- 1Soil Fertility Consortium for Southern Africa (SOFECSA) Research Group, Department of Soil Science and Environment, University of Zimbabwe, Harare, Zimbabwe
- 2Faculty of Agricultural, Life and Environmental Science – Agriculture, Food and Nutrition Science Department, University of Alberta, Edmonton, AB, Canada
- 3Department of Biology and Biotechnology, Botswana International University of Science and Technology, Palapye, Botswana
- 4Faculty of Agriculture Environment and Food Systems, University of Zimbabwe, Harare, Zimbabwe
Traditional cereal crops are important for food and nutrition security in rural communities of southern Africa, but their productivity is often constrained by low soil water largely linked to low seasonal rainfall and long intra-seasonal dry spells. Planting basins (PB), tied ridges (TR), and conventional ploughing (CP) were evaluated, over two cropping seasons (2020/2021 and 2021/2022), for their effects on sorghum [Sorghum bicolor (L.), Moench], pearl millet [Pennisetum glaucum (L.) R.Br.], and finger millet [Eleusine coracana (L.) Gaertn] productivity on degraded (<0.4% soil organic carbon) and productive (>0.6% soil organic carbon) fields under rainfed conditions in Mbire (<450 mm rainfall year−1) and Mutasa (>800 mm rainfall year−1) districts in Zimbabwe. Field trials were established on degraded and productive field sites in each district, with sorghum, pearl millet, and finger millet either sown as monocrops or intercropped with cowpea. The experiments were laid out in a 2 × 3 × 3 factorial in a randomized complete block design (RCBD). The highest sorghum grain yield response of 2100 kg ha−1 was attained under PB on productive soils. Overall, PB and TR increased sorghum, finger millet, and pearl millet grain yields by 43% to 58% compared with CP. Growing sorghum, finger millet, and pearl millet on productive soils increased grain yields by 64%, 33%, and 43%, respectively, compared with degraded soils. Intercropping sorghum, pearl millet, and finger millet with cowpea increased cereal yields by between 23% and 42% over the sole crops. Rainwater use efficiency averaged 1 kg grain mm−1 on productive fields and 0.4 kg grain mm−1 on degraded fields. PB produced the highest net profit of $US408 on a productive field. Overall, production of sorghum and millets on productive soils gave positive economic returns irrespective of rainwater management option and cropping system. Conversely, 63% of the treatments on degraded soils recorded negative economic returns in both districts. We conclude that in-field rainwater management technologies combined with other agronomic practices like intercropping increase the productivity of sorghum and millets under rainfed conditions. However, degraded soils remain a challenge for the increased productivity of traditional cereal crops.
1 Introduction
In Zimbabwe, maize is the dominant cereal crop, occupying 45%–50% of cropped area yearly followed by pearl millet and sorghum with 15%–20% and 10%–15%, respectively (FAO, 2020; 2022). Nevertheless, yields of maize, and other crops, have continually decreased due to the declining soil fertility and the suboptimal use of fertilizers (Mtambanengwe and Mapfumo, 2005; Ncube et al., 2007; Mbanyele et al., 2021a), climate change and variability (Lobell et al., 2008; Blanc, 2012; Rurinda et al., 2014; Masson-Delmotte et al., 2021), pest and diseases (Nyamutukwa et al., 2022), weed pressure (Mandumbu et al., 2017; Mtambanengwe et al., 2015), and poor agronomic practices (Mapfumo and Giller, 2001; Namatsheve et al., 2020). Crop Livestock and Fisheries Assessment (CLAFA) surveys conducted by the Government of Zimbabwe between 2019 and 2023 reported low average grain yields of less than 1 t ha−1 for maize, sorghum, finger millet, and pearl millet and against attainable yields of approximately 3–5 t ha−1 (Crop, Livestock And Fisheries Assessment (CLAFA) Reports for Zimbabwe etl al., 2024). Poor seasonal rainfall distribution limits crop productivity the most (Lobell et al., 2008; Rurinda et al., 2015; Nezomba et al., 2018). Intra-seasonal dry spells occur in virtually 80% of the rainfall seasons, especially in semi-arid regions, exposing crops to severe water stress (Rurinda et al., 2013; Barron et al., 2003). Two related studies conducted in Zimbabwe revealed a shift in the geographical boundaries of rainfall zones, with the traditionally high rainfall areas (>800 mm rainfall year−1), the main crop production areas, reducing in area while the dry regions (<450 mm rainfall year−1) have expanded (Mugandani et al., 2012; Manatsa et al., 2020).
Climate change and variability has led to the promotion of traditional cereal crops such as sorghum and millets in most agroecological regions of Zimbabwe, which hitherto depended on maize production (FAO, 2022). Traditional cereal crops have better drought tolerance than maize (FAO, 2008; Kane-Potaka and Kumar, 2019), require less inputs, particularly fertilizers and pesticides (Mukarumbwa and Mushunje, 2010), exhibit long postharvest life (Chazovachii et al., 2012), and are also used for the production of alcoholic and non-alcoholic beverages (Gabaza et al., 2018). Despite these positive attributes, sorghum and millets are still considered as minor cereal crops by famers and are normally grown on marginal lands with limited use of fertilizers (Mabhaudhi et al., 2019). Furthermore, agronomic research on these traditional cereal crops has lagged behind, with most efforts directed to maize (Rukuni et al., 2004).
Smallholder farming areas in Zimbabwe, where the majority population resides, are largely dominated by sandy soils of low water holding capacity, poor organic carbon content, and relatively low aggregate stability (Mapfumo and Giller, 2001). On such soils, rainfall intensities greater than 13 mm h−1 often lead to runoff (Rockström, 2003a; Nyamadzawo et al., 2012). Water uptake by crops is reported to account for merely 15%–30% of the rainfall as 10%–15% is lost as runoff, 10%–30% as deep percolation, and 30%–50% as evaporation (Miriti et al., 2012). Given the increasing importance of sorghum and millets in the context of climate change coupled with the widespread occurrence of sandy soils, in-field water management options are therefore necessary to increase crop productivity and rainwater use efficiency. A potential option for improving rainwater productivity is to reduce non-productive evaporation from soil in favor of productive plant transpiration (Rockström, 2003b; Rockström et al., 2010). In-field rainwater harvesting has been shown, regionally and locally, to increase rainwater productivity in cropping systems (Biazin et al., 2012; Nyamadzawo et al., 2013). Studies conducted in sub-Saharan Africa (SSA), including semi-arid regions of Zimbabwe, showed that in-field rainwater harvesting technologies such as Fanya ju, infiltration pits (chibatamvura), no-till tied ridges, mulch ripping, and planting basins (zai pits) significantly increased soil water capture, retention, rainwater use efficiency, and crop yields (Mupangwa et al., 2013; Nyamadzawo et al., 2013; Chiturike et al., 2023). However, most of these studies centered on maize and grain legumes, with little work focusing on sorghum and millets either as sole or as intercrops. Combining in-field rainwater management (IRWM) options with intercropping of cereals with legumes increases overall crop productivity (Namatsheve et al., 2020; Mbanyele et al., 2021b). The other benefits of intercrops such as suppression of weeds, diseases, and pests; efficient use of soil water, nutrients, and radiation; and dietary diversification are well documented in literature (Namatsheve et al., 2020; Chaudhary and Kohli, 2020).
In most on-farm studies, performance of IRWM technologies on crop productivity has been found to vary spatially and temporally as dictated by rainfall zone, soil type, and soil fertility gradients (Biazin et al., 2012; Nyamadzawo et al., 2013; Mbanyele et al., 2021a; b; Chiturike et al., 2023). It follows then that IRWM technologies should be evaluated across varying soil fertility gradients and contrasting rainfall patterns to widen typologies for adoption by farmers given the diverse biophysical and socioeconomic settings that characterize smallholder farming systems of SSA. Few studies have been carried out regionally, in SSA and Zimbabwe in particular, to evaluate the productivity and profitability of different IRWM options on sorghum and millets across soil fertility gradients and contrasting rainfall zones. Therefore, the specific objectives of this study were to (1) evaluate the performance of selected IRWM options on sorghum and millet productivity on degraded and productive soils in high- and low-rainfall areas, (2) determine rainwater use efficiency of the different IRWM options, and (3) quantify the economic profitability of the different IRWM options.
2 Methodology
2.1 Study sites
The study was conducted in Mutasa (18°33′S, 32°49′E) (Honde Valley) and Mbire (16°34S′, 30°76′E) districts in Zimbabwe (Figure 1). There are 31 wards in the Mutasa district, 14 of which are located in Honde Valley. Honde Valley is located in the eastern highlands of Zimbabwe. The average annual rainfall for the area is approximately 1,150 mm, received from late October to around end of April. The average daily temperature is 21.5°C. November is the hottest month with an average daily temperature of 24.5°C and July is the coolest month with an average daily temperature of 16.3°C (Kanyangarara et al., 2016). Each farmer owns 2 to 3 ha of land. In recent years, farmers in Honde Valley have been making significant yield losses on perennial crops like tea and coffee, which has led to the shift to drought-tolerant cereals and legumes such as finger millet, sorghum, and cowpea (interviews with local farmers in the year 2020). Conventional ploughing (CP) is the commonly used tillage method for maize production.
The Mbire district (16°34′S, 30°76′E) is approximately 4,700 km2 in area and is characterized by the floodplains of the Zambezi River Basin. The Mbire district receives an average annual rainfall of 450–500 mm. There are 17 wards in the Mbire district. A characteristic of this region is the erratic and unreliable rainfall both between and within seasons. The district records high temperatures (sometimes exceeding 40°C) with a mean annual temperature of 25°C (Bola et al., 2014). Each farmer owns 2 to 4 ha of land. CP is the commonly used tillage method, mainly for maize cropping. Sorghum and millets have widely been adopted in the district despite the fact that they are grown on degraded and marginal croplands under CP.
2.2 Selection of experimental sites and germplasm
Consultative meetings were done with the district agricultural extension office (AGRITEX) in the two study areas to ascertain the commonly used IRWM options. A ward is the smallest planning and administrative unit headed by a councillor. Ward 8 (Mutasa district) and Ward 15 (Mbire district) were selected since they are suited for this study. In each ward, focus group discussions (FGDs)1 were then held to select treatments and suitable field sites for evaluating CP (common farmer practice), planting basins (PB), and no-till tied ridges (TR). The FGDs consisted of men and women. All the participants had local farming experience that exceeded 25 years. PB were considered for evaluation in the two districts because they were being promoted by government and non-governmental organizations under different conservation agriculture initiatives (Corbeels et al., 2014). TR were considered on the basis of literature review of research conducted locally, in the region, and SSA at large (Biazin et al., 2012; Miriti et al., 2012; van Rensburg et al., 2012; Nyamadzawo et al., 2013; Nyagumbo et al., 2019). CP was chosen as a baseline (control) treatment.
Transect walks, guided by the participants, were carried out between February and July 2020 to identify and select fields (both degraded and productive) that were accessible and large enough to accommodate all the treatments. Farmers’ local indicators such as common weed species, crop performance, and soil physical attributes were used to distinguish between productive and degraded fields (Nezomba et al., 2017). A degraded field site and a productive field site were selected in each district. The fields had uniform soil type, were on similar catenal position, and had similar historical management. On each field, 10 soil sub-samples were collected to 0.40 m depth along an X shape covering the experimental field plots. The sub-samples were thoroughly mixed to obtain a composite sample. The composite samples were air-dried and sieved using a 2-mm mesh sieve and analyzed for texture, pH, organic carbon, available P, and total N using methods described by Anderson and Ingram (1993). The laboratory results validated the categorization of fields into productive and degraded. The selected experimental fields had a soil organic carbon content of ≥6 and ≤4 g C kg−1 for productive and degraded fields, respectively, consistent with findings by Nezomba et al. (2017) and Kurwakumire et al. (2014). During the FGDs, seeds of local landraces of sorghum, pear millet, and finger millet were identified for use in the field experiments as hybrid varieties were not available. A hybrid cowpea (CBC2) was, however, obtained for use in the intercropping treatments.
2.3 Treatments and experimental design
Field experiments were established on degraded and productive fields using treatments described in Table 1. Each treatment was replicated three times within a field. The experiments were laid out in a 2 × 3 × 3 factorial in a randomized complete block design. The factors were rainwater management option (×3), crop type (×3), and cropping system (×2). The field plots in the Mbire district were 45 m × 24 m with treatment plots measuring 5 m × 4 m. The field plots in the Mutasa district were 30 m ×12 m with treatment plots of 5 m × 2 m in size. The plot sizes in each district were determined by the availability of land to accommodate all the treatments. Inter-subplot and intercrop plot distance was 1 and 0.5 m, respectively, in both districts. The IRWM options were PB, TR, and CP. For TR, ties were constructed in the furrows at 1.5m intervals to create a microdam. Ridges and ties were constructed to 0.3 and 0.2 m in height, respectively. PB of 15 cm width by 15 cm length by 15 cm depth were dug using hand hoes. For CP, experimental plots were tilled to approximately 30 cm depth.
Retained seeds (local landraces) of sorghum, finger millet, and pearl millet were planted under each IRWM option as sole crops or intercropped with cowpea. A semi-erect cowpea cultivar, CBC2 (approximately 115 days to maturity), was planted in the intercropped treatments. The sorghum and millet landraces were early maturing, approximately 90–120 days to maturity (interviews with local farmers in the year 2020). The rows of sorghum and pearl millet had an inter- and intra-spacing of 75 and 20 cm, respectively. Finger millet was sown at 50 cm inter-row and 20 cm within-row spacing. The sorghum/millet seeds were planted at 50 mm soil depth at a seed rate of 10, 8, and 5 kg/ha for sorghum, pearl millet, and finger millet, respectively. For intercrops, cowpea was planted in between rows of sorghum, pearl millet, and finger millet. For all the crops, planting was manually done by hand-placing seeds in planting stations and covering them with a thin layer of soil. Sowing was done soon after the first effective rains in late November to early December. Cattle manure, NPKS, Zn, and Bo were applied as basal fertilizer at planting, and additional N (ammonium nitrate was used) was added as top dressing to sorghum and the millets. The top dressing N fertilizer was applied during flowering of sorghum and millets at a rate of 75 kg N ha−1. The total fertilizer application rates were 14 kg P ha−1, 7 kg K ha−1, 4.5 kg S ha−1, 5 kg Zn ha−1, 5 kg Bo ha−1, 2 t ha−1 cattle manure, and 90 kg N ha−1. The fertilizer application rates were based on general recommendation rates for sandy soils in Zimbabwe (Agronomy Institute, 2002). All treatments received the same rate of nutrient application. Treatments were maintained in the same plots in the second (2021/2022) cropping season. Between the end of the first cropping season and the start of the second season, experimental fields were not fenced, and crop residues were grazed by livestock. This was done to simulate smallholder farming systems in Zimbabwe where livestock roam freely in farmers’ fields during the dry season.
2.4 Measurements
2.4.1 Seasonal rainfall and dry spells
Seasonal length, rainfall amounts, and numbers of dry spell days were determined during the 2020/2021and 2021/2022 cropping seasons. Seasonal length was determined as the period between season commencement and season cessation. To determine the start of the season, we used the Department of Agricultural Technical and Extension Services (AGRITEX) criterion that planting of summer crops commences when in-field rainfall exceeds 25 mm in 7 days (Raes et al., 2004). The end of the cropping season was in March for both the Mutasa and Mbire districts in 2020/2021 and 2021/2022 cropping seasons. Intra-seasonal dry spells were quantified using a criterion developed by Mbanyele et al. (2021a) in similar smallholder farming areas of Zimbabwe. According to Mbanyele et al. (2021a), intra-seasonal dry spells that negatively affect establishment and growth of rainfed cereal crops in Zimbabwe range from 7 to 21 days and are categorized into three classes based on length (number of dry days) as ≥7, ≥14, and ≥21 days. A dry spell was defined as the consecutive number of days within the growth cycle of the crop in which rainfall did not exceed the agronomic threshold value of 2.95 mm (Stern and Cooper, 2011).
Total seasonal rainfall during the 2020/2021 cropping season was 1,419.5 and 776.5 mm for the Mutasa and Mbire districts, respectively, and characterized by high variation (Figure 2). In the 2021/2022 cropping season, seasonal rainfall for the two districts was relatively low, although the Mbire district had uncharacteristically more rainfall (659.5 mm) than the Mutasa district (641mm). There were no intra-seasonal dry spells throughout the entire cropping season in Mbire during the 2020/2021 cropping season, though rainfall amounts were relatively low (Table 2). For both cropping seasons, the month of January had no dry spells in the Mutasa and Mbire districts. In Mutasa during the 2020/2021 cropping season, dry spells in the range of 7–14 days were observed. Similarly, during the 2021/2022 cropping season, dry spells occurred in the range of 7–14 and 14–21 days throughout the cropping season except in the month of January. In both Mbire and Mutasa districts, dry spells of 14–21 days were observed in the months of December 2021 and February 2022, respectively, during the 2021/2022 cropping season.
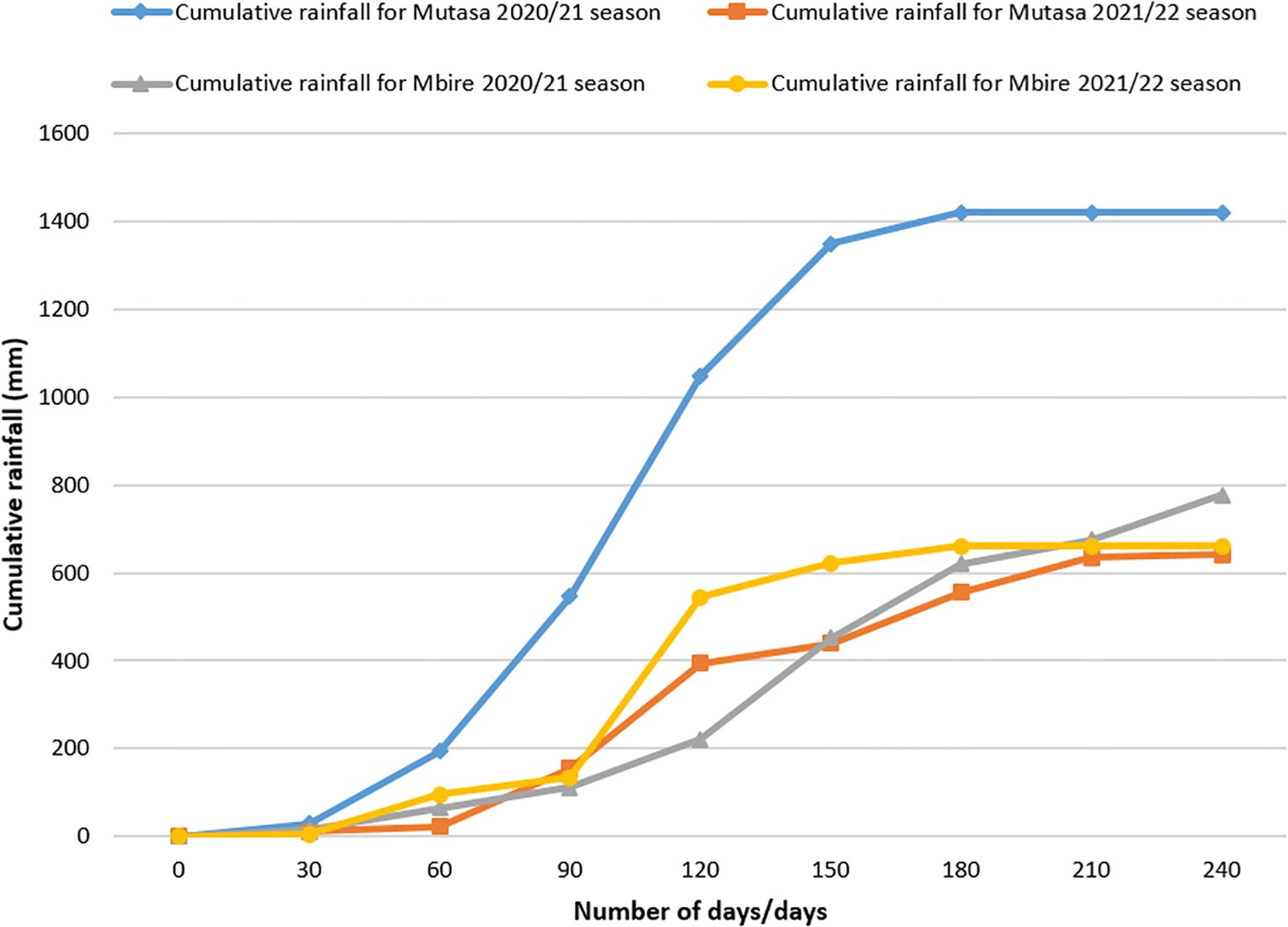
Figure 2. Cumulative seasonal rainfall amounts in the Mutasa and Mbire districts during the 2020/2021 and 2021/2022 cropping seasons.

Table 2. Distribution of intra-seasonal dry spells in the Mutasa and Mbire districts during 2020/2021 and 2021/2022 cropping seasons.
2.4.2 Crop productivity and rainwater use efficiency under IRWM options
Grain yield was quantified at physiological maturity in plots measuring 5 m × 4 m and 5 m × 2 m in the Mbire and Mutasa districts, respectively. For sorghum and millets, ears were removed from the stover and sun-dried to determine dry matter yield, while cowpea grain was separated from the pods. Grain yield was quantified at 12.5% moisture content for sorghum and millets, and 9.5% for cowpea. Overall land productivity was quantified using the partial land equivalent ratio (PLER) equation of Willey and Osiru (1972) as follows:
where Y12 is the grain yield of sorghum/pearl millet/finger millet intercropped with cowpea, whereas Y11 is the yield of sole sorghum/pearl millet/finger millet. Thus, in this case, the PLER expresses how much land in a sole cropping system is needed to produce the same amount of grain yield in an intercropping system.
During the two cropping seasons, daily rainfall was recorded using rain gauges stationed at each of the field sites. The data were used to calculate rainwater use efficiency (RWUE) as grain productivity per total amount of rainfall received between planting and harvesting (in-crop rainfall).
where Y is total grain yield in kg ha−1 and P is in-crop rainfall in mm.
2.4.3 Quantifying economic benefits of treatments
Economic benefits of the different treatments were calculated using gross margins. Economic profitability was calculated as the difference between production costs (labor, seed, herbicides, and fertilizers) and farm gate value of the grain yield. The labor cost per treatment consisted of man days spent on land preparation, planting, weeding, applying fertilizers (inorganic and organic), and crop harvesting. For each activity, starting time, number of people involved, and end time were recorded in farm diaries. The labor hours were converted into monetary value using a local standard wage of US$3 per person per man day (8 h). The cost of mineral fertilizers was based on prices in the local shops, while the cost of sorghum, pearl millet, and finger millet seed was assumed to be the cost of maize seed at the time when the study was being conducted. The value of cattle manure was estimated as many days spent on collecting from cattle kraals and applying to experimental fields since livestock manure is not normally sold in smallholder farming areas in Zimbabwe.
2.5 Statistical analysis
All the data were subjected to normality test using the Shapiro–Wilk method (Shapiro and Wilk, 1965) followed by analysis of variance (ANOVA) using Genstat 15 (VSN International, Hemel Hempstead, UK). In the analysis, which was carried out separately for each cropping season and field type (productive or degraded) to capture variability of seasons, year (season) and replication were considered as random variables and field type and treatments were considered as fixed variables. Treatments’ means were separated at p < 0.05.
3 Results
3.1 Comparative effects of IRWM options on sorghum and millet growth and development
In the Mutasa district, sorghum grain yield under sole crop systems ranged from 200 to 1000 kg ha−1, whereas in intercrop treatments, grain yield ranged from 300 to 1700 kg ha−1 (Figures 3A, B). Overall, sorghum grain yield was significantly (p < 0.05) higher in productive fields compared to degraded fields. Under sole crops, treatment, blocks, and block*treatment interactions were all significant. Sorghum grain yield was highest under PB, followed by TR and least in CP. A similar trend was observed under intercrop systems as treatments and block*treatment interactions were all significant, though yield under intercrop systems was higher (grand mean 900 kg ha−1) compared to sole crops (500 kg ha−1). The productivity of finger millet followed a similar trend to that of sorghum, particularly in the second year (2021/2022), where the highest grain yield of 1200 kg ha−1 was attained under PB on productive fields in both mono- and intercrops. Under low rainfall in the Mbire district, sorghum, pearl millet, and finger millet grain yields were greatly influenced by soil fertility gradient, with productive fields significantly out-yielding degraded fields (Figures 3C, D). Overall, growing sorghum under PB resulted in higher grain yields than under CP. The highest sorghum grain yield of 2100 kg ha−1 was obtained under PB on productive soil in the sorghum–cowpea intercrop. Grain productivity of pearl millet and finger millet followed a similar tend to that of sorghum, with the combination of PB and productive soils attaining better yields than CP in both mono- and intercrops. Aggregated across sites and rainfall seasons, intercropping sorghum, pearl millet, and finger millet with cowpea increased cereal grain productivity by between 23% and 42% compared with the sole crops (Figure 3).
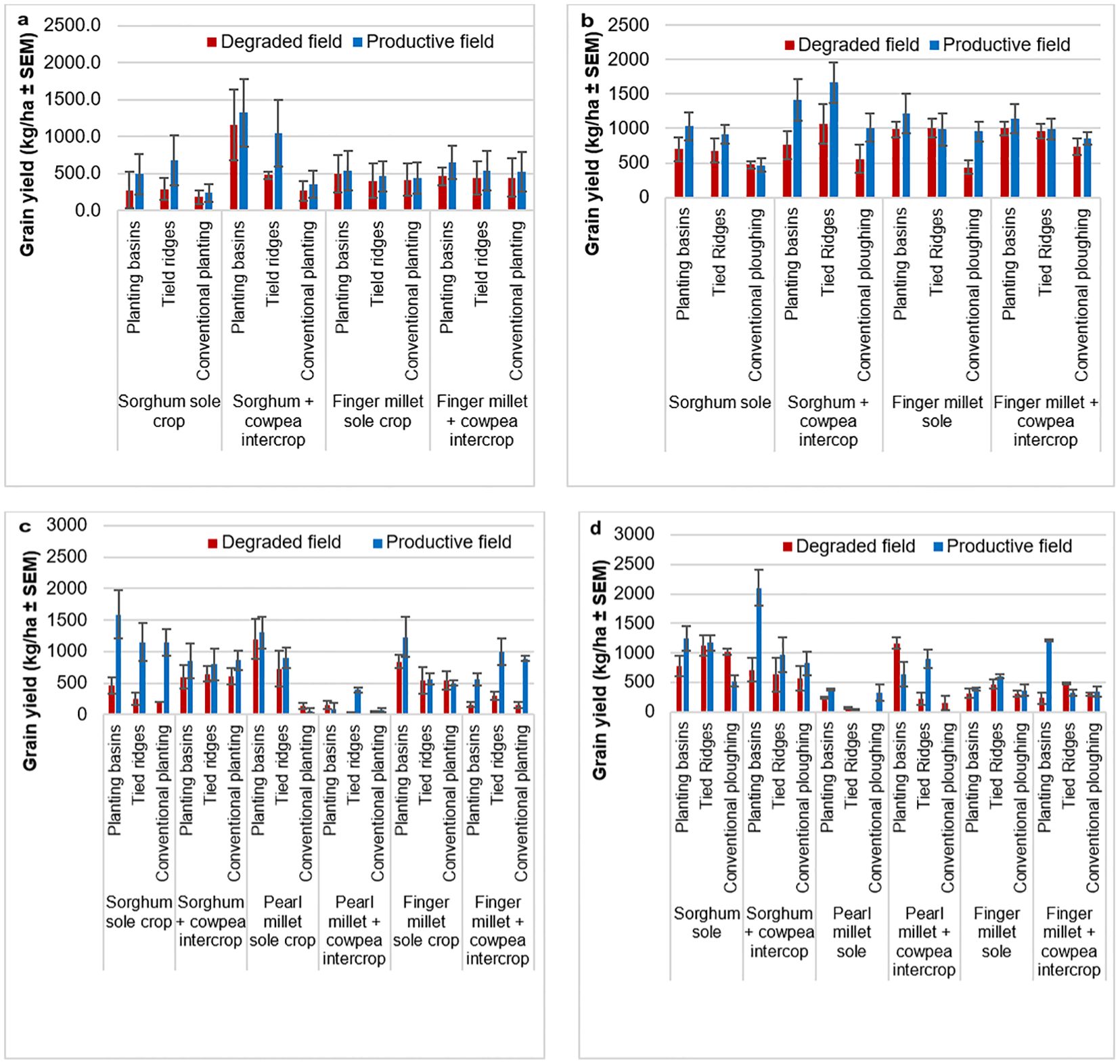
Figure 3. (A, B) Sorghum and finger millet grain yield for the Mutasa district during the (A) 2020/2021 and (B) 2021/2022 cropping seasons. Error bars represent the standard error of mean (SEM). (C, D) Sorghum and millets grain yield for Mbire district during the (C) 2020/2021 and (D) 2021/2022 cropping seasons. Error bars represent SEM.
3.2 Partial land equivalent ratio across treatments
PLERs averaged 1.4 and 1.1 on productive and degraded fields, respectively, indicating the superiority of intercropping over sole cropping (Tables 3A, B). Under low rainfall in the Mbire district (2021/2022 season), PLER was highest when finger millet was intercropped with cowpea on a productive field (Table 3A). During high rainfall in the Mutasa district (2020/2021 season), the highest PLER was recorded in the sorghum–cowpea intercrop on a degraded field (Table 3B).
3.3 Rainwater use efficiency across treatments
In the Mutasa district (2020/2021 season), PB had the best RWUE of 1.9 kg grain mm−1 in the sorghum–cowpea intercrop (productive field) (Figures 4A, B). Overall, PB and TR increased RWUE by 13%–84%, and 7%–46% under sorghum and millet treatments, respectively, during the 2020/2021 cropping season when compared to CP. During the 2021/2022 cropping season, PB and TR increased RWUE by 49%–128% and 30%–69% under sorghum and millet treatments, respectively, compared to CP. There were significant differences in RWUE between TR and PB in the 2020/2021 and 2021/2022 cropping seasons (Figures 4A, B).
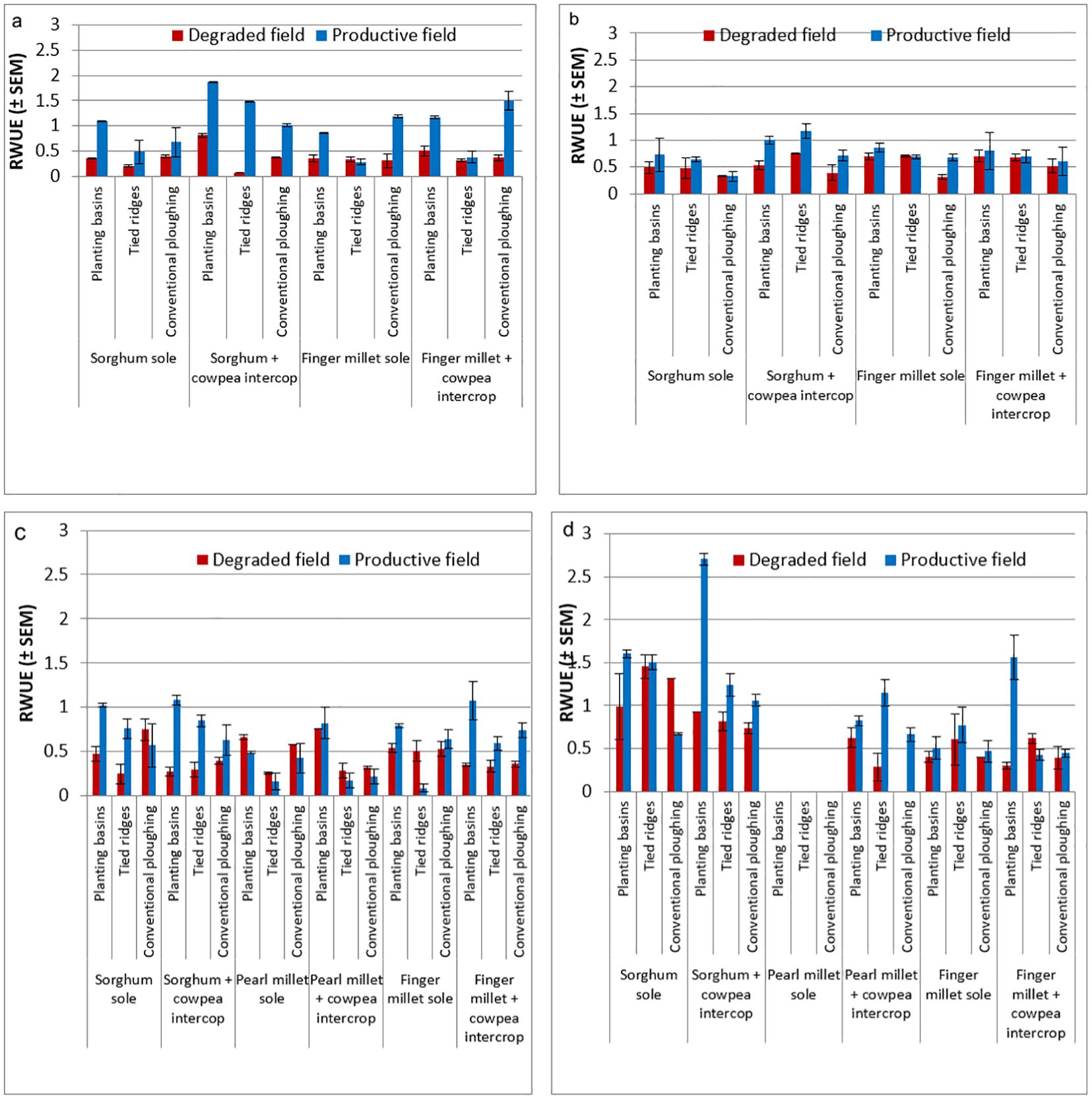
Figure 4. (A, B) Rainwater use efficiency for the Mutasa district for the (A) 2020/2021 and (B) 2021/2022 cropping seasons. Error bars represent the standard error of mean (SEM). (C, D) Rainwater use efficiency for the Mbire district for the (C) 2020/2021 and (D) 2021/2022 cropping seasons. Error bars represent SEM.
In the Mbire district during the 2020/2021 season, PB had the highest RWUE of 1.1 kg grain mm−1 in the sorghum–cowpea intercrop (productive field) (Figures 4C, D). Similarly, the same treatment combination gave the highest RWUE of 2.7 kg grain mm−1 in the 2021/2022 cropping. Overall, PB and TR increased RWUE by 14%–93%, and 6%–33%, respectively, under sorghum and millet treatments during the 2020/2021 cropping season when compared to CP. During the 2021/2022 cropping season, PB and TR increased RWUE by 77%–259%, and 11%–343%, respectively, under sorghum and millet treatments when compared to CP. There were no significant differences in RWUE between TR and PB in the 2020/2021 and 2021/2022 cropping seasons (Figures 4C, D). Aggregated across sites and rainfall seasons, RWUE was significantly higher on productive than on degraded fields.
3.4 Comparative effects of IRWM options on the profitability of sorghum and millet production
In the Mutasa district (2020/2021 season), PB on productive fields produced the highest net profit of $US336 under sole sorghum (Table 4). In the Mbire district, the combination of PB + sorghum–cowpea intercrop on the productive field gave the best economic return of $US180 during the 2020/2021 cropping season. Economic returns were generally low and negative on degraded fields (Table 4). The production of pearl millet on the degraded field in the Mbire district resulted in net losses in both the 2020/2021 and 2021/2022 season, except for the pearl millet–cowpea intercrop +PB treatment, which gave a paltry profitability of $US23 (Table 5). Conversely, most of the treatments gave positive economic returns on the productive field. The finger millet–cowpea intercrop produced the highest net profit of $US408 on a productive field (Table 6). For finger millet, economic profitability averaged $US104 on productive soils and −$US2 on degraded soils.
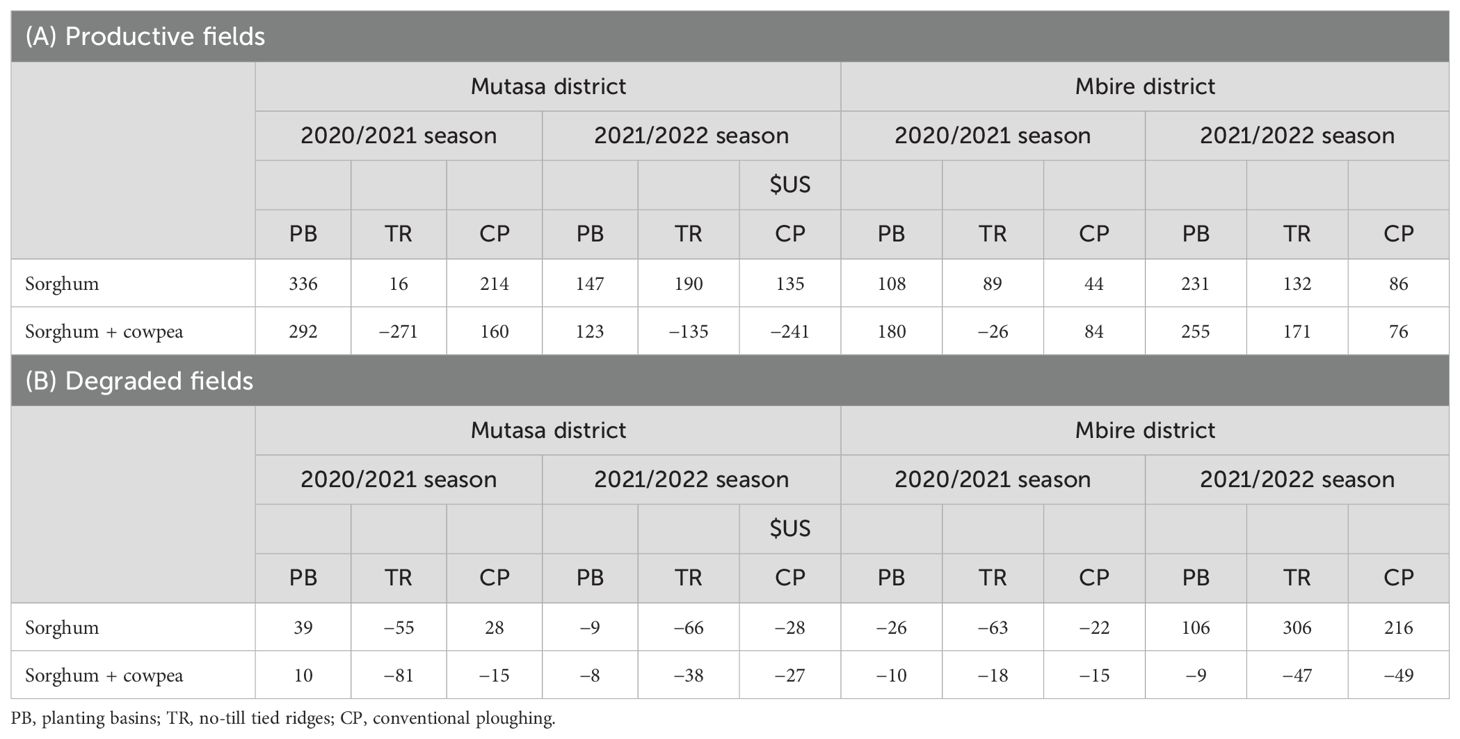
Table 4. Economic profitability ($US) of in-field rainwater management options under sorghum cropping on (A) productive and (B) degraded fields in the Mutasa and Mbire districts.
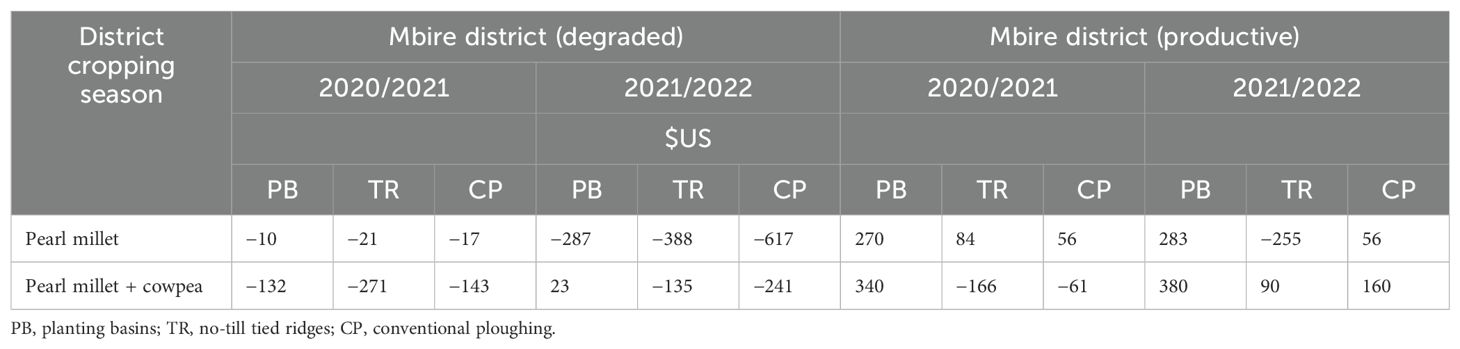
Table 5. Economic profitability ($US) of in-field rainwater management options under pearl millet cropping in the Mutasa and Mbire districts.
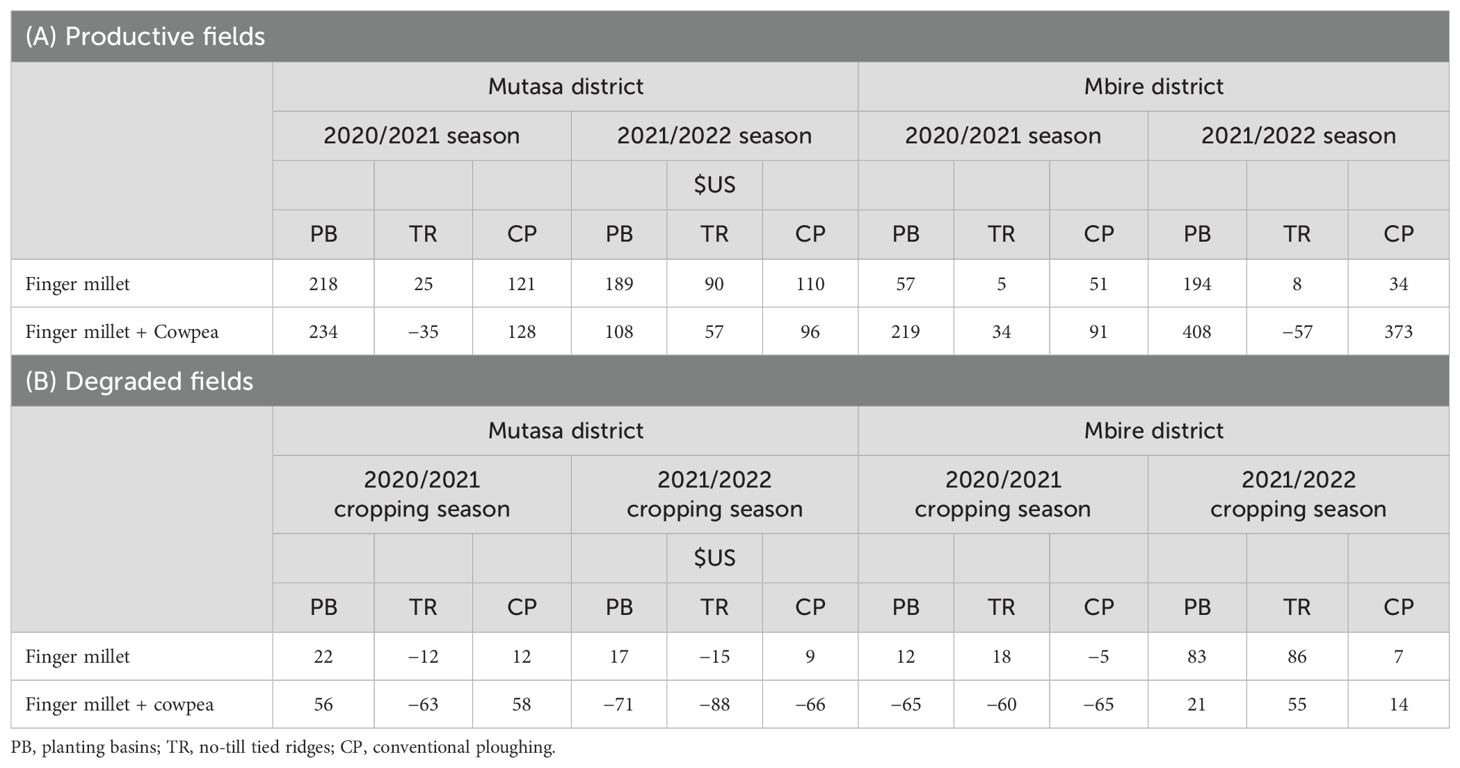
Table 6. Economic profitability ($US) of in-field rainwater management options under finger millet cropping on (A) productive and (B) degraded fields in the Mutasa and Mbire districts.
4 Discussion
Overall, PB and TR increased sorghum, finger millet, and pearl millet grain yield and rainwater use efficiency compared with CP, across sites and cropping seasons. This is in agreement with previous results obtained under similar conditions (Mupangwa et al., 2012a; b; Kubiku et al., 2022; Kugedera et al., 2023). PB intercept, capture, and enhance infiltration and enable excess water to overflow to basin ridges and drain away by diffusion and mass flow to other areas where soil water may be required by plants (Rockström, 2003b; Mupangwa et al., 2012b; Nyamadzawo et al., 2013; Kugedera et al., 2023). The circular nature of PB significantly increases surface area for microbial activity that breaks down organic matter complexes into simpler, digestible, absorbable, and assimilable nutrient elements useful for plant growth, development, and reproductive capacity. The positive impacts of PB on crop productivity bode well for the majority of rural households in Zimbabwe who are widely using PB for in-field soil moisture capture under the government-led Pfumvudza/Intwasa program. Under the Pfumvudza/Intwasa program introduced in the year 2020, the Government of Zimbabwe is assisting smallholder farmers with seeds of sorghum, millets, and other crops to support household crop production in the wake of the changing climate (Mujere, 2021; FAO, 2022; Tanyanyiwa et al., 2022). To date, Pfumvudza/Intwasa has been reported to increase crop yields and household food self-sufficiency in rural communities of Zimbabwe (Mujere, 2021; Parwada et al., 2022; Mavesere and Dzawanda, 2023). The high crop performance under TR could be attributed to the capacity of the IRWM technology to slow down, capture, and tank in-field rain water resulting in increased soil moisture to support crop productivity, particularly during prolonged intra-seasonal dry spells (Mbanyele et al., 2022; Kugedera et al., 2023). In addition to improved water capture, the high grain yield under PB and TR could also be as a result of improved soil fertility from the addition of inorganic and organic nutrient resources close to planting stations. In related studies, placement of fertilizers in planting pits using the “microdosing” concept, as opposed to broadcasting and banding, significantly increased cereal yields in semi-arid in Zimbabwe (Twomlow et al., 2010; Mashingaidze et al., 2013).
Intercrops of sorghum and millets with cowpea increased sorghum and millet productivity and land productivity, particularly on productive fields. These results corroborate with findings in similar cropping systems (Magombeyi et al., 2018; Chaudhary and Kohli, 2020; Namatsheve et al., 2020). Cowpea has been reported to increase dry biomass and grain yield of intercropped sorghum and millets since it is slow growing at early stages of growth, thereby reducing interspecific competition for water, nutrients, and radiation with the companion cereal crop (Chaudhary and Kohli, 2020; Mbanyele et al., 2021b). In addition, cowpea canopy has been reported to provide live mulch cover in cereal–legume intercrops, thereby conserving soil moisture (Mbanyele et al., 2021b) However, there was a decrease in intercrop sorghum and millet productivity in both districts during the wetter season (2020/2021 cropping season) because of severe waterlogging conditions.
Generally, the IRWM options evaluated in this study increased crop productivity on productive fields, but crop yields were rather poor on degraded fields. For example, in both monocrops and intercrops, PB gave significantly higher crop yields and economic returns on productive fields than the counterpart degraded fields in the Mutasa and Mbire districts. Degraded sandy soils are typified by multiple nutrient deficiencies and imbalances and critically low organic matter content, and in some cases, crops respond poorly to the addition of mineral fertilizers on such soils (Nezomba et al., 2015; Zingore et al., 2007; Vanlauwe et al., 2006). Thus, the IRWM options constructed on these fields could not positively impact crop productivity in the short term (two cropping season) most likely because of the soil physicochemical and biological constraints associated with degraded fields. Long-term rehabilitation of these degraded croplands, e.g., through integrated soil fertility management (Nezomba et al., 2015; Zingore et al., 2007), is crucial for improved crop yield response to IRWM. Ironically, degraded fields occupy a large percentage of cropped land in smallholder farming areas in Zimbabwe, and similar areas in SSA (Eswaran et al., 2005; Vlek et al., 2008; Lal, 2009; Zingore et al., 2015). It is thus important for research to develop soil water management and other agronomic typologies targeting productive and degraded fields in order to increase productivity of sorghum and millets and other annual crops in smallholder farming areas of SSA in the wake of soil degradation and the changing climate.
5 Conclusions
We evaluated the effects of IRWM options on the productivity of sorghum and millets under productive and degraded soils in low- and high-rainfall areas in Zimbabwe. Overall, PB achieved the best sorghum and millet productivity on productive fields across rainfall zones, while CP gave the lowest yields. Intercropping of either sorghum or millets with cowpea significantly increased cereal grain yield and net profit in both districts. Sorghum and millet grain yield and net profitability were generally lower on degraded fields than on productive fields. We conclude that IRWM technologies combined with other agronomic practices like intercropping can potentially increase the productivity of sorghum and millets under rainfed conditions, but degraded soils remain a challenge for the increased productivity of traditional cereal crops.
Data availability statement
The original contributions presented in the study are included in the article/supplementary material. Further inquiries can be directed to the corresponding author.
Ethics statement
Ethical review and approval was not required for the study on human participants in accordance with the local legislation and institutional requirements. Written informed consent from the participants was not required to participate in this study in accordance with the national legislation and the institutional requirements.
Author contributions
HN: Conceptualization, Data curation, Formal analysis, Investigation, Methodology, Supervision, Writing – original draft, Writing – review & editing. LM: Conceptualization, Data curation, Formal analysis, Investigation, Methodology, Writing – original draft, Writing – review & editing. AH: Data curation, Formal analysis, Methodology, Writing – original draft, Writing – review & editing. JR: Conceptualization, Data curation, Formal analysis, Investigation, Methodology, Supervision, Writing – original draft, Writing – review & editing. VM: Data curation, Formal analysis, Investigation, Methodology, Software, Writing – original draft, Writing – review & editing. SM: Data curation, Formal analysis, Investigation, Methodology, Software, Writing – original draft, Writing – review & editing. EN: Project administration, Resources, Supervision, Validation, Visualization, Writing – original draft, Writing – review & editing. FM: Funding acquisition, Project administration, Resources, Supervision, Validation, Visualization, Writing – original draft, Writing – review & editing. PM: Conceptualization, Funding acquisition, Project administration, Resources, Validation, Visualization, Writing – original draft, Writing – review & editing.
Funding
The author(s) declare financial support was received for the research, authorship, and/or publication of this article. Funding for this study was provided by the Government of Zimbabwe through the Ministry of Higher and Tertiary Education Innovation Science and Technology Development, and the University of Zimbabwe Vice Chancellor’s Award Challenge Fund under the “Future Grains for Africa” Program.
Conflict of interest
The authors declare that the research was conducted in the absence of any commercial or financial relationships that could be construed as a potential conflict of interest.
Publisher’s note
All claims expressed in this article are solely those of the authors and do not necessarily represent those of their affiliated organizations, or those of the publisher, the editors and the reviewers. Any product that may be evaluated in this article, or claim that may be made by its manufacturer, is not guaranteed or endorsed by the publisher.
Footnotes
- ^ Ethical review and approval was not required for the study on human participants in accordance with the local legislation and institutional requirements. Written informed consent from the participants was not required in accordance with the national legislation and the institutional requirements.
References
Agronomy Institute (2002). Simplified production fact sheets for selected field crops grown in Zimbabwe (Harare, Zimbabwe: Department of Agricultural Research and Extension (AGRITEX), Ministry of Lands, Agriculture and Rural Resettlement). 98 pp.
Anderson J. M., Ingram J. S. I. (1993). Tropical soil biology and fertility: a handbook of methods. 2nd ed. (Wallingford, UK: C.A.B. International).
Barron J., Rockström J., Gichuki F., Hatibu N. (2003). Dry spell analysis and maize yields for two semi-arid locations in east Africa. Agric. For. Meteorol. 117 (1-2), 23–37. doi: 10.1016/S0168-1923(03)00037-6
Biazin B., Sterk G., Temesgen M., Abdulkedir A., Stroosnijder L. (2012). Rainwater harvesting and management in rainfed agricultural systems in sub-Saharan Africa – A review. Phys. Chem. Earth. Parts. A/B/C. 47–48, 139–151. doi: 10.1016/j.pce.2011.08.015
Blanc E. (2012). The impact of climate change on crop yields in sub-saharan africa. AJCC 01, 1–13. doi: 10.4236/ajcc.2012.11001
Bola G., Mabiza C., Goldin J., Kujinga K., Nhapi I., Makurira H., et al. (2014). Coping with droughts and floods: A Case study of Kanyemba, Mbire District, Zimbabwe. Phys. Chem. Earth. Parts. A/B/C. 67–69, 180–186. doi: 10.1016/j.pce.2013.09.019
Chaudhary P., Kohli S. K. (2020). Impact of climate change on smallholder agriculture and role of sustainable land management practices: A review. ISWCR 8, 177–186. doi: 10.1016/j.iswcr.2020.03.004
Chazovachii B., Chigwenya A., Mushuku A. (2012). Adoption of climate resilient rural livelihoods through growing of small grains in Munyaradzi communal area, Gutu District. Afr. J. Agric. Res. 7 (8), 1335–1345. doi: 10.5897/AJAR10.921
Chiturike P., Nyamadzawo G., Gotosa J., Mandumbu R., Nyakudya I. W., Kubiku F. N. M., et al. (2023). Evaluation of different rainwater harvesting techniques for improved maize productivity in semi-arid regions of Zimbabwe with sandy soils. J. Sustain. Agric. Environ. 2, 26–39. doi: 10.1002/sae2.12033
Corbeels M., De Graaff J., Ndah T. H., Penot E., Baudron F., Naudin K., et al (2014). Understanding the impact and adoption of conservation agriculture in Africa: A multi-scale analysis. Agric. Ecosyst. Environ. 187, 155–170. doi: 10.1016/j.agee.2013.10.011
Eswaran H., Almaraz R., van den Berg E., Reich P. (2005). An assessment of the Soil Resources of Africa in Relation to Productivity (Washington, D.C: World Soil Resources, Soil Survey Division, USDA, Natural Resources Conservation Service).
FAO (2008). Climate change adaptation and mitigation in the food and agriculture sector. Technical background document from the expert consultation, (No. CCLXVII.5/4). Rome, Italy: Food and Agriculture Organization of the United Nations (accessed August 2023).
FAO (2020). FAOSTAT crop production. Rome, Italy: Food and Agriculture Organization of the United Nations. Available online at: www.fao.org/faostat/en/home. (accessed July 2023).
FAO (2022). Evaluation of the Zimbabwe livelihoods and food security programme. project evaluation series (Rome, Italy: Food and Agriculture Organization of the United Nations). (accessed July 2023).
Gabaza M., Shumoy H., Louwagie L., Muchuweti M., Vandamme P., Du Laing G., et al. (2018). Traditional fermentation and cooking of finger millet: Implications on mineral binders and subsequent bioaccessibility. J. Food Compos. Anal. 68, 87–94. doi: 10.1016/j.jfca.2017.05.011
Crop, Livestock And Fisheries Assessment (CLAFA) Reports for Zimbabwe. Food Security Cluster (FSC), Food and Agriculture Organization of the United Nations (FAO) and World Food Programme (WFP) (2024). Available at: https://fscluster.org (Accessed May 2024)
Kane-Potaka J., Kumar P. (2019). “Smart food - food that is good for you, the planet and the farmer,” in State of India's Livelihoods Report 2019 (Access Development Services, New Delhi), 71–82. Available at: https://livelihoods-India.org/publications/all-page-soil-report.htmlwww.smartfood.org/wp-content/uploads/2020/05/SOIL-Smart-Foods.pdf.
Kanyangarara M., Mamini E., Mharakurwa S., Munyati S., Gwanzura L., Kobayashi T., et al. (2016). Reduction in malaria incidence following indoor residual spraying with Actellic 300 CS in a setting with pyrethroid resistance: Mutasa District, Zimbabwe. PloS One 11, e0151971. doi: 10.1371/journal.pone.0151971
Kubiku F. N. M., Nyamadzawo G., Nyamangara J., Mandumbu R. (2022). Effect of contour rainwater harvesting and integrated nutrient management on sorghum yield in semi-arid farming environments of Zimbabwe. Acta Agric. Scand. Sect. B- Plant Soil Sci. 72, 364–374. doi: 10.1080/09064710.2021.2005130
Kugedera A. T., Mandumbu R., Nyamadzawo G. (2023). Compatibility of Leucaena leucocephala biomass and cattle manure combination under rainwater harvesting on sorghum (Sorghum bicolor (L.) Moench) productivity in semi-arid region of Zimbabwe. J. Plant Nutr. 46 (8), 1580–1600. doi: 10.1080/01904167.2022.2092512
Kurwakumire N., Chikowo R., Mtambanengwe F., Mapfumo P., Snapp S., Johnston A., et al. (2014). Maize productivity and nutrient and water use efficiencies across soil fertility domains on smallholder farms in Zimbabwe. Field Crops Res. 164, 136–114. doi: 10.1016/j.fcr.2014.05.013
Lal R. (2009). Soil degradation as a reason for inadequate human nutrition. Food Secur. 1, 45–57. doi: 10.1007/s12571-009-0009-z
Lobell D. B., Burke M. B., Tebaldi C., Mastrandrea M. D., Falcon W. P., Naylor R. L. (2008). Prioritizing climate change adaptation needs for food security in 2030. Science 319, 607–610. doi: 10.1126/science.1152339
Mabhaudhi T., Chimonyo V. G. P., Hlahla S., Massawe F., Mayes S., Nhamo L., et al. (2019). Prospects of orphan crops in climate change. Planta 250, 695–708. doi: 10.1007/s00425-019-03129-y
Magombeyi M. S., Taigbenu A. E., Barron J. (2018). Effectiveness of agricultural water management technologies on rainfed cereals crop yield and runoff in semi-arid catchment: a meta-analysis. IJAS 16 (4-5), 418–441. doi: 10.1080/14735903.2018.1523828
Manatsa D., Mushore T. D., Gwitira I., Wuta M., Chemura A., Shekede M. D., et al. (2020). Report on Revised Agroecological Zones of Zimbabwe. Bindura University of Science Education, Bindura, Zimbabwe. 131 pp.
Mandumbu R., Mutengwa C., Mabasa S., Mwenje E., Gotosa J., Munyati V. (2017). The parasitic weeds scourge in northern Zimbabwe: Effects of soil degradation, hosts and food security implications to rural farmers. Scientia 20 (3), 86–91. doi: 10.1080/01904167.2022.2092512
Mapfumo P., Giller K. E. (2001). Soil Fertility Management Strategies and Practices by Smallholder Farmers in Semi-Arid Areas of Zimbabwe (Bulawayo, Zimbabwe: International Crops Research Institute for the Semi-Arid Tropics (ICRISAT). 60 pp.
Masson-Delmotte V., Zhai P., Pirani A., Connors S. L., Péan C., Berger S., et al (2021). Climate change 2021: the physical science basis. Contribution of working group I to the sixth assessment report of the intergovernmental panel on climate change. 2 (1), p.2391.
Mashingaidze N., Belder P., Twomlow S., Hove L., Moyo M. (2013). Improving maize (Zea mays L.) performance in semi-arid Zimbabwe through micro-dosing with ammonium nitrate tablets. Exp. Agric. 49 (2), 179–196. doi: 10.1017/S0014479712001020
Mavesere F., Dzawanda B. (2023). Effectiveness of Pfumvudza as a resilient strategy against drought impacts in rural communities of Zimbabwe. GeoJournal 88, 3455–3470. doi: 10.1007/s10708-022-10812-3
Mbanyele V., Mtambanengwe F., Nezomba H., Jeroen C. J., Groot J. C. J., Mapfumo. P. (2021a). Combinations of in-field moisture conservation and soil fertility management reduce effect of intra-seasonal dry spells on maize under semi-arid conditions. Field Crops Res. 270, 108218. doi: 10.1016/j.fcr.2021.108218
Mbanyele V., Mtambanengwe F., Nezomba H., Jeroen C. J., Groot J. C. J., Mapfumo. P. (2021b). Comparative short-term performance of soil water management options for increased productivity of maize-cowpea intercropping in semi-arid Zimbabwe. J. Agric. Food Res. 5, 100189. doi: 10.1016/j.jafr.2021.100189
Mbanyele V., Mtambanengwe F., Nezomba H., Rurinda J., Mapfumo. P. (2022). Conservation agriculture in semi-arid Zimbabwe: A promising practice to improve finger Millet (Eleusine coracana Gaertn.) productivity and soil water availability in the short term. Agriculture 12, 622. doi: 10.3390/agriculture12050622
Miriti J. M., Kironchi G., Esilaba A. O., Heng L. K., Gachene C. K. K., Mwangi D. M. (2012). Yield and water use efficiencies of maize and cowpea as affected by tillage and cropping systems in semi-arid Eastern Kenya. Agric. Water Manage. 115, 148–155. doi: 10.1016/j.agwat.2012.09.002
Mtambanengwe F., Mapfumo P. (2005). Organic matter management as an underlying cause for soil fertility restoration in smallholder farming systems of Zimbabwe. Nutr. Cycling. Agroecosyst. 73, 227–243. doi: 10.1007/s10705-005-2652-x
Mtambanengwe F., Nezomba H., Tauro T., Chagumaira C., Manzeke M. G., Mapfumo P. (2015). Mulching and fertilization effects on weed dynamics under conservation agriculture-based maize cropping in Zimbabwe. Environments 2, 399–414. doi: 10.3390/environments2030399
Mugandani R., Wuta M., Makarau A., Chipindu B. (2012). Re-classification of agro-ecological regions of Zimbabwe in conformity with climate variability and change. Afr. Crop Sci. J. 20, 361–369.
Mujere N. (2021). Assessing the potential contribution of Pfumvudza towards climate-smart agriculture in Zimbabwe: a review. Preprints, 2021010619 doi: 10.20944/preprints202101.0619.v1
Mukarumbwa P., Mushunje A. (2010). “Potential of sorghum and finger millet to enhance household food security in Zimbabwe’s semi-arid regions: A review,” in Paper presented at the Joint 3rd African Association of Agricultural Economists (AAAE) and 48th Agricultural Economists Association of South Africa (AEASA) Conference, Cape Town, South Africa, September 19-23, 2010.
Mupangwa W., Twomlow S., Walker S. (2012a). Dead level contours and infiltration pits for risk mitigation in smallholder cropping systems of southern Zimbabwe. Phys. Chem. Earth. Parts. A/B/C. 47–48, 166–172. doi: 10.1016/j.pce.2011.06.011
Mupangwa W., Twomlow S., Walker S. (2012b). Reduced tillage, mulching and rotational effects on maize (Zea mays L.), cowpea (Vigna unguiculata (Walp) L.) and sorghum (Sorghum bicolor L. (Moench)) yields under semi-arid conditions. Field Crops Res. 132, 139–148. doi: 10.1016/j.fcr.2012.02.020
Mupangwa W., Twomlow S., Walker S. (2013). Cumulative effects of reduced tillage and mulching on soil properties under semi-arid conditions. J. Arid Environ. 91, 45–52. doi: 10.1016/j.jaridenv.2012.11.007
Namatsheve T., Cardinael R., Corbeels M., Chikowo. R. (2020). Productivity and biological N2-fixation in cereal-cowpea intercropping systems in sub-Saharan Africa. A review. Agron. Sustain. Dev. 40, 30. doi: 10.1007/s13593-020-00629-0
Ncube B., Twomlow S. J., van Wijk M. T., Dimes J. P., Giller K. E. (2007). Productivity and residual benefits of grain legumes to sorghum under semi-arid conditions in southwestern Zimbabwe. Plant Soil 299, 1–15. doi: 10.1007/s11104-007-9330-5
Nezomba H., Mtambanengwe F., Rurinda J., Mapfumo P. (2018). Integrated soil fertility management sequences for reducing climate risk in smallholder crop production systems in southern Africa. Field Crops Res. 224, 102–114. doi: 10.1016/j.fcr.2018.05.003
Nezomba H., Mtambanengwe F., Tittonell P., Mapfumo P. (2015). Point of no return? Rehabilitating degraded soils for increased crop productivity on smallholder farms in eastern Zimbabwe. Geoderma 239–240, 143–155. doi: 10.1016/j.geoderma.2014.10.006
Nezomba H., Mtambanengwe F., Tittonell P., Mapfumo P. (2017). Practical assessment of soil degradation on smallholder farmers' fields in Zimbabwe: Integrating local knowledge and scientific diagnostic indicators. Catena 156, 216–227. doi: 10.1016/j.catena.2017.04.014
Nyagumbo I., Nyamadzawo G., Madembo C. (2019). Effects of three in-field water harvesting technologies on soil water content and maize yields in a semi-arid region of Zimbabwe. Agric. Water Manag. 216, 206–213. doi: 10.1016/j.agwat.2019.02.023
Nyamadzawo G., Nyamugafata P., Wuta M., Nyamangara J., Chikowo R. (2012). Infiltration and runoff losses under fallowing and conservation agriculture practices on contrasting soils, Zimbabwe. Water SA. 38, 233–240. doi: 10.4314/wsa.v38i2.8
Nyamadzawo G., Wuta M., Nyamangara J., Gumbo D. (2013). Opportunities for optimization of in-field water harvesting to cope with changing climate in semi-arid smallholder farming areas of Zimbabwe. SpringerPlus 2, 100. doi: 10.1186/2193-1801-2-100
Nyamutukwa S., Mvumi B. M., Chinwada P. (2022). Sustainable management of fall armyworm, Spodoptera frugiperda (JE Smith): challenges and proposed solutions from an African perspective. Int. J. Pest Manage., 1–19. doi: 10.1080/09670874.2022.2027549
Parwada C., Chipomho J., Mandumbu. R. (2022). “Building resilience to climate change by adopting conservation agriculture in the smallholder farming systems,” in Food Security for African Smallholder Farmers. Eds. Mupambwa H. A., Nciizah A. D., Nyambo P., Muchara B., Gabriel N. N., 281–294. Gateway East, Singapore: Sustainable Agriculture and Food Security. doi: 10.1007/978-981-16-6771-8_17
Raes D., Sithole A., Makarau A., Milford J. (2004). Evaluation of first planting dates recommended by criteria currently used in Zimbabwe. Agric. For. Meteorol. 125, 177–185. doi: 10.1016/j.agrformet.2004.05.001
Rockström J. (2003a). Resilience building and water demand management for drought mitigation. Phys. Chem. Earth. Parts. A/B/C. 28, 869–877. doi: 10.1016/j.pce.2003.08.009
Rockström J. (2003b). Water for food and nature in drought–prone tropics: vapour shift in rain– 626fed agriculture. Phil. Trans. R. Soc Lond. B. 358, 1997–2009. doi: 10.1098/rstb.2003.1400
Rockström J., Karlberg L., Wani S. P., Barron J., Hatibu N., Oweis T., et al. (2010). Managing water in rainfed agriculture—The need for a paradigm shift. Agric. Water Manag. 97, 543–550. doi: 10.1016/j.agwat.2009.09.009
Rukuni M., Tawonezvi P., Eicher C., Munyuki-Hungwe M., Matondi P. (2004). Zimbabwe’s agricultural revolution revisited (Harare, Zimbabwe: University of Zimbabwe Publications).
Rurinda J., Mapfumo P., Van Wijk M. T., Mtambanengwe F., Rufino M. C., Chikowo R., et al. (2013). Managing soil fertility to adapt to rainfall variability in smallholder cropping systems in Zimbabwe. Field Crops Res. 154, 211–225. doi: 10.1016/j.fcr.2013.08.012
Rurinda J., Mapfumo P., van Wijk M. T., Mtambanengwe F., Rufino M. C., Chikowo R., et al. (2014). Comparative assessment of productivity of maize, finger millet and sorghum for household food security in the face of increasing climatic risk. Eur. J. Agron. 55, 29–41. doi: 10.1016/j.eja.2013.12.009
Rurinda J., van Wijk M. T., Mapfumo P., Descheemaeker K., Supit I., Giller K. E. (2015). Climate change and maize yield in southern Africa: what can farm management do? Glob. Change Biol. 21 (2), 4588–4601. doi: 10.1111/gcb.13061
Shapiro S. S., Wilk M. B. (1965). An analysis of variance test for normality (complete samples). Biometrika. 52 (3–4), 591–611. doi: 10.1093/biomet/52.3-4.591
Stern R. D., Cooper P. J. M. (2011). Assessing climate risk and climate change using rainfall data- A case study from Zambia. Exp. Agric. 47, 241–266. doi: 10.1017/S0014479711000081
Tanyanyiwa V. I., Kanyepi T., Katanha A. (2022). “Zimbabwe’s pfumvudza agriculture programme—Reality or rhetoric?,” in Sustainable Agriculture and Food Security. Eds. Filho W. L., Kovaleva M., Popkova E. (Cham, Switzerland: Springer), 327–347. World Sustainability Series. doi: 10.1007/978-3-030-98617-9
Twomlow S., Rohrbach D., Dimes J., Rusike J., Mupangwa W., Ncube B., et al. (2010). Micro-dosing as a pathway to Africa's Green Revolution: Evidence from broad-scale on-farm trials. Nutr. Cycling. Agroecosyst. 88, 3–15. doi: 10.1007/s10705-008-9200-4
Vanlauwe B., Tittonell P., Mukalama J. (2006). Within-farm soil fertility gradients affect response of maize to fertiliser application in western Kenya. Nutr. Cycl. Agroecosyst. 76, 171–182. doi: 10.1007/s10705-005-8314-1
van Rensburg L. D., Botha J. J., Anderson J. J., Hensley M. (2012). The nature and function of the in-field rainwater harvesting system to improve agronomic sustainability. Irrig. Drain. Syst. 61, 34–40. doi: 10.1002/ird.1678
Vlek P., Le Q. B., Tamene L. (2008). Land decline in Land-Rich Africa– A creeping disaster in the making (Rome, Italy: CGIAR Science Council Secretariat). 55 pp.
Willey W., Osiru D. S. O. (1972). Studies on mixtures of maize and beans (Phaseolus vulgaris) with particular reference to plant population. J. Agric. Sci. 79, 517–529. doi: 10.1017/S0021859600025909
Zingore S., Murwira H. K., Delve R. J., Giller K. E. (2007). Influence of nutrient management strategies on variability of soil fertility, crop yields and nutrient balances on smallholder farms in Zimbabwe. Agric. Ecosyst. Environ. 119, 112–126. doi: 10.1016/j.agee.2006.06.019
Keywords: degraded fields, intercrops, productive fields, rainwater use efficiency, smallholder farming systems
Citation: Makuchete L, Hove A, Nezomba H, Rurinda J, Mbanyele V, Mlambo S, Nyakudya E, Mtambanengwe F and Mapfumo P (2024) Productivity of sorghum and millets under different in-field rainwater management options on soils of varying fertility status in Zimbabwe. Front. Agron. 6:1378339. doi: 10.3389/fagro.2024.1378339
Received: 29 January 2024; Accepted: 23 August 2024;
Published: 04 October 2024.
Edited by:
Marloes van Loon, Wageningen University and Research, NetherlandsReviewed by:
Amit Anil Shahane, Central Agricultural University, IndiaCosmas Parwada, Midlands State University, Zimbabwe
Copyright © 2024 Makuchete, Hove, Nezomba, Rurinda, Mbanyele, Mlambo, Nyakudya, Mtambanengwe and Mapfumo. This is an open-access article distributed under the terms of the Creative Commons Attribution License (CC BY). The use, distribution or reproduction in other forums is permitted, provided the original author(s) and the copyright owner(s) are credited and that the original publication in this journal is cited, in accordance with accepted academic practice. No use, distribution or reproduction is permitted which does not comply with these terms.
*Correspondence: Hatirarami Nezomba, aG5lem9tYmFAYWdyaWMudXouYWMuenc=; aGF0aW5lem9tYmFAZ21haWwuY29t