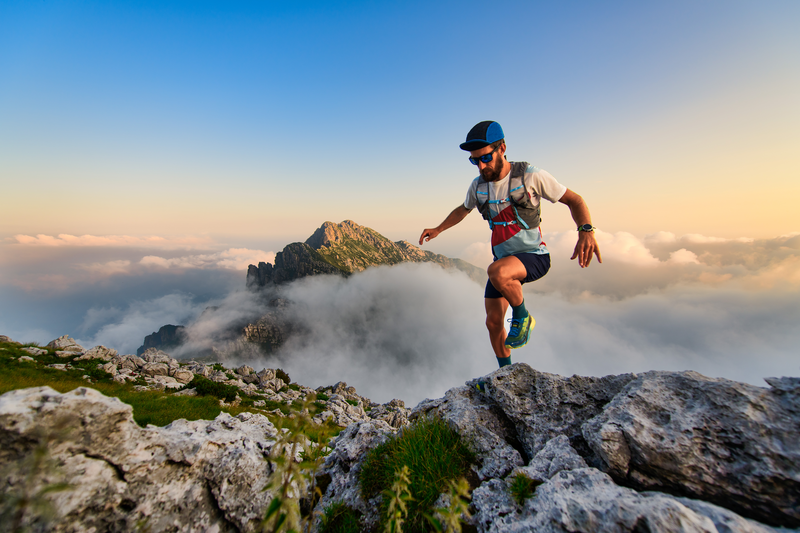
94% of researchers rate our articles as excellent or good
Learn more about the work of our research integrity team to safeguard the quality of each article we publish.
Find out more
ORIGINAL RESEARCH article
Front. Agron. , 16 January 2024
Sec. Weed Management
Volume 5 - 2023 | https://doi.org/10.3389/fagro.2023.1330222
This article is part of the Research Topic Agroecological Management of Weeds – Minimizing Chemical Herbicides in Arable Cropping View all 10 articles
Controlling creeping perennial weeds is challenging throughout all farming systems. The present study distinguished and explored three different methods to control them non-chemically: disturbance with inversion, disturbance without inversion, and competition. Focusing on Cirsium arvense, Elymus repens, and Sonchus arvensis, we conducted a field study (2019–2021) at three northern European sites in Germany, Finland, and Norway. We investigated the effects of the control methods ploughing (inversion disturbance), root cutting (non-inversion disturbance), and cover crops (competition) alone. Root cutting was conducted using a prototype machine developed by “Kverneland”. Eight treatments were tested in factorial designs adapted for each site. Control methods were applied solely and combined. Response variables after treatments were aboveground weed biomass and grain yield of spring cereals. The control method of ploughing was most effective in reducing weed biomass compared to root cutting or cover crops. However, compared to the untreated control, a pronounced additive effect of root cutting and cover crops occurred, reducing weed biomass (−57.5%) similar to ploughing (−66%). Pooled over sites, the response was species-specific, with each species showing a distinct reaction to both control methods. C. arvense was most susceptible to root cutting, followed by E. repens, while S. arvensis showed no susceptibility. Crop yield losses were prevented compared to untreated plots by ploughing (+60.57%) and root cutting (+30%), but not by cover crops. We conclude that the combination of non-inversion disturbance and competition is a promising strategy to reduce the reliance on herbicides or inversion tillage in the management of perennial weeds.
The perennial weed species Cirsium arvense (L.) Scop., Elymus repens (L.) Gould, and Sonchus arvensis L. are widespread in organic and conventional farming systems (Melander et al., 2013; Verwijst et al., 2018; Salonen et al., 2023). All three species thrive through vegetative propagation by either belowground creeping roots (C. arvense, S. arvensis) or rhizomes (E. repens). In this article, the term “roots” is used as a general term for both creeping roots and rhizomes, except when “rhizomes” are addressed explicitly.
In arable farming, creeping perennial weeds require control because crop yield losses occur in all production systems (Buhler et al., 2000; Weber and Gut, 2005). Yield losses are related to perennial weed infestations in general (Hartl, 1989; Brandsæter et al., 2012), or more specifically, with dense stands of C. arvense, E. repens, and S. arvensis reducing crop yield (Behrens and Elakkad, 1981; Melander, 1995; Vanhala et al., 2006).
Creeping perennials are mainly controlled by harrowing and ploughing, or in conventional farming, by applying glyphosate pre-plant as the dominating active ingredient (Håkansson, 2003; Brandsæter et al., 2017). These prevalent control measures are considered to have questionable aspects related to sustainability (Brandsæter et al., 2017; Tavaziva, 2017; Ringselle et al., 2020; Andert et al., 2023). Frequent inversion tillage through ploughing increases the risk of soil erosion and nutrient leaching (Aronsson et al., 2015). Regarding herbicides, it is a general goal to reduce the use not only because of potential environmental and health concerns, but also because of an increasing prevalence of herbicide resistance (Gunnarsson et al., 2017; Chauhan, 2020). Furthermore, glyphosate might face restrictions in the near future (Fogliatto et al., 2020; Tataridas et al., 2022; Triantafyllidis et al., 2023). Hence, controlling creeping perennial weeds without herbicides and intensive tillage would serve both pesticide regulations and environmental concerns.
Non-chemical management of creeping perennials in arable farming follows two general principles: Either disturbing plants or suppressing growth by competition (Weigel et al., 2023). Both disturbance and competition aim to weaken the plants and to reduce the overall infestation level (Håkansson, 2003). However, the methods differ. Disturbance reaches into the soil, affecting creeping roots directly, while competition suppresses creeping roots indirectly. When targeting perennial weeds, cover crops must have the ability to be strong competitors above ground (Bicksler and Masiunas, 2009; Wedryk and Cardina, 2012; Ringselle et al., 2015). Additionally, root competition between crops and cover crops is also important, as evidence suggests that belowground competition is a significant factor in various types of vegetation (Kroon et al., 2003). Disturbance can involve either soil inversion or no soil inversion, leading to the distinction of three different methods: disturbance with inversion, disturbance without inversion, and competition. Any effect of these three control methods will become evident in biannual or longer time periods through changes in the aboveground biomass production of perennial weeds.
Disturbance with inversion (ploughing) fragments creeping roots and buries the fragments to deeper soil layers (Håkansson, 2003). These fragments vary in size from short to long, approximately between 5 and 50 cm (Håkansson, 2003). Fragmentation leads to the depletion of root reserves as it induces root sprouting (Weigel and Gerowitt, 2022). Shoots emerging from buried fragments demand more energy to reach the soil surface (Dock Gustavsson, 1997; Håkansson, 2003). By combining fragmentation and burial, ploughing is an effective and reliable method for controlling perennial weeds (Brandsæter et al., 2011; Melander et al., 2012; Brandsæter et al., 2017).
Disturbance without soil inversion also fragments roots but does not bury them. It is referred to as “root cutting”. Ringselle et al. (2018) used a tool vertically slitting the soil and showed that E. repens shoot numbers decreased by approximately 30%. In a 2-year field study without a crop, Weigel and Gerowitt (2022) demonstrated that root cutting horizontally slitting the soil six times per year reduced shoot numbers of C. arvense by 75%. Although different tools have been used, these two studies indicate that C. arvense is more susceptible to cutting roots than E. repens. Results on S. arvensis are so far missing, but might be similar to C. arvense as both species propagate through creeping adventitious roots, while E. repens creeps through rhizomes (Lalonde and Roitberg, 1994; McClay and Peschken, 2002; Boström et al., 2013). In addition, the depth of the roots differs. Roots of S. arvensis and especially E. repens run shallow at depths of less than 10 cm, while the majority of C. arvense runs considerably deeper. In conjunction with the choice of the applied root cutting depth, the depth of the roots could be crucial. Root fragments of C. arvense resulting from tillage had only a minor role in new shoot development, when these fragments were located above the deeper running and intact root system (Thomsen et al., 2013). Species with a shallow root system (E. repens and S. arvensis) might be less affected by (deep) root cutting than those with a deeper root system (C. arvense).
Competition between weeds and crops is intensified when cover crops close the gap of open soil in the period between two main crops. Included in agronomical concepts, cover crops aim, in addition to other ecosystem services, to reduce perennial weeds (Bakker, 1960; Brandsæter et al., 2012; Brandsæter et al., 2017). Under-sown cover crops alone have been often unable to adequately suppress perennial weeds (Brandsæter et al., 2012; Ringselle et al., 2015; Reimer et al., 2019), except when the cover crops produced a large amount of biomass (Bergkvist et al., 2010).
So far, the combination of disturbance by root cutting with competition by cover crops, as a reasonable concept to work without ploughing, has not been investigated. While ploughing is an established, well-known method inverting the soil, root cutting without inverting the soil is an innovative technology. Field experiments were established on three sites in Northern Europe, in which the factors ploughing, root cutting, and cover crops were combined in eight treatments. The experiments exclusively focused non-chemical weeding. Targeted perennial weed species were C. arvense, E. repens, and S. arvensis. The experiments lasted for two subsequent years to account for biannual effects. We hypothesize that:
1. Root cutting and ploughing reduce weed biomass equally, but reductions vary among S. arvensis, C. arvense, and E. repens (H1).
2. Root cutting controls C. arvense more effectively than S. arvensis and E. repens (H2).
3. Adding cover crops to root cutting or ploughing increases the effectiveness of perennial weed control (H3).
4. All three methods of control by inversion tillage (ploughing), non-inversion tillage (root cutting), or competition effects (cover crops) prevent crop yield losses (H4).
In summer 2019, three field experiments were established, which ran for 2 years until the crop harvest in summer 2021. The experiments were located in Germany (Rostock, 54°01′N 12°14′E, 37 m.a.s.l.), Finland (Ruukki, 64°37′N 25°09′E, 47 m.a.s.l.), and Norway (Ås, 59°40′N 10°47′E, 75 m.a.s.l.). The experiments in Rostock and Ruukki were carried out on a conventional and an organic farm, respectively. The Ås experimental area was certified as organic prior to the experiment but taken out of the certification when starting the experiment. The soil types of the sites were sandy loam (pH = 6.8) in Rostock, fine sand with high soil organic matter (pH = 6.6) in Ruukki and silty clay loam with poor natural drainage in Ås (pH = 5.8), (Table A1). The crop rotations had been dominated by cereal crops (Rostock = Spring wheat, Ruukki = Spring oats, Ås = Spring wheat, spring barley, and spring oat) in the past at all sites. Details on weather conditions can be found in Table A1.
All sites featured spring cereals in both consecutive experimental years. These consisted of spring wheat cv. KWS Mistral in 2020 (400 seeds m−2) and Servus in 2021 (410 seeds m−2), both (180 kg ha−1) in Rostock (Seed drill type Rapid, 4 m, Väderstad, Sweden), spring oats cv. Niklas (215 kg ha−1, 550 seeds m−2) in Ruukki (Seed drill type Junkkari 2.5 m, Junkkari Oy, Finland), and spring barley cv. Brage (200 kg ha−1, 500 seeds m−2) in 2020 and spring wheat cv. Mirakel (225 kg ha−1, 600 seeds m−2) in 2021 in Ås (Seed drill type Nordsten 2.5 m, Nordsten, Denmark).
Seedbed preparation differed among sites; before sowing a field cultivator (Cruiser XL, Horsch, Germany), run a single pass (10 cm depth) in Rostock, run a single pass in Ruukki (power harrow, Kuhn, Germany), and there were two passes (6 cm depth) by a rotary harrow (Kuhn, Germany) in Ås. For each site, the fertilization was as follows: Rostock: cattle manure with 75 kg total N ha−1, Ruukki: meat-bone meal (Ecolan Agra 8-4-8 with 40 kg total N ha−1), and Ås: dried chicken manure, added bone meal (“Marihøne Pluss”), [N (8%)–P (4%)–K (5%), respectively], and pelletized fertiliser, with application corresponding to 100 kg total N ha−1 in the spring of 2019 and 2020, and 150 kg N ha−1 in 2021.
All sites carried the same factors of PL (ploughing), RC (root cutting), and CC (cover crop). A factorial combination of cover crop (with/without), root cutting in spring and autumn (with/without), and ploughing in spring (with/without) resulted in a total of eight treatments:
- Untreated control (UC)
- Cover crop (CC)
- Root cutter (RC)
- Plough (PL)
- Root cutter + cover crop (RC+CC)
- Plough + cover crop (PL+CC)
- Plough + root cutter (PL+RC)
- Plough + root cutter + cover crop (PL+RC+CC)
The treatment operations were repeated annually on the same plots in the period autumn 2019 to crop harvest 2021. Root cutting was done twice and ploughing was done once per year. Table 1 gives details when, how, and where each measure was carried out. Root cutting was done by the “Kverneland horizontal root cutter” (Figure 1). This root cutter is a prototype machine, horizontally cutting and fragmenting belowground root and shoot parts without inverting the soil by using five very flat, wide, and inflexible goosefoot shares (54 cm wide). Depth of root cutting was 20–25 cm in spring and 10 cm in autumn. Ploughing was conducted in spring at 20 cm (Rostock), 23 cm (Ruukki), and 25 cm depth (Ås). During the periods between the cultivation of spring cereals, cover crops of Sinapis alba L. (25 kg ha−1 both years) in Rostock and a ryegrass + clover mixture in Ruukki (15 kg ha−1 in Ruukki both years) and Ås (20 kg and 11 kg ha−1 in 2020 and 2021, respectively) were grown (Ruukki: Lolium multiflorum var. italicum Lam. + Trifolium repens; Ås: Lolium perenne + Trifolium repens).
Figure 1 The “Kverneland horizontal root cutter”; technical drawing by Kverneland Group Norway (left), picture by Marian Malte Weigel (right).
The trial in Rostock had a complete randomized block design, while the trials in Ruukki and Ås had a split-block design with ploughing (with/without) on main plots.
In Ruukki, individual plot size (subplots, gross) was 5.0 by 7.5 m. The main plot size (gross) was 30 by 15 m (300 m2). In Ås, individual plot size (subplots, gross) was 5.0 by 10 m, while the net size was 3.5 by 7.75 m. The main plot size (gross) was 35 by 10 m (350 m2). In Rostock, plot size varied between 9.56 m2 and 99.42 m2. The plot size differed because whole thistle patches were taken as individual plots in Rostock. The whole experimental area covered 50 by 400 m (20,000 m2).
The species C. arvense, S. arvensis, and E. repens were in focus. C. arvense was present in Rostock and Ås, and E. repens and S. arvensis were present in Ruukki and Ås. In Ås, the perennial species Stachys palustris and Vicia cracca also occurred. The biomasses of all mentioned species added together resulted in the variable CRPW (creeping perennial weeds).
At all sites, weed biomass was assessed before crop harvest in 2020 and 2021. In Rostock, weed biomass and shoot densities were assessed in 10 survey areas of 1 m2 each per plot. In Ruukki, two survey areas of 0.5 m2 each per plot and in Ås four survey areas of 0.5 m2 per plot were evaluated. The survey areas were in the same position in both years. Biomass sampling simulated cutting at crop harvest; hence, plants were cut 5 cm above the soil surface. The biomass samples were dried at 70°C for 72 h to determine the dry weight in Ås and Rostock. In Ruukki, air-dry weight was evaluated. Samples were dried in an air flow dryer at 40°C for several days. In the statistical analysis, biomass is always given as dry matter (DM) in g m−2.
Plots were combine harvested. In Ruukki and Ås, plot parts just outside the sample areas for weeds were harvested; in Ruukki, these were 1.5 m wide and 7.5 m long (11.25 m2), while they were 1.5 m wide and 7.75 m long (11.63 m2) in Ås. In Rostock, a plot harvester (1.5 m width) combined through the center of each patch.
In all three countries, grain samples were dried before storage. In Rostock and Ruukki, grain samples were dried in sacks with warm air. After drying, the grain samples were screened by experimental screening machine. In Ås, the grain yield of the plots was weighed at harvest and dried for storage. Grain moisture at harvest, grain weight per hectoliter, and screening percentage were determined. Samples were screened and moisture was measured. The cleaned grain yield was adjusted to 85% dry matter kg ha−1, before statistical analysis.
Data analysis (ANOVA) was conducted using the GLIMMIX package in SAS version 9.4 (SAS Institute Inc.), enabling mixed-effects modeling to accommodate both fixed and random sources of variation. Response variables were perennial aboveground weed biomass (CRPW) or crop grain yield. The Tukey–Kramer pairwise comparison was used to determine differences between treatments.
The initial weed densities assessed before onset of the experiments (end of June to early August 2019 depending on site, cf. Table 1) were always tested as a covariate in the analysis of weed biomass as this was a likely source of variation. Response variables were transformed with either ln(w + 1) or square root (w), where ln(·) is the natural logarithm function, to achieve the response variable analyzed being nearly normally distributed with approximately homogeneous variance, and in order to tone down the influence of certain deviant values.
The following mixed linear models were used. The models assumed that all random effects were independent, normally distributed random variables with an expected value of zero, and their respective variances were estimated from the data.
This model was used to analyze the response variables perennial weed biomass and grain yield (w) when two (Ruukki and Ås) or all (Rostock, Ruukki, and Ås) sites were combined, and assumed a split-block design. The model included a general mean (μ), main effects of ploughing i, root cutting j, cover crop k, year l and site m, their two-, three-, four-, and five-factor interactions, a linear covariate (x), random effects of block n and plot o, their interactions, and an error term (ε). The covariate was only considered when analyzing weed biomass.
The terms Bn(m), (πB)in(m), (τB)jn(m), and (νB)kn(m) are random effects of block n, and its interaction with ploughing i, root cutting j, and cover crop k, respectively. In this model, block n and plot o were nested within site m. Po(m)were included to account for the two observations from the 2 years, and the same plot may be correlated.
This model analyzed the response variables weed biomass or grain yield in Ruukki or Ås separately and assumed a split-block design. The covariate was only considered when analyzing weed biomass.
The terms Bn, (πB)in, (τB)jn, and (νB)kn are random effects of block n, and its interaction with ploughing i, root cutting j, and cover crop k, respectively. Po were included to account for the two observations from the 2 years, and the same plot may be correlated.
This model was used to analyze the response variables weed biomass or grain yield in Rostock separately and assumed a randomized complete block design. The covariate was only considered when analyzing weed biomass.
According to our hypotheses, control effects on total aboveground weed biomass were elaborated first. Results of C. arvense, E. repens, S. arvensis, and creeping perennial weeds (CRPW) are presented separately, across sites and per site. Yield effects are analyzed for each site. Factorial analyses unravelled the effects of PL, RC, and CC. These results are referred to in those tables and figures, addressing the factors and their interactions, hence the combined effects. Additive effects of the control methods on the response variables are analyzed comparing all designed experimental treatments. These results are referred to in those tables and figures addressing the full treatments. Important percentages (%) of an increase or decrease in the given response variable for a factor (with/without) or a treatment [compared to untreated (UC)] are provided in the text.
Although factor site was not significant (Table 2), differences were still observed in the analysis of the control methods for each site (Table 3). In Rostock, biomass reductions were significant for factor PL (−74.5%), RC (−44.3%), and CC (−35.7%) (Table 3; Figures 2A, B). Disturbance treatments, specifically when including PL, were more effective (−77.9%) in reducing biomass than competition by treatment CC (Figure 3A). By adding methods of competition to disturbance in the treatments, biomass was reduced. In particular, the RC+CC treatment exhibited additive effects, resulting in a −72% reduction (RC = −46.38%, CC = −26.2%). Treatment RC+CC achieved the same effects as treatment PL.
Table 2 Effects on the aboveground weed biomass (g m−2) of factors PL (yes/no), RC (yes/no), CC (yes/no), site (Rostock, Ruukki, and Ås), year (2020/2021), and their interactions for C. arvense (Rostock, Ås), E. repens (Ruukki, Ås), S. arvensis (Ruukki, Ås), and the sum of all creeping perennial weeds (CRPW, including S. palustris and V. cracca in Ås) (Model 1), ANOVA table, shoot density assessed before crop harvest in 2019 was used as a covariate (cir19/son19/ely19).
Table 3 Effects on the aboveground weed biomass (g m−2) of factors PL (yes/no), RC (yes/no), CC (yes/no), year (2020/2021), and their interactions for C. arvense, E. repens, and S. arvensis in Rostock (Model 3), Ruukki, or Ås (Model 2), ANOVA table, shoot density assessed before crop harvest in 2019 was used as a covariate (cir19/son19/ely19).
Figure 2 Total aboveground biomass (back-transformed LS means) for factors ploughing (PL), root cutting (RC), cover crops (CC), and year. C. arvense (A–C), E. repens (D–G), S. arvensis (H), in Rostock (A, B), Ruukki (D–F), and Ås (C, G, H). Treatments not sharing the same letter are significantly different (p-value ≤ 0.05).
Figure 3 Total aboveground biomass (back-transformed LS means) of treatment ploughing (PL), root cutting (RC), cover crop (CC), root cutter + cover crop (RC+CC), plough + cover crop (PL+CC), plough + root cutter (PL+RC), plough + root cutter + cover crop (PL+RC+CC), and untreated control (UC). (A) C. arvense, (B) E. repens, and (C) S. arvensis, mean of experimental years, each site separately. Treatments not sharing the same letter are significantly different (Tukey–Kramer, p-value ≤ 0.05).
In Ås, the factor PL reduced C. arvense biomass (−79.4%) but not significantly, while factor RC (−73.5%) was significant (Table 3; Figure 2C). Factor CC (−18%) significantly reduced biomass. PL*RC interacted negatively; therefore, no additive effect in the treatment PL+RC occurred (Table 3; Figure 2C). In general, all treatments including PL, RC, or CC reduced biomass with no differences between these treatments (Figure 3A). C. arvense biomass of PL and RC was lower in 2021 than in 2020 with a significant effect of the year (Table 3).
The effects of factor PL and RC on biomass were more pronounced in Ås than in Rostock (Table 2), underscored by the almost significant interactions Site*RC and Site*PL. A significant three-way interaction of Site*Year*PL is caused as PL was more effective in 2021 compared to 2020 in Ås.
The effect of factor PL differed strongly between the years. In 2020, PL increased biomass of E. repens by +34.3% (Table 3; Figure 2E), while in 2021, PL had a reversed effect, reducing biomass by −23.8%. Notably, across both years, the effect of factor RC was not different to that of PL (Figure 2D). In Ruukki, biomass of E. repens was reduced in factor RC by −19%. RC effect was strongest in 2021 with a decrease of −31.7% (Figure 2F) showing a positive interaction Year*RC.
In Ås, factor PL reduced E. repens biomass by −82.1%. In 2021, factor CC increased biomass of E. repens by +220% (Table 3; Figure 2G). However, compared to no CC, this increase was not significant. The mean biomass of E. repens by CC was significantly lower in 2021 than in 2020.
Across Ruukki and Ås, the factor PL reduced biomass by −38.8%. A difference between the two sites is indicated by the Site*PL interaction (Table 2). Effect of factor RC was significant, reducing biomass by −19.2%. CC reduced biomass neither as an individual treatment nor when added to the control methods ploughing or root cutting. In contrast to C. arvense biomass, only disturbance reduced biomass of E. repens. Both disturbance factors, RC and PL, resulted in lower biomass in 2021 than in 2020, highlighted by Year*RC and Year*PL interactions. Analyzed separately, factor PL had no effect in Ruukki (Table 3).
In Ås, the interaction RC*CC was significant (Table 3). Treatments PL+RC, RC+CC, and PL+RC+CC reduced biomass between 2020 and 2021 significantly, but not across both years (Table 3; Figure 3C). The interaction Year*RC was significant, because RC increased biomass in 2020 but decreased it in 2021 (Table 3). Additionally, Year*PL*RC significantly interacted, resulting in lower biomass values in 2021 than in 2020 for the combined factors PL and RC (Figure 2H; Table 3).
Analyzing Ruukki and Ås together, no significant effects of factors or any treatments occurred (Table 2). None of the results allow for a conclusive, statistical-based evaluation for S. arvensis. An interaction between Site*Year resulted from similar biomass values across the 2 years in Ruukki, unlike the decline observed in Ås in 2021 compared to 2020. Treatment PL+RC+CC was most effective in reducing biomass compared to UC, leading to a non-significant decrease of −55.2%.
When analyzing CRPW data across all three sites, factors PL, RC, and CC, and the interaction PL*RC were significant (Table 2). PL, RC, and CC reduced biomass by −54.4%, −34.5%, and −19.2%, respectively. Owing to the negative interaction of PL*RC, no additive effect occurred for treatment PL+RC (Figure 4). All disturbance treatments reduced CRPW biomass compared to UC (Figure 4). In contrast, treatment CC did not reduce CRPW biomass compared to UC. Nevertheless, by adding the control methods of RC (−43.6%) and CC (−14.4%), treatment RC+CC resulted in a −57.5% reduction, which was only exceeded by PL+RC+CC (−76.1%). No additive effects occurred for the methods of PL and CC.
Figure 4 Total aboveground biomass (back-transformed LS means across both years and all three sites) of all creeping perennial weeds (CRPW) in the treatments ploughing (PL), root cutting (RC), cover crop (CC), root cutter + cover crop (RC+CC), plough + cover crop (PL+CC), plough + root cutter (PL+RC), plough + root cutter + cover crop (PL+RC+CC), and untreated control (UC). Treatments not sharing the same letter are significantly different (Tukey–Kramer, p-value ≤ 0.05).
In Ås and Rostock, factors relying on disturbance (PL and RC) clearly affected the weed biomass, whereas the effects of CC were most notable in Rostock (Site*CC) (Table 2). In Ruukki, results varied depending on species and year. Solely, RC reduced biomass across all sites. In Ruukki, PL even increased weed biomass in 2020 (Site*PL), while PL across both sites reduced CRPW biomass. The year effect was significant with biomass values being lower (−35.2%) in 2021 compared to 2020.
Considering a significant site effect (ANOVA results not shown; Figure 5, see the scale of the ordinate axis), yield was analyzed separately for each site.
Figure 5 Grain yield per year (back-transformed LS means) of treatment ploughing (PL), root cutting (RC), cover crop (CC), root cutter + cover crop (RC+CC), plough + cover crop (PL+CC), plough + root cutter (PL+RC), plough + root cutter + cover crop (PL+RC+CC), and untreated control (UC) in Rostock (A), Ruukki (B), and Ås (C). Treatments not sharing the same letter are significantly different (Tukey–Kramer, p-value ≤ 0.05). Please note the different scale of the ordinate axis.
Yields in Rostock were higher in 2020 than in 2021 (Figure 5A; Table 4). Across both years, only factor PL (+28.72%) significantly increased yield. RC and CC did not prevent yield losses. No differences between treatments could be verified. Nevertheless, in 2021, after 2 years of experimental time, treatments including PL (PL+RC, PL+RC+CC) had the highest yield levels.
Table 4 Effects on grain yield (kg ha−1) of factors PL (yes/no), RC (yes/no), CC (yes/no), year (2020/2021), and their interactions for site Rostock (Model 3), Ruukki, and Ås (Model 2), ANOVA table.
In Ruukki, yields were lower in both years than on the other sites (Figure 5B). Yields were higher for factors PL (+35.5%) and also RC (+21.5%). In 2021, yields in all disturbance treatments delivered higher yields than in 2020. Treatments including disturbance did not differ from each other.
The overall yield level in Ås was the highest (Figure 5C). In both years, disturbance increased yield levels by factors PL (+59.5%) and RC (+15%) (Table 4). All treatments including PL resulted in higher yields compared to UC (Figure 5C). Treatment CC had the lowest yield in both years, which were even lower than in UC. The highest yields were measured in PL (2020) and PL+CC (2021) treatments (Figure 5).
When comparing sites, only factor PL significantly affected grain yields at all three sites. While RC also increased yields, the effect varied between sites, having no effect in Rostock but in Ruukki and Ås. CC alone did not increase yields at any site but reduced yields in Ås.
Controlling creeping perennial weeds is challenging throughout all farming systems. Our study explored three different methods to control them non-chemically: disturbance with inversion, disturbance without inversion, and competition. We analyzed perennial weed control effects of these methods: ploughing as inversion disturbance, root cutting as non-inversion disturbance, and cover crops to perform competition. Three species were investigated, being different in their aboveground growth and belowground clonal system. We discuss the results along the hypotheses stated in the introduction.
Our experimental setup allows one to directly compare the effects of factors PL and RC on perennial weeds (Tables 2, 3; Figure 2). Pooled over sites, statistical analysis revealed no significant differences in the responses of all three species to ploughing or root cutting, although the reaction varied among species. When separated by sites, PL (79.45%) in Rostock reduced C. arvense biomass more than RC (46.38%). In contrast, in Ås, PL did not reduce biomass more than RC. For E. repens, RC gave better effects than PL in Ruukki, while the opposite was the case in Ås. For S. arvensis, both factors, PL and RC, achieved poor and similar effects in Ruukki and Ås. Thus, hypothesis 1 is partly accepted, as the results depended on whether the sites are pooled or not. The variation among the species is evidently supported by the results.
To our knowledge, this study stands out as the first to directly compare the common method of ploughing with the innovative root cutting method for perennial weed control. Ploughing has been the standard for managing perennial weeds through tillage. Other non-inversion cultivation techniques, like different harrows or cultivators, did not achieve comparable results unless they are used in higher frequency—at least three times per year (Verschwele and Häusler, 2004; Lukashyk et al., 2008; Brandsæter et al., 2012). The fact that the non-inversion disturbance tool “Root cutter” provided results almost equal to ploughing is remarkable. We value the potential of the “Root cutter” in reducing perennial weed biomass with non-inversion disturbance as highly promising.
Moreover, root cutting offers potential environmental advantages by not inverting the soil. One environmental benefit is that weed control can commence in autumn using RC, thereby postponing PL until the following spring. Other important aspects such as impacts on soil structure, erosion, and energy consumption are yet to be answered in future studies.
Factor RC reduced biomass of C. arvense (−53.8%) and E. repens (−19.1%) but not S. arvensis. Therefore, hypothesis 2 is accepted. Root fragmentation induces re-sprouting, leading to root reserve depletion (Håkansson, 2003). Different species exhibit varying abilities to re-sprout after fragmentation: Re-sprouting of S. arvensis might have been less vigorous compared to C. arvense and E. repens, and thus, less reserves were depleted especially in autumn. This reluctance to re-sprout, termed bud dormancy, is in contrast between S. arvensis and the other two species, with several studies pointing out this difference (Brandsæter et al., 2010; Tørresen and Gerowitt, 2022). Tørresen and Gerowitt (2022) explored the sprouting ability under conditions resembling the Nordic autumn climate, finding that while C. arvense needed warmer conditions and E. repens sprouted under all/wider conditions, S. arvensis did not sprout at all. To some extent, this explains why S. arvensis, unlike C. arvense, could not be controlled by treatment RC+CC (Figure 3). In contrast to S. arvense, C. arvense exhibits stronger activity and vegetative growth during late summer and autumn. Treatment RC+CC adding competition to disturbance obviously controlled this active growth pattern better than the lagged one of S. arvensis. These results emphasize that timing of disturbance should be in accordance with species specific periods of vigorous re-sprouting in future applications of the root cutter.
Typically, spring ploughing is recognized as an effective tillage method for managing S. arvensis (Brandsæter et al., 2011). Consistent with our findings, the low efficacy of both disturbance and competition on S. arvensis was also observed by Brandsæter et al. (2012).
C. arvense with deep creeping roots and E. repens with shallow rhizomes were susceptible to disturbance, while S. arvensis with shallow and deep creeping roots was not susceptible. As a result, the sensitivity to disturbance could not be clearly attributed to the type of creeping organ (creeping roots, rhizomes) or to the occurrence of deep or shallow roots.
When explaining the better control of root cutting on C. arvense compared to S. arvensis, and also to E. repens, another factor is probably more important. The depth of root cutting in spring was 20–25 cm in our experiments. Research by Thomsen et al. (2013) demonstrated that due to shoots emerging from the intact root system below normal tillage depth, C. arvense was very susceptible to disturbance of its root system caused by deep tilling in the spring or early summer. This is also the reason why deep ploughing (e.g., to 25 cm) in spring controlled C. arvense (Brandsæter et al., 2011).
All three methods of control significantly reduced CRPW biomass in the factorial analysis (Table 2). Most effective in reducing CRPW biomass was treatment PL+RC+CC. Among the different control principles, disturbance proved to be more effective than competition in reducing CRPW biomass. This observation is consistent with the findings of previous studies in which disturbance tended to be more effective in reducing biomass of perennial weeds than competition (Håkansson, 2003; Brandsæter et al., 2012; Reimer et al., 2019; Salonen and Ketoja, 2020).
Treatments PL and RC both reduced CRPW biomass, with PL reducing CRPW biomass more than RC. However, when adding CC to RC (treatment RC+CC) the difference to PL became non-significant (Figure 4). Thus, adding competition through CC to RC amplified the control, resulting in pronounced additive effects. In contrast, adding CC to PL did not result in a comparable additive effect (treatment PL+CC, Figure 4). Therefore, hypothesis 3 can be accepted for root cutting and cover crops (RC+CC) but not for ploughing and cover crops (PL+CC).
The evaluated additive effects of the treatment RC+CC might result from the strategy of shallow root cutting (10 cm depth) in autumn and deep cutting in spring (20–25 cm depth). In contrast, treatments with PL were only ploughed in spring. Shallow cutting, which fragments the underground root and shoot parts, induces intensive re-sprouting of C. arvense (Weigel and Gerowitt, 2022). Frequently employed, such cutting gradually reduces C. arvense infestations. However, the immediate response is a burst of re-sprouting and the emergence of new shoots (Weigel and Gerowitt, 2022). This sprouting depletes root reserves needed to fuel the growth of the new shoots (Håkansson, 2003). While the reserves are depleted, they are accompanied by the emergence of new shoots. These shoots can be then suppressed by cover crops. A dynamic of re-sprouting depleting the reserves and a subsequent control of the emerged shoots can be initiated. As perennial weeds are vulnerable to light competition (Bakker, 1960; Edwards et al., 2000), well-established cover crops post-re-sprouting (via treatment RC+CC) allow to benefit from this dynamic. Unlike RC, adding CC to PL (PL+CC) did not result in enhanced control. Ploughing was conducted in spring, but not in autumn. The dynamic of inducing re-sprouting through disturbance in autumn followed by competition through cover crops was probably precluded through this timing.
Only factor PL resulted in higher yields across all sites; thus, disturbance by ploughing ensured the most reliable yields. Factor RC also increased yields; however, the magnitude varied among sites, having no effect in Rostock but having an effect in Ruukki and Ås. Comparing yields between untreated and control treatments indicates that the methods ploughing or root cutting or both prevented yield losses, but not cover crops alone (Figure 5). Therefore, hypothesis 4 is accepted for PL and RC, but rejected for CC. In Ås, even lower yields were measured in treatment CC than in UC. Notably and in contrast to Ruukki, a winter-hardy ryegrass species was used as cover crop. Its ability to survive winters was crucial. On plots without ploughing, the rotary harrow in spring only partially terminated the ryegrass, which survived the winter. Subsequently, the persistent cover crop competed with both perennial weeds and the new cash crop. Brandsæter et al. (2012) investigated the repeated undersowing of clover in spring cereals and showed that the presence of clover in the cereal crop reduced yield by competing with the crop.
In general, disturbance proved to be the more effective perennial weed control principle compared to competition, with inversion disturbance by ploughing being the most reliable. With respect to perennial weed control, farmers could simply carry on with ploughing. However, with respect to the undesired effects of ploughing on soil health and energy demand, feasible alternatives to ploughing are available for the management of perennial weeds. In our study, the combination of root cutting and cover crops had strong additive effects controlling perennials as reliable as ploughing. The extent of this dynamic varied slightly between species. We conclude that combining non-inversion disturbance with root cutting and competition can become an effective approach to control perennial weeds without inversion tillage.
Our study directly compared the perennial weed control effects of the traditional method of ploughing and the novel method of root cutting with a pilot prototype machine. Although root cutting showed great potential as an alternative method to ploughing in terms of perennial control, further research regarding important aspects like soil structure, erosion, and energy consumption is required to support its widespread use with facts and data about these crucial issues. The advantages of root cutting compared to ploughing are likely also in these aspects and will further fuel practical implementation. The commercial supply of root cutters is a pre-requisite for this. The widespread use of root cutters in practical farming will then ensure that suitable combinations with cover cropping will be on-farm evaluated and improved.
Generated datasets are available by request to the corresponding author with a situation-dependent restriction on who can access them. Requests to access the datasets should be directed tobWFyaWFuLndlaWdlbEB1bmktcm9zdG9jay5kZQ==.
MW: Writing – original draft. TB: Data curation, Visualization, Writing – review & editing, Formal Analysis. JS: Methodology, Validation, Writing – review & editing. TL: Methodology, Writing – review & editing, Validation. BG: Conceptualization, Formal Analysis, Methodology, Supervision, Validation, Writing – review & editing. LB: Conceptualization, Formal Analysis, Methodology, Supervision, Validation, Writing – review & editing.
The author(s) declare financial support was received for the research, authorship, and/or publication of this article. This study has received funding from the European Union’s Horizon 2020 research and innovation programme under grant agreement No 771134. The project “AC/DC-weeds- Applying and Combining Disturbance and Competition for an agro-ecological management of creeping perennial weeds” was funded by ERA-Net Cofund SusCrop/EU Horizon 2020, Grant no. 771134. National funders: DFG (Deutsche Forschungsgemeinschaft), GE 558/3-1; the Research Council of Norway (project no. 299695) and NIBIO; the Finnish MAKERA research grant from the Ministry of Agriculture and Forestry.
We sincerely thank Sabine Andert, Ingolf Gliege, Michael Klaus, Rainer Kittel, Bernd Klingenberg and Rosa Minderlen in the Crop Health group at the University of Rostock for their scientific and technical assistance. We thank Gut Dummerstorf GmbH for providing the experimental field and their technical assistance. We sincerely thank “SKP-Friland”/”Vollebekk research station” for experiment management, especially Vegard Hjerpaasen and Liv Berge, Marit Helgheim and Henrik Antzée-Hyllseth for technical assistance with the Norwegian experiment. A big thank also to the Kverneland Group Norway for making the root cutter prototype available for the experiments in three countries.
The authors declare that the research was conducted in the absence of any commercial or financial relationships that could be construed as a potential conflict of interest.
All claims expressed in this article are solely those of the authors and do not necessarily represent those of their affiliated organizations, or those of the publisher, the editors and the reviewers. Any product that may be evaluated in this article, or claim that may be made by its manufacturer, is not guaranteed or endorsed by the publisher.
Andert S., Guguin J., Hamacher M., Valantin-Morison M., Gerowitt B. (2023). How farmers perceive perennial weeds in Northern France and Eastern Germany. Front. Agron. 5. doi: 10.3389/fagro.2023.1247277
Aronsson H., Ringselle B., Andersson L., Bergkvist G. (2015). Combining mechanical control of couch grass (Elymus repens L.) with reduced tillage in early autumn and cover crops to decrease nitrogen and phosphorus leaching. Nutrient Cycling Agroecosystems 102, 383–396. doi: 10.1007/s10705-015-9712-7
Bakker D. (1960). “The Biology of Weeds,” in A comparative life-history study of Cirsium arvense (L.). Ed. Harper J. L. (Oxford, UK: Blackwell Scientific Publications Ltd).
Behrens R., Elakkad M. A. (1981). Canada thistle interference in crops. Canada Thistle Symposium. Proc. North Cent. Weed Conf. 36, 167–169.
Bergkvist G., Adler A., Hansson M., Weih M. (2010). Red fescue undersown in winter wheat suppresses Elytrigia repens. Weed Res. 50, 447–455. doi: 10.1111/j.1365-3180.2010.00789.x
Bicksler A. J., Masiunas J. B. (2009). Canada Thistle (Cirsium arvense) Suppression with Buckwheat or Sudangrass Cover Crops and Mowing. Weed technology 23, 556–563. doi: 10.1614/WT-09-050.1
Boström U., Andersson L., Forkman J., Hakman I., Liew J., Magnuski E. (2013). Seasonal variation in sprouting capacity from intact rhizome systems of three perennial weeds. Weed Res. 53, 387–398. doi: 10.1111/wre.12035
Brandsæter L. O., Bakken A. K., Mangerud K., Riley H., Eltun R., Fykse H. (2011). Effects of tractor weight, wheel placement and depth of ploughing on the infestation of perennial weeds in organically farmed cereals. Eur. J. Agron. 34, 239–246. doi: 10.1016/J.EJA.2011.02.001
Brandsæter L. O., Fogelfors H., Fykse H., Graglia E., Jensen R. K., Melander B., et al. (2010). Seasonal restrictions of bud growth on roots of Cirsium arvense and Sonchus arvensis and rhizomes of Elymus repens. Weed Res. 50, 102–109. doi: 10.1111/j.1365-3180.2009.00756.x
Brandsæter L. O., Goul Thomsen M., Wærnhus K., Fykse H. (2012). Effects of repeated clover undersowing in spring cereals and stubble treatments in autumn on Elymus repens, Sonchus arvensis and Cirsium arvense. Crop Prot. 32, 104–110. doi: 10.1016/j.cropro.2011.09.022
Brandsæter L. O., Mangerud K., Helgheim M., Berge T. W. (2017). Control of perennial weeds in spring cereals through stubble cultivation and mouldboard ploughing during autumn or spring. Crop Prot. 98, 16–23. doi: 10.1016/j.cropro.2017.03.006
Buhler D. D., Liebman M., Obrycki J. J. (2000). Theoretical and practical challenges to an IPM approach to weed management. Weed Sci. 48, 274–280. doi: 10.1614/0043-1745(2000)048[0274:TAPCTA]2.0.CO;2
Chauhan B. S. (2020). Grand challenges in weed management. Front. Agronomy. 1. doi: 10.3389/fagro.2019.00003
Dock Gustavsson A. (1997). Growth and regenerative capacity of plants of Cirsium arvense. Weed Res. 37, 229–236. doi: 10.1046/j.1365-3180.1997.d01-37.x
Edwards G. R., Bourdot G. W., Crawley M. J. (2000). Influence of herbivory, competition and soil fertility on the abundance of Cirsium arvense in acid grassland. J. Appl. Ecol. 37, 321–334. doi: 10.1046/j.1365-2664.2000.00495.x
Fogliatto S., Ferrero A., Vidotto F. (2020). Current and future scenarios of glyphosate use in Europe: Are there alternatives? Adv. Agron. 163, 219–278. doi: 10.1016/bs.agron.2020.05.005
Gunnarsson M., James T. K., Chynoweth R. J., Rolston M. P. (2017). An evaluation of the resistance of annual and perennial ryegrass to herbicides. New Z. Plant Prot. 70, 165–170. doi: 10.30843/nzpp.2017.70.45
Håkansson S. (2003). Weeds and Weed Management on Arable Land: An Ecological Approach (Cambridge, UK: CABI Pub), 14–219.
Hartl W. (1989). Influence of undersown clovers on weeds and on the yield of winter wheat in organic farming. Agriculture Ecosyst. Environ. 27, 389–396. doi: 10.1016/0167-8809(89)90099-6
Kroon H., de, Mommer L., Nishiwaki A. (2003). Root Competition: Towards a Mechanistic Understanding. 168, 215–234. doi: 10.1007/978-3-662-09784-7_9
Lalonde R. G., Roitberg B. D. (1994). Mating system, life-history, and reproduction in Canada thistle (Cirsium arvense; asteraceae). Am. J. Bot. 81, 21. doi: 10.2307/2445558
Lukashyk P., Berg M., Köpke U. (2008). Strategies to control Canada thistle (Cirsium arvense) under organic farming conditions. Renewable Agric. Food Syst. 23, 13–18. doi: 10.1017/S1742170507002013
McClay A. S., Peschken D. P. (2002). Sonchus arvensis L., perennial sow-thistle (Asteraceae). Biol. Control Programmes Canada 416–424. doi: 10.1079/9780851995274.0416
Melander B. (1995). Pre-harvest assessments of elymus repens (L.) gould interference in five arable crops. Acta Agriculturae Scandinavica Section B — Soil Plant Sci. 45, 188–196. doi: 10.1080/09064719509413103
Melander B., Holst N., Rasmussen I. A., Hansen P. K. (2012). Direct control of perennial weeds between crops – Implications for organic farming. Crop Prot. 40, 36–42. doi: 10.1016/j.cropro.2012.04.029
Melander B., Nørremark M., Kristensen E. F. (2013). Combining mechanical rhizome removal and cover crops for Elytrigia repens control in organic barley systems. Weed Res. 53, 461–469. doi: 10.1111/wre.12042
Reimer M., Ringselle B., Bergkvist G., Westaway S., Wittwer R., Baresel J. P., et al. (2019). Interactive effects of subsidiary crops and weed pressure in the transition period to non-inversion tillage, A case study of six sites across northern and central europe. Agronomy 9, 495. doi: 10.3390/agronomy9090495
Ringselle B., Bergkvist G., Aronsson H., Andersson L. (2015). Under-sown cover crops and post-harvest mowing as measures to control Elymus repens. Weed Res. 55, 309–319. doi: 10.1111/wre.12144
Ringselle B., Bertholtz E., Magnuski E., Brandsæter L. O., Mangerud K., Bergkvist G. (2018). Rhizome fragmentation by vertical disks reduces Elymus repens growth and benefits italian ryegrass-white clover crops. Front. Plant Sci. 8. doi: 10.3389/fpls.2017.02243
Ringselle B., Cauwer B., Salonen J., Soukup J. (2020). A review of non-chemical management of couch grass (Elymus repens). Agronomy 10, 1178. doi: 10.3390/agronomy10081178
Salonen J., Jalli H., Muotila A., Niemi M., Ojanen H., Ruuttunen P., et al. (2023). Fifth survey on weed flora in spring cereals in Finland. Agric. Food Sci. 32, 51–68. doi: 10.23986/afsci.130009
Salonen J., Ketoja E. (2020). Undersown cover crops have limited weed suppression potential when reducing tillage intensity in organically grown cereals. Organic Agric. 10, 107–121. doi: 10.1007/s13165-019-00262-6
Tataridas A., Kanatas P., Chatzigeorgiou A., Zannopoulos S., Travlos I. (2022). Sustainable crop and weed management in the era of the EU green deal: A survival guide. Agronomy 12, 589. doi: 10.3390/agronomy12030589
Tavaziva V. J. (2017). Effects of integrated pest management (IPM) on the population dynamics of the perennial weed species Cirsium arvense (L.) Scop, Vol. 2017 (Uppsala, Sweden: Swedish University of Uppsala), 110.
Thomsen M. G., Brandsæter L. O., Fykse H. (2013). Regeneration of Canada Thistle (Cirsium arvense) from Intact Roots and Root Fragments at Different Soil Depths. Weed Sci. 61, 277–282. doi: 10.1614/ws-d-12-00095.1
Tørresen K. S., Gerowitt B. (2022). Late-autumn ramet sprouting of three arable creeping perennial weed species. Agronomy 12, 2175. doi: 10.3390/agronomy12092175
Triantafyllidis V., Mavroeidis A., Kosma C., Karabagias I. K., Zotos A., Kehayias G., et al. (2023). Herbicide use in the era of farm to fork: strengths, weaknesses, and future implications. Water Air Soil pollut. 234, 94. doi: 10.1007/s11270-023-06125-x
Vanhala P., Lötjönen T., Hurme T. (2006). Managing Sonchus arvensis using mechanical and cultural methods. Agriculutral Food Sci. 15, 444–458. doi: 10.2137/145960606780061498
Verschwele A., Häusler A. (2004). Effect of crop rotation and tillage on infestation of Cirsium arvense in organic farming systems (Lillehammer, Norway: 6th EWRS Workshop on Physical and Cultural Weed Control), 187–194.
Verwijst T., Tavaziva V. J., Lundkvist A. (2018). Assessment of the compensation point of Cirsium arvense and effects of competition, root weight and burial depth on below-ground dry weight - leaf stage trajectories. Weed Res. 58, 292–303. doi: 10.1111/wre.12312
Weber E., Gut D. (2005). A survey of weeds that are increasingly spreading in Europe. Agron. Sustain. Dev. 25, 109–121. doi: 10.1051/agro:2004061
Weigel M. M., Andert S., Gerowitt B. (2023). Monitoring patch expansion amends to evaluate the effects of non-chemical control on the creeping perennial cirsium arvense (L.) scop. in a spring wheat crop. Agronomy 13, 1474. doi: 10.3390/agronomy13061474
Wedryk S., Cardina J. (2012). Smother Crop Mixtures for Canada Thistle (Cirsium arvense) Suppression in Organic Transition. Weed Science 60, 618–623. doi: 10.1614/WS-D-11-00140.1
Weigel M., Gerowitt B. (2022). Mechanical disturbance of Cirsium arvense - Results from a multi-year field study. Julius Kühn-Archiv 468, 79–85. doi: 10.5073/20220117-073804
Keywords: root cutting, ploughing, cover crops, Cirsium arvense, Elymus repens, Sonchus arvensis, sustainable agriculture
Citation: Weigel MM, Berge TW, Salonen J, Lötjönen T, Gerowitt B and Brandsæter LO (2024) Combining disturbance and competition to control creeping perennial weeds in a field study on three northern European sites. Front. Agron. 5:1330222. doi: 10.3389/fagro.2023.1330222
Received: 30 October 2023; Accepted: 19 December 2023;
Published: 16 January 2024.
Edited by:
Md (Asad) Asaduzzaman, Charles Sturt University, AustraliaReviewed by:
Marcelo L. Moretti, Oregon State University, United StatesCopyright © 2024 Weigel, Berge, Salonen, Lötjönen, Gerowitt and Brandsæter. This is an open-access article distributed under the terms of the Creative Commons Attribution License (CC BY). The use, distribution or reproduction in other forums is permitted, provided the original author(s) and the copyright owner(s) are credited and that the original publication in this journal is cited, in accordance with accepted academic practice. No use, distribution or reproduction is permitted which does not comply with these terms.
*Correspondence: Marian Malte Weigel, bWFyaWFuLndlaWdlbEB1bmktcm9zdG9jay5kZQ==
†These authors have contributed equally to this work
Disclaimer: All claims expressed in this article are solely those of the authors and do not necessarily represent those of their affiliated organizations, or those of the publisher, the editors and the reviewers. Any product that may be evaluated in this article or claim that may be made by its manufacturer is not guaranteed or endorsed by the publisher.
Research integrity at Frontiers
Learn more about the work of our research integrity team to safeguard the quality of each article we publish.