- 1Department of Soil and Geological Sciences, College of Agriculture, Sokoine University of Agriculture, Morogoro, Tanzania
- 2Department of Crop Science and Horticulture, College of Agriculture, Sokoine University of Agriculture, Morogoro, Tanzania
- 3The National Environment Management Council (NEMC), Dodoma, Tanzania
Farming practices reliant solely on synthetic agrochemicals face unreliability in the current era marked by unpredictable climate changes and rapid soil health deterioration. Consequently, a shift towards sustainable approaches is imperative to ensure both food security and environmental quality. Molasses and vinasse, abundant organic liquid by-products from sugar processing and distillery industries respectively, have historically served as soil conditioners and biofertilizers. Despite their potential, their effectiveness as organic amendments remain relatively unknown globally. In response, we conducted a systematic literature review to unveil the benefits of molasses and vinasse as organic amendments. Our findings reveal that these by-products consist of both inorganic and organic compounds that enhance soil and aquatic ecosystem performance. These compounds include essential plant nutrients as mineral elements and organic matter, contributing to improved soil physico-chemical and biological properties. Notably, the application of molasses and vinasse in crop production has demonstrated superiority over chemical fertilizers, particularly when combined with other inorganic amendments. Molasses and vinasse have been reported to significantly increase yield in several crops including sugarcane (Saccharum officinarum), tomatoes (Solanum lycopersicum), soybean (Glycine max), maize (Zea mays) and rice (Oryza sativa). Strategic utilization of vinasse has the potential to enhance environmental quality by reducing soil heavy metal loads and mitigating negative impacts associated with synthetic fertilizers. However, it is crucial to note that irregular disposal or misuse of these by-products can result in detrimental effects on the environment and human health. To encourage sustainable utilization on a global scale, it is essential to establish appropriate dosages, raise awareness among farmers and stakeholders regarding judicious use, and develop effective methods for handling and application of molasses and vinasse. This approach ensures cost-effective and environmentally friendly organic amendments, fostering a harmonious balance between agricutural productivity and ecological well-being.
1 Introduction
Utilizing organic amendments in crop production offers a promising solution to challenges associated with excessive agrochemical use (Rahimi et al., 2000; Mohanty et al., 2013; Delcour et al., 2015). These amendments, defined as organic substrates applied to soil, aim to enhance soil physical, biological, and chemical properties, improving plant nutrient uptake (Garbowski et al., 2023). The benefits extend to soil quality and environmental performance, encompassing mechanisms such as carbon sequestration, greenhouse gas emission mitigation, and soil fertility enhancement (González et al., 2010; Singh et al., 2011; Mohanty et al., 2013). Organic liquid byproducts, molasses, and vinasse, remnants from sugar and ethanol processing, possess unique properties (Bento et al., 2019; Dhote et al., 2021). Molasses, a viscous black liquid produced through sugar processing, and vinasse, a dark brown organic effluent from ethanol production, find application in agriculture as biofertilizers, animal feeds, or soil conditioners (Reyes-Cabrera et al., 2017; Ratna et al., 2021).
Sugarcane, along with its byproducts molasses and vinasse, is predominantly produced in developed countries across Asia, America, and Europe (Bordonal et al., 2018; Vandenberghe et al., 2022). However, it is important to note that developing nations in Africa also play a significant role in the production of these commodities. For instance, according to the latest data from FAOSTAT (2024), Tanzania’s sugarcane production ranged from 2.8 to 3.6 million metric tons (Mt), while molasses production varied between approximately 79,000 and 98,000 Mt. Unfortunately, information regarding vinasse production in the country is currently unknown, possibly due to its limited utilization.
When appropriately applied, either in their original or modified forms, alone or in conjunction with other compounds, molasses and vinasse significantly enhance soil health and environmental quality (Zikeli et al., 2017; da Silva et al., 2021; Friedrichsen et al., 2021; Jia et al., 2021). Their contributions include improving soil moisture and nutrient retention, increasing soil organic carbon content, and enhancing nutrient availability. Furthermore, they positively impact soil structure stability, total pore space, and microbial activities (Hossain et al., 2016; Nathaniel et al., 2020; Garbowski et al., 2023). Vinasse, in particular, aids in plant nutrient uptake and facilitates heavy metal mobilization during phytoremediation (Hossain et al., 2016; Awad et al., 2021). For instance, Eissa (2017) reported a significant decrease in soil bulk density (BD) by 28.57% and an increase in porosity by 34.78% in response to vinasse application. On the other hand, Pyakurel et al. (2019) found that the application of molasses at a rate of 2 L ha-1 resulted in a significant increase in spinach yield by 65.57% when compared to control-treated plants under field conditions. These findings collectively demonstrate the positive impact of vinasse and molasses on soil properties and crop performance, reinforcing their potential as beneficial additives in agricultural practices.
However, despite the wealth of knowledge on organic amendments, a notable knowledge gap exists concerning the global recognition and utilization of specific organic by-products, namely molasses and vinasse, in improving soil health. While studies demonstrate the potential of these by-products in enhancing soil moisture, nutrient retention, and overall soil quality (Zikeli et al., 2017; da Silva et al., 2021; Friedrichsen et al., 2021; Jia et al., 2021), there is insufficient acknowledgment on a global scale (Oliveira Filho and Pereira, 2020). This lack of recognition has led to inappropriate discharge and underutilization of molasses and vinasse by farmers in crop production (Dhote et al., 2021), emphasizing the need for a systematic review to bridge this knowledge gap and highlight the potential benefits and best practices associated with these organic by-products.
The existing knowledge gap, as outlined in this background, revolves around the underrecognition and underutilization of specific organic by-products, namely molasses and vinasse, on a global scale in improving soil health. Despite the wealth of knowledge demonstrating the significant contributions of these by-products to soil moisture, nutrient retention, and overall soil quality, there is a notable lack of global acknowledgment. This deficiency in recognition has resulted in inadequate utilization and inappropriate discharge of molasses and vinasse by farmers in crop production, emphasizing the need for a systematic review to address this knowledge gap. The review aims to consolidate existing information, raise awareness on a global scale, and provide insights into the potential benefits and best practices associated with incorporating these organic by-products into agricultural practices. Bridging this knowledge gap is crucial for promoting sustainable and informed agricultural decisions, ensuring that the benefits of molasses and vinasse are harnessed effectively to enhance soil health and contribute to environmental conservation.
Agriculture is undeniably vital for sustaining life on Earth, providing essential resources like food and fiber (Sayer and Cassman, 2013). However, agricultural practices can pose negative impacts on land quality and the environment (Chauvel et al., 1991; Horrigan et al., 2002; Tilman and Clark, 2015). The use of chemical fertilizers, though initially beneficial for improving crop performance, has resulted in excessive application, leading to environmental pollution, greenhouse gas emissions, and ecosystem disturbances (Savci, 2012; Zhao et al., 2013; Kihara et al., 2016; Sellare et al., 2020). In response, sustainable agricultural practices are advocated to minimize environmental deterioration while ensuring sustainable food production (González et al., 2010; Mohanty et al., 2013; Garbowski et al., 2023).
In contrast to fixed nutrient types from chemical fertilizers, molasses and vinasse offer a diverse range of essential nutrients in varying amounts (Fuess et al., 2018; Naveed et al., 2018). When used alongside inorganic fertilizers, they can mitigate negative impacts associated with synthetic chemicals and optimize agronomic use efficiency (Kumar and Chopra, 2014; Eissa, 2017; Sadiq et al., 2021; Raza et al., 2022). Their varied nutrient profile supports a more balanced and comprehensive nutrient supply to crops, enhancing growth and development (Seleem et al., 2022). However, improper handling of these byproducts may lead to environmental pollution, emphasizing the need for their careful incorporation into the agricultural cycle to ensure agronomic utilization while safeguarding the environment (Dhote et al., 2021). Despite their potential, further exploration of molasses and vinasse benefits on crops, soil quality, and the overall environment is warranted (Kumar and Chopra, 2014; Oliveira Filho and Pereira, 2020; Zamarreño et al., 2022), prompting this systematic review to highlight opportunities, gaps, challenges, and a way forward in utilizing them as organic soil amendments.
The existing knowledge gap highlighted herein revolves around the need for a more comprehensive understanding of the benefits, challenges, and environmental implications of using molasses and vinasse as organic soil amendments. While the negative impacts of conventional agricultural practices, particularly the excessive use of chemical fertilizers, are acknowledged, there is a gap in understanding the full potential of molasses and vinasse as alternatives and how their integration into agricultural systems can address environmental concerns. The key knowledge gap areas include: (1) Optimal utilization: There is a need to explore the optimal and sustainable incorporation of molasses and vinasse into agricultural practices to maximize their benefits without causing environmental harm. Understanding the proper handling and application methods is crucial to harness their potential while mitigating pollution risks. (2) Comprehensive impact assessment: While the diverse nutrient profile of molasses and vinasse is recognized, there is a knowledge gap in conducting a comprehensive assessment of their impact on crops, soil quality, and the broader environment. This includes understanding their influence on plant growth, soil health, and potential long-term effects on ecosystems. (3) Balancing nutrient supply: Although it is acknowledged that molasses and vinasse provide a varied nutrient profile, there is a need for a deeper understanding of how these organic amendments contribute to a balanced and sustainable nutrient supply to crops. This involves assessing the specific nutrients supplied, their concentrations, and their effectiveness in supporting overall crop development. (4) Environmental safeguards: The discussion emphasizes the potential for environmental pollution if molasses and vinasse are not handled properly. Bridging the knowledge gap requires a detailed exploration of environmentally friendly practices for integrating these byproducts into agriculture, ensuring their agronomic benefits while minimizing negative environmental consequences. (5) Systematic exploration: While some studies have explored the benefits of molasses and vinasse, this systematic review is proposed to provide a consolidated understanding of opportunities, gaps, challenges, and a clear pathway for utilizing these organic amendments in a sustainable manner. This indicates a broader knowledge gap in synthesizing existing information and identifying areas for future research and application. Addressing these knowledge gaps is crucial for promoting sustainable agricultural practices that balance the need for food production with environmental conservation, thereby contributing to a more resilient and eco-friendly agricultural system.
2 Methodology
This study employs the Preferred Reporting Items for Systematic Reviews and Meta Analyses (PRISMA) framework (Figure 1) to investigate the potential of vinasse and molasses as organic amendments, aiming to enhance soil health and crop performance for environmental conservation amidst the challenges posed by climate change (Macusi et al., 2022; Tlatlaa et al., 2023). Comparable methodologies have been utilized by other researchers in the development of their systematic reviews (Gurwick et al., 2013; Pahlevan-Sharif et al., 2019). To explore information on the potential effects of molasses and vinasse on soil health and crop performance, a comprehensive database search spanning from 2013 to 2023 was conducted. Data were sourced from three databases, namely Scopus, WorldWide Science, and PubMed Central, utilizing search terms like “molasses,” “vinasse,” “spentwash,” “soil organic carbon,” “soil properties,” “soil microorganisms,” “crop growth,” and “crop yield.” These terms were strategically combined using Boolean operators AND/OR to encompass all relevant literature within the defined scope (Farid et al., 2016). Initially, all records, including journal articles, research reports, and review articles published globally in English from 2013 to 2023, were identified through database searches, and subsequent removal of duplicates preceded the screening process. Articles underwent screening and data extraction based on abstracts and full texts, ensuring inclusion only if they met predetermined criteria and fell within the scope of the review.
This study follows eight assumptions: - (1) The PRISMA framework is an effective and widely accepted guideline for systematic reviews and meta-analyses, the study benefits from a standardized and rigorous approach. (2) The comparable methodologies used by other researchers, such as Gurwick et al. (2013) and Pahlevan-Sharif et al. (2019), indicate a level of consistency and reliability in systematic review processes. (3) Scopus, WorldWide Science, and PubMed Central are reputable and comprehensive databases, providing a suitable range of literature to address the research question. (4) The selected search terms, including “molasses,” “vinasse,” and related terms, effectively captures relevant literature on the potential effects of these organic amendments on soil health and crop performance. (5) Relevant information is adequately captured by focusing on articles published globally in English from 2013 to 2023, and that language bias does not significantly impact the findings. (6) The predetermined criteria for screening and data extraction based on abstracts and full texts effectively identify studies that meet the research objectives. (7) The removal of duplicates during the screening process is thorough and accurate, minimizing the risk of redundant information in the final dataset. (8) The predetermined criteria for inclusion ensure that the selected studies are relevant to the research question, focusing on the potential effects of molasses and vinasse on soil health and crop performance.
3 Molasses and spentwash (vinasse) production from raw sugarcane
Sugarcane molasses and vinasse, also known as spentwash, are substantial by-products arising from the sugar and ethanol processing industries, alongside press mud and bagasse. As illustrated in Figure 2, in sugar industries, the production of molasses and vinasse involves a series of intricate processes. The journey begins with the extraction of sugar from sugarcane, where the juice undergoes fermentation. During this stage, molasses is derived through the distillation of the fermented sugarcane juice, resulting in a by-product rich in sugars and other compounds (Cherubin et al., 2020). Simultaneously, the production of vinasse occurs as a consequence of ethanol generation, wherein the fermented broth undergoes distillation, leaving behind the residual liquid known as vinasse (Raza et al., 2021). These processes are integral to the sugar industry’s overall operations, contributing to the substantial quantities of molasses and vinasse generated, albeit presenting challenges in terms of effective management and utilization (Raza et al., 2021; Vandenberghe et al., 2022).
The overall production of sugar and molasses in developed countries greatly surpasses the production levels in developing nations, particularly in Africa. Brazil and India stand out globally as the primary contributors to sugar and molasses production, owing to their expansive sugarcane plantations and advanced processing facilities (Bordonal et al., 2018; Vandenberghe et al., 2022). For instance, between 2013 and 2021, Brazil produced approximately 30 million Mt of sugar, while India registered around 36 million Mt. In contrast, Tanzania’s sugar production during the same period was only 340,000 Mt. Similarly, Brazil and India produced an average of 15 million Mt and 12 million Mt of molasses, respectively, whereas Tanzania’s molasses production was a mere 89,000 Mt from 2013 to 2020. These figures clearly demonstrate a significant production gap between developed and developing countries, with Africa being particularly affected. This highlights the urgent need for increased support and focused efforts in the agricultural sectors of developing nations in order to bridge this gap and foster sustainable growth. Molasses, a derivative of fermented sugarcane juice during sugar extraction, is a key output. Approximately 85-100 kg of sugar and 35–45 kg of molasses are generated for every ton of sugarcane crushed, underscoring the magnitude of production (Solomon, 2011). Molasses finds extensive application as a raw material in alcohol production, encompassing potable spirits, industrial alcohol, and ethanol for fuel blending (Shivaraj and Devidas, 2017).
The growth of distillery industries in Asia and South America, particularly fueled by the mandate for ethanol in gasoline, has elevated the demand for molasses as a crucial feedstock (Manochio et al., 2017; Oceguera-Contreras et al., 2019). Ethanol, a renewable energy resource, offers numerous advantages in terms of net energy value and applications across various sectors. Shelar et al. (2023) report that ethanol has a net energy value ranging from approximately 6 to 6.3 MJ L-1. This versatile fuel has found significant utilization in countries like Brazil, India, China, the United Kingdom, and Thailand, particularly in the transportation sector (Mączyńska et al., 2019). Gasohol, a blend of ethanol and gasoline, has been a notable development in these nations, providing improved environmental cleanliness (Balat and Balat, 2009; Gopal and Kammen, 2009). Gasohol can be formulated with different ethanol proportions, such as 5%, 10%, or 20% (Sakthivel et al., 2018). However, the sustainable adoption of bioethanol as an energy source in African countries like Tanzania has been hindered by inadequate policies and limited technological advancements (Quintero et al., 2012). However, due to its high flammability and volatility, direct use of ethanol in the distillation process for ethanol production is limited, leading to the continued reliance on primary energy sources like coal, crop residues, and biogas (Nguyen et al., 2008; Baeyens et al., 2015; Calvin et al., 2022).
Ethanol production from cane molasses presents a comprehensive solution to address environmental challenges associated with the irregular dispersal of molasses. By utilizing molasses as a feedstock for ethanol production, it not only avoids harmful environmental practices like uncontrolled dumping or improper disposal but also transforms a waste product into a valuable resource (Gopal and Kammen, 2009). This sustainable approach helps to mitigate the negative environmental impacts that would otherwise arise from the mishandling of molasses. Moreover, ethanol derived from cane molasses is considered a renewable energy source due to the renewable nature of sugar cane itself (Shelar et al. (2023). This quality makes it an attractive alternative to fossil fuels, reducing dependence on non-renewable resources and contributing to the mitigation of greenhouse gas emissions.
However, it is important to acknowledge that the process of ethanol production from cane molasses may have some harmful effects on the environment. Notably, the production of ethanol generates significant volumes of vinasse, ranging from 12 to 20 liters for every liter of ethanol produced (Moraes et al., 2014). Inadequate management of the substantial amount of vinasse produced can present environmental concerns. If not handled properly, this waste material has the potential to cause environmental pollution, impacting water bodies and soil, and posing a threat to local ecosystems (Wang et al., 2014). Brazil leads in vinasse production, with an annual output reaching around 300 billion liters, followed by India with approximately 40 billion liters and China contributing about 2.2 million tons annually (Wei and Xu, 2004; Dhote et al., 2021; Carpanez et al., 2022). The past decade has witnessed a substantial global surge in the production and consumption of sugar and ethanol, driven by factors such as population growth, increased demand for renewable energy, government incentives for biofuels, and the depletion of petroleum reserves (Mikucka and Zielińska, 2020). This growth has not only expanded the sugar industry but has also propelled the rapid development of distillery sectors, introducing both opportunities and challenges in the effective management and utilization of molasses and vinasse (Wei and Xu, 2004).
The interlinked expansion of these sectors underscores the imperative for comprehensive policies and practices. These should ensure the safe and sustainable handling of by-products, considering their environmental impact and exploring potential avenues for reuse or dispersal across various sectors (Dhote et al., 2021). Despite significant strides, there still exists a knowledge gap in understanding the full extent of the environmental implications and the optimal utilization of these abundant by-products. Closing this gap is essential for fostering a more sustainable and environmentally conscious approach to the management of sugar and ethanol by-products on a global scale.
4 Composition and properties of molasses
The composition of molasses and vinasse exhibits significant variability influenced by diverse factors, including soil type, management practices, climate, manufacturing processes, crop maturity stage, and crop variety (De Godoi et al., 2019). Both substances, characterized by their highly viscous nature, possess a thick and sticky consistency, with viscosity influenced by factors like temperature and the concentration of soluble and suspended materials. Although their density typically falls within the range of 1.01 to 1.5 g mL-1 (Fuess et al., 2018; Naveed et al., 2018), specific physico-chemical properties are presented in Tables 1 and 2.
Rich in organic compounds, molasses and vinasse serve as valuable resources for soil amendment and agricultural applications. These compounds include residual sugars such as glucose, fructose, and sucrose, along with organic acids like acetic, citric, malic, and lactic acids, originating from microbial activities during fermentation (Fuess et al., 2018; Naveed et al., 2018; Buller et al., 2021; Saeed et al., 2021; Lourenço et al., 2022). The high concentration of organic acids contributes to their acidic nature, reflected in a pH range from extremely acidic (3.5) to slightly acidic (6.5) (Naveed et al., 2018; Lourenço et al., 2022).
Additionally, molasses and vinasse contain varying amounts of mineral elements. Macroelements such as potassium (K), calcium (Ca), magnesium (Mg), phosphorus (P), and nitrogen (N) are present in concentrations ranging from 35 to 4600 mg L-1, while microelements or trace elements like iron (Fe), zinc (Zn), manganese (Mn), copper (Cu), lead (Pb), and aluminum (Al) are found in smaller concentrations ranging from 0.1 to 25 mg L-1 (Fuess et al., 2018; Naveed et al., 2018; Lourenço et al., 2022). The elevated concentration of soluble bases, including K, Ca, Mg, and Na, contributes to the high electrical conductivity observed in molasses and vinasse, ranging from 11 to 51 d Sm-1 (Fuess et al., 2018; Naveed et al., 2018). This comprehensive understanding of the physico-chemical properties of molasses and vinasse underscores their potential as versatile and valuable components in various agricultural and environmental applications.
The existing main knowledge gap in the context of environmental conservation is the lack of detailed information on how the variability in the composition of molasses and vinasse, influenced by diverse factors, may impact environmental outcomes. While the physico-chemical properties of these substances are outlined, there is a need for further research to elucidate the specific environmental implications arising from their variable composition. Understanding how factors such as soil type, management practices, climate, and manufacturing processes influence the composition of molasses and vinasse can provide valuable insights into their environmental effects, especially in terms of soil health, water quality, and overall ecosystem impact. Bridging this knowledge gap would contribute to more informed and sustainable practices when utilizing molasses and vinasse in agricultural and environmental applications.
5 The influence of molasses and vinasse on soil properties
The use of either vinasse or molasses in soil has proven to be beneficial, positively impacting the total organic carbon content and showing promise in restoring organic matter levels in highly degraded soils (Zani et al., 2018; Dos Santos et al., 2020). The high organic matter content in both molasses and vinasse contributes to an increase in total soil organic carbon, enhancing soil fertility, structure, and overall health (Nunes et al., 2021). Studies have demonstrated significant increases in soil organic matter with the co-application of vinasse and other organic amendments, indicating a potential restoration of degraded soil (Raza et al., 2022). The enrichment of soil with soluble minerals such as calcium, magnesium, potassium, and phosphorus through the application of molasses or vinasse further enhances soil fertility (Van Vuuren et al., 2010; Christofoletti et al., 2013; Hoarau et al., 2018; Feder, 2021; Jayashree et al., 2022).
Vinasse application has been linked to a substantial increase in soil base saturation and cation exchange capacity (CEC), positively impacting the availability and exchange of basic cations in the soil (Eissa, 2017; Fuess et al., 2021). Adequate base saturation and CEC are crucial indicators of soil fertility, ensuring a sufficient supply of essential basic cations for plant physiological processes (Aprile and Lorandi, 2012; Chaganti et al., 2021; Culman et al., 2021). Both molasses and vinasse contribute to an increase in soil electrical conductivity, with studies showing no adverse effects on plant growth within optimal ranges (Corwin and Plant, 2005; Jayashree et al., 2022; Raza et al., 2022). The impact on soil pH, however, has been inconsistent, with some studies reporting a decrease and others an increase. Further research is needed to establish conclusive findings on the effects of molasses and vinasse on soil pH (Eissa, 2017; Naveed et al., 2018; Pyakurel et al., 2019; Oak et al., 2021).
Molasses and vinasse influence soil physical strength by enhancing soil aggregation directly and indirectly. They act as cohesive materials, binding soil particles and improving soil structure, leading to better aeration, water retention, and overall soil quality (Hossain et al., 2016; Saneiyan et al., 2019; Litardo et al., 2022). Additionally, the byproducts stimulate microbial-induced calcium precipitation, promoting the formation of stable soil aggregates (Nikseresht et al., 2020; Romano et al., 2020; Behzadipour and Sadrekarimi, 2021; Saneiyan et al., 2021). The application of vinasse has been associated with increased soil thermal conductivity, reduced soil bulk density, and increased soil porosity, all of which contribute to improved soil quality and plant growth (Place et al., 2008; Gutiérrez et al., 2016; Eissa, 2017).
The application of byproducts such as molasses and vinasse in crop production serves as a valuable and long-lasting source of organic carbon and mineral nutrients, which has a positive impact on soil fauna diversity, abundance, and activities (Franzluebbers, 1999; Do Nascimento et al., 2019). These byproducts play a crucial role in enhancing soil fertility and promoting sustainable agricultural practices. Notably, vinasse application has shown significant increases in soil fauna and microbial biomass compared to the use of chemical fertilizers (Behzadipour and Sadrekarimi, 2021; Chuppa-Tostain et al., 2018; Seleem et al., 2022). The rich organic content of vinasse provides an ideal environment for soil organisms, leading to improved soil health and nutrient cycling. Moreover, when vinasse is co-applied with inorganic fertilizers, it has been shown to have a more pronounced effect on increasing soil microbial populations than the use of inorganic fertilizers alone (Navarrete et al., 2015; Durrer et al., 2017; Kaziūnienė et al., 2022). This synergistic effect suggests that combining organic byproducts with inorganic amendments can maximize the benefits for soil microbial communities. These findings underscore the potential of utilizing molasses and vinasse in combination with other inorganic enhancements to promote soil health, enhance nutrient availability, and ultimately improve crop productivity. By adopting such practices, farmers can reduce their reliance on chemical fertilizers while fostering sustainable and environmentally friendly agricultural systems.
One significant knowledge gap regarding soil properties is the inconsistent findings regarding the impact of molasses and vinasse on soil pH. While some studies report a decrease in soil pH following the application of these byproducts, others indicate an increase. This inconsistency highlights the need for further research to establish more definitive conclusions about the effects of molasses and vinasse on soil pH. Understanding the factors influencing these variations and conducting additional studies to clarify the specific conditions under which pH changes occur would contribute to a more comprehensive understanding of the overall impact of these byproducts on soil properties.
6 The influence of molasses and vinasse on crop performance
The use of molasses and vinasse in crop cultivation provides a conducive environment for plant growth, offering various advantages (See Figure 3). These include the adjustment of pH levels, enhanced soil aeration, increased moisture availability, and the reduction of micronutrient toxicity (Scarpare et al., 2016; Reyes-Cabrera et al., 2017; de Souza Oliveira Filho et al., 2021; Litardo et al., 2022). These amendments have demonstrated positive effects on yield and yield quality in a variety of crops, such as tomatoes (Solanum lycopersicum), soybean (Glycine max), maize (Zea mays L.), barley (Hordeum vulgare), rice (Oryza sativa), elephant foot yam (Amorphophallus paeoniifolius), and sugarcane (Saccharum officinarum) (Surendran et al., 2016; Dotaniya et al., 2016; Di Gioia et al., 2017; Naveed et al., 2018; Jayashree et al., 2022; Pinto et al., 2022).
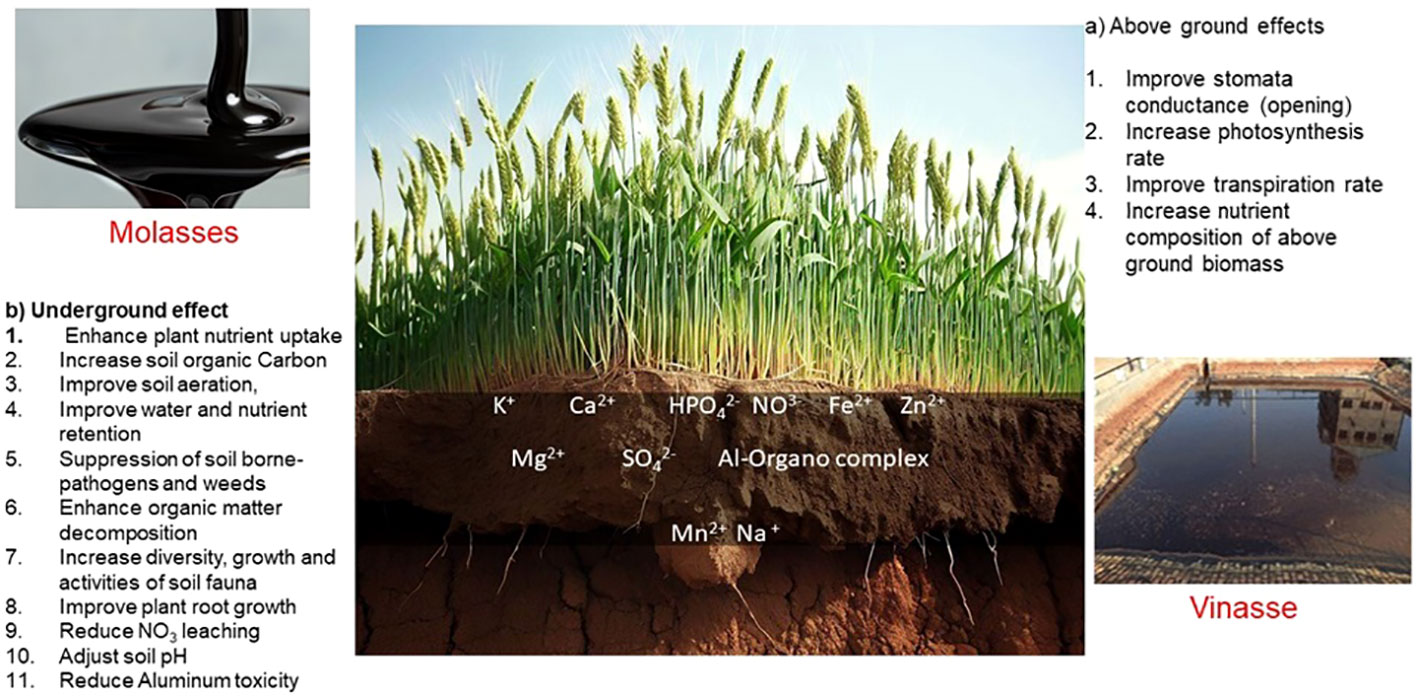
Figure 3 A depiction showcasing the positive effects of vinasse and molasses on soil characteristics and the performance of wheat as a representative crop.
For example, Seleem et al. (2022) reported a 76.91% increase in barley’s total grain weight yield in vinasse-treated crops compared to those treated with chemical fertilizers. Similarly, Naveed et al. (2018) observed enhanced growth and yield parameters in rice, including increased height, panicle length, and number of tillers, grain weight, total grain yield, and grain yield per plant with vinasse application relative to chemical fertilizers. Molasses and/or vinasse play a significant role in improving plant nutrient availability through mechanisms such as direct supply of mineral elements to plants. Molasses contains appreciable amounts of soluble plant nutrients, readily available to plants (Cardin et al., 2016; Omori et al., 2016; Rothe et al., 2019; Kumari et al., 2020; Gowd et al., 2022). Goswami et al. (2023) suggested that the abundant nutrients in molasses and vinasse could directly support plant growth, even without relying on nutrient recycling by microorganisms in the soil. Additionally, co-application of vinasse and poultry manure significantly increased potassium, phosphorus, and nitrogen compared to chemical fertilizers (Seleem et al., 2022). Vinasse also promotes the decomposition of organic matter in the soil, releasing nutrients previously locked up in plant residues or other organic materials (Suleiman et al., 2018; Zhu et al., 2020).
Vinasse plays a crucial role in improving the agronomic efficiency of agricultural inputs, including chemical fertilizers, by mitigating nutrient loss and extending the nutrient release period. Combined application of vinasse or vinasse-biochar with urea has been shown to decrease nitrogen loss through processes like NH3 volatilization, NO3 leaching, and surface run-off (Engström et al., 2014; Jia et al., 2021; Wester-Larsen et al., 2022). Litardo et al. (2022) reported that co-application of vinasse and inorganic fertilizers enhanced plant nutrient uptake by rice crops, indicating increased efficiency of agricultural inputs. Vinasse has been found to promote various plant physiological processes, enhancing photosynthesis rate, stomatal conductance, and respiration rate, leading to improved CO2 utilization, water regulation, and macromolecule synthesis and distribution in plants (Naveed et al., 2018). Furthermore, vinasse contains plant growth-promoting hormones (PGPHs) such as Gibberellic acid (GA), Indole acetic acid (IAA), Abscisic acid (ABA), Zeatin (Z), cis-zeatin riboside (c-ZR), isopentenyladenine (iP), and isopentenyladenosine (iPR), which contribute to enhanced plant growth and yield (Khan et al., 2020; Hassan et al., 2021; Zamarreño et al., 2022).
In recent studies, vinasse has been explored as an organic substrate in controlling soil-borne pathogens through anaerobic soil disinfestation (ASD). Lee et al. (2020) observed a significant reduction in the density of Fusarium oxysporum L. sp. lycopersici, a fungal pathogen causing Fusarium wilt in tomatoes, during ASD with vinasse. This reduction suggests the effectiveness of vinasse, and possibly molasses, in suppressing soil-borne pests through anaerobic decomposition that enhances the antagonistic activities of beneficial microorganisms against pathogens (Shrestha et al., 2016).
While molasses and vinasse have shown several advantages in soil health and crop production, it is important to consider their potential drawbacks. One significant demerit is the risk of nutrient imbalances, mainly due to the varying composition of these byproducts (Fuess et al., 2018; Jamir et al., 2021). This variability makes it difficult to establish a single common nutrient content and ratios, which can lead to over- or under-application of certain nutrients, negatively impacting plant growth. Additionally, the high organic matter content of molasses and vinasse can contribute to an increase in soil acidity, potentially disrupting the pH balance and nutrient availability (Buller et al., 2021; Dhote et al., 2021). Moreover, improper application or excessive use of these byproducts can result in the accumulation of salts and other toxic substances in the soil, ultimately leading to decreased soil productivity (Alves et al., 2015; Naveed et al., 2018). Therefore, to ensure the safe and effective use of molasses and vinasse in crop production, careful management practices are crucial. These practices include proper nutrient analysis, monitoring soil pH, and considering potential contamination risks.
The provided explanation highlights the potential of vinasse and molasses in improving the agronomic efficiency of agricultural inputs, including synthetic fertilizers, by mitigating nutrient loss and extending the nutrient release period. However, a crucial knowledge gap is the lack of specific information on the environmental impacts of synthetic fertilizers and how the use of vinasse and molasses as amendments may contribute to environmental conservation in comparison to synthetic fertilizers. While the benefits of vinasse and molasses in nutrient retention and plant nutrient uptake are emphasized, a comprehensive understanding of the environmental implications of synthetic fertilizers, such as their contribution to water pollution, soil degradation, and greenhouse gas emissions, is missing. To bridge this gap, further research is needed to assess and compare the environmental impacts of using vinasse and molasses in combination with synthetic fertilizers versus the use of synthetic fertilizers alone. This would help determine the potential of these organic amendments in promoting sustainable agricultural practices and minimizing negative environmental effects associated with synthetic fertilizers.
7 Role of vinasse and molasses in enhancing environmental remediation
Sodium (Na) plays a crucial role as a beneficial nutrient in certain crops, enhancing their performance and yield. However, elevated concentrations of sodium can negatively impact plant growth and soil properties (Bauder and Brock, 2001; Leogrande and Vitti, 2019). Sugarcane vinasse application has demonstrated potential benefits in mitigating sodium toxicity in soil. Gutiérrez et al. (2016) found that applying vinasse at 10 L ha-1 significantly reduced exchangeable sodium percentage (ESP) by 23.58% and sodium adsorption ratio (SAR) by 65.83% in sodic soils. This effect is attributed to the enrichment of soil with divalent cations, primarily calcium (Ca2+), as highlighted by Kopittke (2012), Tobe et al. (2003), and Rahimi et al., 2000.
Aluminium, another beneficial nutrient, can be toxic to many crops and soil microorganisms (Aggarwal et al., 2015; Bojó́rquez-Quintal et al., 2017). It is known for its acidifying effect on soil and inhibitory impact on plant nutrient availability, root growth, and development (Rahman et al., 2018). Fortunately, vinasse has shown significant efficacy in reducing aluminum solubility, hindering its availability to plants and soil microorganisms (Fuess et al., 2021). The application of vinasse reduced aluminum saturation by over 20% without compromising soil fertility status. Although specific mechanisms are not fully understood, de Souza Oliveira et al. (2009) and Singh et al. (2021) proposed that the chelating effects of vinasse on aluminum form a less soluble complex, limiting aluminum reactivity in soil and plant roots. Vinasse has also been explored for its potential in phytoremediation, the use of plants to mitigate environmental pollution (Abdelkrim et al., 2019). Heavy metals, persistent pollutants with adverse effects on human health and biodiversity, pose a significant environmental concern (Ashraf et al., 2019). Vinasse application has demonstrated notable removal of lead (Pb) and increased solubility of cadmium (Cd) in various studies (Eissa, 2017; Awad et al., 2021). The efficiency of metal removal by plants, such as Amaranthus tricolor, and quail bush (Atriplex lentiformis), increased significantly with vinasse, showcasing its potential in phytoremediation efforts.
The use of sugarcane vinasse and molasses in crop production has shown promising effects in reducing reliance on chemical fertilizers, which are often associated with environmental pollution and soil disturbances (Ogbodo, 2013; Prashar and Shah, 2016). Vinasse alone contributes a substantial amount of available nutrients, with Cui et al. (2021) highlighting its contribution to about 2.3% of the total nitrogen biomass reservoir in China. Co-applying vinasse with poultry manure has shown notable improvements in plant nutrient availability compared to chemical fertilizers, with increases in potassium, phosphorus, and nitrogen (Seleem et al., 2022). Moreover, vinasse serves as a sustainable and enduring source of nutrients in agriculture, potentially reducing the need for inorganic fertilizers in crop production (See Table 3). Bi et al. (2023) evaluated various nitrogen fertilizer pathways and emphasized the intricate environmental implications of synthetic nitrogenous fertilizers, underscoring the need for sustainable agricultural practices to mitigate nitrogen-related environmental impacts (See Figure 4). Additionally, Tlatlaa et al. (2023) pointed out that the emission of nitrous oxide (N2O) resulting from nitrogenous fertilizers may contribute significantly to atmospheric warming, emphasizing the environmental implications of nitrogen use in agriculture.
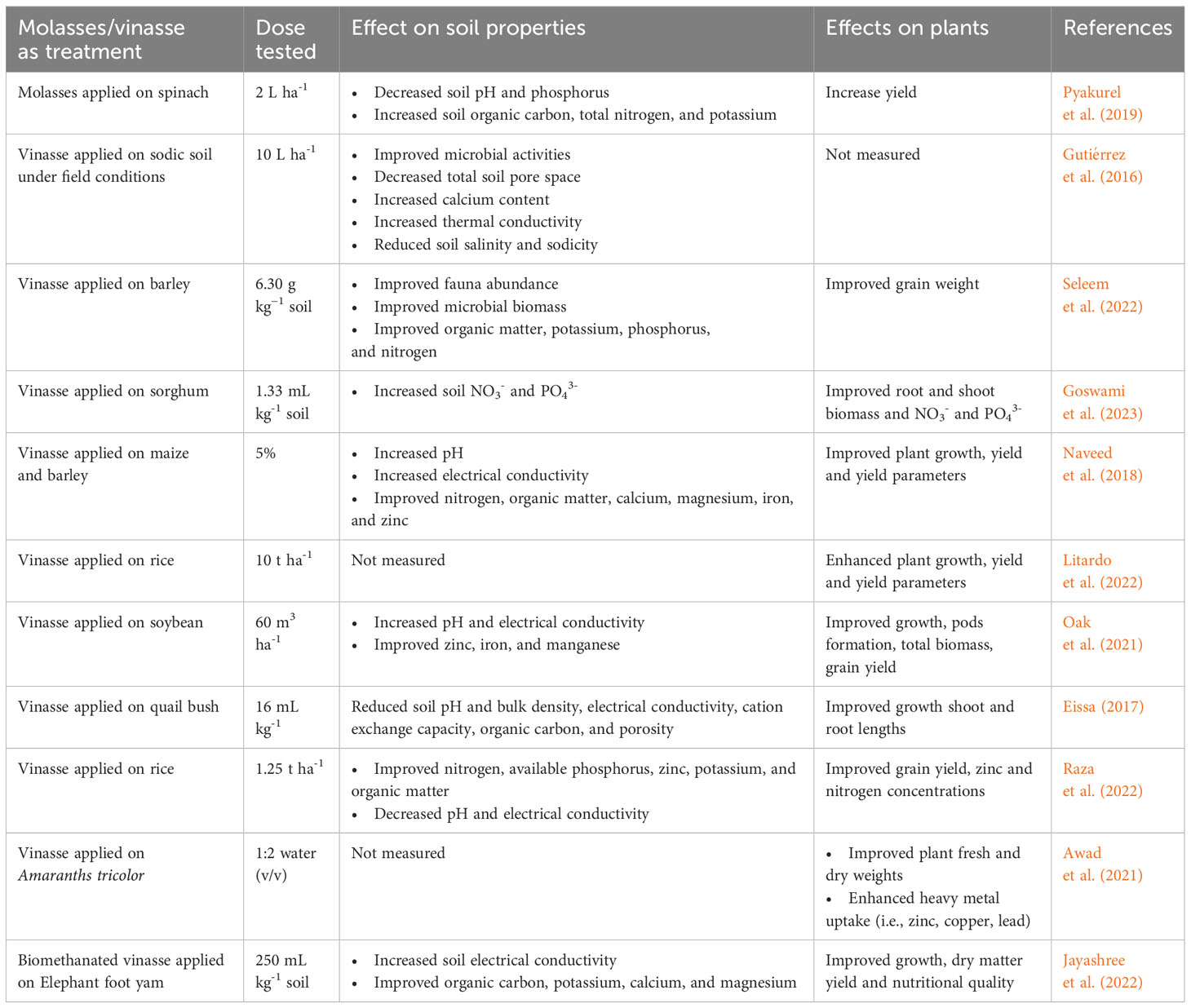
Table 3 Exploring literature discussing the impact of molasses or vinasse application on soil properties and crop performance across various soil conditions.
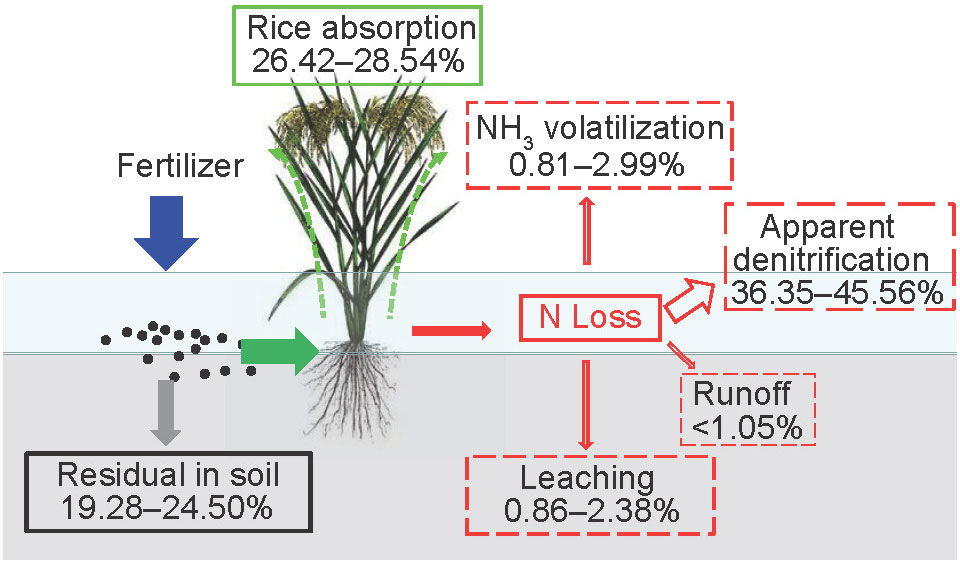
Figure 4 Mapping out the routes of nitrogenous fertilizers in practice. Visual representation reproduced from Figure 6 of 'Fate of fertilizer nitrogen and residual nitrogen in paddy soil in Northeast China' by Bi et al. (2023) under CC BY-NC-ND 4.0 DEED.
The explanation provided highlights several positive aspects of using sugarcane vinasse in agriculture, particularly in addressing issues related to sodium toxicity, aluminum solubility, and heavy metal removal in soils. However, there are notable knowledge gaps that remain and need further exploration for a more comprehensive understanding of the environmental conservation implications. While the studies acknowledge the positive effects of vinasse, the specific mechanisms by which it mitigates sodium toxicity, reduces aluminum solubility, and enhances heavy metal removal are not fully understood. Further research is needed to delve into the underlying biochemical and physiological processes involved in these interactions. The presented information focuses on short-term effects of vinasse application. Long-term studies are crucial to assess the sustainability and persistence of the positive impacts, as well as any potential unintended consequences or accumulative effects on soil health and ecosystem dynamics. Although vinasse shows promise in phytoremediation efforts, the efficiency and reliability of this method across different environmental conditions and plant species need more investigation. Understanding the specific mechanisms by which vinasse enhances metal removal and solubility is essential for optimizing its use in diverse phytoremediation scenarios. The potential environmental impacts of the constituents of vinasse, such as organic matter and other compounds, need thorough examination. This includes studying their breakdown, leaching potential, and effects on water quality, ensuring that the use of vinasse aligns with broader environmental conservation goals. Most studies cited herein are likely conducted under controlled conditions. Large-scale field studies are necessary to validate the efficacy of vinasse in real-world agricultural settings and to assess its broader environmental implications on a landscape scale. While vinasse shows promise as an alternative to chemical fertilizers, more research is needed to explore integrated approaches that combine vinasse with other sustainable practices. This includes studying the synergistic effects of vinasse with cover cropping, agroforestry, or other conservation practices to develop holistic and effective environmental conservation strategies. Therefore, addressing these knowledge gaps will contribute to a more nuanced understanding of the environmental impact of using sugarcane vinasse in agriculture, guiding sustainable practices and policies for environmental conservation in the long run.
While vinasse and molasses hold promise as potential solutions for environmental restoration, it is crucial to recognize that their irregular use can contribute significantly to environmental pollution. The high organic and inorganic load present in molasses and vinasse poses a significant challenge in terms of managing contamination of underground water (Pires et al., 2016). Excessive application of these byproducts can lead to the pollution of water bodies, thereby affecting both underground and aquatic environments. Research conducted by Goswami et al. (2023) and Ortegón et al. (2016) emphasizes the potential for these byproducts to significantly contaminate underground water sources. In addition, irregular use of these byproducts have been linked to greenhouse gasses emission. Although there is limited researches conducted on this subject particularly in agriculture, but available evidence suggests that their sporadic application can result in substantial emissions of greenhouse gasses including carbon dioxide (CO2), methane (CH4), and nitrous oxide (N2O) as indicated by De Figueiredo et al. (2010). For instance, in their study, De Figueiredo et al. (2010) revealed that vinasse, along with other agricultural residues, could emit N2O that is around 50,000 tons of CO2 equivalents, surpassing emissions from fossil fuel consumption (about 12, 000 tons of CO2 equivalents). These findings underscore the importance of carefully managing the utilization of vinasse and molasses to minimize their potential environmental impact and ensure the adoption of sustainable agricultural practices.
8 Institutional guidelines governing the production and utilization of molasses and vinasse
The global concern over environmental pollution stemming from molasses and vinasse in both terrestrial and aquatic ecosystems has prompted the establishment of policies to regulate their production and application. These byproducts, rich in organic matter, nutrients, and pollutants, pose environmental risks if not managed effectively (Raza et al., 2021; Goswami et al., 2023). Despite efforts to address these concerns, the majority of regulations have been formulated and effectively enforced in developed countries, such as Europe, the United States of America, India, and Brazil, leaving a notable gap in policies for many developing nations (Moraes et al., 2014; Wang et al., 2014).
The absence of comprehensive policies in developing countries, particularly in Africa, presents a significant challenge to the sustainable management of molasses and vinasse. Without proper regulations, there is an elevated risk of improper disposal and inadequate treatment, leading to soil, water, and ecosystem pollution with far-reaching consequences (Wang et al., 2014; Ogemdi and Gold, 2018; Iloms et al., 2020). While some African countries, including Nigeria, Namibia, South Africa, Kenya, and Tunisia, have implemented plans and legislations for environmental protection, these are often general and lack specificity for molasses and vinasse (Wang et al., 2014; Ferronato and Torretta, 2019; Onu et al., 2023).
The policies addressing molasses and vinasse aim to establish a framework ensuring their safe reuse or dispersal within the environment. A significant focus lies on the mandatory treatment of these byproducts, aiming to eliminate or reduce organic materials and heavy metals, which can lead to significant environmental pollution (Moran-Salazar et al., 2016; Dhote et al., 2021). These guidelines set standard values for treated molasses and vinasse, ensuring thorough treatment and minimizing potential risks, thus protecting the environment from pollution (Companhia Ambiental do Estado de São Paulo (CETESB), 2006; United States Environmental Protection Agency, 2004). The ultimate goal is the responsible management of these byproducts, mitigating environmental risks associated with their disposal or utilization.
Moreover, these policies advocate for sustainable practices within industries managing molasses and vinasse. This may involve promoting advanced treatment technologies, efficient waste management systems, or innovative methods for the beneficial reuse of these byproducts. By incorporating sustainability principles, governments aim to foster a circular economy, where molasses and vinasse are considered valuable resources rather than mere waste products (Christodoulou and Stamatelatou, 2016). Beyond treatment and reuse, the policies also address proper storage, transportation, and disposal practices. Adequate storage facilities and protocols prevent leaks or spills, safeguarding soil and water sources. Similarly, stringent regulations on transportation ensure the safe conveyance of these byproducts without posing harm to the environment or public health (Buller et al., 2021).
While the provided explanation comprehensively addresses the policies and challenges related to the environmental impact of molasses and vinasse, a notable knowledge gap pertains to the effectiveness and enforcement of these policies. Specifically, there is a need for in-depth research on the implementation and compliance levels of the established regulations in different regions, both in developed and developing countries.
Understanding how well the policies are implemented on the ground is crucial. Research should investigate the extent to which industries adhere to the prescribed guidelines for the treatment, storage, transportation, and disposal of molasses and vinasse. Assessing the effectiveness of regulatory mechanisms in ensuring compliance is essential for evaluating the actual impact of these policies on environmental conservation. This review highlights that many comprehensive policies are developed and enforced in developed countries, leaving a gap in developing nations. Further research should delve into the reasons behind these regional disparities, considering factors such as economic capacities, infrastructure, and institutional capabilities. Addressing this gap will aid in formulating targeted strategies for enhancing environmental conservation practices in developing regions. Research should explore the existence and efficiency of monitoring and reporting systems associated with molasses and vinasse management. Assessing the availability of data on compliance, environmental impact assessments, and incidents related to these byproducts will contribute to a more nuanced understanding of the current state of environmental conservation efforts.
Investigating the level of stakeholder involvement, including industries, local communities, and regulatory bodies, is crucial. Additionally, assessing the awareness levels among these stakeholders regarding the environmental consequences of molasses and vinasse and their understanding of implemented policies will shed light on the potential gaps in knowledge and cooperation. Given the dynamic nature of environmental challenges, there is a need to explore the adaptability of existing policies to changing circumstances. Research should investigate the incorporation of innovative technologies and practices in the treatment, reuse, and disposal of molasses and vinasse, ensuring that policies evolve to address emerging environmental concerns. By addressing these knowledge gaps, future research can contribute to a more holistic understanding of the efficacy of existing policies and provide insights for refining and tailoring environmental conservation measures related to molasses and vinasse on a global scale.
This study sheds light on the future scope of the discipline. In developing countries, particularly in Africa, ensuring the sustainable management of molasses and vinasse requires institutions to commit to comprehensive policies. These policies should address the specific requirements and regulatory frameworks for the safe reuse, treatment, storage, transportation, and disposal of these byproducts. Future research should focus on exploring the unique challenges and opportunities in African contexts to establish effective guidelines and strategies. By doing so, institutions can contribute to sustainable development and economic growth while promoting environmental protection and resource conservation. This holistic approach will help to manage molasses and vinasse responsibly, benefiting both the countries and the environment.
Future research can play a crucial role in evaluating the effectiveness and enforcement levels of existing policies across different regions, encompassing both developed and developing countries. Such research can focus on assessing industry adherence to prescribed guidelines while also evaluating the impact of regulatory mechanisms on environmental conservation efforts. By understanding the barriers and facilitating factors that affect policy compliance, strategies can be developed to enhance implementation and enforcement. Additionally, it is essential to investigate the reasons for regional disparities in policy development and enforcement, considering factors such as economic capacities, infrastructure, and institutional capabilities. This comprehensive analysis can contribute to the formulation of targeted strategies aimed at improving environmental conservation practices in developing regions and bridging the policy gap between developed and developing nations.
In addition to the aforementioned areas of research, it is crucial for future studies to emphasize the role of innovative technologies and practices in the development of policies. Recognizing the ever-changing nature of environmental challenges, research should delve into the integration of innovative technologies and practices in the treatment, reuse, and disposal of molasses and vinasse. This entails exploring emerging technologies that facilitate efficient waste management, advanced treatment methods, and sustainable reuse options. By incorporating these advancements into policies, it becomes possible to address emerging environmental concerns effectively and promote the widespread adoption of sustainable practices. This proactive approach enables the establishment of a more efficient and adaptable regulatory framework that can keep pace with evolving environmental demand.
9 Conclusion and perspectives
Molasses and vinasse have been utilized as biofertilizers and soil conditioners for numerous years, showcasing their potential to enhance soil health and environmental quality. Both substances contribute to the improvement of soil organic carbon, elevate plant nutrient availability, regulate plant growth and physiologies, and enhance the agronomic use efficiency of fertilizers. Additionally, they play a role in alleviating heavy metals and sodium toxicity in soils, further promoting overall soil health.
Despite their positive attributes, it is crucial to highlight that improper use of molasses or vinasse can lead to adverse effects, including salinization, the accumulation of potential toxic elements, and aquatic eutrophication. In the current scenario where agricultural soil productivity is on a decline and inorganic fertilizers exhibit limited effectiveness, molasses and vinasse emerge as cost-effective and environmentally friendly solutions to bolster soil health and environmental quality.
Based on this review, the following recommendations can be made: (i) Establishing the optimal dosage of molasses or vinasse tailored to the agroecological system, specific crops, or soil types. (ii) Raising awareness among farmers and other stakeholders, such as government entities, organizations, and sugar industries, regarding the potential benefits of using molasses and vinasse for soil health and crop performance. (iii) Conducting research on the potential effects of molasses and vinasse on crop yield and nutritional quality, encompassing various crops and soil types. (iv) Investigating appropriate application methods and developing suitable forms of vinasse or molasses to optimize their utilization. (v) Exploring the effectiveness of different mechanisms involved in plant growth promotion and phytoremediation associated with molasses and vinasse. (vi) Formulating or updating specific policies to enforce the safe handling and use of molasses and vinasse, ensuring sustainable environmental quality practices are adhered to.
To ensure responsible and sustainable utilization of molasses and vinasse, future research should prioritize the exploration of appropriate dosage levels that minimize negative impacts on the environment, including soil and aquatic ecosystems, while simultaneously enhancing crop growth and nutritional quality. Understanding the optimal application rates of these byproducts is crucial to prevent potential risks such as nutrient imbalance, soil degradation, and water pollution. Research can focus on determining the ideal quantities that provide sufficient benefits to crops without causing ecological harm. This includes studying the effects of different dosages on soil fertility, water quality, and the overall health of aquatic organisms. By uncovering the appropriate dosage thresholds, researchers can contribute to the development of guidelines and recommendations that support sustainable agricultural practices while safeguarding the environment.
It is also essential to investigate appropriate application methods and develop suitable forms of these byproducts. Future research can focus on exploring innovative techniques that enable efficient and targeted application, such as the use of controlled-release formulations or precision agriculture approaches. By developing advanced application methods, researchers can ensure that the byproducts are distributed in a manner that maximizes their benefits while minimizing potential negative impacts on the environment. Overall, these future researches can contribute to the development of sustainable agricultural practices that enhance the effectiveness and environmental compatibility of vinasse and molasses utilization.
Further exploration of the mechanisms underlying plant growth promotion and phytoremediation associated with molasses and vinasse can yield valuable insights into their mode of action and potential applications. This knowledge is crucial for optimizing their utilization in specific crops, varying soil conditions, and targeted environmental remediation efforts. It can guide the development of tailored application strategies and formulations, ensuring the maximum efficacy of molasses and vinasse in promoting plant growth and facilitating phytoremediation processes. Moreover, understanding the mechanisms involved can shed light on potential synergistic effects when these byproducts are used in combination with other agricultural inputs or remediation techniques. Overall, this research holds significant promise for harnessing the full potential of molasses and vinasse in sustainable agriculture and environmental management.
Data availability statement
The raw data supporting the conclusions of this article will be made available by the authors, without undue reservation.
Author contributions
GS: Conceptualization, Data curation, Formal Analysis, Methodology, Resources, Validation, Visualization, Writing – original draft, Writing – review & editing. MS: Data curation, Methodology, Supervision, Validation, Visualization, Writing – original draft, Writing – review & editing. MB: Data curation, Visualization, Writing – review & editing. CK: Data curation, Visualization, Writing – review & editing. EN: Data curation, Methodology, Supervision, Validation, Visualization, Writing – review & editing.
Funding
The author(s) declare that no financial support was received for the research, authorship, and/or publication of this article.
Conflict of interest
The authors declare that the research was conducted in the absence of any commercial or financial relationships that could be construed as a potential conflict of interest.
Publisher’s note
All claims expressed in this article are solely those of the authors and do not necessarily represent those of their affiliated organizations, or those of the publisher, the editors and the reviewers. Any product that may be evaluated in this article, or claim that may be made by its manufacturer, is not guaranteed or endorsed by the publisher.
References
Abdelkrim S., Jebara S. H., Saadani O., Chiboub M., Abid G., Mannai K., et al. (2019). Heavy metal accumulation in Lathyrus sativus growing in contaminated soils and identification of symbiotic resistant bacteria. Arch. Microbiol. 201, 107–121. doi: 10.1007/s00203-018-1581-4
Aggarwal A., Ezaki B., Munjal A., Tripathi B. N. (2015). “Physiology and biochemistry of aluminum toxicity and tolerance in crops,” in Stress Responses in Plants: Mechanisms of Toxicity and Tolerance (Cham, Switzerland: Springer Cham), 35–57. doi: 10.1007/978-3-319-13368-3_2
Alves P. R. L., Natal-da-Luz T., Sousa J. P., Cardoso E. J. (2015). Ecotoxicological characterization of sugarcane vinasses when applied to tropical soils. Sci. Total Environ. 526, 222–232. doi: 10.1016/j.scitotenv.2015.03.150
Aprile F., Lorandi R. (2012). Evaluation of cation exchange capacity (CEC) in tropical soils using four different analytical methods. J. Agric. Sci. 4, 278. doi: 10.5539/jas.v4n6p278
Ashraf S., Ali Q., Zahir Z. A., Ashraf S., Asghar H. N. (2019). Phytoremediation: Environmentally sustainable way for reclamation of heavy metal polluted soils. Ecotoxicology Environ. Saf. 174, 714–727. doi: 10.1016/j.ecoenv.2019.02.068
Awad M., El-Desoky M. A., Ghallab A., Kubes J., Abdel-Mawly S. E., Danish S., et al. (2021). Ornamental plant efficiency for heavy metals phytoextraction from contaminated soils amended with organic materials. Molecules 26, 3360. doi: 10.3390/molecules26113360
Baeyens J., Kang Q., Appels L., Dewil R., Lv Y., Tan T. (2015). Challenges and opportunities in improving the production of bio-ethanol. Prog. Energy Combustion Sci. 47, 60–88. doi: 10.1016/j.pecs.2014.10.003
Balat M., Balat H. (2009). Recent trends in global production and utilization of bio-ethanol fuel. Appl. Energy 86, 2273–2282. doi: 10.1016/j.apenergy.2009.03.015
Bauder J. W., Brock T. A. (2001). Irrigation water quality, soil amendment, and crop effects on sodium leaching. Arid Land Res. Manage. 15, 101–113. doi: 10.1080/15324980151062724
Behzadipour H., Sadrekarimi A. (2021). Biochar-assisted bio-cementation of a sand using native bacteria. Bull. Eng. Geology Environ. 80, 4967–4984. doi: 10.1007/s10064-021-02235-0
Bento L. R., Castro A. J. R., Moreira A. B., Ferreira O. P., Bisinoti M. C., Melo C. A. (2019). Release of nutrients and organic carbon in different soil types from hydrochar obtained using sugarcane bagasse and vinasse. Geoderma 334, 24–32. doi: 10.1016/j.geoderma.2018.07.034
Bi S., Luo X., Zhang C., Li P., Yu C., Liu Z., et al. (2023). Fate of fertilizer nitrogen and residual nitrogen in paddy soil in Northeast China. J. Integr. Agric. 22, 3535–3548. doi: 10.1016/j.jia.2023.06.010
Bojórquez-Quintal E., Escalante-Magaña C., Echevarría-MaChado I., Martínez-Estévez M. (2017). Aluminum, a friend or foe of higher plants in acid soils. Front. Plant Sci. 8. doi: 10.3389/fpls.2017.01767
Bordonal R. D. O., Carvalho J. L. N., Lal R., de Figueiredo E. B., de Oliveira B. G., La Scala N. (2018). Sustainability of sugarcane production in Brazil. A review. Agron. Sustain. Dev. 38, 13. doi: 10.1007/s13593-018-0490-x
Buller L. S., da Silva Romero C. W., Lamparelli R. A. C., Ferreira S. F., Bortoleto A. P., Mussatto S. I., et al. (2021). A spatially explicit assessment of sugarcane vinasse as a sustainable by-product. Sci. Total Environ. 765, 142717. doi: 10.1016/j.scitotenv.2020.142717
Calvin Y. L., Hariyanto P. A. T., Usman A. I., Masuku M., Wibowo C. S., Anggarani R., et al. (2022). Volatility and physicochemical properties of gasoline-ethanol blends with gasoline RON-based 88, 90, and 92. Fuel 307, 121850. doi: 10.1016/j.fuel.2021.121850
Cardin C. A., Santos C. H. D., Escarmínio M. A. (2016). Impacts of vinasse and methods of sugarcane harvesting on the availability of K and carbon stock of an Argisol. Rev. Ceres 63, 95–102. doi: 10.1590/0034-737X201663010013
Carpanez T. G., Moreira V. R., Assis I. R., Amaral M. C. S. (2022). Sugarcane vinasse as organo-mineral fertilizers feedstock: Opportunities and environmental risks. Sci. Total Environ. 832, 154998. doi: 10.1016/j.scitotenv.2022.154998
Chaganti V. N., Culman S. W., Herms C., Sprunger C. D., Brock C., Leiva Soto A., et al. (2021). Base cation saturation ratios, soil health, and yield in organic field crops. Agron. J. 113, 4190–4200. doi: 10.1002/agj2.20785
Chauvel A., Grimaldi M., Tessier D. (1991). Changes in soil pore-space distribution following deforestation and revegetation: an example from the Central Amazon Basin, Brazil. For. Ecol. Manage. 38, 259–271. doi: 10.1016/0378-1127(91)90147-N
Cherubin M. R., Carvalho J. L., Cerri C. E., Nogueira L. A., Souza G. M., Cantarella H. (2020). Land use and management effects on sustainable sugarcane-derived bioenergy. Land 10, 72. doi: 10.3390/land10010072
Christodoulou A., Stamatelatou K. (2016). Overview of legislation on sewage sludge management in developed countries worldwide. Water Sci. Technol. 73, 453–462. doi: 10.2166/wst.2015.521
Christofoletti C. A., Escher J. P., Correia J. E., Marinho J. F. U., Fontanetti C. S. (2013). Sugarcane vinasse: environmental implications of its use. Waste Manage. 33, 2752–2761. doi: 10.1016/j.wasman.2013.09.005
Chuppa-Tostain G., Hoarau J., Watson M., Adelard L., Shum Cheong Sing A., Caro Y., et al. (2018). Production of Aspergillus Niger biomass on sugarcane distillery wastewater: physiological aspects and potential for biodiesel production. Fungal Biol. Biotechnol. 5, 1–12. doi: 10.1186/s40694-018-0045-6
Companhia Ambiental do Estado de São Paulo (CETESB) (2006). “Vinhaça: critérios e procedimentos para aplicação no solo agrícola,” in Norma Técnica P4.231(São Paulo: Companhia Ambiental do Estado de São Paulo). Available at: https://cetesb.sp.gov.br/wp-content/uploads/2013/11/P4.231_Vinhaca_-Criterios-e-procedimentos-para-aplicacao-no-solo-agricola-3a-Ed-2a-VERSAO.pdf. Visited on 8th September 2023.
Corwin D. L., Plant R. E. (2005). Applications of apparent soil electrical conductivity in precision agriculture. Comput. Electron. Agric. 46, 1–10. doi: 10.1016/j.compag.2004.10.004
Cui X., Guo L., Li C., Liu M., Wu G., Jiang G. (2021). The total biomass nitrogen reservoir and its potential of replacing chemical fertilizers in China. Renewable Sustain. Energy Rev. 135, 110215. doi: 10.1016/j.rser.2020.110215
Culman S. W., Brock C., Doohan D., Jackson-Smith D., Herms C., Chaganti V. N., et al. (2021). Base cation saturation ratios vs. sufficiency level of nutrients: A false dichotomy in practice. Agron. J. 113, 5623–5634. doi: 10.1002/agj2.20787
da Silva J. J., da Silva B. F., Stradiotto N. R., Petrović M., Gros M., Gago-Ferrero P. (2021). Identification of organic contaminants in vinasse and in soil and groundwater from fertigated sugarcane crop areas using target and suspect screening strategies. Sci. Total Environ. 761, 143237. doi: 10.1016/j.scitotenv.2020.143237
De Figueiredo E. B., Panosso A. R., Romão R., La Scala N. (2010). Greenhouse gas emission associated with sugar production in southern Brazil. Carbon balance Manage. 5, 1–7. doi: 10.1186/1750-0680-5-3
De Godoi L. A. G., Camiloti P. R., Bernardes A. N., Sanchez B. L. S., Torres A. P. R., da Conceição Gomes A., et al. (2019). Seasonal variation of the organic and inorganic composition of sugarcane vinasse: main implications for its environmental uses. Environ. Sci. pollut. Res. 26, 29267–29282. doi: 10.1007/s11356-019-06019-8
Delcour I., Spanoghe P., Uyttendaele M. (2015). Literature review: Impact of climate change on pesticide use. Food Res. Int. 68, 7–15. doi: 10.1016/j.foodres.2014.09.030
de Souza Oliveira R. P., Rivas Torres B., Zilli M., de Araújo Viana Marques D., Basso L. C., Converti A. (2009). Use of sugar cane vinasse to mitigate aluminum toxicity to Saccharomyces cerevisiae. Arch. Environ. Contamination Toxicol. 57, 488–494. doi: 10.1007/s00244-009-9287-x
de Souza Oliveira Filho J., dos Santos O. A. Q., Rossi C. Q., Diniz Y. V. D. F. G., de Souza Fagundes H., Pinto L. A. D. S. R., et al. (2021). Assessing the effects of harvesting with and without burning and vinasse application in sugarcane crops: evaluation of soil fertility and phosphorus pools in different ethanol production systems. Agriculture Ecosyst. Environ. 307, 107233. doi: 10.1016/j.agee.2020.107233
Dhote L., Kumar S., Singh L., Kumar R. (2021). A systematic review on options for sustainable treatment and resource recovery of distillery sludge. Chemosphere 263, 128225. doi: 10.1016/j.chemosphere.2020.128225
Di Gioia F., Ozores-Hampton M., Zhao X., Thomas J., Wilson P., Li Z., et al. (2017). Anaerobic soil disinfestation impact on soil nutrients dynamics and nitrous oxide emissions in fresh-market tomato. Agriculture Ecosyst. Environ. 240, 194–205. doi: 10.1016/j.agee.2017.02.025
Do Nascimento M., Battaglia M. E., Rizza L. S., Ambrosio R., Di Palma A. A., Curatti L. (2019). Prospects of using biomass of N2-fixing cyanobacteria as an organic fertilizer and soil conditioner. Algal Res. 43, 101652. doi: 10.1016/j.algal.2019.101652
Dos Santos O. A. Q., Tavares O. C. H., García A. C., Rossi C. Q., de Moura O. V. T., Pereira W., et al. (2020). Fire lead to disturbance on organic carbon under sugarcane cultivation but is recovered by amendment with vinasse. Sci. Total Environ. 739, 140063. doi: 10.1016/j.scitotenv.2020.140063
Dotaniya M. L., Datta S. C., Biswas D. R., Dotaniya C. K., Meena B. L., Rajendiran S., et al. (2016). Use of sugarcane industrial by-products for improving sugarcane productivity and soil health. Int. J. Recycling Organic Waste Agric. 5, 185–194. doi: 10.1007/s40093-016-0132-8
Durrer A., Gumiere T., Taketani R. G., da Costa D. P., e Silva M. D. C. P., Andreote F. D. (2017). The drivers underlying biogeographical patterns of bacterial communities in soils under sugarcane cultivation. Appl. Soil Ecol. 110, 12–20. doi: 10.1016/j.apsoil.2016.11.005
Eissa M. A. (2017). Phytoextraction mechanism of Cd by Atriplex lentiformis using some mobilizing agents. Ecol. Eng. 108, 220–226. doi: 10.1016/j.ecoleng.2017.08.025
Engström L., Stenberg M., Wallenhammar A. C., Ståhl P., Gruvaeus I. (2014). Organic winter oilseed rape response to N fertilisation and preceding agroecosystem. Field Crops Res. 167, 94–101. doi: 10.1016/j.fcr.2014.07.011
FAOSTAT (2024)United Nation data. Available online at: https://data.un.org/Data.aspx?d=FAOandf=itemCode%3A75 (Accessed 4th February 2024).
Farid H., Hakimian F., Nair V., Nair P. K., Ismail N. (2016). Trend of research on sustainable tourism and climate change in 21st century. Worldwide Hospitality Tourism Themes 8 (5), 516-533. doi: 10.1108/WHATT-06-2016-0032
Feder F. (2021). Effects of fertilisation using organic waste products with mineral complementation on sugarcane yields and soil properties in a 4 year field experiment. Agriculture 11, 985. doi: 10.3390/agriculture11100985
Ferronato N., Torretta V. (2019). Waste mismanagement in developing countries: A review of global issues. Int. J. Environ. Res. Public Health 16, 1060. doi: 10.3390/ijerph16061060
Franzluebbers A. J. (1999). Microbial activity in response to water-filled pore space of variably eroded southern Piedmont soils. Appl. Soil Ecol. 11, 91–101. doi: 10.1016/S0929-1393(98)00128-0
Friedrichsen C. N., Hagen-Zakarison S., Friesen M. L., McFarland C. R., Tao H., Wulfhorst J. D. (2021). Soil health and well-being: redefining soil health based upon a plurality of values. Soil Secur. 2, 100004. doi: 10.1016/j.soisec.2021.100004
Fuess L. T., Altoé M. E., Felipe M. C., Garcia M. L. (2021). Pros and cons of fertirrigation with in natura sugarcane vinasse: do improvements in soil fertility offset environmental and bioenergy losses? J. Cleaner Production 319, 128684. doi: 10.1016/j.jclepro.2021.128684
Fuess L. T., Garcia M. L., Zaiat M. (2018). Seasonal characterization of sugarcane vinasse: Assessing environmental impacts from fertirrigation and the bioenergy recovery potential through biodigestion. Sci. Total Environ. 634, 29–40. doi: 10.1016/j.scitotenv.2018.03.326
Garbowski T., Bar-Michalczyk D., Charazińska S., Grabowska-Polanowska B., Kowalczyk A., Lochyński P. (2023). An overview of natural soil amendments in agriculture. Soil Tillage Res. 225, 105462. doi: 10.1016/j.still.2022.105462
González M., Gomez E., Comese R., Quesada M., Conti M. (2010). Influence of organic amendments on soil quality potential indicators in an urban horticultural system. Bioresource Technol. 101, 8897–8901. doi: 10.1016/j.biortech.2010.06.095
Gopal A. R., Kammen D. M. (2009). Molasses for ethanol: the economic and environmental impacts of a new pathway for the lifecycle greenhouse gas analysis of sugarcane ethanol. Environ. Res. Lett. 4, 44005. doi: 10.1088/1748-9326/4/4/044005
Goswami V., Deepika S., Chandra R., Babu C. R., Kothamasi D. (2023). Arbuscular mycorrhizas accelerate the degradation of colour containing organic pollutants present in distillery spent wash leachates. J. Hazardous Materials 452, 131291. doi: 10.1016/j.jhazmat.2023.131291
Gowd S. C., Kumar D., Lin R., Rajendran K. (2022). Nutrient recovery from wastewater in India: A perspective from mass and energy balance for a sustainable circular economy. Bioresource Technol. Rep. 18, 101079. doi: 10.1016/j.biteb.2022.101079
Gurwick N. P., Moore L. A., Kelly C., Elias P. (2013). A systematic review of biochar research, with a focus on its stability in situ and its promise as a climate mitigation strategy. PloS One 8, e75932. doi: 10.1371/journal.pone.0075932
Gutiérrez M. A., Zúñiga O., Ospina-Salazar D. I. (2016). Effect of three biowastes on the productivity potential of a sodic soil. Agronomía Colombiana 34, 250–259. doi: 10.15446/agron.colomb.v34n2.55044
Hassan M. U., Aamer M., Umer Chattha M., Haiying T., Khan I., Seleiman M. F., et al. (2021). Sugarcane distillery spent wash (dsw) as a bio-nutrient supplement: a win-win option for sustainable crop production. Agronomy 11, 183. doi: 10.3390/agronomy11010183
Hoarau J., Caro Y., Grondin I., Petit T. (2018). Sugarcane vinasse processing: Toward a status shift from waste to valuable resource. A review. J. Water Process Eng. 24, 11–25. doi: 10.1016/j.jwpe.2018.05.003
Horrigan L., Lawrence R. S., Walker P. (2002). How sustainable agriculture can address the environmental and human health harms of industrial agriculture. Environ. Health Perspect. 110, 445–456. doi: 10.1289/ehp.02110445
Hossain M. Z., von Fragstein P., Heß J., Heß J. (2016). Plant origin wastes as soil conditioner and organic fertilizer: a review. J. Agricultural Food Environ. Sci. 16, 1362–1371. doi: 10.5829/idosi.aejaes.2016.16.7.12961
Iloms E., Ololade O. O., Ogola H. J., Selvarajan R. (2020). Investigating industrial effluent impact on municipal wastewater treatment plant in Vaal, South Africa. Int. J. Environ. Res. Public Health 17, 1096. doi: 10.3390/ijerph17031096
Jamir L., Kumar V., Kaur J., Kumar S., Singh H. (2021). Composition, valorization and therapeutical potential of molasses: a critical review. Environ. Technol. Rev. 10, 131–142. doi: 10.1080/21622515.2021.1892203
Jayashree R., Sharmi M., Priyanka S. A. (2022). Biomethanated distillery spentwash application and its impact on soil health, growth and yield of Elephant foot yam. Environ. Conserv. J. 23, 49–54. doi: 10.36953/ECJ.2022.2312
Jia Y., Hu Z., Ba Y., Qi W. (2021). Application of biochar-coated urea controlled loss of fertilizer nitrogen and increased nitrogen use efficiency. Chem. Biol. Technol. Agric 8 (3), 1-11. doi: 10.1186/s40538-020-00205-4
Kaziūnienė J., Mažylytė R., Krasauskas A., Toleikienė M., Gegeckas A. (2022). Optimizing the growth conditions of the selected plant-growth-promoting Rhizobacteria Paenibacillus sp. MVY-024 for industrial scale production. Biology 11, 745. doi: 10.3390/biology11050745
Khan N., Bano A. M., Babar A. (2020). Impacts of plant growth promoters and plant growth regulators on rainfed agriculture. PloS One 15, e0231426. doi: 10.1371/journal.pone.0231426
Kihara J., Nziguheba G., Zingore S., Coulibaly A., Esilaba A., Kabambe V., et al. (2016). Understanding variability in crop response to fertilizer and amendments in sub-Saharan Africa. Agriculture Ecosyst. Environ. 229, 1–12. doi: 10.1016/j.agee.2016.05.012
Kopittke P. M. (2012). Interactions between Ca, Mg, Na and K: alleviation of toxicity in saline solutions. Plant Soil 352, 353–362. doi: 10.1007/s11104-011-1001-x
Kumar V., Chopra A. K. (2014). Ferti-irrigational impact of sugar mill effluent on agronomical characteristics of Phaseolus vulgaris (L.) in two seasons. Environ. Monit. Assess. 186, 7877–7892. doi: 10.1007/s10661-014-3974-4
Kumari S., Jose S., Tyagi M., Jagadevan S. (2020). A holistic and sustainable approach for recovery of phosphorus via struvite crystallization from synthetic distillery wastewater. J. Cleaner Production 254, 120037. doi: 10.1016/j.jclepro.2020.120037
Lee C. G., Kunitomo E., Iida T., Nakaho K., Ohkuma M. (2020). Soil prokaryotes are associated with decreasing Fusarium oxysporum density during anaerobic soil disinfestation in the tomato field. Appl. Soil Ecol. 155, 103632. doi: 10.1016/j.apsoil.2020.103632
Leogrande R., Vitti C. (2019). Use of organic amendments to reclaim saline and sodic soils: a review. Arid Land Res. Manage. 33, 1–21. doi: 10.1080/15324982.2018.1498038
Li S., Zhao X., Ye X., Zhang L., Shi L., Xu F., et al. (2020). The effects of condensed molasses soluble on the growth and development of rapeseed through seed germination, hydroponics and field trials. Agriculture 10, 260. doi: 10.3390/agriculture10070260
Litardo R. C. M., Bendezú S. J. G., Zenteno M. D. C., Pérez-Almeida I. B., Parismoreno L. L., García E. D. L. (2022). Effect of mineral and organic amendments on rice growth and yield in saline soils. J. Saudi Soc. Agric. Sci. 21, 29–37. doi: 10.1016/j.jssas.2021.06.015
Lourenço K. S., de Assis Costa O. Y., Cantarella H., Kuramae E. E. (2022). Ammonia-oxidizing bacteria and fungal denitrifier diversity are associated with N2O production in tropical soils. Soil Biol. Biochem. 166, 108563. doi: 10.1016/j.soilbio.2022.108563
Macusi E. D., Estor D. E. P., Borazon E. Q., Clapano M. B., Santos M. D. (2022). Environmental and socioeconomic impacts of shrimp farming in the Philippines: A critical analysis using PRISMA. Sustainability 14, 2977. doi: 10.3390/su14052977
Mączyńska J., Krzywonos M., Kupczyk A., Tucki K., Sikora M., Pińkowska H., et al. (2019). Production and use of biofuels for transport in Poland and Brazil–The case of bioethanol. Fuel 241, 989–996. doi: 10.1016/j.fuel.2018.12.116
Manochio C., Andrade B. R., Rodriguez R. P., Moraes B. S. (2017). Ethanol from biomass: A comparative overview. Renewable and Sustainable Energy Reviews 80, 743–755. doi: 10.1016/j.rser.2017.05.063
Mikucka W., Zielińska M. (2020). Distillery stillage: characteristics, treatment, and valorization. Appl. Biochem. Biotechnol. 192, 770–793. doi: 10.1007/s12010-020-03343-5
Mohanty M., Sinha N. K., Sammi Reddy K., Chaudhary R. S., Subba Rao A., Dalal R. C., et al. (2013). How important is the quality of organic amendments in relation to mineral N availability in soils? Agric. Res. 2, 99–110. doi: 10.1007/s40003-013-0052-z
Moraes B. S., Junqueira T. L., Pavanello L. G., Cavalett O., Mantelatto P. E., Bonomi A., et al. (2014). Anaerobic digestion of vinasse from sugarcane biorefineries in Brazil from energy, environmental, and economic perspectives: Profit or expense? Appl. Energy 113, 825–835. doi: 10.1016/j.apenergy.2013.07.018
Moran-Salazar R. G., Sanchez-Lizarraga A. L., Rodriguez-Campos J., Davila-Vazquez G., Marino-Marmolejo E. N., Dendooven L., et al. (2016). Utilization of vinasses as soil amendment: consequences and perspectives. SpringerPlus 5, 1007. doi: 10.1186/s40064-016-2410-3
Mordenti A. L., Giaretta E., Campidonico L., Parazza P., Formigoni A. (2021). A review regarding the use of molasses in animal nutrition. Animals 11, 115. doi: 10.3390/ani11010115
Nathaniel O., Sam A. R. M., Lim N. H. A. S., Adebisi O., Abdulkareem M. (2020). Biogenic approach for concrete durability and sustainability using effective microorganisms: A review. Construction Building Materials 261, 119664. doi: 10.1016/j.conbuildmat.2020.119664
Navarrete A. A., Diniz T. R., Braga L. P. P., Silva G. G. Z., Franchini J. C., Rossetto R., et al. (2015). Multi-analytical approach reveals potential microbial indicators in soil for sugarcane model systems. PloS One 10, e0129765. doi: 10.1371/journal.pone.0129765
Naveed S., Rehim A., Imran M., Anwar M. F., Hussain S. (2018). Effect of distillery spentwash fertigation on crop growth, yield, and accumulation of potentially toxic elements in rice. Environ. Sci. pollut. Res. 25, 31113–31124. doi: 10.1007/s11356-018-3067-y
Nguyen T. L. T., Gheewala S. H., Garivait S. (2008). Full chain energy analysis of fuel ethanol from cane molasses in Thailand. Appl. Energy 85, 722–734. doi: 10.1016/j.apenergy.2008.02.002
Nikseresht F., Landi A., Sayyad G., Ghezelbash G. R., Schulin R. (2020). Sugarecane molasse and vinasse added as microbial growth substrates increase calcium carbonate content, surface stability and resistance against wind erosion of desert soils. J. Environ. Manage. 268, 110639. doi: 10.1016/j.jenvman.2020.110639
Nunes M. R., Veum K. S., Parker P. A., Holan S. H., Karlen D. L., Amsili J. P., et al. (2021). The soil health assessment protocol and evaluation applied to soil organic carbon. Soil Sci. Soc. America J. 85, 1196–1213. doi: 10.1002/saj2.20244
Oak R. S., Sarode D. D., Joshi J. B., Chavan S. A. (2021). Impact of co-application of biomethanated spent wash with bio-char on soil micronutrients and crop growth. J. Sugarcane Res. 11, 99–109. doi: 10.37580/JSR.2021.1.11.99-109
Oceguera-Contreras E., Aguilar-Juárez O., Oseguera-Galindo D., Macías-Barragán J., Bolaños-Rosales R., Mena-Enríquez M., et al. (2019). Biohydrogen production by vermihumus-associated microorganisms using agro industrial wastes as substrate. Int. J. Hydrogen Energy 44, 9856–9865. doi: 10.1016/j.ijhydene.2018.10.236
Ogbodo E. N. (2013). Impact of the use of inorganic fertilizers to the soils of the Ebonyi State Agro-Ecology, South-Eastern Nigeria. J. Environ. Earth Sci. 3, 33–38. https://api.semanticscholar.org/CorpusID:54945375
Ogemdi I. K., Gold E. E. (2018). Physico-chemical parameters of industrial effluents from a brewery industry in Imo state, Nigeria. Advanced J. Chem. 1, 66–78. doi: 10.29088/sami/AJCA.2018.1.6678
Oliveira Filho J. D. S., Pereira M. G. (2020). Analyzing the research on phosphorus fractions and phosphorus legacy in soil: a bibliometric analysis. J. Soils Sediments 20, 3394–3405. doi: 10.1007/s11368-020-02669-1
Omori W. P., Camargo A. F. D., Goulart K. C. S., Lemos E. G. D. M., Souza J. A. M. D. (2016). Influence of vinasse application in the structure and composition of the bacterial community of the soil under sugarcane cultivation. Int. J. Microbiol. 2016, 1-11. doi: 10.1155/2016/2349514
Onu M. A., Ayeleru O. O., Oboirien B., Olubambi P. A. (2023). Challenges of wastewater generation and management in sub-Saharan Africa: A Review. Environ. Challenges 11, 100686. doi: 10.1016/j.envc.2023.100686
Ortegón G. P., Arboleda F. M., Candela L., Tamoh K., Valdes-Abellan J. (2016). Vinasse application to sugar cane fields. Effect on the unsaturated zone and groundwater at Valle del Cauca (Colombia). Sci. Total Environ. 539, 410–419. doi: 10.1016/j.scitotenv.2015.08.153
Pahlevan-Sharif S., Mura P., Wijesinghe S. N. (2019). A systematic review of systematic reviews in tourism. J. Hospitality Tourism Manage. 39, 158–165. doi: 10.1016/j.jhtm.2019.04.001
Palmonari A., Cavallini D., Sniffen C. J., Fernandes L., Holder P., Fagioli L., et al. (2020). Characterization of molasses chemical composition. J. Dairy Sci. 103, 6244–6249. doi: 10.3168/jds.2019-17644
Pinto L. E., Cordeiro C. F. D. S., de Araujo A. S., Araujo F. F. D. (2022). Vinasse improves soil quality and increases the yields of soybean, maize, and pasture. Rev. Bras. Engenharia Agrícola e Ambiental 26, 335–340. doi: 10.1590/1807-1929/agriambi.v26n5p335-340
Pires J. F., Ferreira G. M., Reis K. C., Schwan R. F., Silva C. F. (2016). Mixed yeasts inocula for simultaneous production of SCP and treatment of vinasse to reduce soil and fresh water pollution. J. Environ. Manage. 182, 455–463. doi: 10.1016/j.jenvman.2016.08.006
Place G., Bowman D., Burton M., Rufty T. (2008). Root penetration through a high bulk density soil layer: differential response of a crop and weed species. Plant Soil 307, 179–190. doi: 10.1007/s11104-008-9594-4
Prashar P., Shah S. (2016). Impact of fertilizers and pesticides on soil microflora in agriculture. Sustain. Agric. Rev. 19, 331–361. doi: 10.1007/978-3-319-26777-7_8
Pyakurel A., Dahal B. R., Rijal S. (2019). Effect of molasses and organic fertilizer in soil fertility and yield of spinach in Khotang, Nepal. Int. J. Appl. Sci. Biotechnol. 7, 49–53. doi: 10.3126/ijasbt.v7i1.23301
Quintero J. A., Cardona C. A., Felix E., Moncada J., Sánchez Ó.J., Gutiérrez L. F. (2012). Techno-economic analysis of bioethanol production in Africa: Tanzania case. Energy 48, 442–454. doi: 10.1016/j.energy.2012.10.018
Rahimi H., Pazira E., Tajik F. (2000). Effect of soil organic matter, electrical conductivity and sodium adsorption ratio on tensile strength of aggregates. Soil Tillage Res. 54, 145–153. doi: 10.1016/S0167-1987(00)00086-6
Rahman M. A., Lee S. H., Ji H. C., Kabir A. H., Jones C. S., Lee K. W. (2018). Importance of mineral nutrition for mitigating aluminum toxicity in plants on acidic soils: current status and opportunities. Int. J. Mol. Sci. 19, 3073. doi: 10.3390/ijms19103073
Ratna S., Rastogi S., Kumar R. (2021). Current trends for distillery wastewater management and its emerging applications for sustainable environment. J. Environ. Manage. 290, 112544. doi: 10.1016/j.jenvman.2021.112544
Raza Q. U. A., Bashir M. A., Rehim A., Raza H. M. A. (2022). Role of sugarcane industrial byproducts on soil physicochemical properties and metal accumulation in rice. Environ. Sci. pollut. Res. 29, 24726–24736. doi: 10.1007/s11356-021-17428-z
Raza Q. U. A., Bashir M. A., Rehim A., Sial M. U., Ali Raza H. M., Atif H. M., et al. (2021). Sugarcane industrial byproducts as challenges to environmental safety and their remedies: A review. Water 13, 3495. doi: 10.3390/w13243495
Reyes-Cabrera J., Leon R. G., Erickson J. E., Rowland D. L., Silveira M. L., Morgan K. T. (2017). Differences in biomass and water dynamics between a cotton-peanut rotation and a sweet sorghum bioenergy crop with and without biochar and vinasse as soil amendments. Field Crops Res. 214, 123–130. doi: 10.1016/j.fcr.2017.09.012
Romano I., Ventorino V., Ambrosino P., Testa A., Chouyia F. E., Pepe O. (2020). Development and application of low-cost and eco-sustainable bio-stimulant containing a new plant growth-promoting strain Kosakonia pseudosacchari TL13. Front. Microbiol. 11. doi: 10.3389/fmicb.2020.02044
Rothe M., Darnaudery M., Thuriès L. (2019). Organic fertilizers, green manures and mixtures of the two revealed their potential as substitutes for inorganic fertilizers used in pineapple cropping. Scientia Hortic. 257, 108691. doi: 10.1016/j.scienta.2019.108691
Sadiq M., Mazhar U., Shah G. A., Hassan Z., Iqbal Z., Mahmood I., et al. (2021). Zinc plus biopolymer coating slows nitrogen release, decreases ammonia volatilization from urea and improves sunflower productivity. Polymers 13, 3170. doi: 10.3390/polym13183170
Saeed Q., Xiukang W., Haider F. U., Kučerik J., Mumtaz M. Z., Holatko J., et al. (2021). Rhizosphere bacteria in plant growth promotion, biocontrol, and bioremediation of contaminated sites: A comprehensive review of effects and mechanisms. Int. J. Mol. Sci. 22, 10529. doi: 10.3390/ijms221910529
Sakthivel P., Subramanian K. A., Mathai R. (2018). Indian scenario of ethanol fuel and its utilization in automotive transportation sector. Resources Conserv. Recycling 132, 102–120. doi: 10.1016/j.resconrec.2018.01.012
Saneiyan S., Ntarlagiannis D., Colwell F. (2021). Complex conductivity signatures of microbial induced calcite precipitation, field and laboratory scales. Geophysical J. Int. 224, 1811–1824. doi: 10.1093/gji/ggaa510
Saneiyan S., Ntarlagiannis D., Ohan J., Lee J., Colwell F., Burns S. (2019). Induced polarization as a monitoring tool for in-situ microbial induced carbonate precipitation (MICP) processes. Ecol. Eng. 127, 36–47. doi: 10.1016/j.ecoleng.2018.11.010
Savci S. (2012). Investigation of effect of chemical fertilizers on environment. Apcbee Proc. 1, 287–292. doi: 10.1016/j.apcbee.2012.03.047
Sayer J., Cassman K. G. (2013). Agricultural innovation to protect the environment. Proc. Natl. Acad. Sci. 110, 8345–8348. doi: 10.1073/pnas.1208054110
Scarpare F. V., Hernandes T. A. D., Ruiz-Corrêa S. T., Kolln O. T., de Castro Gava G. J., dos Santos L. N. S., et al. (2016). Sugarcane water footprint under different management practices in Brazil: Tietê/Jacaré watershed assessment. J. Cleaner Production 112, 4576–4584. doi: 10.1016/j.jclepro.2015.05.107
Seleem M., Khalafallah N., Zuhair R., Ghoneim A. M., El-Sharkawy M., Mahmoud E. (2022). Effect of integration of poultry manure and vinasse on the abundance and diversity of soil fauna, soil fertility index, and barley (Hordeum aestivum L.) growth in calcareous soils. BMC Plant Biol. 22, 492. doi: 10.1186/s12870-022-03881-6
Sellare J., Meemken E. M., Qaim M. (2020). Fairtrade, agrochemical input use, and effects on human health and the environment. Ecol. Economics 176, 106718. doi: 10.1016/j.ecolecon.2020.106718
Shelar M. N., Matsagar V. K., Patil V. S., Barahate S. D. (2023). Net energy analysis of sugarcane based ethanol production. Cleaner Energy Syst. 4, 100059. doi: 10.1016/j.cles.2023.100059
Shivaraj D., Devidas T. (2017). Growth and Performance of Indian Sugar Industry: special reference to Karnataka Sugar Industry. Int. J. Curr. Trends Sci. Technol. 8. https://api.semanticscholar.org/CorpusID:169516318
Shrestha U., Augé R. M., Butler D. M. (2016). A meta-analysis of the impact of anaerobic soil disinfestation on pest suppression and yield of horticultural crops. Front. Plant Sci. 7. doi: 10.3389/fpls.2016.01254
Singh J. S., Pandey V. C., Singh D. P. (2011). Efficient soil microorganisms: a new dimension for sustainable agriculture and environmental development. Agriculture Ecosyst. Environ. 140, 339–353. doi: 10.1016/j.agee.2011.01.017
Singh K., Tripathi S., Chandra R. (2021). Maillard reaction product and its complexation with environmental pollutants: A comprehensive review of their synthesis and impact. Bioresource Technol. Rep. 15, 100779. doi: 10.1016/j.biteb.2021.100779
Solomon S. (2011). Sugarcane by-products based industries in India. Sugar Tech 13, 408–416. doi: 10.1007/s12355-011-0114-0
Suleiman A. K. A., Lourenco K. S., Pitombo L. M., Mendes L. W., Roesch L. F. W., Pijl A., et al. (2018). Recycling organic residues in agriculture impacts soil-borne microbial community structure, function and N2O emissions. Sci. Total Environ. 631, 1089–1099. doi: 10.1016/j.scitotenv.2018.03.116
Surendran U., Ramesh V., Jayakumar M., Marimuthu S., Sridevi G. (2016). Improved sugarcane productivity with tillage and trash management practices in semi arid tropical agro ecosystem in India. Soil Tillage Res. 158, 10–21. doi: 10.1016/j.still.2015.10.009
Tilman D., Clark M. (2015). Food, agriculture and the environment: Can we feed the world and save the earth? Daedalus 144, 8–23. doi: 10.1162/DAED_a_00350
Tlatlaa J. S., Tryphone G. M., Nassary E. K. (2023). Unexplored agronomic, socioeconomic and policy domains for sustainable cotton production on small landholdings: A systematic review. Front. Agron. 5. doi: 10.3389/fagro.2023.1281043
Tobe K., Zhang L., Omasa K. (2003). Alleviatory effects of calcium on the toxicity of sodium, potassium and magnesium chlorides to seed germination in three non-halophytes. Seed Sci. Res. 13, 47–54. doi: 10.1079/SSR2002123
United States Environmental Protection Agency. (2004). “Guidelines for water reuse,” in EPA/625/R-04/108 (Washington, DC.: United States Environmental Protection Agency). Available at: https://www.epa.gov/sites/default/files/2019-08/documents/2004-guidelines-water-reuse.pdf.
Vandenberghe L., Valladares-Diestra K., Bittencourt G., Zevallos Torres L., Vieira S., Karp S., et al. (2022). Beyond sugar and ethanol: The future of sugarcane biorefineries in Brazil. Renewable Sustain. Energy Rev. 167, 112721. doi: 10.1016/j.rser.2022.112721
Van Vuuren D. P., Bouwman A. F., Beusen A. H. (2010). Phosphorus demand for the 1970–2100 period: a scenario analysis of resource depletion. Global Environ. Change 20, 428–439. doi: 10.1016/j.gloenvcha.2010.04.004
Wang H., Wang T., Zhang B., Li F., Toure B., Omosa I. B., et al. (2014). Water and wastewater treatment in Africa–current practices and challenges. CLEAN–Soil Air Water 42, 1029–1035. doi: 10.1002/clen.201300208
Wei Y. A., Xu Y. J. (2004). Eco-friendly management of sugar industry effluents in Guangxi, China. Sugar Tech 6, 285–290. doi: 10.1007/BF02942510
Wester-Larsen L., Müller-Stöver D. S., Salo T., Jensen L. S. (2022). Potential ammonia volatilization from 39 different novel bio-based fertilizers on the European market–a laboratory study using 5 European soils. J. Environ. Manage. 323, 116249. doi: 10.1016/j.jenvman.2022.116249
Wright A. G., Ellis T. P., Ilag L. L. (2014). Filtered molasses concentrate from sugar cane: natural functional ingredient effective in lowering the glycaemic index and insulin response of high carbohydrate foods. Plant Foods Hum. Nutr. 69, 310–316. doi: 10.1007/s11130-014-0446-5
Zamarreño A. M., Valduga G., Garcia-Mina J. M. (2022). Cane vinasses contain bioactive concentrations of auxin and abscisic acid in their composition. Int. J. Mol. Sci. 23, 9976. doi: 10.3390/ijms23179976
Zani C. F., Barneze A. S., Robertson A. D., Keith A. M., Cerri C. E., McNamara N. P., et al. (2018). Vinasse application and cessation of burning in sugarcane management can have positive impact on soil carbon stocks. PeerJ 6, e5398. doi: 10.7717/peerj.5398
Zhao J., Neher D. A., Fu S., Li Z. A., Wang K. (2013). Non-target effects of herbicides on soil nematode assemblages. Pest Manage. Sci. 69, 679–684. doi: 10.1002/ps.3505
Zhu Q., Li G., Jiang Z., Li M., Ma C., Li X., et al. (2020). Investigating the variation of dissolved organic matters and the evolution of autotrophic microbial community in composting with organic and inorganic carbon sources. Bioresource Technol. 304, 123013. doi: 10.1016/j.biortech.2020.123013
Keywords: agro-ecological sustainability, environmental conservation, organic amendments, sweetening sustainability, harnessing underutilized industrial by-product
Citation: Stephen GS, Shitindi MJ, Bura MD, Kahangwa CA and Nassary EK (2024) Harnessing the potential of sugarcane-based liquid byproducts—molasses and spentwash (vinasse) for enhanced soil health and environmental quality. A systematic review. Front. Agron. 6:1358076. doi: 10.3389/fagro.2024.1358076
Received: 20 December 2023; Accepted: 12 February 2024;
Published: 27 February 2024.
Edited by:
Min Huang, Hunan Agricultural University, ChinaReviewed by:
Rajiv Kumar Srivastava, University of Hohenheim, GermanyJiban Shrestha, Nepal Agricultural Research Council, Nepal
Copyright © 2024 Stephen, Shitindi, Bura, Kahangwa and Nassary. This is an open-access article distributed under the terms of the Creative Commons Attribution License (CC BY). The use, distribution or reproduction in other forums is permitted, provided the original author(s) and the copyright owner(s) are credited and that the original publication in this journal is cited, in accordance with accepted academic practice. No use, distribution or reproduction is permitted which does not comply with these terms.
*Correspondence: Gerison Saddick Stephen, c2Fkb2NrZ2VyaXNvbkBnbWFpbC5jb20=
†ORCID: Mawazo Jamson Shitindi, https://orcid.org/0000-0002-5579-902X