- 1Department of Plant Science, The Pennsylvania State University, University Park, PA, United States
- 2Intercollege Graduate Degree Program in Ecology, Penn State University, University Park, PA, United States
The soil microbiome can increase crop resilience to both abiotic and biotic stress, and there is growing interest in uncovering the mechanisms by which we can shape plant associated microbiomes to increase crop yields within stressful environments. Through rhizodeposits, plants influence the composition of microbial communities and abiotic conditions in the rhizosphere, potentially generating plant-soil feedbacks which can increase nutrient availability and competitive ability against neighboring plants. Invasive plants have repeatedly been shown to drive plant-soil feedbacks that increase their ability to adapt to a wide range of environmental conditions and suppress neighboring plants. Using invasive plants as model species, we discuss what is currently known about the mechanisms that generate the plant-soil feedbacks that increase plant productivity, competitive ability, and resilience. Specifically, invasive potential is enhanced through 1) positive direct feedback loops which occur within a species, and can occur through enhanced mutualistic associations; or 2) negative indirect feedback loops, when feedbacks affect heterospecific plants through either allelopathy, disruption of mutualistic associations, and increased pathogen abundance. Knowledge on invasive plant exudate-microbe interactions may increase cropping system resilience through breeding superior crop genotypes, or potentially through soil amendments that disrupt weed-microbe interactions. We argue that because the soil biotic and native plant community often evolve in response to negative indirect feedback loops, focusing breeding efforts on positive direct plant-soil feedbacks, such as those that increase mutualistic associations and nutrient availability in the rhizosphere, are likely to lead to long-term stress resilient crops. Future research should explore to what extent upregulating production of specific exudates in non-invasive crop species generate the same plant-soil feedbacks responsible for invasive plant success.
1 Introduction
Increasing crop tolerance to stress is critical as climate change and a growing population put greater strain on agricultural systems. The soil microbiome can increase crop resilience to both abiotic and biotic stress by increasing soil resource availability, as well as by suppressing pathogens and stimulating plant immunity (Chaparro et al., 2012; Trivedi et al., 2021). Therefore, there is growing interest in uncovering the mechanisms by which we can shape plant associated microbiomes to increase crop yield within stressful environments. However, it is not yet clear to what extent we can manipulate microbiomes to our advantage because numerous factors influence the formation of the soil microbiome, including soil type and climate (Fitzpatrick et al., 2020). Through rhizodeposits, plants can also exert a strong influence over the composition of microbial communities in the rhizosphere, the volume of soil directly surrounding the root (Dastogeer et al., 2020). Plant species (Bouffaud et al., 2014), and even plant genotypes (Liu et al., 2019), vary in their effects on the rhizosphere microbiome, with certain species or genotypes exerting a stronger influence over the rhizosphere and surrounding soil microbiome compared to others (Ulbrich et al., 2022). It is through rhizodeposits, that plants may drive plant-soil feedback loops within the soil that increase both their competitive ability and capacity to tolerate abiotic stress.
Plants allocate a significant portion of fixed C belowground. Depending on the biome in question, anywhere from 20 to 75% of a plant’s photosynthate is translocated into belowground biomass (Poorter et al., 2012), and of this between 5 to 21% can be transferred into the soil via rhizodeposits (Wen et al., 2022). Rhizodeposits, such as mucilage, sloughed off root cells, and exudates (see Box 1), serve as important sources of carbon (C) and energy for active microbes in the rhizosphere (Ma et al., 2022), which is why the rhizosphere has greater biomass and activity of microbes compared to the surrounding bulk soil (Berg and Smalla, 2009). Rhizodeposits shape the composition and abundance of microbes within the rhizosphere by acting as signals for microbial recruitment (Dennis et al., 2010), suppressing phytopathogens (Lamichhane et al., 2023), stimulating microbial sporulation (Weisskopf et al., 2006), altering the soil abiotic environment (Bais et al., 2006), and increasing nutrient availability (Wen et al., 2022). Plant root exudates include a diverse array of sugars, amino acids, hormones, as well as other primary and secondary metabolites (Bais et al., 2006) and because of their tremendous diversity and complexity, they can initiate highly specific plant-microbe interactions (Berg and Smalla, 2009), at times fostering rhizosphere microbial communities that support plant growth. Understanding the mechanisms by which certain plant species manipulate the rhizosphere in ways that increase plant productivity under stressful conditions can ultimately lead to selecting for more resilient crop genotypes.
Box 1. Types of rhizodeposits and their associated functions in the rhizosphere.
Rhizodeposits include a wide variety of substances either passively or actively released from active root cells or released into the rhizosphere via lysis of sloughed off root cells (Dennis et al., 2010).
Border cells and border-like cells – are biologically active cells sloughed off from the root cap either attached to other cells in a layer or sheet (border-like) or as detached cells (border cells) (Driouich et al., 2021). Sloughed off cells may constitute 10% of total C released by roots into the soil, which can protect the apical meristem from physical injury and improve soil aggregation in the rhizosphere (Hawes et al., 2000; Driouich et al., 2021). When the cells lyse, their cellular components provide microbial substrates, as well as release antimicrobial proteins and species specific chemotaxic signals for rhizosphere microbes (Dennis et al., 2010; Driouich et al., 2021).
Mucilage – consists of viscoelastic high molecular weight substance primarily secreted through root caps (Driouich et al., 2021; Nazari et al., 2022); which functions as a biofilm around the root surface. Mucilage has similar properties as in extracellular polymeric substances produced by microorganisms and promotes rhizosphere microbial abundance in the rhizosphere (Nazari et al., 2022). Mucilage consists primarily of carbohydrates (almost 80% of mucilage is carbohydrates), followed by proteins, minerals, and lipids, and these compounds serve as major source of C and energy for rhizosphere associated microbes (Nazari, 2021). Mucilage plays an important role in plant defense, as well as preventing metal toxicity and buffering the root against salinity in the soil (Nazari, 2021).
Metabolites – consist of low molecular weight, bioactive metabolites which interact with other plants or soil biota (Bais et al., 2006). Roots exude a diverse repertoire of metabolites that can either be actively transported or passively secreted across the root surface (Dennis et al., 2010; Vives-Peris et al., 2020).
Primary metabolites – include sugars, amino acids, and organic acids that are passively exuded into the soil through specific efflux channels along a concentration gradient, mostly occurring at the root trip (Canarini et al., 2019). Following source-sink dynamics, plants and microbes can both modulate diffusion of primary metabolites by increasing the concentration of primary metabolites in the plant (Xu et al., 2023), or microbes depleting primary metabolites in the soil. The root tip senses primary metabolite concentrations in the soil, relaying information on both plant nutrient status and nutrient availability in the surrounding environment. Primary exudates can be upregulated by the plant in response to nutrient deficiency in the soil (Xu et al., 2023).
Secondary metabolites – exuded by plants can be incredibly diverse, which some studies suggesting that plants can exude as many as one hundred different metabolites into the soil (Strehmel et al., 2014). Classes of secondary metabolites include phenolics, terpenoids, benzoxazinoids, quinones, and alkaloids (Latif et al., 2022). Secondary metabolites serve as classical signals in root-microbe chemotaxis, wherein secondary phytochemicals serve as signals recruiting specific microbes into the rhizosphere from the surrounding soil. The root exudation profile of a plant varies by species, developmental stage, abiotic stimuli, and biotic interactions, including in response to encounters with pathogens and mutualists (Rathore et al., 2023).
Invasive plants often adapt to a wide range of ecosystems and effectively outcompete other plant species (Gioria and Osborne, 2014), potentially revealing novel plant traits that increase resilience to both abiotic and biotic stressors. Historically, it was thought that certain species were invasive based on their competitive traits in accessing light and soil resources or because of release from natural enemies in their invaded range (Colautti et al., 2004; Van Kleunen et al., 2010; Gioria and Osborne, 2014). However, it is now accepted that plant-soil feedbacks greatly contribute towards the invasive potential of more aggressive plant species (Klironomos, 2002; Levine et al., 2006; Kulmatiski et al., 2008). Plant-soil feedbacks occur when soil pre-conditioned by a focal plant affects growth and fitness of either conspecific or heterospecific plants, even after the focal plant (and thereby direct competition) is removed (Bennett and Klironomos, 2019). Plant-soil feedbacks can result from changes to soil chemical and physical properties, as well as to soil biotic communities (Inderjit and Cahill, 2015). Using invasive plants as model species, we may be able to elucidate the rhizosphere mechanisms, and associated plant traits, that generate plant-soil feedbacks increasing plant productivity, competitive ability, and resilience.
Since discovering the important role that plant-soil feedbacks play in increasing invasive potential, multiple distinct mechanisms have been identified (Coats and Rumpho, 2014; Bennett and Klironomos, 2019). These mechanisms differ from those involved solely in resource competition. Recent work reveals how manipulating the microbiome is an important mechanism by which invaders drive feedback loops that enhance their competitive ability. The soil microbiome associated with invasive plants are often (Coats and Rumpho, 2014; Pickett et al., 2019; Li et al., 2022), but not always (Gibbons et al., 2017), altered from native plant communities in the invaded range. Evidence suggests that invasive or other competitive plants invest more resources towards recruiting and supporting beneficial microbes in the rhizosphere compared to native plants (Kaštovská et al., 2015; Yu et al., 2022), and when invasive plants alter the soil microbiome it often promotes invader success (Coats and Rumpho, 2014). Ultimately, invasive plant species can restructure the soil microbial community in ways that facilitate invasions, and these feedback loops are likely driven by their exudate and rhizodeposit profiles (Delory et al., 2023).
Deepening our understanding of the interactions between invasive plants, root exudates, and the soil microbiome may offer guidance on selecting crop genotypes that support a more beneficial microbiome via root exudation, as well as management practices that buffer against soil legacies from weedy competitors. Previous reviews have covered the diversity and functions of root exudates (Bais et al., 2006) and how the microbiome can increase stress tolerance and crop resilience (Trivedi et al., 2021). Additional reviews have also covered the impacts caused by invasive species (Levine et al., 2003) and the important role that plant-soil feedbacks play in increasing invasive potential (Van Der Putten et al., 2013; Inderjit and Cahill, 2015), including through modifications to the soil microbiome (Coats and Rumpho, 2014). In contrast to these previous reviews, using invasive plants as model species, we will discuss what is currently known about the role exudates and other rhizodeposits play in driving invasive plant feedback loops, and how these interactions increase their competitive ability and adaptation to stressful environments. Finally, we will discuss how an understanding of invasive plant-exudate-microbe interactions can be used to inform management decisions and future breeding efforts to increase crop resilience.
2 Invasive plants and plant-soil feedbacks
Plant-soil feedbacks are classified based on whether effects occur within (conspecific) or between (heterospecific) species, as well as the direction of influence (positive, negative, or neutral) (Inderjit and Cahill, 2015; Bennett and Klironomos, 2019). Direct effects occur when a feedback loop affects conspecific individuals, including that individual plant or other individuals within that species (Van Der Putten et al., 2013). Indirect effects include when a plant’s influence on the soil affects growth of heterospecific individuals (Van Der Putten et al., 2013). Both direct and indirect effects can be positive, negative, or neutral (Bennett and Klironomos, 2019). However, only positive direct (positive effects within a species) or negative indirect (negative effects to other species) feedback loops result in increased competitive ability, population growth, and range expansion of a species (see Figure 1). Therefore, this review will focus on what is currently known about the role of root rhizodeposits in driving both negative indirect and positive direct feedback loops, and the extent to which these effects are mediated through the soil microbiome.
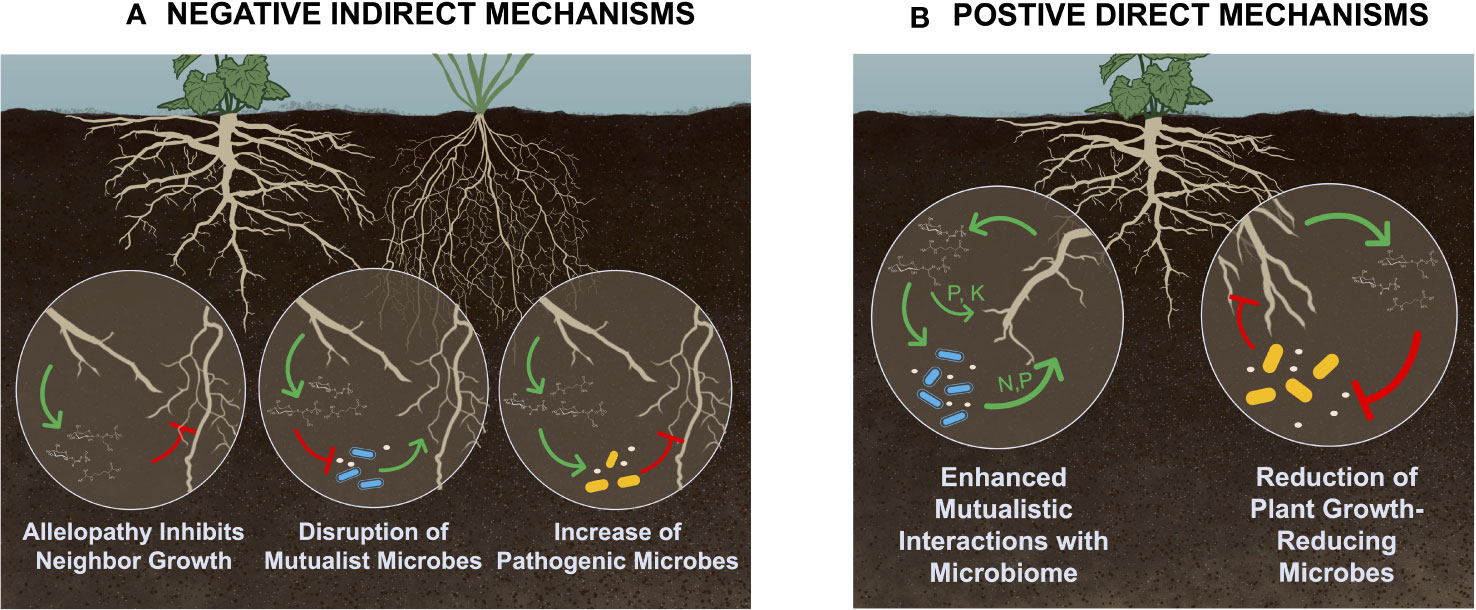
Figure 1 Conceptual model of (A) negative indirect and (B) positive direct plant-soil feedbacks that increase invasive potential, which have been shown to be driven by exudates or other rhizodeposits. Negative indirect plant-soil feedbacks occur when a plant negatively affects other heterospecific plants, and can occur through allelopathy, disruption of mutualistic associations, and increased pathogen abundance. Positive direct feedback loops occur when a plant positively affects other conspecific individuals through changes to the soils biotic or abiotic properties; potential mechanisms include enhanced mutualistic associations, as well as release of antimicrobial compounds into the rhizosphere. However, while enhanced mutualistic associations have repeatedly been demonstrated to increase invasive potential, release of antimicrobial compounds has not. Figure created by K. Blocklove. The green sharp arrows represent increasing levels, while red blunt arrows represent decreasing levels of either exudate production, nutrient availability, or microbial activity.
2.1 Positive direct plant-soil feedbacks
Direct plant-soil feedbacks occur when a plant’s legacy in the soil directly affects itself or other conspecific individuals. The absence of negative direct soil feedbacks (e.g. escape from soil pathogens) frequently characterizes invasive plants (Levine et al., 2006). However, positive direct plant-soil feedbacks, when the soil legacies of a species promote increased fitness and population growth, contribute to community dominance of a single individual (Klironomos, 2002; Van Der Putten et al., 2013). Multiple mechanisms exist by which exudates may drive positive direct soil feedbacks, and while some are microbially mediated (Klironomos, 2002), others are not (Van Der Putten et al., 2013). For example, invasive plants in their introduced range are repeatedly shown to engage in enhanced mutualistic associations that increase access to soil resources. Other positive direct mechanisms are not microbially mediated, such as when exudates directly increase nutrient availability in the soil. A final potential mechanism by which exudation may drive positive direct plant-soil feedbacks is through release of antimicrobial compounds that suppress soil pathogens. However, while research shows that some exudates do have antimicrobial properties (Lamichhane et al., 2023; Xiang et al., 2023), to our knowledge there have not been studies demonstrating antimicrobial exudates driving plant-soil feedbacks that increase invasive potential.
2.1.1 Enhanced mutualistic associations
Invasive plants in their introduced range are often found to support a greater abundance of mutualistic associations compared to their native range (Coats and Rumpho, 2014; Borda et al., 2021). Enhanced mutualist associations are especially valuable, and likely to increase invasive potential, in nutrient limited or stressful environments because they often lead to greater overall nutrient availability or greater capacity to access limited nutrients (Coats and Rumpho, 2014). Additionally, invasive plants are also more likely to increase plant productivity in response to greater nutrient availability compared to native plants (Knauf et al., 2021). Ultimately, these associations serve to increase the plant’s access to limited soil resources and productivity, thereby increasing their potential to suppress neighboring plants (Zhou and Staver, 2019).
Across a range of diverse ecosystems, plant invasions are associated with greater levels of soil nutrient availability (Castro-Diez et al., 2014; Mcleod et al., 2016; Sardans et al., 2017). Surprisingly, even when nodulating plants with endosymbiotic mutualisms that increase nutrient availability, such as legumes, were excluded from an analysis of global plant invasions, nutrients were still found to have increased availability across varying ecosystems (Castro-Diez et al., 2014). Nutrient enrichment, particularly increased nitrogen (N) and phosphorus (P), tends to benefit the invasive plant more than native competitors (Blumenthal et al., 2009; Lee et al., 2013), especially in ecosystems where native plants are adapted to nutrient limited environments (Blumenthal et al., 2009; Sardans et al., 2017). Examples of enhanced mutualisms that increase nutrient availability include: 1) priming microbes in the rhizosphere to mineralize soil organic matter, 2) supporting free-living nitrogen fixers in the rhizosphere, 3) increased access to P via mycorrhizal associations.
Priming organic matter mineralization. Labile root exudates, such as amino acids, sugars and organic acids, can stimulate microbial growth within the rhizosphere (Yan et al., 2023), potentially leading to priming of soil organic matter and nutrient release. When nutrients such as N and P are limiting in the soil solution, plants exude exudates with low N concentrations, mostly simple sugars, and for microbes to utilize these labile sources of C they need to mine soil organic matter for N (Kuzyakov, 2010). Rhizosphere microbes will increase extracellular enzyme production to stimulate decomposition of soil organic matter, thereby increasing nutrient availability (Kuzyakov, 2010; Yan et al., 2023). The ability of the plant to access these newly available nutrient pools depends on its relative ability to compete with rhizosphere microbes. A recent meta-analysis evaluating invasive plants across a range of different species, suggests that soils dominated by invasive plants were associated with higher levels of N and P- releasing enzymes compared to noninvaded sites (Zhou and Staver, 2019).
Free-living N fixers. Free-living nitrogen fixing bacteria include diazotrophs that utilize the nitrogenase enzyme to convert N2 gas to ammonia, but unlike endosymbiotic N fixers, they do not reside and fix N in root nodules. Previously, it was thought that N contribution from free-living N fixers was negligible due to inadequate sources of C because N fixation is energetically costly, and therefore C availability is a major control on the activity of free-living N fixers. However, root exudation provides a constitutive source of labile C, increasing the abundance and activity of free-living N fixers, and thereby N availability, within the rhizosphere. In fact, free-living N fixers can contribute equal or greater amounts of nitrogen across an entire ecosystem compared to endosymbiotic N fixation (Smercina et al., 2019). Recent evidence suggests that certain invasive plants, such as Bromus tectorum, have higher levels of free-living N fixers in the soil directly under the plant compared to native plants in the surrounding vegetation which may explain why B. tectorum invaded sites have higher levels of N compared to noninvaded sites (Reitstetter et al., 2022). Additionally, N-fixing bacteria endophytes were found to increase the productivity of the invasive plants Ageratina adenophora (Spreng.) King & H.Rob (Fang et al., 2019). and Sorghum halepense (L.) Pers (Rout and Chrzanowski, 2008).
Associations with free-living N fixers are ubiquitous among terrestrial plants, however the degree to which free-living N fixers are found in the rhizosphere varies (Smercina et al., 2019) and is likely governed by the quantity and composition of rhizodeposits. It is uncertain how biotic controls of free-living N fixation differ from N fixation within root nodules, however, some overlap has been identified. For example, flavonoids have long been accepted as important signals to initiate interactions with plant roots and rhizobium prior to nodule formation, and recent studies show they are also important signals for interactions with free-living N fixers (Coskun et al., 2017). Specifically, naringenin was shown to stimulate colonization of diazotrophic rhizobacteria in the rhizosphere of wheat roots (Coskun et al., 2017). Certain root exudates, such as arginine and methionine, can increase nitrogenase (the enzyme responsible for N fixation) activity in the soil, thereby increasing nitrogen availability (Medina-De La Rosa et al., 2023). Despite the apparent importance of rhizobacteria that provide nitrogen without nodulation, little is known about the specific influence of rhizodeposits and exudates on free-living N fixers.
Mycorrhizal Associations. Associations with mycorrhizae increase the volume of soil through which a plant can forage, thereby increasing access to soil nutrients and water and ultimately increasing the plant’s capacity to withstand abiotic stress and competition (Figueiredo et al., 2021). Many invasive plants associate with varying types of mycorrhizae, and research suggests that these associations can increase their invasive potential relative to native plants (Klironomos, 2002). For example, populations of Microstegium vimineum (Trin.) A. Camus in its invaded range had greater than twice the mycorrhizal colonization compared to its native range (Lee et al., 2013). Populations of Triadica sebifera (L.) Small from its invaded range had higher concentrations of the flavonoid quercetin in its root exudates, greater AM fungal colonization, and greater biomass compared to populations from its native range (Tian et al., 2021). As expected, greater mycorrhizal colonization was associated with increased P uptake and, and interestingly, also with increased tiller production, thereby increasing the potential of the plant to shade out neighbors. Even when both native and invasive plants are colonized, the invader may benefit more from the association. One study found when the invasive plant Solidago canadensis L. and native Kummerowia striata (Thunb.) Schindl. were interconnected with an arbuscular mycorrhizal network, the mycorrhizae preferentially provided both N and P to the invasive plant (Awaydul et al., 2019). Finally, in a study comparing multiple invasive and native plants, the invasives invested more in exudates known to stimulate mycorrhizal colonization (eg. quercetin and strigolactones), and root exudates from invaders increased AM colonization more than exudates from natives (Yu et al., 2022).
It is uncertain to what extent the greater nutrient availability associated with plant invasions is mediated by exudation; however, these changes are not always linked to litter quality and likely occur through mechanisms that increase plant nutrient access (Zhang et al., 2019). Increasingly, studies have shown that invasive and other highly competitive plants are characterized by greater C investment in mutualistic associations, primarily through exudates or other rhizodeposits (Kaštovská et al., 2015; Yu et al., 2022). For example, the competitive plant Glyceria maxima (Hartm.) Holmb., was characterized by a greater investment into root exudation which increased N turnover and availability to the plant (Kaštovská et al., 2015). A comparison of confamilial native and invasive plants, showed that invasive plants contained higher amounts of known chemotaxic phytochemicals in exudates (specifically, querecin and strigolactones) that enhance AMF colonization compared to native counterparts (Yu et al., 2022). Likewise, introduced populations of Triadica sebifera (L.) Small produced higher amounts of flavonoids, initiating more robust AMF colonization (Tian et al., 2021). Therefore, plant investment towards enhanced symbiotic associations are likely to increase the plant’s ability to adapt and maintain productivity within a wider range of ecosystems, including under limited soil nutrient availability.
2.1.2 Exudate direct control of nutrient availability
It is important to note that root exudates can increase nutrient availability independent from their effects on the soil microbial community through: 1) increasing acidity in the rhizosphere to increase weathering of inorganic minerals and disassociation of cationic nutrients; and 2) release of soil enzymes (Vives-Peris et al., 2020). Specifically, rhizodeposits that acidify the rhizosphere, including protons and organic acids, increase P dissociation from soil minerals, thereby making phosphate available in the soil solution for plant uptake (Chai and Schachtman, 2022). Roots also exude phosphatases and carboxylases, which mobilize P from organic compounds or via ligand exchange, respectively (Chai and Schachtman, 2022). Additionally, white lupin can change the pH in its rhizosphere to make it inhospitable to microbial growth and prevent degradation of exudates that increase P availability, thereby reducing competition with soil microbes for available P (Weisskopf et al., 2006). While most of the research characterizing exudate direct effects on nutrient availability have focused on P, there is some evidence to suggest that exudates can increase potassium availability; however, effects on potassium are less understood (Yang et al., 2019; Ma et al., 2022).
Plants with a greater availability to increase P and potassium (K) within their rhizosphere may have a competitive advantage. In contrast to N, these nutrients are immobile in soil. Plants that invest critical resources towards rhizodeposits that increase local P and K availability are more likely to directly benefit. In contrast, N is a mobile nutrient and more likely to move outside a roots zone of influence (York et al., 2016), which therefore, may result in a close competitor obtaining that limiting nutrient.
2.2 Negative indirect plant-soil feedback
Negative plant-soil feedbacks, mostly characterized by pathogen accumulation over time (Knevel et al., 2004; Flory and Clay, 2013), are thought to play important roles in structuring plant communities (Mommer et al., 2018). In fact, the absence of negative direct soil feedbacks, specifically pathogen release, is often characteristic of invasive plants and a common mechanism for invasions (Levine et al., 2006; Blumenthal et al., 2009). In their introduced range, invasive plants often do not encounter the pathogen loads characteristic of their native range (Klironomos, 2002; Coats and Rumpho, 2014). However, negative indirect effects, specifically when an invader induces plant-soil feedback that negatively impact neighboring plants, can also enhance invasive potential. Negative indirect effects include: 1) release of allelochemicals– the secretion of metabolic compounds into the soil that inhibit growth of competitors (novel weapons hypothesis; (Callaway and Ridenour, 2004); 2) accumulation of competitor pathogens– when an invasive plant increases the abundance of soil pathogens that more negatively affect native or competing species (Eppinga et al., 2006; Mangla and Callaway, 2007); and 3) disruption of mutualistic associations– in which invaders disrupt the beneficial microbes associated with a competitor, thereby decreasing its fitness and competitive ability (Stinson et al., 2006; Callaway et al., 2008; Grove et al., 2017; Roche et al., 2023).
2.2.1 Allelopathy
Exudates can directly affect neighboring plants, thereby influencing plant competition and the composition of plant communities (Nettan et al., 2019; Semchenko et al., 2019). Initial interest in plant-soil feedbacks was largely driven by the realization that living or decomposing plant tissues can release allelopathic compounds into the soil that inhibit plant germination or growth (Callaway and Ridenour, 2004). We now know a diverse array of phytocompounds can have potential allelopathic effects on neighboring plants (Inderjit et al., 2021), some of which are released through the roots as exudates. Many of these compounds have been identified from invasive species, partially explaining their suppression of native competitors. One recent study suggests that allelopathy is pervasive among invasive plant species, with over 51% of invasive plants containing or exuding allelopathic compounds capable of suppressing neighbors (Kalisz et al., 2020). Allelochemicals released by invasive plants can be especially deleterious to the native plant community when these compounds are novel within the invaded range (Portales-Reyes et al., 2015). Compared to species found in their introduced range, allelochemicals from an invasive plant often have less of a deleterious effect on plant species found in their native range, likely because these native plant species have coevolved with that invasive plant, and have therefore, evolved mechanisms to tolerate its associated allelochemicals. In contrast, the native plants in the invaded range do not have this coevolutionary history and therefore, traits conferring tolerance to allelochemicals were not under selection (Leger and Espeland, 2010; Nettan et al., 2019).
Phytotoxins or allelochemicals, can reduce the establishment, growth, or survival of neighboring plants by disrupting fundamental plant processes, ultimately impairing the ability of neighboring plants to compete for limited resources. Allelochemicals have been documented to disrupt photosynthesis, metabolite production, membrane transport, respiration, germination, growth, and initiate cell mortality (Kostina-Bednarz et al., 2023). Common allelochemicals include phenolics, terpenoids, and alkaloids (Kong et al., 2019). One well documented example, Alliaria petiolata (M.Bieb.) Cavara & Grande, exudes glucosinolates, which suppress neighboring plants and can also disrupt mutualistic associations (Cipollini and Cipollini, 2016; Cipollini and Greenawalt Bohrer, 2016). S. halepense roots exude sorgoleone, an inhibitor of photosystem II, respiration and hydroxyphenylpyruvate dioxygenase (HPPD) (Latif et al., 2022). Sorgoleone can be exuded by S. halepense plants shortly after emergence, and is relatively persistent in the soil (Latif et al., 2022). Several studies have demonstrated higher levels of allelochemical production from plants when competition is low or intermediate, but at higher levels of competition, resources are allocated towards plant growth and resource accumulation (Wasternack, 2017; Kong et al., 2019).
While many plant species have been shown to exude deleterious allelochemicals, they often are not found in the soil in high enough concentrations to have an effect (Scavo et al., 2019). Allelochemicals in the soil are often short lived because they are either decomposed by soil organisms or rendered inactive by binding to soil minerals (Scavo et al., 2019). However, among the invasive plants in which allelopathy was determined to be a dominant mechanism, allelochemicals were constitutively exuded by roots so that concentrations built up to the levels at which neighboring plants were inhibited. Finally, invasive plants from the introduced range often produce and exude allelopathic compounds at higher concentrations compared to individuals in the native range (Inderjit et al., 2021), suggesting that the most allelopathic individuals proliferated in the introduced range because of greater invasive potential and fitness.
2.2.2 Increasing competitor pathogens
Invasive plants can have varying effects on soil pathogens, with some studies showing different pathogen communities in invaded soil compared to the uninvaded native plant community (Nelson and Karp, 2013; Wang et al., 2021). A small subset of these studies found a greater abundance of soil pathogens in invaded soils, which greatly suppressed growth of the native plants, potentially increasing invasive potential (Eppinga et al., 2006; Mangla and Callaway, 2007; Day et al., 2015). For example, soil collected beneath an invasive plant, Chromolaena odorata (L.) R.M.King & H.Rob., contained over 25 times the abundance of Fusarium spores, and C. orodrata root leachates increased Fusurium spore density even when the plant was absent (Mangla and Callaway, 2007). Interestingly, growth of native plants was strongly inhibited when grown in the soil collected beneath C. odorata. This effect was eliminated when the soil was autoclaved, which suggests a soil pathogen was responsible. Additionally, theoretical models suggest that accumulation of soil pathogens negatively affecting native species explains both the spatial pattern and rate of spread of Ammophila arenaria (L.) Link, an invasive plant in California (Eppinga et al., 2006). However, other studies examining the effect of invasive plants on pathogen accumulation in the soil and its potential to negatively affect native plant species have found inconsistent effects (Dukes et al., 2018).
Thus far, there is limited evidence that pathogen accumulation, specifically whether an invasive plant can accumulate pathogens that negatively affect neighboring plants more than itself, is a major contributor towards invasive potential. However, it is well established that exudates play a major role in recruiting soil borne pathogens into the rhizosphere, as well as in stimulating germination of pathogen propagules (Pascale et al., 2019). For example, exudation of class III peroxidases recruited Fusurium to the tomato rhizosphere root via hyphae reorientation towards the root (Turra et al., 2015). Two tobacco cultivars that varied in their resistance to Rhizoctonia had exudate profiles that differed in concentrations of key organic acids, specifically oxalic acid, which was shown to induce a chemotactic response in R. solanacearum (Wu et al., 2015; Li et al., 2017a). Other organic acids, specifically fumaric, cinnamic, and myristic acids, were shown to recruit and initiate biofilm formation of R. solanacearum on tobacco roots, ultimately contributing to the development of tobacco bacterial wilt (Li et al., 2017a). In contrast, some exudates have antimicrobial properties, while others can attract biocontrol agents or support the growth of beneficial microbes in the rhizosphere that suppress soil borne pathogens (Lamichhane et al., 2023). Therefore, the effect of exudates on soil pathogens can vary across species, environmental conditions, and the soil biotic community.
2.2.3 Disrupting competitor mutualistic associations
Increasing evidence suggests that a plant’s rhizosphere microbial community depends on the identity of its neighboring plants (Laforgia et al., 2022; Ulbrich et al., 2022), and can ultimately influence the outcome of competition (Fahey and Flory, 2021). The rhizosphere of plants grown in competition will often reflect the rhizosphere of the dominant competitor (Hortal et al., 2017; Ulbrich et al., 2022), and the rhizosphere of more competitive species are less likely to change in response to interspecific competition (Cavalieri et al., 2020). Invasive plants can affect the rhizosphere microbiome of neighboring native plants (Laforgia et al., 2022), potentially disrupting their mutualistic associations (Stinson et al., 2006; Callaway et al., 2008). This is especially detrimental if the neighboring plant is highly dependent on the mutualist (Abbott et al., 2015). Thus far, evidence has shown that invasive plants can disrupt associations with mycorrhizal fungi (Grove et al., 2017) and rhizobia (Portales-Reyes et al., 2015). One well documented example, A. petiolata, is a prolific invader and model for exudate-microbe interactions. High concentrations of glucosinolates and isothiocynates in the soil within A. petiolata invaded sites were associated with reduced mycorrhizal colonization of roots of native trees (Stinson et al., 2006), and lower rhizobia nodulation of a native legume, Amphicarpaea bracteata (L.) Fernald (Portales-Reyes et al., 2015). Interestingly, A. petiolata extracts inhibited mycorrhizae in North American soils, where A. petiolata lacked an evolutionary history, more than mycorrhizae in European soils, which was also associated with a greater inhibition of North American native plants that were dependent on mycorrhizal association (Callaway et al., 2008).
3 Using plant exudate-microbe interactions to improve crop resilience
There are multiple avenues by which knowledge on exudate functions can be applied to increase crop and cropping system resilience. The first, and likely most obvious, would be selecting for crop genotypes with exudate profiles that drive plant-soil feedback loops (microbially mediated or not) which increase plant productivity and adaption to stress (see Table 1 for examples of known root exudates that stimulate plant-soil feedback loops). Additionally, inoculating soils or crops seeds with beneficial microbes potentially increases their abundance and increases the likelihood they will encounter roots of the crop. Finally, while the bulk of our review is on improving crop exudate profiles, the last section will end on using soil amendments to tie exudates from invasive weedy plants that may infest fields and negatively affect crop yields.
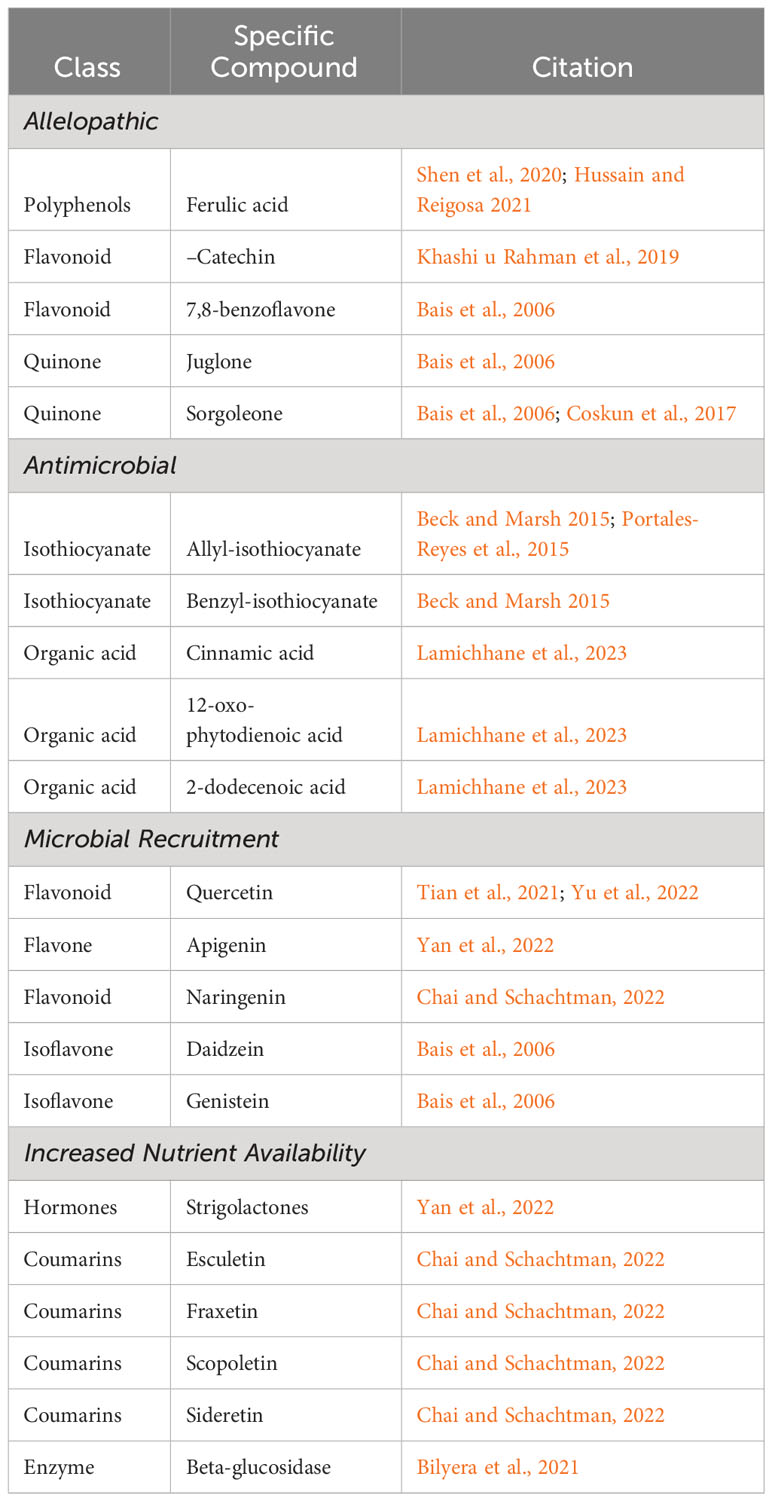
Table 1 Examples of rhizodeposits shown to initiate either direct or indirect plant-soil feedbacks (not meant to be an exhaustive list).
3.1 Breeding resilient crops via targeting exudate profiles
Both negative indirect and positive direct feedback loops can increase a plant’s ability to outcompete neighboring species and adapt to a wide range of environments, including resource deficient environments. However, which approach would be more effective at developing crop genotypes with long-term, robust stress resilience? We argue that focusing breeding efforts on positive direct feedback loops, and the rhizodeposit profiles that drive them, will lead to the development of crop genotypes with persistent stress resilience.
Negative indirect feedback loops can increase invasive potential and species dominance at early stages of invasion; however, these feedback loops are weakened over time (Lankaua et al., 2009; Lankau, 2012). When plant communities encounter a strong selection pressure such as allelopathic compounds or a high abundance of pathogens, populations will either evolve tolerance or be excluded (Leger and Espeland, 2010). Additionally, the soil microbial community will also evolve, increasing their capacity to outcompete plant pathogens and degrade allelopathic compounds (Li et al., 2017b). Therefore, it is likely that these strong negative selection pressures will lead to accelerated evolution of the weedy plants competing with crops in agricultural fields. Additionally, selecting for exudate profiles that accumulate soil pathogens to suppress weedy species is also likely to be ineffective because weed communities are diverse, and it is unlikely that a generalist soil pathogen will be capable of suppressing the diverse array of weed species in a field without also suppressing the crop, which is one limitation of bioherbicides (Hasan et al., 2021).
In contrast to negative feedback loops, positive feedback loops (such as increasing nutrient availability and suppressing pathogens) may be more likely to result in persistent, long-term increases in crop resilience. Developing crops that are more effective at acquiring their own resources, and ultimately more self-sufficient, has an enormous potential to decrease fertilizer inputs, thereby increasing farm profitability and potentially reducing nutrient pollution in waterways (Mariotte et al., 2018). Crop genotypes with increased ability to scavenge soil nutrients would be especially beneficial in agricultural regions where access to fertilizer is limited, and lack of sufficient soil fertility is a main contributor to crop yield gaps.
Ultimately, understanding the mechanisms by which invasive plants increase soil nutrient availability in the rhizosphere, and to what extent these processes are mediated through root exudates, may generate future breeding targets, and the development of crops that are either more capable of mining soil nutrients or supporting the soil microbes capable of providing otherwise-limiting nutrients. Currently, there is a tremendous amount of interest in breeding for crops that have the potential to support N fixers via mutualistic associations (Guo et al., 2023). These efforts include selecting for crop genotypes that are better at supporting free-living N-fixers in the rhizosphere (such as the high level of association with nitrogen-fixers in the mucilage of Sierra Mixe, a landrace which was recently identified in Mexico (Pankievicz et al., 2022), or by engineering non-legume crops to form nodules containing Rhizobium bacteria (Li and Chen, 2020 (Li and Chen, 2020). Compared to the exudate signals that recruit Rhizobium bacteria and initiate nodule formation, we understand much less about exudate signals that recruit free-living N fixers, as well as rhizodeposits necessary to support their growth and functions.
Can we select for improved rhizodeposit profiles within crop breeding programs to develop more resilient crop genotypes? Previous research has shown genotypic variation in exudate profiles among certain plant species (Monchgesang et al., 2016), as well as crop genotypes (Iannucci et al., 2017), suggesting the potential to select for exudate profiles that can increase crop competitiveness and stress resilience. For example, genotypic variation in exudate profiles have been linked to disease resistance in tobacco (Wu et al., 2015), variation in microbial activity in the rhizosphere of maize (Bilyera et al., 2021), phosphorus use efficiency in wheat (Nguyen et al., 2019), and zinc uptake in barley (Rasouli-Sadaghiani et al., 2011). Additionally, specific (but not all) metabolites were found to be heritable (Iannucci et al., 2017), suggesting there is potential to breed crop genotypes with improved exudate profiles.
However, it is widely accepted that selecting for greater performance of one functional trait (e.g. rhizodeposit investment in nutrient scavenging) comes with associated tradeoffs. Plants are limited in photosynthates they can invest towards growth, defense, rhizodeposits, and supporting mutualistic associations. Increasing C investment towards any one of these functions will result in a decrease in C investment to others (Wasternack, 2017). Recently, exudates were shown to constitute a third dimension in the root trait economic spectrum, through which tradeoffs in resource acquisition traits can be described as: 1) investment towards root growth to explore greater soil volume, 2) investment to mutualistic associations, and 3) investment towards exudates to modify the rhizosphere environment to increase nutrient availability (Iannucci et al., 2021; Wen et al., 2022). Therefore, tradeoffs to root exudates and other rhizodeposits are likely to come at a cost to other resource acquisition strategies. Future research is needed to understand the environmental and agronomic conditions for which one of these resource acquisition strategies outperform others.
3.2 Soil inoculants for stress resilient microbiomes
Selecting for crop genotypes with greater capacity to support beneficial microbes that increase crop resilience will only be effective if those microbes are present in the soil. Invasive plants often encounter different microbial taxa in their introduced range compared to their native range, potentially suggesting that microbes are limited via dispersal, and that ‘everything is not everywhere’ (Rout and Callaway, 2012), which could lead to plant-soil feedbacks that vary in magnitude and directionality. Therefore, adding specific beneficial microbial taxa through soil inoculants may increase the probability that a crop plant encounters the microbial mutualist required for positive plant-soil feedbacks.
The development of plant growth promoting soil inoculants is a burgeoning field, with growing interest among both farmers and researchers. Soil inoculants are often bacteria and fungi applied as seed treatments, granules, slurry, or foliar spray to increase the abundance of desired microbes in an ecosystem (O’callaghan et al., 2022). The most well-known example includes inoculating legume seeds with rhizobia for enhanced N-fixation (O’callaghan et al., 2022). Increasingly we are identifying additional beneficial microbes that may be applied as inoculants to increase their abundance and performance of ecosystem functions. For example, supplementing with populations of certain bacteria (e.g. Bacillus sp., Pseudomonas sp., and Burkholderia sp.) may increase availability of phosphorus in the soil (O’callaghan et al., 2022). Soil inoculants that included plant growth promoting bacteria increased fertilizer use efficiency and resulted in similar tomato yields at 50% to 75% reduced fertilizer rates compared to full rates (Chaparro et al., 2012). When applied as a seed inoculant, the beneficial microbes are more accessible to the crop plants and not to competing weeds in the field, thereby increasing crop competitiveness. Inoculants could also support plant health when the soil biotic community is altered by a weedy species, ultimately by fostering competition between the inoculant and deleterious microbes (Pereira et al., 2011).
However, currently there are many challenges with using inoculants. Foremost, the market is largely unregulated and there is a lack of field trials demonstrating product efficacy over a range of environmental conditions (O’callaghan et al., 2022). With widespread marketing of “beneficial” microbial inoculants, there is a risk of unintentionally spreading invasive or pathogenic microbes or shifting the native microbial community in ways that disrupt critical ecosystem functions (Liu et al., 2022; Moore et al., 2022). Inversely, introduced microbes may be outcompeted by native microbes, and effectiveness may vary across diverse environmental conditions (Mallon et al., 2015; Hoek et al., 2016).
3.3 Amendments to reduce impact of weedy exudates
Knowledge of the invasive plant-microbe interactions that drive feedback loops can also aid in developing management practices that decrease the deleterious effects weedy plants have on crop production. For example, soil amendments may be used to decrease the competitive ability of weeds by binding allelochemicals and other exudates and disrupting plant-soil feedbacks. One such example, biochar can reduce the deleterious effects of allelopathic weeds through either sorption or degradation of soil allelochemicals exuded by roots or by fostering diverse microbial communities including allelochemical-degrading soil microbes (Chen et al., 2022b). For example, nanobiochar treated soils reduced the effect of ferulic acid root exudates from Imperata cylindrica (L.) P.Beauv. on rice, with rice seedlings growing with biochar amendments exhibiting three times greater biomass compared to exudate treatments alone (Shen et al., 2020). Japanese knotweed (Polygonum cuspidatum Houtt.) produces polyphenol exudates that make complex nitrogenous soil compounds, thus reducing soil N availability; C amendments increased N mineralization and nitrate concentration was five times greater than control plots possibly due to reduced fungal biomass and faster nutrient cycling (Zhang et al., 2021). In addition to tying up allelochemical compounds, biochar-based amendments can increase soil microbial abundance and diversity (Yan et al., 2021) and is often proposed as a means by which to sequester soil C, improve water holding capacity, as well as filter soil contaminants (Lehmann et al., 2006).
However, there are many challenges with using C amendments, such as biochar to tie up allelochemicals and disrupt plant-soil feedbacks. For example, biochar is prohibitively expensive for use on large scales, so its use would only be effective to suppress small weed infestations (Kettenring and Adams, 2011). Biochar effects can be inconsistent or unreliable (Chen et al., 2022a), and it may end up suppressing the desired crop or other plants more than the weeds themselves. For example, biochar and other C amendments reduced plant available N (Ossanna and Gornish, 2022). And while reductions in soil N reduction can suppress nitrophilic, fast-growing invasives, the reductions in soil N would also decrease crop yields or inhibit restoration of native plants.
4 Conclusion
By elucidating the mechanisms by which root exudates affect microbial communities and abiotic conditions in the rhizosphere, thus generating plant-soil feedbacks which increase resource availability and competitive ability, we can select for crop genotypes that greatly increase the resilience of our crops and cropping systems. Invasive plants have repeatedly been shown to initiate plant-soil feedbacks that increase their ability to adapt to a wide range of soil conditions and suppress neighboring plants. Therefore, they could serve as effective model species to understand which exudate profiles to target when breeding for more resilient crops. While both positive direct and negative indirect feedback loops can increase a plant’s invasive potential, we suggest that focusing breeding efforts on positive direct plant-soil feedbacks, such as those that increase mutualistic associations and nutrient availability in the rhizosphere, are likely to lead to long term stress resilient crops. In contrast, the soil biotic and native plant community often evolve in response to negative indirect feedback loops, and therefore, potential benefits may be short lived. When weedy species are shown to initiate negative indirect feedback loops that suppress crop growth and yield, use of organic amendments, such as biochar, may disrupt these feedback loops.
Utilizing crop genotypes with improved exudate profiles could be one effective component of a diverse and integrated weed management system, creating competitive crops more resilient to abiotic stress. The extent to which these mechanisms translate to the agronomic conditions across agricultural landscapes remains uncertain. Future research is needed to determine whether upregulating specific exudate production can initiate similar plant-soil feedbacks in crop plants, because current research on exudate functions in the rhizosphere have focused on a few model species in limited environmental conditions.
Author contributions
CL: Conceptualization, Writing – original draft, Writing – review & editing. KB: Visualization, Conceptualization, Writing – original draft, Writing – review & editing. KE: Conceptualization, Writing – original draft, Writing – review & editing. NK: Conceptualization, Writing – original draft, Writing – review & editing. ER: Conceptualization, Writing – original draft, Writing – review & editing.
Funding
The author(s) declare financial support was received for the research, authorship, and/or publication of this article. Support for this work was provided by USDA Hatch Appropriations under Project No. 1025327-PEN04759. KB was funded by the College of Agricultural Sciences at the Pennsylvania State University. ER was funded by a NIFA Predoctoral Fellowship (Project # 7240). NK was funded by a USDA NIFA-AFRI grant (# 2021-09936). KE was partially supported by a Northeast SARE Graduate Student Grant (GNE22-298).
Conflict of interest
The authors declare that the research was conducted in the absence of any commercial or financial relationships that could be construed as a potential conflict of interest.
Publisher’s note
All claims expressed in this article are solely those of the authors and do not necessarily represent those of their affiliated organizations, or those of the publisher, the editors and the reviewers. Any product that may be evaluated in this article, or claim that may be made by its manufacturer, is not guaranteed or endorsed by the publisher.
References
Abbott K. C., Karst J., Biederman L. A., Borrett S. R., Hastings A., Walsh V., et al. (2015). Spatial heterogeneity in soil microbes alters outcomes of plant competition. PloS One 10, e0125788. doi: 10.1371/journal.pone.0125788
Awaydul A., Zhu W., Yuan Y., Xiao J., Hu H., Chen X., et al. (2019). Common mycorrhizal networks influence the distribution of mineral nutrients between an invasive plant, Solidago canadensis, and a native plant, Kummerowa striata. Mycorrhiza 29, 29–38. doi: 10.1007/s00572-018-0873-5
Bais H. P., Weir T. L., Perry L. G., Gilroy S., Vivanco J. M. (2006). The role of root exudates in rhizosphere interactions with plants and other organisms. Annu. Rev. Plant Biol. 57, 233–266. doi: 10.1146/annurev.arplant.57.032905.105159
Beck A. M., Marsh T. L. (2015). Inhibition of the general soil microbial community by allyl-isothiocyanate and benzyl-isothiocyanate. Bios 86, 31–37. doi: 10.1893/0005-3155-86.1.31
Bennett J. A., Klironomos J. (2019). Mechanisms of plant-soil feedback: interactions among biotic and abiotic drivers. New Phytol. 222, 91–96. doi: 10.1111/nph.15603
Berg G., Smalla K. (2009). Plant species and soil type cooperatively shape the structure and function of microbial communities in the rhizosphere. FEMS Microbiol. Ecol. 68, 1–13. doi: 10.1111/fem.2009.68.issue-1
Bilyera N., Zhang X., Duddek P., Fan L., Banfield C. C., Schlüter S., et al. (2021). Maize genotype-specific exudation strategies: An adaptive mechanism to increase microbial activity in the rhizosphere. Soil Biol. Biochem. 162. doi: 10.1016/j.soilbio.2021.108426
Blumenthal D., Mitchell C. E., Pysˇek P., Vojtečh J. (2009). Synergy between pathogen release and resource availability in plant invasion. Proc. Natl. Acad. Sci. 106, 7899–7904. doi: 10.1073/pnas.0812607106
Borda V., Longo S., Marro N., Urcelay C. (2021). The global invader Ligustrum lucidum accumulates beneficial arbuscular mycorrhizal fungi in a novel range. Plant Ecol. 222, 397–408. doi: 10.1007/s11258-021-01114-8
Bouffaud M. L., Poirier M. A., Muller D., Moenne-Loccoz Y. (2014). Root microbiome relates to plant host evolution in maize and other Poaceae. Environ. Microbiol. 16, 2804–2814. doi: 10.1111/1462-2920.12442
Callaway R. M., Cipollini D., Barto K., Thelen G. C., Hallett S. G., Prati D., et al. (2008). Novel weapons: invasive plant suppresses fungal mutualists in America but not in its native Europe. Ecology 89, 1043–1055. doi: 10.1890/07-0370.1
Callaway R. M., Ridenour W. M. (2004). Novel weapons: invasive success and the evolution of increased competitive ability. Front. Ecol. Environ. 2, 436–443. doi: 10.1890/1540-9295(2004)002[0436:NWISAT]2.0.CO;2
Canarini A., Kaiser C., Merchant A., Richter A., Wanek W. (2019). Root exudation of primary metabolites: mechanisms and their roles in plant responses to environmental stimuli. Front. Plant Sci. 10, 157. doi: 10.3389/fpls.2019.00157
Castro-Diez P., Godoy O., Alonso A., Gallardo A., Saldana A. (2014). What explains variation in the impacts of exotic plant invasions on the nitrogen cycle? A meta-analysis. Ecol. Lett. 17, 1–12. doi: 10.3390/v14122709
Cavalieri A., Bak F., Garcia-Lemos A. M., Weiner J., Nicolaisen M. H., Nybroe O. (2020). Effects of intra- and interspecific plant density on rhizosphere bacterial communities. Front. Microbiol. 11, 1045. doi: 10.3389/fmicb.2020.01045
Chai Y. N., Schachtman D. P. (2022). Root exudates impact plant performance under abiotic stress. Trends Plant Sci. 27, 80–91. doi: 10.1016/j.tplants.2021.08.003
Chaparro J. M., Sheflin A. M., Manter D. K., Vivanco J. M. (2012). Manipulating the soil microbiome to increase soil health and plant fertility. Biol. Fertil. Soils. 48, 489–499. doi: 10.1007/s00374-012-0691-4
Chen Q., Lan P., Wu M., Lu M., Pan B., Xing B. (2022a). Biochar mitigates allelopathy through regulating allelochemical generation from plants and accumulation in soil. Carbon. Res. 1. doi: 10.1007/s44246-022-00003-7
Chen X., Li Q., Wang Y., Chen F., Zhang X., Zhang F. (2022b). Bacillus promotes invasiveness of exotic Flaveria bidentis by increasing its nitrogen and phosphorus uptake. J. Plant Ecol. 15, 596–609. doi: 10.1093/jpe/rtab046
Cipollini D., Cipollini K. (2016). A review of garlic mustard (Alliaria petiolata, Brassicaceae) as an allelopathic plant. J. Torrey. Bot. Soc. 143, 339–348. doi: 10.3159/TORREY-D-15-00059
Cipollini K., Greenawalt Bohrer M. (2016). Comparison of allelopathic effects of five invasive species on two native species. J. Torrey. Bot. Soc. 143, 427–436. doi: 10.3159/TORREY-D-15-00062.1
Coats V. C., Rumpho M. E. (2014). The rhizosphere microbiota of plant invaders: an overview of recent advances in the microbiomics of invasive plants. Front. Microbiol. 5. doi: 10.3389/fmicb.2014.00368
Colautti R. I., Ricciardi A., Grigorovich I. A., Macisaac H. J. (2004). Is invasion success explained by the enemy release hypothesis? Ecol. Lett. 7, 721–733. doi: 10.1111/j.1461-0248.2004.00616.x
Coskun D., Britto D. T., Shi W., Kronzucker H. J. (2017). How plant root exudates shape the nitrogen cycle. Trends Plant Sci. 22, 661–673. doi: 10.1016/j.tplants.2017.05.004
Dastogeer K. M. G., Tumpa F. H., Sultana A., Akter M. A., Chakraborty A. (2020). Plant microbiome–an account of the factors that shape community composition and diversity. Curr. Plant Biol. 23, 100161. doi: 10.1016/j.cpb.2020.100161
Day N. J., Dunfield K. E., Antunes P. M. (2015). Fungi from a non-native invasive plant increase its growth but have different growth effects on native plants. Biol. Invasions. 18, 231–243. doi: 10.1007/s10530-015-1004-2
Delory B. M., Callaway R. M., Semchenko M. (2023). A trait-based framework linking the soil metabolome to plant-soil feedbacks. New Phytol. doi: 10.1111/nph.19490
Dennis P. G., Miller A. J., Hirsch P. R. (2010). Are root exudates more important than other sources of rhizodeposits in structuring rhizosphere bacterial communities?: Root exudates and rhizosphere bacteria. FEMS Microbiol. Ecol. 72, 313–327. doi: 10.1111/fem.2010.72.issue-3
Driouich A., Gaudry A., Pawlak B., Moore J. P. (2021). Root cap-derived cells and mucilage: a protective network at the root tip. Protoplasma 258, 1179–1185. doi: 10.1007/s00709-021-01660-y
Dukes A. E., Koyama A., Dunfield K. E., Antunes P. M. (2018). Enemy of my enemy: evidence for variable soil biota feedbacks of Vincetoxicum rossicum on native plants. Biol. Invasions. 21, 67–83. doi: 10.1007/s10530-018-1804-2
Eppinga M. B., Rietkerk M., Dekker S. C., De Ruiter P. C., Van Der Putten W. H., Van Der Putten W. H. (2006). Accumulation of local pathogens: a new hypothesis to explain exotic plant invasions. Oikos 114, 168–176. doi: 10.1111/j.2006.0030-1299.14625.x
Fahey C., Flory S. L. (2021). Soil microbes alter competition between native and invasive plants. J. Ecol. 110, 404–414. doi: 10.1111/1365-2745.13807
Fang K., Bao Z. S., Chen L., Zhou J., Yang Z. P., Dong X. F., et al. (2019). Growth-promoting characteristics of potential nitrogen-fixing bacteria in the root of an invasive plant Ageratina adenophora. PeerJ 7, e7099. doi: 10.7717/peerj.7099
Figueiredo A. F., Boy J., Guggenberger G. (2021). Common mycorrhizae network: A review of the theories and mechanisms behind underground interactions. Front. Fungal Biol. 2, 735299. doi: 10.3389/ffunb.2021.735299
Fitzpatrick C. R., Salas-González I., Conway J. M., Finkel O. M., Gilbert S., Russ D., et al. (2020). The plant microbiome: from ecology to reductionism and beyond. Annu. Rev. Microbiol. 74, 81–100. doi: 10.1146/annurev-micro-022620-014327
Flory S. L., Clay K. (2013). Pathogen accumulation and long-term dynamics of plant invasions. J. Ecol. 101, 607–613. doi: 10.1111/1365-2745.12078
Gibbons S. M., Lekberg Y., Mummey D. L., Sangwan N., Ramsey P. W., Gilbert J. A. (2017). Invasive plants rapidly reshape soil properties in a grassland ecosystem. mSystems 2. doi: 10.1128/mSystems.00178-16
Gioria M., Osborne B. A. (2014). Resource competition in plant invasions: emerging patterns and research needs. Front. Plant Sci. 5. doi: 10.3389/fpls.2014.00501
Grove S., Haubensak K. A., Gehring C., Parker I. M., D'antonio C. (2017). Mycorrhizae, invasions, and the temporal dynamics of mutualism disruption. J. Ecol. 105, 1496–1508. doi: 10.1111/1365-2745.12853
Guo K., Yang J., Yu N., Luo L., Wang E. (2023). Biological nitrogen fixation in cereal crops: Progress, strategies, and perspectives. Plant Commun. 4, 100499. doi: 10.1016/j.xplc.2022.100499
Hasan M., Ahmad-Hamdani M. S., Rosli A. M., Hamdan H. (2021). Bioherbicides: an eco-friendly tool for sustainable weed management. Plants (Basel). 10. doi: 10.3390/plants10061212
Hawes M. C., Gunawardena U., Miyasaka S., Zhao X. (2000). The role of root border cells in plant defense. Trends Plant Sci. 5, 128–133. doi: 10.1016/S1360-1385(00)01556-9
Hoek T. A., Axelrod K., Biancalani T., Yurtsev E. A., Liu J., Gore J. (2016). Resource availability modulates the cooperative and competitive nature of a microbial cross-feeding mutualism. PloS Biol. 14, e1002540. doi: 10.1371/journal.pbio.1002540
Hortal S., Lozano Y. M., Bastida F., Armas C., Moreno J. L., Garcia C., et al. (2017). Plant-plant competition outcomes are modulated by plant effects on the soil bacterial community. Sci. Rep. 7, 17756. doi: 10.1038/s41598-017-18103-5
Hussain M. I., Reigosa M. J. (2021). Secondary metabolites, ferulic acid and p-hydroxybenzoic acid induced toxic effects on photosynthetic process in Rumex acetosa L. Biomolecules 11 (2), 233.
Iannucci A., Canfora L., Nigro F., De Vita P., Beleggia R. (2021). Relationships between root morphology, root exudate compounds and rhizosphere microbial community in durum wheat. Appl. Soil Ecol. 158. doi: 10.1016/j.apsoil.2020.103781
Iannucci A., Fragasso M., Beleggia R., Nigro F., Papa R. (2017). Evolution of the crop rhizosphere: impact of domestication on root exudates in tetraploid wheat (Triticum turgidum L.). Front. Plant Sci. 8, 2124. doi: 10.3389/fpls.2017.02124
Inderjit, Cahill J. F. (2015). Linkages of plant-soil feedbacks and underlying invasion mechanisms. AoB. Plants 7, plv022–plv022. doi: 10.1093/aobpla/plv022
Inderjit, Simberloff D., Kaur H., Kalisz S., Bezemer T. M. (2021). Novel chemicals engender myriad invasion mechanisms. New Phytol. 232, 1184–1200. doi: 10.1111/nph.17685
Kalisz S., Kivlin S. N., Bialic-Murphy L. (2020). Allelopathy is pervasive in invasive plants. Biol. Invasions. 23, 367–371. doi: 10.1007/s10530-020-02383-6
Kaštovská E., Edwards K., Picek T., Šantrůčková H. (2015). A larger investment into exudation by competitive versus conservative plants is connected to more coupled plant–microbe N cycling. Biogeochemistry 122, 47–59. doi: 10.1007/s10533-014-0028-5
Kettenring K. M., Adams C. R. (2011). Lessons learned from invasive plant control experiments: a systematic review and meta-analysis. J. Appl. Ecol. 48, 970–979. doi: 10.1111/jpe.2011.48.issue-4
Khashi u Rahman M., Zhou X., Wu F. (2019). The role of root exudates, CMNs, and VOCs in plant–plant interaction. J. Plant Interact. 14, 630–636. doi: 10.1080/17429145.2019.1689581
Klironomos J. N. (2002). Feedback with soil biota contributes to plant rarity and invasiveness in communities. Nature 417, 67–70. doi: 10.1038/417067a
Knauf A. E., Litton C. M., Cole R. J., Sparks J. P., Giardina C. P., Gerow K. G., et al. (2021). Nutrient-use strategy and not competition determines native and invasive species response to changes in soil nutrient availability. Restor. Ecol. 29. doi: 10.1111/rec.13374
Knevel I. C., Lans T., Menting F. B. J., Hertling U. M., Van Der Putten W. H. (2004). Release from native root herbivores and biotic resistance by soil pathogens in a new habitat both affect the alien Ammophila arenaria in South Africa. Oecologia 141, 502–510. doi: 10.1007/s00442-004-1662-8
Kong C. H., Xuan T. D., Khanh T. D., Tran H. D., Trung N. T. (2019). Allelochemicals and signaling chemicals in plants. Molecules 24. doi: 10.3390/molecules24152737
Kostina-Bednarz M., Płonka J., Barchanska H. (2023). Allelopathy as a source of bioherbicides: challenges and prospects for sustainable agriculture. Rev. Environ. Sci. Bio/Technol. 22, 471–504. doi: 10.1007/s11157-023-09656-1
Kulmatiski A., Beard K. H., Stevens J. R., Cobbold S. M. (2008). Plant-soil feedbacks: a meta-analytical review. Ecol. Lett. 11, 980–992. doi: 10.1111/j.1461-0248.2008.01209.x
Kuzyakov Y. (2010). Priming effects: Interactions between living and dead organic matter. Soil Biol. Biochem. 42, 1363–1371. doi: 10.1016/j.soilbio.2010.04.003
Laforgia M. L., Kang H., Ettinger C. L. (2022). Invasive grass dominance over native forbs is linked to shifts in the bacterial rhizosphere microbiome. Microbial. Ecol. 84, 496–508. doi: 10.1007/s00248-021-01853-1
Lamichhane J. R., Barbetti M. J., Chilvers M. I., Pandey A. K., Steinberg C. (2023). Exploiting root exudates to manage soil-borne disease complexes in a changing climate. Trends Microbiol 32 (1). doi: 10.1016/j.tim.2023.07.011
Lankau R. A. (2012). Coevolution between invasive and native plants driven by chemical competition and soil biota. Proc. Natl. Acad. Sci. U.S.A. 109, 11240–11245. doi: 10.1073/pnas.1201343109
Lankaua R. A., Nuzzo V., Spyreas G., Davis A. S. (2009). Evolutionary limits ameliorate the negative impact of an invasive plant. Proc. Natl. Acad. Sci. 106, 15362–15367. doi: 10.1073/pnas.0905446106
Latif S., Gurusinghe S., Weston L. A. (2022). “The potential role of allelopathy in the persistence of invasive weeds,” in Persistence Strategies of Weeds., 1 ed. Eds. Upadhyaya M. K., Clements D. R., Shrestha A. (West Sussex, UK: Wiley).
Lee M. R., Tu C., Chen X., Hu S. (2013). Arbuscular mycorrhizal fungi enhance P uptake and alter plant morphology in the invasive plant Microstegium vimineum. Biol. Invasions. 16, 1083–1093. doi: 10.1007/s10530-013-0562-4
Leger E. A., Espeland E. K. (2010). PERSPECTIVE: Coevolution between native and invasive plant competitors: implications for invasive species management: Managing coevolution. Evol. Appl. 3, 169–178. doi: 10.1111/j.1752-4571.2009.00105.x
Lehmann J., Gaunt J., Rondon M. (2006). Bio-char sequestration in terrestrial ecosystems – A review. Mitigation. Adaptation. Strategies. Global Change 11, 403–427. doi: 10.1007/s11027-005-9006-5
Levine J. M., Pachepsky E., Kendall B. E., Yelenik S. G., Lambers J. H. R. (2006). Plant-soil feedbacks and invasive spread. Ecol. Lett. 9, 1005–1014. doi: 10.1111/j.1461-0248.2006.00949.x
Levine J. M., Vila M., D'antonio C. M., Dukes J. S., Grigulis K., Lavorel S. (2003). Mechanisms underlying the impacts of exotic plant invasions. Proc. Biol. Sci. 270, 775–781. doi: 10.1098/rspb.2003.2327
Li C., Bo H., Song B., Chen X., Cao Q., Yang R., et al. (2022). Reshaping of the soil microbiome by the expansion of invasive plants: shifts in structure, diversity, co-occurrence, niche breadth, and assembly processes. Plant Soil 477, 629–646. doi: 10.1007/s11104-022-05445-x
Li Q., Chen S. (2020). Transfer of nitrogen fixation (nif) genes to non-diazotrophic hosts. Chembiochem 21, 1717–1722. doi: 10.1002/cbic.201900784
Li S., Xu C., Wang J., Guo B., Yang L., Chen J., et al. (2017a). Cinnamic, myristic and fumaric acids in tobacco root exudates induce the infection of plants by Ralstonia solanacearum. Plant Soil 412, 381–395. doi: 10.1007/s11104-016-3060-5
Li Y. P., Feng Y. L., Kang Z. L., Zheng Y. L., Zhang J. L., Chen Y. J., et al. (2017b). Changes in soil microbial communities due to biological invasions can reduce allelopathic effects. J. Appl. Ecol. 54, 1281–1290. doi: 10.1111/1365-2664.12878
Liu F., Hewezi T., Lebeis S. L., Pantalone V., Grewal P. S., Staton M. E. (2019). Soil indigenous microbiome and plant genotypes cooperatively modify soybean rhizosphere microbiome assembly. BMC Microbiol. 19, 201. doi: 10.1186/s12866-019-1572-x
Liu X., Le Roux X., Salles J. F. (2022). The legacy of microbial inoculants in agroecosystems and potential for tackling climate change challenges. iScience 25, 103821. doi: 10.1016/j.isci.2022.103821
Ma W., Tang S., Dengzeng Z., Zhang D., Zhang T., Ma X. (2022). Root exudates contribute to belowground ecosystem hotspots: A review. Front. Microbiol. 13, 937940. doi: 10.3389/fmicb.2022.937940
Mallon C. A., Elsas J. D. V., Salles J. F. (2015). Microbial invasions: the process, patterns, and mechanisms. Trends Microbiol. 23, 719–729. doi: 10.1016/j.tim.2015.07.013
Mangla S., Callaway R. M. (2007). Exotic invasive plant accumulates native soil pathogens which inhibit native plants. J. Ecol. 0, 071031082432001–???. doi: 10.1111/j.1365-2745.2007.01312.x
Mariotte P., Mehrabi Z., Bezemer T. M., De Deyn G. B., Kulmatiski A., Drigo B., et al. (2018). Plant–soil feedback: bridging natural and agricultural sciences. Trends Ecol. Evol. 33, 129–142. doi: 10.1016/j.tree.2017.11.005
Mcleod M. L., Cleveland C. C., Lekberg Y., Maron J. L., Philippot L., Bru D., et al. (2016). Exotic invasive plants increase productivity, abundance of ammonia-oxidizing bacteria and nitrogen availability in intermountain grasslands. J. Ecol. 104, 994–1002. doi: 10.1111/1365-2745.12584
Medina-De La Rosa G., García-Oliva F., López-Lozano N. E. (2023). Arginine and methionine increase the enzymatic activity of microbes involved in N and P cycles in arid soil from the Chihuahuan desert. Eur. J. Soil Biol. 117. doi: 10.1016/j.ejsobi.2023.103517
Mommer L., Cotton T. E. A., Raaijmakers J. M., Termorshuizen A. J., Van Ruijven J., Hendriks M., et al. (2018). Lost in diversity: the interactions between soil-borne fungi, biodiversity and plant productivity. New Phytol. 218, 542–553. doi: 10.1111/nph.15036
Monchgesang S., Strehmel N., Schmidt S., Westphal L., Taruttis F., Muller E., et al. (2016). Natural variation of root exudates in Arabidopsis thaliana-linking metabolomic and genomic data. Sci. Rep. 6, 29033. doi: 10.1038/srep29033
Moore J. A. M., Abraham P. E., Michener J. K., Muchero W., Cregger Melissa A. (2022). Ecosystem consequences of introducing plant growth promoting rhizobacteria to managed systems and potential legacy effects. New Phytol. 234, 1914–1918. doi: 10.1111/nph.18010
Nazari M. (2021). Plant mucilage components and their functions in the rhizosphere. Rhizosphere 18. doi: 10.1016/j.rhisph.2021.100344
Nazari M., Bickel S., Benard P., Mason-Jones K., Carminati A., Dippold M. A. (2022). Biogels in soils: plant mucilage as a biofilm matrix that shapes the rhizosphere microbial habitat. Front. Plant Sci. 12, 798992. doi: 10.3389/fpls.2021.798992
Nelson E. B., Karp M. A. (2013). Soil pathogen communities associated with native and non-native Phragmites australis populations in freshwater wetlands. Ecol. Evol. 3, 5254–5267. doi: 10.1002/ece3.900
Nettan S., Thetloff M., Lepik A., Semchenko M., Zobel K., Tanentzap A. (2019). Manipulation of vegetation with activated carbon reveals the role of root exudates in shaping native grassland communities. J. Vegetation. Sci. 30, 1056–1067. doi: 10.1111/jvs.12808
Nguyen V. L., Palmer L., Roessner U., Stangoulis J. (2019). Genotypic variation in the root and shoot metabolite profiles of wheat (Triticum aestivum L.) indicate sustained, preferential carbon allocation as a potential mechanism in phosphorus efficiency. Front. Plant Sci. 10, 995. doi: 10.3389/fpls.2019.00995
O’callaghan M., Ballard R. A., Wright D. (2022). Soil microbial inoculants for sustainable agriculture: Limitations and opportunities. Soil Use Manage. 38, 1340–1369. doi: 10.1111/sum.12811
Ossanna L. Q. R., Gornish E. S. (2022). Efficacy of labile carbon addition to reduce fast-growing, invasive non-native plants: A review and meta-analysis. J. Appl. Ecol. 60, 218–228. doi: 10.1111/1365-2664.14324
Pankievicz V. C. S., Delaux P. M., Infante V., Hirsch H. H., Rajasekar S., Zamora P., et al. (2022). Nitrogen fixation and mucilage production on maize aerial roots is controlled by aerial root development and border cell functions. Front. Plant Sci. 13, 977056. doi: 10.3389/fpls.2022.977056
Pascale A., Proietti S., Pantelides I. S., Stringlis I. A. (2019). Modulation of the root microbiome by plant molecules: the basis for targeted disease suppression and plant growth promotion. Front. Plant Sci. 10, 1741. doi: 10.3389/fpls.2019.01741
Pereira P., Ibáñez S. G., Agostini E., Etcheverry M. (2011). Effects of maize inoculation with Fusarium verticillioides and with two bacterial biocontrol agents on seedlings growth and antioxidative enzymatic activities. Appl. Soil Ecol. 51, 52–59. doi: 10.1016/j.apsoil.2011.08.007
Pickett B., Irvine I. C., Bullock E., Arogyaswamy K., Aronson E. (2019). Legacy effects of invasive grass impact soil microbes and native shrub growth. Invasive. Plant Sci. Manage. 12, 22–35. doi: 10.1017/inp.2018.32
Poorter H., Niklas K. J., Reich P. B., Oleksyn J., Poot P., Mommer L. (2012). Biomass allocation to leaves, stems and roots: meta-analyses of interspecific variation and environmental control. New Phytol. 193, 30–50. doi: 10.1111/j.1469-8137.2011.03952.x
Portales-Reyes C., Van Doornik T., Schultheis E. H., Suwa T. (2015). A novel impact of a novel weapon: allelochemicals in Alliaria petiolata disrupt the legume-rhizobia mutualism. Biol. Invasions. 17, 2779–2791. doi: 10.1007/s10530-015-0913-4
Rasouli-Sadaghiani M., Sadeghzadeh B., Sepehr E., Rengel Z. (2011). Root exudation and zinc uptake by barley genotpyes differing in Zn efficiency. J. Plant Nutr. 34, 1120–1132. doi: 10.1080/01904167.2011.558156
Rathore N., Hanzelkova V., Dostalek T., Semerad J., Schnablova R., Cajthaml T., et al. (2023). Species phylogeny, ecology, and root traits as predictors of root exudate composition. New Phytol. 239, 1212–1224. doi: 10.1111/nph.19060
Reitstetter R., Yang B., Tews A. D., Barberán A. (2022). Soil microbial communities and nitrogen associated with cheatgrass invasion in a sagebrush shrubland. Plant Soil 479, 325–336. doi: 10.1007/s11104-022-05523-0
Roche M. D., Pearse I. S., Sofaer H. R., Kivlin S. N., Spyreas G., Zaya D. N., et al. (2023). Invasion-mediated mutualism disruption is evident across heterogeneous environmental conditions and varying invasion intensities. Ecography 2023. doi: 10.1111/ecog.06434
Rout M. E., Callaway R. M. (2012). Interactions between exotic invasive plants and soil microbes in the rhizosphere suggest that 'everything is not everywhere'. Ann. Bot. 110, 213–222. doi: 10.1093/aob/mcs061
Rout M. E., Chrzanowski T. H. (2008). The invasive Sorghum halepense harbors endophytic N2-fixing bacteria and alters soil biogeochemistry. Plant Soil 315, 163–172. doi: 10.1007/s11104-008-9740-z
Sardans J., Bartrons M., Margalef O., Gargallo-Garriga A., Janssens I. A., Ciais P., et al. (2017). Plant invasion is associated with higher plant-soil nutrient concentrations in nutrient-poor environments. Glob. Chang. Biol. 23, 1282–1291. doi: 10.1111/gcb.13384
Scavo A., Abbate C., Mauromicale G. (2019). Plant allelochemicals: agronomic, nutritional and ecological relevance in the soil system. Plant Soil 442, 23–48. doi: 10.1007/s11104-019-04190-y
Semchenko M., Nettan S., Sepp A., Zhang Q., Abakumova M., Davison J., et al. (2019). Soil biota and chemical interactions promote co-existence in co-evolved grassland communities. J. Ecol. 107, 2611–2622. doi: 10.1111/1365-2745.13220
Shen Y., Tang H., Wu W., Shang H., Zhang D., Zhan X., et al. (2020). Role of nano-biochar in attenuating the allelopathic effect from Imperata cylindrica on rice seedlings. Environ. Sci.: Nano. 7, 116–126. doi: 10.1039/C9EN00828D
Smercina D. N., Evans S. E., Friesen M. L., Tiemann L. K. (2019). To fix or not to fix: controls on free-living nitrogen fixation in the rhizosphere. Appl. Environ. Microbiol. 85. doi: 10.1128/AEM.02546-18
Stinson K. A., Campbell S. A., Powell J. R., Wolfe B. E., Callaway R. M., Thelen G. C., et al. (2006). Invasive plant suppresses the growth of native tree seedlings by disrupting belowground mutualisms. PloS Biol. 4, e140. doi: 10.1371/journal.pbio.0040140
Strehmel N., Bottcher C., Schmidt S., Scheel D. (2014). Profiling of secondary metabolites in root exudates of Arabidopsis thaliana. Phytochemistry 108, 35–46. doi: 10.1016/j.phytochem.2014.10.003
Tian B., Pei Y., Huang W., Ding J., Siemann E. (2021). Increasing flavonoid concentrations in root exudates enhance associations between arbuscular mycorrhizal fungi and an invasive plant. ISME. J. 15, 1919–1930. doi: 10.1038/s41396-021-00894-1
Trivedi P., Mattupalli C., Eversole K., Leach J. E. (2021). Enabling sustainable agriculture through understanding and enhancement of microbiomes. New Phytol. 230, 2129–2147. doi: 10.1111/nph.17319
Turra D., El Ghalid M., Rossi F., Di Pietro A. (2015). Fungal pathogen uses sex pheromone receptor for chemotropic sensing of host plant signals. Nature 527, 521–524. doi: 10.1038/nature15516
Ulbrich T. C., Rivas-Ubach A., Tiemann L. K., Friesen M. L., Evans S. E. (2022). Plant root exudates and rhizosphere bacterial communities shift with neighbor context. Soil Biol. Biochem. 172, 108753. doi: 10.1016/j.soilbio.2022.108753
Van Der Putten W. H., Bardgett R. D., Bever J. D., Bezemer T. M., Casper B. B., Fukami T., et al. (2013). Plant-soil feedbacks: the past, the present and future challenges. J. Ecol. 101, 265–276. doi: 10.1111/1365-2745.12054
Van Kleunen M., Weber E., Fischer M. (2010). A meta-analysis of trait differences between invasive and non-invasive plant species. Ecol. Lett. 13, 235–245. doi: 10.1111/j.1461-0248.2009.01418.x
Vives-Peris V., De Ollas C., Gómez-Cadenas A., Pérez-Clemente R. M. (2020). Root exudates: from plant to rhizosphere and beyond. Plant Cell Rep. 39, 3–17. doi: 10.1007/s00299-019-02447-5
Wang M., Tang X., Sun X., Jia B., Xu H., Jiang S., et al. (2021). An invasive plant rapidly increased the similarity of soil fungal pathogen communities. Ann. Bot. 127, 327–336. doi: 10.1093/aob/mcaa191
Wasternack C. (2017). A plant’s balance of growth and defense – revisited. New Phytol. 215, 1291–1294. doi: 10.1111/nph.14720
Weisskopf L., Abou-Mansour E., Fromin N., Tomasi N., Santelia D., Edelkott I., et al. (2006). White lupin has developed a complex strategy to limit microbial degradation of secreted citrate required for phosphate acquisition. Plant Cell Environ. 29, 919–927. doi: 10.1111/j.1365-3040.2005.01473.x
Wen Z., White P. J., Shen J., Lambers H. (2022). Linking root exudation to belowground economic traits for resource acquisition. New Phytol. 233, 1620–1635. doi: 10.1111/nph.17854
Wu K., Yuan S., Xun G., Shi W., Pan B., Guan H., et al. (2015). Root exudates from two tobacco cultivars affect colonization of Ralstonia solanacearum and the disease index. Eur. J. Plant Pathol. 141, 667–677. doi: 10.1007/s10658-014-0569-4
Xiang Y., Javed Q., Wu Y., Bo Y., Dai Z., Huang P., et al. (2023). Root exudates of Wedelia trilobata suppress soil-borne pathogenic gungi and increase its invasion. Polish. J. Environ. Stud. 32, 4865–4875. doi: 10.15244/pjoes/168421
Xu K., Liu X., Zhao C., Pan Q., Chen X., Jiang N., et al. (2023). Nitrogen deposition further increases Ambrosia trifida root exudate invasiveness under global warming. Environ. Monit. Assess. 195 (6). doi: 10.1007/s10661-023-11380-w
Yan T., Xue J., Zhou Z., Wu Y. (2021). Biochar-based fertilizer amendments improve the soil microbial community structure in a karst mountainous area. Sci. Total. Environ. 794, 148757. doi: 10.1016/j.scitotenv.2021.148757
Yan D., Tajima H., Cline L. C., Fong R. Y., Ottaviani J. I., Shapiro H.-Y. (2022). Genetic modification of flavone biosynthesis in rice enhances biofilm formation of soil diazotrophic bacteria and biological nitrogen fixation. Plant Biotechnol. J. 20, 2135–2148. doi: 10.1111/pbi.13894
Yan S., Yin L., Dijkstra F. A., Wang P., Cheng W. (2023). Priming effect on soil carbon decomposition by root exudate surrogates: A meta-analysis. Soil Biol. Biochem. 178. doi: 10.1016/j.soilbio.2023.108955
Yang Y., Yang Z., Yu S., Chen H. (2019). Organic acids exuded from roots increase the available potassium content in the rhizosphere soil: A rhizobag experiment in nicotiana tabacum. HortScience 54, 23–27. doi: 10.21273/HORTSCI13569-18
York L. M., Carminati A., Mooney S. J., Ritz K., Bennett M. J. (2016). The holistic rhizosphere: integrating zones, processes, and semantics in the soil influenced by roots. J. Exp. Bot. 67, 3629–3643. doi: 10.1093/jxb/erw108
Yu H., He Y., Zhang W., Chen L., Zhang J., Zhang X., et al. (2022). Greater chemical signaling in root exudates enhances soil mutualistic associations in invasive plants compared to natives. New Phytol. 236, 1140–1153. doi: 10.1111/nph.18289
Zhang Z., Bhowmik P. C., Suseela V. (2021). Effect of soil carbon amendments in reversing the legacy effect of plant invasion. J. Appl. Ecol. 58, 181–191. doi: 10.1111/1365-2664.13757
Zhang P., Li B., Wu J., Hu S. (2019). Invasive plants differentially affect soil biota through litter and rhizosphere pathways: a meta-analysis. Ecol. Lett. 22, 200–210. doi: 10.1111/ele.13181
Keywords: invasive plant, rhizodeposit, plant-soil feedback (PSF), stress tolerance, allelopathy
Citation: Lowry CJ, Blocklove KM, Eckert KE, Koirala N and Rice EK (2024) If you can’t beat them, join them: using invasive plants to understand rhizodeposits as drivers of plant-soil feedbacks. Front. Agron. 6:1363124. doi: 10.3389/fagro.2024.1363124
Received: 29 December 2023; Accepted: 09 February 2024;
Published: 08 March 2024.
Edited by:
Michael Benjamin Kantar, University of Hawaii, United StatesReviewed by:
Qaiser Javed, Jiangsu University, ChinaSajid Latif, Wagga Wagga Agricultural Institute (WWAI), Australia
Yulong Feng, Shenyang Agricultural University, China
Copyright © 2024 Lowry, Blocklove, Eckert, Koirala and Rice. This is an open-access article distributed under the terms of the Creative Commons Attribution License (CC BY). The use, distribution or reproduction in other forums is permitted, provided the original author(s) and the copyright owner(s) are credited and that the original publication in this journal is cited, in accordance with accepted academic practice. No use, distribution or reproduction is permitted which does not comply with these terms.
*Correspondence: Carolyn J. Lowry, Q2Fyb2x5bi5Mb3dyeUBwc3UuZWR1