- Lethbridge Research and Development Centre, Agriculture and Agri-Food Canada, Government of Canada, Lethbridge, AB, Canada
Some plant species retain mature seeds in plant canopies aboveground which are released later during opportune windows for germination and establishment. This process, known as serotiny, can lead to aerial seedbanks that exist simultaneously with soil seedbanks. However, little is known about how serotiny affects the persistence of weed seeds in the aerial seedbank. A randomized-stratified survey of 117 sites in southern Alberta, Canada, was conducted in 2022 to determine whether the summer-annual tumbleweed Russian thistle (Salsola tragus L.) exhibits seed serotiny. The observational study confirmed that Russian thistle plants exhibit serotiny and that the seeds can exist simultaneously in aerial and soil seedbanks. On average, the plants sampled retained 332 ± 62 viable seeds plant-1 seven to eight months after senescence. This time frame followed winter and emergence of the subsequent generation of plants from the soil seedbank. Russian thistle plants that were attached to the soil retained about double (P = 0.0274) the number of seeds (549 ± 133 viable seeds plant-1) than those detached from the soil (270 ± 71 viable seeds plant-1), likely due to seeds dislodging during movement of the tumbleweeds with prevailing winds. Seeds persisting in aerial seedbanks could evade decay, predation, or lethal germination leading to seed mortality in the soil seedbank, and increase the likelihood of seed persistence and successful establishment of new plants in stressful environments.
1 Introduction
Russian thistle (Salsola tragus L.) is a drought- and heat-tolerant annual tumbleweed that was introduced to the Americas in 1873 as a crop seed contaminant (Beckie and Francis, 2009). The plants are highly prolific and can produce >100,000 seeds in the absence of competition (Young, 1986). The seeds persist for about 1 year in the soil seedbank (Burnside et al., 1996). As a C4 plant, Russian thistle thrives in the dry, warm regions of western North America where it is well-adapted to outcompete cool-season C3 crops and other vegetation (Nord et al., 1999). It uses a substantial amount of water and depletes reserves available for crop production (Schillinger and Young, 2000). This tumbleweed can reduce spring wheat (Triticum aestivum L.) yields >50% and completely preclude crop harvest when present at high densities (Young, 1988; Young and Gealy, 1986). In a 2017 mid-season survey of annual crops, Russian thistle was the 5th most abundant weed species in the Mixed Grassland ecoregion of Alberta, Canada, where it was found to the greatest extent in lentil (Lens culinaris Medik.) and durum wheat (Triticum durum Desf.) fields (Leeson et al., 2019). Russian thistle infested about 41 million ha of the western USA by 1990 (Young, 1991) and costs farmers in the Pacific Northwest >$50 million each year in reduced crop yields and additional control measures (Young et al., 2008).
Russian thistle was one of the first known herbicide-resistant weeds in western Canada (Morrison and Devine, 1994). Acetolactate synthase (ALS) inhibitor-resistant Russian thistle were documented in Montana and Washington state in 1987 and in Saskatchewan in 1989 (Heap, 2023). A 2017 survey of Alberta showed that 62% of the Russian thistle populations sampled were ALS inhibitor-resistant (Beckie et al., 2019), which increased to 74% by 2021 (CM Geddes, unpublished research). However, a similar survey of Manitoba did not find ALS inhibitor-resistant Russian thistle (Geddes et al., 2022a). Recent confirmation of glyphosate-resistant Russian thistle in Montana, Washington, and Oregon (Barroso, 2017; Kumar et al., 2017) indicates that continued selection for herbicide resistance is eroding the options available for herbicidal control of this species.
The soil seedbank represents an opportune window for management of most annual weeds because the establishment of subsequent generations relies on seeds entering the soil (Davis, 2006). However, the soil seedbank can be a more-transient target for the management of weeds like kochia [Bassia scoparia (L.) A.J. Scott] where serotiny results in aerial seedbanks that persist simultaneously with soil seedbanks (Geddes and Pittman, 2023). Lamont (1991) defined serotiny as “retention of at least part of the seed crop on the plant in the presence of the succeeding crop”. In this context, non-serotinous plants release all of their seeds at maturity and do not retain seeds in an aerial seedbank. More commonly used to classify tree species where fire triggers seed release from the plant canopy (Lamont and Enright, 2000), the concept of serotiny has been overlooked as a mechanism aiding weed population persistence. Indeed, weed seeds persisting in plant canopies would not be subject to the same microbial decay, predation, or lethal germination often causing seed mortality in the soil seedbank. Since other species in the Amaranthaceae family exhibit serotiny (Gao et al., 2014; Bhatt et al., 2019; Geddes and Pittman, 2023), we set out to determine whether this too could aid in persistence of Russian thistle. The study objectives were to determine (a) whether Russian thistle plants exhibit serotiny by retaining seeds in aerial seedbanks; and if so, (b) how many viable seeds were retained by Russian thistle plants following the winter, seven to eight months after plant senescence, and following emergence of the subsequent generation of plants.
2 Materials and methods
A randomized-stratified survey of 117 sites in southern Alberta, Canada, was conducted in May 2022. The survey coincided with 12 to 13 months after emergence of the Russian thistle plants and about seven to eight months after their senescence in the fall (Beckie and Francis, 2009). It also took place after the subsequent generation of Russian thistle plants had emerged from the soil seedbank (CM Geddes, personal observation). The survey targeted a 1.9 million ha area of southern Alberta, Canada, including the counties of Forty Mile, Lethbridge, Taber, and Warner where Russian thistle was most abundant (Leeson et al., 2019). The number of surveyed sites within each county was stratified based on cultivated area. Locations within each county were chosen at random and the nearest site with visible tumbleweeds was sampled. Further inspection identified the plants as kochia and/or Russian thistle, the two predominant tumbleweed species in the region. When Russian thistle were present, all aboveground biomass from a single plant was sampled and placed in a cotton sac. Each plant sample and site were categorized based on the site classification system outlined by Geddes and Pittman (2023). In brief, classification categories included stem attachment (attached to or detached from the soil), plant status (full plants or partial plants with missing branches or decapitated during crop harvest operations), site description (field access, roadside ditch, fence, field, oil well, or treeline), nearest preceding crop (cereal, oilseed, pulse, pasture, potato (Solanum tuberosum L.), or not applicable), and county (Forty Mile, Lethbridge, Taber, or Warner).
The plant samples were dried at 30°C for a maximum of 7 days and dry weight was determined. Each sample was threshed by hand and the seed bulk weight determined. The number of viable seeds in each sample was quantified using a modified version of the grow-out procedure reported by Geddes and Pittman (2023). A 2.0 g subsample was shallow planted (2 mm depth) in a 52 × 26 × 5 cm greenhouse flat filled with Cornell soil-less potting medium (Sheldrake and Boodley, 1966) supplemented with 750 mg N, 950 mg P, and 500 mg K L-1 mixture. The flats were placed in the greenhouse and watered daily by soaking from the bottom. The greenhouse was set to a 20/18°C temperature regime with a 16 h photoperiod and 230 µmol m-2 s-1 supplemental light from Fluence RAZR 3 light emitting diode bulbs (Fluence, Austin, TX). Emerged Russian thistle seedlings were counted and removed weekly. The grow-out period lasted four weeks, after which the samples were cold stratified at -20°C for four weeks then returned to the greenhouse. The total number of Russian thistle seedlings that emerged throughout three rounds of the grow-out/cold stratification procedure were summed and considered the number of viable seeds in the subsample. This was used with seed bulk weights to back-calculate the number of seeds retained by each Russian thistle plant.
Seed retention data were subject to analysis of variance (ANOVA) using general linear models in R v.4.3.1 (R Core Team, Vienna, Austria). A separate model was fit for each classification category. The assumptions of ANOVA were assessed using the Shapiro-Wilk statistic and by plotting the residuals and fitted values. Data were square-root transformed. Significant differences were determined using α = 0.05. Data were visualized by developing violin plots using the ‘ggplot2’ package. The sampled sites were mapped using QGIS 3.22.2 (QGIS Geographic Information System, Open Source Geospatial Foundation).
3 Results
Russian thistle were present at 45 of the 117 sites surveyed (Figure 1). The Russian thistle plants retained 332 viable seeds plant-1 on average (median of 193 viable seeds plant-1) about seven to eight months after plant senescence (Table 1). This time frame followed the winter period and spring emergence of the subsequent generation of plants. The number of seeds retained ranged from 0 to 2055 viable seeds plant-1. Seed retention was about double (549 viable seeds plant-1) for plants attached to the soil compared with those detached from the soil (270 viable seeds plant-1) (P = 0.0274) (Table 1; Figure 2). Similarly, partial plants that were either missing branches or decapitated during crop harvest had 60% lower seed retention (200 viable seeds plant-1) than full plants (498 viable seeds plant-1) (P = 0.0280). Both detached and partial plant classifications had positively skewed leptokurtic seed retention distributions (Table 1). This was due to a larger proportion of samples with low seed retention compared with the more evenly-distributed seed retention for attached and full plants (Figure 2). Site description, nearest preceding crop, and county were not associated with Russian thistle seed retention (P ≥ 0.1029) (Table 1).
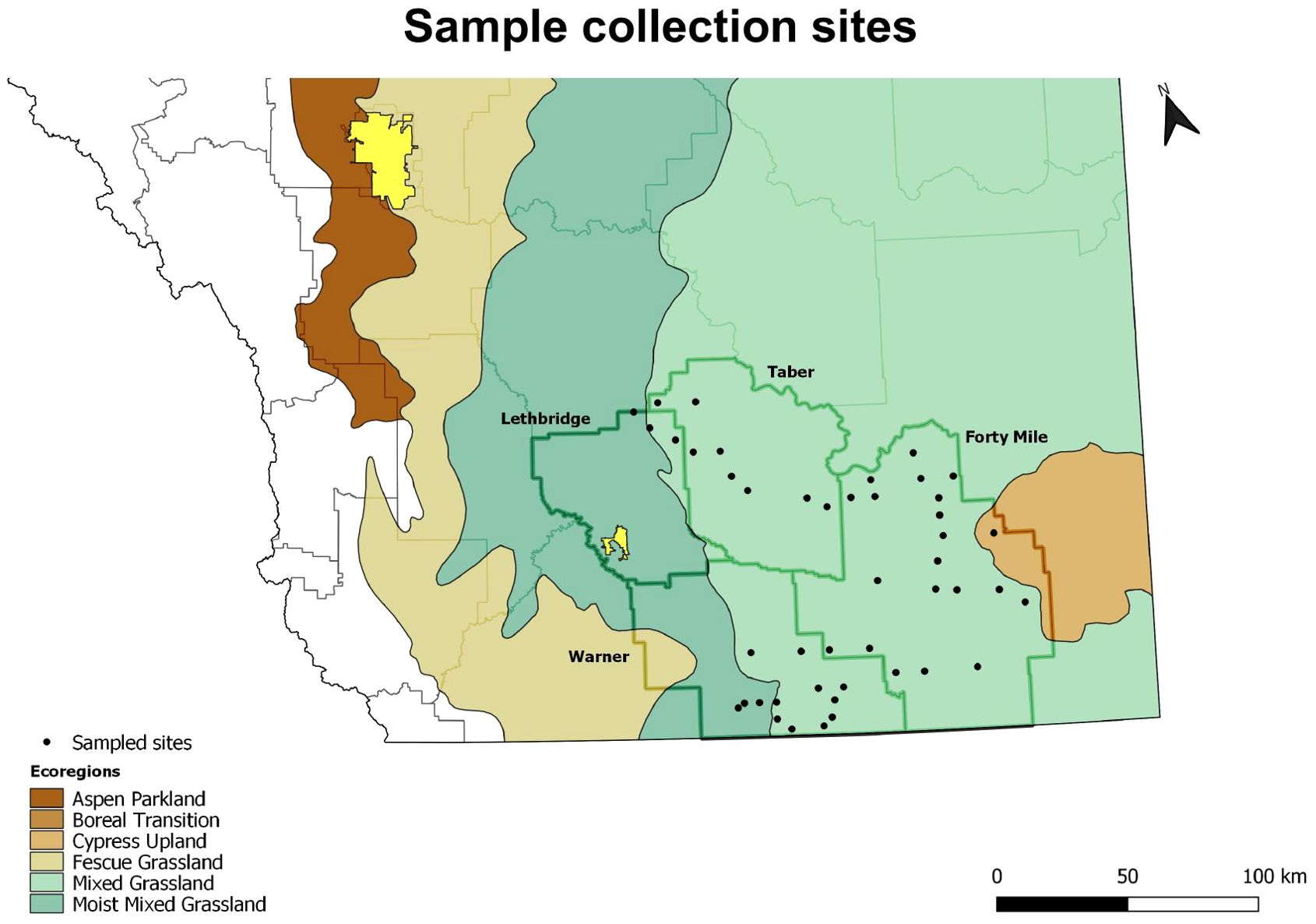
Figure 1. Map of southern Alberta, Canada, showing the locations of the 45 sites sampled in May 2022 to evaluate over-winter seed retention on Russian thistle (Salsola tragus) plants. Names on the map and bold polygons represent the four counties sampled. Base layers: ecoregions (Agriculture and Agri-Food Canada, A National Ecological Framework for Canada: GIS data, http://sis.agr.gc.ca/cansis/nsdb/ecostrat/gis_data.html); municipal boundaries (Altalis Ltd., Calgary, AB, Canada, www.altalis.com); cities (Statistics Canada, http://www12.statcan.gc.ca/census-recensement/index-eng.cfm). Map projection: NAD83/Alberta 10-TM.
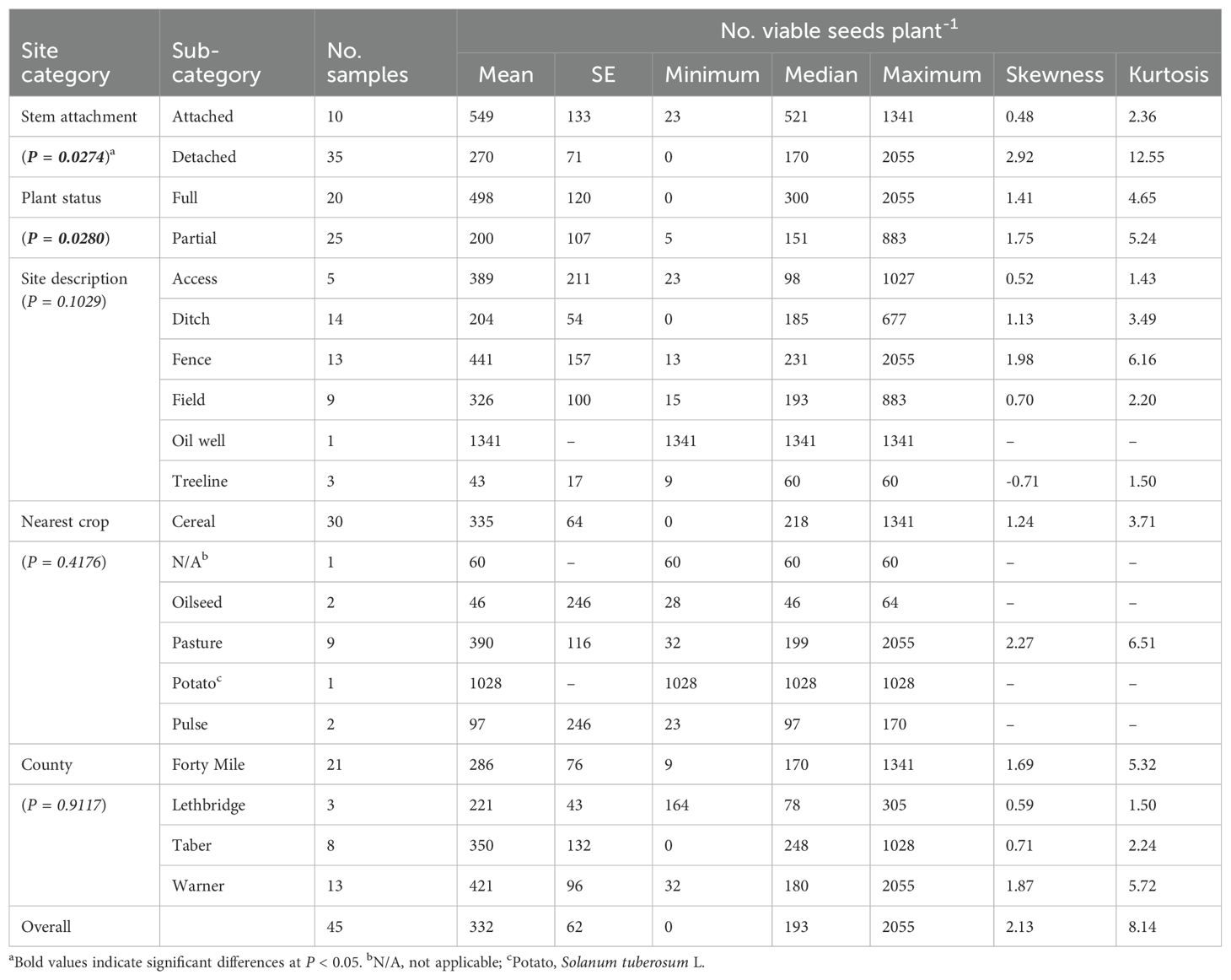
Table 1. Descriptive statistics for the number of seeds retained on Russian thistle (Salsola tragus) plants during the spring (May 2022) after plant senescence the previous fall as a function of stem attachment, plant status, site, nearest preceding crop, and county in southern Alberta, Canada.
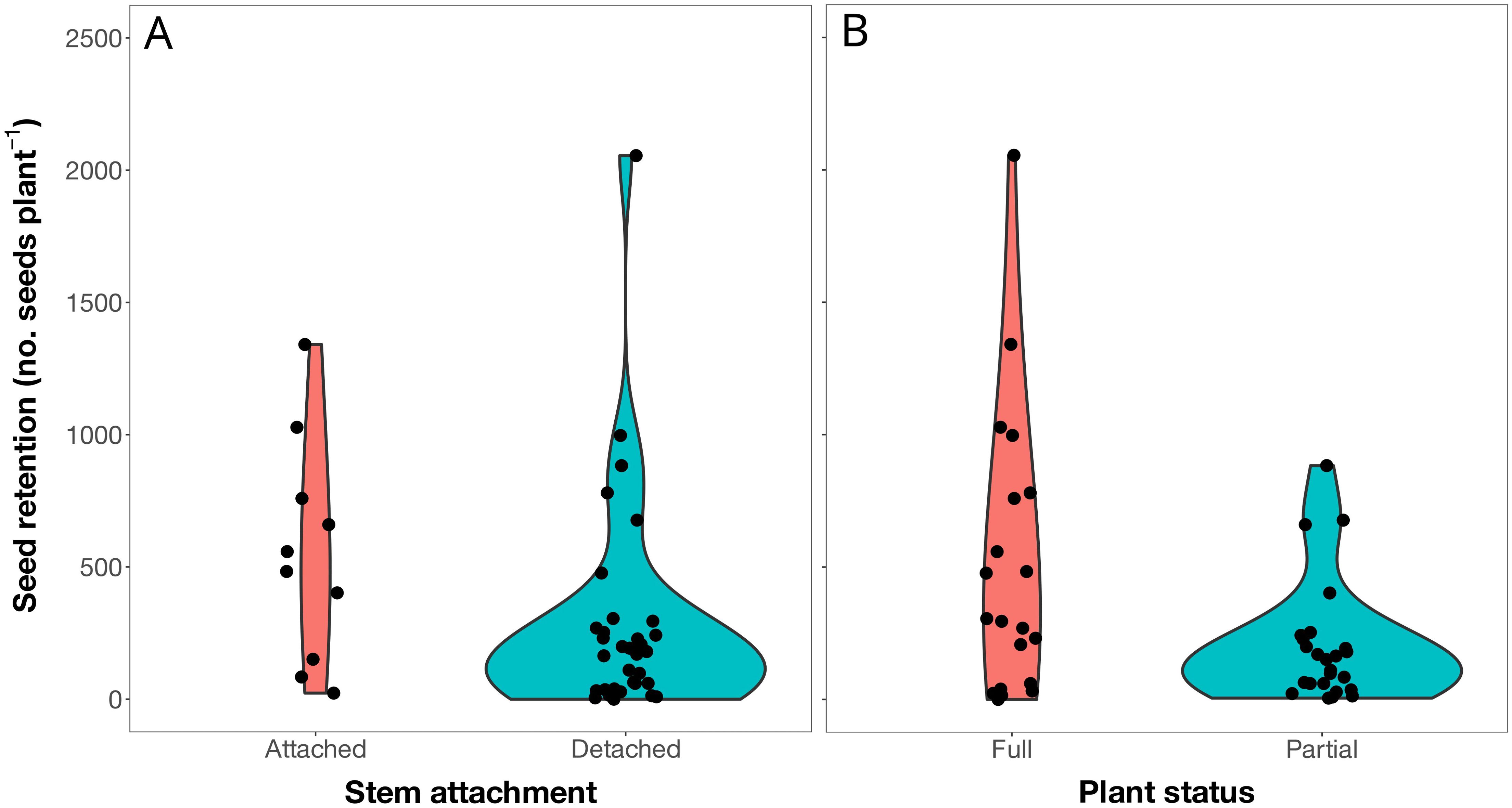
Figure 2. Violin plot showing observed over-winter seed retention on Russian thistle (Salsola tragus) plants evaluated in the spring (May 2022) following plant senescence the previous fall as a function of stem attachment (A; P = 0.0274) and plant status (B; P = 0.0280).
4 Discussion
The current study confirmed that Russian thistle exhibits weak serotiny resulting in simultaneous aerial and soil seedbanks. Russian thistle plants typically produce between 4,600 and 152,100 seeds depending on competition, ranging up to 200,000 seeds plant-1 (Young, 1986; Schillinger and Young, 2000). Based on average plant fecundity reported by Young (1986) and Schillinger and Young (2000) (4,600–152,100 seeds, competition dependent), our results suggest that Russian thistle retained ≤7% of the total number of seeds produced on average, ranging from 0% to 45%. Thus, Russian thistle serotiny was less than the 19% of seeds retained on average (ranging from <1% to 87%) for kochia plants collected during a comparable time frame (Geddes and Pittman, 2023), despite their similar phenology. If serotiny serves as a mechanism to aid in population persistence by enhancing the likelihood of establishment, then the differing magnitude of seed retention exhibited by these species could explain, in part, the more-rapid spread of herbicide resistance traits in kochia compared with Russian thistle (Beckie et al., 2019; Geddes et al., 2022a, b, 2023). Nevertheless, mean Russian thistle seed retention of 332 viable seeds plant-1 during the time of crop seeding in spring represents a substantial aerial seedbank. Seeds in this aerial seedbank could evade decay, predation, or lethal germination often subject to seeds in the soil seedbank. The physical disturbance of senesced plant tissue that occurs during crop seeding may dislodge retained seeds, thereby seeding Russian thistle with the crop and avoidance of non-residual pre-plant weed management tactics.
It was not surprising that over-winter seed retention was lower on Russian thistle plants that were detached from the soil or partial plants that were missing branches or decapitated during crop harvest. Russian thistle stems can break following senescence in the fall, forming a tumbleweed that travels across the landscape with wind (Beckie and Francis, 2009). The disturbance caused by tumbling dislodges seeds and disperses them along the travel transect (Stallings et al., 1995). This could explain the lower number of seeds retained on detached plants compared with those anchored to the soil. Similarly, lower seed retention on partial plants was expected since the loss of seed-producing branches undoubtedly leads to lower seed production in the absence of late-season reproductive compensation.
Prior to the current study, it was assumed that all Russian thistle seeds enter the soil seedbank in the fall where they are subject to processes leading to seed mortality over winter, and that population persistence was a direct function of seeds avoiding mortality in the soil seedbank. However, our research suggests that Russian thistle persistence is a function of seeds persisting in both aerial and soil seedbanks where they are not necessarily subject to the same fates. Therefore, contribution of aerial seedbanks to Russian thistle persistence warrants further investigation. As suggested previously for kochia (Geddes and Pittman, 2023), seed persistence in aerial and soil seedbanks could represent an adaptive bet-hedging strategy that promotes asynchronous seedling recruitment events in the drought-prone environments where Russian thistle is known to occur. A similar mechanism has been noted for other halophytic species in the Amaranthaceae family such as sand rice [Agriophyllum squarrosum (L.) Moq.], saltwort species (Seidlitzia rosmarinus Bunge ex Boiss.), and salt bush (Halothamnus iraqensis Botsch.) that occur in high-stress desert dune ecosystems (Gao et al., 2014; Bhatt et al., 2019).
Simultaneous persistence of Russian thistle seeds in aerial and soil seedbanks could impede the efficacy of management tactics targeting only the soil seedbank. However, post-harvest disturbance in arable agroecosystems, such as fall harrowing, may promote dehiscence of seeds retained in the plant canopy and concentrate the population in the soil. Management tactics targeting the Russian thistle aerial seedbank warrant future research. The current study suggests that persistence of Russian thistle populations from year to year is not a direct function of seed persistence in the soil seedbank as previously thought. Instead, seed persistence in aerial seedbanks must also be considered when developing an integrated weed management program targeting Russian thistle. Since the density of seeds present in both aerial and soil seedbanks is limited by the amount of seed produced by Russian thistle plants, management tactics aimed at reducing seed production could limit replenishment of seed reserves regardless of whether they are present in the soil or plant canopy.
Data availability statement
Data generated or analyzed during this study are available from the corresponding author upon reasonable request (absent of locational information). Requests to access the datasets should be directed to CG, Charles.Geddes@agr.gc.ca.
Author contributions
CG: Conceptualization, Data curation, Formal Analysis, Investigation, Methodology, Project administration, Resources, Software, Supervision, Validation, Visualization, Writing – original draft, Writing – review & editing. MP: Investigation, Writing – review & editing.
Funding
The author(s) declare that no financial support was received for the research, authorship, and/or publication of this article.
Acknowledgments
The authors acknowledge the greenhouse staff at the Lethbridge Research and Development Centre for maintaining the growth environment.
Conflict of interest
The authors declare that the research was conducted in the absence of any commercial or financial relationships that could be construed as a potential conflict of interest.
Publisher’s note
All claims expressed in this article are solely those of the authors and do not necessarily represent those of their affiliated organizations, or those of the publisher, the editors and the reviewers. Any product that may be evaluated in this article, or claim that may be made by its manufacturer, is not guaranteed or endorsed by the publisher.
References
Barroso J. (2017). Identification of glyphosate resistance in Russian thistle in northeastern Oregon. Crops Soils Mag. 50, 26–28. doi: 10.2134/cs2017.50.0211
Beckie H. J., Francis A. (2009). The biology of Canadian weeds. 65. Salsola tragus L. (updated). Can. J. Plant Sci. 89, 775–789. doi: 10.4141/CJPS08181
Beckie H. J., Hall L. M., Shirriff S. W., Martin E., Leeson J. Y. (2019). Glyphosate and acetolactate synthase inhibitor resistance in Russian thistle (Salsola tragus L.) in Alberta. Can. J. Plant Sci. 99, 384–387. doi: 10.1139/cjps-2018-0252
Bhatt A., Chat N. R., Lozano-Isla F., Gallacher D., Santo A., Batista-Silva W., et al. (2019). Germination asynchrony is increased by dual seed bank persistence in two desert perennial halophytes. Botany 87, 639–649. doi: 10.1139/cjb-2019-0071
Burnside O. C., Wilson R. G., Weisberg S., Hubbard K. G. (1996). Seed longevity of 41 weed species buried 17 years in eastern and western Nebraska. Weed Sci. 44, 74–86. doi: 10.1017/S0043174500093589
Davis A. S. (2006). When does it make sense to target the weed seed bank? Weed Sci. 54, 558–565. doi: 10.1614/WS-05-058R.1
Gao R., Yang X., Yang F., Wei L., Huang Z., Walck J. L. (2014). Aerial and soil seedbanks enable populations of an annual species to cope with an unpredictable dune ecosystem. Ann. Bot. 114, 279–287. doi: 10.1093/aob/mcu104
Geddes C. M., Gulden R. H., Jones T., Leeson J. Y., Pittman M. M., Sharpe S. M., et al. (2022a). Baseline survey of herbicide resistance in Russian thistle (Salsola tragus L.) finds no resistance in Manitoba. Can. J. Plant Sci. 102, 246–249. doi: 10.1139/cjps-2020-0260
Geddes C. M., Pittman M. M. (2023). Serotiny facilitates kochia (Bassia scoparia) persistence via aerial seedbanks. Can. J. Plant Sci. 103, 324–328. doi: 10.1139/cjps-2022-0178
Geddes C. M., Pittman M. M., Gulden R. H., Jones T., Leeson J. Y., Sharpe S. M., et al. (2022b). Rapid increase in glyphosate resistance and confirmation of dicamba-resistant kochia (Bassia scoparia) in Manitoba. Can. J. Plant Sci. 102, 459–464. doi: 10.1139/cjps-2021-0169
Geddes C. M., Pittman M. M., Hall L. M., Topinka A. K., Sharpe S. M., Leeson J. Y., et al. (2023). Increasing frequency of multiple herbicide-resistant kochia (Bassia scoparia) in Alberta. Can. J. Plant Sci. 103, 233–237. doi: 10.1139/cjps-2022-0224
Heap I. (2023).The international herbicide-resistant weed database. Available online at: www.weedscience.org (Accessed September 27, 2023).
Kumar V., Spring J. F., Jha P., Lyon D. J., Burke I. C. (2017). Glyphosate-resistant Russian-thistle (Salsola tragus) identified in Montana and Washington. Weed Technol. 31, 238–251. doi: 10.1017/wet.2016.32
Lamont B. B. (1991). Canopy seed storage and release – what’s in a name? Oikos 60, 266–268. doi: 10.2307/3544876
Lamont B. B., Enright N. J. (2000). Adaptive advantages of aerial seed banks. Plant Species Biol. 15, 157–166. doi: 10.1046/j.1442-1984.2000.00036.x
Leeson J. Y., Hall L., Neeser C., Tidemann B., Harker K. N. (2019). “Alberta weed survey of annual crops in 2017” in Weed Survey Series Publ. 19-1 (Agriculture and Agri-Food Canada, Saskatoon, SK), 275. p.
Morrison I. N., Devine M. D. (1994). Herbicide resistance in the Canadian prairie provinces: Five years after the fact. Phytoprot 75, 5–16. doi: 10.7202/706067ar
Nord C. A., Messersmith C. G., Nalewaja J. D. (1999). Growth of Kochia scoparia, Salsola iberica, and Triticum aestivum varies with temperature. Weed Sci. 47, 435–439. doi: 10.1017/S0043174500092043
Schillinger W. F., Young G. L. (2000). Soil water use and growth of Russian thistle after wheat harvest. Agron. J. 92, 167–172. doi: 10.2134/agronj2000.921167x
Sheldrake R. Jr., Boodley J. W. (1966). Plant growing in light-weight artificial mixes. Acta Hortic. 4, 155–157. doi: 10.17660/ActaHortic.1966.4.32
Stallings G. P., Thill D. C., Mallory-Smith C. A., Lass L. W. (1995). Plant movement and seed dispersal of Russian thistle (Salsola iberica). Weed Sci. 43, 63–69. doi: 10.1017/S0043174500080838
Young F. L. (1986). Russian thistle (Salsola iberica) growth and development in wheat (Triticum aestivum). Weed Sci. 34, 901–905. doi: 10.1017/S0043174500068077
Young F. L. (1988). Effect of Russian thistle (Salsola iberica) interference on spring wheat (Triticum aestivum). Weed Sci. 36, 594–598. doi: 10.1017/S0043174500075469
Young F. L., Gealy D. R. (1986). Control of Russian thistle (Salsola iberica) with chlorsulfuron in a wheat (Triticum aestivum) summer-fallow rotation. Weed Sci. 34, 318–324. doi: 10.1017/S0043174500066893
Young F. L., Yenish J. P., Launchbaugh G. K., McGrew L. L., Alldredge J. R. (2008). Postharvest control of Russian thistle (Salsola tragus) with a reduced herbicide applicator in the Pacific Northwest. Weed Technol. 22, 156–159. doi: 10.1614/WT-07-096.1
Keywords: Amaranthaceae, bradyspory, persistence, seed production, seed retention
Citation: Geddes CM and Pittman MM (2024) Russian thistle (Salsola tragus L.) serotiny promotes simultaneous aerial and soil seedbanks. Front. Agron. 6:1352303. doi: 10.3389/fagro.2024.1352303
Received: 07 December 2023; Accepted: 23 August 2024;
Published: 11 September 2024.
Edited by:
Aritz Royo-Esnal, Universitat de Lleida, SpainReviewed by:
Ahmet Uludag, Çanakkale Onsekiz Mart University, TürkiyeArnold Mashingaidze, Chinhoyi University of Technology, Zimbabwe
Copyright © 2024 His Majesty the King in Right of Canada, as represented by the Minister of Agriculture and Agri-Food Canada for the contribution of Charles M. Geddes and Mattea M. Pittman. This is an open-access article distributed under the terms of the Creative Commons Attribution License (CC BY). The use, distribution or reproduction in other forums is permitted, provided the original author(s) and the copyright owner(s) are credited and that the original publication in this journal is cited, in accordance with accepted academic practice. No use, distribution or reproduction is permitted which does not comply with these terms.
*Correspondence: Charles M. Geddes, Charles.Geddes@agr.gc.ca