- Department of Agronomy and Plant Breeding, Faculty of Agriculture, University of Kurdistan, Sanandaj, Iran
The source–sink (S-S) ratio during the grain-filling period is crucial for wheat crop yield. The aim of this study was to investigate the relative sensitivity of grain yield in response to treatments of S-S ratio changes to determine the extent of S-S limitation during grain filling in modern wheat genotypes. The S-S manipulation treatments included four levels: check (CH), removal of flag leaf (RFL), removal of all leaves (RAL), and removal of the upper half of the spikes (RHS). The results showed significant differences between genotypes (pb< 0.001%) in all traits. Drought stress decreased grain weight per spike (GWS) (g) and grain yield (GY) (kg/ha) by 18% and 25%, respectively. The average reduction in GWS under irrigation and rainfed conditions was 8.25% and 6.71% for RFL and 12.25% and 11.15% for RAL, respectively. By RFL and RAL, increasing the remobilization from the stem and spike straw helped to reduce the effects of source limitation. Also, by RHS, the reduction in photosynthetic materials production in both conditions was only equivalent to 38% and 29% of the expected values, respectively, which shows the presence of strong sinks in vegetative organs (stem and spike) compared to grains. Vegetative organs seem to have a larger sink for the uptake of photosynthetic materials than grains when the source–sink ratio increases. However, high-yield genotypes showed more severe source limitation, while low-yield genotypes showed more relative sink limitation. Overall, to increase the yield potential in high-yielding genotypes, photosynthetic sources and sinks in low-yielding genotypes should be improved.
1 Introduction
Bread wheat (Triticum aestivum L.) is one of the most important crops in the world, and its productivity has to be increased significantly to feed the growing world population, which is expected to reach over 9 billion by 2050 (CIMMYT, 2017; Alonso et al., 2018). The cultivated area of this plant in the world is 221 million hectares, and its production amount is 771 million tons (FAO, 2021). In major wheat-growing areas of the world, its productivity is adversely affected by various abiotic stresses, and among them, drought is the major abiotic stress causing serious damage (Saradadevi et al., 2017). In particular, terminal drought refers to the drought after anthesis, and it usually causes grain weight reduction and yield loss (Reynolds et al., 2005). The scenarios of terminal drought also alter the balance between sources and sinks of assimilation and consequently depress the rate and duration of grain filling and sink capacity (Ovenden et al., 2017). In this regard, physiological traits and processes related to drought resistance, including the source–sink (S-S) photosynthetic capacity, should be more accurately evaluated to be used in the breeding process of drought-tolerant cultivars. The growth and grain filling in wheat are controlled by the relationships between source strength and sink capacity (Foulkes et al., 2011). Knowing the physiological relationships between S-S can help to select and improve wheat grain yield (Maydup et al., 2013).
Source tissues are generally responsible for acquiring resources from the external environment, although the remobilization of stored resources may also turn a sink into an internal source. The term source strength refers to the net rate of uptake (mol/s) of a particular resource from the external environment, as seen in Equation 1:
where source size refers to the total biomass of source tissue (g), and source activity is the specific uptake rate of the resource (mol g−1 s−1). Also, sink tissues are net receivers of resources from source tissues. The term sink strength refers to the net rate of uptake (mol/s) of a particular resource by a defined tissue within the plant, as seen in Equation 2:
where sink size is the total biomass of sink tissue (g), and sink activity refers to the specific uptake rate of the resource (mol g−1 s−1). Source tissues thus take up environmental resources and export them to sinks (White et al., 2016). The leaf is the major organ involved in light perception and the conversion of solar energy into organic carbon (Du et al., 2019). The flag leaf is the main component of the canopy in the middle and late growth stages of winter wheat (Liu et al., 2021) and is an important organ that determines the grain-filling rate and the final yield (Vicente et al., 2018). The contribution rate of flag leaves to daily photosynthetic products varies from 50% to 60% (Towfiq et al., 2015), while its defoliation generated grain yield losses of 18% to 30% (Ma et al., 2021). In wheat, the defoliation of the flag leaf blade increased the contribution of assimilates to the grain from the stem and the chaff under normal conditions (Alvaro et al., 2008), and the removal of these affected the grain yields under normal or water-limiting conditions (Cruz-Aguado et al., 1999). Chlorophylls and carotenoids are photosynthetic pigments capable of absorbing light, transmitting energy to the photochemical and biochemical phases of photosynthesis, and accumulating chemical energy that is stored as sugar (Bojovi´c and Stojanovi´c, 2005). Determination of chlorophyll content as an indirect method of estimating the productivity of vegetation represents a good way to gain an understanding of the photosynthetic regime of plants (Niroula et al., 2019).
The sink size of developing yield organs is determined by the number of spikes per unit area, grain number per spike (GNS), and the specific sink size per grain. Source size is related to the production of photo-assimilates, namely, the size, photosynthetic capacity, and duration of leaf area, which drives spike development and grain filling (Jagadish et al., 2015). Grain yield is often limited by sink capacity or lack of photo-assimilates (Maydup et al., 2013). One of the ways to achieve high yield in wheat genotypes is to allocate more photo-assimilates to economic sinks (grains) (Felekori et al., 2014). Manipulation of source strength and sink capacity has been investigated in several studies to determine the mechanisms controlling grain yield. In a balanced situation between S-S, the highest grain yield is produced (Borras and Salfer, 2004). In this regard, various treatments such as removal of leaves and shading indifferent light intensities and for different periods (Wang et al., 2003), increasing the concentration of carbon dioxide (Manderscheid et al., 2003), and removal of spikelets and grains in different parts of the spike (Cruz-Aguado et al., 2000) have been used to investigate S-S relationships in wheat, leading to different results by researchers. The researchers studied the effects of removing the flag leaf and removing the upper half of the spike in 24 durum wheat varieties (both modern and old cultivars). They observed that the treatment of removing half of the spike increased the weight of the remaining grains, indicating a limitation in the supply of photo-assimilates during grain filling under normal conditions (Alvaro et al., 2007). The increase in grain weight in response to the decrease in sink ratio indicates that grains have not reached their maximum growth under normal conditions due to insufficient photosynthesis (Saeidi et al., 2011). In addition, other experiments also show that most improved wheat genotypes have resource limitations.
Depending on the environmental conditions, genotypes have different resource limitations, and it seems that examining the degree of limitation in wheat genotypes in a region shows the degree of compatibility of each genotype with that environment (Ahmadamini et al., 2011). Also, some researchers indicate both S-S limitations in wheat (Abdoli et al., 2013). When the S-S ratio decreases, sink-limited cultivars should be less affected than source-limited cultivars. In other words, defoliation reduces both traits of grain growth rate and grain weight of cultivars, but the relative reduction will be greater for cultivars with limited resources (Abdoli and Saeidi, 2013). Artificial defoliation in wheat may change the photosynthetic characteristics of the remaining tissues (Zhenlin et al., 1998). Researchers reported that after anthesis, source limitation by defoliation of winter wheat increased the net photosynthesis rate and chlorophyll content of wheat leaves (Zhu et al., 2004; Joudi et al., 2006).
The accumulation potential of storage materials in the stems and the rate of remobilization of these materials from the stem to the growing grains are two crucial characteristics in wheat grain yield and related to S-S relationships, which determine the final grain yield under environmental stress (Najafian and Shabani, 2010). In resistant cultivars, remobilization from stem nodes is more significant, especially under drought stress conditions (Saeidi et al., 2012). Under terminal drought stress, stem carbohydrate reserves become the major source of grain filling as leaf photosynthesis ceases (Zhang et al., 2015). These reserves are water-soluble carbohydrates (WSCs), mainly consisting of fructan and glucose, fructose, and sucrose as well as various oligosaccharides (Joudi et al., 2012). The amount of accumulation and remobilization of carbohydrates in the wheat stem can be estimated either by monitoring the changes in stem dry weight (Ma et al., 2014; Thapa et al., 2022) or by measuring the stem WSC content (Liu et al., 2020). Drought stress significantly accelerates the remobilization of pre-anthesis stem water-soluble carbohydrate reserves during the period of grain filling (Liu et al., 2020). The lower grain weight reduction per spike in some genotypes in response to source reduction could be stimulation and remobilization of more storage materials from stem to grains, which partially compensates for yield reduction (Khan et al., 2002). The researchers reported that genotypes with a higher remobilization rate were less affected by drought stress during the final growing season (Yang and Zhang, 2006), and severe drought stress increased the remobilization rate to grow grains due to early maturity and dropping of lower leaves (Bagherikia et al., 2017). In this regard, the researchers obtained a positive and significant correlation between the amount of remobilization and grain weight under stress conditions (Papakosta and Gayianas, 1991).
Most studies to investigate the relationship between S-S in wheat have been conducted on a limited number of cultivars and still need a preliminary evaluation of the relative limitations of S-S in the investigated genotypes. In continuation of the previous research, it is necessary to first identify the relative degree of S-S limitation based on morpho-physiological traits in a broader range of genotypes and then investigate more precise compensatory mechanisms in a smaller range of genotypes. The present study was conducted in order to investigate the S-S relationship and to evaluate the relative sensitivity of grain yield, in response to treatments of reduction S-S ratio, in two conditions of optimal irrigation and rainfall. Also, in order to more closely investigate the S-S relationship, the remobilization values of stem and spike straw affected by the S-S limitation treatments were investigated.
2 Materials and methods
2.1 Plant materials
In the first year, 50 advanced bread wheat lines, the result of Icarda and Simit breeding programs, and six conventional bread wheat cultivars (Baharan, Pishgam, Pishtaz, Sirvan, Heydari, and Mihan) as check were grown under irrigation conditions (Table 1). In the second year, based on the experimental objectives and available diversity, 11 genotypes were selected including nine advanced lines (including three lines with source limitation, three lines with sink limitation, and three intermediate lines with both S-S limitations) to create genetic diversity and two conventional cultivars (Pishgam and Baharan) as check (Table 2). Lines with source limitation were selected based on the flag leaf area, soil plant analysis development (SPAD) values, and date to anthesis traits; lines with sink limitation were selected based on the GNS, grain weight, grain-filling duration, and grain-filling rate traits; lines with both S-S limitations were selected with intermediate traits (Table 1). These lines and cultivars were chosen because they have higher yields than other cultivars of different plant types (Table 2).
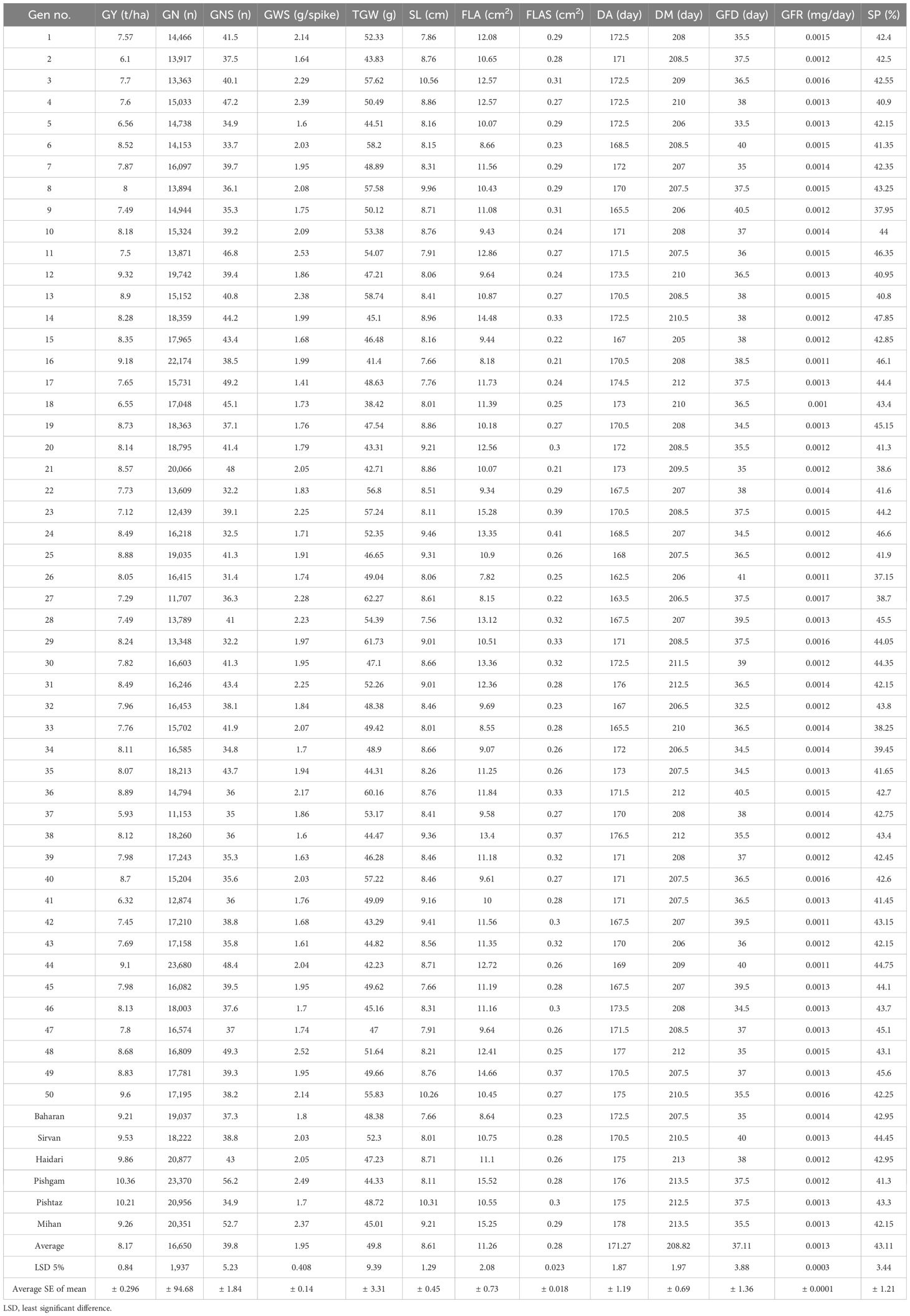
Table 1 The mean comparisons of grain yield (GY), grain number per m2 (GN), grain number per spike (GNS), grain weight per spike (GWS), thousand-grain weight (TGW), spike length (SL), flag leaf area (FLA), flag leaf area per each spike grain (FLAS), date to anthesis (DA), date to maturity (DM), grain-filling duration (GFD), grain-filling rate (GFR), and soil plant analysis development (SPAD) value (SP) in the first year under optimal irrigation conditions.
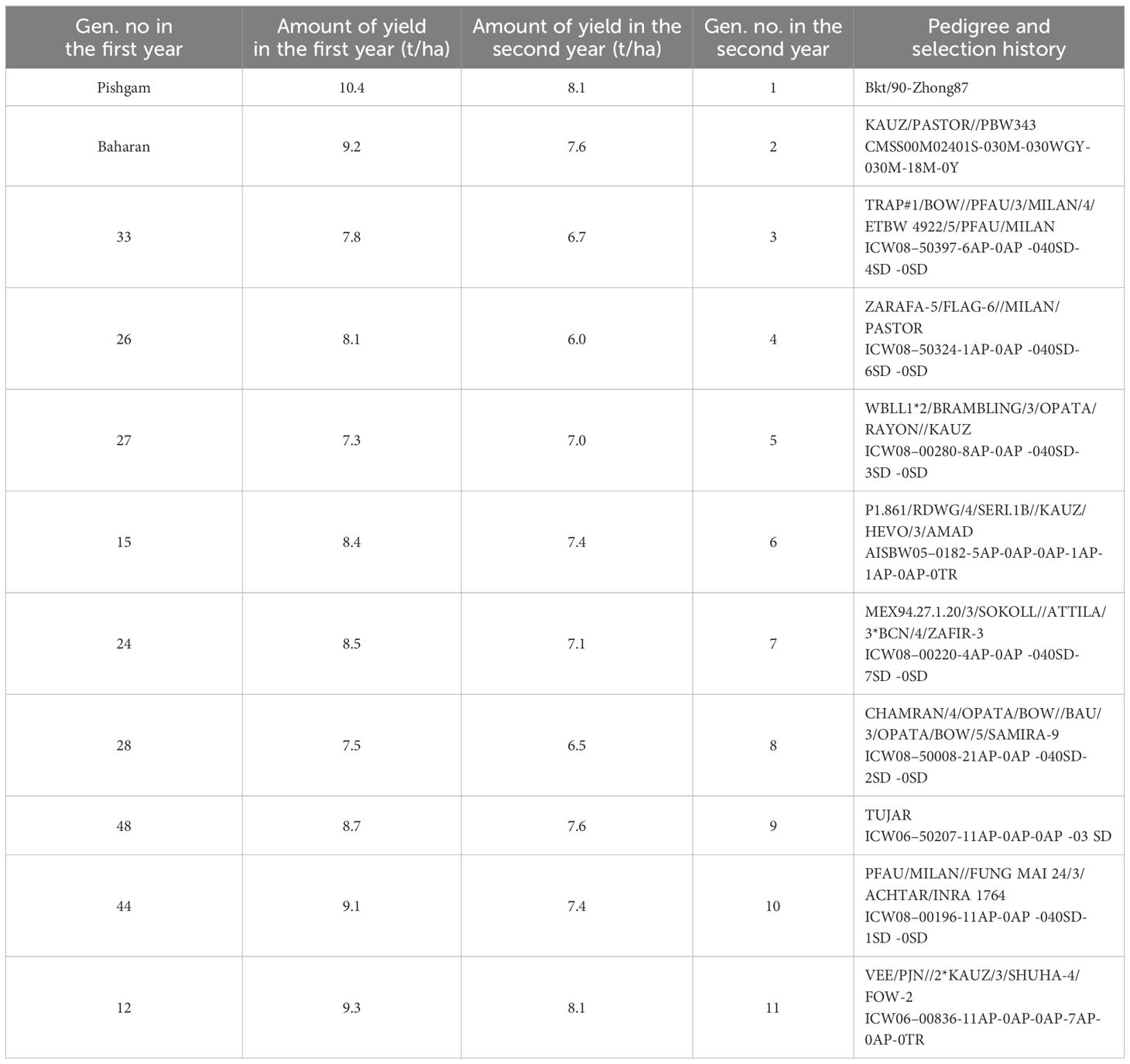
Table 2 Pedigree, selection history, and grain yield (t/ha) of evaluated lines in the first and second years under irrigation conditions.
2.2 Experimental design and field management
This experiment was conducted during the 2017–018 and 2018–2019 cropping years at the Islamabad-e-Gharb Agricultural Research Station (latitude 34°8′ North, longitude 47°26′ East, altitude 1,346 m above sea level). The average annual rainfall was 468 mm, and the average annual temperature was +13°C. The climatic characteristics of the experiment sites are listed in Figures 1A, B. In the first year of the experiment, the desired genotypes were investigated in the format alpha-lattice design in two replicates under irrigation conditions to select suitable genotypes. The genotypes were cultivated on November 5, 2017, in an experimental planter, and the seed rate was 400 seeds/m2. Plots were 3 m long and 1.2 m wide (3.6 m2 total plot area) with six rows in each spaced 0.2 m apart. In the second year, 11 selected genotypes including lines 12, 15, 24, 26, 27, 28, 33, 44, and 48, and two cultivars (Pishgam and Baharan) as check were planted (Table 2). The experimental design was split-plot in a randomized complete block design (RCBD) with three replicates in two separate sites, including i) irrigation and ii) rainfed conditions, using macro plots of 10 m long and 1.2 m wide (12 m2 total plot area) with six rows in each spaced 0.2 m apart, assigning the 11 genotypes to the main plots and the four S-S treatments (CH, RFL, RAL, and RHS) to the subplots. The genotypes were planted in an experimental planter on November 11, 2018, and in both conditions, the seed rate was 400 seeds/m2. Irrigation was done with a fixed classical system, and the irrigation cycle was considered once every 6–8 days based on the conventional agriculture of the region. In rainfed conditions, no irrigation was done during the cropping season, and the amount of rainfall is shown in Figure 1B. The experimental field in the previous crop year was fallow, and the soil type was clay-loam. The physical and chemical characteristics of the experiment site are shown in Table 3. The amount of chemical fertilizers was determined and applied based on the soil test (Table 3), including 200 kg N/ha using urea (46% N) with 50 kg N/ha in sowing, and an additional 150 kg N/ha was applied at the jointing stage. P and K were applied as basal fertilizers with 100 kg P/ha as triple superphosphate (46% P2O5 and 15% Ca) and 50 kg K/ha as potassium sulfate (K2O51% and S 17%). Common herbicides applied to weed control include 2,4-D herbicide for eliminating broad-leaf weeds and clodinafop-propargyl for eliminating narrow-leaf weeds, and for pest control, chlorpyrifos-ethyl insecticide (1,500 mL/ha) was used twice during the crop cycle.
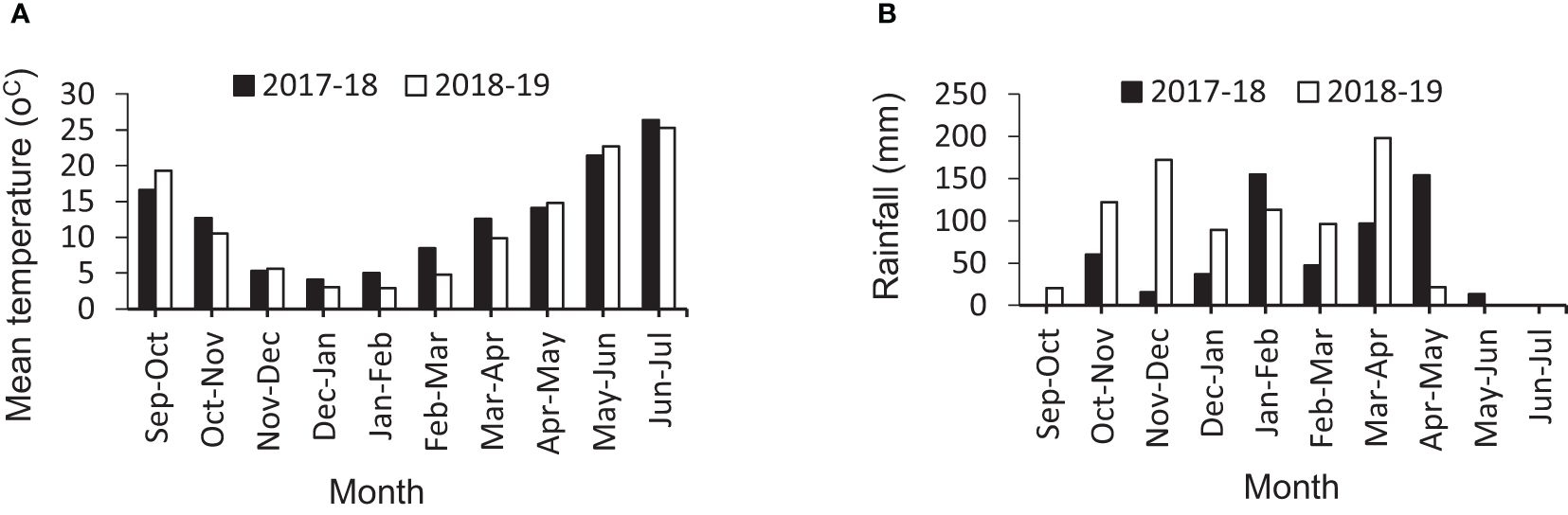
Figure 1 Climate conditions. (A) Changes of temperature in two cropping years, 2017–2018 and 2018–2019. (B) Changes of rainfall in two cropping years, 2017–2018 and 2018–2019.

Table 3 Soil information (0–20-cm depth) of Islamabad-e-Gharb Research Station (2017–2018 and 2018–2019 cropping years).
2.3 Trait measurements and growth analysis
In the first year, the grain yield and its components were recorded at maturity. All plants in each plot (3.6 m2) were harvested to determine grain yield (t/ha). Grain number per spike and grain weight per spike were determined by randomly sampling 10 spikes from each plot. Thousand-grain weight was determined from three subsamples of random 100 grains, and grain number was calculated as the ratio between grain yield and thousand-grain weight. Sink capacity (SICA) was calculated as the product of grain number and potential grain weight (Alonso et al., 2018). The date to anthesis and date to maturity were calculated based on the number of days from planting to anthesis (DC65) and planting to maturity stages (DC95; Zadoks et al., 1974). The grain-filling duration was calculated based on the days between anthesis and maturity. The grain-filling rate was calculated based on the weight of a single grain divided by the grain-filling duration (Wych et al., 1982). Spike length was measured based on the average of five spikes using a ruler. The area of the flag leaf was measured using a scanner as well as ImageJ and Photoshop software. All SPAD measurements were taken using a SPAD-502 PLUS chlorophyll meter (Konica Minolta Sensor, Osaka, Japan). SPAD values of the flag leaf in 50 lines and six cultivars were measured 15 days post-anthesis. The SPAD readings were obtained at the upper, middle, and lower positions of each lamina. Five laminae were measured in each plot, and these values were averaged. According to the above traits and to achieve enough diversity between the genotypes, nine lines and two cultivars (checks) were selected for cultivation in the next year (Table 2). In the second year, to measure the grain yield (GY) (kg/ha), the whole plot was harvested. Also, the desired traits include i) grain weight per spike (GWS), ii) GNS, iii) stem weight (SW), and iv) spike straw weight (SSW). Four S-S treatments were applied to the main shoot, i.e., i) check (CH), ii) removal of flag leaf (RFL), iii) removal of all leaves (RAL), and iv) removal of the upper half of the spikes (RHS); 50% of upper spikelets of the spike were removed by cutting with scissors (Serrago et al., 2013). These treatments were performed 15 days after anthesis (DC75; Zadoks et al., 1974) when the grain number (Abbate et al., 1997) and potential grain weight were mainly defined (i.e., the sink).
In this regard, 10 shoots (main stem) were randomly selected, and the above treatments were applied 15 days after anthesis simultaneously for maximum accumulation of storage materials in the stems and spikes. The samples were partitioned into different organs, including stems and spikes (straw and grains), and oven-dried at 75°C for 48 hours until a constant weight was attained. In this regard, to make the same observations in the data and figures, GWS, GNS, and SSW trait values were doubled in the RHS treatment. Also, in maturity, changes in the SW and SSW were measured, and based on this, remobilization values affected by S-S manipulation treatments were calculated. In this regard, remobilization values were measured according to the reduction in stem and spike straw dry weight from 15 days after anthesis to maturity compared to the check. The decrease in the stems or spikes straw dry weight in the source manipulation treatments (RFL and RAL) was considered the increase in remobilization value affected by source size reduction. Also, the increase in SW or SSW in the sink manipulation (RHS) treatment was considered a reduction in remobilization values affected by sink size reduction. The amount of accumulation and remobilization of carbohydrates in wheat stem was measured by measuring the WSC content (Liu et al., 2020). Accordingly and based on the literature (Ma et al., 2014), the amount of remobilized WSCs was calculated as follows: WSC remobilization of the stem = the maximum WSCs of the stem at 10 days after anthesis minus the WSCs of the stem at maturity. Also, stem remobilization efficiency was estimated using the proportion (%) of the mobilized WSCs relative to the maximum weight of that segment. The following formula was used in order to calculate the amount of source limitation in RHS treatment (Modhej, 2001).
In this formula, SL (%), a, and b are the source limitation percentage, the average weight of the spike in the halved spikes, and the average weight of the spike in the check, respectively. Also, the following relationship was used to calculate the role and influence of the flag leaf and the whole leaves in filling the grains.
In this formula, EL (%), c, and d are the role of leaves (flag leaf or total leaves) in grain-filling percentage, the average spike weight in check, and the average spike weight in defoliated plants (RFL and RAL treatments), respectively. Also, chlorophyll contents, including chlorophyll a, chlorophyll b, total, and carotenoids, were measured 15 days after anthesis on flag leaf samples based on the method (Arnon, 1967).
2.4 Statistical analysis
In the first year, the studied genotypes were investigated in the format alpha-lattice design and with ALPHA software in two replications under irrigation conditions. In the second year, Statistical Analysis System (SAS ver 9.1) software was used to perform analysis of variance (ANOVA), significance analysis, and Pearson’s correlation analysis. The statistical comparisons are indicated by asterisks in the results as significant at the 0.05 (*) and 0.01 (**) probability levels. Mean comparisons among cultivars and S-S manipulations were performed using least significant differences (LSDs) and calculated at the 5% probability level. Finally, the graphs were drawn using EXCEL software.
3 Results
3.1 Selection of genotypes according to yield and morphophysiological traits in the first year
In the first year of the experiment, the effect of cultivar on yield and morphophysiological traits was significant (data not shown). According to the observed diversity of genotypes regarding the above traits, nine out of 50 lines and two out of six cultivars were selected to evaluate the relationship between S-S in the second year (Tables 1, 2). Finally, according to the grain yield and morphophysiological traits, three lines with source limitation (lines 33, 26, and 27), three lines with sink limitation (lines 15, 24, and 28), and three intermediate lines with both relative S-S limitations (lines 48, 44, and 12) were selected. Also, among the conventional cultivars, the two cultivars as check with the highest yield (Pishgam) and the lowest yield (Baharan) and the diversity of morphophysiological traits were chosen for evaluation along with nine selected lines. The general status of the assessed traits of 11 selected genotypes compared to all evaluated genotypes is shown in Tables 1, 2.
3.2 Grain yield and grain number per spike
The data variance analysis showed that the effects of genotype and S-S manipulation on all evaluated traits were significant under irrigation and rainfed conditions (data not shown). Also, drought stress (rainfed conditions) reduced GY (25%), GNS (12%), GWS (18%), SSW (9%), and SW (18%) compared to irrigation conditions (Table 4). Researchers reported that drought stress in the pre-reproductive stage mainly inhibits the formation of wheat grain number per spike but has little impact on spike number and thousand-grain weight (Zhang et al., 2020; Gao et al., 2023). The GY varied in irrigation conditions at 5,949 to 8,133 and rainfed conditions at 4,550 to 6,587 kg/ha, and the results showed that genotypes with high yield in irrigation conditions had a higher yield in rainfed conditions (Figures 2A, B). The range of variation in the GNS was 28 to 65 and 25 to 47 in irrigation and rainfed conditions, respectively, depending on cultivar, treatment, and environment (Figures 3B, 4B). Genotypes 1, 9, 3, 10, and 11 had the highest GNS, and genotypes 4, 5, 2, and 7 had the lowest GNS in both irrigation and rainfed conditions (Table 4). Also, range of variation in GWS was 1.38 to 2.04 (g spike-1) and the most reduction in GNS was related to the RAL treatment in rainfed conditions (Figures 4A, 5B). A significant positive relationship (r = 0.637*) was observed between grain yield and GNS in rainfed conditions (Table 5). In the RFL treatment, the average decrease in GNS was 8% and 3% in irrigation and rainfed conditions, respectively, and in the RAL treatment, it was 17% and 9%, respectively (Table 6). Furthermore, in most investigated lines, RHS treatment did not affect GNS.
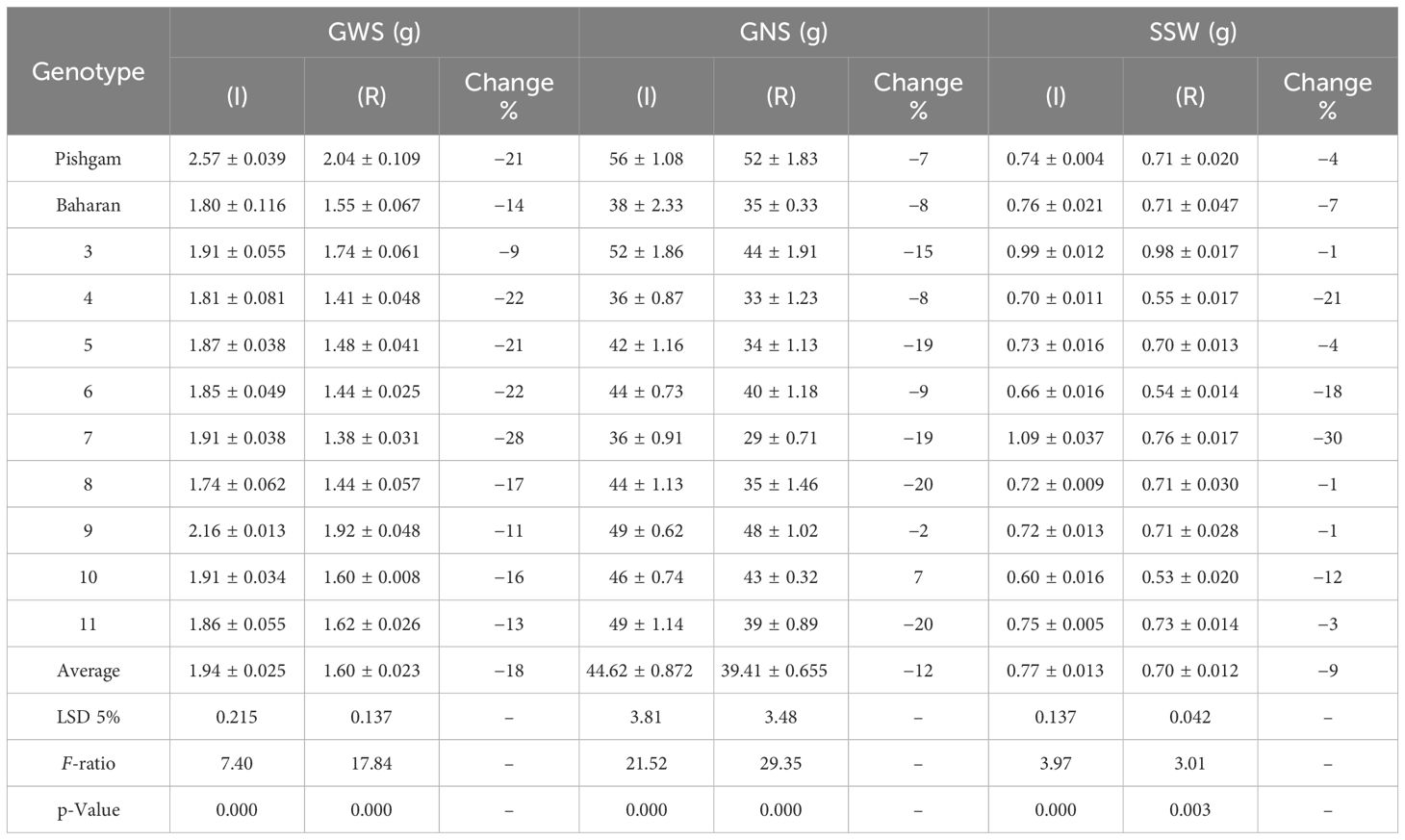
Table 4A The mean comparisons of grain weight per spike (GWS), grain number per spike (GNS), and spike straw weight (SSW) traits in various genotypes (Factor A) in irrigation (I) and rainfed (R) conditions in second year of experiment.
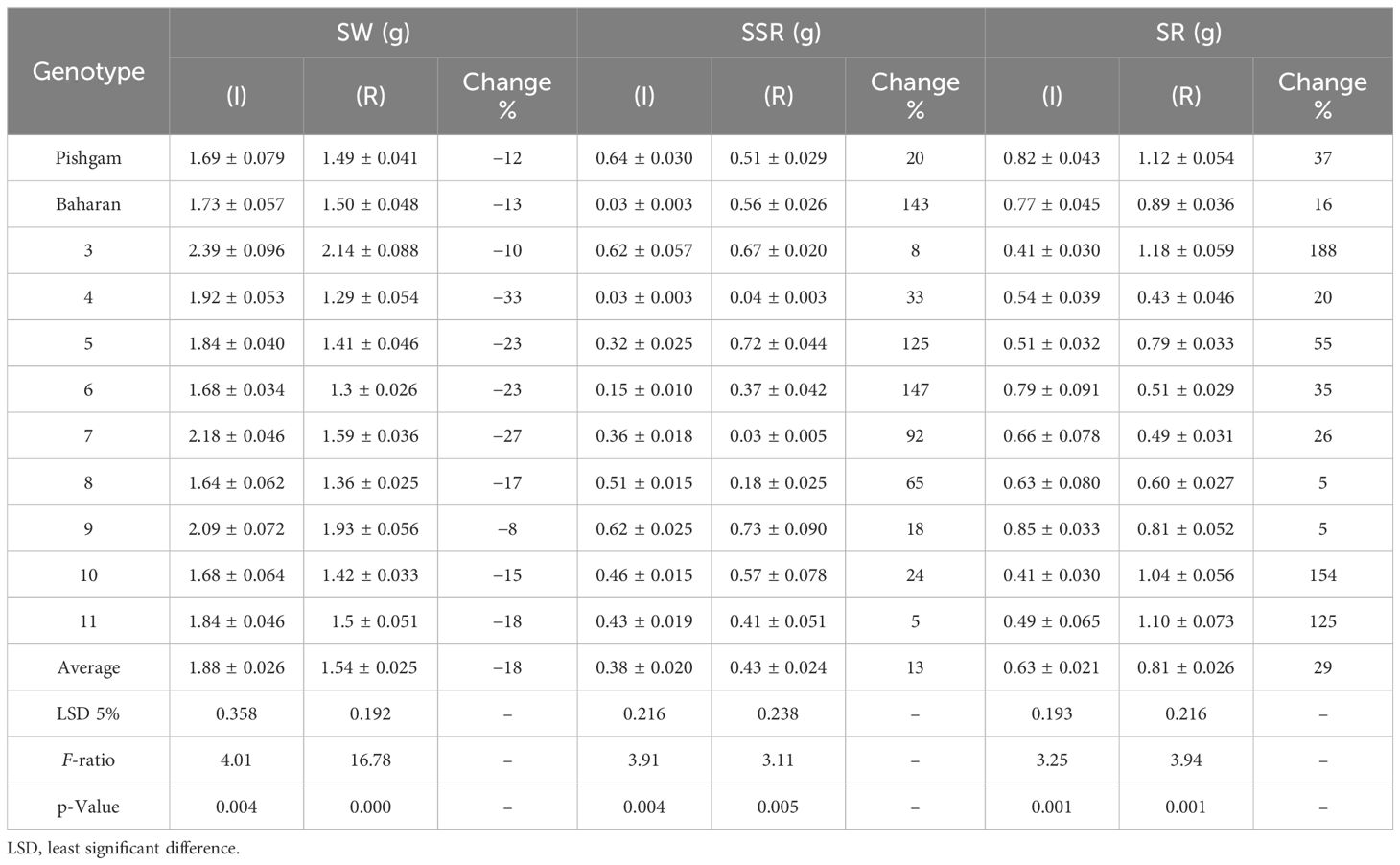
Table 4B The mean comparisons of stem weight (SW), spike straw remobilization (SSR), and stem remobilization (SR) traits in various genotypes (Factor A) in irrigation (I) and rainfed (R) conditions in second year of experiment.
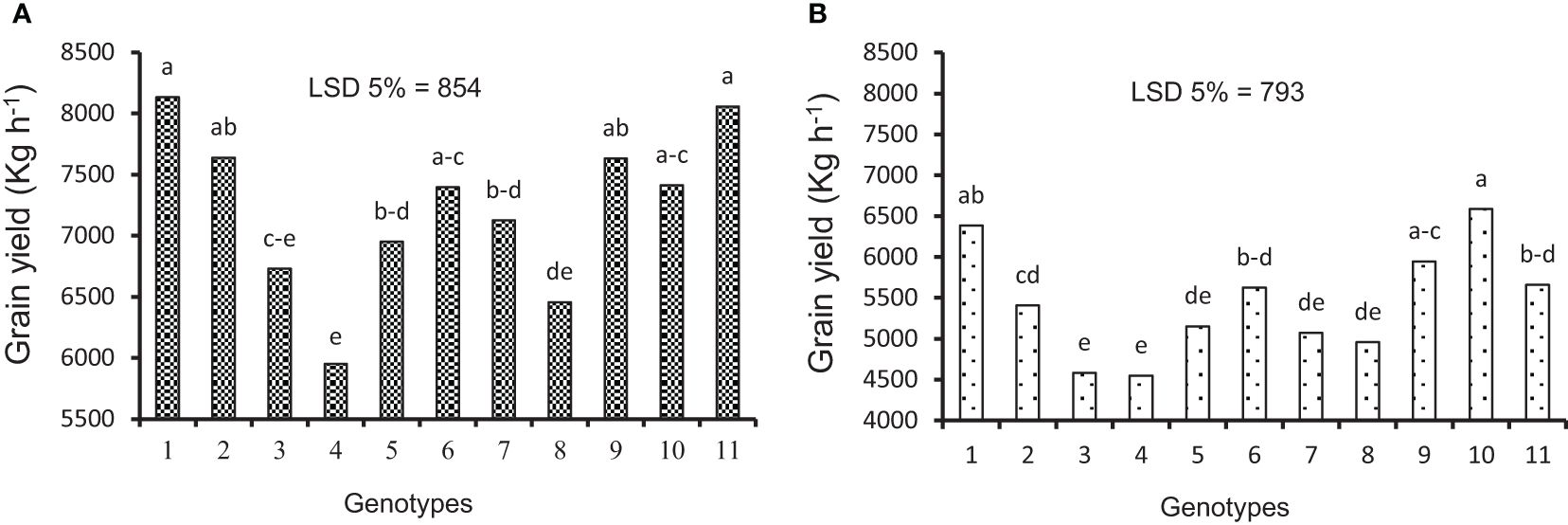
Figure 2 Average grain yield under (A) irrigation and (B) rainfed experiments in the second year. Means followed by the same letter within a column are not significantly different at the 5% probability level according to the least significant difference test.
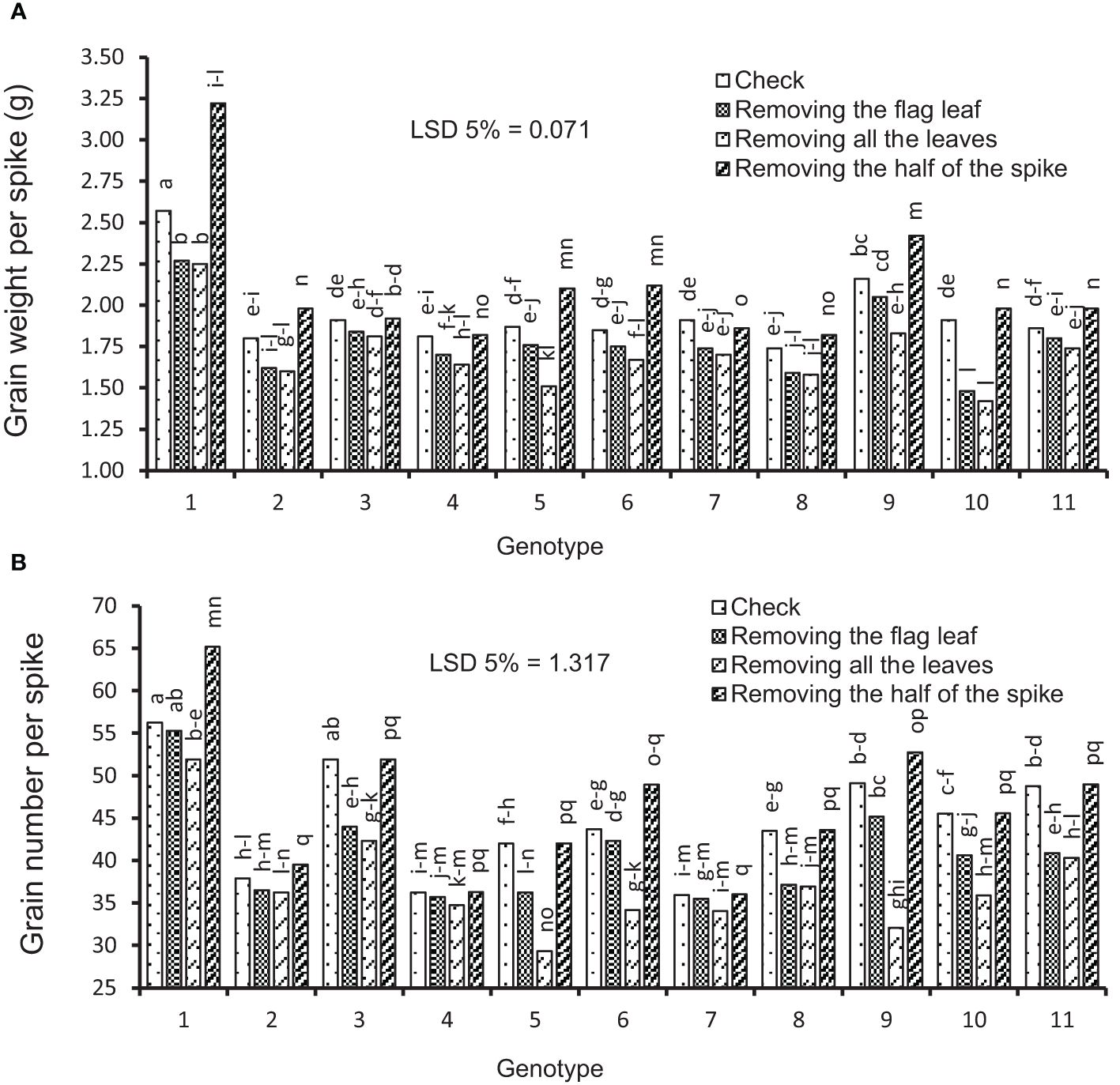
Figure 3 Mean comparison (under irrigation conditions) for interactions of genotypes × source–sink manipulation treatments including i) check, ii) removal of flag leaf, iii) removal of all leaves, and iv) removal of upper half of the spikes. (A) Average of grain weight per spike and (B) average of grain number per spike. Means followed by the same letter within a column are not significantly different at the 5% probability level according to the least significant difference test.
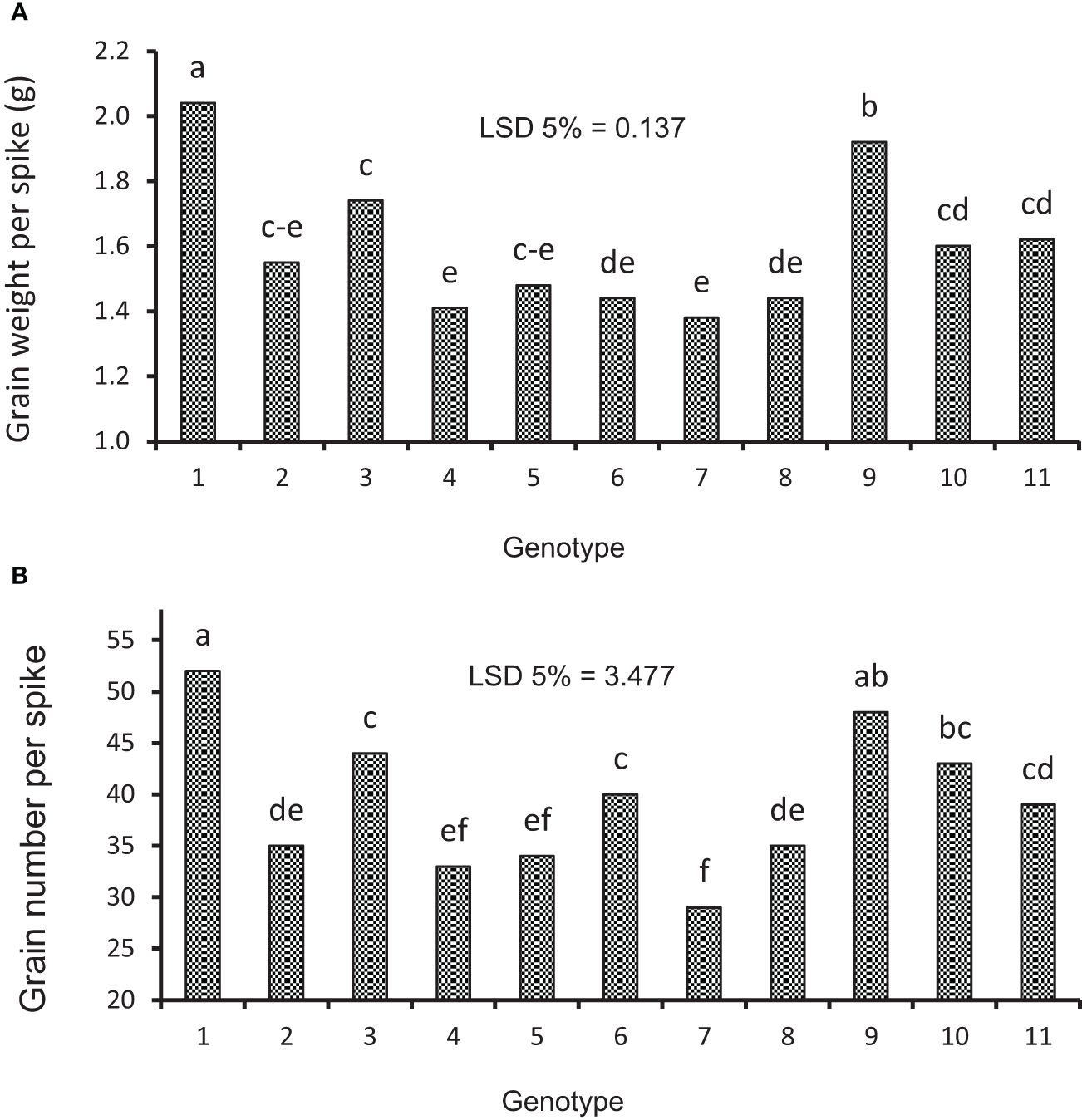
Figure 4 Mean comparison of genotypes under rainfed conditions: (A) average of grain weight per spike and (B) average of grain number per spike. Means followed by the same letter within a column are not significantly different at the 5% probability level according to the least significant difference test.
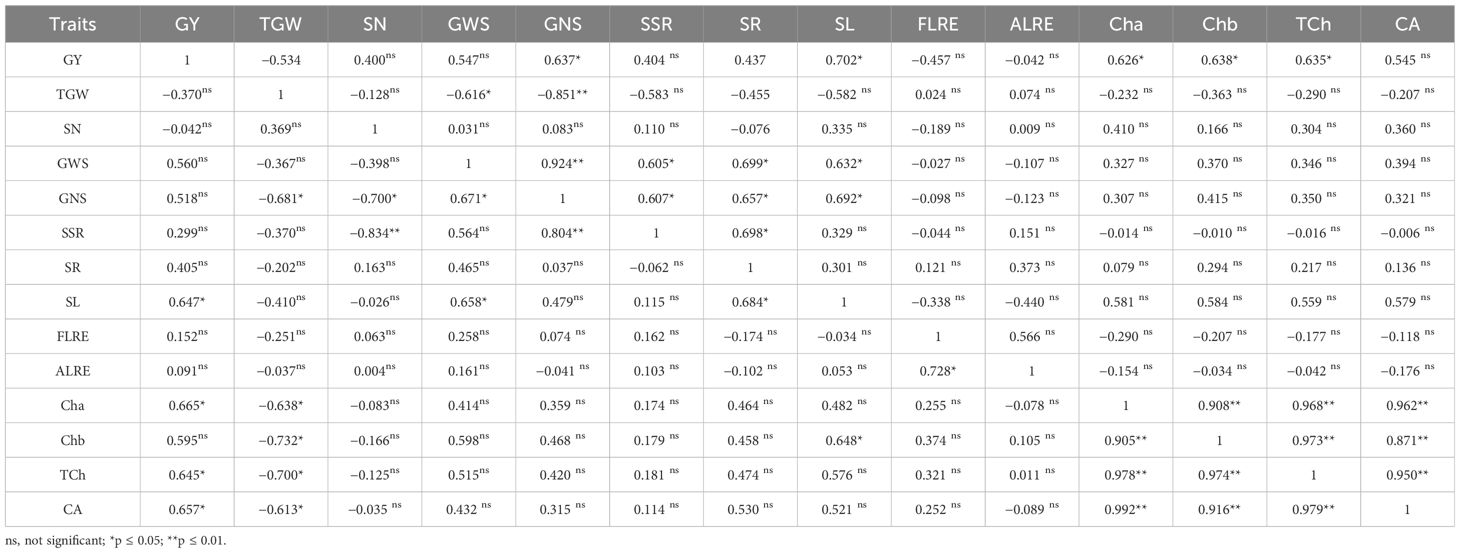
Table 5 Correlation coefficients grain yield (kg/ha) (GY), thousand-grain weight (TGW), spike number per m2 (SN), grain weight per spike (GWS), grain number per spike (GNS), spike straw remobilization (SSR), stem remobilization (SR), source limitation (SL), flag leaf removal effect (FLRE), all leaves removal effect (ALRE), chlorophyll a (Cha), chlorophyll b (Chb), total chlorophyll (TCh), and carotenoids (CA) of wheat in irrigation (down side) and rainfed (up side) conditions.
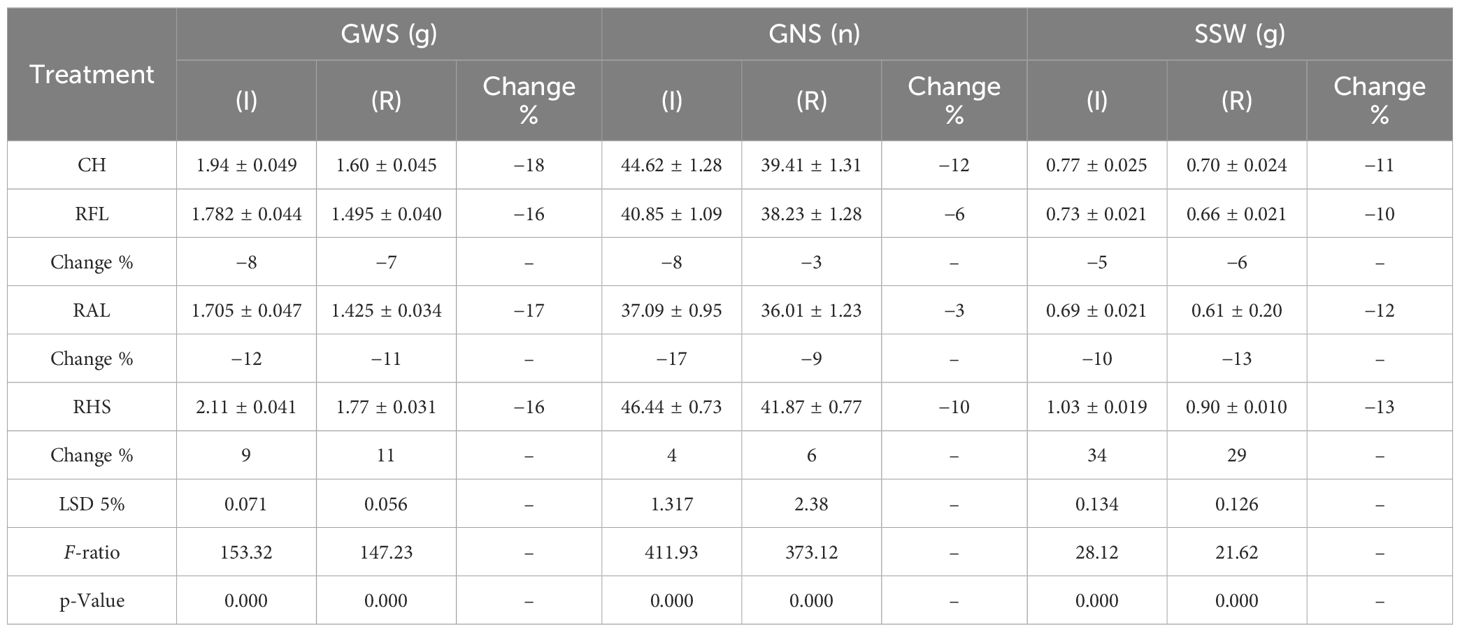
Table 6A The mean comparisons of grain weight per spike (GWS), grain number per spike (GNS), spike straw weight (SSW) traits under check (CH), removal of flag leaf (RFL), removal of all leaves (RAL), and removal of the upper half of the spike (RHS) treatments in irrigation (I) and rainfed (R) conditions.
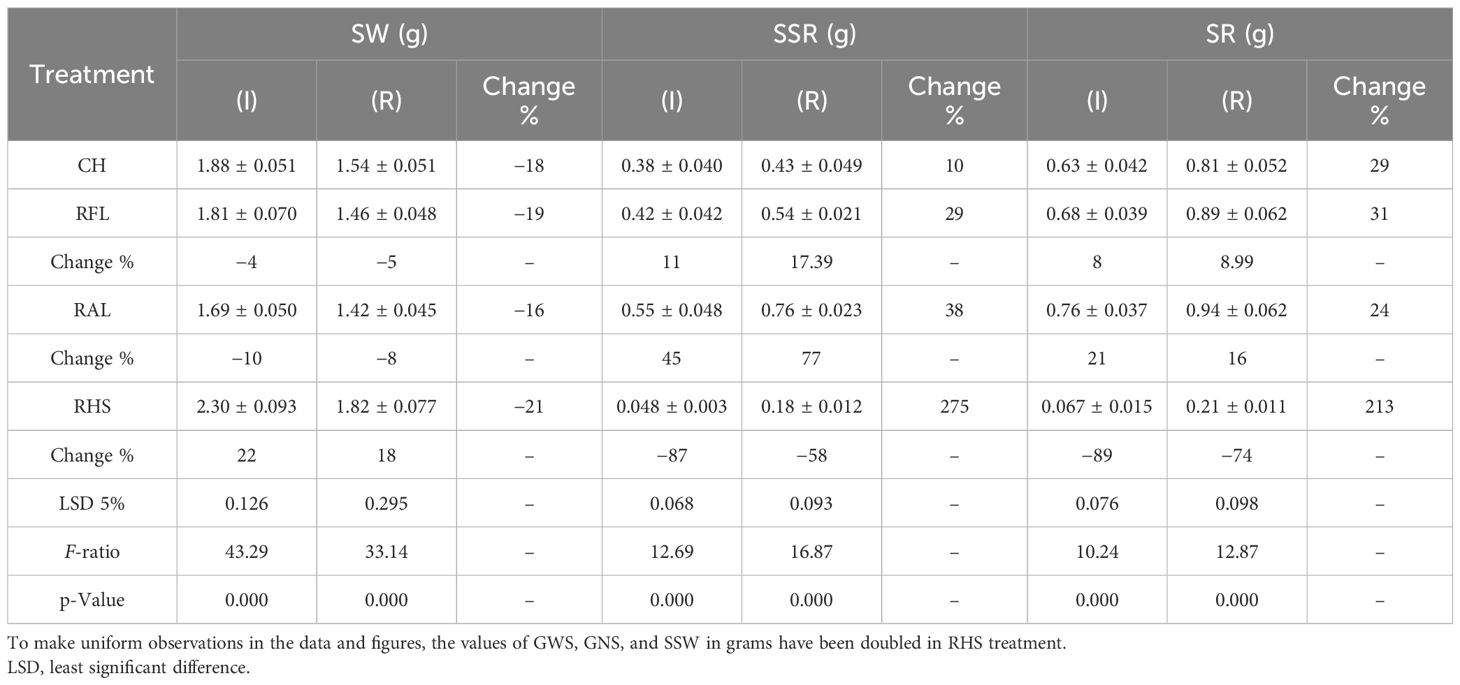
Table 6B The mean comparisons of stem weight (SW), spike straw remobilization (SSR) and stem remobilization (SR) traits under check (CH), removal of flag leaf (RFL), removal of all leaves (RAL), and removal of the upper half of the spike (RHS) treatments in irrigation (I) and rainfed (R) conditions.
3.3 Source and sink S-S limitation
Some levels of source limitation were observed from 8.18% to 10.25% on average in all genotypes. Genotypes showed different reactions to defoliation levels (reduction of source strength) in GWS in both experiments. Defoliation treatments caused a significant decrease in GWS, and GWS decreased by 18% under drought stress in rainfed conditions (Table 6; Figure 5A). In the RFL treatment, the reduction of GWS was 8% and 7% in irrigation and rainfed conditions, respectively. Also, genotypes showed different reactions to the RFL, so genotypes 1 and 10 in irrigation conditions and 4 and 11 in rainfed conditions showed the highest reaction (Table 7). Genotypes 1, 5, and 6 in both irrigation and rainfed experiments showed the highest reaction to the RHS treatment, which indicates the amount of source limitation (SL) and the insufficiency of photosynthetic materials in grain-filling duration. High-yielding genotype 1, with 24% SL in irrigation conditions, showed the highest SL among the genotypes (Table 7). The same situation was observed under drought stress; genotypes 1 and 10 had the highest SL and grain yield in rainfed conditions at 31% and 14%, respectively (Table 7). Also, lines 3 and 7 showed the least response to sink reduction in both irrigation and rainfed conditions, which indicates the relative limitation of the sink in them (Table 7). In this research, a significant positive relationship was observed between SL and GY (kg/ha) in irrigation (r = 0.647*) and rainfed (r = 0.702*) conditions and also with GNS in rainfed conditions (r = 0.692*). Furthermore, the positive relationship between SL and GWS is significantly known in irrigation (r = 0.658*) and rainfed (r = 0.632*) conditions (Table 5).
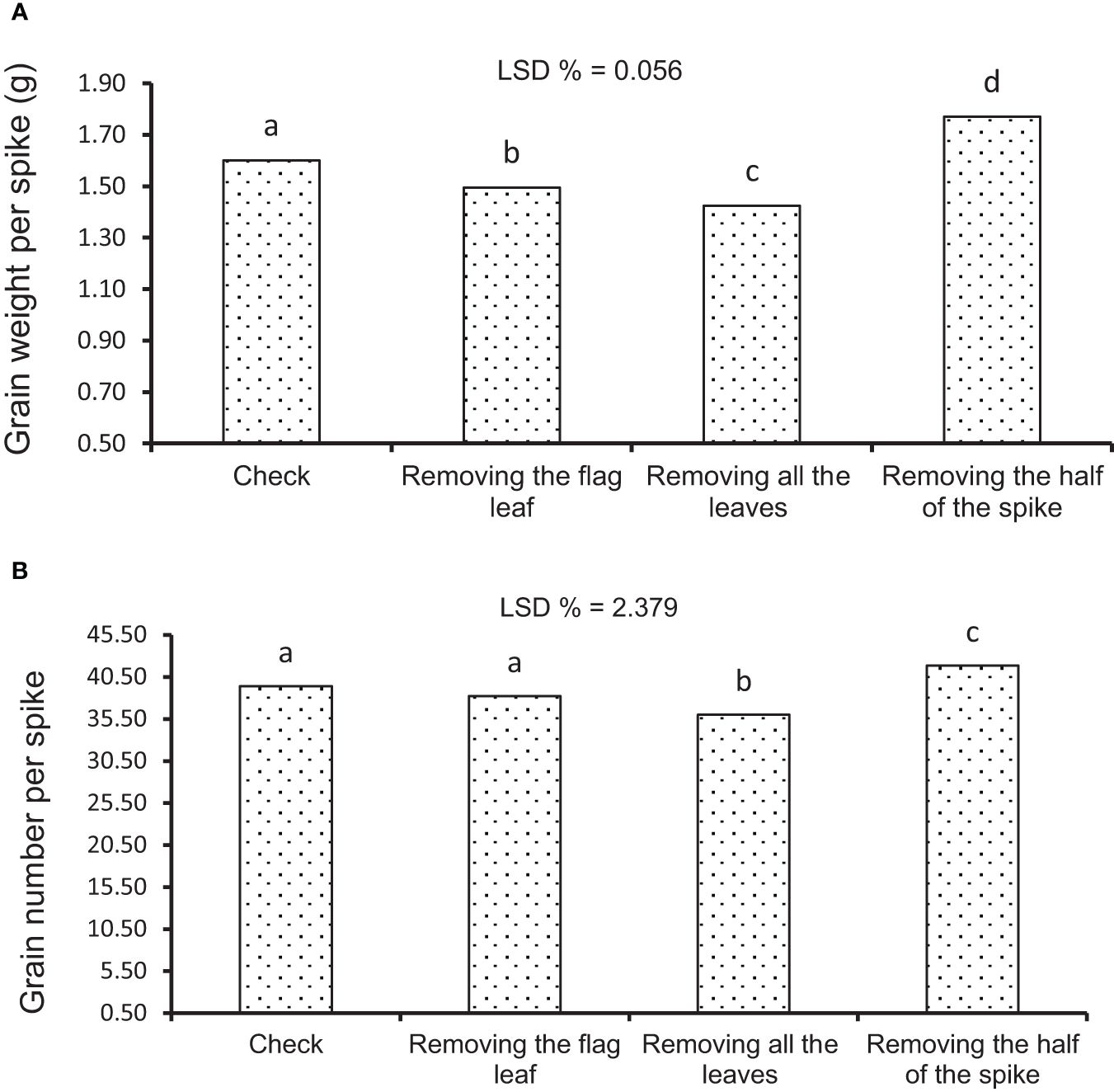
Figure 5 Mean comparison of source–sink treatments under rainfed conditions. (A) Average of grain weight per spike. (B) Average of grain number per spike. Means followed by the same letter within a column are not significantly different at the 5% probability level according to the least significant difference test.
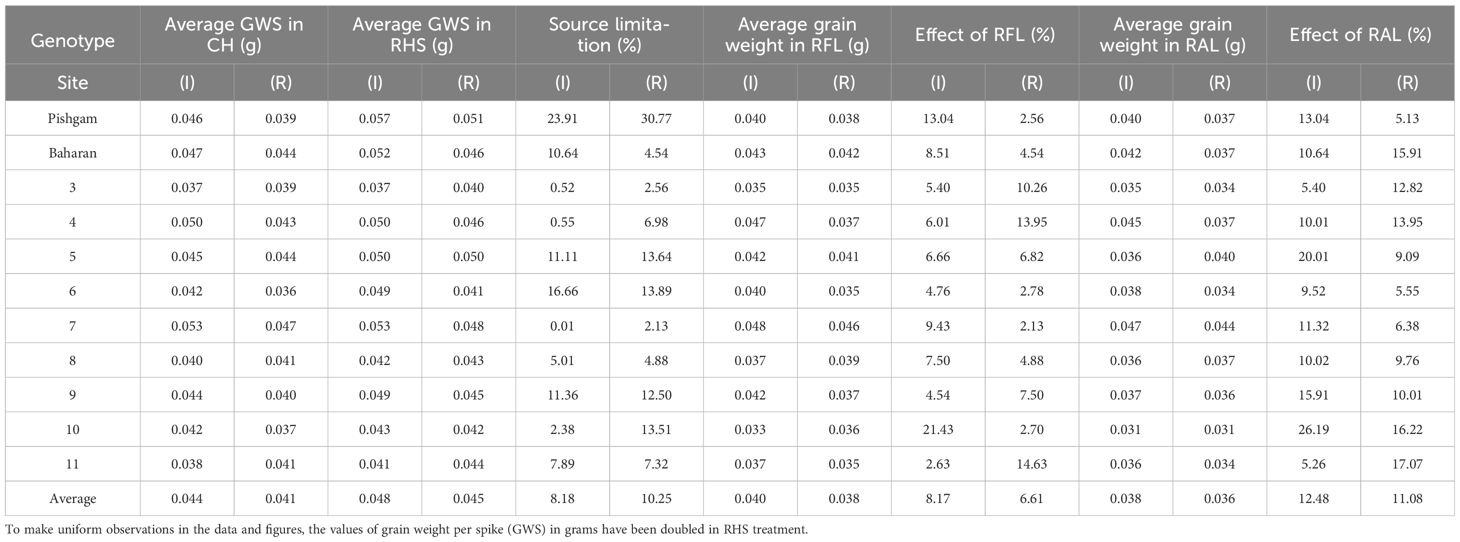
Table 7 Average grain weight per spike (GWS) in check (CH), removal of the upper half of the spikes (RHS), removal of flag leaf (RFL), removal of all leaves (RAL), and source limitation (SL) based on average grain weight per spike (GWS) in RHS treatment compared to check in irrigation (I) and rainfed (I) experiments.
3.4 Stem weight
In the present work, the effects of genotype and defoliation intensities on SW were significant under irrigation and rainfed conditions (data not shown). The SW decreased in RFL and RAL treatments (reduction of source strength), while it increased in RHS treatment (reduction of sink strength) under irrigation and rainfed conditions (Figures 6A, B, 7A, B). Also, the increase in stem remobilization affected by source reduction was calculated by measuring the stem dry weight at maturity in defoliation treatments compared to the check (Table 8). In this regard, the remobilization values from stem to grain in the check (without S-S manipulation) were 0.63 and 0.81 g/stem in irrigation and rainfed conditions, respectively, which were equivalent to 32% and 51%, respectively, of grain weight (Table 4). The increase in stem remobilization (with S-S manipulation) compared to that in the check was 8% (RFL) and 21% (RAL) in irrigation conditions, which was equivalent to 3% and 7% of grain weight, while in rainfed conditions, the increase was 9% (RFL) and 16% (RAL), which was equivalent to 4% and 7% of grain weight, respectively (Table 6).
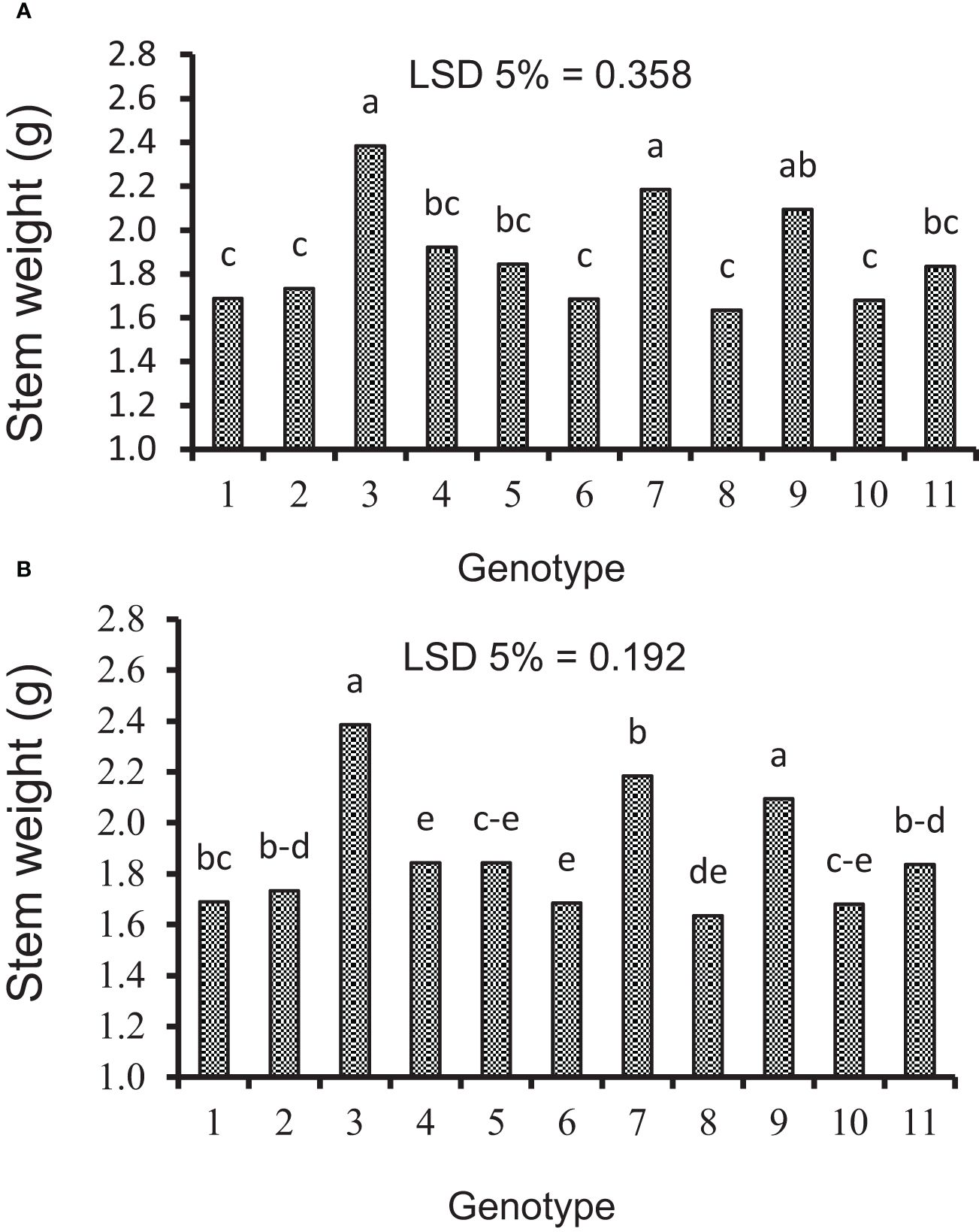
Figure 6 Mean comparison of genotypes for stem weight under (A) irrigation and (B) rainfed conditions. Means followed by the same letter within a column are not significantly different at the 5% probability level according to the least significant difference test.
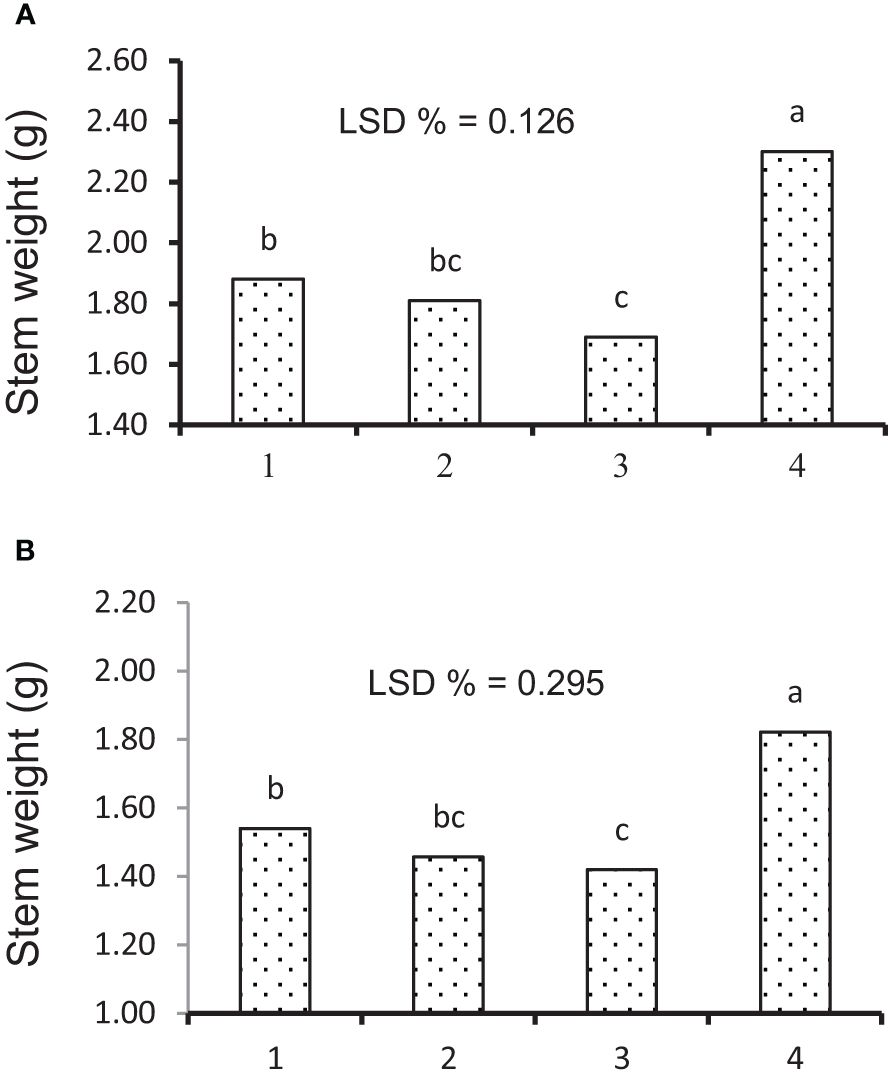
Figure 7 Mean comparison of source–sink treatments for stem weight under (A) irrigation and (B) rainfed conditions. 1 = check; 2 = removing the flag leaf; 3 = removing all the leaves; 4 = removing half of the spike. Means followed by the same letter within a column are not significantly different at the 5% probability level according to the least significant difference test.
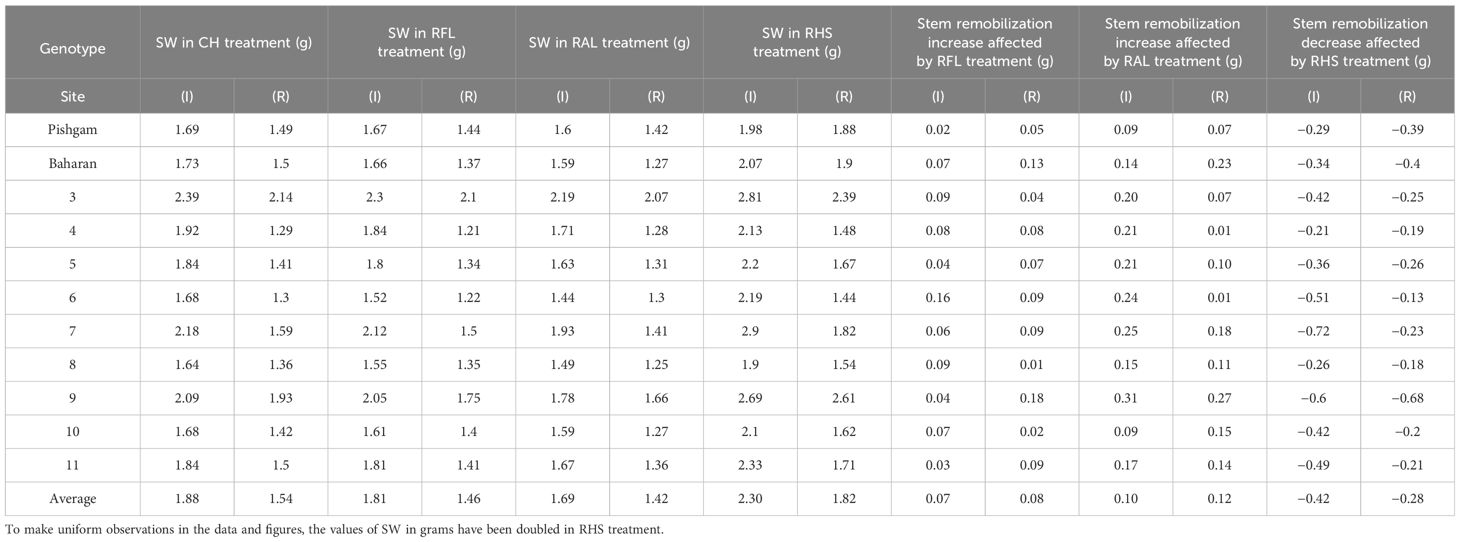
Table 8 Stem weight (SW) in check (CH), removal of flag leaf (RFL), removal of all leaves (RAL) and removal of the upper half of the spike (RHS) treatments, stem remobilization increase affected by RFL and RAL treatments, and stem remobilization decrease affected by RHS in grams (g) in irrigation (I) and rainfed (R) experiments.
3.5 Spike straw weight
In this study, the effects of genotype and defoliation intensities on SSW were significant under irrigation and rainfed conditions (data not shown). The SSW decreased in RFL and RAL treatments (reduction of source strength) while increasing in RHS treatment (reduction of sink strength) under irrigation and rainfed conditions (Figures 8A, B). The remobilization values from spike straw to grains in check (without S-S manipulation) were 0.38 and 0.43 g/spike in irrigation and rainfed conditions, respectively, equivalent to 20% and 27% of grain weight (Table 4). SSW decreased with RFL, and this reduction was more intense in RAL. This reduction means that the increase in remobilization from spike straw to grains was 0.04 and 0.05 g/spike in RFL under irrigation and rainfed conditions, respectively, and 0.08 and 0.09 g/spike in RAL, respectively (Table 9). The role of this increase in remobilization under RFL was 2% and 2.5% in grain yield per spike in irrigation and rainfed conditions, respectively, and in RAL, it was 4% and 6%, respectively. Removing a part of the spike and reducing sink size reduced the need for photosynthetic materials and remobilization value from the spike straw to the grains. The increase in the GWS under the influence of RHS was 0.17 and 0.16 g/spike in irrigation and rainfed conditions, respectively (Table 6).
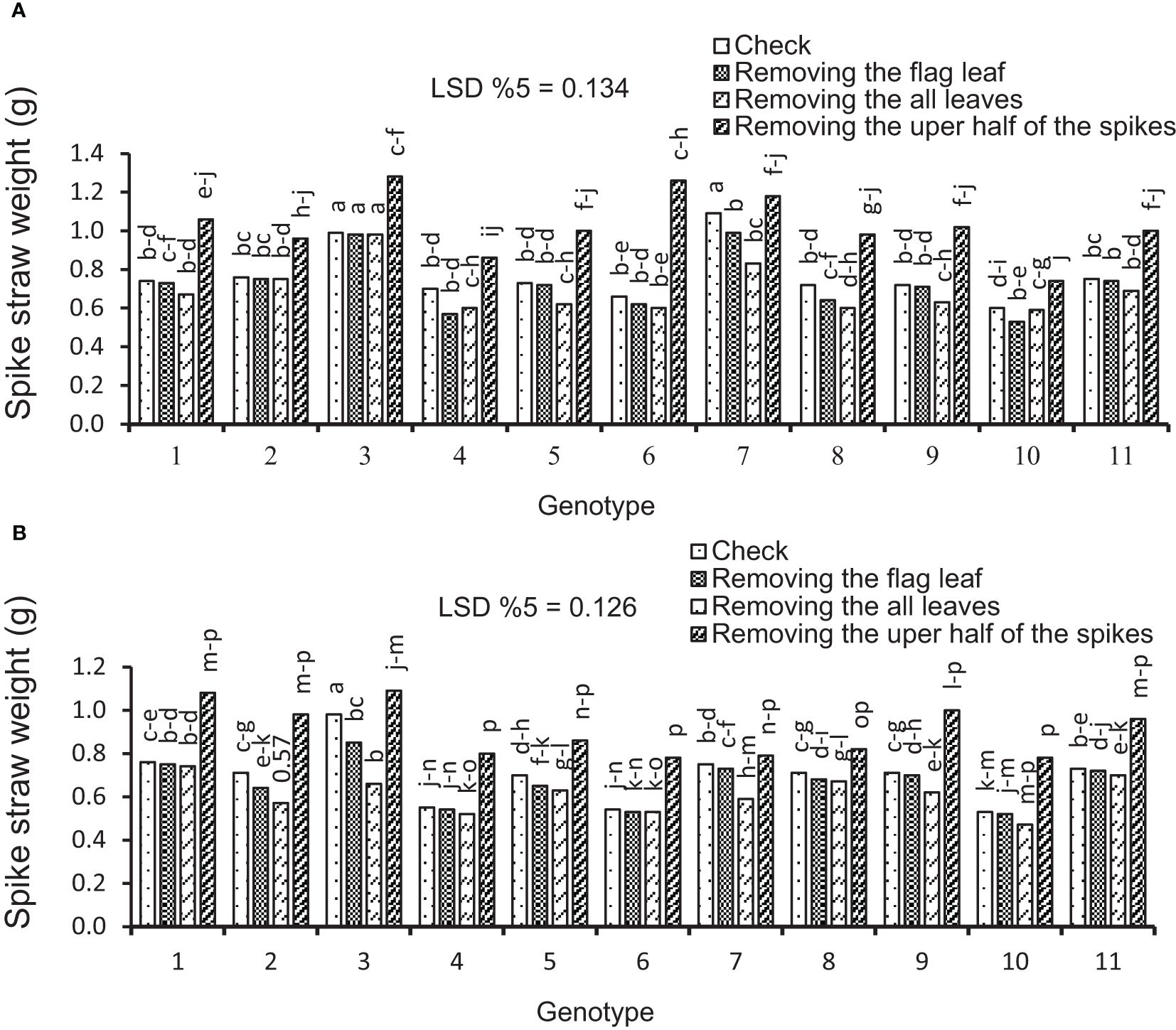
Figure 8 Mean comparison for interactions of genotypes × source–sink manipulation treatments including i) check, ii) removal of flag leaf, iii) removal of all leaves, and iv) removal of the upper half of the spikes. (A) Average spike straw weight under irrigation conditions. (B) Average spike straw weight under rainfed conditions. Means followed by the same letter within a column are not significantly different at the 5% probability level according to the least significant difference test.
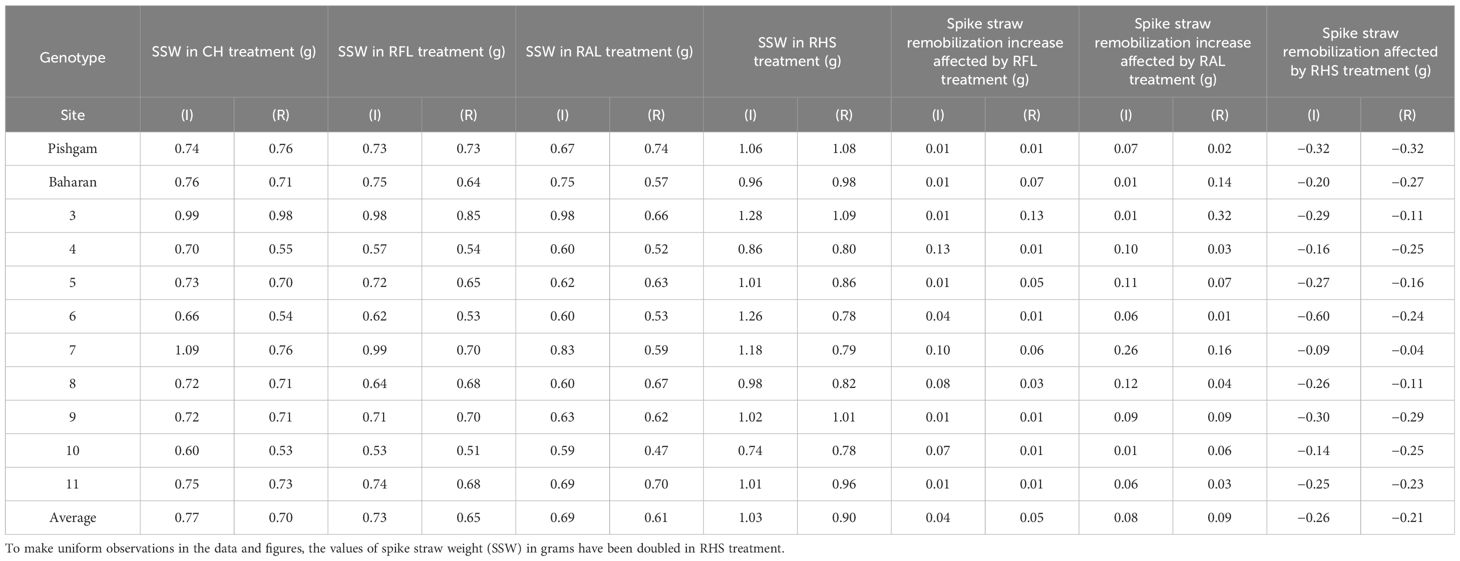
Table 9 Spike straw weight (SSW) in check (CH), removal of flag leaf (RFL), removal of all leaves (RAL) and removal of the upper half of the spikes (RHS) treatments, spike straw remobilization increase affected by RFL and RAL treatments, and spike straw remobilization decrease affected by RHS treatment in grams (g) in irrigation (I) and rainfed (R) experiments.
3.6 The compensatory role of vegetative organs in grain filling
In our study, under irrigation conditions, by RFL treatment, GWS, SW, and SSW decreased by 0.16, 0.07, and 0.04 g per plant, respectively (Table 6), equivalent to 0.27 g per plant of photosynthesis reduction. The decrease in the SW and SSW by 0.11 g per plant means an increase in the remobilization from the stem and spike straw to the grains by 0.11 g per plant, and although the photosynthesis decreased by 0.27 g per plant, grain weight decreased by only 0.16 g. Therefore, the increase in remobilization from the stem and spike straw was compensated by 41% of the decrease in photosynthesis caused by RFL in irrigation conditions. In rainfed conditions, due to the reduction of 0.11, 0.08, and 0.04 g per plant in the GWS, SW, and SSW, respectively, the role of remobilization from vegetative organs in compensating for the decrease in photosynthesis was equal to 52%. Also, under irrigation conditions, in RAL treatment, GWS, SW, and SSW decreased by 0.24, 0.19, and 0.08 g per plant, respectively, which was equivalent to 0.51 g per plant, reducing photosynthesis. The increase in remobilization from the stem and spike straw to the amount of 0.27 g caused the grain weight to decrease by only 0.24 g. Therefore, remobilization equivalent to 53% photosynthesis reduction due to the RAL was compensated in irrigation conditions. Hence, the compensatory effect of increasing remobilization under stress conditions due to the decrease of 0.17, 0.12, and 0.09 g per plant in GWS, SW, and SSW, respectively, was 56% (Table 6). Furthermore, under irrigation conditions, by RHS, grain weight in the remaining half of the spike should have been halved and reduced from 1.94 g to 0.97 g, but it reached 1.06 g. This means that 0.09 g of material was stored. However, SW and SSW in this treatment increased by 0.51 g, and GWS, SW, and SSW increased by 0.60 g per plant. In rainfed conditions, the grain weight in the half spike in the intact plant was 0.8 g, but by RHS, the GWS in the remaining half of the spike reached 0.89 g. Also, SW and SSW increased by 0.48 g per plant, while GWS, SW, and SSW increased by 0.57 g per plant. Therefore, the production of photosynthetic materials decreased by only 0.23 g per plant, equivalent to 29% of the weight of the half spike (demand reduction). Results in this study showed a significant positive relationship between stem remobilization with the GWS (r = 0.699*) and the GNS (r = 0.657*) as well as a significant positive relationship between the spike straw remobilization with the GWS (r = 0.605*) and the GNS (r = 0.607*) in rainfed conditions (Table 5). Moreover, a significant positive relationship (r = 0.684*) was observed between the remobilization of the stem under irrigation conditions and source limitation (Table 5).
3.7 Remobilization of water-soluble carbohydrates in the stem
The obtained results in this study revealed the substantial genetic variations of the WSC remobilization and efficiency from the stems (Table 10). This is consistent with the findings of other studies (Ehdaie et al., 2006a; Ehdaie et al., 2006b; Vosoghi Rad et al., 2022), which, accordingly, corroborate the manipulation of this trait in wheat breeding programs. Accordingly, depending on the cultivars and the environmental conditions (irrigation and rainfed), the amount of WSC content at 10 days after anthesis was estimated at 61 to 117 and at maturity at 23 to 58 g/m2. Also, the average values of WSC remobilization in irrigation and rainfed conditions were estimated at 52.85 and 47.66 g/m2, respectively. The average WSC remobilization in all genotypes in irrigation and rainfed conditions was 52.85 and 47.66 g/m2, respectively. Furthermore, the average remobilization efficiency (%) in irrigation and rainfed conditions was 53.10% and 59.18%, respectively, which increased by 11.45% in rainfed conditions (Table 10). Also, the contribution of WSC remobilization in grain yield (%) in rainfed conditions has increased by 19% compared to that in irrigation conditions. Results show the effect and importance of WSC remobilization in drought stress conditions. No strong correlation was observed between the above traits and grain yield.
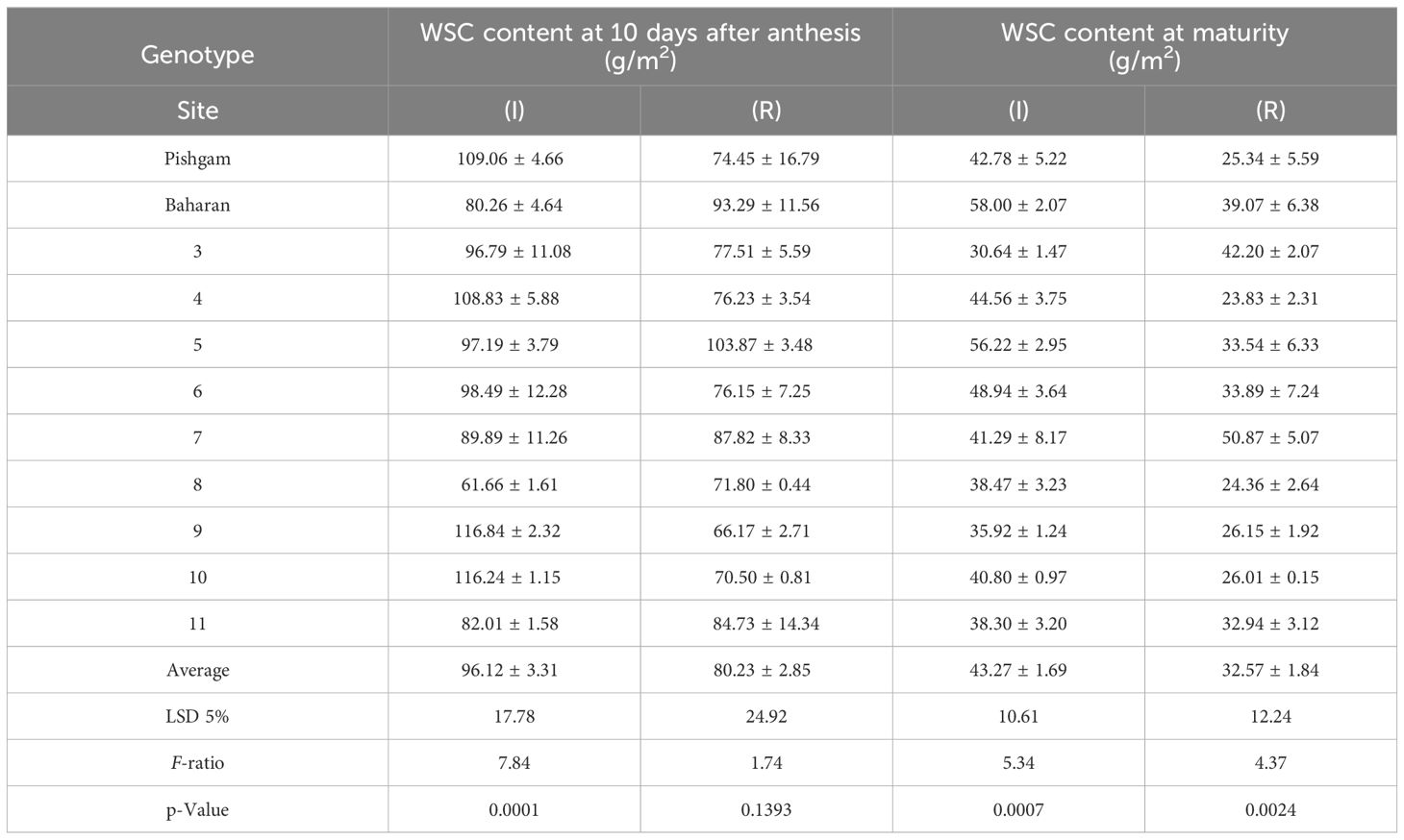
Table 10A Means of the WSC content at 10 days after anthesis and maturity (g/m2) under irrigation and rainfed conditions.
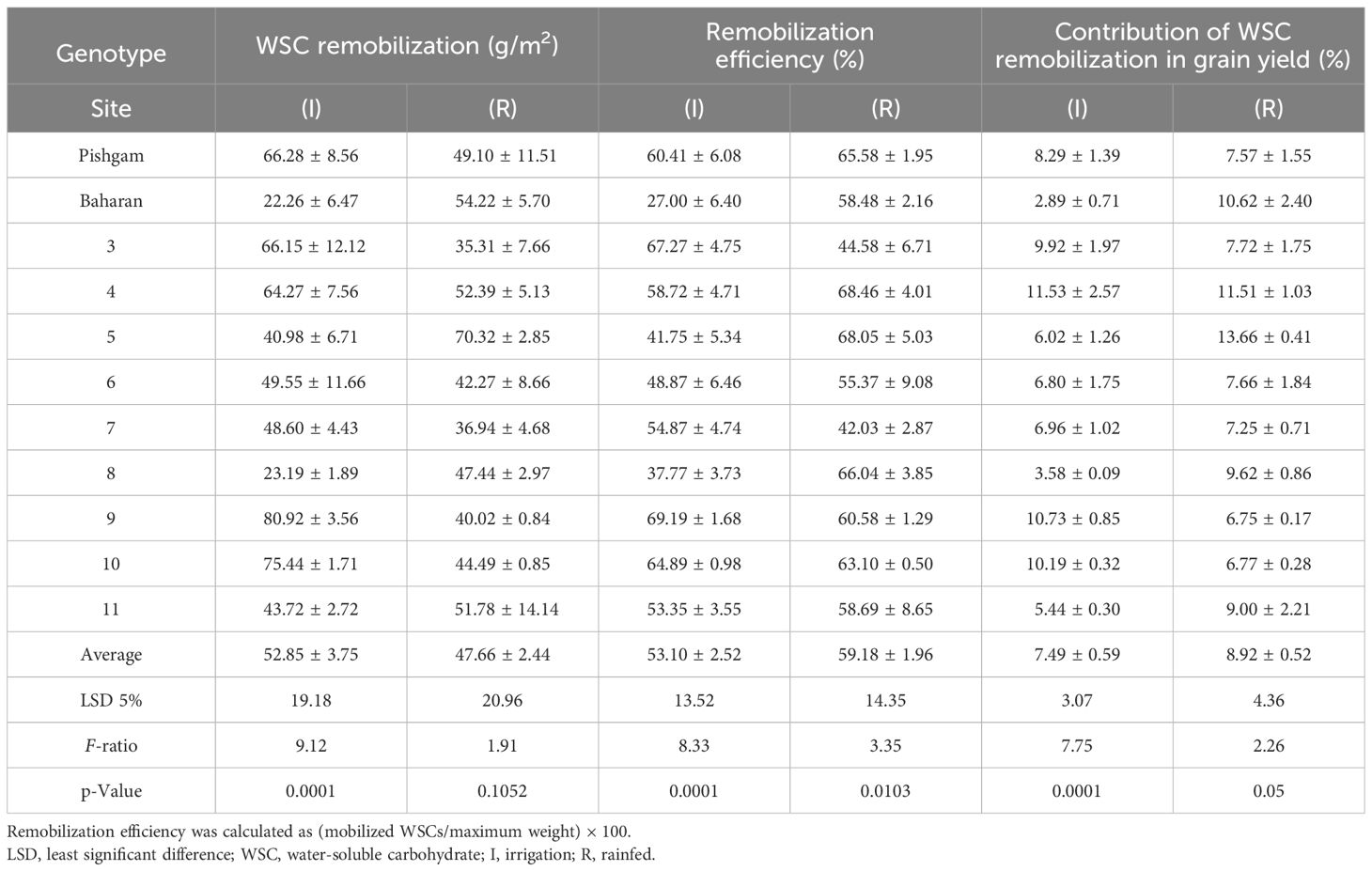
Table 10B Means of the WSC remobilization (g/m2) of the stem, remobilization efficiency (%), and contribution of WSC remobilization in grain yield (%) under irrigation and rainfed conditions.
3.8 Chlorophyll
The results showed that in both conditions, the effect of genotype on chlorophyll a, chlorophyll b, total chlorophyll, and carotenoids was significant (data not shown), and there was a significant difference between the genotypes (Table 11). Also, drought stress caused a decrease in chlorophyll contents so that the values of chlorophyll a, chlorophyll b, total, and carotenoids in all genotypes decreased to 20.7%, 24.10%, 21.02%, and 16%, respectively (Table 11; Figures 9A–D). In addition, the values of chlorophyll and carotenoids had a significant positive correlation with grain yield (ha−1) in both irrigation and rainfed conditions. In this regard, genotypes 1, 2, 6, 9, and 10 with the highest grain yield (ha−1) had the highest amounts of chlorophyll. Also, the values of chlorophyll b showed a significant positive relationship (r = 0.648*) with source limitation in irrigation conditions (Table 5).
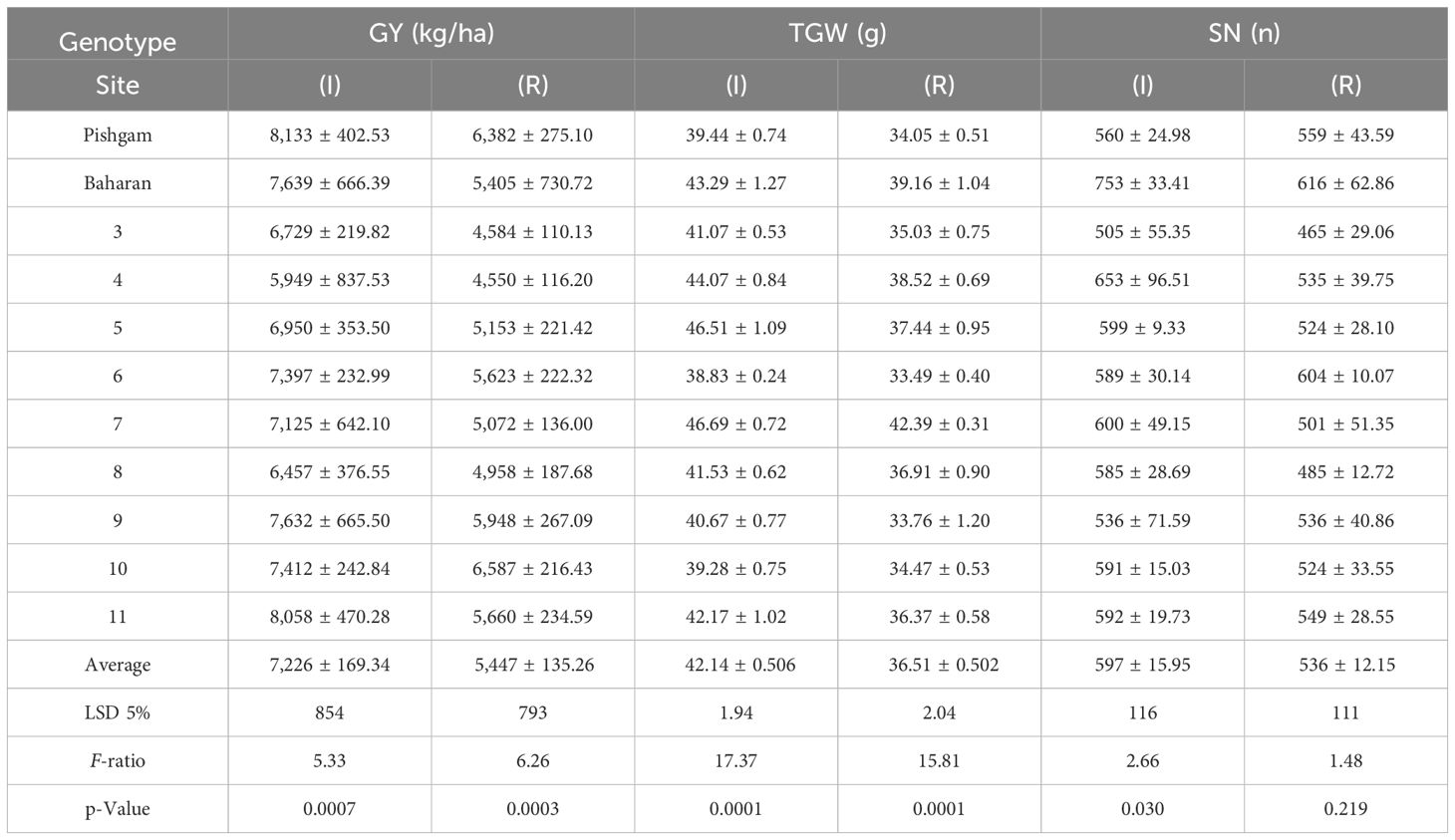
Table 11A The mean comparisons of grain yield (GY), thousand-grain weight (TGW), and spike number per m2 (SN) in irrigation (I) and rainfed (R) conditions in second year of experiment.
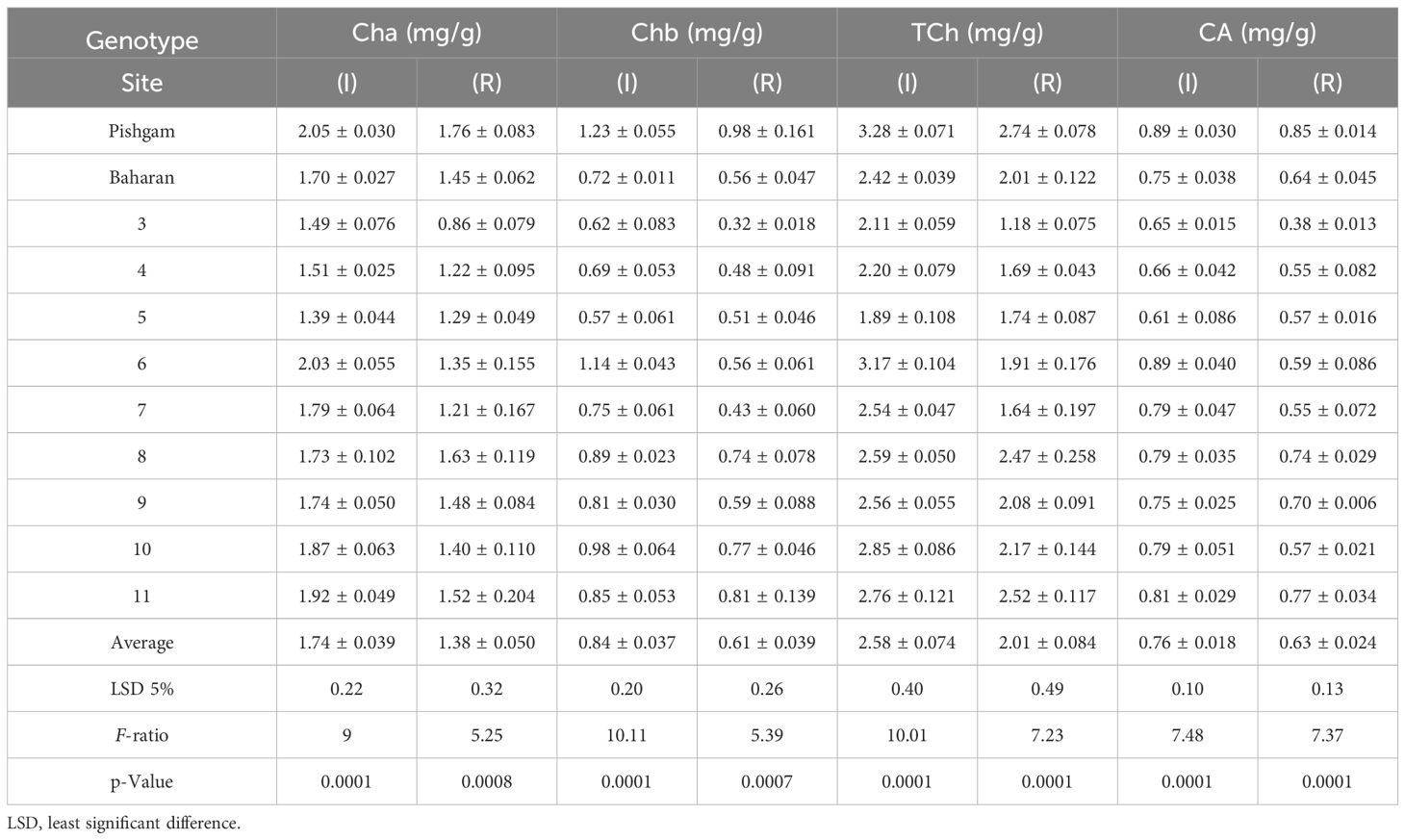
Table 11B The mean comparisons of chlorophyll a (Cha), chlorophyll b (Chb), total chlorophyll (TCh), and carotenoids (CA) in irrigation (I) and rainfed (R) conditions in second year of experiment.
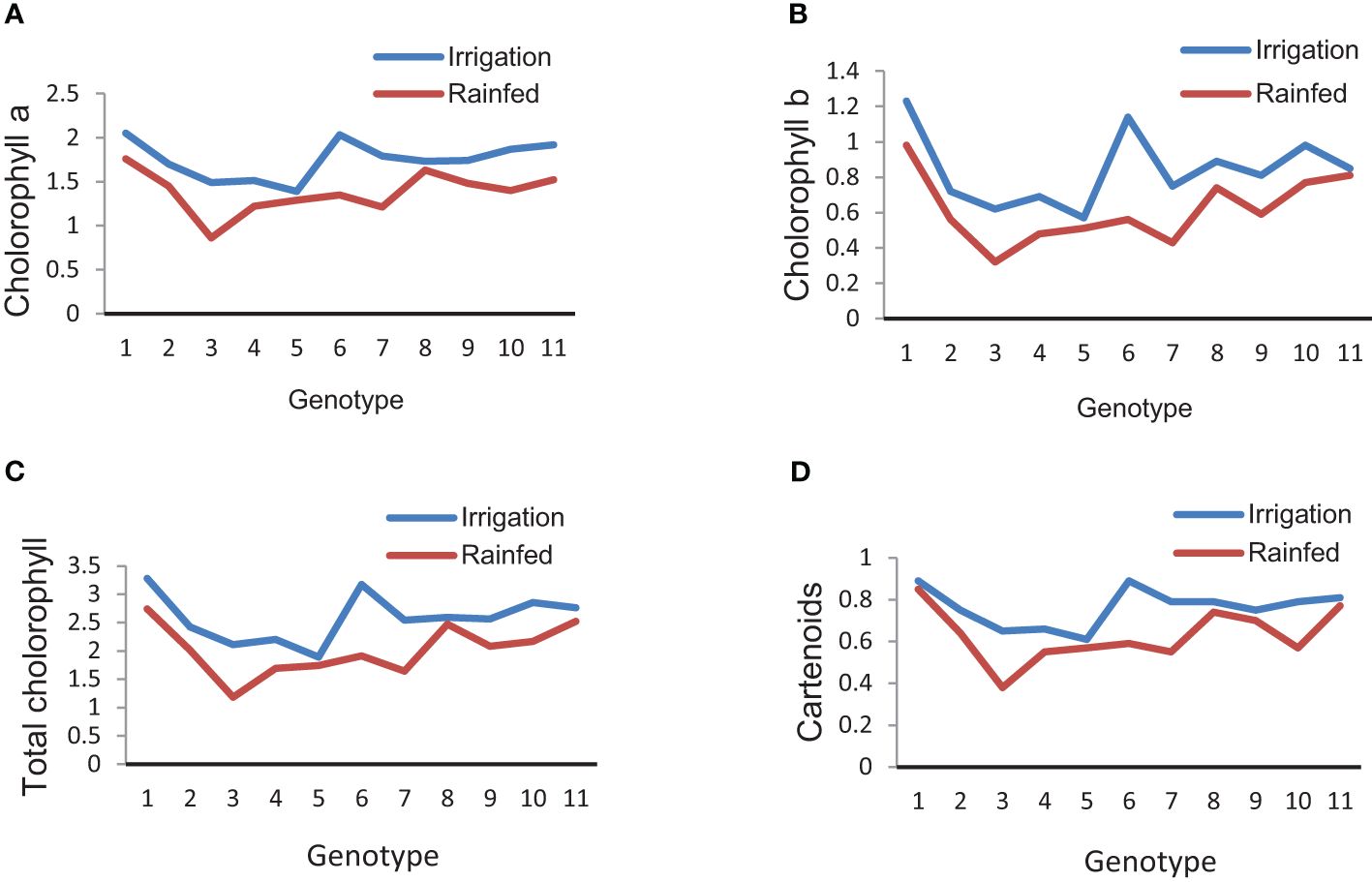
Figure 9 Mean comparison for interactions of genotypes × chlorophyll. (A) Average of chlorophyll a under irrigation and rainfed conditions. (B) Average of chlorophyll b under irrigation and rainfed conditions. (C) Average of total chlorophyll under irrigation and rainfed conditions. (D) Average of carotenoids under irrigation and rainfed conditions.
4 Discussion
4.1 Relationships between source–sink affected by RFL, RAL, and RHS treatments
As expected, grain yield was closely related to GNS. The results showed that lines with more GNS had higher GWS and higher grain yield (per ha−1). Grain yield is strongly related to the number of grains harvested at physiological maturity (Peltonen-Sainio et al., 2007; Fischer, 2008). Although grain number is the dominant component of grain yield determination, it is evident that for any given number, there is a wide range of achievable yield due to variations in grain weight (Slafer et al., 2014). Drought stress caused a significant decrease in GNS (12%) and GWS (18%) compared to irrigation conditions (Table 4). The grain weight of Ghods wheat cultivars significantly decreased under drought stress (Ahmadi et al., 2009). In RFL, the average decrease in GNS was 8% and 3% in rainfed and irrigation conditions, respectively, and in RAL, it was 17% and 9%, respectively (Table 6). Considering that leaf removal treatments were applied 2 weeks after anthesis, and at this stage, pollination and grain number were not affected, it can be said that the decrease in grain number was due to the abortion of grains or the formation of tiny grains that were not considered in the counting process. It has been reported that the removal of all leaves partially reduced the grain number of wheat by 3% to 6% (Zhenlin et al., 1998). In a study with 20 cultivars and lines of wheat, it was shown that GNS was significantly reduced by the removal of all leaves after pollination (Alam et al., 2008). Also, the RHS in some lines (lines 1, 6, and 9) increased the number of the grains in the remaining half of the spike by 4% and 6% in irrigation and rainfed, respectively (Table 6), which seems to be due to competition reduction between grains in absorbing assimilation and better growth of grains and prevention of grain abortion.
The contribution rate of flag leaves to daily photosynthetic products varies from 50% to 60% (Towfiq et al., 2015), while its defoliation generated grain yield losses of 18% to 30% (Ma et al., 2021). It has been reported that different intensities of leaf removal in the beginning stage of sink capacity formation cause a significant reduction in grain weight in different wheat cultivars (Bijanzadeh and Emam, 2010), and through the reduction in photosynthesis, it causes a decrease in grain yield (Albacete et al., 2014). Singh and Singh 1992) showed that source restriction reduced the 30% to 40% yield of wheat cultivars. Bijanzadeh and Emam (2010) announced that in the Shiraz cultivar, defoliation of all leaves decreased the main shoot yield by 40.75%. This demonstrated that the Shiraz cultivar was sensitive to source restriction under well-watered conditions. Generally, genetic diversity was observed among wheat cultivars when they were imposed on source restriction and drought stress.
It has been stated that the removal of all leaves and flag leaves caused a 28% and 17% decrease in grain weight per spike, respectively (Alam et al., 2008), which indicates the limitation of the source strength. In this study, it seems that in lines 1 and 10 under irrigation conditions, which had more yield and more oversized sink, under the influence of RFL, the limitation of photosynthetic materials in them increased, and yield reduction has been more severe. In addition, lines 3 and 4, which had lower grain yield potential, showed less sensitivity to source reduction probably due to relative sink limitation (Figures 3A, B; Tables 4, 6, 11). Therefore, the flag leaf is decisive in the S-S relationship. Researchers observed a 7%–9% decrease in grain weight, 10.7% in grain yield, and 11.1% in GNS during an experiment by removing the flag leaf after spike formation (Sharma et al., 2003). In the RAL, the average decrease in GWS compared to check was 12% and 11% in irrigation and rainfed conditions, respectively, and genotypes 3 and 11 in irrigation conditions and genotypes 6 and 7 in rainfed showed the least reaction to the RAL, which was probably due to the low source limitation and the relative sink limitation in them (Tables 6, 7). Therefore, considering that source reduction in most of the studied lines caused a significant reduction in grain weight per spike, these genotypes have some source limitations (Table 7). The role of RAL in the reduction of GWS was stronger than that of RFL which was calculated through Equation 4. However, no significant difference was observed in most of the genotypes, which shows the importance and role of the flag leaf in photosynthesis and the production of assimilates (Table 7). Investigating S-S relationships in more than 150 new bread wheat genotypes showed that all genotypes have some source limitation in sink capacity levels (Alonso et al., 2018). In general, the results showed that the leaves had a lesser role in grain filling in rainfed conditions, and grain filling under drought stress is more dependent on the remobilization of photosynthetic materials. Moreover, results showed an increase in GWS in the RHS treatment, which is interpreted as source limitation. Of course, these results indicate the limitation of the sink because, with the doubling of available photosynthetic materials under RHS treatment, the grain weight in the remaining half of the spike increased by only 10% on average. There is a significant positive relationship between source limitation and grain yield (kg/ha) in both irrigation (r = 0.647*) and rainfed (r = 0.702*) conditions, according to the results of Modhej (2011) (Table 5).
Hence, it can be said that in genotypes with higher grain yield (kg/ha), more GNS, or higher GWS (due to the more oversized sink), photosynthetic material limitation is more severe in them, and as a result, the reduction of grain yield in these genotypes under defoliation treatments will be higher. All of these genotypes are included in the category of limited source (Table 7). In this regard, it has been reported that the genotypes with a more oversized sink (grain number) will have more source limitations due to the increased competition of the sinks to receive photosynthetic materials (Satorre and Slafer, 2000). Some researchers have stated that due to the role of sources during the grain-filling period, genotypes that have less source limitation under normal and drought stress conditions have a higher genetic potential for grain yield (Janmohammadi et al., 2010). However, despite this issue, the investigated genotypes in this experiment, which are from the advanced lines resulting from the breeding experiments of Icarda and Simit, had a relative source limitation due to favorable sink potential. The studies conducted on improved wheat cultivars for different regions show that the source limitation has increased during the improvement programs in the direction of more grains and more yield in wheat and that the newly modified varieties of wheat have source limitations due to the increase in sink strength and related traits such as more oversized spikes and more GNS (Koshkin and Tararina, 2003; Alonso et al., 2018; Kuzay et al., 2019).
In recent research, the understanding of the genetic basis of source-related traits has been emphasized because the increase of spikelets in the spike can lead to more yield only when the source is adapted to the rise in the sink at the same time (Kuzay et al., 2019). It has been reported that defoliation reduces both the traits of grain growth rate and grain weight of cultivars. However, the relative reduction for sensitive cultivars to spike removal (limited source cultivars) is greater than that for insensitive cultivars (Abdoli and Saeidi, 2013). Complementary crosses between genotypes with high sink capacity and those with high source capacity resulted in progeny with substantial yield improvement (Reynolds et al., 2017), suggesting the co-limitation of S-S on yield. It has been suggested that selecting crossing partners based on physiological traits is a promising strategy to achieve higher crop productivity through breeding, which is facilitated by the increasingly automated phenotyping techniques (Reynolds et al., 2017; Furbank et al., 2019). The co-limitation of S-S implies that their breeding progress should be achieved parallelly. However, the interactions of source characteristics with the sink traits and thereby their role in the breeding progress of winter wheat are unclear, especially for the capacity of the canopy to stay green (Jagadish et al., 2015).
4.2 Remobilization of stem and spike straw under RFL, RAL, and RHS treatments and the compensatory role of vegetative organs in grain filling
In the grain-filling duration, the current photosynthesis is not enough for the grain’s needs, so grains rely on the remobilization of stem storage materials. In this sense, remobilization is one of the plant’s compensatory processes in facing the weakness of source strength, especially under drought stress. This situation may occur under source limitation conditions caused by defoliation. In wheat, the defoliation of the flag leaf blade increased the contribution of assimilates to the grain from the stem and the chaff under normal conditions (Alvaro et al., 2008), and the removal of these affected the grain yields under normal or water-limiting conditions (Cruz-Aguado et al., 1999). In this study, the RFL treatment caused a significant decrease in SW compared to the check, as well as in RAL; the reduction in SW and the increase in remobilization to grains were intensified (Table 8). Leaf removal treatment increases the remobilization of non-structural carbohydrates in the stem (Noshin et al., 1996). This decrease in SW affected by reduction in photosynthesis rate stimulates compensatory mechanisms including the remobilization of storage materials from the stem, especially at higher intensities of leaf removal and in drought stress conditions. Other findings demonstrated a significant increase in the rate and efficiency of assimilate remobilization from the stem internodes under drought stress (Ma et al., 2014; Vosoghi Rad et al., 2022). Hence, more storage materials are sent from the stems to the economic sinks (grains) and, as a result, will cause a further reduction in the SW. The researchers stated that under low-to-medium conditions of source limitation, the plant resists nutrient deficiency stress by physiological mechanisms including more optimally using the reserves in the aerial organs, balancing the distribution of photosynthetic materials, and more efficiently using the remaining leaf surfaces (Lopes and Reynolds, 2010; Emam et al., 2013).
The observed diversity in remobilization values from the stems indicates that some genotypes (due to the stronger sink) send more carbohydrates to the grains by stimulating the mechanism of remobilization (Table 4). In this case, a drought-tolerant genotype had a stronger capacity for accumulation and higher remobilization efficiency of pre-anthesis stem water-soluble carbohydrate reserves under terminal drought, resulting in better grain filling and effective compensation for the loss of grain weight, especially in lower internodes (Liu et al., 2020). Saeidi et al. (2012) suggested that the amount of remobilization of storage materials through the stem nodes is higher in resistant cultivars, especially in drought stress conditions. The noteworthy point in this study was the significant increase in SW in all investigated lines in RHS treatment by 22% and 18% in irrigation and rainfed conditions, respectively. With the reduction of the physiological sinks, the current photosynthesis has provided the grain requirement, and the surplus photosynthetic materials have been stored in the stem (Table 6). For instance, genotype 9, which has the highest increase in remobilization from the stem under RAL in irrigation (0.31 g/stem) and rainfed (0.27 g/stem), was ranked second in terms of grain weight and GNS in both conditions (Table 8). This shows the compatibility and resistance of these genotypes to adverse environmental conditions. Of course, this compatibility was not observed in the high-yielding Pishgam cultivar. The amount of remobilization during environmental stress determines the final grain yield (Najafian and Shabani, 2010).
Also, by removing the leaves and reducing the source size, part of the deficit of photosynthetic materials may be compensated by increasing the remobilization from the vegetative organs such as spike straw. In our study, the remobilization values from spike straw to grains in CH (without S-S manipulation) were 0.38 and 0.43 g/spike in irrigation and rainfed conditions, respectively. Therefore, remobilization from the spike straw compared to the stem on a smaller scale can effectively fill the grains. Overall, when the photosynthetic capacity is reduced due to drought stress or source reduction (leaves), grain filling is significantly dependent on the remobilization from storage organs such as stems and spikes straw, and if the size of the sink is reduced, the survival of storage compounds increases. Removing a part of the spike (reduction of sink size) reduces the need for photosynthetic materials and remobilization from the spike straw to the grains. According to Table 9, line 3 showed the highest increase in remobilization from spike straw in RFL (0.13 g/spike) and RAL (0.32 g/spike) in rainfed conditions. It had the least effect of drought stress on GWS (8.90%) compared to irrigation conditions. This shows the important role of intensification of remobilization from the spike straw in grain filling under critical conditions (Table 4). At the same time, genotypes 1, 4, and 6, which had the lowest increase in remobilization from spike straw in both RFL and RAL treatments in rainfed conditions, were more severely affected by drought stress (Table 9). Generally, source reduction through leaf removal treatments leads to a more favorable utilization of the storage materials in spike straw. The amount of remobilization from spike straw to grains in RAL compared to RFL showed a double increase in irrigation and rainfed conditions. The remobilization from vegetative organs to grains moderates the effect of severe defoliation on grain growth (Emam and Niknejad, 2004).
It is noteworthy that the genotypes in irrigation conditions could increase the remobilization by 0.27 g per plant in RAL, but in RFL, only 0.11 g of these reserve materials was used for remobilization; therefore, it can be said that due to the energy required for decomposition and remobilization from the stem and spike straw to the grain, most of these compounds are transferred to the grain only in critical conditions.
4.3 Changes in photosynthesis under RHS treatment
Due to competition for assimilates among reproductive organs, it is an important approach to control the source–sink ratio to reduce this competition by removing the reproductive organs and consequently the plant’s reproductive potential and yield (Wu et al., 2022). In the present study, the reduction of total photosynthesis due to the halving of grain demand (under RHS treatment) was only 0.37 g instead of 0.97 g (half spike weight) per spike, indicating a negative feedback due to the saturation of photosynthetic materials in the remaining half of the spike (Table 6). This shows that photosynthesis decreased by 38%. Also, in rainfed conditions, the production of photosynthetic materials decreased by only 0.23 g per plant, equivalent to 29% of the weight of half spike (demand reduction). This indicates the presence of stronger sinks in vegetative organs compared to grains for the uptake of photosynthetic materials when the source–sink ratio increases. The source–sink relationship analysis of wheat after anthesis showed that the sink capacity affects the production and distribution of photosynthetic products, and a larger sink capacity can promote the leaf photosynthetic potential and transport of photosynthetic products to spike (Kumar et al., 2017). The amount of dry matter accumulation after anthesis may affect the grain weight, indicating that there was a feedback regulation between the sink and the source after wheat anthesis, and the source can affect the enrichment of the sink (Asseng et al., 2017; Ibrahim et al., 2021). Moreover, the distribution of assimilates was affected by the source–sink ratio. The proportion of assimilates allocated to the spike (sink) was relatively small when the source–sink ratio was large (Abeledo et al., 2020). Moreover, it shows the need to pay attention to increasing the size of the sink to improve grain yield, especially in irrigation conditions if the source is increased. Several researchers proposed analyzing the wheat yield in terms of sink capacity and the degree of sink limitation (Abbate et al., 2005; Lázaro et al., 2010; Alonso et al., 2018), finding that the source for grain filling becomes a limiting factor when the sink capacity increases. Grain yield was highly associated with sink capacity, grain number, biomass, SPAD values, and leaf area index during grain filling, indicating a higher degree of source limitation with an increase in sink capacity. Therefore, source limitation should be taken into account by breeders when sink capacity is increased, especially under non-limiting conditions (Wu et al., 2022). There was a positive relationship between the stem remobilization with the GWS (r = 0.699*) and the GNS (r = 0.657*) and also between the spike straw remobilization with the GWS (r = 0.605*) and the GNS (r = 0.607*) in rainfed conditions. This shows the role and importance of carbohydrate transfer in traits affecting yield (Table 5).
4.4 Remobilization of water-soluble carbohydrates in the stem
During the early grain filling, if the current leaf photosynthesis is unable to thoroughly meet the sink/grain demand, part of the required photo-assimilates for the grain filling would be supplied by the dry matter remobilization from the lower internodes, which have already reached their maximum weight. Also, under terminal drought stress, stem carbohydrate reserves become the major source of grain filling as leaf photosynthesis ceases (Zhang et al., 2015). Research has shown that reserve pools can potentially contribute to approximately 20% of the final grain weight and up to 50% of the grain yield under favorable conditions and drought stress during the grain-filling period, respectively (Hou et al., 2018). Also, a positive and significant correlation has been reported between grain weight per main spike and remobilization rate in wheat under terminal drought stress (Li et al., 2020).
In this study, the average remobilization efficiency (%) in irrigation and rainfed conditions was 53.10% and 59.18%, respectively, which increased by 11.45% in rainfed conditions (Table 10). Also, the contribution of WSC remobilization in grain yield (%) in rainfed conditions has increased by 19% compared to irrigation conditions. These results show the effect and importance of WSC remobilization in drought stress conditions (Table 10). This contribution of the sucrose flux from the stem to the grain seems to be more important for yield maximization under drought conditions (Joudi et al., 2012). Nevertheless, other findings demonstrated a significant increase in the rate and efficiency of assimilate remobilization from the stem internodes under drought stress (Ma et al., 2014; Vosoghi Rad et al., 2022). In addition, the amount of remobilization among the wheat population can be influenced by the amount of accumulated reserves in the stem as well as the remobilization efficiency, which, in turn, depends on the strength of the sink (Thapa et al., 2022). Hence, it can be argued that the remobilization amount and efficiency are differently influenced by the cultivar and the severity of the drought stress.
4.5 Effect of drought stress on the chlorophyll content of flag leaf
Furthermore, drought stress decreased the contents of chlorophyll in all genotypes (Table 11; Figures 9A–D). Researchers registered slight flag leaf senescence after anthesis in the optimal conditions, which can be accelerated by drought conditions (Liu et al., 2009), while others affirm that post-anthesis drought significantly accelerated chlorophyll loss (Martinez et al., 2003). Drought stress can destroy or reduce chlorophyll content and inhibit its synthesis (Hassanzadeh et al., 2009). Decreased yield in drought-sensitive genotypes might be due to a reduction in chlorophyll as well as photosynthetic parameters (Perdomo et al., 2017). Drought-tolerant genotypes retained many photosynthetic pigments under drought stress (Epee Misse, 2018). Also, it has been observed that the chlorophyll content was highly maintained during the initial grain-filling period, and their photosynthetic capacity gradually decreased after this period (Fan et al., 2021). The significant relationship between chlorophyll content with resource limitation seems to be due to stronger sinks in these genotypes.
5 Conclusion
Some levels of source limitation were observed in all genotypes, and vegetative organs seem to have a larger sink than grains to uptake photosynthetic materials when the source–sink ratio increases. However, high-yielding genotypes had more severe source limitations, and low-yielding genotypes had more relative sink limitations. Therefore, source limitation does not necessarily occur due to the smallness of the source, and in high-yielding genotypes, it may be due to the largeness of the sinks. Hence, to increase the yield potential of high-yielding cultivars, the size of photosynthetic sources and, in cultivars with lower yields, the size of sinks should be improved. Also, by RFL, increasing remobilization from vegetative organs (stem and spike straw) to grains moderates the effects of source limitation, and it compensates decrease in grain weight. This role of remobilization was more intense in RAL. Furthermore, depending on the cultivars and environmental conditions, the amount of WSC retransplantation was calculated from 22 to 81 g/m2, Accordingly, WSC remobilization in critical conditions shows stronger positive effects on grain yield. This is also recommended to be considered in physiological and molecular studies focusing on carbohydrate remobilization of wheat stems. Also, by RHS, the decrease in the production of photosynthetic materials was only equivalent to 38% and 29% of the expected values in both conditions, which shows the presence of strong sinks in vegetative organs (stem and spike straw) compared to grains, and it is necessary to pay attention to them in order to improve wheat genotypes. Taken together, investigating the S-S relationship along with the ability to remobilization in optimal and critical conditions displayed a promising perspective in decreasing growth limitations and selecting potential genotypes in wheat in temperate regions.
Data availability statement
The raw data supporting the conclusions of this article will be made available by the authors, without undue reservation.
Author contributions
KE: Data curation, Formal analysis, Writing – original draft. AS: Conceptualization, Funding acquisition, Resources, Supervision, Writing – original draft. FH: Investigation, Validation, Writing – review & editing.
Funding
The author(s) declare financial support was received for the research, authorship, and/or publication of this article. The authors are indebted to the University of Kurdistan (UOK) for the financial support given to carry out this study.
Acknowledgments
The authors sincerely thank Mosayeb Norozi and Rasol Jaliliyan of the Islamabad-e-Gharb Agricultural Research Station for their help in implementing the experiments. They also deeply thank Reza Amiri and Layegh Moradi for their help and support.
Conflict of interest
The authors declare that the research was conducted in the absence of any commercial or financial relationships that could be construed as a potential conflict of interest.
Publisher’s note
All claims expressed in this article are solely those of the authors and do not necessarily represent those of their affiliated organizations, or those of the publisher, the editors and the reviewers. Any product that may be evaluated in this article, or claim that may be made by its manufacturer, is not guaranteed or endorsed by the publisher.
References
Abbate P. E., Andrade F. H., Culot J. P., Bindraban P. S. (1997). Grain yield in wheat: effects of radiation during spike growth period. Field Crops Res. 54, 245–257. doi: 10.1016/S0378-4290(97)00059-2
Abbate P. E., Lázaro L., Montenegro A. A., Bariffi J. H., Gutheim F. (2005). “Potential yield of Argentine vs. foreign wheat cultivars,” in 7th International Wheat Conference, Mar del Plata, Bs. As, Argentina.
Abdoli M., Saeidi M. (2013). Evaluation of water deficiency at the post anthesis and source limitation during grain filling on grain yield, yield formation, somemorphological and phonological traits and gas exchange bread wheat cultivar. Albanian J. Agric. Sci. 12, 255–265.
Abdoli M., Saeidi M., Jalali-Honarmand S., Mansourifar S., Ghobadi M. E., Cheghamirza K. (2013). Effect of source and sink limitation on yield and some agronomiccharacteristics in modern bread wheat cultivars under post anthesis water deficiency. Acta Agric. Slovenica 101, 173–182. doi: 10.2478/acas-2013–0013
Abeledo L. G., Savin R., Slafer G. A. (2020). Maize senescence under contrasting source-sink ratios during the grain filling period. Environ. Exp. Bot. 180, 104263. doi: 10.1016/j.envexpbot.2020.104263
Ahmadamini T., Kamkar B., Soltani A. (2011). The effect of planting date on partitoning coefficient in sospecies of wheat. Electronic J. Crop Production 4, 131–150.
Ahmadi A., Joudi M., Janmohammadi M. (2009). Late defoliation and wheat yield: Little evidence of post-anthesis source limitation. Field Crops Res. 113, 90–93. doi: 10.1016/j.fcr.2009.04.010
Alam M. S., Rahman A. H. M. M., Nesa M. N., Khan S. K., Siddquie N. A. (2008). Effect of Source and/or Sink Restriction on the grain yield in wheat. J. Appl. Sci. Res. 4, 258–261.
Albacete A. A., Cristina M. A., Francisco P. A. (2014). Hormonal and metabolic regulation of source sink relations under salinity and drought: From plant survival to crop yield stability. Bio. Advances 32, 12–30. doi: 10.1016/j.biotechadv.2013.10.005
Alonso M. P., Abbate P. E., Mirabella N. E., Aramburu Meralos F., Panelo J. S., Pontaroli A. C. (2018). Analysis of sink/source relations in bread wheat recombinant inbred lines and commercial cultivars under a high yield potential environment. Eur. J. Agron. 93, 82–87. doi: 10.1016/j.eja.2017.11.007
Alvaro F., Royo C., García Del Moral L. F., Villegas D. (2007). Grain filling and dry matter translocation responses to source-sink modifications in a historical series of durum wheat. Crop Sci. 48, 1523–1531. doi: 10.2135/cropsci2007.10.0545
Alvaro F., Royo C., García del Moral L. F., Villegas D. (2008). Grain filling and dry matter translocation responses to source–sink modifications in a historical series of durum wheat. Crop Sci. 48, 1523.
Asseng S., Kassie B. T., Labra M. H., Amador C., Calderini D. F. (2017). Simulating the impact of source-sink manipulations in wheat. Field Crops Res. 202, 47–56. doi: 10.1016/j.fcr.2016.04.031
Bagherikia S., Pahlevani M. H., Yamchi A., Zenalinezhad K., Mostafaie A. (2017). Molecular and physiological analysis of flag leaf senescence and remobilization of assimilates in bread wheat under terminal drought stress. Agric. Biotechnol. J. 8, 1–16. doi: 10.22103/jab.2017.1630
Bijanzadeh E., Emam Y. (2010). Effect of source-sink manipulation on yield components and photosynthetic characteristic of wheat cultivars (Triticum aestivum and T. durum L.). J. Appl. Sci. 10, 564–569. doi: 10.3923/jas.2010.564.569
Bojovi´c B., Stojanovi´c J. (2005). Chlorophyll and carotenoid content in wheat cultivars as a function of mineral nutrition. Arch. Biol. Sci. 57, 283–290. doi: 10.2298/ABS0504283B
Borras L., Salfer G. A. (2004). Seed dry weight response to source-sink manipulation in wheat, maize and soybean: a quantitative reappraisal. Field Crops Res. 86, 131–146. doi: 10.1016/j.fcr.2003.08.002
CIMMYT (2017) Global Wheat Research. [2017–05-09]. Available online at: http://www.cimmyt.org/globalwheat-research.
Cruz-Aguado J., Reyes F., Rodes R., Perez I., Dorado M. (1999). Effect of source-to-sink ratio on partitioning of dry matter and 14 C-photoassimilates in wheat during grain filling. Ann. Bot. 83, 655–665. doi: 10.1006/anbo.1999.0869
Cruz-Aguado J. A., Rodes R., Perez I. P., Dorado M. (2000). Morphological characteristics and yield components associated with accumulation and loss of drymass in the internodes of wheat. Field Crops Res. 66, 129–139. doi: 10.1016/S0378-4290(00)00070-8
Du B., Liu L., Wang Q., Sun G., Ren X., Li C., et al. (2019). Identification of QTL underlying the leaf length and area of different leaves in barley. Sci. Rep. 9, 4431. doi: 10.1038/s41598-019-40703-6
Ehdaie B., Alloush G. A., Madore M. A., Waines J. G. (2006a). Genotypic variation for stem reserves and mobilization in wheat: II. Postanthesis changes in internode water soluble carbohydrates. Crop Sci. 46, 2093–2103. doi: 10.2135/cropsci2006.01.0013
Ehdaie B., Alloush G. A., Madore M. A., Waines J. G. (2006b). Genotypic variation for stem reserves and mobilization in wheat: I. postanthesis changes in internode dry matter. Crop Sci. 46, 735–746. doi: 10.2135/cropsci2005.04-0033
Emam Y., Niknejad M. (2004). An introduction to the physiology of crop yield (Shiraz, Iran: Shiraz University Press), 571.
Emam Y., Sedaghat M., Bahrani H. (2013). Responses of maize (SC704) yield and yield components to source restriction. Iran Agric. Res. 32, 31–40. doi: 10.22099/IAR.2013.1815
Epee Misse P. T. (2018). Wheat Seedling Physiological Adaptation to Overcome Water Stress (SSRN) (Elsevier BV), 2–11. doi: 10.2139/ssrn.3307427
Fan Y., Lv Z., Ge T., Li Y., Yang W., Zhang W., et al. (2021). Night-warming priming at the vegetative stage alleviates damage to the flag leaf caused by post-anthesis warming in winter wheat (Triticum aestivum L.). Front. Plant Sci. 12. doi: 10.3389/fpls.2021.706567
FAO (2021). Available online at: https://www.fao.org/faostat/en/#data.
Felekori H., EghbalGhobadi M., Ghobadi M., JalaliHonarmand S., Saeidi M. (2014). The effect of post anthesis source and sink limitation in wheat cultivars under moderate conditions. Int. J. Biosci. 5, 52–59. doi: 10.12692/ijb/5.5.52-59
Fischer R. A. (2008). The importance of grain or kernel number in wheat: a reply to Sinclair and Jamieson. Field Crops Res. 105, 15–21. doi: 10.1016/j.fcr.2007.04.002
Foulkes M. J., Slafer G. A., Davies W. J., Berry P. M., Sylvester–Bradley R., Martre P., et al. (2011). Raising yield potential of wheat. III. Optimizing partitioning to grain while maintaining lodging resistance. J. Exp. Bot. 62, 469–486. doi: 10.1093/jxb/erq300
Furbank R. T., Jimenez-Berni J. A., George-Jaeggli B., Potgieter A. B., Deery D. M. (2019). Field crop phenomics: enabling breeding for radiation use efficiency and biomass in cereal crops. J. New. Phytol. 223, 1714–1727. doi: 10.1111/nph.15817
Gao J., Zhang Y., Xu C., Wang X., Wang P., Huang S. (2023). Abscisic acid collaborates with lignin and flavonoid to improve pre-silking drought tolerance by tuning stem elongation and ear development in maize (Zea mays L.). Plant J. 114, 437–454.
Hassanzadeh H., Ebadi A., Panahyan-e-Kivi M., Eshghi A. G., Jamaati-e-Somarin Sh., Saeidi M., et al. (2009). Evaluation of drought stress on relative water content and chlorophyll content of sesame (Sesamum indicum L.) genotypes at early flowering stage. Res. J. Environ. Sci. 3, 345–350. doi: 10.3923/rjes.2009.345.350
Hou J., Huang X., Sun W., Du C., Xie Y., Ma Y. (2018). Accumulation of water-soluble carbohydrates and gene expression in wheat stems correlates with drought resistance. J. Plant Physiol. 231, 182–191.
Ibrahim H. M., Ali B., El-Keblawy A., Ksiksi T., El-Esawi M. A., Josko I., et al. (2021). Effect of source–sink ratio manipulation on growth, flowering, and yield potential of soybean. Agr. 11, 926. doi: 10.3390/agriculture11100926
Jagadish K. S. V., KaviKishor P. B., Bahuguna R. N., von Wirén N., Sreenivasulu N. (2015). Staying alive or going to die during terminal Senescence-An enigma surrounding yield stability. Front. Plant Sci. 6. doi: 10.3389/fpls.2015.01070
Janmohammadi M., Movahedi Z., Sabaghnia N. (2010). Multivariate statistical analysis of some traits of bread wheat for breeding under rainfed conditions. J. Agric. Sci. 59, 1–14. doi: 10.2298/JAS1401001J
Joudi M., Ahmadi A., Mohamadi V., Abbasi A., Vergauwen R., Mohamadi H., et al. (2012). Comparison of fructan dynamics in two wheat cultivars with different capacities of accumulation and remobilization under drought stress. Physiol. Plant 144, 1–12. doi: 10.1111/j.1399-3054.2011.01517.x
Joudi M., Ahmadi A., Poustini K., Shrifzadeh F. (2006). Effect of leaf elimination on the effectiveness of flag leaf photosynthesis and seed growth in bread wheat. Iranian J. Agric. Sci. 2, 203–211.
Khan N. A., Khan M., Ansari H. R., Samiullah H. (2002). Auxin and defoliation effects on photosynthesis and ethylene evolution in mustard. Scientia Horticulture 96, 43–51. doi: 10.1016/S0304-4238(02)00094-8
Koshkin E. I., Tararina V. V. (2003). Yield and source/sink relations of spring wheat cultivars. Field Crops Res. 22, 297–306. doi: 10.1016/0378-4290(89)90029-4
Kumar R. R., Goswami S., Shamim M. (2017). Biochemical defense response: characterizing the plasticity of source and sink in spring wheat under terminal heat stress. Front. Plant Sci. 8, 1603. doi: 10.3389/fpls.2017.01603
Kuzay S., Xu Y., Zhang J., Katz A., Pearce S., Su Z. (2019). Identification of a candidate gene for a QTL for spikelet number per spike on wheat chromosome arm 7AL by high-resolution genetic mapping. Theor. Appl. Genet. 132, 2689–2705. doi: 10.1007/s00122-019-03382-5
Lázaro L., Abbate P. E., Cogliatti D. H., Andrade F. H. (2010). Relationship between yield, growth and spike weigth in wheat under phosphorus deficiency and shading. J. Agric. Sci. 148, 83–93. doi: 10.1017/S0021859609990402
Li M., Liu Y., Ma J., Zhang J., Wang C., Su J., et al. (2020). Genetic dissection of stem WSC accumulation and remobilization in wheat (Triticum aestivum L.) under terminal drought stress. BMC Genet. 21, 50. doi: 10.1186/s12863-020-00855-1
Liu Y., Li M., Li J., Li X., Yang X., Tong Y., et al. (2009). Dynamic changes in flag leaf angle contribute to high photosynthetic capacity. Chin. Sci. Bulletin 54, 3045–3052. doi: 10.1007/s11434-009-0470-2
Liu X., Yin B., Hu Z., Bao X., Wang Y., Zhen W. (2021). Physiological response of flag leaf and yield formation of winter wheat under different spring restrictive irrigation regimes in the Haihe Plain, China. J. Integr. Agric. 20, 2343–2359. doi: 10.1016/S2095-3119(20)63352-4
Liu Y., Zhang P., Li M., Chang L., Cheng H., Chai Sh., et al. (2020). Dynamic responses of accumulation and remobilization of water soluble carbohydrates in wheat stem to drought stress. Plant Physiol. Biochem. 155, 262–270. doi: 10.1016/j.plaphy.2020.07.024
Lopes M. S., Reynolds M. P. (2010). Partitioning of assimilates to deeper roots is associated with cooler canopies and increased yield under drought in wheat. Funct. Plant Biol. 37, 147–156. doi: 10.1071/FP09121
Ma J., Huang G. B., Yang D. L., Chai Q. (2014). Dry matter remobilization and compensatory effects in various internodes of spring wheat under water stress. Crop Sci. 54, 331–339. doi: 10.2135/cropsci2013.03.0141
Ma J., Xiao Y., Hou L., He Y. (2021). Combining Protein content and grain yield by genetic dissection in bread wheat under low-input management. Foods. 10, 1058. doi: 10.3390/foods10051058
Manderscheid R., Burkart S., Bramm A., Weigel H. J. (2003). Effect of CO2 enrichment on growth and daily radiation use efficiency of wheat in relation to temperature and growth stage. Eur. J. Agron. 19, 411–425. doi: 10.1016/S1161-0301(02)00133-8
Martinez D. E., Luquez V. M., Bartoli C. G., Guiamet J. J. (2003). Persistence of photosynthetic components and photochemical efficiencyin ears of water-stressed wheat (Triticum aestivum). Physiologia Plantarum 119, 519–525. doi: 10.1046/j.1399-3054.2003.00195.x
Maydup M. L., Antonietta M., Guiamet J. J., Graciano C., Lopez J. R., Tambussi E. A. (2013). The contribution of ear photosynthesis to grain filling in bread wheat (Triticum aestivum L.). Field Crops Res. 119, 48–58. doi: 10.1016/j.fcr.2010.06.014
Modhej A. (2001). Investigating the effects of heat stress after pollination on the resource limitation of wheat and barley genotypes in Ahvaz weather conditions. Islamic Azad University, Dezful branch, 132.
Modhej M. (2011). The relationship between source and sink physiological wheat (Triticum aestivum and T.durum) and triticale (Triticale hexaploid Lart.) In Ahvaz environmental conditions. J. Agric. Res. 9, 258–264.
Najafian G., Shabani A. (2010). The effects of terminal water stress on physiological cahractersitics and sink-source relations in two bread wheat (Triticum aestivum L.) cultivars. Iranian J. Crop Sci. 12, 392–408.
Niroula A., Khatri S., Timilsina R., Khadka D., Khadka A., Ojha P. (2019). Profile of chlorophylls and carotenoids of wheat (Triticum aestivum L.) and barley (Hordeum vulgare L.) microgreens. J. Food Sci. Technol. 56, 2758–2763. doi: 10.1007/s13197-019-03768-9
Noshin B., Hac I. U., Shap P. (1996). Source reduction and comparative sink enhancement effects on remobilization of assimilates during grain filling of old and new wheat varieties. Rachis. 15, 20–23.
Ovenden B., Milgate A., Lisle C., Wade L. J., Rebetzke G. J., Holland J. B. (2017). Selection for water-soluble carbohydrate accumulation and investigation of genetic × environment interactions in an elite wheat breeding population. Theor. Appl. Genet. 130, 2445–2461. doi: 10.1007/s00122-017-2969-2
Papakosta D. K., Gayianas A. A. (1991). Nitrogen and dry matter accumulation, remobilization and losses for Mediterranean wheat during grain filling. Agrono. J. 83, 864–870. doi: 10.2134/agronj1991.00021962008300050018x
Peltonen-Sainio P., Kangas A., Salo Y., Jauhiainen L. (2007). Grain number dominates grain weight in temperate cereal yield determination: evidence based on 30 years of multi-location trials. Field Crops Res. 100 (2-3), 179–188. doi: 10.1016/j.fcr.2006.07.002
Perdomo J. A., Capó-Bauçà S., Carmo-Silva E., Galmés J. (2017). Rubisco and rubisco activase play an important role in the biochemical limitations of photosynthesis in rice, wheat, and maize under high temperature and water deficit. Front. Plant Sci. 8. doi: 10.3389/fpls.2017.00490
Reynolds M. P., Mujeeb-Kazi A., Sawkins M. (2005). Prospects for utilising plant-adaptive mechanisms to improve wheat and other crops in drought-and salinity-prone environments. Ann. Appl. Biol. 146, 239–259. doi: 10.1111/j.1744-7348.2005.040058.x
Reynolds M. P., Pask A. J. D., Hoppitt W. J. E., Sonder K., Sukumaran S., Molero G., et al. (2017). Strategic crossing of biomass and harvest index—source and sink—achieves genetic gains in wheat. Euphytica. 213, 257. doi: 10.1007/s10681-017-2040-z
Saeidi M., Moradi F., Ahmadi A., Sepehri R., Najafiyan G., Shabani A. (2011). The effect of terminal water stress on physiological cahracteristics and sink-source relations in two bread wheat (Triticum aestivum L.) cultivars. Iranian J. Field Crop Sci. 12, 392–408.
Saeidi M., Moradi F., Jalali-Honarmand S. (2012). The effect of post anthesis source limitation treatments on wheat cultivars under water deficit. Australian J. Crop Sci. 6, 1179–1187.
Saradadevi R., Palta J. A., Siddique K. H. M. (2017). ABA-mediated stomatal response in regulating water use during the development of terminal drought in wheat. Front. Plant Sci. 8. doi: 10.3389/fpls.2017.01251
Satorre H. E., Slafer G. A. (2000). Wheat, Ecology and Physiology of Yield Determination (New York. London. Oxford: Food Products Press), 503.
Serrago R. A., Alzueta I., Savin R., Slafer G. A. (2013). Understanding grain yield responses to source–sink ratios during grain filling in wheat and barley under contrasting environments. Field Crops Res. 150, 42–51. doi: 10.1016/j.fcr.2013.05.016
Sharma S. N., Sain R. S., Sharma R. K. (2003). Genetic analysis of flag leaf area in durum wheat over environments. Wheat Inf. Service 96, 5–10.
Singh D., Singh D. (1992). Effect of leaf blade and awn on grain yield of rainfed wheat (Triticum aestivum L.) at different stages of spike development. Ind. J. Agric. Sci. 62, 468–471.
Slafer G. A., Savin R., Sadras V. O. (2014). Goarse and fine regulation of wheat yield components in response to genotype and environment. Field Crops Res. 157, 71–83. doi: 10.1016/j.fcr.2013.12.004
Thapa S., Rudd J. C., Jessup K. E., Liu S., Baker J. A., Devkota R., et al. (2022). Middle portion of the wheat culm remobilizes more carbon reserve to grains under drought. J. Agron. Crop Sci. 208, 795–804. doi: 10.1111/jac.12508
Towfiq S. I., Abdulqader S. H., Ahmad K. R., Hama S. J. (2015). Response of grain yield and its components to organic matter and removal of some photosynthetic organs of durum wheat (Triticum aestivum L.) in two years of Sulaimani—Iraq region. Int. J. Plant Anim. Environ. Sci. 5, 134–140.
Vicente R., Vergara-Díaz O., Medina S., Chairi F., Kefauver S. C., Bort J., et al. (2018). Durum wheat ears perform better than the flag leaves under water stress: Gene expression and physiological evidence. Environ. Exp. Bot. 153, 271–285. doi: 10.1016/j.envexpbot.2018.06.004
Vosoghi Rad M., Jami Moeini M., Taherian M., Mohammad A. (2022). Accumulation and remobilization of assimilates in different genotypes of durum wheat under terminal drought stress. J. Crop Sci. Biotech. 25, 191–214. doi: 10.1007/s12892-021-00123-3
Wang Z., Yin Y., He M., Zhang Y., Lu S., Li Q., et al. (2003). Allocation of photosynthates and grain growth of two wheat cultivars with different potential grain growth in response to pre- and post-anthesis shading. J. Agron. Crop Sci. 189, 280–285. doi: 10.1046/j.1439-037X.2003.00041.x
White A. C., Rogers A., Rees M., Osborne C. P. (2016). How can we make plants grow faster? A source–sink perspective on growth rate. J. Exp. Bot. 67, 31–45. doi: 10.1093/jxb/erv447
Wu X. L., Liu M., Li C. S., Mchugh A. D. J., Li M., Xiong T., et al. (2022). Source-sink relations and responses to sink-source manipulations during grain filling in wheat. J. Integr. Agric. 21, 1593–1605. doi: 10.1016/S2095–3119(21)63640–7
Wych R. D., Mc Grawm R. L., Stuthman D. D. (1982). Genotype×Year interaction for length and rate of grain filling in oats. Crop Sci. 22, 1025–1028. doi: 10.2135/cropsci1982.0011183X002200050031x
Yang J., Zhang J. (2006). Grain filling of cereals under soil drying. New Phytologist 169, 223–236. doi: 10.1111/j.1469-8137.2005.01597.x
Zadoks J. C., Chang T. T., Konzak C. F. (1974). A decimal code for the growth stages of cereals. Weed Res. 14, 415–421. doi: 10.1111/j.1365-3180.1974.tb01084.x
Zhang J., Chen W., Dell B., Vergauwen R., Zhang X., Mayer J. E., et al. (2015). Wheat genotypic variation in dynamic fluxes of WSC components in different stem segments under drought during grain filling. Front. Plant Sci. 6, 624. doi: 10.3389/fpls.2015.00624
Zhang Z., Huang J., Gao Y., Liu Y., Li J., Zhou X., et al. (2020). Suppressed ABA signal transduction in the spike promotes sucrose use in the stem and reduces grain number in wheat under water stress. J. Exp. Bot. 71, 7241–7256. doi: 10.1093/jxb/eraa380
Zhenlin W., Yin Y., He M., Cao H. (1998). Source-sink manipulation effects on post anthesis photosynthesis and grain setting on spik in winter wheat. Photos. 35, 453–459. doi: 10.1023/A:1006976605148
Keywords: chlorophyll, grain yield, photosynthetic materials, remobilization, source and sink limitation, water soluble carbohydrates
Citation: Ershadimanesh K, Siosemardeh A and Hoseeinpanahi F (2024) Evaluation of source–sink manipulation through defoliation treatments in promising bread wheat lines under optimal irrigation and rainfed conditions. Front. Agron. 6:1393267. doi: 10.3389/fagro.2024.1393267
Received: 28 February 2024; Accepted: 08 May 2024;
Published: 05 June 2024.
Edited by:
Danilo Scordia, University of Messina, ItalyReviewed by:
Muhammad Ahsan Asghar, Aarhus University, DenmarkIoannis Roussis, Agricultural University of Athens, Greece
Copyright © 2024 Ershadimanesh, Siosemardeh and Hoseeinpanahi. This is an open-access article distributed under the terms of the Creative Commons Attribution License (CC BY). The use, distribution or reproduction in other forums is permitted, provided the original author(s) and the copyright owner(s) are credited and that the original publication in this journal is cited, in accordance with accepted academic practice. No use, distribution or reproduction is permitted which does not comply with these terms.
*Correspondence: Adel Siosemardeh, YTMzQHVvay5hYy5pcg==