- 1Developmental Biology, Institute of Biology, Faculty of Natural Science I, Martin-Luther University Halle-Wittenberg, Halle, Germany
- 2Biocenter, Martin-Luther University Halle-Wittenberg, Halle, Germany
- 3PTC Phage Technology Center GmbH, Bönen, Germany
- 4BASF Coordination Center Belgium CommV, Zwijnaarde, Belgium
Several species of Thysanoptera are considered agricultural pests, especially Frankliniella occidentalis (Western Flower Thrips, WFT). The damage WFT causes to plants can either be direct by feeding and oviposition activity or indirect by WFT serving as vectors for several plant viruses, bacteria, and fungi. Properties of WFT like polyphagy, cryptic lifestyle, fast reproduction and the ability to quickly develop insecticide resistance make them difficult to control. Alternative pest management tactics are thus in high demand. Potential targets are the facultative or obligate endosymbionts that affect the life history parameters of WFT. The known endosymbionts of WFT: BFo1/BFo2 (B = bacteria, Fo = Frankliniella occidentalis, number indicate different types) positively influence their fertility and development. Elimination of these endosymbionts could result in a decreased individual reproduction level and decreased population growth. Bacteriophages specific against BFo1/BFo2 were isolated and characterized and were applied in a no-choice feeding assay to female WFT. Antibiotic and sugar solutions served as controls. Phage-treated thrips showed no significant difference in survival, fecundity, oviposition rate or developmental time of the progeny compared to the control, whereas antibiotic treatment caused a decline in survival, fecundity, and oviposition rate, but not in the developmental time of offspring. The results showed a rapid decline of the bacteriophage concentration in the feed solution, which the WFT salivary gland products might cause. The complex relationship between thrips, endosymbionts and bacteriophages, needs further attention before bacteriophages could be used as potential management tools.
1 Introduction
The Western Flower Thrips, Frankliniella occidentalis (Thysanoptera: Thripidae), cause billions of dollars of damage to crop plants every year (Kirk et al., 2021). Their cryptic lifestyle, rapid reproduction and ability to quickly develop insecticide resistance make WFT difficult to control (Reitz et al., 2020). Up to now, 175 cases of resistance against 30 active ingredients of insecticides are described (Mota-Sanchez and Wise, 2022). To prevent the further emergence of resistance and avoid excessive pesticide use, novel management tactics are urgently needed. Optimally, these tactics should be tailored to the target organism and act without any environmental or non-target organism damage. Such a novel management tactic can aim at combating specific endosymbionts. Endosymbionts in insects have different functions (Feldhaar, 2011). Especially gut symbionts are known to improve life history and are partly obligatory for the animals by adopting synthesis pathways.
Frankliniella occidentalis shows a permanent association with intestinal bacteria (de Vries et al., 2001a; de Vries et al., 2001b). Most striking are the bacterial strains known as BFo1 and BFo2 (de Vries et al., 2001a; Chanbusarakum and Ullman, 2008), which show a worldwide stable distribution within Frankliniella populations (Chanbusarakum and Ullman, 2008). While BFo1 seems to belong to the Erwinia spp. bacteria, BFo2 is a novel species related to Pantoea (Facey et al., 2015). These bacteria influence the reproduction and development of their Frankliniella host. Aposymbiotic insects showed a reduced oviposition rate on certain forage plants and a slower development time. Supplementation with pollen could partly compensate for these negative effects (de Vries et al., 2004).
A management tactic could therefore try to reduce or eradicate the symbiotic gut bacteria and thus cause reduced population growth. A possibility to trigger such a reduction can be managed by bacteriophages introduced into the gut through the ingestion of a feed solution. Bacteriophages are viruses that are able to propagate within bacterial cells. Their non-toxicity to eukaryotic cells, self-replication, host specificity, and ease of production made them an interesting agent for biocontrol (Kering et al., 2019). Up to now, phages are a promising biocontrol agent of phytobacteria (Kering et al., 2019; Vu and Oh, 2020; Grace et al., 2021). While these authors showed that phages can specifically attack phytobacteria, a focused approach to attacking endosymbionts of herbivorous insects and vector species represents a completely new dimension of possible applications of phages in plant protection.
The work aimed to see if specifically produced phages against the Western Flower Thrips endosymbionts BFo1/BFo2 could be used to disturb the endosymbiont system and induce a negative effect on population growth parameters. To deliver phages via a feed solution represents a simple and possibly commercially viable form of application.
2 Materials and methods
2.1 Bacterial strains and growth conditions
The five Erwinia and Pantoea strains used in this study were donated by the University of Amsterdam, where the strains BFo1 and BFo-2 were detected, isolated and characterized (de Vries et al., 2001a; Facey et al., 2015). They were used for phage isolation and propagation. The strains were grown in LB Lennox Medium (Roth, Karlsruhe) at 30 °C with shaking or on agar plates of LB medium.
2.2 Phage isolation and sequencing
Over 100 environmental samples and samples of plant origin, like WFT-infected vegetable leaves and rose leaves, rotten vegetables and soil, were used for the isolation of bacteriophages on the five Erwinia and Pantoea host strains. After enrichment, phages were detected by a double agar overlay technique (Clokie and Kropinski, 2009). A table in the Supplementary Material (Supplementary 1) provides the data for six phages chosen for this study, including information on the phage origin, host range and GenBank accession numbers.
2.3 Phage cocktail against Erwinia and Pantoea strains
By combining six phages, a phage cocktail with a broad, overlapping host range and a wide genetic diversity was generated (see Supplementary 1).
2.4 Bioassay
Frankliniella occidentalis specimens were obtained from the main culture held at the Martin-Luther University Halle-Wittenberg. Thrips were maintained in 50 x 50 x 50 cm acrylic cages with 2 sides closed with fine mesh under light:dark cycle of 16:8 h, 5000 lux at 23 ± 1 °C. Thrips were fed on Phaseolus vulgaris var. Jutta plants.
In 6 different experimental conditions, the insects were offered different feeding solutions in a no-choice assay (feeding phase, 48 h) and allowed to oviposit afterwards (oviposition period, 96 h). The influence on survival probability, fecundity and larval development was investigated. The experiments were conducted in three independent biological replicates.
Experimental scheme:
(1) control – 2.5% sucrose with 0.5% patent blue V
(2) control + pollen – 2.5% sucrose with 0.5% patent blue V followed by pollen during the oviposition phase
(3) antibiotic – 2.5% sucrose with 5 mg/mL tetracycline and 0.5% patent blue V
(4) antibiotic + pollen – 2.5% sucrose with 5 mg/mL tetracycline and 0.5% patent blue V, followed by additional pollen during the oviposition phase
(5) test solution – 2.5% sucrose with 1 x 109 PFU/mL and 0. 5% patent blue V
(6) test solution + pollen – 2.5% sucrose with 1 x 109 PFU/mL and 0.5% patent blue V, followed by pollen during the oviposition phase
To obtain age-synchronized females for the experiments, females from the main culture were allowed to lay eggs for 2 x 24 h in a petri-dish filled with 1.4% agar and a bean leaf. Hatched larvae were transferred to 12-well cell culture plates at the L2 stage. Well plates were equipped with 1.4% agar and a piece of a bean leaf.
Age-synchronized adult female Western Flower Thrips (24–48 h post-emergence) were placed in the no-choice arena (Figure 1). The arena was made from a plastic cylinder (3 cm x 3 cm), the bottom of which was sealed with gauze. After the insects were placed inside, the top of the cylinder was sealed with parafilm, which was stretched to twice its length. The prepared arena was hung in a second cylinder (3.2 cm x 4 cm), the bottom of which was filled with 2 mL 1.4% agar to maximize humidity. After a 24 h starvation period, 200 µL feeding solution according to the experimental scheme was applied onto the parafilm layer and sealed with a second layer of parafilm. The arena was then placed in a plastic box equipped with moist cellulose to prevent drying of the feeding solution. The feed solutions were offered to the insects for 48 h (feeding phase). All feeding solutions were made on a distilled water basis. Patent Blue V was used as a tracer dye to monitor the food intake. To ensure the water solubility of the antibiotic to the necessary extent tetracycline hydrochloride (Sigma-Aldrich, T7660, CAS 64-75-5) was used. The feeding phase was performed in the dark at 23 ± 1 °C. During the starving and feeding phase, males were present.
After the feeding phase females were individually transferred to wells of a 12-well cell culture plate, each equipped with 1.4% agar and a punched bean leaf disc, for oviposition for 4 days (oviposition phase). The group with pollen supplementation received a brush tip (size 00) of pollen applied to the bean leaf (commercially available pollen from Pinus massoniana, Blue Diamond UGhb, Stuttgart, Germany). The oviposition phase was performed in a climatic chamber at the same conditions as the main culture. Leaf discs were changed every 24 h.
The survival of the mothers was recorded every day until the end of the experiments. The oviposition plates were checked daily for hatching larvae. Larvae were placed individually in cell culture plates (prepared as mentioned above). The documentation of the developmental stage was done every 24 h until the adult stage. Eight days after the onset of egg laying, the remaining eggs on the bean leaves were counted. For this purpose, the leaves were briefly heated in the microwave so that the eggs appeared as white dots and could be counted under a binocular. The data was used to determine fecundity (eggs/female), number of non-hatched eggs per female, total fecundity (eggs + non-hatched eggs/female), and oviposition rate (total fecundity/female/day).
2.5 Determination of bacteriophage concentrations
To test the stability of the phages during the process, the bacteriophage concentration was measured at different points in time after the first biological repetition of the experiment. We measured the concentration in the feeding solution before thrips feeding and after 48 h feeding period. A retained sample from the same age and storage served as a control.
Bacteriophage concentrations were measured by a standard double agar overlay plaque assay (Clokie and Kropinski, 2009).
2.6 Statistics
Data analysis was performed with the software SPSS 27 (IBM, Armonk, USA). Survival analyses were conducted with the Kaplan-Meier method and pairwise checked with Log-Rank-test (p = 0.05). Fecundity data were tested for variance homogeneity (Levene’s test, p = 0.05). Due to the failure of this assumption, even after ln-transformation, the data were analyzed by Welch-Anova and Games-Howell, or the Dunnett-T3 post hoc test (p = 0.05).
3 Results
3.1 Survival
A total of 33 individual female insects (24–48 h old) were set for the feeding phase per experimental condition. The antibiotic-treated insects, as well as antibiotic + pollen-treated insects have a significantly shorter survival time compared to the test-solution groups (Figure 2). The antibiotic group also has a significantly shorter survival time compared to the control-group, whereas the groups antibiotic + pollen and control + pollen are marginally non-significant (Kaplan-Meier method, log-rank test, p < 0.05, Figures 1A, B; for details see Supplementary 2, 3).
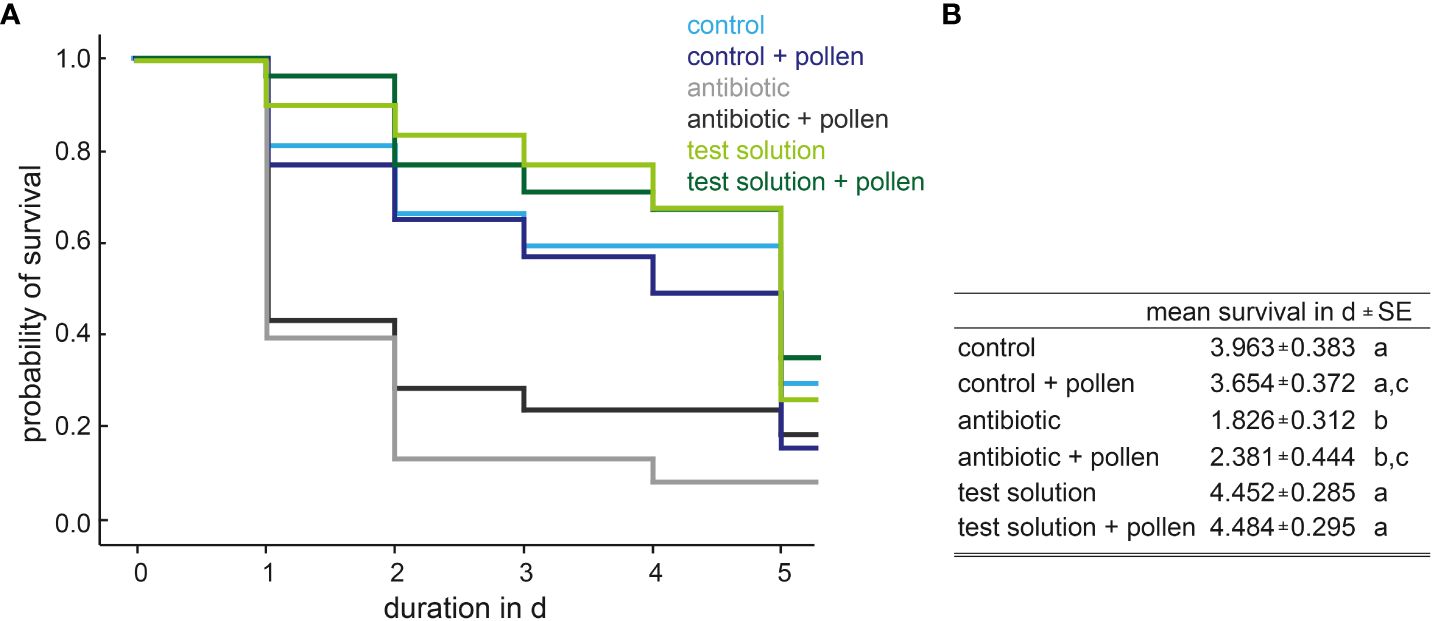
Figure 2 (A) Probability of survival depending on experimental design and (B) estimated mean survival in d ± SE, Kaplan-Meier-analysis with Log-Rank-test, different letters indicate significant differences.
3.2 Fecundity
The significant difference between the experimental groups is also evident in fecundity, total fecundity, oviposition rate, as well as the number of non-hatched eggs (Welch-ANOVA, all p < 0.001, post hoc: Dunnett’s T3 test, p < 0.05), Figure 3; Supplementary 4). The antibiotic-treated insects (with and without pollen) show a significantly reduced oviposition rate and total fecundity (Figure 3). The also significant reduction in non-hatched eggs in antibiotic-treated insects (Supplementary 4) can be attributed to low to no fecundity. The control insects did not differ in the above parameters from the insects administered the test solution.
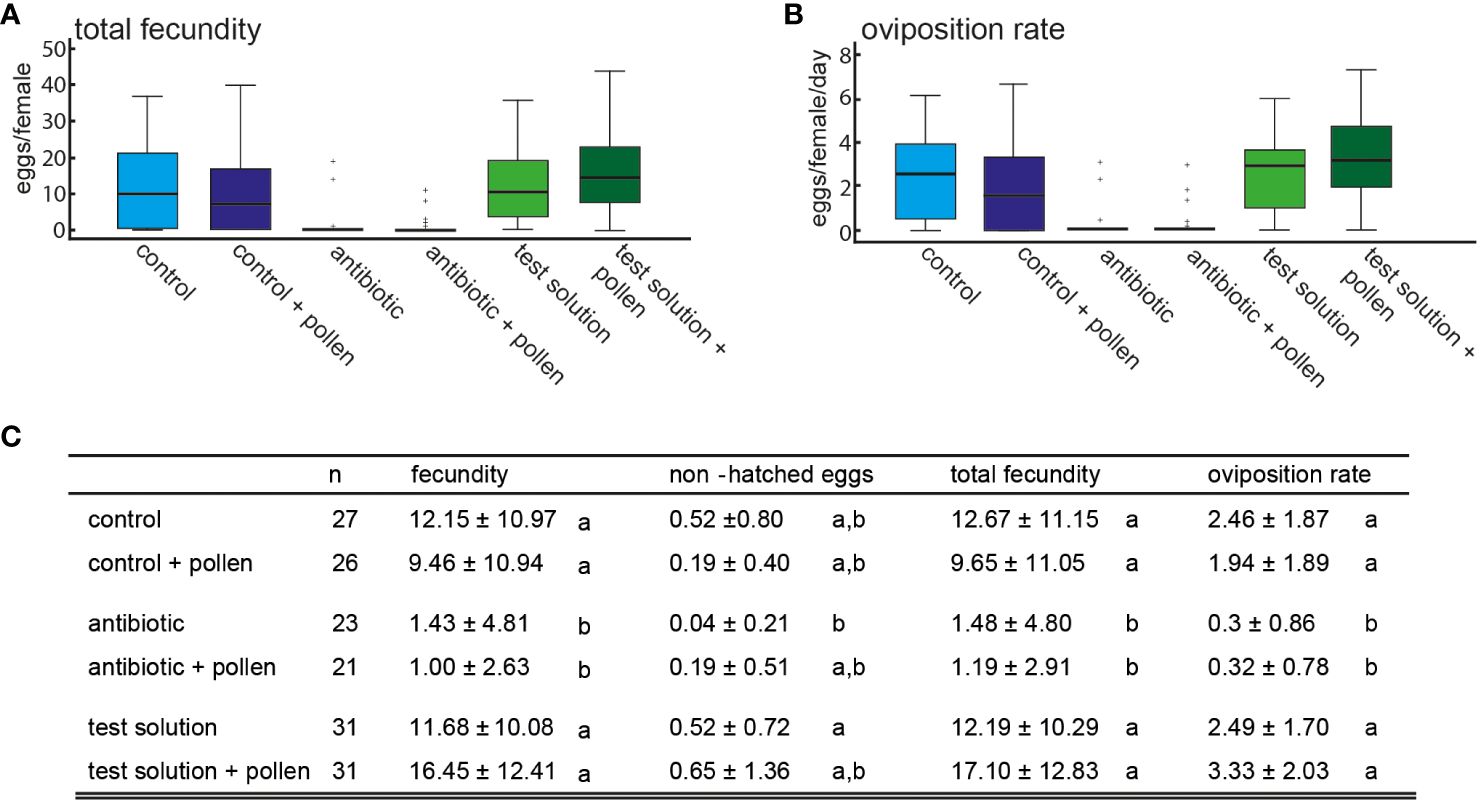
Figure 3 (A) Mean total fecundity and (B) oviposition rate depending on experimental design; (C) table with mean fecundity (eggs/female), mean number of non-hatched eggs, total fecundity (eggs + non-hatched eggs/female) and mean oviposition rate (eggs/female/day) ± SD, Welch-Anova and Dunnett-T3 post hoc test, p < 0.05, different letters indicate for significant differences.
3.3 Development of offspring, sex ratio
The duration of the developmental stages did not differ between the experimental conditions (Figure 4) with one exception: there was a significant difference in the duration of the embryogenesis phase (Welch-ANOVA, F = 3.642, df1 = 5, df2 = 44.944, p = 0.008) (Supplementary 5, 6). However, this difference refers only to the two experimental conditions of the test solution and test solution + pollen (Games-Howell, p < 0.001) (Figure 4; Supplementary 6).
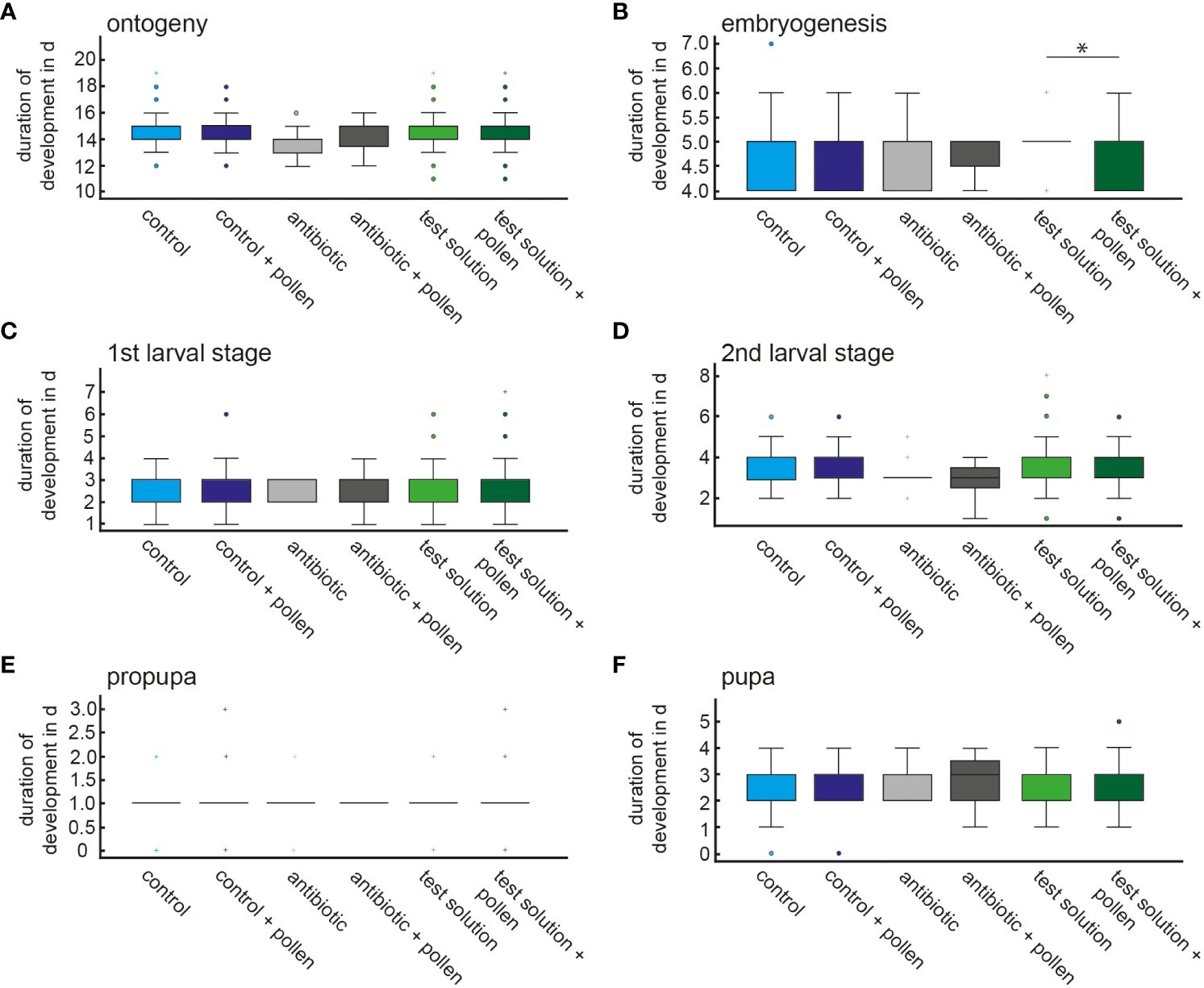
Figure 4 Mean developmental time of the F1 generation within the different developmental stages (A–F) depending on the experimental design, significant difference is marked by * (embryogenesis, Welch-ANOVA, Games-Howell post hoc p<0.001).
3.4 Bacteriophage concentrations before and after the feeding period
After the feeding phase of the first repetition, the titer of the remaining phage concentration was determined for the test solution. There was a reduction of the bacteriophage concentration by several powers of ten (Supplementary 7).
4 Discussion
Antibiotic treatment showed significant changes in the fecundity and oviposition rate of the insects. Similar results are shown in the study of de Vries et al. (2004), where the authors could demonstrate that symbiont-free insects showed a reduced oviposition rate, which, however, could be improved by pollen supplementation in this study. However, in our experimental setup, there was no prolongation of the larval development time, as observed by de Vries et al. (2004) in their aposymbiont insects.
While the antibiotic treatment non-specifically kills all antibiotic-sensitive bacteria in the thrips gut, the bacteriophage treatment should specifically target only the gram-negative intestinal symbionts. It was the goal of this study to determine if bacteriophages could substitute antibiotics by reducing the fecundity of thrips. However, with our feeding approach the insects showed no differences in fecundity, oviposition rate or larval development between the bacteriophage treatment and the control.
The major hurdle to the successful application of bacteriophages was most likely due to the rapid decline in bacteriophage activity observed during the feeding phase. The results showed a direct link between the bacteriophage activity loss and the thrips feeding process (see Supplementary 7). Thrips possess two types of salivary glands and salivation is known to occur during feeding activity (Ullman et al., 1989; Stafford et al., 2011). Numerous enzymes are found in the saliva of F. occidentalis, including proteases, carbohydrate-degrading enzymes, calcium-binding proteins, detoxification enzymes and several uncharacterized proteins. They function in detoxification, inhibition of plant defense, plant cell-wall degradation, extra-oral digestion and protein metabolism (Stafford-Banks et al., 2014; Rajarapu et al., 2022). It is tempting to speculate that the used bacteriophages might be sensitive to the proteolytic activity of the thrips saliva. It would then be an interesting option to isolate protease-resistant bacteriophages or formulate the bacteriophages in a manner that might withstand extra-oral digestion and reach the gut of the thrips.
The problem of extra-oral digestion of the feed solution could be avoided with alternative application methods like spraying or dipping. But the sensitivity of bacteriophages against sunlight and temperature (Kering et al., 2019), and the hidden lifestyle, with thigmotactic behavior, of thrips argue against such application forms. Therefore, there are still too many unanswered questions when trying to understand the complex relationship between thrips, endosymbionts and bacteriophages, and thus the use of bacteriophages as a novel pest management tool to reduce the damage done by Western Flower Thrips does not appear to be straightforward.
Another problem could be associated with the host specificity of bacteriophages. The tested bacteriophages were grown on bacterial strains originating from a different Frankliniella population (de Vries et al., 2001a) than the one used in the experiments. Although the symbiosis of the intestinal bacteria BFo1 and BFo2 seem to be generally widespread (Chanbusarakum and Ullman, 2008), there are regional differences regarding their expression (Chanbusarakum and Ullman, 2009) and also between strains (Andongma et al., 2022). Though we observed bacteriophage activity against the bacterial strains present in our Frankliniella population, it was beyond the scope of these experiments to specifically adapt the bacteriophages to their new target bacteria. We also had the simple applicability in the agricultural sector in mind, where it is not always possible to determine the symbionts beforehand. Bacteriophage cocktails have been used in phytobacterial control experiments to overcome the problem of specificity (Kering et al., 2019). Despite using a genetically diverse bacteriophage cocktail against Erwinia and Pantoea hosts, our approach was not successful.
In hindsight also the influence of the forage plants should have been considered. The negative effects of loss of gut bacteria were much more evident on pumpkin than on bean plants (de Vries et al., 2004), which were used in this set of experiments.
The endosymbiont-thrips system is sensible with several variances, which might be critical for the useful, broad commercial application of phages in pest management.
Data availability statement
The original contributions presented in the study are included in the article/Supplementary Material. Further inquiries can be directed to the corresponding author.
Ethics statement
The manuscript presents research on animals that do not require ethical approval for their study.
Author contributions
SK: Conceptualization, Investigation, Writing – original draft. AK: Investigation, Writing – review & editing. TL: Investigation, Writing – review & editing. HL: Investigation, Writing – review & editing. MS: Conceptualization, Writing – review & editing.
Funding
The author(s) declare financial support was received for the research, authorship, and/or publication of this article. The study was funded by a research assignment issued by BASF. The funder was not involved in the study design, collection, analysis, interpretation of data, the writing of this article or the decision to submit it for publication. The APC was covered by the Publication Fund of the Martin Luther University Halle-Wittenberg.
Acknowledgments
We thank Gerald Moritz, Martin-Luther-University Halle-Wittenberg, for critically discussing the experiments and manuscript, as well as Johanna Libnow and Emil Cyranka for laboratory support.
Conflict of interest
The authors AK, TL, and HL are employed by PTC Phage Technology Center GmbH. The author MS is employed by BASF Coordination Center Belgium CommV.
The remaining author declares that the research was conducted in the absence of any commercial or financial relationships that could be construed as a potential conflict of interest.
This study received funding from BASF. The funder was involved in conceptualization of the study. The experiments were conducted and evaluated independently of the funders.
Publisher’s note
All claims expressed in this article are solely those of the authors and do not necessarily represent those of their affiliated organizations, or those of the publisher, the editors and the reviewers. Any product that may be evaluated in this article, or claim that may be made by its manufacturer, is not guaranteed or endorsed by the publisher.
Supplementary material
The Supplementary Material for this article can be found online at: https://www.frontiersin.org/articles/10.3389/fagro.2024.1334561/full#supplementary-material
References
Andongma A. A., Whitten M. M. A., Del Sol R., Hitchings M., Dyson P. J. (2022). Bacterial competition influences the ability of symbiotic bacteria to colonize western flower thrips. Front. Microbiol. 13. doi: 10.3389/fmicb.2022.883891
Chanbusarakum L., Ullman D. E. (2008). Characterization of bacterial symbionts in Frankliniella occidentalis (Pergande), Western flower thrips. J. Invertebrate Pathol. 99 (3), 318–325. doi: 10.1016/j.jip.2008.09.001
Chanbusarakum L. J., Ullman D. E. (2009). Distribution and ecology of Frankliniella occidentalis (Thysanoptera: Thripidae) bacterial symbionts. Environ. Entomology 38 (4), 1069–1077. doi: 10.1603/022.038.0414
Clokie M. R. J., Kropinski A. M. (2009). “Bacteriophages,” in Methods and protocols (New York, NY: Humana Pr).
de Vries E. J., Breeuwer J. A., Jacobs G., Mollema C. (2001a). The association of Western flower thrips, Frankliniella occidentalis, with a near Erwinia species gut bacteria: transient or permanent? J. Invertebrate Pathol. 77 (2), 120–128. doi: 10.1006/jipa.2001.5009
de Vries E. J., Jacobs G., Breeuwer J. A. (2001b). Growth and transmission of gut bacteria in the Western flower thrips, Frankliniella occidentalis. J. Invertebrate Pathol. 77 (2), 129–137. doi: 10.1006/jipa.2001.5010
de Vries E. J., Jacobs G., Sabelis M. W., Menken S. B. J., Breeuwer J. A. J. (2004). Diet-dependent effects of gut bacteria on their insect host: the symbiosis of Erwinia sp. and western flower thrips. . Proc. R. Soc. B: Biol. Sci 271 (1553), 2171–2178. doi: 10.1098/rspb.2004.2817
Facey P. D., Méric G., Hitchings M. D., Pachebat J. A., Hegarty M. J., Chen X., et al. (2015). Draft genomes, phylogenetic reconstruction, and comparative genomics of two novel cohabiting bacterial symbionts isolated from frankliniella occidentalis. Genome Biol. Evol. 7 (8), 2188–2202. doi: 10.1093/gbe/evv136
Feldhaar H. (2011). Bacterial symbionts as mediators of ecologically important traits of insect hosts. Ecol. Entomology 36 (5), 533–543. doi: 10.1111/j.1365-2311.2011.01318.x
Grace E. R., Rabiey M., Friman V.-P., Jackson R. W. (2021). Seeing the forest for the trees: Use of phages to treat bacterial tree diseases. Plant Pathol. 70 (9), 1987–2004. doi: 10.1111/ppa.13465
Kering K. K., Kibii B. J., Wei H. (2019). Biocontrol of phytobacteria with bacteriophage cocktails. Pest Manage. Sci. 75 (7), 1775–1781. doi: 10.1002/ps.5324
Kirk W. D. J., Kogel W., Koschier E. H., Teulon D. A. J. (2021). Semiochemicals for thrips and their use in pest management. Annu. Rev. Entomology 66, 101–119. doi: 10.1146/annurev-ento-022020-081531
Mota-Sanchez D., Wise C. J. (2022) The Arthropod Pesticide Resistance Database. Available at: http://www.pesticideresistance.org (Accessed 6/7/2022).
Rajarapu S. P., Ben-Mahmoud S., Benoit J. B., Ullman D. E., Whitfield A. E., Rotenberg D. (2022). Sex-biased proteomic response to tomato spotted wilt virus infection of the salivary glands of Frankliniella occidentalis, the western flower thrips. Insect Biochem. Mol. Biol. Vol 149, 103843. doi: 10.1016/j.ibmb.2022.103843
Reitz S. R., Gao Y., Kirk W. D. J., Hoddle M. S., Leiss K. A., Funderburk J. E. (2020). Invasion biology, ecology, and management of western flower thrips. Annu. Rev. Entomology 65, 17–37. doi: 10.1146/annurev-ento-011019-024947
Stafford C. A., Walker G. P., Ullman D. E. (2011). Infection with a plant virus modifies vector feeding behavior. Proc. Natl. Acad. Sci. United States America 108 (23), 9350–9355. doi: 10.1073/pnas.1100773108
Stafford-Banks C. A., Rotenberg D., Johnson B. R., Whitfield A. E., Ullman D. E. (2014). Analysis of the salivary gland transcriptome of Frankliniella occidentalis. PloS One 9 (4), e94447. doi: 10.1371/journal.pone.0094447
Ullman D. E., Westcot D. M., Hunter W. B., Mau R. F. L. (1989). Internal anatomy and morphology of Frankliniella occidentalis (Pergande) (Thysanoptera: Thripidae) with special reference to interactions between thrips and tomato spotted wilt virus. Int. J. Insect Morphology Embryology 18 (5-6), 289–310. doi: 10.1016/0020-7322(89)90011-1
Keywords: Thysanoptera, insecticides, gut bacteria, Erwinia, phages, alternative management tactics
Citation: Krueger S, Kroj A, Lehnherr T, Lehnherr H and Stuiver M (2024) Could bacteriophages be used as a novel pest management tool to control gut endosymbionts in Western Flower Thrips? Front. Agron. 6:1334561. doi: 10.3389/fagro.2024.1334561
Received: 07 November 2023; Accepted: 08 January 2024;
Published: 26 April 2024.
Edited by:
Domenico Bosco, University of Turin, ItalyReviewed by:
Marika Rossi, National Research Council (CNR), ItalyElena Gonella, University of Turin, Italy
Copyright © 2024 Krueger, Kroj, Lehnherr, Lehnherr and Stuiver. This is an open-access article distributed under the terms of the Creative Commons Attribution License (CC BY). The use, distribution or reproduction in other forums is permitted, provided the original author(s) and the copyright owner(s) are credited and that the original publication in this journal is cited, in accordance with accepted academic practice. No use, distribution or reproduction is permitted which does not comply with these terms.
*Correspondence: Stephanie Krueger, c3RlcGhhbmllLmtydWVnZXJAYmlvemVudHJ1bS51bmktaGFsbGUuZGU=