds, grain yield, and economic returns in corn (Zea mays). J. Sustain. Agric. 26, 3. doi: 10.1300/J064v26n03_11
Lal R. (2004). Soil carbon sequestration impacts on global climate change and food security. Science 304, 1623–1627. doi: 10.1126/science.1097396
Lenth R. V. (2022). emmeans: Estimated marginal means, aka least–squares means. Available online at: https://CRAN.R–project.org/package=emmeans (Accessed October 14, 2023).
Liebl R., Simmons F., Wax L., Stoller E. (1992). Effect of rye (Secale cereale) mulch on weed control and soil moisture in soybean (Glycine max). Weed Technol. 6, 838–846. doi: 10.1017/S0890037X00036356
Lin B. B. (2011). Resilience in agriculture through crop diversification: adaptive management for environmental change. BioScience 61, 183–193. doi: 10.1525/bio.2011.61.3.4
Marcillo G. S., Miguez F. E. (2017). Corn yield response to winter cover crops: An updated meta-analysis. J. Soil Water Conserv. 72, 226–239. doi: 10.2489/jswc.72.3.226
Martinez-Feria R. A., Dietzel R., Liebman M., Helmers M. J., Archontoulis S. V. (2016). Rye cover crop effects on maize: A system-level analysis. Field Crops Res. 196, 145–159. doi: 10.1016/j.fcr.2016.06.016
Miguez F. E., Bollero G. A. (2005). Review of corn yield response under winter cover cropping systems using meta–analytic methods. Crop Sci. 45, 2318–2329. doi: 10.2135/cropsci2005.0014
Mirsky S., Curran W., Mortenseny D., Ryany M., Shumway D. (2011). Timing of cover–crop management effects on weed suppression in no–till planted soybean using a roller–crimper. Weed Sci. 59, 380–389. doi: 10.1614/WS-D-10-00101.1
Mitchell W. H., Tell M. R. (1977). Winter–annual cover crops for no–tillage corn production. Agron. J. 69, 569–573. doi: 10.2134/agronj1977.00021962006900040011x
Munawar A., Blevins R. L., Frye W. W., Saul M. R. (1990). Tillage and cover crop management for soil water conservation. Agron. J. 82, 773–777. doi: 10.2134/agronj1990.00021962008200040024x
Norsworthy J. K., Ward S. M., Shaw D. R., Llewellyn R. S., Nichols R. L., Webster T. M., et al. (2012). Reducing the risks of herbicide resistance: Best management practices and recommendations. Weed Sci. 60, 31–62. doi: 10.1614/WS-D-11-00155.1
Ormeño–Núñez J., Pino–Rojas G., Garfe–Vergara F. (2008). Inhibition of yellow nutsedge (Cyperus esculentus L.) and Bermudagrass (Cynodon dactylon (L.) Pers.) by a mulch derived from rye (Secale cereale L.) in grapevines. Chil. J. Agric. Res. 68, 238–247. doi: 10.4067/S0718-58392008000300003
Osipitan O. A., Dille J. A., Assefa Y., Knezevic S. (2018). Cover crop for early season weed suppression in crops: systematic review and meta–analysis. Agron. J. 110, 2211–2221. doi: 10.2134/agronj2017.12.0752
Pittman K. B., Barney J. N., Flessner M. L. (2020). Cover crop residue components and their effect on summer annual weed suppression in corn and soybean. Weed Sci. 68, 301–310. doi: 10.1017/wsc.2020.16
Poeplau C., Don A. (2015). Carbon sequestration in agricultural soils via cultivation of cover crops–a meta–analysis. Agric. Ecosyst. Environ. 200, 33–41. doi: 10.1016/j.agee.2014.10.024
Price A. J., Balkcom K. S., Duzy L. M., Kelton J. A. (2012). Herbicide and cover crop residue integration for Amaranthus control in conservation agriculture cotton and implications for resistance management. Weed Technol. 26, 490–498. doi: 10.1614/WT-D-11-00127.1
Raimbault B. A., Vyn T. J., Tollenaar M. (1990). Corn response to rye cover crop management and spring tillage systems. Agron. J. 82, 1088–1093. doi: 10.2134/agronj1990.00021962008200060012x
R Core Team (2024). R: A language and environment for statistical computing (Vienna, Austria: R Foundation for Statistical Computing). Available online at: https://www.R–project.org/ (Accessed October 14, 2023).
Reed H. K., Karsten H. D., Curran W. S., Tooker J. F., Duiker S. W. (2019). Planting green effects on corn and soybean production. Agron. J. 111, 2314–2325. doi: 10.2134/agronj2018.11.0711
Reese C. L., Clay D. E., Clay S. A., Bich A. D., Kennedy A. C., Hansen S. A., et al. (2014). Winter cover crops impact on corn production in semiarid regions. Agron. J. 106, 1479–1488. doi: 10.2134/agronj13.0540
Runck B. C., Khoury C. K., Ewing P. M., Kantar M. (2020). The hidden land use cost of upscaling cover crops. Commun. Biol. 3, 300. doi: 10.1038/s42003-020-1022-1
Sainju U. M., Singh B. P. (2001). Tillage, cover crop, and kill–planting date effects on corn yield and soil nitrogen. Agron. J. 93, 878–886. doi: 10.2134/agronj2001.934878x
Sainju U. M., Whitehead W. F., Singh B. P., Wang S. (2006). Tillage, cover crops, and nitrogen fertilization effects on soil nitrogen and cotton and sorghum yields. Eur. J. Agron. 25, 372–382. doi: 10.1016/j.eja.2006.07.005
Schulz M., Marocco A., Tabaglio V., Macias F. A., Molinillo J. M. G. (2013). Benzoxazinoids in rye allelopathy: From discovery to application in sustainable weed control and organic farming. J. Chem. Ecol. 39, 154–174. doi: 10.1007/s10886-013-0235-x
Searle S. R., Speed F. M., Milliken G. A. (1980). Population marginal means in the linear model: An alternative to least squares means. Am. Stat. 34, 216–221. doi: 10.1080/00031305.1980.10483031
Seifert C. A., Azzari G., Lobell D. B. (2018). Satellite detection of cover crops and their effects on crop yield in the Midwestern United States. Environ. Res. Lett. 13, 064033. doi: 10.1088/1748-9326/aac4c8
Snapp S. S., Swinton S. M., Labarta R., Mutch D., Black J. R., Leep R., et al. (2005). Evaluating cover crops for benefits, costs and performance within cropping system niches. Agron. J. 97, 322–332. doi: 10.2134/agronj2005.0322a
Strock J. S., Porter P. M., Russelle M. P. (2004). Cover cropping to reduce nitrate loss through subsurface drainage in the northern US Corn Belt. J. Environ. Qual. 33, 1010–1016. doi: 10.2134/jeq2004.1010
Stroup W. W. (2015). Rethinking the analysis of non–normal data in plant and soil science. Agron. J. 107, 811–827. doi: 10.2134/agronj2013.0342
Studebaker G., Bateman N., Davis J., Cato A., Thrash B., Lee J., et al. (2022). MP144 2022, insecticide recommendation for arkansas (Little Rock: University of Arkansas System Division of Agriculture, Research and Extension), 332.
Teasdale J. R., Mohler C. L. (1993). Light transmittance, soil temperature, and soil moisture under residue of hairy vetch and rye. Agron. J. 85, 673–680. doi: 10.2134/agronj1993.00021962008500030029x
Teasdale J. R., Mohler C. R. (2002). The quantitative relationship between weed emergence and the physical properties of mulches. Weed Sci. 48, 385–392. doi: 10.1614/0043-1745
Tollenaar M., Mihajlovic M., Vyn T. J. (1993). Corn growth following cover crops: Influence of cereal cultivar, cereal removal, and nitrogen rate. Agron. J. 85, 252–255. doi: 10.2134/agronj1993.00021962008500020017x
Tonitto C., David M. B., Drinkwater L. E. (2006). Replacing bare fallows with cover crops in fertilizer–intensive cropping systems: a meta–analysis of crop yield and N dynamics. Agric. Ecosyst. Environ. 112, 58–72. doi: 10.1016/j.agee.2005.07.003
Wagger M. G. (1989). Time of desiccation effects on plant composition and subsequent nitrogen release from several winter annual cover crops. Agron. J. 81, 236–241. doi: 10.2134/agronj1989.00021962008100020020x
Ward S. M., Webster T. M., Steckel L. E. (2013). Palmer amaranth (Amaranthus palmeri): A review. Weed Technol. 27, 12–27. doi: 10.1614/WT-D-12-00113.1
Webster T. M., Simmons D. B., Culpepper A. S., Grey T. L., Bridges D. C., Scully B. T. (2016). Factors affecting potential for Palmer amaranth (Amaranthus palmeri) suppression by winter rye in Georgia, USA. Field Crops Res. 192, 103–109. doi: 10.1016/j.fcr.2016.04.020
Westo L. (1996). Utilization of allelopathy for weed management in agroecosystems. Agron. J. 88, 860–866. doi: 10.2134/agronj1996.00021962003600060004x
Wiggins M. S., Hayes R. M., Steckel L. E. (2016). Evaluating cover crops and herbicides for glyphosate-resistant Palmer amaranth (Amaranthus palmeri) control in cotton. Weed Technol. 30, 415–422. doi: 10.1614/WT-D-15-00113.1
Keywords: cereal rye, cover crop, integrated weed management, herbicide selection pressure, cover crop termination timings
Citation: Godar AS, Norsworthy JK and Barber LT (2024) Effect of cereal rye cover crop termination timings on weed control and corn yield under a two-pass herbicide program. Front. Agron. 6:1419228. doi: 10.3389/fagro.2024.1419228
Received: 17 April 2024; Accepted: 18 October 2024;
Published: 15 November 2024.
Edited by:
Shibu Jose, University of Missouri, United StatesReviewed by:
Gourav Sharma, Bayer Crop Science, United StatesAnnu Kumari, FMC Agricultural Solutions, United States
Sougata Bardhan, Lincoln University, United States
Copyright © 2024 Godar, Norsworthy and Barber. This is an open-access article distributed under the terms of the Creative Commons Attribution License (CC BY). The use, distribution or reproduction in other forums is permitted, provided the original author(s) and the copyright owner(s) are credited and that the original publication in this journal is cited, in accordance with accepted academic practice. No use, distribution or reproduction is permitted which does not comply with these terms.
*Correspondence: Amar S. Godar, YWdvZGFyQHVhcmsuZWR1