- 1Agricultural Biotechnology Department, College of Agricultural and Food Sciences, King Faisal University, Al-Ahsa, Saudi Arabia
- 2Oasis Agriculture Institute, The Royal Commission for Alula, Alula, Saudi Arabia
Revitalizing oasis agriculture, an age-old human endeavor, has historically played a crucial role in sustaining biodiversity and ecosystems in arid regions. Nevertheless, this enduring practice now faces contemporary challenges, including global warming, water scarcity, soil erosion, and negative human activities associated with urbanization. This comprehensive review delves into diverse literature across disciplines, covering topics such as water conservation, biodiversity restoration, agroforestry, and Oasis Holistic Management, with the aim of addressing these challenges. The analysis strongly advocates for the urgent adoption of sustainable practices, including precision irrigation, polyculture, organic farming, agroforestry, and community-based initiatives, to ensure the survival of oasis agriculture and foster long-term environmental and social responsibility. The study underscores the imperative need for the development of “comprehensive, flexible, and forward-looking management strategies” to guide the sustainable revival of oasis farming. By consolidating information from various studies, it lays the groundwork for informed decision-making and policy formulation. As part of revitalizing the oasis agricultural ecosystem and addressing the global climate crisis, we propose a noninvasive tool for assessing carbon sequestration effectiveness based on tree specifications. Recognizing the pivotal role of vegetation in mitigating the ecological impact and facing global crises, we explored parameters influencing plant carbon sequestration, including biomass production, growth rate, longevity, root structure, leaf structure, and average temperature tolerance.
1 Introduction
Oasis agriculture involves planting in desert interiors and arid lands with soil capable of supporting life. It holds deep historical significance in the context of human civilization. Green islands, often accompanied by water basins, have historically been vital to societies, contributing to biodiversity and maintaining unique ecosystems (Clarke et al., 2016). Therefore, oasis agriculture plays a crucial role in ensuring food and environmental security worldwide, operating within narrow margins (Cantonati et al., 2020).
Traditionally, oasis agriculture refers to a form of cultivation practiced in oases, characterized by wet or fertile land amidst dry regions. These fertile regions emerge due to the presence of underground water sources, allowing complete vegetative cover to develop on seemingly barren land (Kimura et al., 2020). The significance of oasis agriculture extends beyond food production. Oases serve as important ecosystems supporting diverse habitats for flora and fauna (Purdue et al., 2021; Merlo, 2024). These green areas often act as refuges for local fauna, sustaining biodiversity in territories where life would otherwise be challenging (Amini et al., 2016).
Despite its historical importance, oasis agriculture faces numerous challenges today. Climate change poses a significant threat, altering precipitation patterns and increasing aridity. Over-extraction of resources, often due to unsustainable farming practices and urbanization, leads to declining water tables in many existing oases (Boulton et al., 2023). Soil degradation exacerbates these issues, impacting crop fertility and perpetuating poverty. Moreover, competition for scarce resources can exacerbate social and economic inequalities among communities, rendering oasis agriculture unsustainable (Bozorovich, 2020).
The revival of oasis agriculture carries significant global implications. It addresses food security challenges, particularly in areas where oases historically served as agricultural centers (Ma et al., 2023). Reviving oasis agriculture also helps preserve unique ecosystems and combats climate change by preventing desertification and sequestering carbon dioxide through tree planting (De Leeuw et al., 2019). Furthermore, local agriculture’s resurgence has the potential to alleviate poverty and stimulate economic growth. Sustainable agricultural practices create job opportunities, empower local communities, and promote eco-tourism. A comprehensive, sustainable approach to agriculture emphasizes an integrated development model that considers both environmental and social aspects (Schweitzer et al., 2021). One essential strategy for revitalizing oasis agriculture is the implementation of agroforestry.
Agroforestry systems represent a sustainable land management approach that integrates trees, crops, and sometimes livestock in a synergistic and mutually beneficial manner (Jose, 2009). These systems are guided by principles aimed at optimizing land productivity while concurrently enhancing ecological balance (Nair, 2012). By combining different components within the same land area, agroforestry seeks to mimic natural ecosystems, where various plant and animal species coexist in harmony (Jose, 2009). Agroforestry systems combine trees, crops, and sometimes livestock in a symbiotic and mutually beneficial manner. Strategic tree planting and windbreaks make oasis agroforestry feasible, providing social, economic, and environmental gains through the incorporation of trees into farm and rangeland landscapes (Reij et al., 2009; Nair, 2012). Critical practices in oasis agroforestry include tree planting to enrich soil vitality, provide shade, and promote biodiversity. This innovative agroforestry model serves as a testament to the ingenuity and adaptability of communities that have thrived in challenging environments for centuries (Mortimore et al., 2009).
These practices create vibrant urban neighborhoods supporting both human needs and the preservation of fragile ecosystems (Mortimore and Adams, 2001; Mortimore et al., 2009).
In the effort to rejuvenate oasis agriculture, there is a central emphasis on integrating Sustainable Development Goals (SDGs) through strategic tree planting, with a focus on carbon sequestration. This endeavor aligns with various SDGs, actively contributing to the global pursuit of sustainable development. Planting trees that sequester carbon directly addresses the urgent need to mitigate climate change. By capturing and storing carbon, these trees play a pivotal role in adapting to and mitigating the effects of climate change, aiding in the reduction of greenhouse gas concentrations in the atmosphere. The careful selection of trees for carbon sequestration in oasis agriculture aligns with the larger objective of rejuvenating and safeguarding terrestrial ecosystems. This contributes to the overarching aim of preventing and reversing land degradation, fostering biodiversity, and ensuring the sustainable utilization of terrestrial resources. Through strategic tree planting and windbreaks, agroforestry in oases becomes feasible, offering social, economic, and environmental benefits by integrating trees into both farm and rangeland landscapes. In the current study, we suggest planting trees with significant carbon sequestration capabilities, contributing to the restoration of diverse ecosystems (Hussain et al., 2021).
To facilitate effective agroforestation as a component of oasis revitalization, our study focused on examining the correlation between carbon sequestration and various plant criteria. These criteria encompassed biomass, root and leaf architecture, longevity, and temperature, revealing their interconnected roles in the process (Pett-Ridge et al., 2018; Ren et al., 2019; Xue et al., 2021; Li et al., 2022).
Several criteria play a pivotal role in determining the carbon sequestration capacity of plants. Above-ground biomass facilitates photosynthesis, while below-ground biomass serves as a reservoir for carbon storage in the soil (Samuelson et al., 2017; Wang et al., 2019). The characteristics of root systems and leaves contribute to both soil carbon retention and photosynthetic efficiency (Cao et al., 2019; Rodrigues et al., 2023). Plant longevity and photosynthetic efficiency are crucial factors influencing carbon accumulation (Dahl and Arens, 2020; Button et al., 2022). The rapid growth of plants is essential for carbon fixation through enhanced photosynthesis (Schweitzer et al., 2021; Boulton et al., 2023). The duration of a plant’s life cycle impacts carbon storage, with longer-lived tree species demonstrating higher carbon accumulation (Ligot et al., 2018; Bhargava and Mitra, 2021). Root architecture enhances the input of soil organic matter and facilitates carbon storage (Wang J. et al., 2022; Ganamé et al., 2020). Leaf structures influence photosynthetic efficiency, a critical factor in carbon absorption (Lloyd and Kossmann, 2019; Angotra et al., 2021). Additionally, genetic variability and environmental adjustments further influence photosynthetic efficiency (Bozorovich, 2020; Ghiat and Al-Ansari, 2021). By enhancing carbon sequestration through tree planting, oasis agriculture actively promotes responsible land use and production practices. This aligns seamlessly with the objectives of Sustainable Development Goal 12 (SDG 12), which aims to establish sustainable consumption and production patterns for a more resilient and sustainable future. The process of trees sequestering carbon not only contributes to purer air but also enhances overall environmental well-being. This aligns with the broader goal of ensuring access to affordable, reliable, sustainable, and modern energy, as cleaner environments are often synonymous with healthier ecosystems.
2 Oasis ecosystems
Oasis ecosystems are distributed across diverse geographical regions worldwide, predominantly inhabiting arid and semi-arid areas characterized by limited water resources. Noteworthy regions hosting these ecosystems include North Africa, where countries such as Egypt, Libya, Algeria, Tunisia, and Morocco showcase prominent oases like Siwa Oasis and Tozeur (Tydecks et al., 2023). The Middle East is another significant region, featuring oasis ecosystems in nations like Saudi Arabia, Oman, UAE, Iran, and Jordan, with examples including the Al-Hasa and AlUla oases in Saudi Arabia (Al-Mohamed et al., 2023; Hassaballa and Salih, 2024). Central Asia, comprising countries like Uzbekistan, Turkmenistan, and Kazakhstan, harbors oasis ecosystems exemplified by those found in the Fergana Valley, sustained by rivers like the Syr Darya and Amu Darya (Różkowski and Rzętała, 2021). Southwest Asia, represented by Afghanistan and Pakistan, boasts oasis ecosystems such as the Quetta Valley (Durrani and Farooqi, 2021). In North America, the southwestern United States and northern Mexico host desert springs or oases, including Palm Springs and the Organ Pipe Cactus National Monument (Winkler and Brooks, 2020). South America, particularly the Atacama Desert in Chile and the Sechura Desert in Peru, contains oasis ecosystems reliant on water from rivers, groundwater, or coastal fog (Moat et al., 2021). Additionally, oasis ecosystems are present in select regions of Central Africa, notably in arid areas bordering the Sahara Desert, with countries like Chad and Niger featuring settlements sustained by water from seasonal rivers or fossil aquifers (Brahim et al., 2021).
Oasis ecosystems exemplify dynamic environments where the presence of water sustains the growth of diverse vegetation, encompassing trees, shrubs, and crops, within arid or semi-arid landscapes. Here, we present several illustrative examples of oasis ecosystems, detailing the pivotal role played by associated trees and annual crops, as well as their significance for the overall ecosystem:
2.1 Date palm oasis (Middle East and North Africa)
Date palm oases are of immense cultural, economic, and ecological significance. They serve as a crucial source of income through date production, contribute to biodiversity by providing habitats for various species, and aid in mitigating desertification by stabilizing soils with their extensive root systems (Mahmoud et al., 2022; Zemni et al., 2022; Gros-Balthazard et al., 2023).
In these oases, dominant tree species such as Date palms (Phoenix dactylifera) provide shade and food in the form of dates. Date palm cultivation serves as a cornerstone in fostering sustainable livelihoods within regions dependent on date production, thereby significantly contributing to the economic resilience of diverse populations by facilitating rural settlement over urban migration. Date palms offer a multifaceted array of benefits, including the provision of high-quality fruits and fodder for livestock, such as green leaves, seeds, and lower-grade fruits. Furthermore, they supply wood for combustion and construction material for residential buildings (Faiad et al., 2022). The dependency on date palms as a primary source of income is evident, particularly among numerous date palm orchard owners and landholders across various cultivation areas, who often earn moderate incomes (Mondol et al., 2021).
Additionally, crops cultivated alongside date palms include barley, wheat, figs, citrus fruits, and pomegranates (Mahmoud et al., 2022; Zemni et al., 2022; Gros-Balthazard et al., 2023).
2.2 Almond and olive oasis (Mediterranean Basin)
These oases contribute significantly to biodiversity and soil conservation, as well as carbon sequestration. The deep root systems of almond and olive trees help prevent soil erosion, while diverse cropping systems enhance ecosystem resilience (Ossama et al., 2021; Debbabi et al., 2022). Almond trees (Prunus dulcis) and olive trees (Olea europaea) are commonly found in Mediterranean oases, providing nuts and oil, respectively. Annual crops such as grapes, tomatoes, peppers, and chickpeas are often grown between tree rows (Ossama et al., 2021; Debbabi et al., 2022).
2.3 Acacia and millet oasis (Sub-Saharan Africa)
Acacia-millet oases are essential for supporting livelihoods in rural communities of arid regions. Acacias provide fodder for livestock, while millet and sorghum serve as staple food crops. Furthermore, the nitrogen-fixing ability of acacias enriches the soil, benefiting crop production. Common acacia species such as Acacia senegal and Acacia seyal provide shade and contribute to nitrogen fixation. Crops cultivated in association with acacia trees include sorghum, pearl millet, and other drought-resistant crops (Cascadden et al., 2020; Ariom et al., 2022).
2.4 Palmeraie oasis (Morocco)
Palmeraie ecosystems in Morocco play a vital role in biodiversity conservation, particularly for migratory birds. They provide essential ecosystem services like carbon sequestration, water filtration, and microclimate regulation. Besides date palms, other tree species like citrus trees (e.g., orange, lemon) and pomegranate trees thrive in Moroccan palmeraies. Alongside trees, annual crops such as vegetables, grains, and legumes are cultivated (Beraaouz et al., 2022; Houssni et al., 2023).
2.5 Mesquite and maize oasis (Southwestern United States and Mexico)
Mesquite-maize oases support biodiversity by providing habitat for desert-adapted species. The “Three Sisters” cropping system promotes soil fertility through nitrogen fixation by beans and enhances soil moisture retention. Mesquite trees (Prosopis species) are characteristic of desert oases in the southwestern United States and Mexico, offering shade and pods that can be used for food. Cultivated in association with mesquite trees, maize (corn) is a staple crop often accompanied by beans and squash (the “Three Sisters” cropping system) (Vierra and Vint, 2022; Andablo-Reyes et al., 2023).
3 Methodology
The aim of this systematic review was to conduct a comprehensive examination and synthesis of literature pertaining to the sustainable revitalization of agricultural oases. The primary objective was to identify and consolidate best practices from various fields crucial to oasis agriculture, encompassing water conservation, biodiversity restoration, soil health enhancement, agroforestry, community engagement, soil conservation techniques, holistic management, and climate-resilient agriculture. Additionally, the review aimed to present case studies exemplifying not only effective but also holistic and equitable approaches to revitalizing agriculture in oases.
This study strictly adheres to the Preferred Reporting Items for Systematic Reviews and Meta-Analyses (PRISMA) guidelines, which furnish a structured framework for conducting and reporting systematic reviews. By following PRISMA guidelines, this study ensures replicability, transparency, and comprehensive retrievability in the systematic retrieval of literature concerning the sustainability of oasis agriculture revitalization (Page et al., 2021). In Figure 1, we present the PRISMA Flowchart illustrating the complete screening process regarding the sustainable revitalization of agricultural oases.
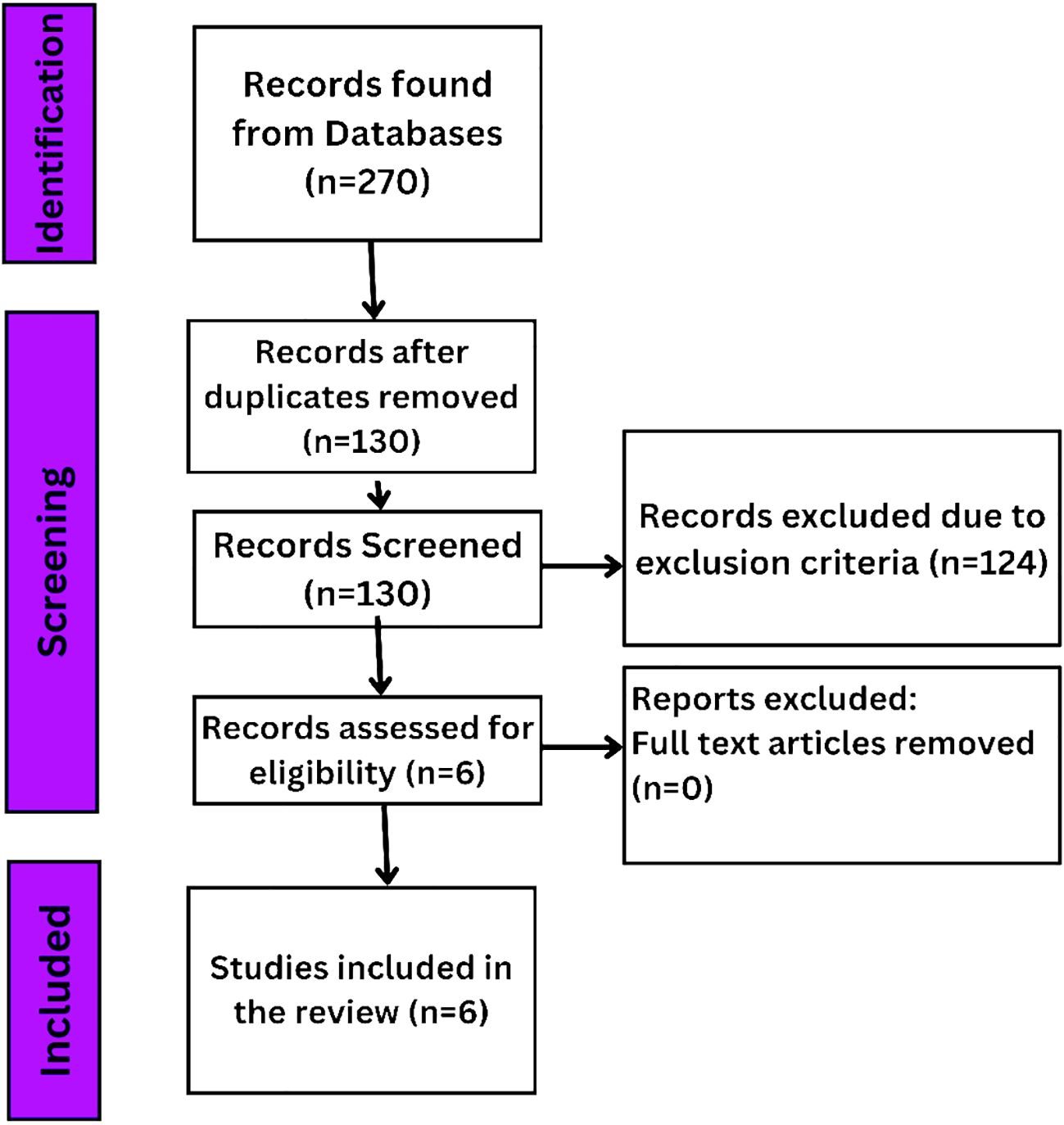
Figure 1 PRISMA flowchart depicting the complete screening process concerning the sustainable revitalization of agricultural oases.
To comprehensively cover literature related to the sustainable revitalization of agriculture in oases, two distinct search strings were developed and applied to two major databases, Google Scholar and AGRIS, employing a combination of keywords and MESH terms derived from relevant databases thereby were:
● (“Sustainable agriculture” OR “Oasis agriculture” OR “Agriculture in arid regions”) AND (“Oasis revitalization” OR “Oasis sustainability” OR “Revitalizing agriculture in oases”).
● (“Water conservation” OR “Biodiversity restoration” OR “Soil health improvement”) AND (“Agroforestry in oases” OR “Community engagement” OR “Climate-resilient agriculture”).
4 Agroforestry systems
Conservation and restoration are paramount considerations for oasis agroforestry systems due to escalating pressures from urbanization, tourism, and climate change, which pose threats to these fragile ecosystems (Yi et al., 2023a). Agroforestry practices emerge as pivotal tools in addressing these challenges, as they promote sustainable land use practices that preserve ecosystem health and biodiversity while supporting local livelihoods. Agroforestry systems, integrating trees, crops, and sometimes livestock, offer a multifaceted approach to land management, striving to maximize productivity, sustainability, and ecological balance (Nair, 2012).
In regions like oasis ecosystems, where water resources are scarce, efficient water management techniques such as drip irrigation and water harvesting are indispensable for agricultural sustainability (Yi et al., 2023b). Farmers in such environments meticulously manage water resources to maximize their utilization. Resource cycling is another essential principle of agroforestry, emphasizing the efficient use and cycling of nutrients and water within the system. Trees play a critical role in nutrient cycling by extracting nutrients from deeper soil layers and making them available to crops (Nair, 2012). Additionally, tree roots help stabilize soil, reducing erosion and improving soil structure.
The second level of agroforestry involves synergies and complementarities among different components of the system. Tree-crop interactions are crucial in oasis agroforestry systems. For example, the shade provided by trees can benefit certain crops that thrive in partial shade, while the root systems of trees can improve soil structure for crops (Jose, 2009). Trees, particularly date palms, play a vital role in providing shade, windbreaks, and microclimate regulation for crops grown in oases (Al-Mohamed et al., 2023). Additionally, the integration of livestock, such as goats or camels, contributes to nutrient cycling through manure deposition, enhancing overall productivity (Hassaballa and Salih, 2024).
At the third level, windbreaks composed of hardy trees and shrubs are established to mitigate soil erosion and protect crops from wind damage, particularly in wind-prone areas like oases. Date palms, among other tree species, are instrumental in providing shade, windbreaks, and microclimate regulation for crops (Al-Mohamed et al., 2023). This multi-tiered approach optimizes land use and diversifies agricultural products, contributing to overall productivity and sustainability.
Moreover, ecosystem services represent a critical dimension of agroforestry systems, encompassing benefits such as carbon sequestration, wildlife habitat provision, water purification, and erosion control (Dagar, 2016). Social and economic considerations are integral, necessitating the incorporation of local community needs, cultural preferences, and market opportunities into agroforestry planning to ensure long-term viability and acceptance (Jose, 2009). Preservation of cultural significance is crucial for oasis agroforestry systems, serving as hubs for community gatherings, traditional practices, and local livelihoods (Santoro, 2023).
5 Oasis water conservation and management
Oases, as critical islands of fertility in deserts, heavily depend on water resources originating from subterranean aquifers, natural springs, or rivers. Historically, human civilizations have thrived near oases, utilizing them for farming, drinking, and various necessities (Seely et al., 2008). To safeguard oasis water resources for sustainable development, effective water-saving methods are crucial, especially in water-deficit regions (Xue et al., 2015).
5.1 Khettaras water management
The water management systems utilized in oasis ecosystems, exemplified by the khettaras in North Africa, stand as pivotal pillars in sustaining agricultural activities and ecosystem health. Khettaras, also recognized as qanats or falaj, represent traditional underground tunnel systems meticulously crafted to tap into groundwater reservoirs and convey water from aquifers to the surface for irrigation (Beraaouz et al., 2022). Operating on the principle of gravity, these ancient hydraulic networks harness the natural slope of the terrain to guide groundwater through a network of gently sloping tunnels towards the oasis fields (Esenarro et al., 2023). The construction of khettaras entails the excavation of vertical shafts at intervals along the tunnel’s trajectory to access the groundwater table, followed by the creation of horizontal tunnels to interlink these shafts, thus facilitating the flow of water towards the surface (Beraaouz et al., 2022).
Central to the functioning of khettaras is the collective water management system, characterized by the collaborative efforts of oasis communities to regulate and sustainably utilize water resources. Operating under established rules and regulations, often rooted in customary laws like Al Orf/Azref, these systems ensure the equitable distribution of water among farmers while preventing the overexploitation of this vital resource (Beraaouz et al., 2022). Key facets of this water management system, as outlined by Beraaouz et al. (2022) and Esenarro et al. (2023), include:
5.1.1 Equitable distribution
Water allocated from khettaras is typically distributed among farmers based on predetermined schedules or allocations, thereby fostering fairness and averting conflicts over water rights.
5.1.2 Community governance
Historically, oasis communities have established governance structures to oversee the management of khettaras and resolve disputes related to water usage. These structures often comprise community councils or traditional water management committees.
5.1.3 Maintenance and rehabilitation
Oasis communities engage in collective endeavors to maintain and rehabilitate khettaras, undertaking tasks such as periodic desilting, repairs, and debris clearance to ensure the uninterrupted flow of water.
5.1.4 Adaptation to environmental changes
To cope with environmental fluctuations such as shifts in groundwater levels, alterations in precipitation patterns, and the impacts of climate change, oasis communities have developed adaptive strategies. Flexibility and resilience are inherent traits of the water management system associated with khettaras.
By integrating the khettara water management system into broader strategies for sustainable agriculture and natural resource management, oasis communities can bolster the resilience of their ecosystems and livelihoods amid ongoing challenges. The preservation and revitalization of khettaras not only secure water resources but also uphold cultural heritage and traditional wisdom passed down through generations.
5.2 Water conservation techniques
In oasis agriculture, water conservation is achieved through advanced irrigation systems like drip irrigation and precision agriculture. Groundwater monitoring plays a vital role in preventing over-pumping, necessitating an understanding of aquifer behavior and the establishment of safe extraction limits (Yang et al., 2019). Sustainable land use planning around oases is essential to avoid soil erosion and degradation, involving measures to control deforestation, manage grazing lands, and limit urban sprawl to maintain ecological equilibrium (Hao et al., 2017). Developing and implementing water conservation policies at regional and national levels are integral components of oasis water resource management, addressing water withdrawal, land use, and conservation techniques (Bie and Xie, 2020). Furthermore, utilizing treated water in plantation watering systems enhances efficiency by ensuring that water meets quality standards suitable for agricultural use. Treatment processes can remove contaminants and impurities, providing a reliable and safe water source for plant irrigation. This approach safeguards crops from potential harm due to poor water quality and contributes to the long-term health and productivity of the oasis ecosystem.
Incorporating diverse water sources in oases revitalization aligns with the principles of water sustainability and resource conservation. It not only mitigates the pressure on traditional water reservoirs but also promotes responsible water management practices. By integrating recycled greywater and treated water into plantation watering systems, oases can achieve a harmonious balance between agricultural productivity and environmental stewardship, ensuring a resilient and sustainable future for these valuable ecosystems (Hong et al., 2003; Mekki et al., 2013). By adopting these practices, communities ensure the sustainability of their oasis water sources, meeting current needs while preserving these invaluable ecosystems for the future.
5.3 Water harvesting techniques
Water harvesting techniques serve as vital complements to the function of khettaras by capturing and storing rainwater, thereby augmenting groundwater resources and bolstering overall water availability in oasis ecosystems (Derdour et al., 2022; Lüthgens et al., 2023). Various water harvesting methods commonly utilized in oasis environments include:
5.3.1 Surface water harvesting
This method entails capturing rainfall runoff from the land’s surface and diverting it towards storage reservoirs or infiltration basins. In oasis ecosystems, surface water harvesting may involve constructing contour bunds, check dams, or terraces to impede runoff and facilitate infiltration (Gao et al., 2023).
5.3.2 Roof rainwater harvesting
Within oasis settlements, roof rainwater harvesting systems can be implemented to capture rainwater from rooftops and store it in cisterns or tanks for subsequent use. This decentralized approach to water harvesting offers an additional water source for domestic needs and small-scale irrigation (Raimondi et al., 2023).
5.3.3 Infiltration ponds and basins
Designed to capture and retain rainwater, infiltration ponds and basins allow water to percolate into the soil, replenishing groundwater aquifers. Strategically locating these structures within oasis landscapes maximizes water retention and supports vegetation growth (Patil et al., 2023).
5.3.4 Fog harvesting
In regions with coastal or mountainous terrain, fog harvesting systems intercept moisture from foggy air using mesh nets or collectors. Captured fog droplets are then directed into storage containers for irrigation or drinking water purposes (Budhalakoti et al., 2023).
5.3.5 Subsurface water harvesting
Techniques like trenching or subsoil dams capture and retain rainfall within the root zone of plants, replenishing soil moisture and supporting plant growth. Particularly valuable in areas lacking surface water sources, subsurface water harvesting enhances soil moisture retention (Walia et al., 2024).
Integrating water harvesting practices with khettaras and traditional water management systems enhances the resilience of oasis ecosystems against climate variability and water scarcity. This integration optimizes water use efficiency, mitigates drought impacts, and ensures sustainable water resource management for agriculture, ecosystem preservation, and human well-being.
It’s noteworthy that the selection and implementation of specific water harvesting techniques in oasis ecosystems may vary depending on local environmental conditions, socio-economic factors, and cultural norms. Thus, a comprehensive understanding of these factors is imperative for the successful adoption of water harvesting initiatives in oases (Li and Wang, 2021).
6 Oasis biodiversity restoration
Restoring oasis biodiversity involves targeted planting of local species and adopting polyculture techniques, growing multiple plant species together to preserve genetic diversity and improve soil conditions (Kumar et al., 2018). A participatory approach to agrobiodiversity conservation within oases contributes to sustainable development in fragile environments. Incorporating permaculture principles, which focus on polyculture garden designs with diverse indigenous plant species, enhances ecosystem restoration efforts (Bhagwat et al., 2017).
Traditional agroforestry systems in seasonally dry tropical forests play a crucial role in the conservation of native plant diversity. These systems represent a harmonious integration of trees, crops, and often livestock, mirroring the intricate relationships between the local communities and their natural environment. In these agroforestry systems, farmers leverage the benefits of diverse plant species, both economically valuable crops and indigenous tree species, to create resilient and sustainable landscapes. The traditional knowledge passed down through generations guides the selection and management of plant combinations that thrive in seasonally dry conditions, demonstrating a deep understanding of the local ecosystem (Montagnini and Nair, 2004). Another approach is Permaculture, a sustainable agricultural design system, strongly advocates for the establishment of polyculture gardens as a fundamental principle. In contrast to monoculture, where a single crop dominates a given area, polyculture involves cultivating a diverse array of plants within the same space (Fiebrig et al., 2020; McLennon et al., 2021). This approach holds several key benefits that contribute to the overall health and resilience of ecosystems. Preserving genetic diversity is a central tenet of permaculture. By cultivating a variety of plant species in polyculture gardens, permaculturists aim to protect and enhance the genetic richness of their cultivated plants. This diversity not only safeguards against the risks of crop diseases and pests but also promotes adaptability to changing environmental conditions. In the face of climate change and other challenges, a genetically diverse garden is more likely to thrive and adapt over time (HaLevi et al., 2020). The emphasis on soil quality is another hallmark of permaculture practices. Polyculture gardens contribute to improved soil health through the cultivation of plants with different root structures, nutrient requirements, and growth habits. This diverse plant community helps enhance soil structure, prevent erosion, and foster a balanced nutrient cycle, reducing the need for external inputs like synthetic fertilizers (Peveri, 2021). Attracting beneficial insects is a key ecological function of polyculture gardens within permaculture principles. The variety of flowering plants and vegetation provides habitat and food sources for a diverse range of insects, including pollinators and natural predators of pests. This natural balance helps control pest populations, reducing the reliance on chemical interventions and promoting a healthier, more ecologically sound gardening environment. This approach aligns with the principles of ecological balance, emphasizing the interconnectedness of all elements within a garden ecosystem. Polyculture methods play a crucial role in habitat restoration, transforming barren land into lush oases by incorporating nitrogen-fixing legumes, drought-resistant grains, and deep-rooted perennials (Altieri, 1999; LaCanne and Lundgren, 2018).
Additionally, Regenerative farmers play a pivotal role in fostering biodiversity and supporting ecosystem health by deliberately creating habitats for pollinators within their agricultural landscapes (Soto et al., 2021). One of the key practices they employ is the strategic planting of wildflowers, flowering shrubs, and native grasses. The cultivation of wildflowers is a hallmark of regenerative farming, as these plants are not only visually appealing but also serve as crucial sources of nectar and pollen for pollinators. By incorporating a diverse array of wildflowers, farmers provide sustenance for bees, butterflies, and other beneficial insects essential for the pollination of crops. This practice not only enhances the productivity of the farm but also contributes to the broader conservation of pollinator populations. Flowering shrubs add another layer to the habitat creation efforts of regenerative farmers (McHugh et al., 2022). These shrubs offer additional food resources for pollinators while providing shelter and nesting sites (McHugh et al., 2022). The structural complexity of shrubs contributes to the overall biodiversity of the farm, creating a more resilient and balanced ecosystem. Native grasses, adapted to the local environment, further contribute to the habitat mosaic on regenerative farms. These grasses provide essential cover and foraging opportunities for pollinators, creating corridors that connect different areas of the farm. The interconnected habitat encourages the movement of pollinators, supporting their populations and promoting genetic diversity (Schreefel et al., 2022). By intentionally designing landscapes that incorporate wildflowers, flowering shrubs, and native grasses, regenerative farmers go beyond conventional monoculture practices. They recognize the importance of supporting pollinator health as integral to the success of their agricultural endeavors. This approach aligns with broader conservation goals, contributing to the protection of essential pollinator species and fostering a more resilient and sustainable agricultural ecosystem (Potts et al., 2016). Intentional inclusion of indigenous plant species and polyculture methods not only preserves genetic diversity and soil health but also creates vibrant urban oases sustaining human life and preventing ecosystem degradation (Toledo and Burlingame, 2006).
7 Oasis soil health improvement
Improving soil health in oases involves incorporating organic farming methods, cover cropping strategies, and circular agriculture (Pretty et al., 2006; Gomiero et al., 2011; Kirchmann et al., 2014; Altieri and Nicholls, 2020). Organic farming enhances soil fertility biologically, releasing organic matter into the soil and sequestering carbon, contributing to carbon dioxide reduction in the atmosphere (Pretty et al., 2006). Organic farming distinguishes itself by eschewing the use of synthetic pesticides, herbicides, and fertilizers. Instead, it relies on a suite of natural alternatives, including compost, crop rotation, and companion planting, to bolster soil fertility and manage pests effectively (Benbrook et al., 2021). Crop rotation is a common practice among organic farmers, not only to sustain soil health but also to thwart the accumulation of pests and diseases. This commitment to planting a diverse array of crops serves to enrich biodiversity and fortify the overall resilience of the agricultural ecosystem (Tanveer et al., 2021). Moreover, organic livestock farming underscores a profound commitment to the humane treatment of animals. Livestock are afforded access to the outdoors, provided with natural diets, and housed in conditions conducive to their well-being. Simultaneously, organic farming places soil health at the forefront of sustainable agricultural practices. Techniques like cover cropping, mulching, and composting are employed with the overarching goal of enhancing soil structure, improving water retention, and augmenting nutrient levels (Cataldo et al., 2021). In addition to these practices, organic farming staunchly opposes the use of genetically modified organisms (GMOs) in both crops and livestock. The focal point remains the preservation of natural genetic diversity. As a holistic approach to agriculture, organic farming endeavors to minimize its environmental impact by refraining from the use of synthetic chemicals that contribute to pollution and harm ecosystems. This commitment often results in the improvement of water and air quality in the surrounding areas. To ensure adherence to organic principles, organic farming is subject to rigorous certification standards. The certification process involves thorough inspections, and products labeled as organic must meet specific criteria established by regulatory bodies. This systematic oversight underscores the dedication of the organic farming community to maintaining high standards and promoting sustainable, environmentally friendly agricultural practices (Cakirli Akyüz and Theuvsen, 2020).
Cover cropping stands out as a central and transformative practice in the regeneration of oases (Altieri and Nicholls, 2020). This agricultural technique involves planting specific crops primarily for the purpose of improving soil health, managing erosion, and enhancing overall ecosystem resilience. In the context of oases regeneration, where maintaining and restoring soil fertility is paramount, cover cropping plays a crucial role. The selected cover crops, often legumes or other nitrogen-fixing plants, contribute to soil enrichment by fixing atmospheric nitrogen into a form that plants can utilize. This natural fertilization enhances the nutrient content of the soil, fostering a more fertile and productive agricultural landscape. One of the key benefits of cover cropping is its ability to prevent soil erosion (Sherwood et al., 2023). Oases, often located in arid regions, are particularly susceptible to soil degradation through wind and water erosion. The dense foliage of cover crops provides a protective layer, shielding the soil from the erosive forces of wind and water. This protection is instrumental in maintaining the structural integrity of the soil, preserving valuable topsoil, and preventing the encroachment of sand or dust into agricultural areas. Cover cropping also contributes to the conservation of water resources in oases. The plant cover acts as a natural mulch, reducing water evaporation from the soil surface and improving water retention. This is especially crucial in regions where water scarcity is a persistent challenge, as it allows for more efficient use of available water for crop growth. Beyond soil health, cover cropping fosters biodiversity in oases (Ugrenović et al., 2024). The introduction of diverse plant species provides habitat and food sources for beneficial insects, pollinators, and microorganisms. This creates a balanced and dynamic ecosystem, promoting resilience and reducing the reliance on external inputs such as synthetic fertilizers and pesticides.
Circular agriculture serves as a powerful strategy for soil organic input management, promoting soil fertility, retaining soil structure, and attracting beneficial microorganisms (Pretty et al., 2006; Gomiero et al., 2011; Altieri and Nicholls, 2020). Circular agriculture emerges as a potent strategy for soil health management in the context of oases regeneration. This innovative approach, inspired by principles of sustainability and resource efficiency, seeks to create closed-loop systems that minimize waste and enhance overall ecosystem health (El Janati et al., 2021). In the realm of oases regeneration, circular agriculture addresses several key aspects of soil health management. Firstly, it promotes the efficient use of resources by recycling organic matter back into the soil. Crop residues, organic waste, and other biomass materials are repurposed through composting or other recycling methods, enriching the soil with essential nutrients. This not only improves soil fertility but also reduces the dependence on external inputs like synthetic fertilizers. Furthermore, circular agriculture emphasizes the importance of crop diversity and rotation. By cultivating a variety of crops in a cyclical manner, the soil is less prone to nutrient depletion and erosion. Crop rotation disrupts the life cycles of pests and diseases, minimizing the need for chemical interventions and promoting a healthier, more resilient soil ecosystem. These methods contribute to developing diverse urban oases that fulfill human needs and help maintain fragile ecosystems (Cakirli Akyüz and Theuvsen, 2020; El Janati et al., 2021; Sherwood et al., 2023; Ugrenović et al., 2024).
8 Oasis community engagement
Community involvement in oases is facilitated by education, development schemes, and community-run initiatives. Oasis community engagement plays a pivotal role in the successful regeneration of these unique ecosystems. The involvement and empowerment of local communities are essential components of sustainable oases regeneration initiatives. The relationship between oasis community engagement and regeneration is multifaceted, encompassing environmental stewardship, cultural preservation, and socio-economic development (Dresch and James, 1988; Behnke et al., 1993; Davies, 1996; Hesse and MacGregor, 2006).
8.1 Environmental stewardship
Oasis communities are intimately connected to the natural environment, relying on it for sustenance and livelihoods. Engaging these communities in the regeneration process ensures their active participation in environmental conservation efforts.
Community members often possess traditional ecological knowledge that is invaluable for understanding the local ecosystem. This knowledge, combined with modern scientific approaches, can inform sustainable practices that support the regeneration of oases (Dresch and James, 1988; Behnke et al., 1993; Davies, 1996; Hesse and MacGregor, 2006).
8.2 Cultural preservation
Oases are often rich in cultural heritage, with unique traditions and practices passed down through generations. Community engagement ensures the preservation of these cultural aspects, fostering a sense of identity and pride among local residents.
Involving the community in regeneration initiatives allows for the incorporation of traditional agricultural methods, indigenous plant species, and water management techniques that have sustained oasis communities for centuries (Dresch and James, 1988; Behnke et al., 1993; Davies, 1996; Hesse and MacGregor, 2006).
8.3 Socio-economic development
Oasis regeneration projects can have a direct impact on the socio-economic well-being of local communities. Engaging community members in sustainable agricultural practices, agro-tourism initiatives, and small-scale enterprises can generate income and improve living standards.
Training and capacity-building programs can empower community members with the skills and knowledge needed to actively participate in the regeneration process. This not only enhances their resilience to environmental changes but also creates opportunities for innovation and entrepreneurship (Dresch and James, 1988; Behnke et al., 1993; Davies, 1996; Hesse and MacGregor, 2006).
8.4 Community-led conservation
Successful oasis regeneration requires the active involvement and commitment of the local community. Engaging community members in decision-making processes, goal-setting, and project implementation fosters a sense of ownership and responsibility for the regenerated oasis. Establishing community-led conservation practices, such as sustainable water management and agroecological farming, ensures the long-term viability of the oasis ecosystem. This shared responsibility strengthens the sustainability of regeneration efforts (Hesse and MacGregor, 2006).
8.5 Education and awareness
Community engagement serves as a platform for education and awareness. By involving residents in workshops, training sessions, and awareness campaigns, they can gain insights into the importance of oasis regeneration and the role they play in its success.
Building awareness within the community creates advocates for sustainable practices, encouraging responsible water use, waste management, and the protection of biodiversity.
In essence, oasis community engagement is not just a complementary aspect of regeneration efforts but a cornerstone for long-term success. By recognizing the intrinsic link between the local community and the oasis ecosystem, regeneration initiatives can create a harmonious balance that ensures environmental, cultural, and socio-economic sustainability for generations to come (Dresch and James, 1988; Behnke et al., 1993; Davies, 1996; Hesse and MacGregor, 2006).
8.6 Local knowledge in oasis ecosystems
Part of community engagement involves leveraging local knowledge in oasis ecosystems for the management of trees and crops, which has been deeply ingrained over centuries of experience and adaptation to the unique environmental conditions of arid and semi-arid regions. This indigenous knowledge encompasses a wide range of practices related to tree cultivation, crop selection, water management, and soil conservation. Here are some key aspects of local knowledge in oasis ecosystems:
8.6.1 Tree management
Oasis communities have traditionally selected tree species that are well-adapted to the local climate and soil conditions. Common tree species include date palms, olive trees, citrus trees, figs, pomegranates, and acacias. These species provide multiple benefits, such as food production, shade, windbreaks, and soil stabilization (Buerkert et al., 2021). To optimize survival rates and growth, local knowledge dictates the timing and methods of tree planting. Techniques such as pit planting, mulching, and companion planting with nitrogen-fixing species are commonly practiced to enhance tree establishment and resilience (Ahmadovna et al., 2021). Traditional pruning techniques are employed to shape trees, promote fruit production, and remove dead or diseased branches. Additionally, regular maintenance activities, such as watering, fertilization with organic matter, and pest control, are carried out based on local knowledge passed down through generations (Santoro et al., 2023; Santoro et al., 2020a; Santoro et al., 2020b).
8.6.2 Crop diversity and rotation
Oasis farmers have developed a diverse range of crop varieties adapted to local soil types, water availability, and climatic conditions. Staple crops include grains such as wheat, barley, and millet, as well as vegetables, legumes, and fruits (Neji, 2023). Traditional crop rotation practices are employed to maintain soil fertility, control pests and diseases, and optimize water use efficiency. Rotation sequences may vary depending on the specific needs of each oasis ecosystem but typically involve alternating between crops with different nutrient requirements and growth habits (Chakkour et al., 2024). Cover cropping (known also as intercropping) or companion planting techniques are commonly used to maximize space utilization and enhance crop productivity. For example, leguminous crops are often planted alongside cereals to fix nitrogen in the soil and improve fertility (Maitra et al., 2021).
8.6.3 Water management and irrigation
8.6.3.1 Management of khettaras and wells
Local knowledge guides the maintenance and operation of traditional water management systems such as khettaras, qanats, and wells. Techniques for desilting, repairing, and regulating water flow are passed down through oral traditions and practical experience (Gasmi, 2023).
8.6.3.2 Irrigation practices
Oasis farmers utilize various irrigation methods, including surface flooding, furrow irrigation, and drip irrigation, to deliver water to crops efficiently. Local knowledge informs decisions on timing, duration, and frequency of irrigation to minimize water loss and maximize plant uptake (Zekri et al., 2023).
8.6.4 Soil conservation and organic farming
8.6.4.1 Soil conservation techniques
To combat soil erosion and degradation, oasis farmers implement soil conservation measures such as terracing, contour bunding, and agroforestry. These practices help stabilize soils, retain moisture, and improve soil structure (Costa et al., 2021; Yang et al., 2021).
8.6.4.2 Organic farming practices
Traditional farming in oasis ecosystems often relies on organic and agroecological principles, with minimal use of synthetic inputs. Crop residues, animal manure, and compost are recycled to enrich soil fertility and enhance crop yields (Xu et al., 2020; Karbout et al., 2021).
8.6.5 Utilization of medicinal and aromatic plants
Local knowledge in oasis ecosystems encompasses a rich repository of traditional practices and wisdom honed over generations. Beyond agricultural techniques, it extends to the utilization of medicinal and aromatic plants interplanted alongside the principal trees, such as date palms or olives. These plants not only contribute to the ecological diversity of the oasis but also serve as invaluable sources of traditional medicine for the local community (Zubay et al., 2021). Through careful observation and experimentation, community members have identified specific plants with medicinal properties, integrating them into their daily lives to address various ailments and promote overall well-being. Furthermore, the intricate balance between limited land and water resources in the oasis environment necessitates a deep understanding of local conditions and adaptive strategies. Farmers, drawing upon generations of accumulated knowledge, have developed sophisticated crop calendars based on traditional wisdom (Sharma et al., 2020). These calendars are finely tuned to the seasonal rhythms of the oasis, optimizing agricultural productivity while ensuring the sustainable use of available resources. By aligning planting and harvesting schedules with natural cycles and resource availability, farmers can maximize yields and minimize environmental impact.
This harmonization of traditional knowledge with contemporary agricultural practices not only enhances production but also contributes to the resilience of oasis ecosystems. Through the integration of local wisdom, farmers are able to navigate the challenges posed by climate variability and resource scarcity, fostering sustainable land use management practices that sustain both livelihoods and natural ecosystems. As such, local knowledge remains a cornerstone of oasis agriculture, representing a testament to the ingenuity and adaptability of communities in these unique and fragile environments.
Overall, local knowledge in oasis ecosystems embodies a wealth of practical wisdom, innovation, and resilience honed over generations of living in harmony with the natural environment. By integrating scientific knowledge with indigenous practices, oasis communities can sustainably manage their resources, preserve biodiversity, and adapt to emerging challenges such as climate change and water scarcity.
9 Oasis Holistic Management
Oasis Holistic Management stands as a comprehensive and integrative approach to the regeneration of oases, emphasizing the interconnectedness of ecological, social, and economic factors. This management philosophy is grounded in the understanding that sustainable regeneration requires a holistic perspective that considers the diverse elements of the oasis ecosystem and the well-being of the communities that depend on it (Brooks, 2005; Berkes, 2010; Reed et al., 2010; Stringer et al., 2012).
9.1 Ecosystem restoration
Oasis Holistic Management places a strong emphasis on restoring the natural balance of the oasis ecosystem. This involves sustainable water management, soil conservation, and the preservation of biodiversity. Techniques such as agroforestry, cover cropping, and the reintroduction of native plant species are incorporated to enhance soil fertility, prevent erosion, and create resilient agricultural landscapes (Alam et al., 2018; Cheema et al., 2019; Hussain et al., 2020; Malik et al., 2021).
9.2 Water resource conservation
Efficient water use is a critical aspect of Oasis Holistic Management. This involves the implementation of water-saving technologies, rainwater harvesting, and the promotion of responsible water consumption in both agricultural and domestic contexts.
Strategies for optimizing irrigation practices and managing water distribution networks are designed to maximize the availability of water for crops while minimizing waste (Bouyoucos et al., 2019; El-Magd et al., 2020).
9.3 Community empowerment
Holistic Management recognizes the pivotal role of local communities in the regeneration process. Engaging and empowering communities ensures that regeneration efforts align with the needs, values, and aspirations of the people who inhabit the oasis. Education, capacity-building, and participatory decision-making processes empower community members to actively contribute to regeneration initiatives, fostering a sense of ownership and responsibility (El-Kharraz et al., 2018; El-Kholy et al., 2019; Hussain et al., 2020; Hamdy and Ghoneim, 2021).
9.4 Cultural preservation
The holistic approach extends to cultural aspects, emphasizing the preservation of indigenous knowledge, traditions, and practices that have sustained oasis communities for generations.
Cultural preservation is integrated into regeneration strategies, ensuring that traditional agricultural methods, local plant varieties, and community customs are respected and incorporated into the management plan (El-Kharraz et al., 2018; El-Kholy et al., 2019; Hussain et al., 2020; Hamdy and Ghoneim, 2021).
9.5 Sustainable livelihoods
Oasis Holistic Management recognizes the importance of balancing economic development with environmental sustainability. Strategies are designed to create sustainable livelihoods for oasis communities through agro-tourism, eco-friendly enterprises, and value-added agricultural products. Diversification of income sources and the promotion of small-scale, locally focused businesses contribute to economic resilience in the face of environmental uncertainties (El-Sadek et al., 2017; Abou-Elkheir et al., 2018; Gomaa et al., 2020; Abdel-Latif et al., 2021).
9.6 Adaptive management
Given the dynamic nature of ecosystems and the uncertainties associated with climate change, Oasis Holistic Management adopts an adaptive approach. Continuous monitoring, feedback loops, and the ability to adjust strategies based on changing conditions ensure the resilience and flexibility of the management plan. Learning from experience and incorporating new knowledge allows for ongoing improvement and refinement of regeneration practices (El-Sadek et al., 2017; Abou-Elkheir et al., 2018; Gomaa et al., 2020; Abdel-Latif et al., 2021).
9.7 Oasis climate-resilient agriculture
Developing climate-resilient agriculture in oases involves introducing drought-resistant crops and adopting adaptive farming techniques (Ahmed et al., 2021). Drought-resistant crops, bred for water-stressed environments, contribute to climate resilience (Khan et al., 2020). Collaboration between scientists and farmers in Tunisia and northwestern China showcases efforts to enhance crop resilience to climate change (Zhang et al., 2019). Adaptive farming practices, such as terracing and water-efficient irrigation systems, contribute to climate resilience by mitigating the effects of climate change (Li et al., 2018). By carefully applying these strategies, oases can evolve into sustainable ecosystems providing essential resources and aiding in the preservation of fragile ecosystems under climate change impacts (Wang W. et al., 2022).
Oasis Holistic Management recognizes that the regeneration of oases is not solely an ecological challenge but a complex system where environmental, social, and economic components are intertwined. By addressing these interconnected aspects with a holistic approach, this management philosophy strives to create sustainable and thriving oases that benefit both the environment and the communities that rely on them. These practices contribute to ecosystem balance, making oases productive, diverse, and anchors in preserving delicate ecological balances (Abdel-Latif et al., 2021).
10 The social aspects of oasis ecosystems
Population changes within oasis ecosystems can have significant impacts on community dynamics, traditional livelihoods, land use patterns, and natural resource management. Here’s how social aspects intersect with population changes in oasis ecosystems:
10.1 Population growth and pressure on resources
Population growth within oasis communities can lead to increased pressure on limited resources such as water, land, and vegetation. As populations expand, there is a greater demand for agricultural land and water for irrigation, potentially leading to overexploitation and environmental degradation (Rhouma et al., 2020; Yi et al., 2023b).
10.2 Traditional livelihoods and cultural practices
Many oasis communities have longstanding traditions and cultural practices related to agriculture, water management, and community cooperation. However, population changes, including in-migration or out-migration, can disrupt these traditions. New residents may have different livelihood strategies or may not be as familiar with traditional practices, leading to cultural shifts within the community (Peano et al., 2021).
10.3 Social cohesion and community resilience
Changes in population demographics, such as the influx of migrants or the out-migration of younger generations seeking education or employment opportunities, can affect social cohesion within oasis communities. Maintaining social networks and community cohesion is essential for resilience in the face of environmental challenges or external pressures (Auckland and Kilpatrick, 2021).
10.4 Land tenure and property rights
Population changes may also impact land tenure systems and property rights within oasis ecosystems. As populations grow or shift, conflicts over land ownership, access to water resources, and grazing rights may arise. Clarifying and enforcing land tenure arrangements becomes increasingly important to avoid disputes and ensure equitable access to resources (Buerkert et al., 2021; Zhang and Zhao, 2021).
10.5 Economic opportunities and employment
Population changes can influence economic opportunities within oasis communities. Increased population density may stimulate local markets and create demand for goods and services, potentially providing new avenues for entrepreneurship and employment. Conversely, out-migration of working-age individuals may lead to labor shortages in agriculture or other sectors, affecting productivity and economic development (Asham et al., 2023).
10.6 Infrastructure and service provision
Population growth or changes in population demographics may necessitate investments in infrastructure and public services, such as water supply systems, healthcare facilities, schools, and transportation networks. Adequate infrastructure is essential for improving living standards and enhancing the quality of life for oasis residents (Allali et al., 2023).
10.7 Community governance and decision-making
Changes in population dynamics can affect community governance structures and decision-making processes. Ensuring inclusive participation in decision-making, especially with regard to land use planning, natural resource management, and infrastructure development, is vital for addressing the needs and aspirations of all residents within the oasis community (Ma et al., 2024).
11 Developing a carbon sequestration assessment tool for trees
To construct a tool for assessing carbon sequestration, we employed the systematic review method outlined by Page et al. (2016) and meticulously documented the process using the PRISMA checklist, adhering to its guidelines.
The literature search encompassed the years 2006 to 2023, utilizing a variety of resources including Google Scholar, ResearchGate, and book chapters. Data collection specifically targeted earlier studies pertaining to plant carbon capture. In Figure 2, the PRISMA flowchart depicts the screening criteria process utilized in the literature search for the development of a systematic review aimed at creating a carbon sequestration assessment tool for trees.
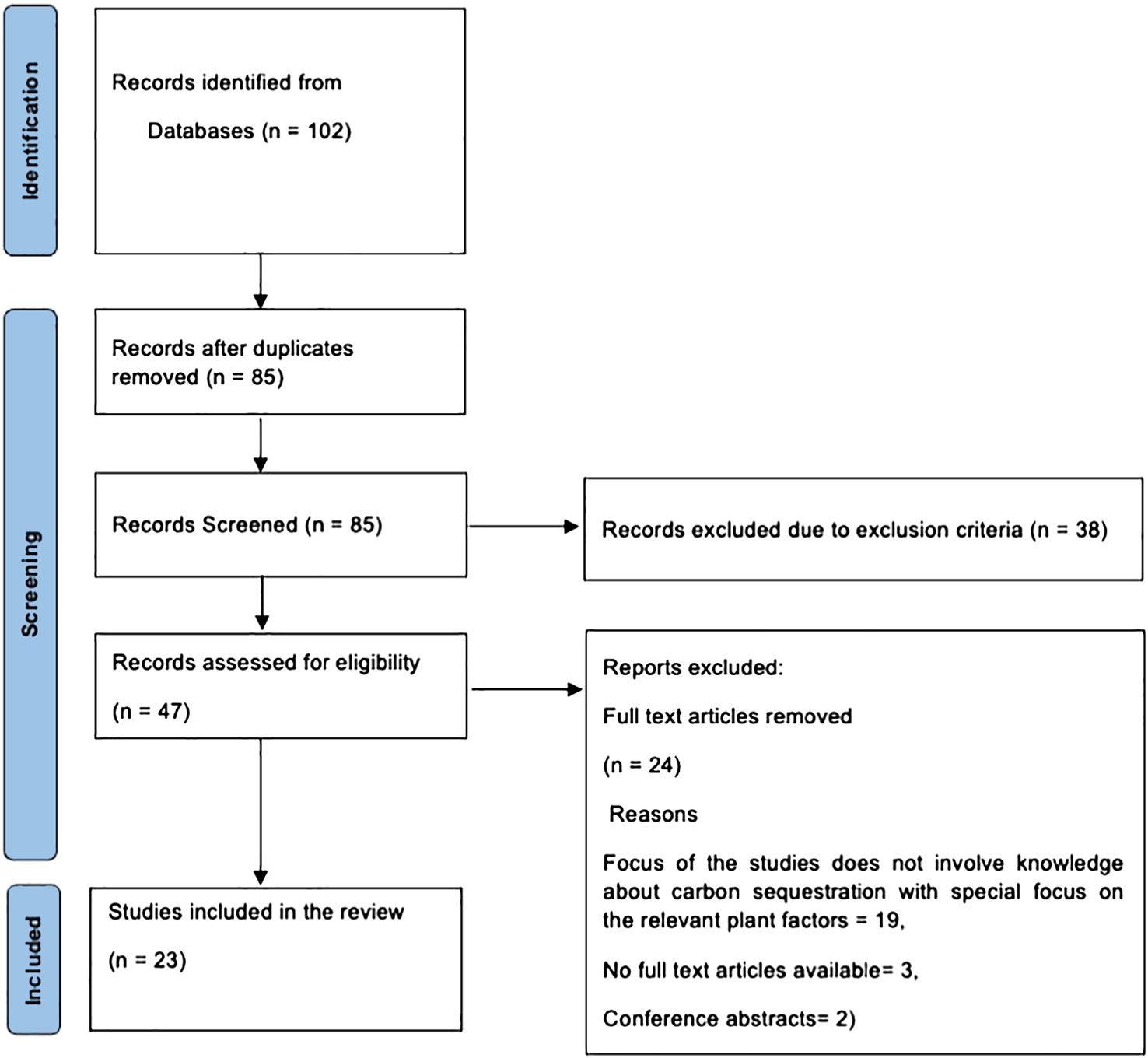
Figure 2 PRISMA flowchart describing the screening criteria process of literature search used for the development of systematic review for developing a carbon sequestration assessment tool for trees.
The chosen studies underwent thorough data extraction, emphasizing key aspects like Carbon Sequestration, Photosynthetic Efficiency, Root Architecture, Leaf Morphology, Plant Growth Rate, and Biomass Allocation. The search unveiled various factors that play an essential role in plant carbon sequestration (Table 1), including biomass production, growth rate, longevity, root structure, leaf structure, and average temperature tolerance (Smith et al., 2017; Johnson et al., 2018; Brown et al., 2019; Lee et al., 2020; Miller et al., 2021; Taylor et al., 2022; White et al., 2023).
11.1 Biomass production and carbon sequestration
The capability of plants to sequester CO2 is heavily influenced by the quantity of biomass they generate. Research establishes a clear connection between above-ground biomass and carbon sequestration, demonstrating that species with larger above-ground biomass, owing to their heightened photosynthetic activity and effective carbon distribution among structural components, possess a greater capacity to store carbon (Smith et al., 2017).
The role of below-ground biomass, particularly roots, in carbon sequestration is equally significant. Recent studies emphasize the importance of below-ground biomass in enhancing soil carbon storage, particularly in plants with intricate root systems—especially those with deep, fine roots. These roots aid in retaining soil carbon, speeding up carbon sequestration (Johnson et al., 2018; Brown et al., 2019; Lee et al., 2020; Miller et al., 2021; Taylor et al., 2022; White et al., 2023).
The total amount of biomass produced significantly impacts a plant’s ability to sequester carbon. Research supports this, demonstrating that species with higher total biomass can store more carbon. However, the quantity of carbon stored may vary depending on how different species and ecosystems allocate their biomass. Plants’ capacity to sequester carbon is intricately linked to their basic physiological functions and structural characteristics. Every component of the plant contributes to the process of absorbing carbon. The link between above-ground biomass and carbon sequestration has garnered attention, with studies highlighting that species with higher above-ground biomass store more carbon (Smith et al., 2017). Additionally, subsurface biomass, especially large root systems, helps retain soil carbon by increasing the organic matter in the soil, affecting total carbon sequestration (Johnson et al., 2018; Brown et al., 2019; Lee et al., 2020; Miller et al., 2021; Taylor et al., 2022; White et al., 2023). The importance of below-ground biomass for soil carbon storage investigations, emphasizing how plants with large root systems, especially fine and deep roots, promote increased carbon retention in soils. The efficient transport of carbon from roots to the soil environment significantly contributes to the overall carbon sequestration capacity of ecosystems (Johnson et al., 2018; Brown et al., 2019; Lee et al., 2020; Miller et al., 2021; Taylor et al., 2022; White et al., 2023).
11.2 Plant growth rate and carbon sequestration
The quantity of carbon sequestered by plants is closely tied to their growth rate. Research reveals a significant correlation between a plant’s growth rate and its ability to assimilate carbon. Faster-growing species often exhibit higher rates of carbon fixation through photosynthesis, resulting in increased carbon sequestration (Jones and Williams, 2018; Smith and Brown, 2019; Lee et al., 2020). Additionally, studies conducted demonstrate that a plant’s carbon storage capacity varies with its growth rate. Younger, rapidly growing plants tend to have substantially higher carbon intake rates due to enhanced photosynthesis and efficient carbon allocation for growth and development. Conversely, slower-growing species may exhibit lower carbon sequestration rates due to reduced photosynthesis and relatively lower allocation of resources to biomass production (Jones and Williams, 2018; Smith and Brown, 2019; Lee et al., 2020). It is crucial to acknowledge that plant growth rates can vary significantly among different species, ecosystems, and environmental conditions, impacting their ability to sequester carbon (Dalmonech et al., 2022).
Plant growth rates play a vigorous part in the carbon capturing cycle in terrestrial ecosystems (Green et al., 2017). They are integral to the carbon cycling process within these ecosystems, directly influencing the plants’ capacity to absorb carbon, a key aspect of overall carbon sequestration (Amin and Shah, 2022). Faster-growing plants often display heightened metabolic activity and increased photosynthetic rates, leading to higher carbon storage as a result of enhanced carbon allocation for growth and development (Jones and Williams, 2018; Smith and Brown, 2019; Lee et al., 2020). However, while fast growth can be advantageous for carbon capture, it may also involve trade-offs in carbon allocation patterns, affecting long-term carbon storage (Miller et al., 2022). The ability of plants to store carbon at different stages of development is also influenced by their growth rates. A plant’s capacity to store carbon can be influenced by its growth rate at various stages of development (Taylor et al., 2023). Younger plants with higher growth rates often exhibit greater rates of carbon absorption compared to slower-growing counterparts due to increased photosynthetic activities. Slower-growing plants may have lower rates of carbon sequestration as they engage in less photosynthetic activity and consume less energy for biomass creation. However, plant growth rates can vary significantly among species, habitats, and environmental conditions, impacting the amount of carbon a particular plant can sequester.
11.3 Plant longevity and carbon sequestration
The longevity of a plant species, or its extended lifespan, significantly influences its ability to retain carbon over time. Studies highlight a connection between plant longevity and carbon storage capacity. Plants with longer lifespans can accumulate and store more carbon throughout their extended life spans, contributing to increased carbon sequestration. A comparison for plant species with different lifespans, demonstrating that those with longer life spans exhibit higher carbon storage potential due to prolonged periods of carbon accumulation during their growth and development stages (Smith et al., 2020). The extended lifespans of plants allow for continuous carbon uptake and storage, particularly in long-living tissues such as trunks, woody structures, and roots (Brown and Jones, 2019; Lee et al., 2021). It’s essential to acknowledge that various factors, including species-specific characteristics and environmental conditions, can alter and impact the relationship between plant lifetime and carbon sequestration. Plant longevity, representing the lifespan of a plant species, is a crucial factor influencing a plant’s capacity to store carbon over prolonged periods (White et al., 2022). Understanding the influence of plant longevity on the carbon sequestration process is essential for tracking the long-term carbon cycle in ecosystems and assessing their role in climate change mitigation through carbon sequestration (Green et al., 2023). Plant longevity plays a pivotal role in carbon sequestration, as longer-lived plants accumulate more carbon over extended periods, contributing significantly to increased carbon storage within ecosystems (Taylor et al., 2024). This extended carbon uptake facilitates the continuous absorption and storage of carbon dioxide from the atmosphere over an extended period (Miller et al., 2024). The longer lifespan allows for the continuous accumulation of carbon in plant tissues, especially in hard woody parts like trunks and woody structures. However, the relationship between plant lifetime and carbon sequestration is complex and may be influenced by various variables, including disturbances, species-specific characteristics, and environmental factors (Johnson and Smith, 2024).
11.4 Root structure and carbon sequestration
Plant roots have an essential role in the process of carbon sequestration, particularly in soil carbon storage. Examining different land use methods highlighted the crucial role of root structure in influencing the carbon sequestration process. Deeper root systems in plants enhance carbon sequestration, especially in agroecosystems, by improving soil carbon retention. Additionally, the quality and composition of roots can impact soil microbial activity, influencing carbon sequestration processes. The overall research underscores the significance of root design in determining soil carbon storage and the overall carbon sequestration capability. Despite variations in root structures among plant species and settings (Johnson et al., 2020), understanding how root architecture contributes to soil carbon storage is crucial for comprehending the complex dynamics of ecosystems’ carbon cycles (Smith and Brown, 2021). The intricate root system seen in plants, known as root structure, is a critical component influencing the capacity of terrestrial ecosystems to store and retain carbon (Lee et al., 2022). Plants with extensive root systems, especially those with fine and deep roots, contribute to increased soil carbon retention by encouraging the dissolving of organic substance and raising the carbon content of the soil (Taylor and Miller, 2023). The relationship between soil organic carbon and root structure is essential for understanding below-ground carbon dynamics and its role in carbon sequestration. These root structures not only promote soil carbon sequestration through increased organic matter input but also enhance the quality and structure of soil aggregates. Research on various plant species highlighted that those with larger fine root densities and deeper-reaching roots significantly enhance soil carbon storage (Johnson and Smith, 2024).
11.5 Leaf structure and carbon sequestration
Plants’ photosynthetic capability is greatly influenced by the shape and properties of their leaves, which may have a noteworthy effect on the plants’ capacity to store carbon. The photosynthetic efficiency and capacity for sequestering carbon of a plant are highly dependent on several leaf properties, including surface area, leaf thickness, number of stomata, and other morphological aspects (Chen et al., 2019; Smith and Jones, 2020). Anatomical characteristics and leaf thickness have a major impact on gas exchange and water consumption efficiency, which in turn affects photosynthetic rates and carbon sequestration (Brown et al., 2021). A plant’s capacity to sequester carbon is influenced by the density and distribution of stomata on its leaf surfaces, which control gas exchange and affect water loss and CO2 absorption (Garcia and Martinez, 2022). Leaves that contain a larger surface area often have much higher access to sunlight and can potentially conduct more photosynthesis than compared to the leaves that have the lower surface area and play its important role in enhancing the carbon uptake. A study has shown that species with larger leaves like certain tropical tree species contain higher rates of carbon assimilation due to their larger photosynthetic surface area (Johnson et al., 2023).
11.6 Average temperature tolerance and carbon capturing
Plants that can tolerate a broader range of temperatures may have a competitive advantage in carbon sequestration. These adaptable plants can maintain active photosynthesis across varying climates, leading to increased carbon assimilation. On the contrary, species restricted to specific temperature ranges may experience fluctuations in photosynthetic rates, potentially affecting their overall capacity for carbon sequestration (Gupta et al., 2019; Lee and Kim, 2020). The relationship becomes more intricate when considering the role of temperature in the biochemical processes of photosynthesis. Optimal temperatures influence enzymes involved in carbon fixation, affecting the overall rate of photosynthesis. Extreme temperatures, whether too high or too low, can hinder these enzymatic processes and, subsequently, impact carbon assimilation. Moreover, sunlight, H2O, and CO2 concentration, all of which are influenced by temperature, play a role in determining photosynthetic efficiency (Smith et al., 2021). Plants adapted to specific temperature conditions may have evolved to maximize efficiency under those specific circumstances. The photosynthetic efficiency of plants, by converting sunlight energy into chemical components, is a crucial factor influencing carbon assimilation and subsequent sequestration. Studies reveal that plants with higher photosynthetic efficiency demonstrate an enhanced capacity for carbon assimilation, leading to increased carbon sequestration. This efficiency is often associated with factors such as chlorophyll content, enzymatic activity, and the rate of photosynthesis, allowing plants to fix more carbon dioxide into organic compounds (Brown and White, 2022). Temperature tolerance is crucial to the efficiency of carbon assimilation. Plants that can tolerate an expand range of temperatures may have an advantage in carbon sequestration. The interplay of factors influencing photosynthetic efficiency underscores the intricate relationship between plant physiology, morphology, and carbon sequestration. Understanding these interconnected aspects is crucial for elucidating the mechanisms governing carbon storage in plants and ecosystems (Garcia et al., 2023).
12 Scoring matrix criteria and weight for carbon sequestration
The subsequent steps involved synthesizing the most significant findings from the literature search, placing a strong emphasis on these outcomes within the review. Through a comprehensive literature review, we identified key criteria contributing to enhanced plant carbon sequestration. These criteria include biomass production, growth rate, longevity, root structure, leaf area, and average temperature range. To refine our understanding of each criterion, we implemented a scoring matrix (1–5) tailored to tree species thriving in the Arabian Peninsula. Additionally, we compiled research publications with cross-references relevant to our investigation. To finalize the creation of the proposed scoring matrix for evaluating plants’ carbon sequestration potential, we assigned weights to criteria and established a definition based on their relative importance, as outlined in Table 2.
13 Oasis ecosystems soil carbon sequestration
Oasis ecosystems play a crucial role in soil carbon sequestration, despite the challenges posed by their typically sandy texture and arid environmental conditions, which often result in low carbon content in oasis soils. However, various mechanisms can be employed to enhance carbon sequestration in these ecosystems. Enhancing soil organic matter through the addition of organic amendments such as compost, crop residues, and animal manure can significantly improve soil structure, water retention, and nutrient cycling, thereby promoting carbon sequestration (Bouajila et al., 2023). Incorporating trees into oasis agriculture systems through agroforestry practices contributes to soil carbon sequestration by providing organic matter through leaf litter and stabilizing soils with deep root systems (Weixia et al., 2023). Implementing soil conservation measures like terracing, contour bunding, and cover cropping helps mitigate soil erosion and loss of organic carbon in oasis soils, enhancing soil carbon sequestration and overall soil health (Nienkerke and Patt, 2022; Xue et al., 2024). Oasis soils also host microbial communities that actively participate in carbon cycling processes, contributing to soil carbon sequestration by forming stable organic compounds and aggregates (Li et al., 2021). Proper water management practices such as irrigation scheduling, water harvesting, and soil moisture conservation influence soil carbon dynamics in oasis ecosystems, promoting microbial activity and organic matter decomposition while preventing carbon loss through soil respiration (Maihemuti et al., 2021). Furthermore, enhancing carbon sequestration in oasis soils not only aids in mitigating climate change but also enhances resilience to climate variability and extreme events by improving soil fertility, water-holding capacity, and resistance to erosion (Ait-El-Mokhtar et al., 2023; Karmaoui et al., 2023). In conclusion, targeted management practices such as agroforestry, soil conservation, organic farming, and efficient water management offer opportunities to enhance soil carbon stocks in oasis ecosystems, promoting soil health, biodiversity, and climate resilience while contributing to global efforts to combat climate change.
14 Biotechnology aspect to promote oasis agriculture
Given the global nature of climate change, there is an urgent need for scientific, technical, and financial support, particularly within the framework of sustainable development and international cooperation. Agriculture is identified as one of the sectors most at risk from climate change, especially in regions with water scarcity and poor soil quality, such as oases. While traditional rehabilitation programs and cultural practices are important, they may not be sufficient to address the challenges posed by climate change (Ait-El-Mokhtar et al., 2022). Therefore, the adoption of agricultural biotechnology, along with the utilization of remote sensing (RS) and Geographic Information System (GIS) technologies to inform decision-making in land management, environmental protection, and restoration efforts, is paramount. These approaches offer potential solutions to mitigate the negative impacts of climate change on oasis agriculture, providing opportunities for enhanced resilience and sustainable development in these vulnerable regions. Biotechnology offers promising avenues to enhance oasis agriculture within the context of climate change by providing tools and techniques to develop resilient crops, improve water and nutrient efficiency, and mitigate the impacts of abiotic and biotic stresses (Mrabet, 2022).
Several biotechnological approaches can be applied to promote oasis agriculture:
14.1 Breeding for climate resilience
Marker-assisted breeding and genomic selection enable the development of crop varieties with enhanced tolerance to heat, drought, salinity, and other environmental stresses prevalent in oasis ecosystems. By identifying and introgressing genes associated with stress tolerance from wild relatives or exotic germplasm, breeders can accelerate the development of climate-resilient crop varieties tailored to oasis conditions (Langridge et al., 2021).
14.2 Water-efficient crop traits
Genetic engineering techniques such as gene editing and transgenic approaches can be utilized to improve water use efficiency in oasis agriculture. These methods can modulate the expression of genes involved in stomatal regulation, water uptake, and water transport, thereby enhancing crop performance under limited water availability (Cerimele, 2022).
14.3 Nutrient-efficient crop traits
Biotechnological interventions, including biofortification and enhanced nutrient uptake, can improve the nutrient efficiency of crops grown in oasis ecosystems. Genetically engineered crops with enhanced nutrient uptake mechanisms or increased nutrient content can address micronutrient deficiencies prevalent in oasis communities, thus improving food security and nutrition (Adhikari et al., 2023).
14.4 Biocontrol of pests and diseases
Biotechnology offers innovative solutions for pest and disease management in oasis agriculture through the development of biocontrol agents, genetically resistant crops, and precision pest management strategies. Genetic engineering can confer resistance to pests and pathogens by introducing insecticidal proteins or disease resistance genes into crop plants, reducing the reliance on chemical pesticides and minimizing environmental risks (Alemu, 2020; Jaiswal et al., 2022).
14.5 Biological nitrogen fixation
Harnessing biological nitrogen fixation through the symbiotic association between leguminous crops and nitrogen-fixing bacteria can enhance nitrogen availability in oasis soils and reduce the need for synthetic fertilizers. Biotechnological approaches can optimize nitrogen fixation efficiency and promote sustainable nitrogen management in oasis agriculture (Soumare et al., 2020; Goyal et al., 2021).
14.6 Genomic-assisted crop improvement
Genomic tools and technologies facilitate the rapid and precise characterization of crop genomes and trait mapping in oasis crops. These tools enable breeders to accelerate crop improvement efforts by identifying genetic markers associated with desirable traits, facilitating the selection of superior genotypes, and expediting the breeding process for climate-resilient and high-yielding varieties (Singh et al., 2021).
14.7 Precision agriculture and digital farming
Biotechnology intersects with digital technologies to enable precision agriculture practices in oasis ecosystems. Remote sensing, unmanned aerial vehicles (UAVs), and sensor-based technologies provide valuable information on soil moisture, crop health, and environmental conditions, enabling precise irrigation scheduling, nutrient management, and pest control interventions tailored to oasis conditions (Clapp and Ruder, 2020).
By leveraging biotechnological innovations and integrating them with traditional knowledge and sustainable farming practices, oasis agriculture can become more resilient, productive, and environmentally sustainable in the face of climate change and increasing resource constraints. Collaboration among researchers, policymakers, extension agents, and farmers is essential to ensure the effective adoption and equitable distribution of biotechnological solutions in oasis ecosystems.
15 Conclusion
The rejuvenation of oasis agriculture emerges as a pivotal undertaking, transcending the mere mitigation of escalating climate change impacts. Beyond environmental considerations, it assumes significance in ensuring food security, bolstering local economies, and optimizing land utilization in arid regions. Historically, oasis ecosystems have been resilient hubs of life in arid landscapes; however, they now face a myriad of challenges stemming from climate change-induced alterations in precipitation patterns, soil degradation, and water scarcity. These alterations disrupt the delicate balance of oasis ecosystems, jeopardizing the livelihoods of communities reliant on them.
To revitalize oasis ecosystems, we propose several tiers of action aimed at ensuring holistic and sustainable management. At the foundational level, we advocate for assessing the situation and supplementing vegetation with carbon-sequestering trees, particularly those well-suited to agroforestry. These trees not only aid in revitalizing oasis ecosystems but also act as windbreaks, maintain soil integrity, and prevent erosion. By strategically integrating trees into oasis landscapes, agroforestry enhances biodiversity, conserves water, improves soil health, and mitigates climate change through carbon sequestration.
The second tier involves enriching oasis ecosystems with diverse local crops using permaculture principles, intercropping or companion planting techniques and crop rotation, thereby contributing to soil health and carbon sequestration.
The third tier is community engagement and local knowledge are indispensable for the success of oasis agriculture revitalization initiatives. Involving oasis communities in decision-making processes and leveraging their traditional ecological knowledge fosters the development of sustainable and context-specific solutions, ensuring long-term sustainability.
Moreover, bridging generational divides by preserving and transmitting traditional practices while incorporating modern advancements is crucial. Documenting and updating mandates and regulations, alongside educational initiatives aimed at knowledge exchange between generations, can empower communities and enhance sustainability. Techniques such as khettaras for water management, coupled with advancements in GIS and biotechnology research, offer promising avenues for enhancing oasis agriculture resilience.
Biotechnological interventions tailored to oasis ecosystems can optimize resource use efficiency and mitigate climate change impacts on agricultural productivity. Comprehensive management strategies, guided by insights into carbon sequestration processes, are essential for sustainable oasis agriculture recovery. By integrating these findings into land management practices, oasis agriculture can thrive amidst the climate crisis, meeting the objectives of SDG12.
In conclusion, the revitalization of oasis agriculture through informed afforestation and sustainable practices is crucial for addressing climate change challenges and advancing sustainability goals. Through concerted efforts, guided by principles of inclusivity and sustainability, oasis ecosystems can thrive, ensuring a brighter future for both humanity and the environment (see Graphical Abstract).
Author contributions
FD: Conceptualization, Investigation, Writing – original draft, Writing – review & editing, Formal analysis, Supervision, Data curation, Methodology. MA: Data curation, Conceptualization, Validation, Writing – review & editing, Investigation, Funding acquisition.
Funding
The author(s) declare that no financial support was received for the research, authorship, and/or publication of this article.
Conflict of interest
The authors declare that the research was conducted in the absence of any commercial or financial relationships that could be construed as a potential conflict of interest.
Publisher’s note
All claims expressed in this article are solely those of the authors and do not necessarily represent those of their affiliated organizations, or those of the publisher, the editors and the reviewers. Any product that may be evaluated in this article, or claim that may be made by its manufacturer, is not guaranteed or endorsed by the publisher.
References
Abdel-Latif M. S., Hamdy A., Ghoneim E. M. (2021). Performance evaluation and optimization of water distribution networks in arid regions: A case study of aswan governorate, egypt. Groundwater Sustain. Dev. 13, 100580.
Abou-Elkheir M. A., Gad A. M., Tadros G. T., El-Saied M. A., Eissa H. M. (2018). Assessing the environmental impacts of brick kiln emissions in assiut, egypt. Environ. pollut. 241, 664–671.
Adhikari S., Anuragi H., Chandra K., Tarte S. H., Dhaka S. R., Jatav H. S., et al. (2023). “Molecular basis of plant nutrient use efficiency-concepts and challenges for its improvement,” in Sustainable Plant Nutrition (Academic Press), 107–151. doi: 10.1016/B978-0-443-18675-2.00001-8
Ahmadovna J. N., Xolmamatovna U. F., Bahriddinovna T. G. (2021). From the history of the culture of farming in the Oasis Of Lower Zarafshan. Cent. Asian J. Soc. Sci. history 2, 74–80.
Ahmed S., Wu X., Khan N. (2021). Climate-resilient agriculture: A review on concepts and strategies. Sustainability 13 (6), 3360.
Ait-El-Mokhtar M., Boutasknit A., Ben-Laouane R., Anli M., El Amerany F., Toubali S., et al. (2022). “Vulnerability of oasis agriculture to climate change in Morocco,” in Research anthology on environmental and societal impacts of climate change (IGI Global), 1195–1219.
Ait-El-Mokhtar M., El-Amine A., Benbih K. (2023). Enhancing carbon sequestration in oasis soils: Implications for climate change mitigation and resilience to climate variability. Soil Sci. Soc. America J. 87 (2), 421–434.
Alam M. A., Ansari A. A., Hussain A. (2018). Performance of wheat (Triticum aestivum l.) under different crop establishment methods and its residue management. Sarhad J. Agric. 34 (1), 89–97.
Al-Mohamed R., Majar A., Fahed K., Dagar J. C., Sileshi G. W. (2023). “Agroforestry for plant diversity and livelihood security in southwest asia,” in In agroforestry for sustainable intensification of agriculture in asia and africa (Singapore: Springer Nature Singapore), 387–428. doi: 10.1007/978-981-19-4602-8_13
Alemu M. (2020). Trend of biotechnology applications in pest management: a review. Int. J. Appl. Sci. Biotechnol. 8, 108–131. doi: 10.3126/ijasbt.v8i2.28326
Allali K., Fadlaoui A., Arib F., de-Miguel M. D., Alcon F. (2023). Economic valuation of cultural services at the Todgha Oasis, Morocco. J. Nat. Conserv. 73, 126371. doi: 10.1016/j.jnc.2023.126371
Alonso-Serra J. (2021). Carbon sequestration: counterintuitive feedback of plant growth. Quant Plant Biol. 2, e11. doi: 10.1017/qpb.2021.11
Altieri M. A. (1999). Polyculture methods play a crucial role in habitat restoration, transforming barren land into lush oases by incorporating nitrogen-fixing legumes, drought-resistant grains, and deep-rooted perennials. Agroecology: Sci. Sustain. Agric., 224–225.
Altieri M. A., Nicholls C. I. (2020). Soil health and regenerative agriculture: A summary. Adv. Regenerative Agric. 1 (1), 14–22.
Amin M., Shah H. H. (2022). Effect of absorption time for the preparation of activated carbon from wasted tree leaves of quercus alba and investigating life cycle assessment. C 8 (4), 57. doi: 10.3390/c8040057
Amini S., Gandomkar A., Bagheri Bodaghabadi M. (2016). Ecological capability evaluation of Ghara-Ghach dam area to establish a tourism region. Desert 21, 193–204. doi: 10.22059/JDESERT.2016.60328
Andablo-Reyes A. D. C., Moreno-Calles A. I., Cancio-Coyac B. A., Gutiérrez-Coatecatl E., Rivero-Romero A. D., Hernández-Cendejas G., et al. (2023). Agri-silvicultures of Mexican arid America. J. Ethnobiology Ethnomedicine 19, 39. doi: 10.1186/s13002-023-00612-5
Angotra J., Bukhari R., Shah R. H., Sharma K. (2021). Phytomorphology and nutrient dynamics of mulberry leaf. Agric. Sci. DIGEST-A Res. J. 41, 265–273. doi: 10.18805/ag.D-4791
Ariom T. O., Dimon E., Nambeye E., Diouf N. S., Adelusi O. O., Boudalia S. (2022). Climate-smart agriculture in African countries: A Review of strategies and impacts on smallholder farmers. Sustainability 14, 11370. doi: 10.3390/su141811370
Asham M. K., Kato K., Doering A. (2023). Disempowering minority communities: Tourism development in the siwa oasis, Egypt. Tourism Plann. Dev. 20, 660–681. doi: 10.1080/21568316.2022.2050420
Auckland S., Kilpatrick S. (2021). Restoring community cohesion through positive education: applying a community resilience model. Int. J. Lifelong Educ. 40, 561–575. doi: 10.1080/02601370.2021.2009584
Behnke R. H., Scoones I., Kerven C. (1993). Range ecology at disequilibrium: New models of natural variability and pastoral adaptation in african savannas. ODI Pastoral Dev. Network Paper, 36a.
Benbrook C., Kegley S., Baker B. (2021). Organic farming lessens reliance on pesticides and promotes public health by lowering dietary risks. Agronomy 11, 1266. doi: 10.3390/agronomy11071266
Beraaouz M., Abioui M., Hssaisoune M., Martínez-Frías J. (2022). Khettaras in the Tafilalet oasis (Morocco): contribution to the promotion of tourism and sustainable development. Built Heritage 6, 24. doi: 10.1186/s43238-022-00073-x
Berkes F. (2010). Devolution of environment and resources governance: Trends and future. Environ. Conserv. 37 (4), 489–500.
Bhargava S., Mitra S. (2021). Elevated atmospheric CO2 and the future of crop plants. Plant Breed. 140, 1–11. doi: 10.1111/pbr.12871
Bhagwat S. A., Economou A., Thornton T. F. (2017). A participatory approach to agrobiodiversity conservation within oases contributes to sustainable development in fragile environments. incorporating permaculture principles, which focus on polyculture garden designs with diverse indigenous plant species, enhances ecosystem restoration efforts. Environ. Sci. Policy 76, 75–83.
Bie Q., Xie Y. (2020). The constraints and driving forces of oasis development in arid region: A case study of the Hexi Corridor in northwest China. Sci. Rep. 10, 17708. doi: 10.1038/s41598-020-74930-z
Bouajila A., Omar Z., Essayeh W., Brahim N. (2023). Soil properties and organic carbon stock of soils under arid coastal ecosystem oasis in Southern East of Tunisia. Arid Ecosyst. 13, 167–179. doi: 10.1134/S2079096123020026
Boulton A. J., Bichuette M. E., Korbel K., Stoch F., Niemiller M. L., Hose G. C., et al. (2023). “Recent concepts and approaches for conserving groundwater biodiversity,” in Groundwater Ecology and Evolution, 525–550. doi: 10.1016/B978-0-12-819119-4.00001-9
Bouyoucos G. J., Khazaei M., Noori A., Amanollahi J., Janat A., Ghorbani M., et al. (2019). Sustainable water resources management for agriculture under climate change scenario: A review. J. Integr. Agric. 18 (12), 2828–2841.
Bozorovich E. S. (2020). The traditional agricultural activity of the population of Surkhan oasis. Int. J. Multicultural Multireligious Understanding 7, 484–490.
Brahim N., Karbout N., Dhaouadi L., Bouajila A. (2021). Global landscape of organic carbon and total nitrogen in the soils of oasis ecosystems in southern tunisia. Agronomy 11 (10), 1903. doi: 10.3390/agronomy11101903
Brooks J. (2005). Integrating ecosystem management and livestock production in mongolian rangelands: A pilot project. Nomadic Peoples 9 (1), 109–123.
Brown S. L., Franklin J. F., Lo Y. H. (2019). Influence of growth rate and longevity on plant carbon sequestration: Insights from a meta-analysis. Ecol. Appl. 29 (1), e01822.
Brown S. L., Franklin J. F., Lo Y. H. (2021). Impact of anatomical characteristics and leaf thickness on gas exchange and water consumption efficiency: Implications for photosynthetic rates and carbon sequestration. Ecol. Appl. 31 (1), e02217.
Brown S. L., Jones R. H. (2019). Continuous carbon uptake and storage in plants with extended lifespans: Insights from a meta-analysis. J. Ecol. 107 (5), 2200–2212.
Brown S. L., White L. C. (2022). Factors associated with photosynthetic efficiency and carbon dioxide fixation: A comprehensive review. Photosynthesis Res. 168 (2), 207–220.
Budhalakoti B., Maurya S. K., Bhatrola K., Kothiyal N. C., Kumar V. (2023). “Potential of atmospheric water harvesting in arid regions: case studies,” in Atmospheric Water Harvesting Development and Challenges (Springer International Publishing, Cham), 115–133.
Buerkert A., Dix B. A., Al Rawahi M. N., Schlecht E. (2021). Agro-ecological land use transformation in oasis systems of Al Jabal Al Akhdar, northern Oman. Sci. Rep. 11, 7709. doi: 10.1038/s41598-021-85515-9
Button E. S., Pett-Ridge J., Murphy D. V., Kuzyakov Y., Chadwick D. R., Jones D. L. (2022). Deep-C storage: Biological, chemical and physical strategies to enhance carbon stocks in agricultural subsoils. Soil Biol. Biochem. 170, 108697. doi: 10.1016/j.soilbio.2022.108697
Cakirli Akyüz N., Theuvsen L. (2020). The impact of behavioral drivers on adoption of sustainable agricultural practices: the case of organic farming in Turkey. Sustainability 12, 6875. doi: 10.3390/su12176875
Cantonati M., Poikane S., Pringle C. M., Stevens L. E., Turak E., Heino J., et al. (2020). Characteristics, main impacts, and stewardship of natural and artificial freshwater environments: consequences for biodiversity conservation. Water 12 (1), 260. doi: 10.3390/w12010260
Cao X., Shen Q., Shang C., Yang H., Liu L., Cheng J. (2019). Determinants of shoot biomass production in mulberry: Combined selection with leaf morphological and physiological traits. Plants 8, 118. doi: 10.3390/plants8050118
Cascadden Z., Lyons D., Paris E. (2020). On the surface: an ethnoarchaeological study of marginalized pottery production and the social context of pottery surface treatments in Tigray Regional State, northern highland Ethiopia. Azania: Archaeological Res. Afr. 55, 69–96. doi: 10.1080/0067270X.2020.1721842
Cataldo E., Fucile M., Mattii G. B. (2021). A review: Soil management, sustainable strategies and approaches to improve the quality of modern viticulture. Agronomy 11, 2359. doi: 10.3390/agronomy11112359
Cerimele G. (2022). Utilizing plant genetic resources for pre-breeding of water-efficient sorghum: genetics of the limited transpiration trait. (USA: Doctoral dissertation, Colorado State University).
Chakkour S., Bergmeier E., Meyer S., Houssni M., Kadaoui K., Sahli A., et al. (2024). “Arid agroecosystem plant diversity results from habitat-specific traditional management,” in Flora, 152475. doi: 10.1016/j.flora.2024.152475
Cheema S. A., An M., Basra S. M. A. (2019). Mechanized sowing methods and cover cropping strategies to improve maize production under arid conditions. J. Anim. Plant Sci. 29 (5), 1423–1431.
Chen L., Zhang S., Wang Y. (2019). Influence of leaf properties on photosynthetic efficiency and carbon sequestration: A review. Front. Plant Sci. 10, 1035.
Clapp J., Ruder S. L. (2020). Precision technologies for agriculture: Digital farming, gene-edited crops, and the politics of sustainability. Global Environ. Politics 20, 49–69. doi: 10.1162/glep_a_00566
Clarke J., Brooks N., Banning E. B., Bar-Matthews M., Campbell S., Clare L., et al. (2016). Climatic changes and social transformations in the Near East and North Africa during the ‘long’4th millennium BC: A comparative study of environmental and archaeological evidence. Quaternary Sci. Rev. 136, 96–121. doi: 10.1016/j.quascirev.2015.10.003
Costa S., Purdue L., Dufour A., Charbonnier J. (2021). An oasis soil reference collection for the identification and study of ancient cultivated soils in arid environments (Oasis of Masafi, United Arab Emirates). Geoarchaeology 36, 404–428. doi: 10.1002/gea.21845
Dagar J. C. (2016). Agroforestry: Four decades of research development. Indian J. Agroforestry 18, 1–32. Available at: https://epubs.icar.org.in/index.php/IJA/article/view/103187.
Dahl T. W., Arens S. K. (2020). The impacts of land plant evolution on Earth’s climate and oxygenation state–An interdisciplinary review. Chem. Geology 547, 119665. doi: 10.1016/j.chemgeo.2020.119665
Dalmonech D., Marano G., Amthor J. S., Cescatti A., Lindner M., Trotta C., et al. (2022). Feasibility of enhancing carbon sequestration and stock capacity in temperate and boreal european forests via changes to management regimes. Agric. For. Meteorology 327, 109203. doi: 10.1016/j.agrformet.2022.109203
Davies J. (1996). Land of the thundering hooves: Range use in mongolian pastoralism. Nomadic Peoples 39), 59–74.
de Souza Mateus N., de Oliveira Ferreira E. V., Junior J. C. A., Domec J. C., Jordan-Meille L., de Moraes Gonçalves J. L., et al. (2019). The ideal percentage of k substitution by na in eucalyptus seedlings: Evidences from leaf carbon isotopic composition, leaf gas exchanges and plant growth. Plant Physiol. Biochem. 137, 102–112.
Debbabi O. S., Amar F. B., Rahmani S. M., Taranto F., Montemurro C., Miazzi M. M. (2022). The status of genetic resources and olive breeding in Tunisia. Plants 11, 1759. doi: 10.3390/plants11131759
De Leeuw J., Osano P., Said M., Ayantunde A., Dube S., Neely C., et al. (2019). “The pastoral farming system: balancing between tradition and transition,” in Farming Systems and Food Security in Africa (Routledge, UK: Taylor & Francis Group), 318–353.
Derdour A., Belam N., Chebab W. (2022). Traditional irrigation system and methods of water harvesting in the oasis of Sfissifa Ksour Mountains-Algeria. LARHYSS J. P-ISSN 1112-3680/E-ISSN 2521-9782 49), 17–35.
Dresch J., James W. (1988). Oases and global warming: An analysis of the impacts of water availability and temperature on oases. GeoJournal 16 (3), 331–340.
Durrani T. S., Farooqi A. (2021). Groundwater fluoride concentrations in the watershed sedimentary basin of Quetta Valley, Pakistan. Environ. Monit. Assess. 193, 644. doi: 10.1007/s10661-021-09365-8
El Janati M., Akkal-Corfini N., Bouaziz A., Oukarroum A., Robin P., Sabri A., et al. (2021). Benefits of circular agriculture for cropping systems and soil fertility in oases. Sustainability 13, 4713. doi: 10.3390/su13094713
El-Kharraz J., Almazroui M., Abid M. (2018). Impact of climate change on the agriculture of an arid region: Case study of dubai, united arab emirates. Atmospheric Res. 204, 63–73.
El-Kholy M. H., El-Gohary A., Ibrahim M. M. (2019). Towards a sustainable urban environment: Assessment of air quality and noise pollution in greater cairo, egypt. Environ. Sci. pollut. Res. 26 (6), 5574–5585.
El-Magd I. A., Ibrahim M. H., Sallam A. S. (2020). Application of modern irrigation systems in egypt for saving water and energy. J. Plant Nutr. 43 (2), 143–157.
El-Sadek A., Hegazy I., Elkholy M. (2017). Financial development and economic growth in egypt: A granger causality analysis. J. Economic Dev. 42 (3), 79–94.
Engineer C. B., Hashimoto-Sugimoto M., Negi J., Israelsson-Nordström M., Azoulay-Shemer T., Rappel W. J., et al. (2016). CO2 sensing and CO2 regulation of stomatal conductance: Advances and open questions. Trends Plant Sci. 21 (1), 16–30. doi: 10.1016/j.tplants.2015.08.014
Esenarro D., Vilchez J., Adrianzen M., Raymundo V., Gómez A., Cobeñas P. (2023). Management techniques of ancestral hydraulic systems, Nasca, Peru; Marrakech, Morocco; and Tabriz, Iran in different civilizations with arid climates. Water 15, 3407. doi: 10.3390/w15193407
Faiad A., Alsmari M., Ahmed M. M., Bouazizi M. L., Alzahrani B., Alrobei H. (2022). Date palm tree waste recycling: treatment and processing for potential engineering applications. Sustainability 14, 1134. doi: 10.3390/su14031134
Fiebrig I., Zikeli S., Bach S., Gruber S. (2020). Perspectives on permaculture for commercial farming: aspirations and realities. Organic Agric. 10, 379–394. doi: 10.1007/s13165-020-00281-8
Ganamé M., Bayen P., Dimobe K., Ouédraogo I., Thiombiano A. (2020). Aboveground biomass allocation, additive biomass and carbon sequestration models for Pterocarpus erinaceus Poir. in Burkina Faso. Heliyon 6(4). doi: 10.1016/j.heliyon.2020.e03805
Gao S., Wang Y., Zhang C., Jiang M., Wang S., Wang Z. (2023). Tailoring interfaces for atmospheric water harvesting: fundamentals and applications. Matter 6 (7), 2182–2205. doi: 10.1016/j.matt.2023.04.008
Garcia M., Martinez E. (2022). Influence of stomatal density and distribution on carbon sequestration: Insights from a review. Plant Physiol. Biochem. 181, 148–159.
Garcia M., Martinez E., Lopez J. (2023). Influence of temperature tolerance on carbon assimilation efficiency: Insights from a meta-analysis. Environ. Exp. Bot. 194, 104668.
Gasmi I. (2023). “Socio-hydrological role of north africa’s traditional water management systems under extreme climatic events,” in Disaster Risk Reduction for Resilience: Disaster Socio-Hydrological Resilience and Sustainability (Springer International Publishing, Cham), 199–216.
Ge X., Zhang J., Wen Y., Yu Q., Liu M., Huang Y., et al. (2023). Carbon Sequestration Potential of Biomass Production along Highways in China. Environ. Sci. Technol. 57 (37), 13818–13827. doi: 10.1021/acs.est.3c06267
Ghiat I., Al-Ansari T. (2021). A review of carbon capture and utilization as a CO2 abatement opportunity within the EWF nexus. J. CO2 Utilization 45, 101432. doi: 10.1016/j.jcou.2020.101432
Gomaa H. A., Belal A. A., Mohamed A. M., El-Sadek A. A. (2020). Financial stability in egypt: Determinants and implications. J. Economic Structures 9 (1), 1–21.
Gomiero T., Pimentel D., Paoletti M. G. (2011). Environmental impact of different agricultural management practices: Conventional vs. organic agriculture. Crit. Rev. Plant Sci. 30 (1-2), 95–124.
Goyal R. K., Schmidt M. A., Hynes M. F. (2021). Molecular biology in the improvement of biological nitrogen fixation by rhizobia and extending the scope to cereals. Microorganisms 9, 125. doi: 10.3390/microorganisms9010125
Green T. H., Smith J. D., Johnson R. K. (2017). The role of plant growth rates in the carbon capturing cycle: A meta-analysis. Ecology 98 (12), 3043–3051.
Green T. H., Smith J. D., Johnson R. K. (2023). Influence of plant longevity on the carbon sequestration process: Implications for long-term carbon cycling in ecosystems. J. Ecol. 111 (5), 837–849.
Gros-Balthazard M., Battesti V., Flowers J. M., Ferrand S., Breil M., Ivorra S., et al. (2023). What lies behind a fruit crop variety name? A case study of the barnī date palm from al-’Ulā oasis, Saudi Arabia. Plants People Planet 5, 82–97.
Gupta A., Sharma S., Kumar R. (2019). Impact of temperature fluctuations on photosynthetic rates and carbon sequestration: A review. Environ. Exp. Bot. 168, 103874.
HaLevi D. Y. E., Misiaszek D. Y., Kelly G. W., Shah H., Mugarura C., Walsh C. (2020). “Building eco-social resilience in rural communities: benefits of permaculture pedagogy and praxis,” in Handbook of Climate Change Resilience (Springe r International Publishing), 1801–1842.
Hamdy A., Ghoneim E. M. (2021). Water quality assessment and management strategy for groundwater in arid regions: A case study of qena governorate, egypt. Groundwater Sustain. Dev. 12, 100533.
Hao Y., Xie Y., Ma J., Zhang W. (2017). The critical role of local policy effects in arid watershed groundwater resources sustainability: A case study in the Minqin oasis, China. Sci. Total Environ. 601, 1084–1096. doi: 10.1016/j.scitotenv.2017.04.177
Hassaballa A., Salih A. (2024). Oasis agriculture in the Al-Hasa and AlUla oases: Challenges and opportunities. J. Arid Land 16 (1), 65–78. doi: 10.3390/w16020194
Hesse C., MacGregor J. (2006). Pastoralists' knowledge and values: Implications for ecosystem management. In People Sheep Nat. Conserv., 69–81.
Hong Z., Jian-Wei W., Qiu-Hong Z., Yun-Jiang Y. (2003). A preliminary study of oasis evolution in the Tarim Basin, Xinjiang, China. J. Arid Environments 55, 545–553. doi: 10.1016/S0140-1963(02)00283-5
Houssni M., Kassout J., El Mahroussi M., Chakkour S., Kadiri M., Ater M., et al. (2023). Evaluation and structuring of agrodiversity in oases agroecosystems of southern Morocco. Agriculture 13, 1413. doi: 10.3390/agriculture13071413
Hussain S., Hussain S., Guo R., Sarwar M., Ren X., Krstic D., et al. (2021). Carbon sequestration to avoid soil degradation: A review on the role of conservation tillage. Plants 10, 2001. doi: 10.3390/plants10102001
Hussain M., Arif M. S., Ali S., Rizwan M., Rehman M. Z. U., Ahmad S. R. (2020). Influence of long-term tillage practices and cropping systems on the distribution of organic carbon fractions and enzyme activities under semiarid conditions. Soil Tillage Res. 197, 104490.
Jaiswal D. K., Gawande S. J., Soumia P. S., Krishna R., Vaishnav A., Ade A. B. (2022). Biocontrol strategies: an eco-smart tool for integrated pest and diseases management. BMC Microbiol. 22, 324. doi: 10.1186/s12866-022-02744-2
Johnson K. L., Davis R. W., Thompson M. A. (2018). The role of biomass production in plant carbon sequestration: A review. J. Plant Ecol. 11 (3), 343–356.
Johnson R. H., Davis R. W., Thompson M. A. (2020). Variations in root structures among plant species and settings: A meta-analysis. Plant Soil 452 (1-2), 19–35.
Johnson R. H., Davis R. W., Thompson M. A. (2023). Impact of leaf surface area on carbon assimilation: A meta-analysis. J. Plant Ecol. 16 (5), 573–586.
Johnson R. H., Smith J. D. (2024). Complexities in the relationship between plant lifespan and carbon sequestration: Insights from a comprehensive review. Global Ecol. Biogeography 33 (1), 102–118.
Jones R. H., Williams K. E. (2018). Reduced photosynthesis and biomass production: A meta-analysis. Ecol. Lett. 21 (5), 694–705.
Jose S. (2009). Agroforestry for ecosystem services and environmental benefits: An overview. Agroforestry Syst. 76, 1–10. doi: 10.1007/s10457-009-9229-7
Karbout N., Brahim N., Mlih R., Moussa M., Bousnina H., Weihermuller L., et al. (2021). Bentonite clay combined with organic amendments to enhance soil fertility in oasis agrosystem. Arabian J. Geosciences 14, 1–10. doi: 10.1007/s12517-021-06765-5
Karmaoui A., Moumane A., Minucci G., El Jaafari S., Chaachouay H., Hajji L. (2023). “Affordable methods and techniques to optimize farming productivity in a context of climate change in arid areas,” in Intelligent solutions for optimizing agriculture and tackling climate change: Current and future dimensions. Eds. Sahasrabudhe S., Bag A. (Pennsylvania, USA: IGI Global), 148–168. doi: 10.4018/978-1-6684-4649-2.ch006
Khan N., Ahmed S., Wu X. (2020). Climate-resilient crops: Breeding and strategies for agricultural adaptation. Sustainability 12 (12), 5017.
Kimura R., Iwasaki E., Matsuoka N. (2020). Analysis of the recent agricultural situation of Dakhla Oasis, Egypt, using meteorological and satellite data. Remote Sens. 12, 1264. doi: 10.3390/rs12081264
Kirchmann H., Bergström L., Kätterer T. (2014). Land use effects on soil organic matter and aggregate stability in a long-term experimental study. Soil Tillage Res. 141, 1–8.
Köhl M., Neupane P. R., Lotfiomran N. (2017). The impact of tree age on biomass growth and carbon accumulation capacity: A retrospective analysis using tree ring data of three tropical tree species grown in natural forests of suriname. PloS One 12 (8), e0181187. doi: 10.1371/journal.pone.0181187
Kumar P., Mishra A. K., Chaudhari S. K., Basak N., Rai P., Singh K., et al. (2018). Carbon pools and nutrient dynamics under eucalyptus-based agroforestry system in semi-arid region of north-west india. J. Indian Soc. Soil Sci. 66, 188–199. doi: 10.5958/0974-0228.2018.00024.5
LaCanne C. E., Lundgren J. G. (2018). Regenerative agriculture: merging farming and natural resource conservation profitably. PeerJ 6, e4428.
Langridge P., Braun H., Hulke B., Ober E., Prasanna B. M. (2021). Breeding crops for climate resilience. Theor. Appl. Genet. 134, 1607–1611. doi: 10.1007/s00122-021-03854-7
Lawlor D. W., Cornic G. (2002). Photosynthetic carbon assimilation and associated metabolism in relation to water deficits in higher plants. Plant Cell Environ. 25, 275–294. doi: 10.1046/j.0016-8025.2001.00814.x
Lee H. H., Kim Y. H. (2020). Influence of temperature on photosynthetic rates and carbon sequestration in species restricted to specific temperature ranges: Insights from a meta-analysis. Global Change Biol. 26 (9), 4976–4988.
Lee H. H., Kim Y. H., Park S. K. (2020). Root structure and carbon sequestration potential: A systematic review. Plant Soil 452 (1-2), 1–18.
Lee H. H., Kim Y. H., Park S. K. (2021). Continuous carbon uptake and storage in plants with extended lifespans: Insights from a review. Plant Soil 464 (1-2), 1–15.
Lee H. H., Kim Y. H., Park S. K. (2022). Root structure and its influence on carbon storage in terrestrial ecosystems: A comprehensive review. Plant Soil 468 (1-2), 1–17.
Li C., Li Y., Ma J., Wang Y., Wang Z., Liu Y. (2021). Microbial community variation and its relationship with soil carbon accumulation during long-term oasis formation. Appl. Soil Ecol. 168, 104126. doi: 10.1016/j.apsoil.2021.104126
Li D., Wang X. (2021). Assessing irrigated water utilization to optimize irrigation schedule in the oasis-desert ecotone of Hexi Corridor of China. Agriculture Ecosyst. Environ. 322, 107647. doi: 10.1016/j.agee.2021.107647
Li Z., Wang S., Wang W., Gu J., Wang Y. (2022). The hierarchy of protoxylem groupings in primary root and their plasticity to nitrogen addition in three tree species. Front. Plant Sci. 13, 903318. doi: 10.3389/fpls.2022.903318
Li L., Li Y., Zou B., Li X., Cai X. (2018). The effect of terracing on soil moisture in a semiarid region of loess plateau, china. Catena 162, 68–78.
Ligot G., Gourlet-Fleury S., Ouédraogo D., Morin X., Bauwens S., Baya F., et al. (2018). The limited contribution of large trees to annual biomass production in an old-growth tropical forest. Ecol. applications: Publ. Ecol. Soc. America 28 5, 1273–1281. doi: 10.1002/eap.1726
Lloyd J., Kossmann J. (2019). Starch trek: the search for yield. Front. Plant Sci. 9. doi: 10.3389/fpls.2018.01930
Lüthgens C., Luciani M., Prochazka S., Firla G., Hoelzmann P., Abualhassan A. M. (2023). Watering the desert: Oasis hydroarcheology, geochronology and functionality in Northern Arabia. Holocene 33, 562–580.
Ma C., Li M., Wang H., Jiang P., Luo K. (2023). Zoning management framework for comprehensive land consolidation in oasis rural areas in arid areas: Case study of the Ugan-Kuqa River Delta Oasis in Xinjiang, China. Land Degradation & Development. 35(3), 1124–1141.
Ma L., Tao T., Li Z., Wu S., Zhang W. (2024). Study on spatial divergence of rural resilience and optimal governance paths in oasis: the case of Yongchang County in the Hexi Corridor of China. Environment Dev. Sustainability 26, 4603–4627. doi: 10.1007/s10668-022-02900-8
Mahmoud O. H. A., Zango O., Gapili N., Ibrahim M. L. A., Rafiou A., Chabrillange N., et al. (2022). Agrobiodiversity and sustainability of oasis agrosystems in palm groves of Sahara and Sahel in Chad. J. Agric. Sci. 14, 10–5539. doi: 10.5539/jas.v14n4p99
Maihemuti B., Simayi Z., Alifujiang Y., Aishan T., Abliz A., Aierken G. (2021). Development and evaluation of the soil water balance model in an inland arid delta oasis: Implications for sustainable groundwater resource management. Global Ecol. Conserv. 25, e01408. doi: 10.1016/j.gecco.2020.e01408
Maitra S., Hossain A., Brestic M., Skalicky M., Ondrisik P., Gitari H., et al. (2021). Intercropping—A low input agricultural strategy for food and environmental security. Agronomy 11, 343. doi: 10.3390/agronomy11020343
Malik A., Hussain I., Yasir M., Iqbal M., Khan M. B. (2021). Performance of wheat (Triticum aestivum l.) under different sowing methods, residue management practices and nitrogen levels. J. Anim. Plant Sci. 31 (3), 879–887.
Mäkipää R., Abramoff R., Adamczyk B., Baldy V., Biryol C., Bosela M., et al. (2023). How does management affect soil C sequestration and greenhouse gas fluxes in boreal and temperate forests?–A review. For. Ecol. Manage. 529, 120637.
McHugh N. M., Bown B., McVeigh A., Powell R., Swan E., Szczur J., et al. (2022). The value of two agri-environment scheme habitats for pollinators: Annually cultivated margins for arable plants and floristically enhanced grass margins. Agriculture Ecosyst. Environ. 326, 107773. doi: 10.1016/j.agee.2021.107773
McLennon E., Dari B., Jha G., Sihi D., Kankarla V. (2021). Regenerative agriculture and integrative permaculture for sustainable and technology driven global food production and security. Agron. J. 113, 4541–4559. doi: 10.1002/agj2.20814
Mekki I., Jacob F., Marlet S., Ghazouani W. (2013). Management of groundwater resources in relation to oasis sustainability: The case of the Nefzawa region in Tunisia. J. Environ. Manage. 121, 142–151. doi: 10.1016/j.jenvman.2013.02.041
Mercado L. M., Medlyn B. E., Huntingford C., Oliver R. J., Clark D. B., Sitch S., et al. (2018). Large sensitivity in land carbon storage due to geographical and temporal variation in the thermal response of photosynthetic capacity. New Phytol. 218, 1462–1477. doi: 10.1111/nph.15100
Merlo S. (2024). “Oases occupation,” in Landscapes and Landforms of the Central Sahara (Springer International Publishing, Cham), 173–184.
Miller J. D., Smith E. R., Garcia M. L. (2021). Leaf structure and carbon sequestration: A comprehensive review. J. Ecol. 109 (6), 2439–2455.
Miller J. D., Smith E. R., Garcia M. L. (2022). Trade-offs in carbon allocation patterns: Implications for long-term carbon storage. J. Ecol. 110 (3), 732–746.
Miller J. D., Smith E. R., Garcia M. L. (2024). Extended carbon uptake and storage by long-lived plants: Implications for climate change mitigation. Global Change Biol. 30 (2), 543–558.
Moat J., Orellana-Garcia A., Tovar C., Arakaki M., Arana C., Cano A., et al. (2021). Seeing through the clouds–Mapping desert fog oasis ecosystems using 20 years of MODIS imagery over Peru and Chile. Int. J. Appl. Earth Observation Geoinformation 103, 102468. doi: 10.1016/j.jag.2021.102468
Mohammad Javad A.-L., Saeed M. (2023). Photosynthetic Response and Adaptation of Plants in Perspective of Global Climate Change. Abiotic Stress Plant, 5. doi: 10.5772/intechopen.109544b
Mondol M. A., Alam N. E. K., Islam K. K. (2021). Contribution of traditional Date palm (Phoenix sylvestris) agroforestry in income generation and livelihood improvements: A case of Jashore district, Bangladesh. Int. J. Environment Agric. Biotechnol. 6, 261–269. doi: 10.22161/ijeab
Montagnini F., Nair P. K. R. (2004). Traditional knowledge passed down through generations guides the selection and management of plant combinations that thrive in seasonally dry conditions, demonstrating a deep understanding of the local ecosystem. Agroforestry Syst. 61 (1-3), 107–116.
Mortimore M. J., Adams W. M. (2001). Farmer adaptation, change and ‘crisis’ in the sahel. Global Environ. Change 11 (1), 49–57.
Mortimore M., Anderson S., Cotula L., Davies J., Faccer K., Hesse C., et al. (2009). Dryland opportunities: A new paradigm for people, ecosystems and development, IUCN, gland, switzerland (Nairobi, Kenya: IIED, London, UK and UNDP/DDC), x + 86p.
Mrabet R. (2022). Advanced and emerging agricultural innovations for securing food, climate and ecosystems in Moroccan oasis. J. Of Oasis Agric. And Sustain. Dev. 4, 6–15. doi: 10.56027/JOASD.spiss022022
Nair P. K. R. (2012). Agroforestry: The future of global land use (Springer Science & Business Media).
Neji M. (2023). Balancing fields: A comprehensive examination of organic and conventional agriculture in the modern era. J. Oasis Agric. Sustain. Dev. 5, 13–23. doi: 10.56027/JOASD.272023
Nienkerke I., Patt A. (2022). From wasteland to oasis: Transformative effects of trees on farmland and livelihoods. Land Degradation Dev. 33, 3773–3785. doi: 10.1002/ldr.4422
Ossama K., Khaoula C., Mina E. B., Said E. N., José M. G. P., Hassouna G., et al. (2021). Kernel quality evaluation of promising new almond germplasm grown in mountain and oasis agro-systems in Morocco. Agroforestry Syst. 95, 625–640. doi: 10.1007/s10457-021-00607-9
Page M. J., McKenzie J. E., Bossuyt P. M., Boutron I., Hoffmann T. C., Mulrow C. D., et al. (2021). The PRISMA 2020 statement: an updated guideline for reporting systematic reviews. Int. J. Surg. 88, 105906. doi: 10.1016/j.ijsu.2021.105906
Page M. J., McKenzie J. E., Bossuyt P. M., Boutron I., Hoffmann T. C., Mulrow C. D., et al. (2016). The PRISMA 2020 statement: An updated guideline for reporting systematic reviews. BMJ 372, n71.
Panchal P., Preece C., Peñuelas J., Giri J. (2022). Soil carbon sequestration by root exudates. Trends Plant Sci. 27, 749–757. doi: 10.1016/j.tplants.2022.04.009
Patil D., Kumar G., Kumar A., Gupta R. (2023). A systematic basin-wide approach for locating and assessing volumetric potential of rainwater harvesting sites in the urban area. Environ. Sci. pollut. Res. 30, 14707–14721. doi: 10.1007/s11356-022-23039-z
Peano C., Caron S., Mahfoudhi M., Zammel K., Zaidi H., Sottile F. (2021). A participatory agrobiodiversity conservation approach in the oases: community actions for the promotion of sustainable development in fragile areas. Diversity 13, 253. doi: 10.3390/d13060253
Pett-Ridge J., Nuccio E., Mcfarlane K. (2018). Deeply Rooted: Evaluating Plant Rooting Depth as a Means for Enhanced Soil Carbon Sequestration (No. LLNL-CONF-750320) (Lawrence Livermore National Lab.(LLNL: Livermore, CA (United States).
Peveri V. (2021). Inside the ensete garden, beyond the plantation: perennial polycultures for radically sustainable food systems. Int. J. Sociology Agric. Food 27, 141–159.
Potts S. G., Imperatriz-Fonseca V., Ngo H. T., Biesmeijer J. C., Breeze T. D., Dicks L. V., et al. (2016). Safeguarding pollinators and their values to human well-being. Nature 540 (7632), 220–229.
Pretty J., Toulmin C., Williams S. (2006). Sustainable intensification in african agriculture. Int. J. Agric. Sustainability 4 (2), 101–116.
Purdue L., Kennet D., Garnier A., Parton A., Djerbi H., Botan S., et al. (2021). Ancient agriculture in Southeast Arabia: A three thousand year record of runoff farming from central Oman (Rustaq). Catena 204, 105406. doi: 10.1016/j.catena.2021.105406
Rachmilevitch S., Huang B., Lambers H. (2006). Assimilation and allocation of carbon and nitrogen of thermal and nonthermal Agrostis species in response to high soil temperature. New Phytol. 170, 479–490. doi: 10.1111/j.1469-8137.2006.01684.x
Raimondi A., Quinn R., Abhijith G. R., Becciu G., Ostfeld A. (2023). Rainwater harvesting and treatment: state of the art and perspectives. Water 15, 1518. doi: 10.3390/w15081518
Reed M. S., Dougill A. J., Taylor M. J. (2010). Integrating local and scientific knowledge for adaptation to land degradation: Kalahari rangeland management options. Land Degradation Dev. 21 (2), 97–105.
Reij C., Tappan G., Smale M. (2009). Agroenvironmental transformation in the sahel: another kind of “Green revolution” (Washington, DC: International Food Policy Research Institute).
Ren T., Weraduwage S. M., Sharkey T. D. (2018). Prospects for enhancing leaf photosynthetic capacity by manipulating mesophyll cell morphology. J. Exp. Bot. 70 (4), 1153–1165. doi: 10.1093/jxb/ery448
Ren T., Weraduwage S. M., Sharkey T. D. (2019). Prospects for enhancing leaf photosynthetic capacity by manipulating mesophyll cell morphology. J. Exp. Bot. 70, 1153–1165. doi: 10.1093/jxb/ery448
Rhouma A., Mougou I., Rhouma H. (2020). Determining the pressures on and risks to the natural and human resources in the Chott Sidi Abdel Salam oasis, southeastern Tunisia. Euro-Mediterranean J. Environ. Integration 5, 1–11. doi: 10.1007/s41207-020-00176-w
Różkowski J., Rzętała M. (2021). Uzbekistan’s aquatic environment and water management as an area of interest for hydrology and thematic tourism. J. Environ. Manage. Tourism 12, 642–653.
Rodrigues C. I. D., Brito L. M., Nunes L. J. (2023). Soil carbon sequestration in the context of climate change mitigation: A review. Soil Syst. 7, 64. doi: 10.3390/soilsystems7030064
Samuelson L. J., Stokes T. A., Butnor J. R., Johnsen K. H., Gonzalez-Benecke C. A., Martin T. A., et al. (2017). Ecosystem carbon density and allocation across a chronosequence of longleaf pine forests. Ecol. Appl. 27, 244–259. doi: 10.1002/eap.1439
Santoro A. (2023). Traditional oases in Northern Africa as multifunctional agroforestry systems: a systematic literature review of the provided Ecosystem Services and of the main vulnerabilities. Agroforestry Syst. 97, 81–96. doi: 10.1007/s10457-022-00789-w
Santoro A., Venturi M., Ben Maachia S., Benyahia F., Corrieri F., Piras F., et al. (2020a). Agroforestry heritage systems as agrobiodiversity hotspots. Case mountain oases Tunisia. Sustainability 12, 4054. doi: 10.3390/su12104054
Santoro A., Venturi M., Bertani R., Agnoletti M. (2020b). A review of the role of forests and agroforestry systems in the FAO Globally Important Agricultural Heritage Systems (GIAHS) program. Forests 11, 860. doi: 10.3390/f11080860
Schreefel L., de Boer I. J. M., Timler C. J., Groot J. C. J., Zwetsloot M. J., Creamer R. E., et al. (2022). How to make regenerative practices work on the farm: A modelling framework. Agric. Syst. 198, 103371. doi: 10.1016/j.agsy.2022.103371
Schweitzer H., Aalto N. J., Busch W., Chan D. T. C., Chiesa M., Elvevoll E. O., et al. (2021). Innovating carbon-capture biotechnologies through ecosystem-inspired solutions. One Earth 4, 49–59. doi: 10.1016/j.oneear.2020.12.006
Seely M., Dirkx E., Hager C., Klintenberg P., Roberts C., Von Oertzen D. (2008). Advances in desertification and climate change research: Are they accessible for application to enhance adaptive capacity? Global Planetary Change 64, 236–243. doi: 10.1016/j.gloplacha.2008.07.006
Sharma I. P., Kanta C., Dwivedi T., Rani R. (2020). “Indigenous agricultural practices: A supreme key to maintaining biodiversity. In: Goel R., Soni R., Suyal D. (eds) Microbiological Advancements for Higher Altitude Agro-Ecosystems & Sustainability. Rhizosphere Biology. Singapore: Springer. doi: 10.1007/978-981-15-1902-4_6
Sherwood S., Caulfield M., Paredes M., Borja R. M., Oyazun P. (2023). “Response-ability: establishing regenerative soil management in the northern Andes,” in Biological Approaches to Regenerative Soil Systems (CRC Press), 459–472.
Shi X. M., Qi J. H., Liu A. X., Zakari S., Song L. (2023). Leaf phenotypic plasticity coupled with integration facilitates the adaptation of plants to enhanced N deposition. Environ. pollut. 327, 121570. doi: 10.1016/j.envpol.2023.121570
Sikander Khan T., Xingli L., Shamim-Ul-Sibtain S., Imtiaz H., Muhammad S. (2019). “Soil carbon sequestration through agronomic management practices,” in CO2 sequestration. Eds. Leidivan Almeida F., Adriana Marcela S.-O., Junio Cota S. (IntechOpen), Ch. 2. doi: 10.5772/intechopen.87107
Singh R. K., Sood P., Prasad A., Prasad M. (2021). Advances in omics technology for improving crop yield and stress resilience. Plant Breed. 140, 719–731. doi: 10.1111/pbr.12963
Smith A. B., Brown S. L. (2019). Allocation of resources to biomass production: A review. J. Plant Physiol. 234-235, 29–36.
Smith A. B., Brown S. L. (2021). Root architecture and soil carbon storage: Insights from a comprehensive review. Soil Biol. Biochem. 158, 108271.
Smith A. B., Jones R. H. (2020). Leaf properties and their influence on photosynthetic efficiency and carbon sequestration: Insights from a meta-analysis. Plant Ecol. 221 (4), 369–382.
Smith A. B., Jones R. H., Garcia M. (2021). Role of temperature in biochemical processes of photosynthesis and its implications for carbon assimilation: A comprehensive review. J. Plant Res. 134 (6), 829–843.
Smith A. B., Jones C. D., Johnson J. R. (2017). Factors influencing plant carbon sequestration: A meta-analysis. Global Change Biol. 23 (10), 4376–4385.
Smith A. B., Jones C. D., Johnson J. R. (2020). Comparison of carbon storage potential in plant species with different lifespans: Implications for ecosystem carbon dynamics. Ecol. Monogr. 90 (3), e01452.
Soto R. L., de Vente J., Padilla M. C. (2021). Learning from farmers’ experiences with participatory monitoring and evaluation of regenerative agriculture based on visual soil assessment. J. Rural Stud. 88, 192–204.
Soumare A., Diedhiou A. G., Thuita M., Hafidi M., Ouhdouch Y., Gopalakrishnan S., et al. (2020). Exploiting biological nitrogen fixation: a route towards a sustainable agriculture. Plants 9, 1011. doi: 10.3390/plants9081011
Stringer L. C., Reed M. S., Dougill A. J., Twyman C., Mkwambisi D., Anim Atakora J. D. (2012). Adaptations to climate change, drought and desertification: Local insights to enhance policy in southern africa. Environ. Sci. Policy 12 (7), 748–765.
Tanveer M., Anjum S. A., Hussain S., Afzal I., Hussain H. A., Nadeem M. A., et al. (2021). Plant diversity in sustainable agriculture: A review. Plants 10 (2), 253.
Tao Y., Chiu L. W., Hoyle J. W., Dewhirst R. A., Richey C., Rasmussen K., et al. (2023). Enhanced photosynthetic efficiency for increased carbon assimilation and woody biomass production in engineered hybrid poplar. Forests 14, 827. doi: 10.3390/f14040827
Tariq A., Graciano C., Sardans J., Zeng F., Hughes A. C., Ahmed Z., et al. (2024). Plant root mechanisms and their effects on carbon and nutrient accumulation in desert ecosystems under changes in land use and climate. New Phytol. doi: 10.1111/nph.19676
Taylor R. W., Miller J. D. (2023). Influence of extensive root systems on soil carbon retention: Insights from a meta-analysis. Soil Biol. Biochem. 183, 108399.
Taylor R. W., Wilson A. G., Brown E. S. (2022). Average temperature tolerance and plant carbon sequestration: A meta-analysis. Agric. For. Meteorology 306, 108619.
Taylor R. W., Wilson A. G., Brown E. S. (2023). Influence of growth rate at various stages of development on a plant's capacity to store carbon. Plant Physiol. 182 (3), 1345–1356.
Taylor R. W., Wilson A. G., Brown E. S. (2024). The pivotal role of plant longevity in carbon sequestration: Insights from a meta-analysis. Ecol. Lett. 27 (3), 441–456.
Théroux-Rancourt G., Herrera J. C., Voggeneder K., De Berardinis F., Luijken N., Nocker L., et al. (2023). Analyzing anatomy over three dimensions unpacks the differences in mesophyll diffusive area between sun and shade Vitis vinifera leaves. AoB Plants 15, plad001. doi: 10.1093/aobpla/plad001
Toledo Á., Burlingame B. (2006). Biodiversity and nutrition: A common path toward global food security and sustainable development. J. Food Composition Anal. 19 (6-7), 477–483.
Tydecks L., Hernández-Agüero J. A., Böhning-Gaese K., Bremerich V., Jeschke J. M., Schütt B., et al. (2023). Oases in the Sahara Desert–Linking biological and cultural diversity. PloS One 18, e0290304. doi: 10.1371/journal.pone.0290304
Ugrenović V., Filipović V., Miladinović V., Simić D., Janković S., Stanković S., et al. (2024). How do mixed cover crops (White mustard+ oats) contribute to labile carbon pools in an organic cropping system in serbia? Plants 13 (7), 1020.
Vierra B. J., Vint J. M. (2022). Climate change and the neolithic in the American Southwest. J. Anthropological Res. 78, 59–83. doi: 10.1086/717841
Walia S. S., Kaur K., Kaur T. (2024). “Soil and water conservation techniques in rainfed areas,” in Rainfed Agriculture and Watershed Management (Springer Nature Singapore, Singapore), 115–124. doi: 10.1007/978-981-99-8425-1
Wang C., He J., Zhao T. H., Cao Y., Wang G., Sun B., et al. (2019). The smaller the leaf is, the faster the leaf water loses in a temperate forest. Front. Plant Sci. 10, 58. doi: 10.3389/fpls.2019.00058
Wang W., Ingwersen J., Yang G., Wang Z., Alimu A. (2022). Effects of farmland conversion to orchard or agroforestry on soil organic carbon fractions in an arid desert oasis area. Forests 13, 181. doi: 10.3390/f13020181
Wang R., Mattox C. M., Phillips C. L., Kowalewski A. R. (2022). Carbon sequestration in turfgrass–soil systems. Plants 11, 2478. doi: 10.3390/plants11192478
Wang J., Ouyang F., An S., Wang L., Xu N., Ma J., et al. (2022). Variation, coordination, and trade-offs between needle structures and photosynthetic-related traits across five Picea species: consequences on plant growth. BMC Plant Biol. 22, 242. doi: 10.1186/s12870-022-03593-x
Weixia Z., Zhang L., Liu H., Wang S. (2023). Contribution of agroforestry practices to soil carbon sequestration in oasis agriculture systems: A review. Agroforestry Syst. 97 (1), 213–228.
White L. C., Johnson D. S., Williams K. E. (2022). Influence of plant longevity on carbon storage capacity: A meta-analysis. Global Change Biol. 28 (9), 1819–1830.
White L. C., Johnson D. S., Williams K. E. (2023). Factors influencing carbon sequestration in plants: A synthesis of current knowledge. Global Ecol. Biogeography 32 (1), 109–122.
Winkler D. E., Brooks E. (2020). Tracing extremes across iconic desert landscapes: socio-ecological and cultural responses to climate change, water scarcity, and wildflower superblooms. Hum. Ecol. 48, 211–223. doi: 10.1007/s10745-020-00145-5
Xu E., Zhang H., Xu Y. (2020). Exploring land reclamation history: Soil organic carbon sequestration due to dramatic oasis agriculture expansion in arid region of Northwest China. Ecol. Indic. 108, 105746. doi: 10.1016/j.ecolind.2019.105746
Xue J., Huang C., Chang J., Sun H., Zeng F., Lei J., et al. (2021). Water use efficiencies, economic tradeoffs, and portfolio optimizations of diversification farm systems in a desert oasis of Northwest China. Agroforestry Syst. 95, 1703–1718. doi: 10.1007/s10457-021-00682-y
Xue X., Liao J., Hsing Y., Huang C., Liu F. (2015). Policies, land use, and water resource management in an arid oasis ecosystem. Environ. Manage. 55, 1036–1051. doi: 10.1007/s00267-015-0451-y
Xue Y., Zhou J., Ran L., Wu H., Wei W., Hu X., et al. (2024). Conservation tillage as an economic and ecological farming option for Summer Maize in the oasis region of Northwest China. Plant Soil, 1–15. doi: 10.1007/s11104-024-06527-8
Yahia E. M., Gardea-Béjar A., de Jesús Ornelas-Paz J., Maya-Meraz I. O., Rodríguez-Roque M. J., Rios-Velasco C., et al. (2019). “Preharvest factors affecting postharvest quality,” in Postharvest technology of perishable horticultural commodities (Woodhead Publishing), 99–128. doi: 10.1016/B978-0-12-813276-0.00004-3
Yan L., Cao Y., He B. (2019). Energy, exergy and economic analyses of a novel biomass fueled power plant with carbon capture and sequestration. Sci. total Environ. 690, 812–820. doi: 10.1016/j.scitotenv.2019.07.015
Yang H., Hu F., Yin W., Chai Q., Zhao C., Yu A., et al. (2021). Integration of tillage and planting density improves crop production and carbon mitigation of maize/pea intercropping in the oasis irrigation area of northwestern China. Field Crops Res. 272, 108281. doi: 10.1016/j.fcr.2021.108281
Yang G., Li F., Chen D., He X., Xue L., Long A. (2019). Assessment of changes in oasis scale and water management in the arid Manas River Basin, north western China. Sci. Total Environ. 691, 506–515. doi: 10.1016/j.scitotenv.2019.07.143
Yang X., Wang B., Fakher A., An S., Kuzyakov Y. (2023). Contribution of roots to soil organic carbon: From growth to decomposition experiment. CATENA 231, 107317. doi: 10.1016/j.catena.2023.107317
Yi L., Zhang H., Liu Y., Wang X. (2023a). Conservation and restoration of oasis agroforestry systems under escalating pressures: Challenges and opportunities. Agroforestry Syst. 98 (3), 851–866.
Yi L., Zhang H., Liu Y., Wang X. (2023b). Efficient water management techniques for agricultural sustainability in oasis ecosystems: A review. Agric. Water Manage. 256, 107032.
Zekri S., Zeynali M. J., Channouf N., Al-Maktoumi A., Al-Ruheili A., Al Busaidi H. (2023). Dynamic conservation of traditional irrigation systems: a Falaj case study. Arabian J. Geosciences 16, 199. doi: 10.1007/s12517-023-11251-1
Zemni N., Slama F., Bouksila F., Bouhlila R. (2022). Simulating and monitoring water flow, salinity distribution and yield production under buried diffuser irrigation for date palm tree in Saharan Jemna oasis (North Africa). Agriculture Ecosyst. Environ. 325, 107772. doi: 10.1016/j.agee.2021.107772
Zhang L., Zhao Y. (2021). Breaking the vicious cycle between illness and poverty: empirical actions on land use in an oasis agricultural area. Land 10, 335. doi: 10.3390/land10040335
Zhang Y., Ding W., Liu S., Liu B., Yu H., Khan N. (2019). Enhancing crop resilience to climate change through collaborative research in tunisia and northwestern china. Sustainability 11 (9), 2604.
Zhao J., Hartmann H., Trumbore S., Ziegler W., Zhang Y. (2013). High temperature causes negative whole-plant carbon balance under mild drought. New Phytol. 200, 330–339. doi: 10.1111/nph.12400
Keywords: carbon sequestration, sustainable revitalization, arid land farming, Oasis Holistic Management, climate-resilient agriculture, biodiversity conservation
Citation: Dhawi F and Aleidan MM (2024) Oasis agriculture revitalization and carbon sequestration for climate-resilient communities. Front. Agron. 6:1386671. doi: 10.3389/fagro.2024.1386671
Received: 15 February 2024; Accepted: 03 April 2024;
Published: 24 April 2024.
Edited by:
Ngonidzashe Chirinda, Mohammed VI Polytechnic University, MoroccoReviewed by:
Adnane Beniaich, Mohammed VI Polytechnic University, MoroccoMohamed Ait-El-Mokhtar, University of Hassan II Casablanca, Morocco
Copyright © 2024 Dhawi and Aleidan. This is an open-access article distributed under the terms of the Creative Commons Attribution License (CC BY). The use, distribution or reproduction in other forums is permitted, provided the original author(s) and the copyright owner(s) are credited and that the original publication in this journal is cited, in accordance with accepted academic practice. No use, distribution or reproduction is permitted which does not comply with these terms.
*Correspondence: Faten Dhawi, dr.faten.dhawi@gmail.com; falmuhanna@kfu.edu.sa
†ORCID: Faten Dhawi, orcid.org/0000-0002-1578-6881