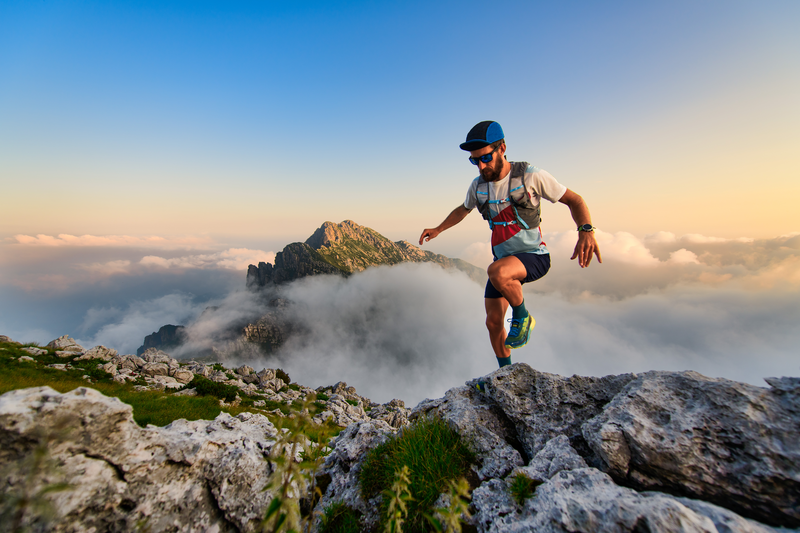
94% of researchers rate our articles as excellent or good
Learn more about the work of our research integrity team to safeguard the quality of each article we publish.
Find out more
ORIGINAL RESEARCH article
Front. Agron. , 15 May 2024
Sec. Climate-Smart Agronomy
Volume 6 - 2024 | https://doi.org/10.3389/fagro.2024.1383613
This article is part of the Research Topic Innovative Approaches in Nutrient Management for Sustainable Cropping Systems View all 5 articles
Graphene has unique properties for improving soil health properties such as nutrient availability, soil physical and chemical properties, and controlled release of essential elements. This research aimed at determining the impact of graphene amendment on the decomposition of oil palm frond mulching and on soil health status. The study was conducted using a factorial experiment in completely randomized design with two main factors: (i) covering conditions: cover with plastic sheet and no cover, and (ii) graphene application that included T1 (control): oil palm frond mulching (OFM), T2: OFM + graphene (G), T3: OFM + G + chemical fertilizer, and T4: OFM + G + goat manure. The results indicated that there were significant differences among graphene applications, between cover conditions, and in interactions between graphene applications and cover conditions for all soil characteristics in the most observed month. In the third month of soil analysis, the treatment of graphene applications showed higher electrical conductivity (T2: 151.7 ± 6.8 µS cm−1), available phosphorus (T3: 9.0 ± 6.7 mg kg−1), exchangeable potassium (T2: 67.1 ± 24.9 mg kg−1), and exchangeable calcium (T3: 95.4 ± 5.1 mg kg−1), compared to control. The cover condition showed suitable soil pH (5.0 ± 0.2), higher soil available phosphorus (7.1 ± 5.0 mg kg−1), and exchangeable calcium (599.1 ± 235.2 mg kg−1), but the no-cover condition presented higher soil organic matter (0.7% ± 0.2%), exchangeable potassium (60.3 ± 19.1 mg kg−1), and exchangeable magnesium (96.7 ± 11.4 mg kg−1). Correlation results indicated that most soil characteristics were correlated under graphene applications. Principal component analysis showed that the treatments of graphene application dominated most soil characteristics. The results suggest that graphene has potential for improving soil health properties and can be applied as an alternative sustainable amendment to accelerate the decomposition of oil palm frond mulch and enhance nutrient availability for oil palm. In addition, the authors suggest that further investigations should consider more soil health parameters in long-term field studies for a better understanding and to provide recommendations to farmers.
Oil palm (Elaeis guineensis Jacq.) as a key economic oil crop in Thailand and other tropical regions is famous for its high yield potential, averaging 3.3 tons per hectare (Woittiez et al., 2017). Initially, oil palm plantations were confined to tropical regions due to their optimal growing conditions (Corley and Tinker, 2003). However, driven by the increased demand for palm oil in both food and non-food industrial sectors, its cultivation has expanded to include marginal lands with poor soil fertility. As of 2023, Thailand had approximately 1.03 million hectares of oil palm cultivation, with 86% located in the southern region (Office of Agricultural Economics, 2023). The soils in southern Thailand are generally less fertile, compelling efficient nutrient management for successful crop cultivation (Hussain et al., 2022). The perennial nature of oil palm crops, with fruiting cycles extending up to 30 years, coupled with improper fertilizer management, can adversely affect soil health (Duangpan et al., 2022). Despite the desire for high yields, associated management costs pose challenges. Soil health and nutrient availability are crucial considerations, as they directly influence yield potential. Although farmers apply fertilizers, seasonal applications often lead to significant nutrient losses, particularly during heavy rainfall intervals in the rainy season, impacting fertilizer efficiency. Given these challenges, it is imperative to explore alternative approaches to improve soil health and nutrient status. Innovative methods such as an efficient utilization of oil palm frond mulch and organic amendments may offer promising solutions.
Oil palm fronds are waste products obtained during pruning and oil palm bunch harvesting (Ajayi et al., 2023). Typically, farmers utilize these fronds as mulch in oil palm plantations, where these fronds are decomposed to provide organic matter (OM) and essential nutrients for plants (Pulunggono et al., 2019). Oil palm fronds contain various substances that decompose into plant-available nutrients, including cellulose (31.5% ± 0.3%), lignin (14.0% ± 0.5%), hemicellulose (19.2% ± 0.1%), protein (9.4% ± 0.1%), and ash (12.3% ± 0.2%) (Hong et al., 2012). In current management practices of oil palm plantations in Southern Thailand, farmers often apply a bulk of fertilizers, including urea, muriate of potash, and manure. This method requires less labor and time compared to conventional broadcasting methods. Oil palm trees receive nutrients and moisture through the dissolution of fertilizers and decomposition of oil palm fronds. However, this fertilization method leads to nutrient losses through leaching, particularly during heavy rainfall in the region’s rainy season (Hussain et al., 2021), with annual rainfall averaging 2,521 mm. Nutrient losses, especially nitrate leaching, significantly impact the sustainability of cropping systems, resulting in substantial losses in southern Thailand (Hussain et al., 2023). Therefore, it is essential to control these losses and improve nutrient use efficiency. Alternative approaches, such as adding substances and amendments to fertilizers, can enhance fertilizer absorption and maintain nutrient levels in the soil for longer periods. According to Hossain et al. (2020), substances with properties such as porosity, electrical charge, high surface area, and controlled release of nutrients are crucial for prolonging nutrient availability in the soil.
Graphene is considered a suitable material for maintaining and enhancing nutrient levels in oil palm frond mulch. Some farmers have begun to incorporate graphene into oil palm mulch for prolonged nutrient availability and reduced fertilizer usage; however, there is currently no specific research evidence available on this matter which is the basis of this study. Graphene is a single sheet of carbon atoms arranged in a honeycomb lattice and joined by sp2 hybridization (Asif and Saha, 2023). Because of its outstanding 2-D structure, large surface area, and exceptional mechanical, thermal, optical, and electrical properties that make it useful for a variety of applications, graphene has garnered a great deal of research interest (Kabiri et al., 2017). Graphene can be utilized in agriculture to increase soil clay content and improve soil texture, thereby enhancing nutrient absorption capacity and nutrient storage (Wang et al., 2023). This leads to reduced nutrient losses caused by volatilization, surface runoff, and deep seepage (Andelkovic et al., 2018; He et al., 2018), which are critical components of soil water and nitrogen balance (Hussain et al., 2023). Additionally, graphene can enhance soil physicochemical properties by increasing the concentration of nutrient ions in soil colloids, reducing nutrient loss, boosting the levels of ammonium nitrogen, effective phosphorus, and fast-acting potassium in the soil after leaching, and improving the stability of soil aggregates following leaching (Liu et al., 2023). It can also enhance the soil’s electrochemical properties, promote the absorption of nutrients by root systems, increase fertilizer application rates, reduce agricultural surface pollution, and enhance fertilizer efficiency and conservation (Wang et al., 2023), ultimately leading to improved productivity and sustainability of agricultural soils.
Keeping in view the importance of the above-mentioned findings and the lack of scientific evidence on the impacts of graphene application in oil palm mulch for prolonged nutrient availability, the objective of this study was to evaluate the impact of graphene amendment on the decomposition of oil palm frond mulch and soil health status through enhanced nutrient availability. The results of this study will provide scientific evidence for long-term research studies and will help farmers to revise the fertilizer management strategies for improved soil health by optimizing fertilizer use and associated costs.
The research experiment was conducted between March and September 2023 at the School of Agricultural Technology and Food Industry’s oil palm experimental field (8°38′43.9" N, 99°54′03.2" E), at Walailak University, Thailand. The soil at the experimental field is classified as Gleysols (IUSS Working Group WRB, 2022). Clay, silt, and sand components of clayey soil were 62%, 28%, and 10%, respectively, whereas soil pH was 4.7. The weather data were recorded using the weather station installed at the experimental site. During the experimental period, the average monthly rainfall was 60 mm, and the highest and lowest rainfall were observed in May and March, respectively. The relative humidity ranged between 83% and 91% with the highest and lowest humidity observed in May and June. The temperature ranged between 27.9°C and 28.9°C, and the highest and lowest temperatures were observed in August and April, respectively. The experiment was organized using a completely randomized design with a factorial arrangement having two factors. The first factor was a covering condition including covering with black plastic sheet and no covering. The second factor included the treatments of graphene application including T1: oil palm frond mulching, T2: oil palm frond mulching amended with graphene, T3: oil palm frond mulching amended with graphene + 15-15-15 chemical fertilizer, and T4: oil palm frond mulching amended with graphene + goat manure. Each experimental unit consisted of three replications (oil palm frond mulching). The mulching was prepared by approximately 10–12 oil palm fronds. The ground of the mulching was prepared by fine river sand. The experimental arrangement is illustrated in Figure 1.
Figure 1 Experimental arrangement having experimental treatments including (i) graphene applications and (ii) covering conditions. T1: oil palm frond mulching, T2: oil palm frond mulching amended with graphene, T3: oil palm frond mulching amended with graphene + 15-15-15 chemical fertilizer, and T4: oil palm frond mulching amended with graphene + goat manure.
The soil samples were collected at 0–30 cm under the oil palm frond mulching every 2 months (M1: May, M2: July, and M3: September). The samples were then analyzed for the soil properties including pH, electrical conductivity, OM, available phosphorus, exchangeable potassium, exchangeable calcium, and exchangeable magnesium. The OM was analyzed according to the in-house method (He et al., 2020). The available phosphorus was extracted using Bray No II (Wuenscher et al., 2015) and analyzed by spectrophotometer (Jasco V-630). The exchangeable potassium, calcium, and magnesium were extracted with 1.0 M NH4OAc pH 7.0 and analyzed with ICP-OES (AVIO 200) (Food and Agriculture Organization, 2020). All soil analyses were performed at the Center for Scientific and Technological Equipment of Walailak University, Thailand.
Data were analyzed and analysis of variance (ANOVA) was performed. The assumption of normality was tested according to Shapiro–Wilk test using the dplyr package (Wickham et al., 2023). Means of the data (± standard derivations) were compared according to Duncan’s multiple range test and reported at p < 0.05. Statistical analysis and data visualization were carried out using the R program (Version R 4.3.2) with the Agricolae package (De Mendiburu, 2023). Correlation analysis and principal component analysis (PCA) were performed to further evaluate the association and their significance among studied attributes using the ggbiplot package (Vu et al., 2024).
Weight loss percentage of oil palm frond under different graphene applications is presented in Table 1. The highest weight loss percentage was observed at T1 (32.1%) and T3 (32.1%) under the cover condition, and the lowest percentage was obtained at T3 under the no-cover condition. As compared between both conditions, the cover condition (31.2%) showed higher weight loss percentage than the no-cover condition (27.6%). Statistical results for soil characteristics are presented in Table 2. The ANOVA revealed that there were significant differences (p < 0.05) among the treatments of graphene application and cover conditions for all soil characteristics in the most observed months, except for the soil pH in May. In addition, there were statistical interactions between graphene applications and cover conditions for all soil characteristics in the most observed times, except for soil pH in May and September. The coefficient of variation (CV) ranged between 1.7% and 9.6%, with the highest and lowest CV observed from soil pH in May and soil electrical conductivity in September.
Table 2 Mean squares of analysis of variance and significance for soil characteristics observed under various applied treatments.
Between the covering conditions and the four treatments of graphene application, there were no significant differences in the soil pH in May. T4 provided the highest pH of any treatment in July (5.89 ± 1.1) and the appropriate pH level for plants (5.5–6.5). The soil pH from the cover group (6.0 ± 0.6) was higher than that of the no-cover group (4.5 ± 0.3). While all pH values in September were below 5.5, T4 yielded the plant-suitable levels closest to 5.1 ± 0.2, which was also higher than other treatments. The cover group (5.0 ± 0.2) had a higher value than the no-cover group (4.9 ± 0.2), like July (Table 3, Figure 2).
Figure 2 Variation in soil pH under different graphene applications (T1, T2, T3, and T4) observed at different months. M1, M2, and M3 refer to May, July, and September, respectively.
The soil from the no-cover group (155.6 ± 11.0 µS cm−1) had a greater electrical conductivity (EC) in May than the cover group (147.7 ± 8.4 µS cm−1) and T3 produced the highest EC at 163.4 ± 7.67 µS cm−1. T4 produced the highest EC in July, measuring 171.0 ± 20.7 µS cm−1. Moreover, soil from the cover group (161.5 ± 17.7 µS cm−1) produced EC that was higher than that of the no-cover group (158.0 ± 13.9 µS cm−1). T2 yielded the highest EC in September (151.7 ± 6.8 µS cm−1) but there were no significant differences between EC from covering conditions (Table 3, Figure 3).
Figure 3 Variation in soil electrical conductivity under different graphene applications (T1, T2, T3, and T4) observed at different months. M1, M2, and M3 refer to May, July, and September, respectively.
Treatment of oil palm frond mulching (T1) provided the most OM in May, resulting in 0.6% ± 0.2%, while the soil from the cover group (0.6% ± 0.1%) had more OM than the soil from the no-cover group (0.5% ± 0.1%). T4 yielded the greatest OM in July, at 0.8% ± 0.2%, while the soil from the no-cover group (0.7% ± 0.2%) produced more OM than the cover group (0.7% ± 0.2%). The soil from the no-cover group (0.7% ± 0.2%) had more OM than the cover group (0.7% ± 0.1%) in September, with T1 producing the highest OM at 0.9% ± 0.2% (Table 3, Figure 4).
Figure 4 Variation in soil organic matter under different graphene applications (T1, T2, T3, and T4) observed at different months. M1, M2, and M3 refer to May, July, and September, respectively.
The soil from the cover group (3.4 ± 3.2 mg kg−1) produced more available phosphorus (P) than the soil from the no-cover group (2.4 ± 0.6 mg kg−1) in May, with T3 producing the maximum amount of P at 6.0 ± 3.0 mg kg−1. T4 produced the most P in July (15.7.0 ± 5.3 mg kg−1), while the cover group (6.2 ± 5.8 mg kg−1) produced P that was higher than that of the no-cover group (4.2 ± 2.4 mg kg−1). T3 yielded the maximum P in September, measuring 9.0 ± 6.7 mg kg−1, while the soil from the cover group (7.1 ± 5.0 mg kg−1) produced more P than the soil from the no-cover group (4.2 ± 4.0 mg kg−1) (Table 3, Figure 5).
Figure 5 Variation in soil available phosphorus under different graphene applications (T1, T2, T3, and T4) observed at different months. M1, M2, and M3 refer to May, July, and September, respectively.
Oil palm frond mulching amended with graphene (T2) yielded the maximum exchangeable potassium (K) in May, July, and September, at 66.6 ± 20.2, 60.6 ± 8.3, and 67.1 ± 24.9 mg kg−1, respectively. The soil from the no-cover group (71.8 ± 14.9, 54.2 ± 11.5, and 60.3 ± 19.1 mg kg−1) had higher K levels than the cover group (47.1 ± 4.5, 49.5 ± 6.0, and 52.6 ± 8.8 mg kg−1) in all the observed months (Table 3, Figure 6).
Figure 6 Variation in soil exchangeable potassium under different graphene applications (T1, T2, T3, and T4) observed at different months. M1, M2, and M3 refer to May, July, and September, respectively.
Oil palm frond mulching amended with graphene + 15-15-15 chemical fertilizer (T3) provided the highest exchangeable calcium (Ca) in May (528.1 ± 115.7 mg kg−1). T4 produced the most Ca in July (635.4 ± 362.1 mg kg−1). T1 produced the most Ca in September (607.1 ± 287.7 mg kg−1). Compared to the no-cover group (310.2 ± 77.2, 295.6 ± 35.7, and 309.2 ± 26.7 mg kg−1), the soil from the cover group (536.0 ± 93.8, 594.2 ± 284.8, and 599.1 ± 235.2 mg kg−1) had greater Ca levels in all observed months (Table 3, Figure 7).
Figure 7 Variation in soil exchangeable calcium under different graphene applications (T1, T2, T3, and T4) observed at different months. M1, M2, and M3 refer to May, July, and September, respectively.
Oil palm frond mulching (T1) and oil palm frond mulching amended with graphene + 15-15-15 chemical fertilizer (T3) provided the most exchangeable magnesium (Mg) in September and May, at 95.4 ± 5.1 mg kg−1 and 93.7 ± 14.5 mg kg−1, respectively. T2 produced the most Mg in July, 91.8 ± 14.3 mg kg−1. Values of Mg in the soil from the no-cover group was greater (92.7 ± 14.7, 92.1 ± 10.3, and 96.7 ± 11.4 mg kg−1) than that of the cover group (78.4 ± 7.1, 78.1 ± 4.4, and 75.8 ± 12.1 mg kg−1) in all observed months (Table 3, Figure 8).
Figure 8 Variation in soil exchangeable magnesium under different graphene applications (T1, T2, T3, and T4) observed at different months. M1, M2, and M3 refer to May, July, and September, respectively.
The scatter plot with Pearson correlation coefficient showed that the highly significant positive correlation coefficients were obtained between soil P and EC (0.56), soil P and Ca (0.51), and soil pH and Ca (0.47). Highly significant negative correlation coefficients were obtained between soil pH and Mg (−0.31), soil OM and K (−0.24), and soil Ca and Mg (−0.23) (Figure 9). In the principal component (PC) analyses, the first three components were described by 29.5%, 23.0%, and 18.6% variation. The biplot of PC1 and PC2 revealed that the largest group was obtained from T4 under the cover condition and the smallest group was obtained from T1 under the no-cover condition. T3 under the cover condition dominated in soil OM, soil pH, Ca, P, and EC. T4 under the cover condition dominated in soil pH and Ca. T2, T3, and T4 under the no-cover condition dominated in soil Mg and K (Figure 10A). From the biplot of PC1 and PC3, T4 under the cover condition was also the biggest group and T1 under the no-cover condition was the smallest group. T2, T3, and T4 under the cover condition dominated in soil EC, pH, P, and Ca. T4 under the no-cover condition dominated in soil K (Figure 10B).
Figure 9 Scatter plot with Pearson correlation coefficients among soil characteristics. The distribution of each variable is presented diagonally. The upper triangular matrix shows the Pearson correlation coefficient with significance. 0.05 (*), 0.01 (**), and 0.001 (***). The lower triangular matrix shows the bivariate scatter plots with a fitted smooth line.
Figure 10 Principal component analysis of soil characteristics under different graphene applications. Plotted between PC1 and PC2 (A) and plotted between PC1 and PC3 (B).
This study revealed that the application of graphene in oil palm frond mulching resulted in higher electrical conductivity, available phosphorus, exchangeable potassium, and exchangeable calcium compared to conditions without graphene application. This suggests that graphene can increase essential plant nutrients and improve soil properties. Graphene amendment alone yielded high values of soil electrical conductivity and exchangeable potassium, while graphene combined with chemical fertilizer (15-15-15) exhibited high values for available phosphorus and exchangeable potassium. Cover conditions also played a significant role and influenced soil characteristics. Previous investigations into graphene’s structure have highlighted its unique 2-D structure, large surface area, and extraordinary mechanical, optical, thermal, and electrical capabilities (Wang et al., 2011). These features have led to its utilization in agriculture for various purposes, including stimulating plant development, removing contaminants from pesticide and insecticide applications, serving as a component of fertilizer, nano-encapsulation, and providing controlled release (May et al., 2021). When placed around plant roots, graphene, with its negative charge, absorbs cations and aids in the roots’ adsorption and transport of essential cations required by plants (Hu et al., 2017). Moreover, evidence suggests that graphene has the potential to regulate the soil microenvironment and has a specific activating influence on soil enzyme activity, both of which contribute to improved nutrient availability (Lin et al., 2022).
The findings of this study are supported by recent reports. Kabiri et al. (2017) investigated the loading capacity, release properties in soil and solution, and plant availability of unique graphene-based micronutrient fertilizers. Their results demonstrated that graphene oxide sheets slowly and continuously delivered micronutrients such as zinc and copper. Hammerschmiedt et al. (2023) evaluated the combined impact of elemental nano-sulfur and graphene oxide on the pH, biological characteristics, and dry biomass of lettuce plants in controlled environments. They found that graphene usage did not significantly affect soil pH, above-ground plant biomass, or the root system. Graphene oxide alone notably enhanced soil respiration and arylsulfatase activity, and when combined with nano-sulfur, it increased arylsulfatase, urease, and phosphatase activity in the soil. Wang et al. (2023) assessed the effect of varying amounts of graphene amendment on soil physicochemical parameters, nutritional content, and maize seedling development. They observed an increase in the proportion of large agglomerates in the soil due to graphene, leading to a considerable increase in geometric mean diameter and mean weight diameter. Water-stable agglomerates also showed increased concentration, enhancing soil nutrient availability. Additionally, graphene increased the aboveground levels of total nitrogen, total phosphorus, and total potassium in maize plants, improving their nutrient absorption capacity and resulting in higher aboveground fresh weight, dry weight, plant height, and stalk thickness. In a recent study, Alessandrino et al. (2023) examined three differently textured soils that were amended with traditional soil conditioners (compost, biochar, and zeolites) along with graphene, which provided interesting results. The conditioners were applied at two different doses: 10% and 5% dry weight for compost, biochar, and zeolites, and 1.0% and 0.5% dry weight for graphene. Their findings suggested that an increase in drainage porosity due to the conditioner application might be accompanied by a reduction in storage porosity. This implies that the use of graphene to improve soil aeration and drainage conditions is feasible, particularly in fine soils. The enhancement of soil fertility retention was the primary manifestation of graphene’s promoting effect. In comparison to soil amended with graphene, which facilitated nutrient uptake and utilization, the experiment revealed that soil lacking graphene experienced greater soluble nutrient runoff following rainfall and washing. Additionally, the soil’s structure underwent alterations during leaching. Graphene, when added to the soil, strengthened the soil’s agglomeration structure and microagglomerates. This bolstered the soil’s resistance to erosion and increased soil fertility. Consequently, graphene contributes significantly to soil fertility preservation (Liu et al., 2023).
Our findings revealed that the application of graphene accelerated the decomposition of oil palm frond mulch, as evidenced by the weight loss percentages of the frond mulches and changes in nutrient status over time. This research provides initial scientific evidence on the impact of graphene amendment on the decomposition of oil palm frond mulch and soil health status, demonstrating enhanced nutrient availability. The results of this pilot study are valuable for planning long-term research experiments and offer practical insights for farmers to efficiently utilize graphene for managing oil palm waste, such as frond mulches, to improve soil health. Depending on the availability of frond biomass, farmers can adjust their fertilizer management plans by incorporating graphene and oil palm fronds, thereby reducing fertilizer input costs. However, further research is needed to explore the long-term effects of graphene on nutrient cycling, microbial activity, and the economic implications for farm management.
Different graphene amendments in oil palm frond mulching and cover conditions affected soil properties throughout the observed months. Graphene alone in oil palm frond mulching resulted in higher soil electrical conductivity and exchangeable potassium. Combining graphene with chemical fertilizer led to increased soil available phosphorus and exchangeable calcium. Cover conditions influenced soil pH, available phosphorus, and exchangeable calcium, but did not significantly affect soil OM, exchangeable potassium, or exchangeable magnesium. Correlation results indicated significant relationships among soil characteristics under graphene applications. Principal component analysis revealed that graphene treatments dominated most soil property characteristics, including OM, pH, electrical conductivity, phosphorus, potassium, calcium, and magnesium. Graphene emerges as a sustainable soil amendment, enhancing nutrient availability and accelerating frond decomposition. This study provides scientific evidence supporting graphene’s positive impact on soil health properties, suggesting its adoption for sustainable soil, nutrients, and frond waste management in oil palm farms. Furthermore, the authors suggest long-term experimentation to assess graphene’s effects on crucial soil properties in oil palm farm soils.
The original contributions presented in the study are included in the article/supplementary material. Further inquiries can be directed to the corresponding author.
TK: Conceptualization, Formal analysis, Methodology, Project administration, Writing – original draft, Writing – review & editing. TH: Formal analysis, Writing – original draft, Writing – review & editing.
The author(s) declare financial support was received for the research, authorship, and/or publication of this article. This research was partially funded by the Program Management Unit on Area Based Development, Office of National Higher Education Science Research and Innovation Policy Council (grant number A17F650109).
The experimental materials, experiment field, and facilities were supported by the School of Agricultural Technology and Food Industry (SAF) and the Center for Scientific and Technological Equipment, Walailak University, which is gratefully acknowledged by the authors. We are thankful to the undergraduate students of SAF for preparing the experimental field, collecting soil samples, and analyzing soil properties.
The authors declare that the research was conducted in the absence of any commercial or financial relationships that could be construed as a potential conflict of interest.
All claims expressed in this article are solely those of the authors and do not necessarily represent those of their affiliated organizations, or those of the publisher, the editors and the reviewers. Any product that may be evaluated in this article, or claim that may be made by its manufacturer, is not guaranteed or endorsed by the publisher.
Ajayi S. M., Olusanya S. O., Didunyemi A. E., Olumayede E. G. (2023). Assessment of cellulose from oil palm waste for paper-based applications. Materials Lett. 349, 134782. doi: 10.1016/j.matlet.2023.134782
Alessandrino L., Pavlakis C., Colombani N., Mastrocicco M., Aschonitis V. (2023). Effects of graphene on soil water-retention curve, van Genuchten parameters, and soil pore size distribution – A comparison with traditional soil conditioners. Water 15, 1297. doi: 10.3390/w15071297
Andelkovic I. B., Kabiri S., Tavakkoli E., Kirby J. K., McLaughlin M. J., Losic D. (2018). Graphene oxide-Fe (III) composite containing phosphate–A novel slow release fertilizer for improved agriculture management. J. Clean. Prod 185, 97–104. doi: 10.1016/j.jclepro.2018.03.050
Asif F. C., Saha G. C. (2023). Graphene-like carbon structure synthesis from biomass pyrolysis: a critical review on feedstock–process–properties relationship. C 9, 31. doi: 10.3390/c9010031
Corley R. H. V., Tinker P. B. (2003). The oil palm. 4th ed (Oxford, UK: Blackwell Publishing), 284. doi: 10.1002/9780470750971
De Mendiburu F. (2023) Agricolae: statistical procedures for agricultural research. Available online at: https://cran.r-project.org/web/packages/agricolae/index.html.
Duangpan S., Tongchu Y., Hussain T., Eksomtramage T., Onthong J. (2022). Beneficial effects of silicon fertilizer on growth and physiological responses in oil palm. Agronomy 12, 413. doi: 10.3390/agronomy12020413
Food and Agriculture Organization (2020). Soil Testing Methods-Global Soil Doctors Programme-A farmer-to-farmer training programme (Rome: Food and Agriculture Organization of the United Nations). doi: 10.4060/ca2796en
Hammerschmiedt T., Holatko J., Zelinka R., Kintl A., Skarpa P., Bytesnikova Z., et al. (2023). The combined effect of graphene oxide and elemental nano-sulfur on soil biological properties and lettuce plant biomass. Front. Plant Sci. 14. doi: 10.3389/fpls.2023.1057133
He Y., Qian L., Liu X., Hu R., Huang M., Liu Y., et al. (2018). Graphene oxide as an antimicrobial agent can extend the vase life of cut flowers. Nano Res. 11, 6010–6022. doi: 10.1007/s12274-018-2115-8
He C., Zhang Y., Li Y., Zhuo X., Li Y., Zhang C., et al. (2020). In-house standard method for molecular characterization of dissolved organic matter by FT-ICR mass spectrometry. ACS Omega. 5, 11730–11736. doi: 10.1021/acsomega.0c01055
Hong L. S., Ibrahim D., Che-omar I. (2012). Oil palm frond for the production of bioethanol. Int. J. Biochem. Biotechn 1, 007–011.
Hossain M. Z., Bahar M. M., Sarkar B., Donne S. W., Ok Y. S., Palansooriya K. N., et al. (2020). Biochar and its importance on nutrient dynamics in soil and plant. Biochar 2, 379–420. doi: 10.1007/s42773-020-00065-z
Hu M. C., Yao Z. H., Wang X. Q. (2017). Graphene-based nanomaterials for catalysis. Ind. Eng. Chem. Res. 56, 3477–3502. doi: 10.1021/acs.iecr.6b05048
Hussain N., Ahmed M., Duangpan S., Hussain T., Taweekun J. (2021). Potential impacts of water stress on rice biomass composition and feedstock availability for bioenergy production. Sustainability 13, 10449. doi: 10.3390/su131810449
Hussain T., Gollany H. T., Hussain N., Ahmed M., Tahir M., Duangpan S. (2022). Synchronizing nitrogen fertilization and planting date to improve resource use efficiency, productivity, and profitability of upland rice. Front. Plant Sci. 13. doi: 10.3389/fpls.2022.895811
Hussain T., Gollany H. T., Mulla D. J., Ben Z., Tahir M., Ata-Ul-Karim S. T., et al. (2023). Assessment and application of EPIC in simulating upland rice productivity, soil water, and nitrogen dynamics under different nitrogen applications and planting windows. Agronomy 13, 2379. doi: 10.3390/agronomy13092379
IUSS Working Group WRB (2022). “World reference base for soil resources,” in International soil classification system for naming soils and creating legends for soil maps, 4th edition (International Union of Soil Sciences (IUSS, Vienna, Austria).
Kabiri S., Degryse F., Tran D. N. H., Silva R. C., McLaughlin M. J., Losic D. (2017). Graphene oxide: A new carrier for slow release of plant micronutrients. ACS Appl. Mater. Interfaces. 9, 43325–43335. doi: 10.1021/acsami.7b07890
Lin J. J., Ma K. Y., Chen H. H., Chen Z. L., Xing B. S. (2022). Influence of different types of nanomaterials on soil enzyme activity: A global meta-analysis. Nano Today 42, 101345. doi: 10.1016/j.nantod.2021.101345
Liu Z., Zhou M., Liao W., Liu J., Luo C., Lu C., et al. (2023). Fertilizer-holding performance of graphene on soil colloids based on double electric layer theory. Materials 16, 2578. doi: 10.3390/ma16072578
May A., Coelho L. F., Silva E. H. F. M., Viana R. S., Junior N. A. V., Ferreira W. P. M. (2021). Graphene: A new technology for agriculture. Res. Soc Dev. 10, e56610212827. doi: 10.33448/rsd-v10i2.12827
Office of Agricultural Economics (2023) Oil palm data. Available online at: https://www.oae.go.th/view/1/%E0%B8%AB%E0%B8%99%E0%B9%89%E0%B8%B2%E0%B9%81%E0%B8%A3%E0%B8%81/TH-TH.
Pulunggono H., Anwar S., Mulyanto B., Sabiham S. (2019). Decomposition of oil palm frond and leaflet residues. AGRIVITA J. Agric. Sci. 41, 524–536. doi: 10.17503/Agrivita
Vu V. Q., Friendly M., Tavadyan A. (2024) Package ‘ggbiplot’: A grammar of graphics implementation of biplots. Available online at: https://github.com/friendly/ggbiplot.
Wang S., Liu Y., Wang X., Xiang H., Kong D., Wei N., et al. (2023). Effects of concentration-dependent graphene on maize seedling development and soil nutrients. Sci. Rep. 13, 2650. doi: 10.1038/s41598-023-29725-3
Wang K., Ruan J., Song H., Zhang J., Wo Y., Guo S., et al. (2011). Biocompatibility of graphene oxide. Nanoscale Res. Lett. 6, 1–8. doi: 10.1007/s11671-010-9751-6
Wickham H., François R., Henry L., Müller K., Vaughan D., Software P. (2023) dplyr: A grammar of data manipulation. Available online at: https://cran.r-project.org/web/packages/dplyr/index.html.
Woittiez L. S., Van Wijk M. T., Slingerland M., Van Noordwijk M., Giller K. E. (2017). Yield gaps in oil palm: A quantitative review of contributing factors. Eur. J. Agron. 83, 57–77. doi: 10.1016/j.eja.2016.11.002
Keywords: graphene, oil palm, frond mulching, decomposition, soil fertility
Citation: Khomphet T and Hussain T (2024) Utilization of graphene as an alternative sustainable amendment in improving soil health through accelerated decomposition of oil palm mulch and enhanced nutrient availability. Front. Agron. 6:1383613. doi: 10.3389/fagro.2024.1383613
Received: 07 February 2024; Accepted: 19 April 2024;
Published: 15 May 2024.
Edited by:
Domenico Ronga, University of Salerno, ItalyReviewed by:
Muhammad Saqlain Zaheer, Khwaja Fareed University of Engineering and Information Technology (KFUEIT), PakistanCopyright © 2024 Khomphet and Hussain. This is an open-access article distributed under the terms of the Creative Commons Attribution License (CC BY). The use, distribution or reproduction in other forums is permitted, provided the original author(s) and the copyright owner(s) are credited and that the original publication in this journal is cited, in accordance with accepted academic practice. No use, distribution or reproduction is permitted which does not comply with these terms.
*Correspondence: Thanet Khomphet, dGhhbmV0LmtoQG1haWwud3UuYWMudGg=
Disclaimer: All claims expressed in this article are solely those of the authors and do not necessarily represent those of their affiliated organizations, or those of the publisher, the editors and the reviewers. Any product that may be evaluated in this article or claim that may be made by its manufacturer is not guaranteed or endorsed by the publisher.
From Frontiers
Learn more about the work of our research integrity team to safeguard the quality of each article we publish.