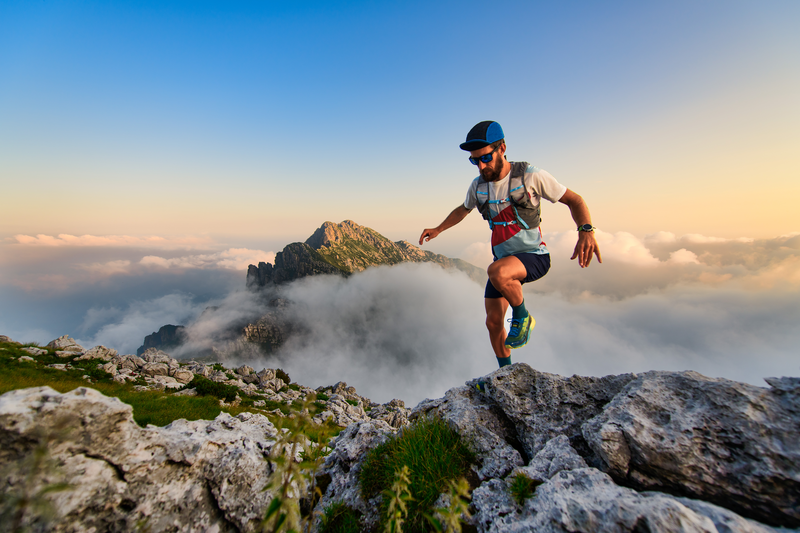
94% of researchers rate our articles as excellent or good
Learn more about the work of our research integrity team to safeguard the quality of each article we publish.
Find out more
ORIGINAL RESEARCH article
Front. Agron. , 29 April 2024
Sec. Pest Management
Volume 6 - 2024 | https://doi.org/10.3389/fagro.2024.1385740
This article is part of the Research Topic Latest Research Advances in Biology, Ecology, and Integrated Pest Management of Invasive Insects View all 4 articles
For spotted-wing drosophila, Drosophila suzukii Matsumura, the standard larval sampling protocol from the fruits involves immersing the fruits in hot water, salt, or sugar solution followed by enumerating the larvae that exit from the fruit. In this study, using the fruit samples collected from commercial blueberry farms, we validated the efficacy of a novel D. suzukii larval sampling technique, the vacuum extraction protocol. The larval extraction efficacy of the vacuum extraction method was compared with the salt extraction method using paired fruit samples collected from three different fields for four consecutive weeks. Overall, larval counts detected through the vacuum extraction were significantly higher than those detected through salt extraction in two out of three blueberry fields. Additionally, among the five blueberry fruit sample volumes tested (118, 177, 237, 355, and 473 mL), we identified 355 mL (12 fl. oz.) as the optimum fruit sample volume for vacuum extraction that maximized sampling precision for unit sampling cost. Since D. suzukii larvae exited from fruits after vacuum extraction remain alive and active, a delay in separating the larvae from the sample with coffee filter extraction may result in larval movement back into the fruit flesh. However, compared with immediate processing, delaying coffee filter extraction up to 60 minutes after vacuum incubating the fruit samples at -98 kPa for 60 minutes did not significantly reduce the larval count. Overall, the results of validation trials indicate that vacuum extraction is a promising sampling method for growers to detect D. suzukii larval infestation in blueberries.
The spotted-wing drosophila, Drosophila suzukii Matsumura (Diptera: Drosophilidae), is an invasive pest of small fruit crops in the United States. Adult females lay eggs inside the ripe and ripening fruit (Atallah et al., 2014), and developing larvae inside the fruit make it unmarketable. Repeated insecticide applications are the primary control tactic to manage D. suzukii infestation in small fruit crops. However, other non-chemical management options are being developed and integrated into the D. suzukii management operations (Tait et al., 2021). Currently, the D. suzukii pest management decisions in small fruit crops are primarily based on larval quantification in fruit using salt or sugar extraction (Shaw et al., 2019; Van Timmeren et al., 2021), followed by separating the larvae from the fruit and incubation liquid to a coffee filter (Van Timmeren et al., 2017). Adult sampling using the monitoring traps with attractants are also used to detect the D. suzukii adult population in the field (Larson et al., 2021; Panthi et al., 2022). While the adult monitoring traps are a valuable tool for early detection of D. suzukii adult activity in the field, these trap catches are generally less reliable in accurately predicting the level of D. suzukii larval infestation in the fruit across the field season (Burrack et al., 2020). Thus, assessing the D. suzukii larval count in the fruit is crucial for a grower to make timely pest management decisions, evaluate the efficacy of management tactics employed, and assess the marketability of fruits (Burrack et al., 2020).
Laboratory-based studies conducted by Babu et al. (2023) demonstrated that D. suzukii larvae from the infested blueberry fruit can be extracted by subjecting the fruit samples to vacuum pressure. The vacuum pressure and fruit incubation duration that maximize the D. suzukii larval counts from blueberries has been identified as -98 kPa for 60 minutes. Moreover, for laboratory-infested blueberry fruit samples, the larval extraction efficacy of vacuum extraction was comparable or superior to salt or sugar extraction protocol incubated for the same duration. However, the effectiveness of the vacuum sampling method in comparison with the widely implemented salt extraction method has yet to be tested in the grower’s field settings. As growers actively manage the population of D. suzukii in their field, often using frequent insecticide applications, the larval count in fruit is typically much lower in field-collected samples compared to laboratory-infested fruit samples. Validating the effectiveness of vacuum extraction in the grower’s field settings is thus important. Moreover, we observed that fruit directly collected from the production field are generally firmer than the store-purchased laboratory-infested fruit (A. Babu, pers. obser.), probably because the field-collected fruit did not experience any postharvest delay of packaging and marketing. Since the physical properties of the fruit might influence the larval extraction efficacy when subjecting the fruit sample to vacuum pressure, a field-based efficacy validation of vacuum sampling is required before recommending this method for grower adoption. Additionally, identifying a cost-effective sample unit size (sample volume) suitable for vacuum extraction is also required.
The sample unit size can significantly influence the cost and precision of a sampling program (Buntin, 1994). The optimum fruit sample volume that balances the sampling cost and precision needs to be established for the vacuum-based sampling program in blueberries. Selecting the optimum sample size for a specific sampling technique early on in its development is ideal for several reasons: 1) This provides growers with the most efficient sampling unit size associated with the sampling method for a given pest and crop, 2) this allows future researchers conducting studies on vacuum sampling of D. suzukii larvae in blueberries to run their trials following optimum sample size without wasting time and resources on an inefficient sampling procedure, and 3) following a previously optimized sample unit size will ensure comparability in study procedures and results across various related research (Krebs, 1999).
When larvae are extracted using vacuum or sugar methods, they remain alive and active, whereas they do not survive salt extraction (Babu et al., 2023). The larval movement of alive larvae in the sugar or vacuum-extracted samples facilitates easy detection of larvae from fruit fibers when extracted to a coffee filter (Shaw et al., 2019; Babu et al., 2023). The larval movement is beneficial for detecting first-instars and smaller second-instar larvae among fruit debris. In sugar extraction, larvae that exited from the fruit during incubation are suspended in a sugar solution. Thus, for sugar extraction, larval movement back to the fruit is of less concern. However, a delay in processing the fruit sample after vacuum incubation, if left in room condition, might result in some of the larvae moving back to the fruit before conducting coffee filter extraction. When processing a batch of 8-12 samples, for samples that get processed towards the end of the queue, a typical delay of up to 10-15 minutes is anticipated for performing coffee filter extraction. However, the effect of a further extended delay of 30 to 60 minutes on the larval count needs assessment.
With an overall goal of developing vacuum extraction as a practical and efficient larval sampling method in blueberries that growers can adopt to monitor the D. suzukii field infestation level, we conducted research with the following objectives: 1) to validate the efficacy of the vacuum extraction method in separating D. suzukii larvae from the blueberry fruit and compare it with the efficacy of salt extraction method, 2) identify an optimum fruit sample volume for D. suzukii larval sampling in blueberries using vacuum extraction, and 3) determine impact of delay in processing (coffee-filter extraction) the sample after vacuum incubation on the D. suzukii larval recovery rate.
The larval extraction efficacy of novel vacuum extraction is compared with the salt extraction method using paired rabbiteye blueberry (Vaccinium virgatum L.) fruit samples collected from 3 commercial fields, one in Bacon Co., (31.433, -82.388) and two in Appling Co., GA (31.718, -82.432, and 31.787, -82.255). In each field, ten sampling locations, where adjacent sampling locations separated 10 meters apart, were marked at the row edge. From each sampling location, paired fruit samples, each with 50 berries, were collected. Samples were collected in 237 mL clear polypropylene deli containers (ChoiceHD 8 oz. deli containers, www.webstaurantstore.com) weekly for four consecutive weeks and transported to the laboratory for processing. From the paired sample, one sample was subjected to a vacuum extraction (Babu et al., 2023), while the other was subjected to salt extraction (Van Timmeren et al., 2021). Before salt or vacuum incubation, fruit weights in each sample were recorded.
The vacuum extraction setup used in our experiments (Figure 1) was previously described in a related paper (Babu et al., 2023). Briefly, the vacuum incubation setup consists of an 11.35 L steel vacuum chamber with a removable tempered glass lid with a rubber gasket edge (model # P0199, BACOENG, Richfield, MN, USA). The steel chamber’s side wall has an air inlet connecting to a 3-way valve body outside. A liquid-filled gauge that measures the vacuum pressure inside the chamber is connected to one arm of the valve body. The other two arms are equipped with shut-off valves to regulate air movement. One valve, the vent valve, is connected to a particulate filter to draw air into the chamber to terminate the vacuum process when needed. The other arm with a hose barb connects the chamber to a diaphragm vacuum pump (model # FB-DVP-353, Fisher Scientific, Pittsburg, PA, USA) using a rubber hose. Before subjecting fruit samples to vacuum pressure, the deli container’s lids were replaced with a similar but modified lid with a ≈ 2 cm diameter opening for air extraction from samples during vacuum pump operation. Fruit samples were then stacked inside the vacuum chamber over a nonstick silicone mat. The vacuum pump was operated until the chamber reached the -98 kPa (~ -29 Hg) vacuum level. The samples were incubated in this vacuum level for 60 minutes.
Figure 1 Vacuum extraction setup consists of a vacuum pump and vacuum chamber. (1) an 11.35 L steel vacuum chamber, (2) tempered glass lid with a rubber gasket edge, (3) pressure gauge, (4) vent valve connected to a particulate filter, (5) valve connecting the vacuum chamber to the vacuum pump, and (6) diaphragm vacuum pump.
For salt extraction, berries from the samples were lightly squeezed to break the fruit skin. The samples were then incubated in 8.2% (wt/vol) salt solution for 60 minutes (82.5g salt per 1 L water, Free-Flowing Salt, Kroger Co. Cincinnati, OH) (Van Timmeren et al., 2021). Fruit were kept well covered with salt solution throughout the incubation.
After salt or vacuum incubation, the larvae exited the fruit were separated using the coffee-filter method described by Van Timmeren et al. (2017). Briefly, the fruit sample containing fruit, larvae, and liquid ooze from the sample was strained with a course filter glued inside a funnel placed on top of the coffee filter. The coarse filter retains berries and large fruit debris, allowing liquid and larvae to pass to a reusable coffee filter where larvae were retained for counting. Additionally, the berries retained on the coarse filter were rinsed with 2 L of tap water to dislodge any remaining larvae. The larvae retained in the coffee filter were counted using a zoom stereo microscope (Meji RZ 3010, Meiji Techno America, San Jose, CA, USA) at 7.5x magnification (10× eyepiece lens, 1× objective lens, and zoom knob set to 7.5x actual magnification). The samples were illuminated with a dual gooseneck halogen light source at maximum brightness (Schott ACE 1, Schott North America Inc. Southbridge, MA, USA). The time required for various steps in processing the sample, including to set up the vacuum chamber and vacuum pump, for vacuum chamber to attain -98 kPa vacuum level during vacuum incubation, for preparation of the salt solution, for squeezing the berries to break the fruit skin (only for salt incubated samples), for coffee filter extraction, and for counting the larvae, were recorded. Based on these data, the total time investment per sample for salt and vacuum extraction was calculated. Since the time required to collect the fruit samples from the field and the time duration of salt or vacuum incubation of fruit samples are identical for salt and vacuum extractions, these time investments were not included in the total time investment calculations.
To optimize the fruit sample volume for vacuum extraction that results in the highest precision in D. suzukii larval counts per unit time investment, we tested 5 different (118, 177, 237, 355, and 473 mL) fruit sample volumes (Table 1). These selected sample volumes correspond to fruit volumes that can fit in 4, 6, 8, 12, and 16 fl. oz. deli containers, respectively, and represent the standard disposable deli container sizes in the US that are readily available to a grower for sample collection and measurement. The total fruit sample volume collected for each sample size category is fixed to 1.42 L (48 fl. oz.) per field per sampling date. Thus, the number of sample units taken per field for each sample volume category varies (Table 1) and ranges from 12 counts of 118 mL sample to 3 counts of 473 mL sample to a total of 33 samples per field per sampling date.
Table 1 Fruit sample volumes tested to determine the optimum sample size for the vacuum-based larval sampling of D. suzukii in blueberry.
Three rabbiteye fields, two in Bacon Co. (31.433, -82.388, and 31.535, -82.510) and one in Appling Co. GA (31.787, -82.255) were selected for this study. Among the 3 fields, one field in the Bacon. Co. (31.535, -82.510) was not the part of the earlier study that compared the salt and vacuum extraction methods, and was located at the Blueberry Research and Demonstration Farm, Alma, GA. In each field, 33 adjacent rabbiteye blueberry rows that share the edge with the natural area were marked with flags. Each row is randomly assigned with one of the 33 samples without replacement. The randomization schemes generated from an online website (www.random.org) were used to assign samples to a particular row. Separate randomization schemes were used for each field on each sampling date. Sample collection was restricted to the first three blueberry plants in a row. Two sets of weekly samples were taken from 2 fields, and a single set of samples was taken from a third field for a total of 5 sample sets. The time to walk between sampling points and to collect each sample was recorded. Samples were transported to a laboratory, and fruit weights were recorded. Samples were then subjected to -98 kPa vacuum pressure for 60 minutes, and larvae were extracted without delay using the coffee filter method (Van Timmeren et al., 2017). For washing the sample during the coffee-filter extraction procedure, water volume per sample was determined based on the fruit sample volume being processed: 0.5 L increment for each 30 mL (≈ 1 fl. oz.) of sample volume. The larvae retained in the coffee filter were counted using a zoom stereo microscope at 7.5x magnification. The time required for coffee filter extraction and counting the larvae for each sample was recorded.
The D. suzukii larvae exit blueberry fruit during vacuum incubation. The larvae that exit the fruit remain alive and active after a vacuum incubation, even when the samples are subjected to -98 kPa for 60 minutes. Samples removed from the vacuum chamber after this predetermined incubation duration can be immediately processed with coffee filter extraction to separate the larvae from the fruit debris. However, a delay in the coffee filter extraction after vacuum incubation, i.e., placing the vacuum-incubated samples in room conditions for an extended time before extraction, might favor larval movement back to the fruits. While such delays are not part of the protocol or required for sample processing, they can nevertheless happen in the growers’ setting. To test how delayed processing of the sample affects the D. suzukii larval count in the coffee filter, fruit samples were collected from a grower’s rabbiteye blueberry field in Bacon Co., GA (31.433, -82.388). Samples were collected from 16 sampling locations that were separated ≈ 10 meters apart. Three identical samples (weighing ≈200 g each), randomly assigned to one for each of the three treatments (a coffee-filter processing delay of 0, 30, or 60 minutes), were collected from each sampling location. Sample collection is confined to 2-3 blueberry plants on a row edge. Samples were then vacuum incubated at -98 kPa for 60 minutes and were stacked on a laboratory bench. Depending on the treatment, the coffee filter extraction of the sample was delayed to either 0, 30, or 60 minutes. After coffee filter extraction, the larval counts were recorded with the aid of a zoom stereo microscope at 7.5x magnification. Samples were processed as a batch of 4 samples. It should be noted that when processing a batch of vacuum-incubated samples containing four samples, it takes up to 5-7 minutes to complete the coffee-filter extraction of all the samples, and thus, depending on the order of processing, samples within a treatment undergone an additional delay of 0-7 minutes. This processing delay was uniform across the treatments.
Both the larval count data and total sample processing time data collected to compare the efficacy of vacuum extraction with the salt extraction were analyzed separately for each field. Data was analyzed using GraphPad Prism Software V.10 (GraphPad Software, San Diego, CA). The normality assumption was tested with the Shapiro-Wilk test at α = 0.05. Data pass the normality assumptions were subjected to Student’s two-tailed paired t-test data, and non-normal data was subjected to a Wilcoxon matched-pairs signed rank test at α = 0.05.
The optimum sample volume with the highest sampling precision for the given time investment is identified using Wiegert’s method (Wiegert, 1962). Wiegert’s method was initially developed to optimize the quadrat area used for sampling vegetation. We adapted the method for identifying an optimum fruit sample volume for efficient D. suzukii larval detection from blueberry fruit using the vacuum extraction method. The optimum sample volume is the sample size with the minimal product of relative variance x relative cost (Krebs, 1999). The relative variance and relative cost estimate required for identifying the optimum sample volume were calculated as follows:
Where minimum standard deviation is the least standard deviation of larval count among the sample volumes tested. Before calculating the relative variance, the standard deviation of larval count from all the sample volume categories was standardized to larval count per 100 mL fruit sample volume (Krebs, 1999). The optimum sample volume identified using the above method varied across the sampling dates and fields. Thus, the average product of relative variance x relative cost across 5 sample dates for each sample volume category was calculated (Schoenly et al., 2003). The sample volume with the least mean product of relative variance x relative cost across 5 sample dates was identified as the optimum for D. suzukii larval population estimation in blueberries using vacuum extraction. Additionally, time investment per larvae detected by vacuum extraction of each sample volume category was calculated. Since the number of sample units taken per field for each sample volume category varies (Table 1), but the total sample volume taken for a sample volume category per field per sampling week is fixed at 1.42 L, the time investment per larvae was calculated per field per sampling date basis. For this, the total time investment to sample all the individual samples of that sample volume category per field per sampling week was estimated and divided by the total larval count of each sample volume category per field per sampling week. Data on time investment per larvae was then analyzed using a generalized linear mixed model (PROC GLIMMIX). Data was fitted with a Poisson distribution model. The fixed effect in the model was time investment per larvae in minutes. Sampling week nested within the field was considered a random factor. Means were separated post-analysis of variance (ANOVA) using the Tukey-Kramer test (α = 0.05).
Larval counts from the sample processed after a 0, 30, or 60-minute delay were analyzed using a generalized linear mixed model (PROC GLIMMIX). Data was fitted with a Poisson distribution model. The fixed effect in the model was sample processing delay. Replication was considered a random factor. Means were separated post-analysis of variance (ANOVA) using the Tukey-Kramer test (α = 0.05).
Compared with salt extraction, the vacuum extraction of blueberry samples detected significantly more larvae in two out of three fields (Field 1, W = -183; n = 40; P = 0.0182; Field 3, W = -176; n = 40; P = 0.0030; Figure 2). No significant difference in larval counts between salt and vacuum extraction was observed at the remaining field (Field 2, W = -60; n = 40; P = 0.3083; Figure 2), probably due to the low natural infestation level in this field and the sample variability.
Figure 2 Mean (± SEM) number of D. suzukii larvae recovered per 50 blueberries collected from 3 different fields in Georgia when following the salt and vacuum extraction protocols. Fruits were incubated in a -98 kPa vacuum or 8.2% (wt/vol) salt solution for 60 minutes. 'ns' no statistical difference (P>0.05); ** significant statistical difference (P<0.01).
Additionally, across the three fields, compared with samples processed with salt extraction, the vacuum extraction resulted in a higher proportion of samples with at least one D. suzukii larva. The vacuum extraction detected at least one larva in 56.67 ± 0.05 (mean ± SEM) percentage of the sample tested, while salt extraction detected larvae only in 46.67 ± 0.05 percent sample. Overall, for D. suzukii larval detection in blueberry, 21.4% more samples detected larva when processed using vacuum extraction method as compared to the salt extraction method.
A comparison of the mean total time investment per sample required to process the blueberry fruit samples using salt and vacuum extraction protocols indicates that salt extraction, in general, requires a significantly longer sample processing time than vacuum extraction in all three growers’ fields tested (Field 1, W = 820; n = 40; P<0.0001; Field 2, t = 18.80; df = 39; P<0.0001; Field 3, W = 820; n = 40; P<0.0001; Figure 3).
Figure 3 Mean (± SEM) processing time per sample of 50 blueberries collected from 3 different fields in Georgia when following the salt and vacuum extraction protocols. **** significant statistical difference (P<0.0001).
Across the five sampling trials, no single blueberry sample volume emerged as an optimum blueberry fruit volume that maximizes the sample count precision for the unit cost, suitable for D. suzukii larval extraction using the vacuum extraction procedure (Table 2). A 473, 355, and 177 mL fruit volume was identified as the optimum sampling volume in 2, 2, and 1 sampling trials, respectively. However, averaging the product of relative variance and relative cost over the five sampling trials suggested that, on average, 355 mL (12 fl. oz.) fruit sample is the optimum sampling size for vacuum extraction for detecting D. suzukii larvae from blueberries (Figure 4).
Table 2 Mean larval count, mean sample processing time, and optimum sample volume based on lowest relative variance x relative cost (indicated by an asterisk) for D. suzukii larval sampling in blueberry when using vacuum extraction protocol.
Figure 4 Mean (± SEM) of the product of relative variance and relative cost, averaged across 3 fields. The optimum fruit volume, which has the highest sampling precision for the unit sampling cost for sampling D. suzukii larvae from blueberries when using the vacuum extraction method, is identified with a square.
The above calculation of optimum sample volume is based on the relative variance calculation as a measure of sampling precision and time investment as a measurement of cost. However, 473 mL samples had the least time requirement for detecting a D. suzukii larva using vacuum extraction protocol, and no significant difference is observed between 177 mL, 237 mL, and 473 mL sample volumes (F = 10.70; df = 4, 16; P = 0.0002; Figure 5).
Figure 5 Mean (± SEM) processing time in minutes spent per successful detection of a larva when samples were processed using vacuum extraction protocol. Bars marked with different letters indicate significant differences between treatments (Tukey–Kramer).
A sample processing delay of 30 or 60 minutes after vacuum incubation did not significantly reduce the larval recovery success (F = 4.08; df = 2, 1; P = 0.3303; Figure 6). However, it should be noted that compared with the sample that processed immediately after vacuum incubation, i.e., 0-minute delay, overall, there was a 31.7 and 25.0% numerical reduction in the mean larval counts when sample processing is delayed for 30 and 60 minutes after a 60-minute vacuum incubation.
Figure 6 Mean (± SEM) number of D. suzukii larvae recovered per 200 grams of blueberries collected from a commercial field in Georgia using vacuum extraction at -98 kPa incubated for 60 minutes and then placed in laboratory bench for 0, 30, or 60 minutes before coffee-filter extraction. Bars marked with the same letter are not significantly different (Tukey–Kramer).
The results from the validation trials of vacuum extraction in blueberry demonstrated that: 1) compared with the current salt extraction protocol, the vacuum extraction is a quicker and equally or more efficient D. suzukii larval sampling method in blueberry; 2) A 355 mL blueberry fruit sample volume minimizes the sample variance per unit cost, and thus identified as the optimum sample volume for D. suzukii population estimation; 3) a 473 mL sample size minimizes the time investment per successful detection of larvae, and the growers can use this sample volume if their objective is to maximize the larval detection efficacy in terms of sampling cost, and finally; 4) a delay in coffee-filter extraction for 30 minutes or even up to 60 minutes after vacuum incubation did not significantly reduced larval recovery count from the samples. Together, results from our vacuum sampling protocol validation and sample size optimization trials suggest that vacuum extraction is a practical and efficient sampling method for growers to detect the D. suzukii larval infestation in blueberry fruit. Moreover, identifying the optimum sample volume for both population estimation and pest detection ensures the field usability of this novel sampling technique for D. suzukii pest management decision-making. Since a delay of up to 60 minutes for coffee filter extraction after vacuum incubation does not significantly influence the larval recovery success, the vacuum extraction protocol provides some flexibility for the growers when processing multiple batches of samples without negatively affecting the larval assessment outcome.
For making D. suzukii management decisions in blueberries, both adult trapping (Cha et al., 2018; Larson et al., 2020; Panthi et al., 2022) as well as larval sampling from the infested fruits (Shaw et al., 2019; Van Timmeren et al., 2021; Babu et al., 2023) are available as pest monitoring methods. In regions where D. suzukii adults are not detected in late winter and spring, adult monitoring is helpful for growers to detect adult activity in the field earlier in the season during the onset of the fruit-susceptible window (Cha et al., 2018). Thus, adult detection can serve as an early warning system. However, a reliable relationship between the adult trap captures from the field and fruit infestation has not been established. Additionally, in southern locations, including Georgia, where D. suzukii adults are active most of the year, adult detection in the field to signal growers to initiate insecticide application is of less relevance. Moreover, pest management by calendar-based insecticide applications after the initial detection of adult insects in the field, without quantifying the larval infestation that results in actual damage, does not agree with the IPM principles. Therefore, a larval sample-based method, which directly measures damage potential, is more appropriate for D. suzukii management in small fruit crops. Accordingly, a recent study on the economic impact of larval monitoring-based D. suzukii management in organic blueberries in Oregon suggested that management strategies incorporating larval sampling from fruits resulted in higher average profit than the calendar-based insecticide spray strategy (Yeh et al., 2023). For developing larval monitoring-based D. suzukii management, a recent research introduced vacuum extraction protocol as an efficient larval sampling technique (Babu et al., 2023). Along with the results from this previous related study, this study demonstrated that compared with other D. suzukii larval extraction protocols, vacuum extraction is less laborious, quicker, and equally or more efficient in extracting D. suzukii larvae from the infested blueberries. Therefore, among the various sampling methods available, vacuum extraction can be considered as one of the most promising method for sampling D. suzukii in blueberries.
Irrespective of the larval sampling technique, the treatment decision-making for D. suzukii management in blueberries is complicated by the absence of an economic threshold. The lack of a research-based economic threshold coupled with a low tolerance for larval infestation diminishes the perceived value of an accurate larval population estimation in the field. Therefore, presently, the fruit sampling for D. suzukii monitoring has primarily been aimed at detecting D. suzukii larval infestation rather than accurately quantifying the larval population mean. We identified 473 mL as the optimum blueberry fruit sample volume that minimizes the sampling cost per larval detection. Blueberry growers can use this sample volume for vacuum extraction to detect D. suzukii larvae for pest management decision-making until an economic threshold is established. Additionally, to maximize the D. suzukii larval detection probability, growers can restrict the fruit sampling from the field edges where the infestation is usually the highest (Rice et al., 2017; Rodriguez-Saona et al., 2020).
The incubation of fruit samples infested with D. suzukii at -98 kPa vacuum pressure triggers the larval exit from the fruit. Most of these larvae remain alive and active, even after 60 minutes of incubation at -98 kPa. Since the larvae are alive, we were concerned that keeping the vacuum-incubated sample outside the vacuum for a longer duration before proceeding to the coffee filter extraction would result in a portion of the larval population moving back to the fruit flesh, affecting the overall efficacy of the vacuum protocol. Such a delay can be expected when growers are processing multiple batches of fruits or when busy with other farm operations. However, we didn’t observe any substantial reduction in larval counts when the coffee filter extraction of vacuum-incubated samples was delayed up to 60 minutes. This will provide growers with some flexibility during the sample processing. For example, after removing a batch of fruit samples from the vacuum chamber, growers can take time to initiate the vacuum incubation of the next batch before proceeding to the coffee filter extraction of the recently incubated samples. This flexibility will allow growers to minimize the waiting period between processing the successive samples after the typical 30 or 60 minutes of vacuum incubation.
In conclusion, vacuum extraction is a promising larval extraction method for growers, and crop consultants to detect and quantify the D. suzukii infestation in the blueberries in real-time and make informed pest management decisions. This research confirmed the usefulness of vacuum extraction in the blueberry field setting and defined the optimum sample size that maximizes larval detection efficacy. The results from this study will help growers to fully adopt vacuum extraction as an efficient larval sampling protocol. Future research should explore the usefulness of vacuum extraction for D. suzukii larval monitoring in other small fruit crops, including raspberry, strawberry, blackberry, and cherries, where D. suzukii is a major pest. Additionally, research should also be conducted to develop an economic threshold for this pest in these crops to encourage the implementation of more sustainable IPM programs to control this pest.
The original contributions presented in the study are included in the article/Supplementary Material. Further inquiries can be directed to the corresponding author.
The manuscript presents research on animals that do not require ethical approval for their study.
AB: Conceptualization, Data curation, Formal analysis, Investigation, Methodology, Project administration, Validation, Visualization, Writing – original draft, Writing – review & editing. AS: Data curation, Formal analysis, Funding acquisition, Methodology, Project administration, Resources, Software, Supervision, Validation, Writing – review & editing.
The author(s) declare financial support was received for the research, authorship, and/or publication of this article. This research was supported by funding from the USDA Specialty Crops Research Initiative (Award # 2015-51181-24252 and 2020-51181-32140), the USDA Organic Agriculture Research and Extension Initiative (Award # 2018-51300-28434), Extension Implementation Program (Award # 2017-70006-27202), Georgia Berry Exchange, and Georgia Agricultural Commodity Commission for Blueberries.
We thank Cera Jones, Jacob Athon, Paige Hackleman, and Tyler Washburn for their technical assistance.
The authors declare that the research was conducted in the absence of any commercial or financial relationships that could be construed as a potential conflict of interest.
All claims expressed in this article are solely those of the authors and do not necessarily represent those of their affiliated organizations, or those of the publisher, the editors and the reviewers. Any product that may be evaluated in this article, or claim that may be made by its manufacturer, is not guaranteed or endorsed by the publisher.
The Supplementary Material for this article can be found online at: https://www.frontiersin.org/articles/10.3389/fagro.2024.1385740/full#supplementary-material
Supplementary Data Sheet 1 | Datasets analysed for this study.
Atallah J., Teixeira L., Salazar R., Zaragoza G., Kopp A. (2014). The making of a pest: the evolution of a fruit-penetrating ovipositor in Drosophila suzukii and related species. Proc. R. Soc. B: Biol. Sci. 281, 20132840. doi: 10.1098/rspb.2013.2840
Babu A., Adhikari R., Sial A. A. (2023). Vacuum extraction: an effective larval sampling method for spotted-wing drosophila in small fruit crops. J. Econ. Entomol. 116, 1750–1759. doi: 10.1093/jee/toad160
Buntin G. D. (1994). “Developing a primary sampling program,” in Handbook of sampling methods for arthropods in agriculture. Eds. Pedigo L. P., Buntin G. D. (CRC, Boca Raton, FL), 99–115.
Burrack H., Lee J. C., Rodriguez-Saona C., Loeb G. (2020). “Progress and challenges in building monitoring systems for Drosophila suzukii,” in Drosophila suzukii management. Ed. Garcia F. R. M. (Springer Nature Switzerland AG, Cham, Switzerland), 111–132.
Cha D. H., Hesler S. P., Wallingford A. K., Zaman F., Jentsch P., Nyrop J., et al. (2018). Comparison of commercial lures and food baits for early detection of fruit infestation risk by Drosophila suzukii (Diptera: Drosophilidae). J. Econ. Entomol. 111, 645–652. doi: 10.1093/jee/tox369
Larson N. R., Strickland J., Shields V. D., Rodriguez-Saona C., Cloonan K., Short B. D., et al. (2021). Field evaluation of different attractants for detecting and monitoring Drosophila suzukii. Front. Ecol. Evol. 9, 175. doi: 10.3389/fevo.2021.620445
Larson N. R., Strickland J., Shields V. D., Zhang A. (2020). Controlled-release dispenser and dry trap developments for Drosophila suzukii detection. Front. Ecol. Evol. 8, 45. doi: 10.3389/fevo.2020.00045
Panthi B., Cloonan K. R., Rodriguez-Saona C., Short B. D., Kirkpatrick D. M., Loeb G. M., et al. (2022). Using red panel traps to detect spotted-wing drosophila and its infestation in US berry and cherry crops. J. Econ. Entomol. 115, 1995–2003. doi: 10.1093/jee/toac134
Rice K. B., Jones S. K., Morrison W., Leskey T. C. (2017). Spotted wing drosophila prefer low hanging fruit: insights into foraging behavior and management strategies. J. Insect Behav. 30, 645–661. doi: 10.1007/s10905-017-9646-9
Rodriguez-Saona C., Firbas N., Hernández-Cumplido J., Holdcraft R., Michel C., Palacios-Castro S., et al. (2020). Interpreting temporal and spatial variation in spotted-wing drosophila (Diptera: Drosophilidae) trap captures in highbush blueberries. J. Econ. Entomol. 113, 2362–2371. doi: 10.1093/jee/toaa153
Schoenly K. G., Domingo I., Barrion A. T. (2003). Determining optimal quadrat sizes for invertebrate communities in agrobiodiversity studies: a case study from tropical irrigated rice. Environ. Entomol. 32, 929–938. doi: 10.1603/0046-225X-32.5.929
Shaw B., Cannon M. F. L., Buss D. S., Cross J. V., Brain P., Fountain M. T. (2019). Comparison of extraction methods for quantifying Drosophila suzukii (Diptera: Drosophilidae) larvae in soft- and stone-fruits. Crop Prot. 124, 104868. doi: 10.1016/j.cropro.2019.104868
Tait G., Mermer S., Stockton D., Lee J., Avosani S., Abrieux A., et al. (2021). Drosophila suzukii (Diptera: Drosophilidae): a decade of research towards a sustainable integrated pest management program. J. Econ. Entomol. 114, 1950–1974. doi: 10.1093/jee/toab158
Van Timmeren S., Davis A. R., Isaacs R. (2021). Optimization of a larval sampling method for monitoring Drosophila suzukii (Diptera: Drosophilidae) in blueberries. J. Econ. Entomol. 114, 1690–1700. doi: 10.1093/jee/toab096
Van Timmeren S., Diepenbrock L. M., Bertone M. A., Burrack H. J., Isaacs R. (2017). A filter method for improved monitoring of Drosophila suzukii (Diptera: Drosophilidae) larvae in fruit. J. Integrated Pest Manage. 8, 1–7. doi: 10.1093/jipm/pmx019
Wiegert R. G. (1962). The selection of an optimum quadrat size for sampling the standing crop of grasses and forbs. Ecology 43, 125–129. doi: 10.2307/1932047
Keywords: Drosophila suzukii, spotted-wing drosophila, blueberry, sample volume, vacuum extraction, salt extraction
Citation: Babu A and Sial AA (2024) Validation of vacuum extraction protocol for larval sampling of spotted-wing drosophila in blueberries. Front. Agron. 6:1385740. doi: 10.3389/fagro.2024.1385740
Received: 13 February 2024; Accepted: 17 April 2024;
Published: 29 April 2024.
Edited by:
Mohannad Ismail, Université Catholique de Louvain, BelgiumReviewed by:
Alberto Pozzebon, University of Padua, ItalyCopyright © 2024 Babu and Sial. This is an open-access article distributed under the terms of the Creative Commons Attribution License (CC BY). The use, distribution or reproduction in other forums is permitted, provided the original author(s) and the copyright owner(s) are credited and that the original publication in this journal is cited, in accordance with accepted academic practice. No use, distribution or reproduction is permitted which does not comply with these terms.
*Correspondence: Arun Babu, QXJ1bkJhYnVAdWdhLmVkdQ==
Disclaimer: All claims expressed in this article are solely those of the authors and do not necessarily represent those of their affiliated organizations, or those of the publisher, the editors and the reviewers. Any product that may be evaluated in this article or claim that may be made by its manufacturer is not guaranteed or endorsed by the publisher.
Research integrity at Frontiers
Learn more about the work of our research integrity team to safeguard the quality of each article we publish.