- 1Department of Agronomy and Horticulture, University of Nebraska-Lincoln, Lincoln, NE, United States
- 2Department of Horticulture, Oregon State University, Corvallis, OR, United States
- 3Department of Entomology, University of Nebraska-Lincoln, Lincoln, NE, United States
Palmer amaranth is a relatively recent arrival in Western Nebraska, where acetolactate synthase (ALS)-inhibitor-resistant biotypes are common in the region. With limited effective postemergence (POST) herbicides for controlling ALS-inhibitor-resistant Palmer amaranth in dry edible bean, a sequential preemergence (PRE) followed by (fb) POST program of very long-chain fatty acid (VLCFA)-inhibiting herbicides shows promise. Currently, dimethenamid-P is the only VLCFA-inhibiting herbicide registered for POST use in dry edible bean in Nebraska. The objective of this study was to assess the crop safety and effectiveness in weed control of sequential PRE fb POST programs, including pendimethalin + dimethenamid-P applied PRE fb dimethenamid-P POST, pendimethalin + S-metolachlor PRE fb S-metolachlor POST, and pendimethalin + pyroxasulfone PRE fb POST, in comparison with pendimethalin + dimethenamid-P applied PRE fb imazamox + bentazon + fomesafen applied POST in dry edible bean. Results showed that sequential PRE fb POST programs were more effective in reducing both the density and biomass of Palmer amaranth compared to PRE-alone programs. Pendimethalin + dimethenamid-P applied PRE fb dimethenamid-P POST, along with pendimethalin + S-metolachlor PRE fb S-metolachlor POST, resulted in over 85% control of Palmer amaranth, similar to the effectiveness of pendimethalin + dimethenamid-P PRE fb fomesafen + imazamox + bentazon applied POST. Pendimethalin + pyroxasulfone applied PRE-alone and the application of pendimethalin + pyroxasulfone PRE fb pyroxasulfone POST showed inconsistent control of Palmer amaranth, causing high crop injury, stand loss, and delayed maturity, ultimately leading to yield loss. Dimethenamid-P and S-metolachlor demonstrated excellent crop safety when applied either PRE-only or sequentially. Pendimethalin + S-metolachlor PRE fb S-metolachlor POST provided control of Palmer amaranth comparable to that achieved with POST applications of dimethenamid-P and imazamox + bentazon + fomesafen.
1 Introduction
Dry edible bean is an important crop globally (Adjesiwor et al., 2020). The United States ranks as the fourth-largest producer of dry edible bean worldwide (Yonts et al., 2018; Lucier and Davis, 2020), generating over 1.49 million tons valued at over $1 billion in 2020 alone (Statista, 2021a, 2021b). Nebraska stands as the fourth-leading producer of dry edible bean in the United States, alongside North Dakota, Michigan, and Minnesota, which collectively account for 86% of the country’s production in 2020 (Lucier and Davis, 2020). However, weed management poses a significant challenge in dry edible bean production due to the emergence and proliferation of herbicide-resistant weeds (Heap, 2024) and the lack of effective herbicides (Poling et al., 2009). Compared to corn or soybeans, dry edible bean crops are more vulnerable to weed interference, primarily due to their slower growth rate and shorter stature (Soltani et al., 2016, 2017, 2018a).
Palmer amaranth, a relatively recent arrival in Western Nebraska, is becoming increasingly more prevalent, particularly in irrigated areas, with acetolactate synthase (ALS)-resistant biotypes becoming widespread (Beiermann et al., 2021a). This summer annual weed species is highly competitive and poses a challenge to control efforts due to its ability to emerge throughout the growing season and the prevalence of herbicide-resistant biotypes (Chandi et al., 2012; Ward et al., 2013; Korres et al., 2019). Interference from Palmer amaranth has been extensively studied across various row crops, revealing significant yield reductions regardless of crop type, density, or emergence timing (Klingaman and Oliver, 1994; Massinga et al., 2001; Burke et al., 2007; Chandi et al., 2012). In dry edible beans, Palmer amaranth interference can lead to a substantial yield decrease, with studies showing up to a 77% reduction in yield at a density of 2 plants m−1 row (Miranda et al., 2022a). Even at lower densities, 0.04 plants m−2, Palmer amaranth interference can lead to a 5% yield reduction (Miranda et al., 2022a). Miranda et al. (2022a) further highlighted the prolific seed production of Palmer amaranth, reporting seed yields ranging from 91,000 to 376,000 seeds plant−1 and 140,000 seeds m−2 in a two-year study conducted in western Nebraska.
While effective preplant-incorporated (PPI) and PRE herbicides are available in Nebraska for controlling Palmer amaranth in dry edible beans, there is a notable lack of effective labeled POST herbicides (Knezevic et al., 2020; Beiermann et al., 2021b). This scarcity underscores the need for new herbicide programs. Commonly used dry edible bean POST herbicides for controlling pigweed species (Amaranthus spp.) include halosulfuron, imazamox, and imazethapyr, which are ALS-inhibiting herbicides (WSSA group 2). However, these herbicides are ineffective against ALS-inhibitor-resistant biotypes. Similarly, bentazon, a PS II-inhibitor herbicide (WSSA group 6), commonly used, fails to provide adequate control of pigweed species (Chahal et al., 2017). Currently, the only effective POST herbicide for controlling ALS-inhibitor-resistant pigweed species in dry edible beans is fomesafen, a PPO-inhibitor (Wilson, 2005). However, label guidelines were modified in 2021, restricting its rotation to corn to 18 months after application (Anonymous, 2021). Since corn is the primary rotational crop following dry edible beans in Western Nebraska, fomesafen is no longer a viable option for most growers in the region.
A sequential PRE followed by (fb) POST application, also known as a layby application program, of soil-residual herbicides presents a promising alternative to POST herbicides. The objective of this approach is to overlap and prolong residual control until crop canopy closure (McCloskey, 2001). Very long chain fatty acid (VLCFA)-inhibiting herbicides, categorized under Weed Science Society of America (WSSA) Group 15 herbicides, are integral components of sequential PRE fb POST programs, providing effective control of several herbicide-resistant pigweed species (Steckel et al., 2002; Jhala et al., 2015; Chahal et al., 2018; Goodrich et al., 2018; Miranda et al., 2022b). Pigweed species often exhibit resistance to multiple herbicide modes of action, making the evaluation of VLCFA-inhibiting herbicides critical (Jhala et al., 2024) These herbicides, including dimethenamid-P, S-metolachlor and pyroxasulfone, offer a potential solution for effective season-long control of Palmer amaranth in dry edible beans (Wilson and Sbatella, 2014; Soltani et al., 2018b). They have demonstrated efficacy against a broad spectrum of weeds, including grass weeds, nutsedges, and small-seeded broadleaf weeds such as pigweed species, including those resistant to glyphosate, ALS, and PSII inhibitors, across multiple crops (Soltani et al., 2006; Mueller and Steckel, 2011; Li et al., 2017, 2014b; Stephenson et al., 2017). Despite their broad efficacy, concerns about resistance are increasing. As of April 2024, there have been 13 weed species identified with resistance to these herbicides (Heap, 2024). While instances of resistance to VLCFA inhibitors have been reported less frequently than with glyphosate, ALS inhibitors, and PSII inhibitors, the rising occurrence of resistance, particularly in waterhemp and Palmer amaranth, underscores the urgency for proactive resistance management strategies. The increasing trend of resistance requires diligent surveillance and the implementation of integrated weed management approaches to maintain the effectiveness of these herbicides in crop protection (Jhala et al., 2024).
In dry edible bean, dimethenamid-P is registered for PRE, PPI, and POST use (Anonymous, 2019), while S-metolachlor is registered for PRE and PPI (Anonymous, 2020). Pyroxasulfone is not currently registered for use in dry edible beans (Anonymous, 2017). Previous research has shown that dimethenamid-P applied sequentially PRE fb POST is as effective as POST programs containing fomesafen for Palmer amaranth management in dry edible bean (Miranda et al., 2022b). There is potential to expand the utilization of S-metolachlor and pyroxasulfone in dry edible bean cultivation if their efficacy in weed control and crop safety can be demonstrated as viable alternatives to dimethenamid-P and fomesafen. The objective of this field study was to assess crop safety and efficacy of dimethenamid-P, S-metolachlor, and pyroxasulfone for control of ALS-inhibitor-resistant Palmer amaranth in dry edible bean.
2 Materials and methods
2.1 Site description
This field study was conducted during the growing seasons of 2020 and 2021 at the University of Nebraska-Lincoln Panhandle Research and Extension Center in Scottsbluff, Nebraska (41.893 °N, 103.680 °W). Soil type was a Tripp fine sandy loam (coarse-silty, mixed, superactive, mesic Aridic Haplustolls) (NCSS, 2006; USDA-NRCS, 2019) with a pH range from 8.1 to 8.3 and an organic matter content range from 1.1% to 1.7%. Common weed species at the experiment site include bristly foxtail (Setaria verticillata (L.) Beauv.), common lambsquarters (Chenopodium album L.), hairy nightshade (Solanum physalifolium Rusby), kochia (Bassia scoparia (L.) A. J. Scott), and Palmer amaranth. The Palmer amaranth population present at the experiment location is resistant to ALS-inhibiting herbicides. The preceding crop in the experiment site was sugar beet (Beta vulgaris L.) in both years.
2.2 Treatments and experimental design
This field study was designed as a randomized complete block design with four replications. The dry edible bean cultivar ‘SV6139GR’ (Seminis, 800 N Lindbergh Blvd, St. Louis, MO 63167) was planted on May 26 in 2020 and the cultivar ‘Cowboy’ (ADM Seedwest, 4666 Faries Parkway Decatur, IL 62526) was planted on June 1 in 2021. Both dry edible bean cultivars are upright pinto with indeterminate growth habits. Dry edible beans were planted at a density of 210,000 seeds ha−1, using a 0.56 m row spacing. Each plot consisted of four 9.1 m long rows. Fertilizer in the form of N and P2O5 were dry applied at 112 kg ha−1 and 45 kg ha−1 prior to experiment establishment, respectively. Glyphosate was applied at 1,261 g ae ha−1 + ammonium sulfate (AMS) at 3% vol/vol + nonionic surfactant (NIS) at 0.25% vol/vol within one day after dry edible bean planting as a burndown treatment to control emerged weeds. Irrigation was provided throughout the season using an overhead lateral-move irrigation system.
The treatments included a nontreated control (weedy), pendimethalin + dimethenamid-P applied PRE-alone, pendimethalin + S-metolachlor applied PRE-alone, pendimethalin + pyroxasulfone applied PRE-alone, pendimethalin + dimethenamid-P applied PRE fb dimethenamid-P POST at V1 (first trifoliate growth stage), pendimethalin + S-metolachlor applied PRE fb S-metolachlor POST at V1, pendimethalin + pyroxasulfone applied PRE fb pyroxasulfone POST at V1, and pendimethalin + dimethenamid-P PRE fb imazamox + bentazon + fomesafen at V1 (Table 1). The inclusion of pendimetalin + dimethenamid-P PRE fb imazamox + bentazon + fomesafen at V1 was specifically chosen for its high efficacy in weed control, as fomesafen provides excellent control of ALS-inhibitor-resistant Palmer amaranth.
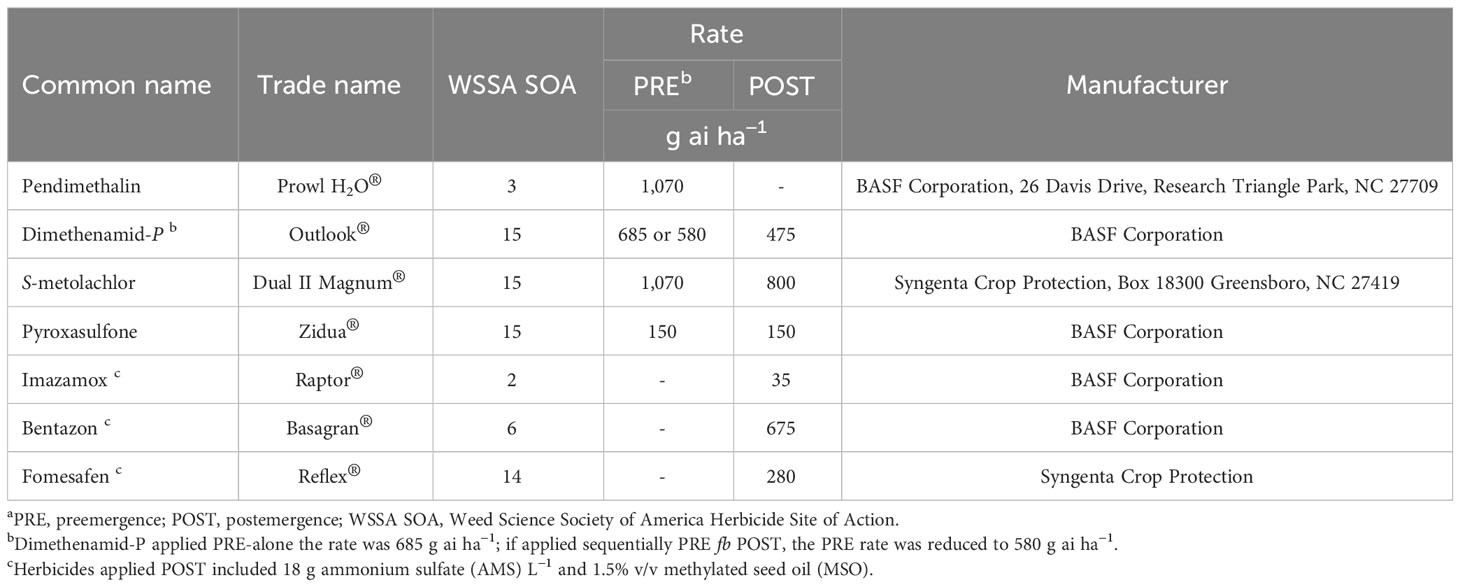
Table 1 Common names, trade names, WSSA classification, application rates for PRE or POST application timings, and manufacturers of herbicides evaluated in dry edible bean for ALS-inhibitor-resistant Palmer amaranth control in field experiments conducted at the University of Nebraska Panhandle Research and Extension Center, Scottsbluff, NEa.
In 2020, PRE applications occurred on May 28, while in 2021, PRE applications occurred on June 2. Herbicides applied PRE were incorporated into the soil with 12.5 mm of irrigation within 24 h after their application. In 2020, POST applications occurred on June 15, while in 2021, POST applications occurred on June 17. Soil-residual herbicides applied POST were incorporated into the soil with 6.3 mm of irrigation within 24 h after their application. AMS (WinField United, 4001 Lexington Ave N, Arden Hills, MN 55126) at 18 g L−1 and methylated seed oil (MSO) (WinField United, 4001 Lexington Ave N, Arden Hills, MN 55126) at 1.5% v/v were used with all POST applications which included imazamox, bentazon, and fomesafen. Herbicides were applied with a CO2 pressurized backpack sprayer equipped with four TeeJet 11002 AIXR nozzles (TeeJet® Technologies, Spraying Systems Co., P.O. Box 7900, Wheaton, IL 60187) spaced at 51 cm and calibrated to deliver 140 L hectare–1 of spray solution at 160 kPa.
2.3 Data collection
Palmer amaranth control was visually estimated every 14 days after POST treatment (DAT) until harvest, using a scale ranging from 0 to 100%, where 0% represented no control and 100% represented complete control. The assessment of injury to dry edible beans was assessed at 0, 14, 28, and 42 DAT. The degree of injury, noting typical responses to PPO-inhibitors and VLCFA-inhibiting herbicides such as bronzing, necrosis, leaf deformation, and stunting, was visually rated using a percentage scale from 0%, indicating no visible injury to the beans, to 100%, indicating complete plant mortality. The evaluation at 0 DAT was conducted within 4–6 hours after POST treatment. Weed density was recorded every 14 DAT until harvest by randomly placing two 0.5 m2 quadrants in each plot. At harvest, density was assessed, and above-ground Palmer amaranth biomass was also determined from the same quadrants. The Palmer amaranth aboveground biomass was oven-dried at 60°C for one week before being weighed. To streamline the presentation of results, only Palmer amaranth density measurements and control ratings at harvest, as well as crop injury at 0, 14, and 28 DAT, were reported. Dry edible beans were harvested on September 16, 2020, and September 8, 2021, by hand-pulling all plants from 6.1 m of row in the second and third rows. These hand-pulled dry edible bean plants were threshed using a Zurn 150 plot combine (ZURN USA Inc., 9210 Wyoming Ave N, Brooklyn Park, MN 55445), which served as a static threshing machine. Yield and moisture per plot were collected using a H2 Classic GrainGage (Juniper Systems & HarvestMaster Inc., 1132 W 1700 N Logan, UT, 84321) placed on the plot combine. Yields were adjusted to 15% moisture content.
2.4 Statistical analysis
The data were analyzed using R version 4.0.2 (R Core Team, 2020). Visual estimated Palmer amaranth control and crop injury data were transformed to a beta-distribution (values between 0 and 1) to handle percent data more effectively (Douma and Weedon, 2019). These data were then analyzed using a beta regression with the ‘glmmTMB’ function in the ‘glmmTMB’ package version 1.0.2.1 (Brooks et al., 2017), along with Analysis of Variance (ANOVA) at the 5% level of significance. For the analysis of crop injury, only data from treatments containing pyroxasulfone and fomesafen were included, as no injury was recorded in the remaining treatments (Sarangi and Jhala, 2018). Dry edible bean yield and Palmer amaranth dry biomass data were analyzed using a linear mixed model (LMM) with the ‘lmer’ function in the ‘lme4’ package version 1.1-23 (Bates et al., 2015), along with ANOVA at the 5% level of significance. Palmer amaranth dry biomass residuals were square-root transformed to improve normality and homogeneity. Back-transformed mean values and their standard errors were presented based on transformed data for better interpretation (Onofri et al., 2010). Palmer amaranth density was analyzed using a generalized linear mixed-effect model (GLMM) with a Poisson error distribution, utilizing the ‘lme4’ package version 1.1-23 (Bates et al., 2015), along with ANOVA at the 5% level of significance. The ANOVA was performed using the ‘Anova’ function in the ‘car’ package version 3.0-10 (Fox and Weisberg, 2019). For LMM models, Type III Wald F-tests were conducted in the ANOVA, while Type III Wald chi-square tests were conducted for GLMM and glmmTMB models. Herbicide treatment was considered a fixed effect, and replications were considered random effects. Response variables from each year of the experiment were analyzed separately due to variations in treatments between years. ANOVA assumptions of normality and homogeneity of variance were evaluated by plotting residuals for models assuming normal distributions (Onofri et al., 2010; Kniss and Streibig, 2018). Whenever a significant herbicide treatment effect was found, means were separated using estimated marginal means (also known as least-squares means) with the ‘emmeans’ function in the ‘emmeans’ package version 1.5.1 (Lenth, 2020), and the 5% Fisher’s protected least significant difference (LSD) test was calculated using the ‘cld’ function in the ‘multcomp’ package version 1.4-14 (Hothorn et al., 2008).
3 Results
3.1 Environmental conditions and irrigation
In both 2020 and 2021, the average temperatures were higher than the 30-year average by 0.9°C and 1.1°C, respectively (Table 2). During the months of June and July in 2021, average temperatures were higher compared to those in 2020 by 1°C and 0.5°C, respectively. Additionally, rainfall recorded in 2020 and 2021 was lower than the 30-year average by 46% and 33%, respectively. Consequently, the growing seasons of both 2020 and 2021 were notably warmer and drier compared to the long-term average. Irrigation was consistently provided throughout both growing seasons, and despite the variation in rainfall between 2020 and 2021, its impact on the study outcomes in either year would have been minimal.
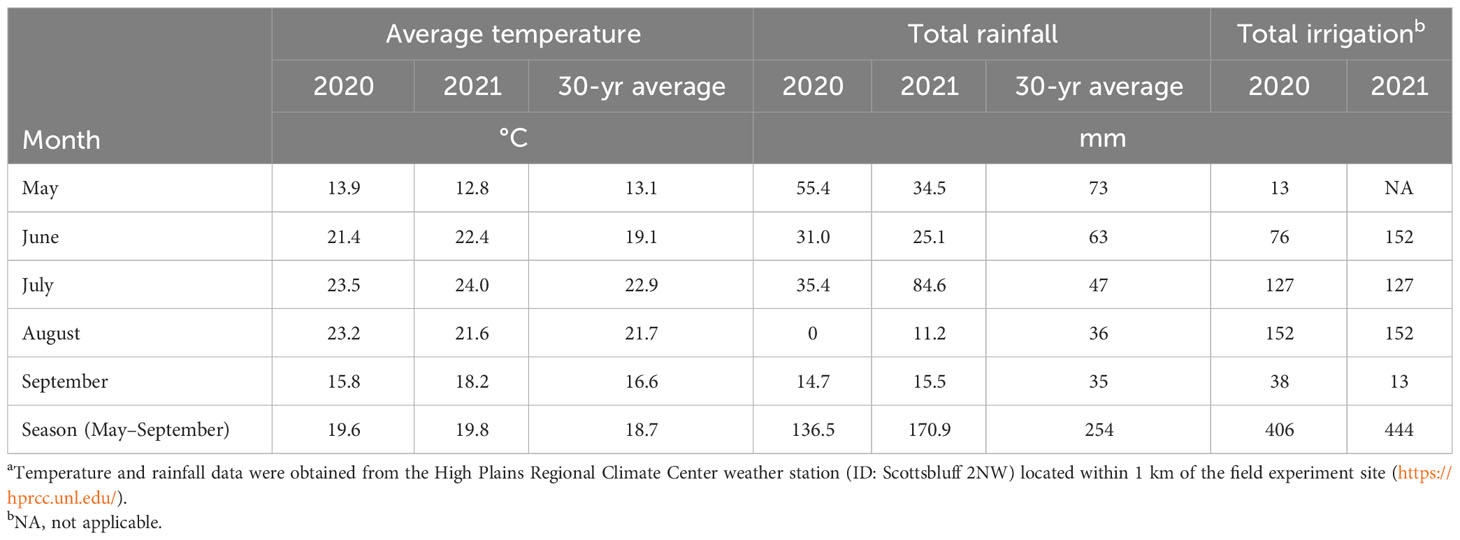
Table 2 Comparison of average monthly temperature, total rainfall, and total irrigation for the 2020 and 2021 growing seasons with the 30-year average at the University of Nebraska Panhandle Research and Extension Center, Scottsbluff, NEa.
3.2 Palmer amaranth control
Pendimethalin + dimethenamid-P applied PRE-alone, along with pendimethalin + S-metolachlor applied PRE-alone, controlled <73% Palmer amaranth in 2020 and 2021 (Table 3). Sequential PRE fb POST programs, including pendimethalin + dimethenamid-P applied PRE fb dimethenamid-P POST, pendimethalin + S-metolachlor applied PRE fb S-metolachlor POST, and pendimethalin + pyroxasulfone applied PRE fb pyroxasulfone POST, exhibited enhanced control of Palmer amaranth compared to PRE-alone programs, surpassing 87% control in both years (Table 3).
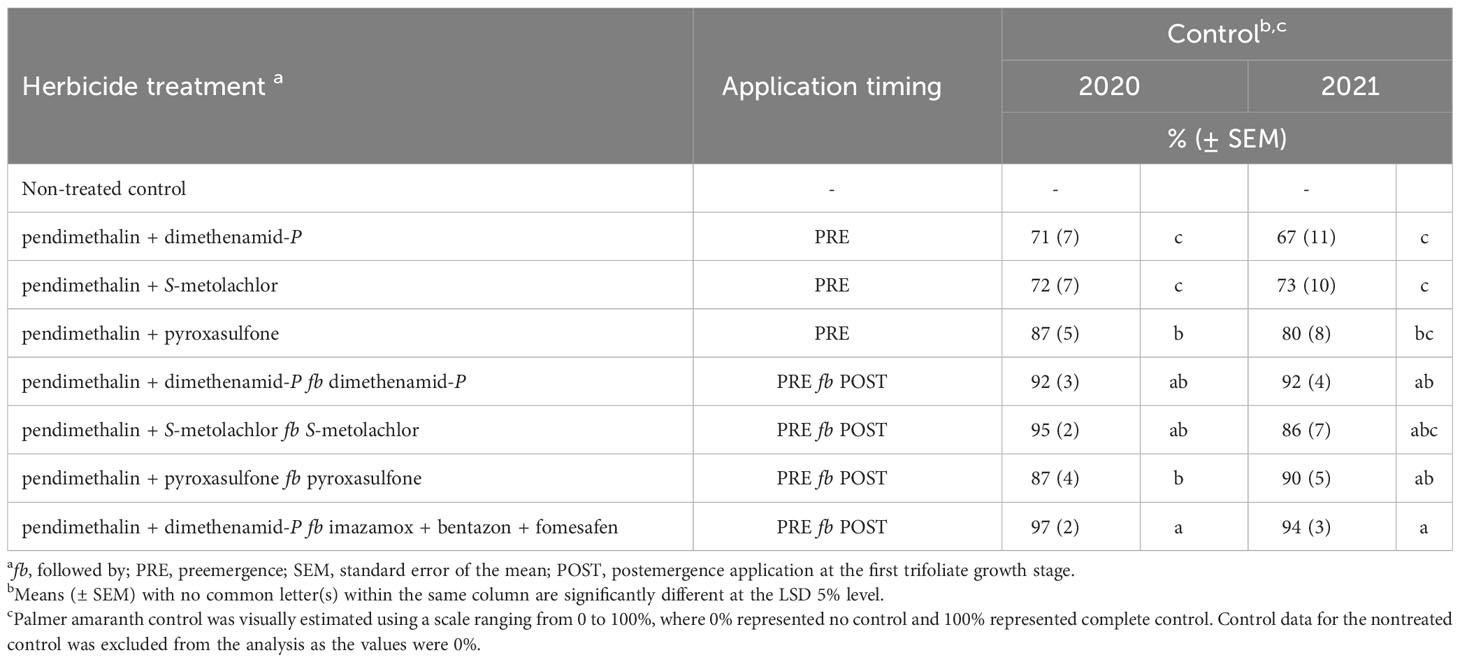
Table 3 Impact of herbicide programs on the control of ALS-inhibitor-resistant Palmer amaranth in dry edible bean at harvest, derived from field experiments conducted at the University of Nebraska Panhandle Research and Extension Center, Scottsbluff, NEa.
In 2020, pendimethalin + dimethenamid-P applied PRE-alone along with pendimethalin + S-metolachlor applied PRE-alone, provided 71% to 72% Palmer amaranth control (Table 3). Pendimethalin + pyroxasulfone applied PRE-alone and pendimethalin + pyroxasulfone applied PRE fb pyroxasulfone POST, provided 87% control of Palmer amaranth. Although pendimethalin + pyroxasulfone PRE fb pyroxasulfone POST did not improve the control compared to using pendimethalin + pyroxasulfone PRE-alone, applying pendimethalin + dimethenamid-P PRE fb dimethenamid-P POST and pendimethalin + S-metolachlor PRE fb S-metolachlor POST significantly improved control compared to their PRE-alone counterparts, achieving 21–23% greater control. These sequential PRE fb POST treatments provided >92% control of Palmer amaranth, similar to the fomesafen-containing treatment.
In 2021, both pendimethalin + S-metolachlor and pendimethalin + pyroxasulfone applied PRE-alone provided 73–80% control of Palmer amaranth, similar to the control achieved by pendimethalin + dimethenamid-P applied PRE-alone (67%) (Table 3). The application of pendimethalin + pyroxasulfone PRE fb pyroxasulfone POST significantly enhanced Palmer amaranth control to 90% compared to its PRE-alone application. Pendimethalin + dimethenamid-P PRE fb dimethenamid-P POST, pendimethalin + S-metolachlor PRE fb S-metolachlor POST, and pendimethalin + pyroxasulfone PRE fb pyroxasulfone POST, yielded similar levels of Palmer amaranth control (>90%) as the fomesafen-containing treatment. Interestingly, in 2021, pendimethalin + dimethenamid-P PRE fb dimethenamid-P POST provided similar Palmer amaranth control (92%) as in 2020, whereas pendimethalin + pyroxasulfone PRE fb pyroxasulfone POST showed slightly greater control, 90% in 2021 compared to 87% in 2020. Overall, the sequential PRE fb POST programs provided similar control levels as the fomesafen-containing treatment, with minor variations in performance between years.
3.3 Palmer amaranth density and biomass
The nontreated control had the greatest Palmer amaranth densities and biomass in both 2020 and 2021 (Table 4). Palmer amaranth biomass and densities in the nontreated control were greater in 2020 by 14% and 9% compared to 2021, respectively. The application of herbicides significantly reduced both Palmer amaranth densities and biomass accumulation in the nontreated control. While Palmer amaranth was the primary focus due to its dominance, it is important to note that the treatments also effectively controlled other weeds such as bristly foxtail, common lambsquarters, and hairy nightshade, which were present in the plots. This highlights the broad-spectrum efficacy of the herbicides used.
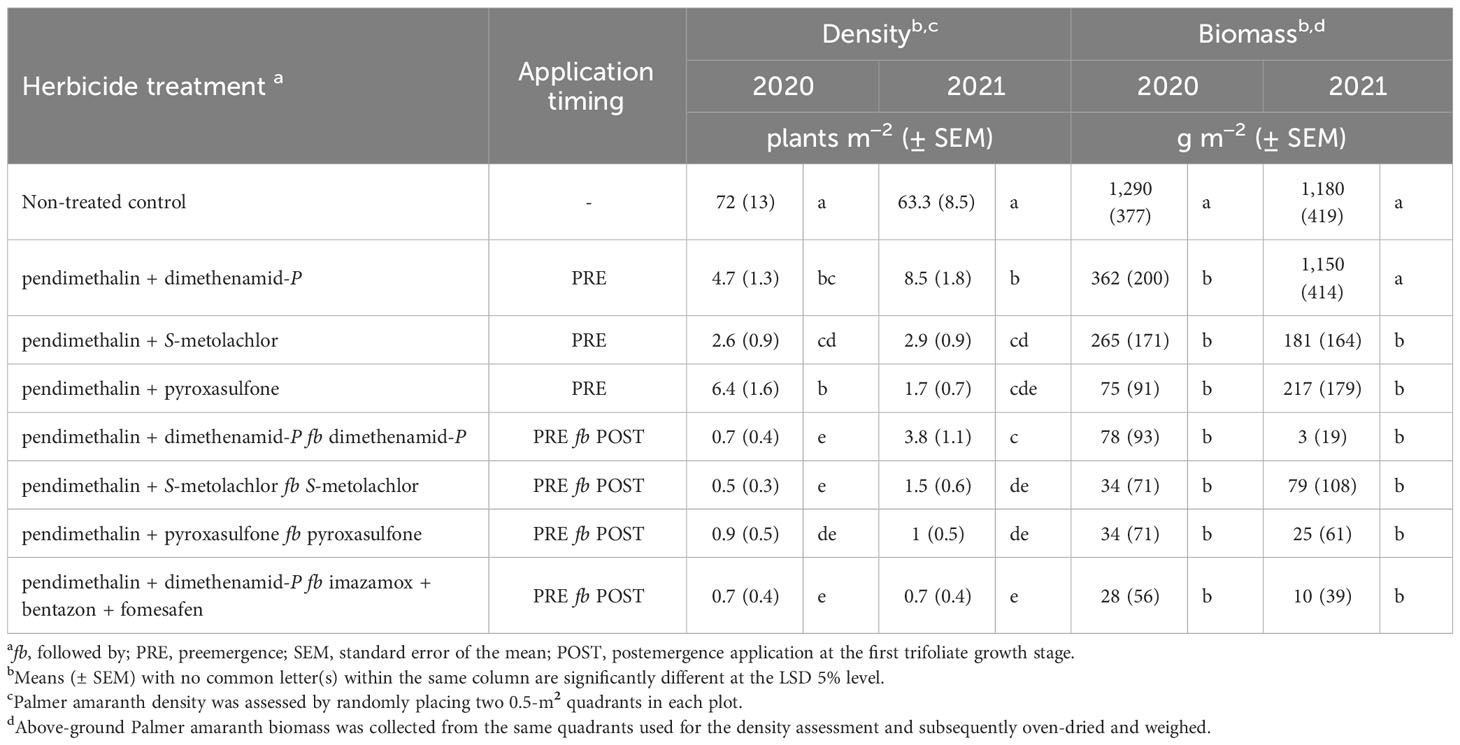
Table 4 Impact of herbicide programs on the density and biomass of ALS-inhibitor-resistant Palmer amaranth at harvest, as observed in field experiments conducted at the University of Nebraska Panhandle Research and Extension Center, Scottsbluff, NEa.
In 2020, pendimethalin + dimethenamid-P, pendimethalin + S-metolachlor, and pendimethalin + pyroxasulfone applied PRE-alone resulted in greater Palmer amaranth densities compared to pendimethalin + dimethenamid-P PRE fb dimethenamid-P POST, pendimethalin + S-metolachlor PRE fb S-metolachlor POST, and pendimethalin + pyroxasulfone PRE fb pyroxasulfone POST (Table 4). Pendimethalin + dimethenamid-P PRE fb dimethenamid-P POST (0.7 plants m−2), pendimethalin + S-metolachlor PRE fb S-metolachlor POST (0.5 plants m−2), and pendimethalin + pyroxasulfone PRE fb pyroxasulfone POST (0.9 plants m−2), provided similar Palmer amaranth densities as the fomesafen-containing treatment (0.7 plants m−2). Despite statistical differences among treatments in terms of Palmer amaranth densities, Palmer amaranth biomass was similar for all herbicide treatments. The application of pendimethalin + dimethenamid-P PRE fb dimethenamid-P POST, pendimethalin + S-metolachlor PRE fb S-metolachlor POST, and pendimethalin + pyroxasulfone PRE fb pyroxasulfone POST reduced Palmer amaranth dry biomass to 78, 34, and 34 g m−2, respectively.
In 2021, among the herbicide treatments, pendimethalin + dimethenamid-P applied PRE-alone provided the greatest Palmer amaranth densities, with 8.5 plants m−2 (Table 4). Pendimethalin + S-metolachlor and pendimethalin + pyroxasulfone applied PRE-alone and pendimethalin + S-metolachlor PRE fb S-metolachlor POST and pendimethalin + pyroxasulfone PRE fb pyroxasulfone POST resulted in similar Palmer amaranth densities, with fewer than 2.9 plants m−2. Pendimethalin + S-metolachlor PRE fb S-metolachlor POST, and pendimethalin + pyroxasulfone PRE fb pyroxasulfone POST yielded Palmer amaranth densities comparable to the fomesafen-containing treatment, with fewer than 1.5 plants m−2. Although the pendimethalin + dimethenamid-P PRE fb dimethenamid-P POST treatment improved the reduction of Palmer amaranth densities compared to pendimethalin + dimethenamid-P PRE-alone, it still provided greater densities (3.8 plants m−2) than the fomesafen-containing treatment. Despite the greater densities in the pendimethalin + dimethenamid-P PRE fb dimethenamid-P POST treatment, Palmer amaranth biomass was similar to that of the fomesafen-containing treatment. This suggests that although pendimethalin + dimethenamid-P PRE fb dimethenamid-P POST led to greater densities due to late-emerging flushes, the overall biomass remained comparable to the fomesafen-containing treatment. The most effective treatments in terms of Palmer amaranth biomass reduction were pendimethalin + dimethenamid-P PRE fb dimethenamid-P POST (3 g m−2), pendimethalin + S-metolachlor PRE fb S-metolachlor POST (79 g m−2), and pendimethalin + pyroxasulfone PRE fb pyroxasulfone POST (25 g m−2), which provided similar biomass reduction as the fomesafen-containing treatment.
3.4 Dry edible bean injury and yield
Only treatments containing pyroxasulfone and fomesafen caused injury to dry edible beans in both years, despite the differences in cultivars, which can vary significantly in their sensitivity to herbicides (Figure 1) (Soltani et al., 2006, 2014a, 2014b, 2018b). The symptoms observed were characteristic of PPO-inhibitors, including bronzing and necrosis, as well as symptoms typical of VLCFA-inhibiting herbicides such as leaf deformation, stand loss, and stunting. The greatest dry edible bean injury at 0 DAT was caused by pendimethalin + pyroxasulfone applied PRE-alone (47%) and pendimethalin + pyroxasulfone PRE fb pyroxasulfone POST (58–62%). The injury caused by pyroxasulfone decreased over time (Figure 1), with dry edible beans recovering faster in the pendimethalin + pyroxasulfone PRE-alone treatment compared to pendimethalin + pyroxasulfone PRE fb pyroxasulfone POST. In 2020, dry edible bean injury caused by pyroxasulfone decreased faster than in 2021, with injury rates at 27% and 48% at 14 DAT, respectively. By 28 DAT, no injury was observed in pendimethalin + pyroxasulfone applied PRE-alone, whereas minimal injury (1%) was detected in the pendimethalin + pyroxasulfone PRE fb pyroxasulfone POST treatment. The decrease in observed injury over time from treatments containing pyroxasulfone is likely due to the surviving plants growing and filling in the canopy. Fomesafen-containing treatment also caused greater injury in 2021 compared to 2020, with injury rates decreasing faster in 2020 than in 2021. Dry edible bean injury decreased from 6% at 0 DAT to 1% at 14 DAT to no injury at 28 DAT when fomesafen was applied POST in 2020. In 2021, dry edible injury decreased from 11% at 0 DAT to 6% at 14 DAT to 0.4% at 28 DAT. The observed recovery of the plants in fomesafen-containing treatments can be attributed to the gradual diminishing of leaf burning and necrosis over time, as new leaf tissue developed. Overall, pendimethalin + dimethenamid-P PRE fb dimethenamid-P POST and pendimethalin + S-metolachlor PRE fb S-metolachlor POST provided excellent crop safety.
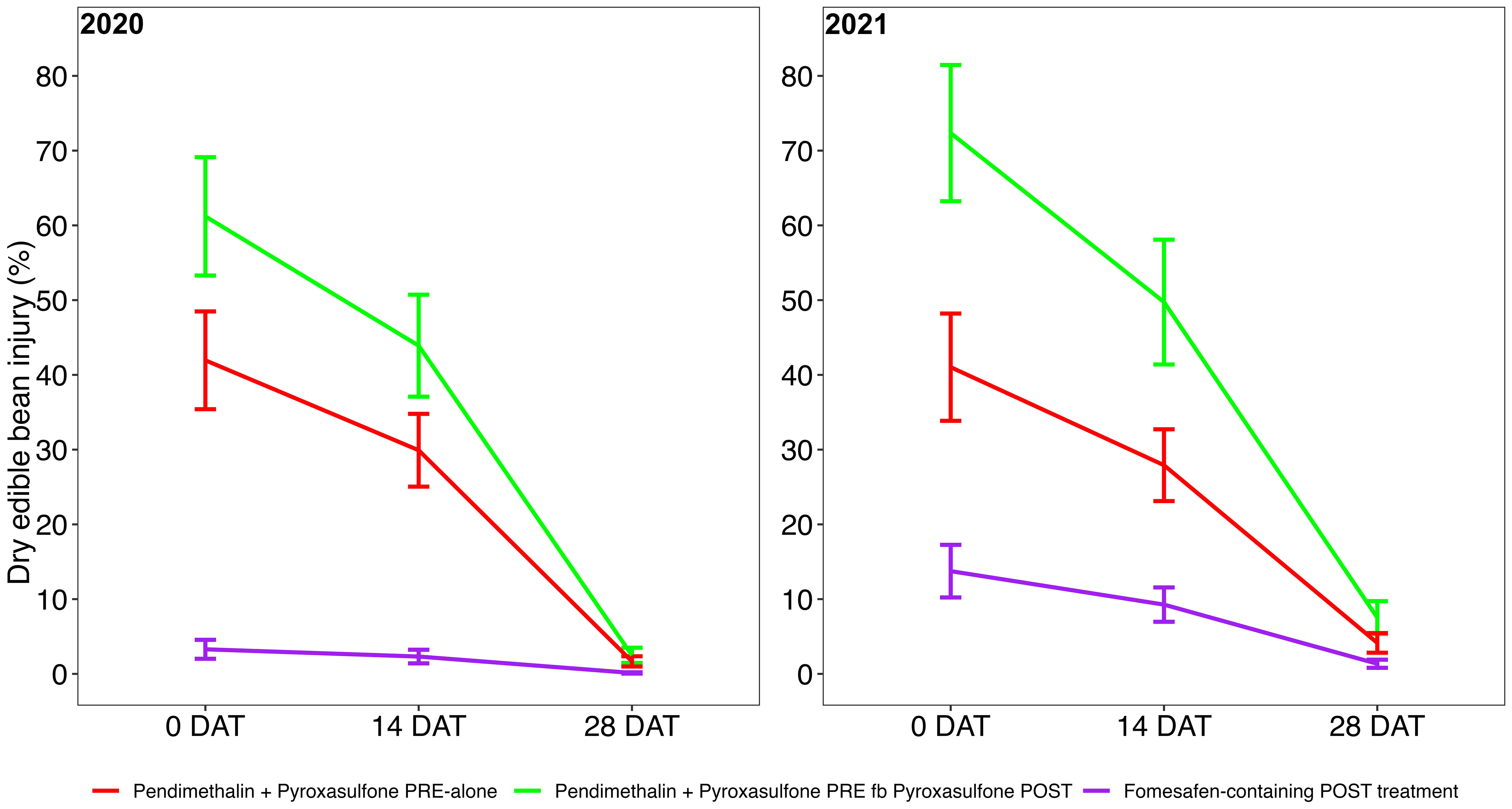
Figure 1 Percentages of dry edible bean injury [± SEM; standard error of the mean] at 0, 14, and 28 days after POST treatment (DAT). The evaluation at 0 DAT was conducted within 4–6 hours after POST treatment. The data were collected from field experiments conducted in 2020 (left) and 2021 (right) at the University of Nebraska Panhandle Research and Extension Center, Scottsbluff, NE.
The substantial reduction in dry edible bean yield in the nontreated control in both 2020 and 2021 underscores the significant impact of weed interference on crop productivity (Table 5). The greater yield in 2021 compared to 2020 can be attributed to the lower Palmer amaranth density in 2021 (Table 4). Among the herbicide treatments in 2020, pendimethalin + dimethenamid-P applied PRE-alone, pendimethalin + dimethenamid-P PRE fb dimethenamid-P POST, pendimethalin + S-metolachlor PRE fb S-metolachlor POST, and pendimethalin + pyroxasulfone applied PRE-alone exhibited the greatest dry edible bean yields, surpassing 3,740 kg ha−1, comparable to the fomesafen-containing treatment. Pendimethalin + S-metolachlor applied PRE-alone and pendimethalin + pyroxasulfone PRE fb pyroxasulfone POST resulted in the lowest yields, with 2,680 kg ha−1 and 1,930 kg ha−1, respectively. Similarly, in 2021, pendimethalin + pyroxasulfone PRE fb pyroxasulfone POST resulted in the lowest dry edible bean yield (1,860 kg ha−1), demonstrating substantial reductions compared to the top-performing treatments. The application of pendimethalin + dimethenamid-P, pendimethalin + S-metolachlor, and pendimethalin + pyroxasulfone PRE-alone led to notable yield decreases of about 40% when compared to the top-performing treatments. Pendimethalin + dimethenamid-P PRE fb dimethenamid-P POST (4,850 kg ha−1) and pendimethalin + S-metolachlor PRE fb S-metolachlor POST (4,730 kg ha−1) provided yields similar to the fomesafen-containing treatment.
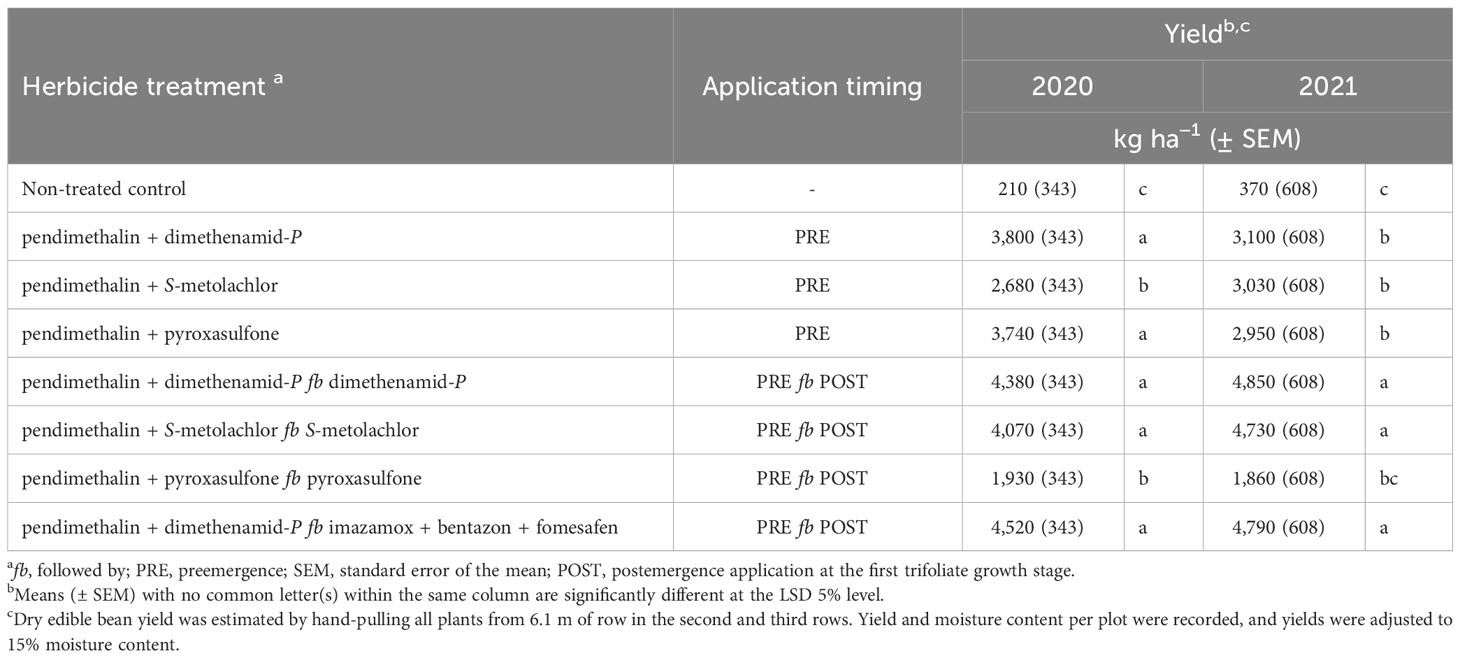
Table 5 Impact of herbicide treatments on dry edible bean yield (kg ha−1) in field experiments conducted at the University of Nebraska Panhandle Research and Extension Center, Scottsbluff, NEa.
4 Discussion
Previous studies have shown the efficacy of PRE-alone applications of VLCFA-inhibiting herbicides on pigweed species control, such as pyroxasulfone providing 88–96% control of waterhemp (Amaranthus tuberculatus (Moq.) J. D. Sauer) in sorghum (Goodrich et al., 2018). However, in our study, pendimethalin + pyroxasulfone applied PRE-alone provided lower control of Palmer amaranth (79–87%), likely due to stand loss and stunted crop growth, which allowed Palmer amaranth to emerge later in the season (Figure 2). Miranda et al. (2022b) reported inconsistent control of Palmer amaranth, ranging from 0% to 86%, when pendimethalin + dimethenamid-P were applied PRE-alone in dry edible beans. Our findings, along with those of Miranda et al. (2022b), suggest that PRE-alone programs in dry edible beans for controlling ALS-inhibitor-resistant Palmer amaranth are insufficient to manage late-emerging flushes. Sequential PRE fb POST programs of VLCFA-inhibiting herbicides have shown promise in controlling pigweed species, with acetochlor, dimethenamid-P, and S-metolachlor providing effective control of waterhemp and multiple herbicide-resistant Palmer amaranth when applied sequentially PRE fb POST in corn (Steckel et al., 2002; Chahal et al., 2018). These studies collectively emphasize the limitations of relying solely on PRE-alone herbicide programs for effective control of pigweed species, highlighting the need of sequential PRE fb POST applications of VLCFA-inhibiting herbicides for effective season-long control of problematic weed species such as waterhemp and herbicide-resistant Palmer amaranth in row cropping systems.
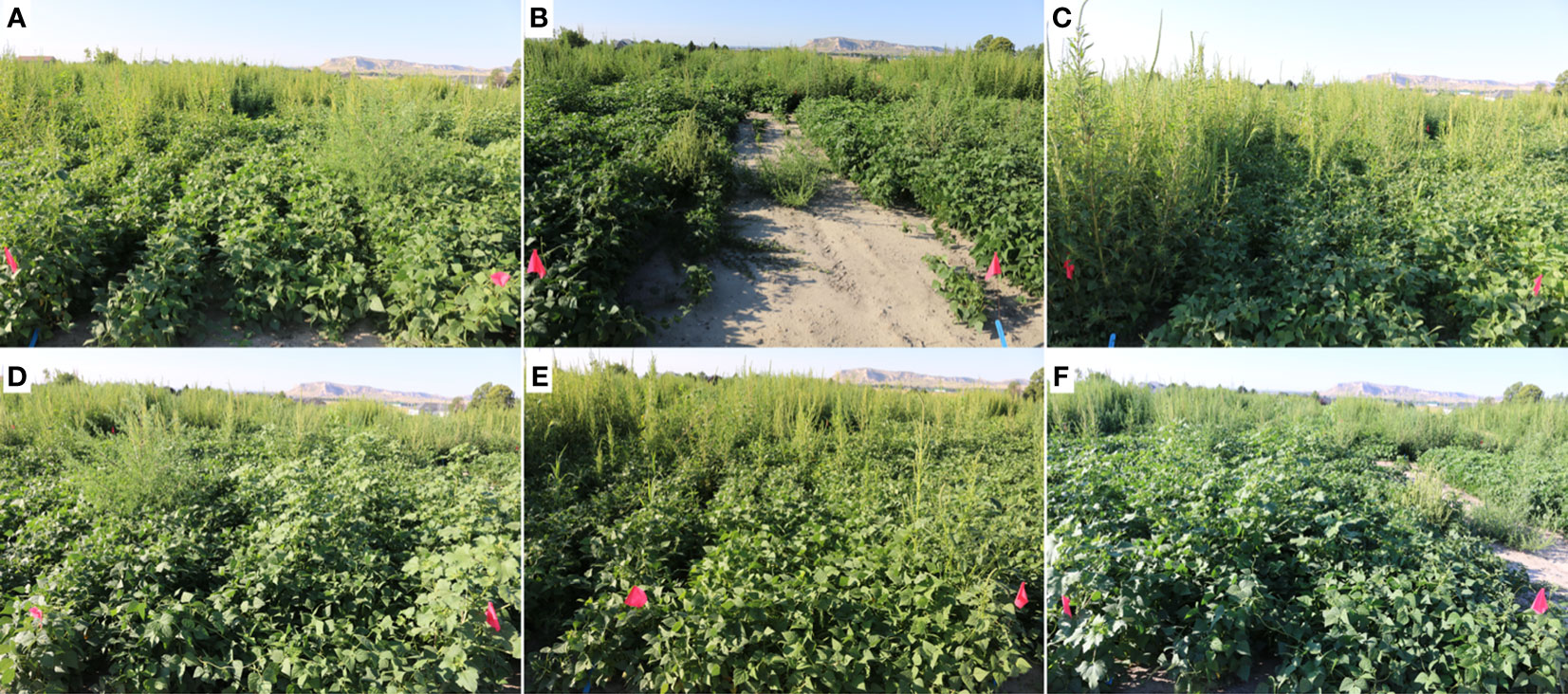
Figure 2 Photos from selected treatments illustrating the control of Palmer amaranth and injury to dry edible beans. The treatments include pyroxasulfone applied PRE-alone (A), pyroxasulfone PRE followed by pyroxasulfone POST (B), dimethenamid-P applied PRE-alone (C), dimethenamid-P PRE followed by dimethenamid-P POST (D), S-metolachlor applied PRE-alone (E), and S-metolachlor PRE followed by S-metolachlor POST (F). Note the stand loss and stunted growth observed in dry edible bean following the application of pyroxasulfone in both PRE and POST. These photos were taken during field experiments conducted in 2020 and 2021 at the University of Nebraska Panhandle Research and Extension Center in Scottsbluff, NE.
Reductions in Palmer amaranth biomass are crucial indicators of herbicide program effectiveness, as biomass strongly correlates with seed production (Webster and Grey, 2015; Spaunhorst et al., 2018; Mahoney et al., 2021; Miranda et al., 2022a). Even small late-emerging plants, which may have minimal impact on crop yield, can produce enough seeds to replenish the soil seedbank. Miranda et al. (2022a) demonstrated that for every gram of female Palmer amaranth dry biomass, seed production increased by 270 seeds when competing with dry edible bean. Therefore, either pendimethalin + dimethenamid-P PRE fb dimethenamid-P POST or pendimethalin + S-metolachlor PRE fb S-metolachlor POST, may represent the best alternatives in the absence of foliar-active POST herbicides, as they consistently provided the greatest reduction in Palmer amaranth biomass.
Regarding crop safety, dimethenamid-P and S-metolachlor have been shown to provide excellent crop safety, with injury levels below 3%, as reported by Procópio et al. (2001) and Soltani et al. (2014a). However, pyroxasulfone can cause high injury in dry edible bean, as observed in our study, consistent with the findings of Soltani et al. (2018b). This emphasizes the significance of balancing herbicide efficacy with crop safety. The observed reduction in dry edible bean yield associated with the application of pyroxasulfone, aligns with previous studies indicating high crop injury and maturity delay caused by this herbicide. Figure 2 further supports this conclusion regarding crop stand loss and maturity delay. The significant yield reduction observed in our study contrasts with the findings of Soltani et al. (2018b), who reported a 17% yield reduction associated with pyroxasulfone application in dry edible bean. In our study, the yield reduction was notably higher, around 40% in 2021 compared to the top-yielding treatment, underscoring the substantial impact of pyroxasulfone on dry edible bean yield. These variations underscore the complexity of weed management decisions and the necessity to assess the risks and benefits of different herbicide options. While certain herbicides may offer effective control of problematic weeds such as Palmer amaranth, they can also pose risks to crop health and yield. Additionally, failure to effectively control Palmer amaranth can lead to substantial yield reductions in dry edible bean crops (Miranda et al., 2022a), jeopardizing farm profitability and food security. Providing growers with effective weed management tools that do not compromise crop health and yield is crucial for sustainable agriculture practices. This highlights the need for further research to explore alternative weed management options that offer effective control while minimizing adverse effects on crop health and productivity. Finding a balance between weed control efficacy and crop safety is essential for sustainable agriculture practices, as it ensures both effective weed management and optimal crop yield, thereby safeguarding farm profitability and contributing to food security.
4.1 Conclusion and practical implications
Effective management of herbicide-resistant Palmer amaranth requires an integrated approach aimed at reducing the soil seedbank and implementing diverse control strategies (Harker and O’Donovan, 2013; Jhala et al., 2014; Mahoney et al., 2021). Crop rotation plays a crucial role in long-term management, with tall-stature crops like corn offering competitive advantages against Palmer amaranth (Mahoney et al., 2021). Additionally, the greater diversity of herbicide options available in corn compared to dry edible bean allows for the use of multiple modes of action, enhancing weed control efficacy. Given the rotational restrictions imposed by fomesafen, alternatives such as pendimethalin + dimethenamid-P PRE fb dimethenamid-P POST and pendimethalin + S-metolachlor PRE fb S-metolachlor POST emerge as promising options for integrated Palmer amaranth control. These VLCFA-inhibitor-containing programs offer flexibility in crop rotation while delivering comparable control levels to fomesafen-containing treatments. However, it is essential to exercise caution and implement mixtures with foliar-active herbicides to mitigate the risk of herbicide resistance and maintain the effectiveness of these herbicide programs. By adopting such integrated approaches and expanding the spectrum of controlled weed species, growers can effectively manage herbicide-resistant Palmer amaranth while preserving crop health and productivity in dry edible bean production systems.
Data availability statement
The raw data supporting the conclusions of this article will be made available by the authors, without undue reservation.
Author contributions
JM: Data curation, Formal Analysis, Investigation, Methodology, Software, Visualization, Writing – original draft, Writing – review & editing. AJ: Writing – review & editing. JB: Writing – review & editing. NL: Conceptualization, Funding acquisition, Project administration, Resources, Supervision, Writing – review & editing.
Funding
The author(s) declare that financial support was received for the research, authorship, and/or publication of this article. This study received financial support from the Nebraska Dry Bean Commission. The funders were not involved in the study design, data collection, analysis, interpretation, writing of the article, or decision to submit it for publication.
Acknowledgments
The authors gratefully acknowledge the Nebraska Dry Bean Commission for providing funding to conduct this study, and the PHREC Weed Science research group for their assistance in establishing the study.
Conflict of interest
The authors declare that the research was conducted in the absence of any commercial or financial relationships that could be construed as a potential conflict of interest.
Publisher’s note
All claims expressed in this article are solely those of the authors and do not necessarily represent those of their affiliated organizations, or those of the publisher, the editors and the reviewers. Any product that may be evaluated in this article, or claim that may be made by its manufacturer, is not guaranteed or endorsed by the publisher.
Nomenclature
Bentazon; dimethenamid-P; fomesafen; imazamox; S-metolachlor; Palmer amaranth, Amaranthus palmeri S. Watson; dry edible bean, Phaseolus vulgaris L.
References
Adjesiwor A. T., Claypool D. A., Kniss A. R. (2020). Dry bean response to preemergence flumioxazin. Weed Technol. 34, 197–201. doi: 10.1017/wet.2019.87
Anonymous (2020). Dual II Magnum® herbicide product label (Research Triangle, NC: Syngenta Crop Protection).
Anonymous (2021). Section 24 (c) special local need label. Reflex® herbicide for control of weeds in dry beans (Research Triangle, NC: Syngenta Crop Protection).
Bates D., Mächler M., Bolker B., Walker S. (2015). Fitting linear mixed-effects models using lme4. J. Stat. Software 67, 1-48. doi: 10.18637/jss.v067.i01
Beiermann C. W., Creech C. F., Knezevic S. Z., Jhala A. J., Harveson R., Lawrence N. C. (2021a). Response of Palmer amaranth (Amaranthus palmeri S. Watson) and sugarbeet to desmedipham and phenmedipham. Weed Technol. 35, 440–448. doi: 10.1017/wet.2021.1
Beiermann C. W., Miranda J. W. A., Creech C. F., Knezevic S. Z., Jhala A. J., Harveson R., et al. (2021b). Critical timing of weed removal in dry bean as influenced by the use of preemergence herbicides. Weed Technol. 36, 168–176. doi: 10.1017/wet.2021.99
Brooks M. E., Kristensen K., van Benthem K. J., Magnusson A., Berg C. W., Nielsen A., et al. (2017). glmmTMB balances speed and flexibility among packages for zero-inflated generalized linear mixed modeling. R J. 9, 378–400. doi: 10.32614/RJ-2017-066
Burke I., Schroeder M., Thomas W. E., Wilcut J. W. (2007). Palmer amaranth interference and seed production in peanut. Weed Technol. 21, 367–371. doi: 10.1614/WT-06-058.1
Chahal P. S., Ganie Z. A., Jhala A. J. (2018). Overlapping residual herbicides for control of photosystem (PS) II- and 4-Hydroxyphenylpyruvate Dioxygenase (HPPD)-inhibitor-resistant Palmer amaranth (Amaranthus palmeri S. Watson) in glyphosate-resistant maize. Front. Plant Sci. 8. doi: 10.3389/fpls.2017.02231
Chahal P. S., Varanasi V. K., Jugulam M. (2017). Glyphosate-resistant Palmer amaranth (Amaranthus palmeri) in Nebraska: confirmation, EPSPS gene amplification, and response to POST corn and soybean herbicides. Weed Technol. 31, 80–93. doi: 10.1614/WT-D-16-00109.1
Chandi A., Jordan D. L., York A. C., Milla-Lewis S. R., Burton J. D., Culpepper A. S., et al. (2012). Interference of selected Palmer amaranth (Amaranthus palmeri) biotypes in soybean (Glycine max). Int. J. Agron. 2012, 168267. doi: 10.1155/2012/168267
Douma J. C., Weedon J. T. (2019). Analysing continuous proportions in ecology and evolution: A practical introduction to beta and Dirichlet regression. Methods Ecol. Evol. 10, 1412–1430. doi: 10.1111/2041-210X.13234
Fox J., Weisberg S. (2019). An R Companion to Applied Regression. 3rd edn (Thousand Oaks, CA: Sage). Available at: https://socialsciences.mcmaster.ca/jfox/Books/Companion/.
Goodrich L. V., ButtS-Wilmsmeyer C. J., Bollero G. A., Riechers D. E. (2018). Sequential pyroxasulfone applications with fluxofenim reduce sorghum injury and increase weed control. Agron. J. 110, 1915–1924. doi: 10.2134/agronj2018.01.0014
Harker K. N., O’Donovan J. T. (2013). Recent weed control, weed management, and integrated weed management. Weed Technol. 27, 1–11. doi: 10.1614/WT-D-12-00109.1
Heap I. (2024) The International Herbicide-Resistant Weed Database. Available online at: http://www.weedscience.org/.
Hothorn T., Bretz F., Westfall P. (2008). Simultaneous inference in general parametric models. Biom J. 50, 346–363. doi: 10.1002/bimj.200810425
Jhala A. J., Knezevic S. Z., Ganie Z. A., Singh M. (2014). “Integrated weed management in maize,” in Recent Advances in Weed Management. Eds. Chauhan B. S., Mahajan G. (Springer New York, New York, NY), 177–196. doi: 10.1007/978-1-4614-7744-9_8
Jhala A. J., Malik M. S., Willis J. B. (2015). Weed control and crop tolerance of micro-encapsulated acetochlor applied sequentially in glyphosate-resistant soybean. Can. J. Plant Sci. 95, 973–981. doi: 10.4141/cjps-2014-422
Jhala A. J., Singh M., Shergill L. (2024). Very long chain fatty acid–inhibiting herbicides: Current uses, site of action, herbicide-resistant weeds, and future. Weed Technol. 38, 1–16. doi: 10.1017/wet.2023.90
Klingaman T. E., Oliver L. R. (1994). Palmer amaranth (Amaranthus palmeri) interference in soybeans (Glycine max). Weed Sci. 42, 523–527. doi: 10.1017/S0043174500076888
Knezevic S. Z., Klein R., Ogg C., Creech C., Kruger G. R., Lawrence N., et al. (2020). Guide for weed, disease, and insect management in Nebraska (EC130) (Lincoln, NE: University of Nebraska-Lincoln), 99–101.
Kniss A. R., Streibig J. C. (2018) Statistical analysis of agricultural experiments using R. Available online at: http://Rstats4ag.org.
Korres N. E., Norsworthy J. K., Mauromoustakos A. (2019). Effects of Palmer amaranth (Amaranthus palmeri) establishment time and distance from the crop row on biological and phenological characteristics of the weed: implications on soybean yield. Weed Sci. 67, 126–135. doi: 10.1017/wsc.2018.84
Lenth R. (2020) emmeans: Estimated marginal means, aka least-squares means. R package version 1.5.1. Available online at: https://CRAN.R-Project.org/package=emmeans.
Li Z., Van Acker R., Robinson D., Soltani N., Sikkema P. (2017). Managing weeds with herbicides in white bean in Canada: a review. Can. J. Plant Sci. 97, 755–766. doi: 10.1139/CJPS-2017-0030
Lucier G., Davis W. (2020). Economic Research Service. Situation and outlook report. Vegetables and pulses outlook VGS-364 (Washington D.C.: USDA, Economic Research Service).
Mahoney D. J., Jordan D. L., Hare A. T., Leon R. G., Roma-Burgos N., Vann M. C., et al. (2021). Palmer amaranth (Amaranthus palmeri) growth and seed production when in competition with peanut and other crops in North Carolina. Agronomy 11, 1734. doi: 10.3390/agronomy11091734
Massinga R. A., Currie R. S., Horak M. J., Boyer J. (2001). Interference of Palmer amaranth in corn. Weed Sci. 49, 202–208. doi: 10.1614/0043-1745(2001)049[0202:IOPAIC]2.0.CO;2
McCloskey W. B. (2001). Using layby herbicides for weed control in cotton (Tucson, Arizona: University of Arizona, College of Agriculture and Life Sciences, Cooperative Extension). Available at: http://cals.arizona.edu/crops/cotton/weeds/laybyherbicides.html.
Miranda J. W. A., Bradshaw J., Jhala A. J., Lawrence N. C. (2022b). Control of acetolactate synthase-inhibiting herbicide-resistant Palmer amaranth (Amaranthus palmeri) with sequential applications of dimethenamid–P in dry edible bean. Weed Technol. 36, 325–333. doi: 10.1017/wet.2022.23
Miranda J. W. A., Jhala A. J., Bradshaw J., Lawrence N. C. (2022a). Palmer amaranth (Amaranthus palmeri) interference and seed production in dry edible bean. Weed Technol. 35, 995–1006. doi: 10.1017/wet.2022.39
Mueller T. C., Steckel L. E. (2011). Efficacy and dissipation of pyroxasulfone and three chloroacetamides in a Tennessee field soil. Weed Sci. 59, 574–579. doi: 10.1614/WS-D-11-00003.1
NCSS (2006). Tripp Series (National Cooperative Soil Survey). Available at: https://soilseries.sc.egov.usda.gov/OSD_Docs/T/TRIPP.html.
Onofri A., Carbonell E. A., Piepho H.-P., Mortimer A. M., Cousens R. D. (2010). Current statistical issues in Weed Research. Weed Res. 50, 5–24. doi: 10.1111/j.1365-3180.2009.00758.x
Poling K. W., Renner K. A., Penner D. (2009). Dry edible bean class and cultivar response to dimethenamid and metolachlor. Weed Technol. 23, 73–80. doi: 10.1614/WT-07-092.1
Procópio S. O., Silva A., Ferreira L. R., Miranda G., Santos J. (2001). Dry bean to S–metolachlor tolerance under different spray conditions. Planta Daninha 19, 263–271. doi: 10.1590/S0100-83582001000200008
R Core Team (2020). R: A Language and Environment for Statistical Computing (Vienna, Austria: R Foundation for Statistical Computing).
Sarangi D., Jhala A. J. (2018). Comparison of a premix of atrazine, bicyclopyrone, mesotrione, and S–metolachlor with other preemergence herbicides for weed control and corn yield in no-tillage and reduced-tillage production systems in Nebraska, USA. Soil Tillage Res. 178, 82–91. doi: 10.1016/j.still.2017.12.018
Soltani N., Dille J. A., Burke I. C., Everman W. J., VanGessel M. J., Davis V. M., et al. (2016). Potential corn yield losses from weeds in North America. Weed Technol. 30, 979–984. doi: 10.1614/WT-D-16-00046.1
Soltani N., Dille J. A., Burke I. C., Everman W. J., VanGessel M. J., Davis V. M., et al. (2017). Perspectives on potential soybean yield losses from weeds in North America. Weed Technol. 31, 148–154. doi: 10.1017/wet.2016.2
Soltani N., Dille J. A., Gulden R. H., Sprague C. L., Zollinger R. K., Morishita D. W., et al. (2018a). Potential yield loss in dry bean crops due to weeds in the United States and Canada. Weed Technol. 32, 342–346. doi: 10.1017/wet.2017.116
Soltani N., Nurse R. E., Sikkema P. H. (2014a). Weed management in kidney bean with tank mixes of S–metolachlor, imazethapyr and linuron. Agric. Sci. 5, 611–617. doi: 10.4236/as.2014.57064
Soltani N., Robinson D. E., Shropshire C., Sikkema P. H. (2006). Otebo bean (Phaseolus vulgaris) sensitivity to pre-emergence herbicides. Crop Prot. 25, 476–479. doi: 10.1016/j.cropro.2005.08.002
Soltani N., Shropshire C., Sikkema P. H. (2014b). Sensitivity of dry bean to dimethenamid–P, saflufenacil and dimethenamid–P/saflufenacil. Am. J. Plant Sci. 5, 3288–3294. doi: 10.4236/ajps.2014.521343
Soltani N., Shropshire C., Sikkema P. H. (2018b). Dry bean sensitivity to VLCFA herbicides applied preemergence. Am. J. Plant Sci. 9, 1414–1423. doi: 10.4236/ajps.2018.97103
Spaunhorst D. J., Devkota P., Johnson W. G., Smeda R. J., Meyer C. J., Norsworthy J. K. (2018). Phenology of five Palmer amaranth (Amaranthus palmeri) populations grown in northern Indiana and Arkansas. Weed Sci. 66, 457–469. doi: 10.1017/wsc.2018.12
Statista (2021a) Production of dry edible beans in the United States from 2000 to 2020 (in 1,000 cwt). Available online at: https://www.statista.com/statistics/194283/us-bean-production-since-2000/.
Statista (2021b) Production value of dry edible beans in the United States from 2000 to 2020 (in 1,000 United States dollars). Available online at: https://www.statista.com/statistics/194284/us-bean-production-value-since-2000/.
Steckel L. E., Sprague C. L., Hager A. G. (2002). Common Waterhemp (Amaranthus rudis) control in corn (Zea mays) with single preemergence and sequential applications of residual herbicides. Weed Technol. 16, 755–761. doi: 10.1614/0890-037X(2002)016[0755:CWARCI]2.0.CO;2
Stephenson D. O., Blouin D. C., Griffin J. L., Landry R. L., Woolam B. C., Hardwick J. M. (2017). Effect of pyroxasulfone application timing and rate on soybean. Weed Technol. 31, 202–206. doi: 10.1017/wet.2016.25
USDA-NRCS (2019) Soil Survey Staff, Natural Resources Conservation Service, United States Department of Agriculture. Web Soil Survey. Available online at: http://websoilsurvey.sc.egov.usda.gov/.
Ward S., Webster T., Steckel L. (2013). Palmer amaranth (Amaranthus palmeri): A review. Weed Technol. 27, 12–27. doi: 10.1614/WT-D-12-00113.1
Webster T. M., Grey T. L. (2015). Glyphosate-resistant Palmer amaranth (Amaranthus palmeri) morphology, growth, and seed production in Georgia. Weed Sci. 63, 264–272. doi: 10.1614/WS-D-14-00051.1
Wilson R. G. (2005). Response of dry bean and weeds to fomesafen and fomesafen tank mixtures. Weed Technol. 19, 201–206. doi: 10.1614/WT-04-166R
Wilson R. G., Sbatella G. M. (2014). Integrating irrigation, tillage, and herbicides for weed control in dry bean. Weed Technol. 28, 479–485. doi: 10.1614/WT-D-13-00173.1
Keywords: chloroacetamide, crop injury, Group 15 herbicides, layby application, pinto bean, residual herbicides
Citation: Miranda JWA, Jhala AJ, Bradshaw J and Lawrence NC (2024) Crop safety and control of acetolactate synthase inhibitor-resistant Palmer amaranth (Amaranthus palmeri) with very long-chain fatty acid-inhibiting herbicides in dry edible bean. Front. Agron. 6:1401865. doi: 10.3389/fagro.2024.1401865
Received: 16 March 2024; Accepted: 24 April 2024;
Published: 15 May 2024.
Edited by:
Lynn M. Sosnoskie, Cornell University, United StatesReviewed by:
Stephen Christopher Marble, University of Florida, United StatesAgnieszka Synowiec, University of Agriculture in Krakow, Poland
Copyright © 2024 Miranda, Jhala, Bradshaw and Lawrence. This is an open-access article distributed under the terms of the Creative Commons Attribution License (CC BY). The use, distribution or reproduction in other forums is permitted, provided the original author(s) and the copyright owner(s) are credited and that the original publication in this journal is cited, in accordance with accepted academic practice. No use, distribution or reproduction is permitted which does not comply with these terms.
*Correspondence: Joshua W. A. Miranda, am9zaC5taXJhbmRhQG9yZWdvbnN0YXRlLmVkdQ==
†ORCID: Joshua W. A. Miranda, orcid.org/0000-0003-3591-5190
Amit J. Jhala, orcid.org/0000-0001-8599-4996
Nevin C. Lawrence, orcid.org/0000-0003-3836-323X