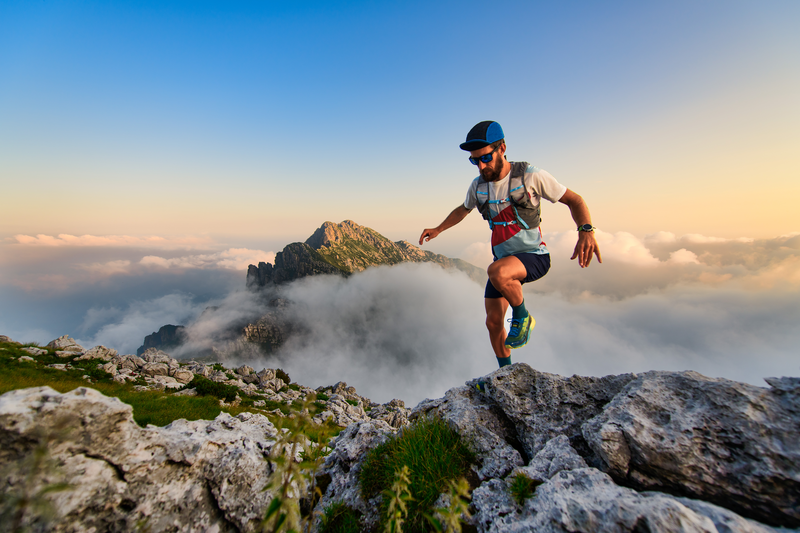
94% of researchers rate our articles as excellent or good
Learn more about the work of our research integrity team to safeguard the quality of each article we publish.
Find out more
BRIEF RESEARCH REPORT article
Front. Agron. , 09 April 2024
Sec. Climate-Smart Agronomy
Volume 6 - 2024 | https://doi.org/10.3389/fagro.2024.1394143
This article is part of the Research Topic Sustainable Nutrient Management under Climate Change View all 6 articles
Urea is widely used as nitrogen (N) source for rice fertilization in Costa Rica, despite its low efficiency linked to ammonia losses. To assess urea management alternatives, two field experiments were conducted in the Central Pacific region of Costa Rica to study the effect of N-(n-butyl) thiophosphoric triamide (NBPT) on rice yield and N use efficiency (NUE). In Experiment 1 (Exp1) three tillage treatments (commercial-CT-, reduced-RT-, and reduced tillage with previous subsoiler-RTS-) were evaluated with three N managements: control (without N), urea at 124 kg N ha-1 with and without NBPT. In Experiment 2 (Exp2), a 100 kg N ha-1 rate (with and without NPBT) was evaluated along with a control (without N). NUE was estimated using 15N urea isotopic labeling technique for both trials. In Exp1, a significant difference of 4.8% in NUE for grain was observed among urea with and without NBPT, but no tillage effect was observed. No statistically significant differences were observed in yield among the fertilization treatments (Exp1: 3.56 ± 0.98 t ha-1 for urea and 3.85 ± 0.85 t ha-1 for urea with NBPT; Exp2: 3.38 ± 0.39 t ha-1 for urea and 3.40 ± 0.58 t ha-1 for urea with NBPT) or due to different tillage practices (CT: 3.33 ± 0.79 t ha-1, RT: 3.56 ± 0.74 t ha-1, and RTS: 4.23 ± 0.98 t ha-1). Although the NBPT is a viable option to reduce ammonia losses, its adoption in tropical conditions might be restricted by the small impact on yield.
Tillage practices and nitrogen (N) management are essential factors for rice production. Urea is the most used fertilizer worldwide (Li et al., 2017), although its efficiency is usually low (Liao et al., 2015). Decreased N use efficiency (NUE) associated with the N loss via ammonia (NH3) volatilization, reduces crop productivity and its subsequent deposition generates an indirect greenhouse gas effect, eutrophication of water bodies and soil acidification (de Vries, 2021; Skorupka and Nosalewicz, 2021). In the tropics, NH3 volatilization is enhanced by high humidity and temperature and low carbon content in the soil (Martins et al., 2017).
Several techniques have been tested to improve NUE, including the use of urease inhibitors as N-(n-butyl) thiophosphoric triamide (NBPT), that seeks a slower release of ammonium (NH4+), to reduce the concentration of NH3 near the soil surface (Guardia et al., 2018; Klimczyk et al., 2021). Retaining N as urea for longer time reduces N loss as NH3 from the soil-plant system and could potentially provide the plant with a longer period for uptake, especially under high application rates (Koci and Nelson, 2016). Soil properties such as pH, the total exchange capacity, the type and structure of soil, and moisture content impact N-volatilization from urea and the efficacy of NBPT (Klimczyk et al., 2021; Soares and Cantarella, 2023).
Although, studies focused on the effect of the NBPT inhibitor according to soil type have established the potential of NBPT to reduce up 52% NH3 loss (Sanz-Cobena et al., 2014; Silva et al., 2017) its effect on yields and its cost-benefit ratio have been less predictable. The utilization of urease inhibitors, including NBPT, showed variable results in paddy rice. For instance, in two recent meta-analyses, Linquist et al. (2013) found rice yield increases ranging from 3.5% to 9%, and Cantarella et al. (2018) reported an average yield increment of 7.6%, but with values ranging from nearly -10% to over 50%. Moreover, the potential benefit of NBPT in rice depends on its degradation rate affected by soil temperature or pH, presence of coarse-textured soils, and whether the urea was applied to the soil well before the field was flooded (Silva et al., 2017; Cantarella et al., 2018).
A few field trials have studied the interactions between slow-release N fertilizer and tillage practices on NUE and rice yield (Jian-She et al., 2011; Cantarella et al., 2018; Liu et al., 2018). No-tillage or conservation tillage have been pointed out to promote NH3 volatilization compared with conventional intensive tillage (Rochette et al., 2009; Uddin et al., 2021; Santos et al., 2023). However, Liu et al. (2018) suggested a combination of no-tillage and slow-release N fertilizer plus inorganic N fertilizer as a strategy for mitigating NH3 emissions and improving grain yield and NUE in paddy fields of central China. This is because they did not find obvious effects on total NH3 volatilization or a significant effect on grain yield and NUE in a two growing seasons study period under intensive tillage and no-tillage.
In Costa Rica, rice cultivation spans 35,301 ha across five geographical regions for the 2021/2022 harvest. The Central Pacific region, comprising 18% of the planting area, ranks second in importance. While rainfed rice production covers 57% of the national planting area, this proportion remains at around 96% in the Central Pacific region between 2018 and 2022 (CONARROZ, 2023a). Planting in this region typically occurs at the onset of the rainy season (usually between April and May), with second plantings during the dry season being rare due to the absence of irrigation districts. The average annual yield in the Central Pacific was 3.85 t/ha from 2018 to 2022 (CONARROZ, 2023a). Rice cultivation in this region heavily relies on intensive urea application, predominantly under high temperatures and wet soil conditions, which may lead to N losses as NH3 (CONARROZ, 2023b). However, there is a lack of published research on the impact of NBPT on NUE and rice yield, as well as its influence under various tillage practices.
We carried out two field trials with the objective of assessing the potential of NBPT to improve NUE and rice yield under varying environmental conditions, including different rice varieties, N rates, and production seasons (rainy/dry) in Costa Rican rice production, according to its ability to mitigate ammonia (NH3) volatilization from urea fertilization. Secondly, to investigate the interaction effects of NBPT application and different tillage on NH3 volatilization, NUE, and rice yield to determine optimal management strategies for rice production in tropical conditions, and finally, to provide insights into the cost-benefit ratio of NBPT application in tropical rice production systems, considering factors such as soil properties, climate conditions, and potential yield improvements.
Two field trials were carried out in Parrita in the Central Pacific of Costa Rica, in two farms located 8.2 km from each other. The area is classified as Tropical Humid Forest (L. Holdridge life zone system), with an annual average temperature of 28°C (for period 2013-2021), which oscillates from 26°C to 29°C. The annual accumulated average rainfall ranges from 2000 to 3000 mm per year with a maximum in October (mean 613.6 mm) and a marked rainfall reduction during the dry season that peaks in February (mean 13.6 mm).
The first field trial (La Bandera) was located at CONARROZ’ Experimental Farm in La Bandera, Parrita (9°30’55.02” N, 84°22’2.56” W). The second experiment (Barbudal) was carried out in a producer farm located in Barbudal, Parrita (9°30’59.15” N, 84°17’27.20” W). The soils classified as alluvial Inceptisol, under ustic climatic regime, are clay loam in texture, characterized by low organic matter (1.6 and 1.7% in La Bandera and Barbudal respectively) and medium to high fertility. Other soil characteristics for both sites, prior to the onset of the experiment, are shown in Supplementary Table 1.
The experiment was conducted from December 4th, 2019, to March 25th, 2020 during the dry season (Supplementary Figure 1). Lazarroz variety was sown in a seed density of 110 kg ha-1 under a wet-dry irrigation system. Fifteen 12 m x 60 m plots were established under a split plot design of a randomized complete block, with five repetitions for each treatment combination. Tillage was assigned to the main plot and fertilizer to the subplot. The following tillage systems were applied: commercial tillage (CT), reduced tillage (RT) and reduced tillage with previous subsoiling (RTS). The CT consisted of two passes with coarse harrow discs in the top 20-30 cm of soil, followed by one pass with fine harrows at a depth of 10-20 cm. In contrast, the Reduced Tillage (RT) only included a single pass with fine harrows at the 10-20 cm depth. Furthermore, the Reduced Tillage with Subsoiling (RTS) method included a subsoiler pass at a depth of 30-40 cm, conducted eight months before the RT application.
Three fertilization treatments (plots 12 m x 20 m) were randomly applied in main plots (Supplementary Table 2), namely: control (CK1, without N fertilization), urea (U1, 124 kg N ha-1 as urea) and urea with inhibitor (UI1, 124 kg N ha-1 as urea coated with NBPT commercial brand Nitro Xtend).
In the entire experimental area following subsoiling in May 2019, weed seed bank depletion was carried out by mowing weeds with a brush cutter, supplemented by the application of Paraquat. This weed seed bank depletion process was repeated after plant reemergence. Finally, due to weed emergence, primarily broadleaf, a mixture of 2,4-D and metsulfuron was applied.
Before sowing the crop, an exhaustion of weeds was conducted using the post-emergent contact herbicide Gramoxone (active ingredient Paraquat) along with the non-selective systemic herbicide Roundup (active ingredient Glyphosate-isopropylamine). Following sowing, the selective rice herbicide Sirius (active ingredient Pyrazosulfuron ethyl) was applied to control of Cyperaceae and broadleaf weeds. Based on monitoring, one reseal was applied with the post-emergent systemic sealant herbicide Sempra (active ingredient Halosulfuron Methyl).
Soil penetration resistance was measured using a Penetrometer (Eijkelkamp, model 06.01.SA) at a depth of 5, 10, 15, 20, 25, 30, 35 and 40 cm in 28 sampling points per plot (120 per soil preparation treatment).
To assess soil available water (SAW) for plants, undisturbed samples were taken in cylinders of 20 cm3, at 10 cm and 30 cm soil depth. With 5 replicates per soil preparation treatment, SAW was estimated as the difference of water content at field capacity (between 60-330 hPa) and at permanent wilting point (15 000 hPa) in the water retention curve.
The experiment was conducted from May 6th to August 19th, 2021 during the rainy season (Supplementary Figure 1). The Inta-Puita variety was sown in a seed density of 120 kg ha-1 under a rainfed rice system. Eighteen 4.5 m x 4.5 m plots prepared with minimal tillage (only one coarse harrow pass) were delimited in six complete random blocks. Three fertilization treatments were randomly distributed in the experimental area (Supplementary Table 2), namely: control (CK2, without N fertilization), urea (U2, 100 kg N ha-1 as urea), and urea with inhibitor (UI2, 100 kg N ha-1 as urea coated with NBPT commercial brand Nitro Xtend).
Due to the low weed pressure in this experimental area, only the non-selective systemic herbicide Roundup (active ingredient Glyphosate-isopropylamine) was applied. Subsequently, a single pass of heavy harrowing was performed before planting. The high seed density used and the precipitation conditions limited weed growth, eliminating the need for the application of a seal or post-seal herbicide during crop development. Few weeds that were not controlled chemically were removed through manual weeding.
In La Bandera, 15 subplots of 1 m2 were harvested per treatment (U1 and UI1) (small size due to the restrictions imposed by the COVID-19 pandemic), while in Barbudal, six subplots of 8 m2 per treatment (U2 and UI2) were harvested for yield estimation.
In both experiments, the weight of each subplot’s fresh grain was determined immediately after cutting. Samples were homogenized and air-dried to determine dry biomass yield. To determine the moisture content of rice grain, two composite samples of 2.5 kg of each treatment were collected and analyzed by the Laboratory of Quality Control in Rice in CONARROZ, following the INTE-ISO/IEC 17025:2005 and Technical Regulation of Rice “RTCR 406-2007”. Finally, the dry grain was normalized to 13% moisture (percentage of moisture in Costa Rican commercial grain) to calculate the yield.
The estimation of NUE was conducted using the 15N urea isotopic labeling technique (Tubert-Brohman et al., 2013; Meng et al., 2020). Microplots of 1 m2 were delimited inside the U and UI plots. Microplots were fertilized with urea and urea impregnated with NBPT, both enriched with 15N at 1.9384 atoms in excess. During harvest, a sample of four plants was cut from the center of each microplot, separating the biomass from the grain. Subsequently, the grain and the foliage were dried in room air for 48 h and dried in an oven at 70 °C for 72 h. These samples were ground in a blade mill (Retsch GM 200). A subsample was taken to determinate the total N content by the dry combustion method using a MACRO cube CN vario analyzer (Elementar Analyze system GmbH, Germany) (Horneck and Miller, 2019). The rest of each subsample was ground to powder in a pellet mill (Retsch MM 400), then packed in tin capsules and sent to the Stable Isotope Laboratory, at the University of California, Davis to determine the 15N composition. To determine the dry biomass in each treatment a subsample of 200 g of foliage were dried at 70 °C by 72 hours.
The amount of N from the fertilizer in the plant (Ndfp), in grams (g), was evaluated according to the Equation 1:
where, NAC is the accumulated N or N uptake (g) calculated using NAC = (MD*CN)/100 where MD is the dry matter (g), and CN is its content of total N (%), and Ndff the N derived from the fertilizer (%) calculated by Equation 2:
where, AN is the 15N atoms in excess of 0.3663 in the plant or in the fertilizer.
Finally, NUE (%) was calculated by Equation 3:
The effect of NBPT on NH3 volatilization was corroborate in two different phases. In La Bandera, the aim was to capture the greatest risk of NH3 volatilization due to the combination of a higher application rate with less development of the plant (16 days after sown- DAS). In Barbudal monitoring in the active tillering stage was chosen to see the effect of NBPT under conditions of lower NH3 volatilization risk due to the peak of crop development (31 DAS). Throughout the NH3 monitoring periods, average temperature and wind speed were 26.9 ± 3.2 °C, (26.0-29.0 °C), and 1.68 ± 1.1 m s-1 (0.09-5.16 m s-1) for dry season respectively, and for rainy season were 26.1 ± 2.7 °C (25.0-26.7 °C), and 1.86 ± 0.5 m s-1 (0.14-5.20 m s-1) respectively.
Measurements of NH3 volatilization potential were determined by semi-static open chambers made of transparent polyethylene terephthalate bottles (Araújo et al., 2009; Jantalia et al., 2012; Martins et al., 2017). One NH3 chamber was installed in each plot on the soil surface. Inside each chamber, a foam strip (2.5 cm × 25 cm, 3 mm thick) was presoaked in a solution of 1 mol L−1 H2SO4 with 5% (v/v) glycerol and kept moist during sampling periods with 25 mL of the acid solution contained in a plastic jar suspended inside the chamber. When foam strips were removed, they were transported inside the plastic jar to the laboratory in a cooler (4-6 °C) box. The trap volume was adjusted to 50 mL with deionized water and extracted by shaking for 30 min at 220 revolutions per minute on an orbital shaker (Martins et al., 2021). A 1.2 mL aliquot of this solution was diluted to 25 mL, and NH4+ was analyzed as indophenol in an alkaline medium by spectrophotometric analysis (Bolleter et al., 1961).
In La Bandera, traps were installed just after the second N fertilization (15 DAS) and replaced by new traps on days 1, 3, 5, 8 and 11 after N application. The last trap was removed on day 14. In Barbudal, the traps were installed just after the third N fertilization (30 DAS) and replaced by new traps on days 2, 5 and 8 after fertilization. The last trap was removed on day 11. In both trials, one NH3 chamber was installed per plot of the CK, U, and UI treatments; however due to an issue with N fertilization in the block 1 of the split plot design of a randomized complete block, NH3 volatilization was followed only in four repetitions in La Bandera. The purpose of installing and replacing the traps was to prevent saturation, particularly during the initial sampling days when emissions are higher. This precautionary measure aimed to maintain optimal trapping efficiency and prevent a decline in the percentage of NH3 captured throughout the sampling period.
For comparing volatilization between treatments, partial cumulative NH3 volatilization potential (EcNH3, g ha−1) was calculated by Equation 4 following the procedure stated by Jantalia et al. (2012).
where mNi (mg N) is the i value of N captured from one plot by each period of sampling, CF is the conversion factor for converting N present in trap into volatilized N equal to 1.74 (Jantalia et al., 2012), A is the area covered by the chamber (m2), and 10-2 is a factor to express in kg ha-1 the result.
Normality and homogeneity of variances were assessed with Shapiro-Wilk and the Levene test, respectively. The outliers were identified and corrected with the Cook´s distance method. Data analysis in La Bandera was assessed using a two-way ANOVA, and the means comparison between treatments was evaluated by Least Significant Difference (LSD Fisher) by the split plot design. In Barbudal data was evaluated by one-way ANOVA, and the means comparison treatments were performed by Tukey´s Honest significance difference. Statistical significance differences between treatments were considered at p values less than 0.05. The software Rstudio 4.2.3 was used for statistical data analysis.
The rice yield in La Bandera and Barbudal trials did not present statistical differences at p< 0.05 between fertilization treatments (Figure 1). In La Bandera, tillage treatments factor CT (3.33 ± 0.79 t ha-1), RT (3.56 ± 0.74 t ha-1) and RTS (4.23 ± 0.98 t ha-1) or the combined effect of tillage and N-fertilization treatments did not show differences in yield (Supplementary Figure 2A).
Figure 1 Rice yield at 13% humidity and nitrogen use efficiency (NUE) for two field trials in Parrita, Costa Rica: (A) La Bandera: Var. Lazarroz with 120 kg N ha-1 applied as urea (U1) and urea with NBPT (UI 1) (n=15) (B) Barbudal: Var. Inta-Puita produced with 100 kg N ha-1 applied as urea (U2) and urea with NBPT (UI 2), (n=6). Error bars indicate standard error. In both experiments, the rice yield was not significantly different according to LSD Fisher test (p > 0.05). The grain bars in panel (A) identified with different letters are significantly different according to LSD Fisher test at p< 0.05 (Giraldo-Sanclemente, 2024).
Soil compaction was evident in the CT and RT tillage treatments in La Bandera, showing values near to 1 MPa in the top 10-15 cm, and values above 1.66 to 1.72 MPa at a depth of 20-30 cm (Figure 2A) where the deep roots of rice are developed.
Figure 2 (A) Soil penetration resistance (MPa) of plots with conventional tillage (CT), reduced tillage (RT) and reduced tillage with previous subsoil (RTS) (n = 120). (B) Average available soil water (%) at 10 cm and 30 cm of depth in same tillage treatments: CT, RT and RTS. The error bars indicate standard error (n=5). La Bandera, Parrita, Costa Rica.
Soil available water in the top 10 cm in La Bandera ranged from 7% to 22% and at 30 cm from 6% to 27%, with a high variability among repetitions of the same treatment what precluded the detection of significant differences among tillage treatments. However, there was a clear tendency towards higher values in the RT treatment particularly at 30 cm depth (Figure 2B).
In the split plot field trial in La Bandera, the treatment UI1 increase significantly the NUE grain percentage in 4.8% at p = 0.029 in comparison with U1, but not for foliage (Figure 1A). Regarding the ANOVA analysis, no significant differences were observed with different tillage management in NUE (p = 0.64) (Supplementary Figure 2B) or in the interaction between tillage and fertilization treatments (p = 0.068).
In Barbudal, grain and foliage did not present NUE percentage statistical differences between UI2 and U2 (Figure 1B).
In contrast to the controls, the NH3 potential volatilization increased after the urea application with or without NBPT, where volumetric soil water content higher than 25% favored urea hydrolysis. The NH3 potential volatilization remained consistently higher in the urea treatments (Figure 3A), and maintained similar emission intervals, with the rates of NH3 volatilization reaching the baseline level by day 12 after N-fertilization.
Figure 3 Potential NH3 volatilization rate (mg NH3-N m-2 h-1) for control (CK), urea (U) and urea with NBPT (UI) in two rice crops in Parrita, Costa Rica. In La Bandera: Wet/dry irrigated rice crop with Lazarroz variety and 42 kg N ha-1 applied 15 d after sowing (n=12). In Barbudal: Rainfed rice crop with Inta-Puita variety and 30 kg N ha-1 applied 30 d after sowing (n=6). (A) NH3 potential volatilization for control (CK), urea (U) and urea with NBPT (UI). (B) NH3 potential volatilization showing the interaction of N-fertilizer [urea (U1) and urea plus NBPT (UI1)] and tillage treatments [commercial tillage (CT), reduced tillage (RT) and reduced tillage with previous subsoiler (RTS)]. The error bars indicate standard error. Different letters indicate significant differences between means (p< 0.05) according to Tukey’s test (Giraldo-Sanclemente, 2024).
The highest NH3 volatilization rates occurred within 3-5 days and 0–2 days after N fertilization in La Bandera and Barbudal, respectively (Figure 3A). In La Bandera, the use of NBPT delayed the loss of NH3. The maximum NH3 volatilization rate was reached in the case of U1 between days 3 and 5, while UI1 showed the highest emission on day 8, and the loss of 50% of the total NH3 was reached after up to 4.4 and 6.0 days, respectively (See Supplementary Figure 3). On the other hand, although U2 and UI2 presented differences in the maximum potential volatilization rate of around 40%, both reached their peak on the second day after N application, which may have been observed in this way due to the lower sampling frequency in Barbudal.
The partial cumulative NH3 emissions showed differences between U and UI treatments in both experiments (Table 1). Although, there were no significant statistical differences between the tillage treatments, and there was no interaction between fertilization and tillage treatments in La Bandera, most of the time the RT-NH3 emissions were higher than in RTS and CT treatments (Figure 3B). Which can be related to the higher available soil water in the RT treatment (Figure 2B).
Table 1 Partial cumulative volatilization of NH3-N (mean ± standard deviation) after broadcast fertilization for one split only, in La Bandera and Barbudal, Parrita, Costa Rica.
The rice yield obtained in La Bandera and Barbudal (Figure 1) was similar to the average yield reported for the region Parrita (2019: 4.13 t ha-1 and 2021: 3.77 t ha-1) (CONARROZ, 2023a).
This research did not show any yield benefits when using urea coated with NBPT instead of urea alone, which is consistent with results reported by Rector et al. (2018) in flooded rice. However, the no effect of NBPT on yield contrasts with the average yield gain of 5.3% and 5.7% reported for soils with different pH and organic carbon content regardless of the N rate applied (Linquist et al., 2013; Silva et al., 2017). The effectiveness of NBPT in tropical soils may be reduced by factors such as pH, temperature, texture, and cation exchange capacity (Soares and Cantarella, 2023). Firstly, the pH levels of the soils in La Bandera and Barbudal (Supplementary Table 1) could explain the ineffectiveness of NBPT in improving crop yield; as these more acid pH levels do not favor the acid-base balance shift towards NH3- formation compared to soils with a pH ≥ 8, that are more represented in meta-analysis studies on the effectiveness of urease inhibitors like NBPT (Linquist et al., 2013; Matczuk and Siczek, 2021). Furthermore, pH modulates the stability and persistence of NBPT (less than a week at pH 5.5-6.4), with the degradation of NBPT being two to four times greater in acidic soils than in neutral or alkaline soils (Lasisi and Akinremi, 2022; Soares and Cantarella, 2023).
Secondly, the lower stability of NBPT at the high temperatures of tropical conditions, where degradation has been reported to begin just two or four days after application contrasts with the stability of the inhibitor in temperate climates (Soares and Cantarella, 2023). Finally, the clayloam texture and a high effective cation exchange capacity (Supplementary Table 1) might be key factors to fix NH4+ to the exchangeable cation specific sites in the clay and reduce faster volatilization of NH3 in both experimental field trials (Abalos et al., 2014; Silva et al., 2017; Götze et al., 2023).
In La Bandera, the tillage treatments did not result in significant differences in yield. This discrepancy to previous reports in literature of increased yield (Wang et al., 2020b), associated to improvement in soil physical properties (structure, aeration, water retention) with subsoiling (Gürsoy, 2021), might be due to the fact that there was no water stress, an aspect that has been mentioned as a key factor for yield increment with subsoiling (Sun et al., 2018).
The limited increase in NUE in La Bandera’s grain, achieved by using urea with NBPT compared to urea alone, was insufficient to impact crop yield, and contrasts with the reported 12% NUE increments (Linquist et al., 2013). As previously mentioned, the lower effectiveness of NBPT could be attributed to the high temperature and acid nature of the soils in the experimental areas as the most significant benefits of the inhibitor in rice have been observed in temperate soils with a pH ≥ 7.5 (Abalos et al., 2014; Meng et al., 2020; Soares and Cantarella, 2023).
The increase in NUE in grain during the dry season observed in La Bandera is attributed to the reduction under favorable conditions of NH3-N volatilization (Santos et al., 2023), and the translocation of N from foliage to grain. It is well-documented that enhancing the N utilization in stages preceding floral differentiation in rice contributes to approximately 50% of the final grain content (Wang et al., 2022). In the case of experiment in Barbudal conducted during the rainy season, C/N ratio of less than 7.5 suggests that N is readily available to the rice, and accordingly, most N is likely taken up from the soil, rather than from fertilizer. Therefore, the N saved by NBPT may serve as a complement that does not translate into NUE increases (Cantarella et al., 2018).
Tillage treatments did not affect NUE even though it is recognized that the removal of a compact soil layer could promote root development and subsequently enhance plant N uptake (Jug et al., 2019). What might be linked to soil disturbance promoting N mineralization rate and favoring N absorption from soil instead of from the fertilizer (Govindasamy et al., 2023).
The observed delay in the maximum rate of NH3 volatilization (Figure 3A) in UI1 compared to U1, as well as the decrease in the potential loss of NH3 -N with the application of NBPT in both La Bandera and Barbudal farms were due to the recognized delay in urea hydrolysis caused by NBPT (Wang et al., 2020a). This led to the dilution of the urea into the soil, limiting the availability of the NH4+ and hence its loss by volatilization (Silva et al., 2017; Uddin et al., 2021). Ammonia volatilization from the N-fertilized plots (U1 and UI1), independently of the tillage treatment, maintained similar emission intervals (Figure 3B) due to the short period of effective urease inhibition of NBPT under high temperatures and slightly acidic soils (Cantarella et al., 2018), which aligns with NBPT half-life in non-sterilized soil of only 0.59 d at pH 6.1 reported by Engel et al. (2015).
The difference in the timing of the highest NH3 emission rate after urea application between La Bandera and Barbudal trials (Figure 3A) was associated with the lower soil water content in La Bandera, as this factor affects urease activity and thus the hydrolysis rate of the applied urea (Tian et al., 2015; Cantarella et al., 2018). As a result, the potential NH3 losses increased substantially in La Bandera until day three when irrigation was applied, increasing the soil water content, and promoting urea hydrolysis. Otherwise, in Barbudal, the maximum rate of NH3 losses from urea fertilizer occurred within the first three days after broadcast application, which is consistent with Uddin et al. (2021).
Is worth noticing that the differences observed in NH3 emissions in la Bandera, where emissions were higher in RT than in the CT or the RTS. This condition could be caused by several factors, first an increment in available water (RT reaching up to 5% higher than the CT, Figure 2B) as indicate by Alvarez and Steinbach (2009), that could provide better conditions for urea hydrolysis before and after irrigation. Second, an increase in soil urease activity resulting from the presence of vegetation from crop residues, which leads to a higher concentration of NH4+. Third, the presence of crop residues can prevent soil contact and reduce NH4+ adsorption. Finally, it is possible that the reduced penetration of N fertilizer applied to RT plots contributed to this condition, as these plots showed fewer shallow soil cracks, particularly when compared to RTS plots (Rochette et al., 2009; Uddin et al., 2021).
As it is already accepted, the urease inhibitor NBPT application reduces N losses through NH3 volatilization. However, as it was mentioned in the previous sections, it was not a viable alternative for significantly increasing grain yield or NUE in our experimental conditions. The effectiveness of the urease inhibitor depends on environmental factors such as rainfall, temperature, and soil properties (like pH, and ECEC), as when urea diffuses and hydrolyses, the NH4+ originated may undergo sorption to clays in the soil matrix, while NH3 can interact with soil acidity (Cantarella et al., 2018; Matczuk and Siczek, 2021).
Further research is necessary to determine the conditions that justify the application of NBPT from an economic point of view.
Exploring the use of urease inhibitors in conjunction with split or reduced rates of N application, as well as implementing proper soil management practices, could be crucial in reducing management costs and improving rice yields in tropical soils.
The raw data supporting the conclusions of this article will be made available by the authors, without undue reservation.
AP-C: Conceptualization, Data curation, Formal analysis, Funding acquisition, Investigation, Methodology, Project administration, Resources, Supervision, Validation, Visualization, Writing – original draft, Writing – review & editing. WG-S: Data curation, Formal Analysis, Investigation, Methodology, Validation, Visualization, Writing – original draft, Writing – review & editing. MM-M: Conceptualization, Formal analysis, Investigation, Methodology, Writing – original draft, Writing – review & editing. CC-S: Conceptualization, Formal analysis, Visualization, Writing – review & editing. MA-M: Conceptualization, Investigation, Writing – review & editing. MZ: Conceptualization, Funding acquisition, Resources, Writing – review & editing.
The author(s) declare financial support was received for the research, authorship, and/or publication of this article. This work was funded by the International Atomic Energy Agency (IAEA), Vienna, Austria, through a Coordinated Research Project (No. CRP D1 50 20) of the Soil and Water Management and Crop Nutrition Section, Joint FAO/IAEA Division of Nuclear Techniques in Food and Agriculture, through the Technical Cooperation Project (No. COS5035), and by the Research Vice Presidency of the Universidad de Costa Rica (No. VI-802-B8-501).
We kindly acknowledge the support of Corporación Arrocera Nacional (CONARROZ), to Eng. Max Carballo Salazar and the rice producer Daniel Fernández for their help with the field experiments, and to the Costa Rica National Meteorological Institute (IMN) for the climate data shared, contract IMN-DIM-CM-056-2022. Support of student Roberto Aguirre for penetration resistance measurement in La Bandera Farm is acknowledged. Partial content of the manuscript has previously appeared online in the master’s thesis titled “Efecto de un inhibidor de la ureasa (NBPT) en la eficiencia de uso del nitrógeno, las pérdidas de amoníaco y las emisiones de óxido nitroso y metano en el cultivo de arroz de secano (Giraldo-Sanclemente, 2024) in Spanish.
The authors declare that the research was conducted in the absence of any commercial or financial relationships that could be construed as a potential conflict of interest.
All claims expressed in this article are solely those of the authors and do not necessarily represent those of their affiliated organizations, or those of the publisher, the editors and the reviewers. Any product that may be evaluated in this article, or claim that may be made by its manufacturer, is not guaranteed or endorsed by the publisher.
The Supplementary Material for this article can be found online at: https://www.frontiersin.org/articles/10.3389/fagro.2024.1394143/full#supplementary-material
Abalos D., Jeffery S., Sanz-Cobena A., Guardia G., Vallejo A. (2014). Meta-analysis of the effect of urease and nitrification inhibitors on crop productivity and nitrogen use efficiency. Agr. Ecosyst. Environ. 189, 136–144. doi: 10.1016/j.agee.2014.03.036
Alvarez R., Steinbach H. S. (2009). review of the effects of tillage systems on some soil physical properties, water content, nitrate availability and crops yield in the Argentine Pampas. Soil Tillage Res. 104, 1–15. doi: 10.1016/j.still.2009.02.005
Araújo S. E., Marsola T., Miyazawa M., Soares H. L., Urquiaga S., Boddey R., et al. (2009). Calibration of a semi-opened static chamber for the quantification of volatilized ammonia from soil. Pesqui. Agropecu. Bras. 44, 769–776. doi: 10.1590/S0100-204X2009000700018
Bolleter W. T., Bushman C. J., Tidwell P. W. (1961). Spectrophotometric determination of ammonia as indophenol. Anal. Chem. 33, 592–594. doi: 10.1021/ac60172a034
Cantarella H., Otto R., Soares R. J., Silva G. A. (2018). Agronomic efficiency of NBPT as a urease inhibitor: A review. J. Adv. Res. 13, 19–27. doi: 10.1016/j.jare.2018.05.008
CONARROZ (2023a) Informes Estadísticos. Available online at: https://www.conarroz.com/estadisticasarroceras.php (Accessed March 21, 2024).
CONARROZ (2023b) Fertilización. Available online at: https://www.conarroz.com/revistaarrocera.php (Accessed March 21, 2024).
de Vries W. (2021). Impacts of nitrogen emissions on ecosystems and human health: A mini review. Curr. Opin. Environ. Sci. Health 21, 100249. doi: 10.1016/j.coesh.2021.100249
Engel R. E., Towey B. D., Gravens E. (2015). Degradation of the urease inhibitor NBPT as affected by soil pH Soil Sci. Soc Am. J. 79, 1674–1683. doi: 10.2136/sssaj2015.05.0169
Giraldo-Sanclemente W. (2024). Efecto de un inhibidor de la ureasa (NBPT) en la eficiencia de uso del nitrógeno, las peérdidas de amoní́aco y las emisiones de óxido nitroso y metano en cultivo de arroz de secano (Thesis). Ciudad Universitaria Rodrigo Facio, Costa Rica: Universidad de Costa Rica. Available at: the Universidad de Costa Rica institutional repository, named Kérwá.
Götze H., Saul M., Jiang Y., Pacholski A. (2023). Effect of incorporation techniques and soil properties on NH3 and N2O emissions after urea application. Agronomy 13, 2632. doi: 10.3390/agronomy13102632
Govindasamy P., Muthusamy S. K., Bagavathiannan M., Mowrer J., Jagannadham P. T. K., Maity A., et al. (2023). Nitrogen use efficiency—a key to enhance crop productivity under a changing climate. Front. Plant Sci. 14. doi: 10.3389/fpls.2023.1121073
Guardia G., Sanz-Cobena A., Sanchez-Martin L., Fuertes-Mendizabal T., Gonzalez-Murua C., Alvares J. M., et al. (2018). Urea-based fertilization strategies to reduce yield-scaled N oxides and enhance bread-making quality in a rainfed Mediterranean wheat crop. Agr. Ecosyst. Environ. 265, 421–431. doi: 10.1016/j.agee.2018.06.033
Gürsoy S. (2021). “Soil compaction due to increased machinery intensity in agricultural production: its main causes, effects and management,” in Technology in Agriculture. Eds. Ahmad F., Sultan M. (London: IntechOpen), 95–112. doi: 10.5772/intechopen.98564
Horneck D. A., Miller R. O. (2019). “Determination of total nitrogen in plant tissue,” in Handbook of Reference Methods for Plant Analysis, Soil and Plant Analysis. Ed. Kalra Y. P. (Boca Raton: CRC Press), 75–83.
Jantalia C. P., Halvorson D. A., Follet R., Alves J. B., Polidiro C. J., Urquiaga S. (2012). Nitrogen source effects on ammonia volatilization as measured with semi-static chambers. Agron. J. 104, 1595–1603. doi: 10.2134/agronj2012.0210
Jian-She Z., Fu-Ping Z., Jin-Hua Y., Jin-Ping W., Ming-Li C., Li C. F., et al. (2011). Emissions of N2O and NH3, and nitrogen leaching from direct seeded rice under different tillage practices in central China. Agr. Ecosyst. Environ. 140, 164–173. doi: 10.1016/j.agee.2010.11.023
Jug D., Brozović B., Đurđević B., Jug I., Lipiec J., Birkás M., et al. (2019). Effect of conservation tillage on crop productivity and nitrogen use efficiency. Soil Till Res. 194, 104327. doi: 10.1016/j.still.2019.104327
Klimczyk M., Siczek A., Schimmelpfennig L. (2021). Improving the efficiency of urea-based fertilization leading to reduction in ammonia emission. Sci. Total Environ. 771, 145483. doi: 10.1016/j.scitotenv.2021.145483
Koci J., Nelson P. N. (2016). Tropical dairy pasture yield and nitrogen cycling: Effect of urea application rate and a nitrification inhibitor, DMPP. Crop Pasture Sci. 67, 766–779. doi: 10.1071/CP15400
Lasisi A., Akinremi O. (2022). Degradation of N-(n-butyl) thiophosphoric triamide (NBPT) with and without nitrification inhibitor in soils. Nitrogen. 3, 161–169. doi: 10.3390/nitrogen3020012
Li Y., Huang L., Zhang H., Wang M., Liang Z. (2017). Assessment of ammonia volatilization losses and nitrogen utilization during the rice growing season in alkaline salt-affected soils. Sustainability. 9, 132. doi: 10.3390/su9010132
Liao L., Shao X., Ji R., Wen T., Junzen X. (2015). Ammonia volatilization from direct seeded later-rice fields as affected by irrigation and nitrogen managements. Int. J. Agric. Biol. 17, 582−588. doi: 10.17957/IJAB/17.3.14.636
Linquist B. A., Liu L., Van Kessel C., Van Groenigen K. J. (2013). Enhanced efficiency nitrogen fertilizers for rice systems: Meta-analysis of yield and nitrogen uptake. Field Crops Res. 154, 246–254. doi: 10.1016/j.fcr.2013.08.014
Liu T., Huang J., Chai K., Cao C., Li C. (2018). Effects on N fertilizer sources and tillage practices on NH3 volatilization, grain yield, and N use efficiency of rice fields in Central China. Front. Plant Sci. 9. doi: 10.3389/fpls.2018.00385
Martins M. R., Sant’ Anna S. A., Zaman M., Santos R., Monteiro R. C., Alves B., et al. (2017). Strategies for the use of urease and nitrification inhibitors with urea: impact on N2O and NH3 emissions, fertilizer-15N recovery and maize yield in a tropical soil. Agr. Ecosyst. Environ. 247, 54–62. doi: 10.1016/j.agee.2017.06.021
Martins M. R., Sarkis L. F., Guareschi R. F., Santos C. A., Sant’Anna S. A. C., Zaman M., et al. (2021). A simple and easy method to measure ammonia volatilization: accuracy under field conditions. Pedosphere 31, 255–264. doi: 10.1016/S1002-0160(20)60077-7
Matczuk D., Siczek A. (2021). Effectiveness of the use of urease inhibitors in agriculture: A review. Int. Agrophys. 35, 197–208. doi: 10.31545/intagr/139714
Meng X., Li Y., Yao H., Wang J., Dai F., Wu Y., et al. (2020). Nitrification and urease inhibitors improve rice nitrogen uptake and prevent denitrification in alkaline paddy soil. Appl. Soil Ecol. 154, 103665. doi: 10.1016/j.apsoil.2020.103665
Rector C., Brye K., Humphreys J., Norman R., Slaton N., Gbur E., et al. (2018). Tillage and coated-urea effects on nitrous oxide emissions from direct-seeded, delayed-flood rice production in Arkansas. J. Rice Res. developments 1, 25–37. doi: 10.36959/973/417
Rochette P., Angers D. A., Chantigny M. H., MacDonald J. D., Gasser M. O., Bertrand N. (2009). Reducing ammonia volatilization in a no-till soil by incorporating urea and pig slurry in shallow bands. Nutr. Cycling Agroecosyst. 84, 71–80. doi: 10.1007/s10705-008-9227-6
Santos C., Pinto S. I. D. C., Guelfi D., Rosa S. D., da Fonseca A. B., Fernandes T. J., et al. (2023). Corn cropping system and nitrogen fertilizers technologies affect ammonia volatilization in Brazilian tropical soils. Soil Syst. 7, 54. doi: 10.3390/soilsystems7020054
Sanz-Cobena A., Lassaleta L., Estellés F., Del Prado A., Guardia G., Abalos D., et al (2014). Yield-scaled mitigation of ammonia emissions from N fertilization: the Spanish case. Environ. Res. Lett. 9, 12. doi: 10.1088/1748-9326/9/12/125005
Silva A., Sequeira C. H., Sermarini R., Otto R. (2017). Urease inhibitor NBPT on ammonia volatilization and crop productivity: A meta-analysis. Agr. J. 119, 1–13. doi: 10.2134/agronj2016.04.0200
Skorupka M., Nosalewicz A. (2021). Ammonia volatilization from fertilizer urea—A new challenge for agriculture and industry in view of growing global demand for food and energy crops. Agriculture 11, 822. doi: 10.3390/agriculture11090822
Soares J. R., Cantarella H. (2023). Dynamics of ammonia volatilization from NBPT-treated urea in tropical acid soils. Sci. Agric. 80, 01403. doi: 10.1590/1678-992x-2022-0076
Sun M., Ren A. X., Gao Z. Q., Wang P. R., Mo F., Xue L. Z., et al. (2018). Long-term evaluation of tillage methods in fallow season for soil water storage, wheat yield and water use efficiency in semiarid southeast of the Loess Plateau. Field Crop Res. 218 pp, 24–32. doi: 10.1016/j.fcr.2017.12.021
Tian Z., Wang J., Liu S., Zhang Z., Dodla S. K., Myers G. (2015). Application effects of coated urea and urease and nitrification inhibitors on ammonia and greenhouse gas emissions from a subtropical cotton field of the Mississippi delta region. Sci. Total Environ. 533, 329–338. doi: 10.1016/j.scitotenv.2015.06.147
Tubert-Brohman I., Sherman W., Repasky M., Beuming T. (2013). Improved docking of polypeptides with glide. J. Chem. Inf. Model. 53, 1689–1699. doi: 10.1021/ci400128m
Uddin S., Nitu T. T., Milu U. M., Nasreen S. S., Hossenuzzaman M., Haque M. E., et al. (2021). Ammonia fluxes and emission factors under an intensively managed Wetland rice ecosystem. Environ. Sci. Processes Impacts 23, 132). doi: 10.1039/D0EM00374C
Wang Y., Chen S., Zhang D., Yang L., Cui T., Jing H., et al. (2020b). Effects of subsoiling depth, period interval and combined tillage practice on soil properties and yield in the Huang-Huai-Hai Plain, China. J. Integr. Agriculture. 19, 1596–1608. doi: 10.1016/S2095-3119(19)62681-X
Wang H., Köbke S., Dittert K. (2020a). Use of urease and nitrification inhibitors to reduce gaseous nitrogen emissions from fertilizers containing ammonium nitrate and urea. Glob. Ecol. Conserv. 22, e00933. doi: 10.1016/j.gecco.2020.e00933
Keywords: NBTP, nitrogen use efficiency, nutrient management practices, rice yield, tropical soils, tillage
Citation: Pérez-Castillo AG, Giraldo-Sanclemente W, Monge-Muñoz M, Chinchilla-Soto C, Alpízar-Marín M and Zaman M (2024) Rice yield in Costa Rican Central Pacific did not improve with a urease inhibitor. Front. Agron. 6:1394143. doi: 10.3389/fagro.2024.1394143
Received: 01 March 2024; Accepted: 26 March 2024;
Published: 09 April 2024.
Edited by:
Hanuman Singh Jatav, Sri Karan Narendra Agriculture University, IndiaReviewed by:
Ankit Saini, Eternal University, IndiaCopyright © 2024 Pérez-Castillo, Giraldo-Sanclemente, Monge-Muñoz, Chinchilla-Soto, Alpízar-Marín and Zaman. This is an open-access article distributed under the terms of the Creative Commons Attribution License (CC BY). The use, distribution or reproduction in other forums is permitted, provided the original author(s) and the copyright owner(s) are credited and that the original publication in this journal is cited, in accordance with accepted academic practice. No use, distribution or reproduction is permitted which does not comply with these terms.
*Correspondence: Ana Gabriela Pérez-Castillo, YW5hLnBlcmV6Y2FzdGlsbG9AdWNyLmFjLmNy
†These authors have contributed equally to this work
Disclaimer: All claims expressed in this article are solely those of the authors and do not necessarily represent those of their affiliated organizations, or those of the publisher, the editors and the reviewers. Any product that may be evaluated in this article or claim that may be made by its manufacturer is not guaranteed or endorsed by the publisher.
Research integrity at Frontiers
Learn more about the work of our research integrity team to safeguard the quality of each article we publish.