- 1Lacombe Research and Development Centre, Agriculture and Agri-Food Canada (AAFC), Lacombe, AB, Canada
- 2Department of Plant Sciences, University of Saskatchewan, Saskatoon, SK, Canada
- 3Department of Plant Science, University of Manitoba, Winnipeg, MB, Canada
- 4Lethbridge Research and Development Centre, Agriculture and Agri-Food Canada (AAFC), Lethbridge, AB, Canada
- 5Beaverlodge Research Farm, Agriculture and Agri-Food Canada, Beaverlodge, AB, Canada
- 6Scott Research Farm, Agriculture and Agri-Food Canada, Scott, SK, Canada
Although herbicides have been a dominant and effective weed control strategy for decades in Western Canada, herbicide resistance and the lack of new modes of action have resulted in weed management challenges. Integrated weed management strategies have been shown to be successful in controlling certain weed species that are problematic in cropping systems. The objective of this study was to investigate integrated weed management strategies that have been successful on individual species to determine their applicability to a multiple weed species that may coexist in a field. In addition, harvest weed seed control was incorporated into these integrated weed management strategies to determine its impact in western Canadian cropping systems. A 5-year rotational study was conducted from 2016 to 2020 at Beaverlodge, Lacombe, and Lethbridge, AB; Scott and Saskatoon, SK; and Carman, MB, that incorporated integrated weed management strategies such as rotational crop diversity (including winter annuals and perennials), increased seeding rates, crop silaging, chaff collection, and with or without in-crop herbicides. This research confirmed success in managing some species of weeds such as wild oat when increased seeding rates, 2 years of early cut silage barley, and competitive winter cereals were incorporated into a cropping system, even when no in-crop herbicides were applied. However, some weed growth morphologies (e.g., twining weeds) or life cycles (e.g., facultative winter annuals) were not managed successfully with this combination of strategies. Chaff collection provided incremental weed control benefits but did not serve as a replacement for herbicidal weed control. Weed densities had an apparent impact on the success of these integrated weed management strategies, suggesting that the sooner they are adopted, the more likely they are to be successful at maintaining or reducing weed densities. This study not only showed the ability to reduce reliance on herbicides with strategies that can be effective in Western Canada but also highlighted the need for further understanding of different weed species and their responses to integrated weed management strategies, as well as the complexity of managing a weed community with integrated weed management.
1 Introduction
Weeds cause substantial crop yield losses in western Canadian cropping systems. These losses reduce the net returns that farmers receive. Herbicide-resistant weeds can be difficult to control, resulting in increased costs due to reduced yields, ineffective management, and the need for additional control strategies. From 2014 to 2017, it was estimated that 9.6 million ha in a total area of 16.2 million ha in Western Canada was occupied by herbicide-resistant weeds (Beckie et al., 2020). The perceived cost of herbicide resistance for producers in that time frame averaged $33/ha or an annual cost of $530 million dollars (Beckie et al., 2020). Preliminary results of surveys conducted more recently indicate that the frequency and extent of infestation by herbicide-resistant weeds has increased, as has the annual cost to producers (C. Geddes, unpublished data).
Integrated weed management (IWM) strategies, or systems that combine chemical, physical, cultural, and/or biological control methods, can be effective at managing weeds, including those with herbicide resistance. However, weed management plans that integrate herbicide management tactics, including rotation of herbicide mode of action, tank mixing, and layering pre-seeding and post-emergence herbicides are often mistaken for IWM (Harker and O'Donovan, 2013). True IWM incorporates not only herbicide-based management but also cultural, physical, or biological control strategies. Successful IWM strategies incorporate life-cycle diversity, rotation design, competitive crop canopies, no or reduced tillage cropping systems, and maintenance of crop residues on the soil to help disrupt weed population dynamics (Anderson, 2005). In Western Canada, cultural practices including diverse crop rotations, utilizing competitive crop cultivars, incorporating silage production into the rotation, and increasing crop seeding rates have been shown to effectively suppress wild oat (Avena fatua L.) (O'Donovan et al., 1999; O'Donovan et al., 2000; Harker et al., 2003; Harker et al., 2009; Harker et al., 2016). Incorporating those strategies into a defined IWM system can result in synergistic improvements to weed management (O’Donovan et al., 2007; Blackshaw et al., 2008; Harker et al., 2009; Harker et al., 2016). A study combining many of those strategies with competitive winter annuals or perennials, rotations that included early cut silage crops and excluded wild oat herbicides for 3 years, still maintained similar wild oat density and biomass as a canola–wheat rotation that incorporated a full herbicide regime annually (Harker et al., 2016).
Although there are combinations of IWM strategies that can be effective, it is important to also look at the integration of novel cultural strategies into these IWM systems to increase their robustness and likelihood of long-term success. There has been a recent upsurge of interest in harvest weed seed control (HWSC) strategies and their potential to be incorporated into global cropping systems (Walsh et al., 2018; Shergill et al., 2020; Akhter et al., 2023). Although many of the previously mentioned strategies directly focus on limiting disturbance and increased crop competition with emerged weeds, HWSC focuses on preventing seed-bank input from weeds that have survived to crop maturity (Walsh et al., 2018). Incorporation of silaging strategies targets a different weed life-cycle stage (seed production) with a similar end goal of reducing the weed seed bank (Harker et al., 2003), but the marketable commodity from that rotational cycle is silage. If a producer is not operating a mixed operation (livestock and grain production), then they may have limited opportunity to market silage. Land trading between adjacent grain and mixed operations may provide a solution on a local scale; however, this is rare. Incorporation of HWSC technologies allows for prevention of weed seed-bank inputs while still allowing production of a marketable grain commodity. In addition, these strategies could both be incorporated into a rotation, in different rotational years/crops in the overall system. Studies on HWSC in Canada have demonstrated that efficacy was species-dependent based on seed retention levels (Burton et al., 2016; Burton et al., 2017; Beckie et al., 2018), but no studies have looked at benefits or risks of incorporating HWSC into a crop rotation or IWM program in Western Canada. Available HWSC methods include chaff collection, impact mills, narrow windrow burning, chaff lining or tramlining, and bale-direct systems (Walsh et al., 2018)
Many of the IWM cropping system studies in Western Canada have focused on management of wild oat (Harker et al., 2003; Harker et al., 2009; O'Donovan et al., 2013; Harker et al., 2016). A recent study found that rotations that included a perennial crop, or with two winter cereal crops in rotation or with incorporation of silage crops in a flax (Linum usitatissimum L.) rotation, were successful in managing wild oat and false cleavers (Galium spurium L.) with reduced herbicide applications (Benaragama et al., 2022). Although true IWM strategies can increase costs and complexity of managing weeds (Norsworthy et al., 2012; Ervin and Frisvold, 2016; Owen, 2016), these strategies are needed to provide growers options and strategies to reduce their reliance on herbicides, particularly in the face of continuously increasing herbicide-resistance pressures (Heap, 2023).
It must be recognized that weeds do not appear in isolation in producer fields but in weed communities where multiple species are coexisting simultaneously in a field. Therefore, the objective of this study was to investigate impacts of effective IWM strategies that have been studied for a single species for their efficacy levels on a weed community. The weed community included a common broadleaf and a common grass weed species across locations, in combination with other locally dominant weed species. In addition, a secondary objective was to evaluate the efficacy chaff collection as a HWSC strategy impact on weed community management in Western Canada. IWM strategy rotations were compared on weed and crop variables to the most common spring annual crop rotation on the Canadian Prairies: a repeated canola–wheat rotation at recommended seeding rates with a full herbicide regime (Beckie and Harker, 2017).
2 Materials and methods
The experiments were conducted at six locations across Western Canada from 2016 to 2020 [Beaverlodge, AB (55.2°N, 119°W); Lacombe, AB (52.5°N, 113.7°W); Lethbridge, AB (49.7°N, 112.8°W); Scott, SK (52.4°N, 108.8°W); Saskatoon, SK (52.5°N, 106.5°W); and Carman, MB (49.5°N, 98°W)]. In 2016, plot areas at all locations were treated pre-seeding with glyphosate (900 g ae ha−1) and bromoxynil (290 g ai ha−1) to manage early emerging weeds. After the pre-seeding applications, the plot areas were supplemented with 100 seeds m−2 of wild oat and 250 seeds m−2 of wild buckwheat (Fallopia convolvulus (L.) Á Löve) to ensure consistent grass and broadleaf species for comparison among the locations. In addition, location-specific weed species were also supplemented on the basis of local weed flora for development of a weed community (Table 1). All plots were direct seeded in long-term no-till or reduced tillage field areas.
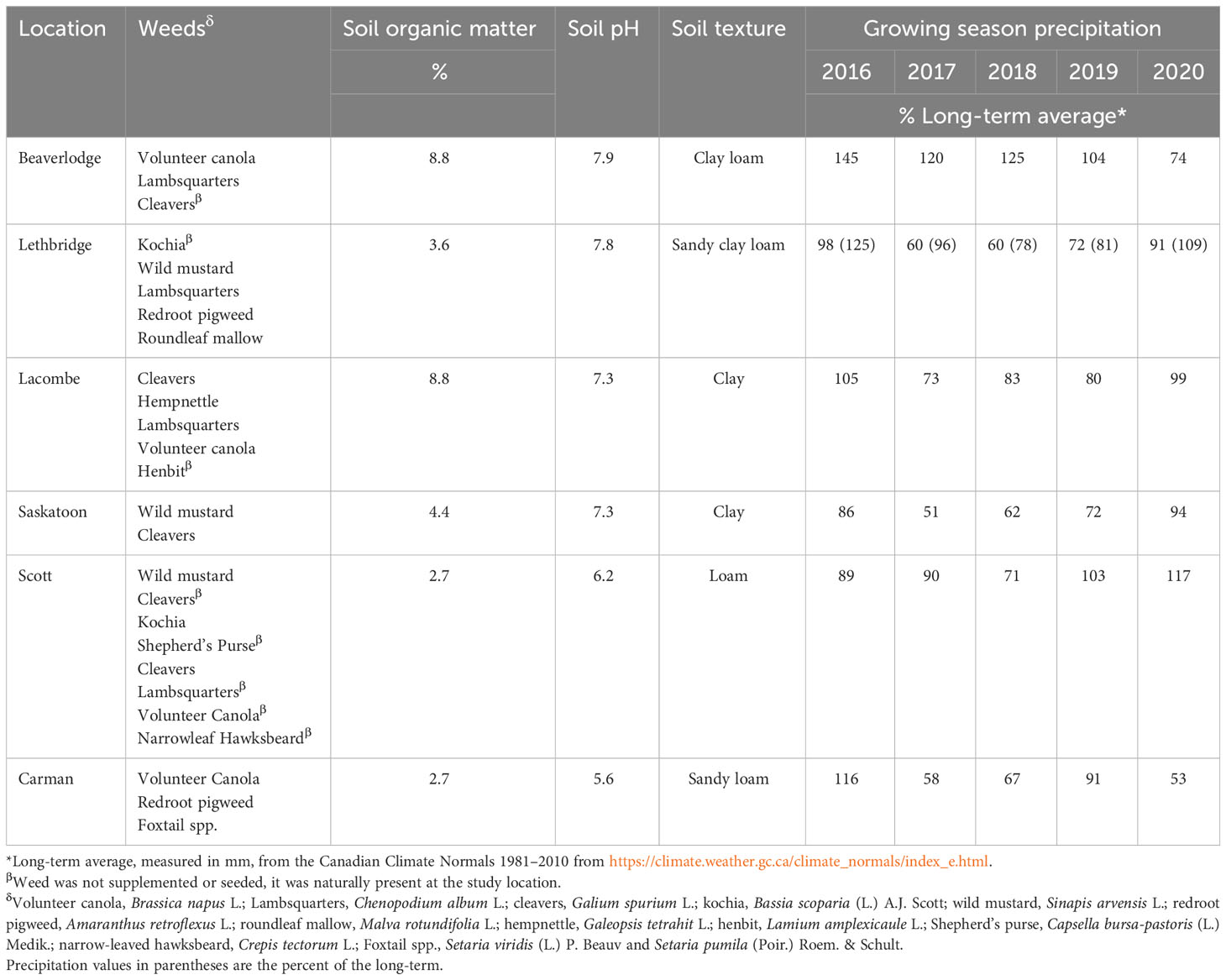
Table 1 Location characteristics where the rotational study was conducted between 2016 and 2020, including target weeds that were included in the study at each location, soil characteristics, and growing season precipitation for each year as a percent of the long-term average.
Soil samples were collected at each location each year before seeding and analyzed for soil nutrients at commercial laboratories. Fertilizer additions and blends were made to achieve the recommendations resulting from the soil tests for macronutrients. Fertilizer was primarily side-banded with some seed-placed starter fertilizer; however, this practice did vary slightly on the basis of seeding equipment at each location. Seeding equipment was primarily knife opener drills or air seeders with 25.4 cm (Saskatoon and Scott) or 30.5 cm (Beaverlodge, Lethbridge, and Lacombe) row spacing. Carman seeding equipment was a disc opener seeder with 19-cm row spacing. Fungicides and insecticides were applied as needed according to local disease and pest insect infestations. Pre-seed herbicides after the establishment year were location-specific and based on weed species and density. Plot dimensions were 3 m × 10 m at Scott, 2.3 m × 8 m in Saskatoon, 4 m × 8 m at Carman, 4.3 m × 12 m at Lethbridge, and 3.7 m × 15 m in Lacombe and Beaverlodge. Additional location characteristics including soil organic matter, soil type, and soil pH are described in Table 1.
At each location, 14 treatments were arranged in a randomized complete block design with four replications (Table 2). Crop seeding rates were at 1× (typical recommended seeding rate), 1.5× or 2× (increased rates as an IWM strategy) as follows: spring cereals, 200 seeds m−2 (1×) and 400 seeds m−2 (2×); canola (Brassica napus L.), 100 seeds m−2 (1×) and 150 seeds m−2 (1.5×); field pea (Pisum sativum L.), 80 seeds m−2 (1×) and 120 seeds m−2 (1.5×); fababeans (Vicia faba L.), 40 seeds m−2 (1×) and 60 seeds m−2 (1.5×); winter cereals, 300 seeds m−2 (1×) and 600 seeds m−2 (2×); and alfalfa (Medicago sativa L.), 9 kg ha−1 (1×). Although doubling seeding rates in all crops would have been ideal from an IWM strategy perspective, the large seed size of fababean and pea can cause logistical challenges at high seeding rates, and the high seed cost of canola seed renders this an economically irrelevant strategy from a production perspective. Incorporation of 1.5× rates allowed us to continue to incorporate increased seeding rates as an IWM strategy while balancing logistic and practical considerations. In-crop herbicide product selections, where applications were required in the treatment regime, were location-specific based on the weed community present (Table 1). Early-cut barley (Hordeum vulgare L.) silage was cut 1 week after head emergence (Zadoks 65) (Zadoks et al., 1974) to leverage as much weed control out of this technique as possible (Harker et al., 2003). Each treatment was initiated (2016) with the same crop and IWM strategies across all treatments. The trial was initially seeded at a 2× seeding rate of spring wheat (Triticum aestivum L.) and no in-crop herbicide applications in 2016 to ensure establishment and naturalization of targeted weed species. The treatments then integrated different factors including crop species in rotation, crop life cycles (incorporation of winter annuals and perennials), herbicide regime (no in-crop or conventional practice which includes one to two in-crop herbicide applications per season), incorporation of silage harvest, and chaff collection (yes or no) (Table 2) over three growing seasons (2017 to 2019). The only exception to the no in-crop herbicide regime was the initial establishment year of alfalfa where a single in-crop herbicide treatment was allowed to assist in establishment, with no herbicides applied thereafter.
Chaff collection was chosen as the HWSC strategy in this trial. Ideally, a physical impact mill would have been incorporated as they are currently of most interest to Western Canada producers, but they are not currently available for plot sized combines. By collecting and removing the chaff from the plot area, we simulated the effect of an impact mill on the weed populations; although, residue removal is unique to chaff collection (Walsh et al., 2017). Chaff collection is a less common HWSC technique (Walsh et al., 2018) but still has the potential to be equally effective in terms of weed management, and it is far simpler to implement in plot scale research. Chaff collection equipment was designed to match the harvesting equipment available at each location. Examples of some of the chaff collection systems are shown in Figure 1.
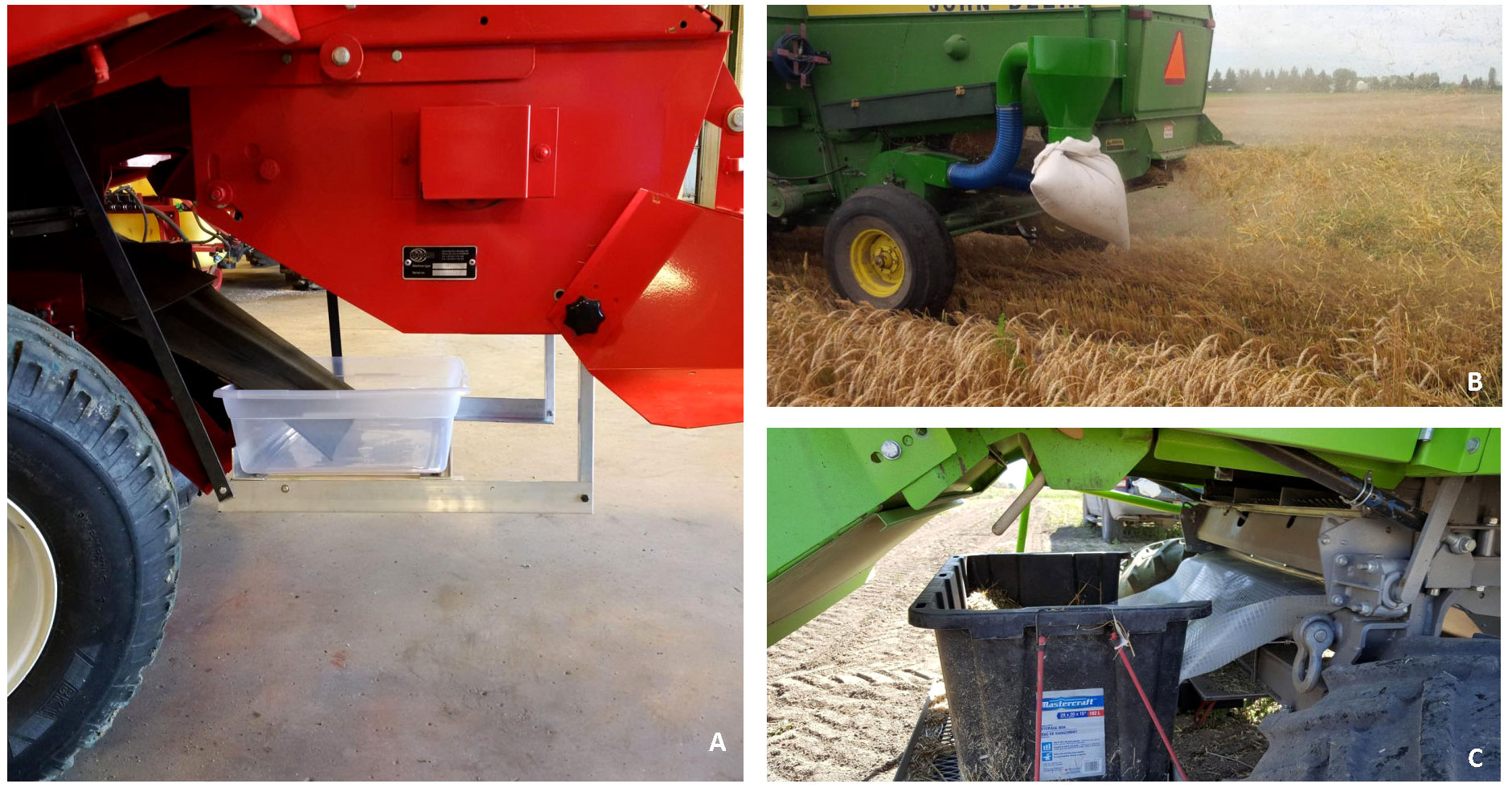
Figure 1 Chaff collection systems used in applicable treatments at (A) Beaverlodge, (B) Lacombe, and (C) Lethbridge. Systems were designed to fit the harvesting equipment available for the study at each location.
The cumulative effects of the treatments were determined after the 3 years of differentiated treatments. In 2020, the final year of the study, all treatments were seeded to a 2× seeding rate of spring wheat, and no herbicides were applied. This strategy allowed examination of the weed communities with no confounding effects of crop types or severely reduced weed populations from in-crop herbicide applications. Treatment 2, a canola–wheat rotation with full in-crop herbicide application regime represents a common crop rotation sequence on the Canadian Prairies. As such, the other treatments were compared with this treatment as a “standard” of many farmers would be doing and the level of weed control that would be expected or desired by producers.
Weed densities were determined approximately 2–3 weeks after crop emergence and prior to in-crop herbicide application, each year in two 0.5-m2 quadrats in each plot. Weeds were counted and identified to species, where possible. Prior to early-cut silage barley harvest, crop and weed biomass (separated by broadleaf and grass weeds) were determined from the same quadrats. For each of the study years, the location of the quadrat was shifted to ensure no confounding effects of biomass removal in the prior year. The biomass samples were dried at 60°C until moisture content stabilized for a dry weight measurement. For the silage barley, the plants were swathed, and the material was removed from the plot at the appropriate time. Grain plots were swathed or left standing until maturity and harvested with plot combines, at which time chaff was collected by treatment when indicated. Seed was cleaned and dockage recorded for each plot. For the initial year of alfalfa growth, it was cut only once; subsequently two to three cuts were harvested each year depending on location and the respective growing conditions, and total dry weight biomass was determined. Alfalfa was terminated at the end of the growing season in 2019 using a mixture of clopyralid (166 g ai ha−1) and glyphosate (445 g ae ha−1).
Weed seed-bank samples were collected in the fall of 2020, after grain harvest. A “W” pattern was utilized to take 12 soil samples per plot to a depth of 8 cm using a circular core sampler with a diameter of 10 cm. Subsamples were bulked into a single sample. Soil was dried at 30°C, sieved, and washed with a 250-μm screen. Large-seeded weeds like wild oat were removed and counted by hand. The remaining sample was mixed with approximately 5 L of potting soil (JiffyMix, Professional Gardener, Calgary, AB) with fertilizer (Harrell’s ProFertilizer [14-14-14: N-P2O5-K2O], Lakeland, FL), placed in trays of 55 cm × 28 cm × 1.3 cm, under light emitting diode (LED) germination lights (Monios-L T5 Grow Lights 120W, Monios-L, online) with a 16-h/8-h light:dark period in a temperature-controlled room at ~25°C. Weed seedlings were identified, counted, and removed. After 3 weeks of growth, the tray was placed into a −18°C freezer for 3 weeks to promote breaking of dormancy through cold stratification. After 3-week cold treatment, samples were removed, mixed by hand, and placed back under the LED germination lights. Recruited seedlings were again identified and removed. Trays were exposed to a final cold treatment for a minimum of 6 weeks, and the growth was repeated once more. The data were converted to seeds m−2 for analysis (O'Donovan et al., 2013).
2.1 Treatment modifications
Treatment modifications because of logistical challenges became necessary at specific locations and years, as were pesticide applications for additional pests. Insecticidal controls were applied in Beaverlodge in 2017 and 2019 for flea beetles (Phyllotreta cruciferae Goeze and Phyllotreta striolata F.), in Lacombe in 2017 and 2019 for flea beetles, and in Lacombe and Lethbridge in 2017 for pea leaf weevil (Sitona lineatus L.). These applications generally allowed us to maintain treatments, but, in some cases, abiotic and biotic stresses required treatment changes. Decisions were made to maintain the integrity of the treatment as much as possible. In Lacombe in 2017, an infestation of pea leaf weevil resulted in a complete decimation of emerging alfalfa seedlings at approximately the second trifoliate stage. In order to not cause further disturbance and stimulation of the seed bank and based on the weather forecast, a 2× seeding rate of alfalfa (18 kg ha−1) was broadcast on the plots prior to a day of rain. It was anticipated that, without incorporation, the alfalfa germination/emergence would be reduced compared with seeded alfalfa, hence the doubling of the seeding rate. This seeding rate also allowed for some additional predation of the alfalfa by remaining pea leaf weevils while allowing the alfalfa to establish. Drought issues resulted in a similarly poor alfalfa stand at Saskatoon and poor establishment for unknown reasons were also observed in Scott, so the same reseeding strategy was utilized. At Lethbridge, a late season drought in 2017 resulted in supplemental seed being broadcast (regular seeding rate) later in the summer as the first cut of alfalfa resulted in unusually high plant mortality. At Saskatoon in 2018, the winter wheat in treatments 8–10 and the winter triticale (× Triticosecale Wittmack) in treatments 11 and 12 suffered a high level of winter kill (Table 2). In treatments 8–10, spring barley grown for grain was substituted to simulate an earlier harvest than typical spring cereals. In treatments 11 and 12, the rotational sequence was switched to silage barley in 2018 and winter triticale being the primary crop in 2019. However, winter kill again compromised the treatment and spring barley for grain was grown in 2019 instead. In Scott in 2018, the winter wheat and winter triticale also showed high levels of winter kill; however, the densities met the industry-recommended plant stand to not reseed and were left to grow for the growing season. Fababean in Carman in 2017 was sprayed out and reseeded later than is typical (June 12) due to incorrect seeding rates at initial seeding. Weed densities in Carman were not assessed in 2020 due to COVID-19 restrictions, and so weed densities in 2020, including wild oat and wild buckwheat, were analyzed across the five other locations.
2.2 Statistical analysis
Data, including wild oat and wild buckwheat densities, grass weed biomass, broadleaf weed biomass, crop biomass, wheat yield, and dockage, were analyzed with the PROC GLIMMIX procedure of SAS, version 9.4 (Littell et al., 2006; SAS Institute, 2013). Treatment was considered a fixed effect, and location and replicate nested in location were considered random effects. Because the locations covered the broad geography of the Canadian Prairies and the desire was to make treatment inferences beyond the study location, it was appropriate to consider location effects and their interactions with the treatments as random (Yang, 2010). Experimental treatment effects were considered fixed. A log-normal distribution was utilized on the basis of Akaike’s corrected information criterion (AICc) (Hurvich and Tsai, 1989) and fit of residuals. A comparison of means was conducted utilizing a Dunnett’s test with the canola–wheat full herbicide regime (treatment 2) used as the control or comparison treatment and a p-value α = 0.05. Data and standard errors were back-transformed for presentation in the original scale.
In consideration of the fact that weed community composition differed among locations,and to determine consistency of treatment efficacy, data were also analyzed for each variable within each location. The same log-normal distribution was utilized, treatments were fixed effects, and replication was a random effect. A comparison of means was conducted as above. A “site compliance” comparison was done by summarizing the number of mean comparisons from the by-location analysis that agreed or disagreed with the same comparison from the combined location analysis mean comparisons to provide descriptive information on the consistency of treatment differences among locations (Harker et al., 2016).
Analysis of wild oat and wild buckwheat densities in the seed bank followed the same procedure as above; however, a negative binomial distribution was utilized to improve the fit of the residuals. Other weed species’ seed-bank densities were analyzed by location as at each location the weed community composition differed. Similarly, analysis of seedling weed densities aside from wild buckwheat and wild oat were conducted by location due to differences in weed community composition between locations. On a few occasions [wild mustard (Sinapis arvensis L.) and false cleavers (hereafter referred to as cleavers) in Saskatoon, cleavers in Scott], normal distributions were used for individual seedling densities, and, on one occasion (henbit (Lamium amplexicaule L.) in Lacombe), a lognormal distribution provided the best model fit, based on AICc and residuals. Similarly, seed-bank densities of most weed species best fit a negative binomial error distribution; however, a log-normal error distribution was used for hempnettle (Galeopsis tetrahit L.) in Lacombe, wild mustard in Lethbridge, cleavers in Saskatoon and Scott, and kochia [Bassia scoparia (L.) A.J. Scott.] in Scott, as well as a normal distribution for wild mustard in Saskatoon.
3 Results
3.1 Notable weather anomalies
Notable divergences in precipitation occurred at Beaverlodge in 2016 and 2020; Lethbridge, Lacombe, and Saskatoon in 2017–2019 (somewhat mitigated in Lethbridge through the use of supplemental irrigation); Scott in 2018; and Carman in 2017, 2018, and 2020 (Table 1). These deviations from the normal may have played a role in densities of various weed species. For example, at Lacombe and the surrounding area in 2016, it was noted as a year that seemed to encourage the growth of wild oat. At Lacombe and Beaverlodge in 2018, it snowed in the second week of September, and, at Lacombe, snow continued until early October. This delayed harvest and reduced the crop quality in those environments (data not shown).
3.2 Weed densities
Wild oat was the common grass weed across locations. Densities in the “standard” comparison treatment averaged 46 m−2. In general, the treatments investigated resulted in either static wild oat densities in comparison with treatment 2 or increases where in-crop herbicides were removed. Wild oat densities in the final year of the rotational study were affected by treatment (p < 0.0001). Densities increased in the canola–wheat rotation with chaff collection at a 1× seeding rate (treatment 4), in the diversified spring annual rotation with chaff collection at a 1× seeding rate (treatment 6), in the diversified spring annual rotation with increased seeding rates (treatment 7), in the diversified life cycle (incorporating winter cereals) combined with chaff collection at a 1× seeding rate (treatment 9), and in the diversified life-cycle treatment describe previously but incorporating chaff collection (treatment 10) (Figure 2). All the listed treatments included no in-crop herbicides. However, at three out of the five locations, in treatments 9 and 10, which included no in-crop herbicide and winter wheat in rotation, densities were not greater than the standard comparison (treatment 2). Interestingly, treatments without in-crop herbicides that included winter triticale or fall rye (Secale cereale L.) in combination with silage barley (treatment 11: silage barley twice plus winter triticale at increased seeding rates; treatment 12: silage barley twice, winter triticale, chaff collection, and increased seeding rate; and treatment 14: silage barley, fall rye, and canola; chaff collection; increased seeding rate) had wild oat densities that were statistically similar to the “standard” treatment where a full in-crop herbicide regime was used. These winter cereals had higher winter survival than winter wheat across locations and were therefore more competitive. At three locations in the diversified spring annual treatment with chaff collection and increased seeding rate (treatment 7), densities were similar compared with that in treatment 2. The alfalfa treatment showed some variability with similar wild oat densities across locations, and, at three individual locations, lower wild oat density at one location and greater wild oat density at one location. Across locations, no treatment was successful in reducing wild oat densities below those observed in treatment 2.
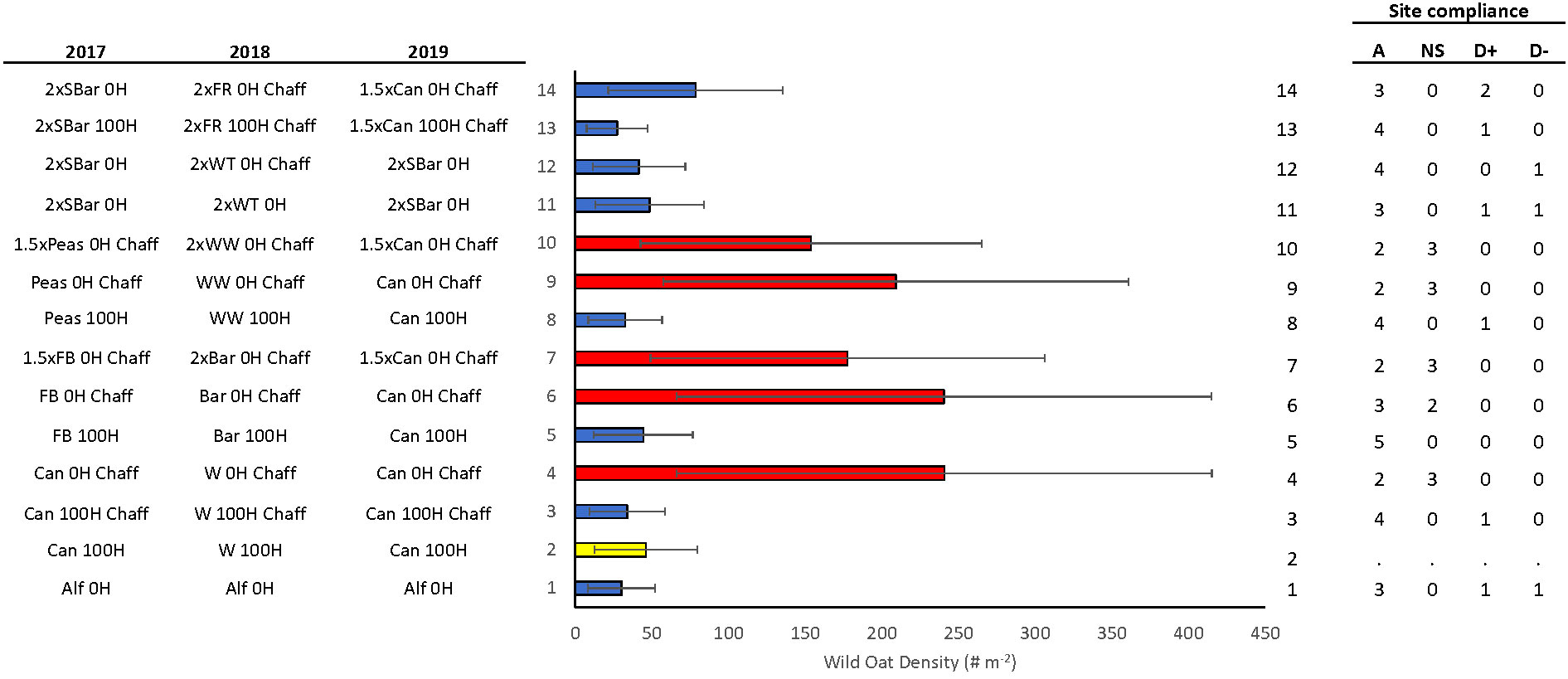
Figure 2 Wild oat density across locations by treatment in the final year of the rotational study. The yellow-highlighted treatment is the canola–wheat rotation with standard seeding rate, full herbicide, and no harvest weed seed control, to which all the other treatments are contrasted. Significant increases in wild oat density are red, whereas significant reductions in densities are green, based on a Dunnett’s comparison of means using an α = 0.05. Error bars indicate standard errors. Site compliance indicates the number of individual locations with Dunnett’s comparison of means that are in agreement with across-location comparisons to the yellow-highlighted treatment: A, agreement; NS, not significant when across-location comparison is significant; D+, significant difference (same pattern) when across-location comparison is not significant; D−, significant difference (opposite pattern) when across-location comparison is not significant. Alf, alfalfa; Can, canola; W, wheat; FB, fababean; Bar, barley (grain); WW, winter wheat; SBar, silage barley; WT, winter triticale; FR, fall rye. If the numbers are present in front of the crop, then they indicate an increased seeding rate. 0H and 100H indicate no or full herbicide regimes, respectively, and if the word “chaff” is present, then it indicates that chaff was collected.
Wild buckwheat was the common broadleaf weed across locations, and densities in the standard comparison treatment (treatment 2) averaged 6 m−2. Wild buckwheat showed little response to the integrated weed management strategies chosen. Although wild buckwheat was affected by treatment (p = 0.0483), no treatments differed in wild buckwheat densities compared with that in treatment 2 (Figure 3). At one out of the five locations, the diversified life cycle rotation with chaff collection, no herbicides, and a baseline seeding rate (treatment 9); the diversified life cycle rotation as described previously but with an increased seeding rate (treatment 10); and the rotation incorporating silage barley, fall rye, and canola with an increased seeding rate, no in-crop herbicide, and chaff collection (treatment 14) all resulted in increases in wild buckwheat densities.
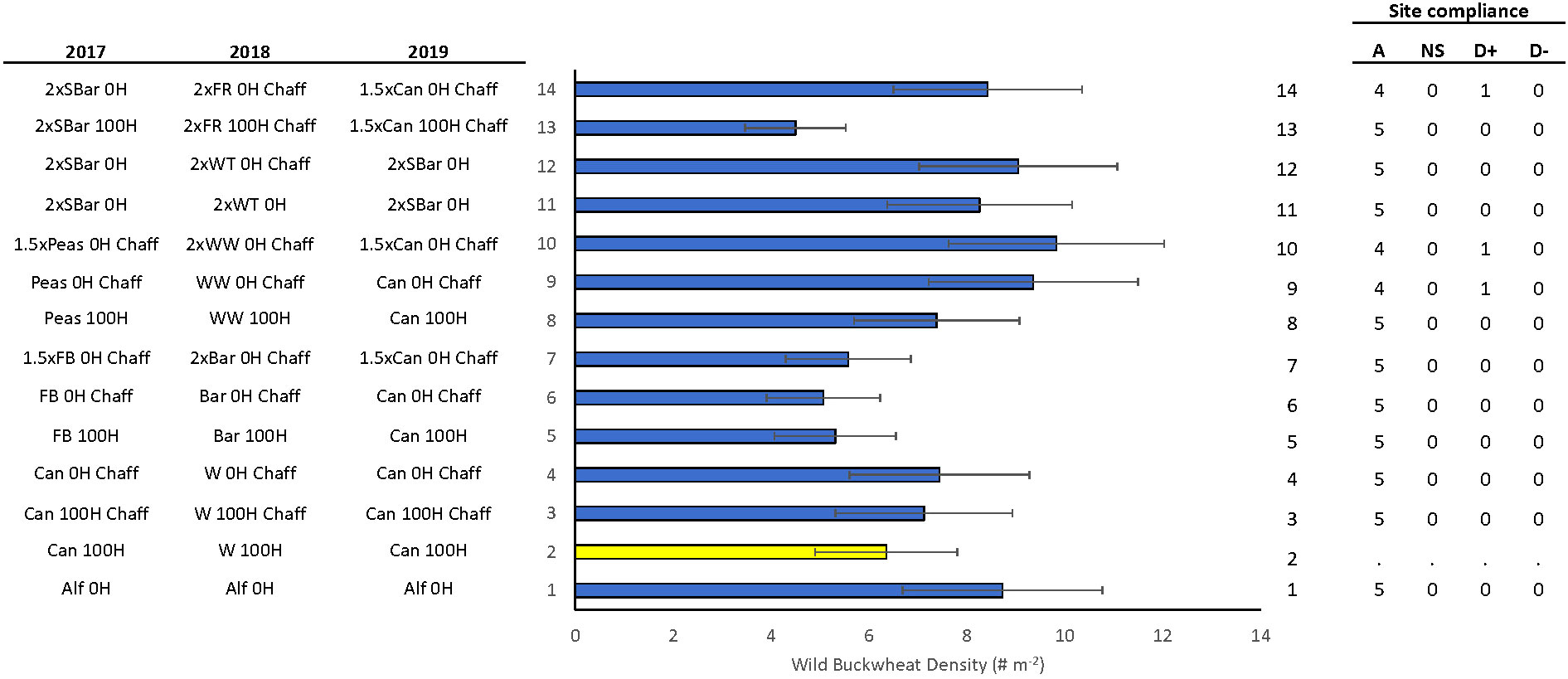
Figure 3 Wild buckwheat density across locations by treatment in the final year of the rotational study. The yellow-highlighted treatment is the canola–wheat rotation with standard seeding rate, full herbicide, and no harvest weed seed control, to which all the other treatments are contrasted. Increased densities are highlighted in red, whereas decreased densities treatment are highlighted in green, based on a Dunnett’s comparison of means using an α = 0.05. Error bars indicate standard errors. Site compliance indicates the number of individual locations with Dunnett’s comparison of means that are in agreement with across-location comparisons to the yellow-highlighted treatment: A, agreement; NS, not significant when across-location comparison is significant; D+, significant difference (same pattern) when across-location comparison is not significant; D−, significant difference (opposite pattern) when across-location comparison is not significant. Alf, alfalfa; Can, canola; W, wheat; FB, fababean; Bar, barley (grain); WW, winter wheat; SBar, silage barley; WT, winter triticale; FR, fall rye. If the numbers are present in front of the crop, then they indicate an increased seeding rate. 0H and 100H indicate no or full herbicide regimes, respectively, and if the word “chaff” is present, then it indicates that chaff was collected.
Weed species chosen as targets in many cases had similar densities among treatments (p > 0.05), or none of the treatments differed in comparison with the standard treatment 2. Only those weed species that were affected by treatment and showed significant differences are presented here. Cleaver density at Beaverlodge was reduced in the diversified life-cycle rotation with a baseline seeding rate and full in-crop herbicides (treatment 8); in the rotation with silage barley twice, in combination with winter triticale, increased seeding rate, and no in-crop herbicide (treatment 11); in the rotation that included silage barley, fall rye, and canola at an increased seeding rate, with full in-crop herbicides and chaff collection (treatment 13) (Supplementary Figure 1A), at Lacombe in the alfalfa treatment (treatment 1); in the standard rotation with no in-crop herbicides but with chaff collection added (treatment 4); in the diversified spring annual rotation with no in-crop herbicides but with chaff collection added (treatment 6); in the diversified spring annual rotation as previously described but with an increased seeding rate (treatment 7); in the diversified life-cycle treatment with full in-crop herbicides (treatment 8); in the rotation with silage barley twice in addition to winter triticale, no in-crop herbicides, and increased seeding rates (treatment 11); in the silage barley and triticale rotation as previously described but including chaff collection (treatment 12); and in the silage barley, fall rye, and canola rotation with full in-crop herbicides, chaff collection, and increased seeding rate (treatment 13) (Supplementary Figure 1B). In Lacombe, cleavers densities increased in the diversified spring annual rotation with no in-crop herbicide, chaff collection, and a baseline seeding rate (treatment 6), and, in Saskatoon, densities increased in the diversified life-cycle rotation with no in-crop herbicides, chaff collection, and an increased seeding rate (treatment 10) (Supplementary Figure 1C) when compared with the standard treatment densities at the respective locations. Many of the treatments showing reduced cleaver densities include full in-crop herbicide regime, chaff collection, increased seeding rates, winter cereals, and silage barley in combination. Increased densities at Saskatoon were observed in treatments with no in-crop herbicide, increased seeding rates, and chaff collection. The perennial alfalfa treatment was variable in terms of efficacy on cleaver density with no difference to the standard at Beaverlodge (although numerically lower), significantly lower density compared with that in the standard at Lacombe and statistically similar although numerically higher density at Saskatoon. Volunteer canola was lower in treatments that did not include canola in rotation the year previous, as well as in some canola-containing rotations where chaff collection was included [treatment 6 (diversified spring annual, no in-crop herbicides, and chaff collection) at all three locations, treatment 9 (diversified life cycle, no in-crop herbicide, and chaff collection) at Lacombe and Scott, and numerous treatments at Scott] (Supplementary Figure 2). Kochia densities at Lethbridge were only reduced compared with that in the standard in the diversified life-cycle treatment with no in-crop herbicides, chaff collection, and an increased seeding rate (treatment 10), although no other treatments increased densities, even in the absence of herbicides (Supplementary Figure 3A). Wild mustard at Saskatoon was reduced in the perennial alfalfa (treatment 1), as well as the diversified spring annual treatment with no in-crop herbicide, chaff collection, and increased seeding rates (treatment 7), and the diversified life-cycle treatment with no in-crop herbicide, chaff collection, and increased seeding rates (treatment 10), whereas densities increased in the standard canola–wheat rotation with full in-crop herbicide but chaff collection added (treatment 3) (Supplementary Figure 3B). Shepherd’s purse [Capsella bursa-pastoris (L.) Medik.] densities at Scott were greater in the standard canola–wheat rotation with no in-crop herbicide and with chaff collection (treatment 4); in the diversified life cycle with no in-crop herbicide, chaff collection, and an increased seeding rate (treatment 10); and in the silage barley and triticale rotation with no in-crop herbicide but an increased seeding rate (treatment 11) (Supplementary Figure 3C).
3.3 Weed and crop biomass
Weed biomass was separated by grass and broadleaf weeds, and, in both categories, weed biomass was affected by the cropping system treatments. Grass weed biomass was influenced by the treatment (p < 0.001) and, in comparison with the canola–wheat standard treatment, increased in treatment 6, a diversified spring annual treatment with no in-crop herbicides, baseline seeding rates, and chaff collection (Figure 4). In two locations, the alfalfa treatments reduced grass weed biomass, as did treatment 8, a more diversified annual cropping rotation. There were single locations where the winter cereals and silage barley in rotation reduced grass weed biomass. Across locations, differences from the standard canola–wheat rotation were limited; most treatments did not differ in grass weed biomass.
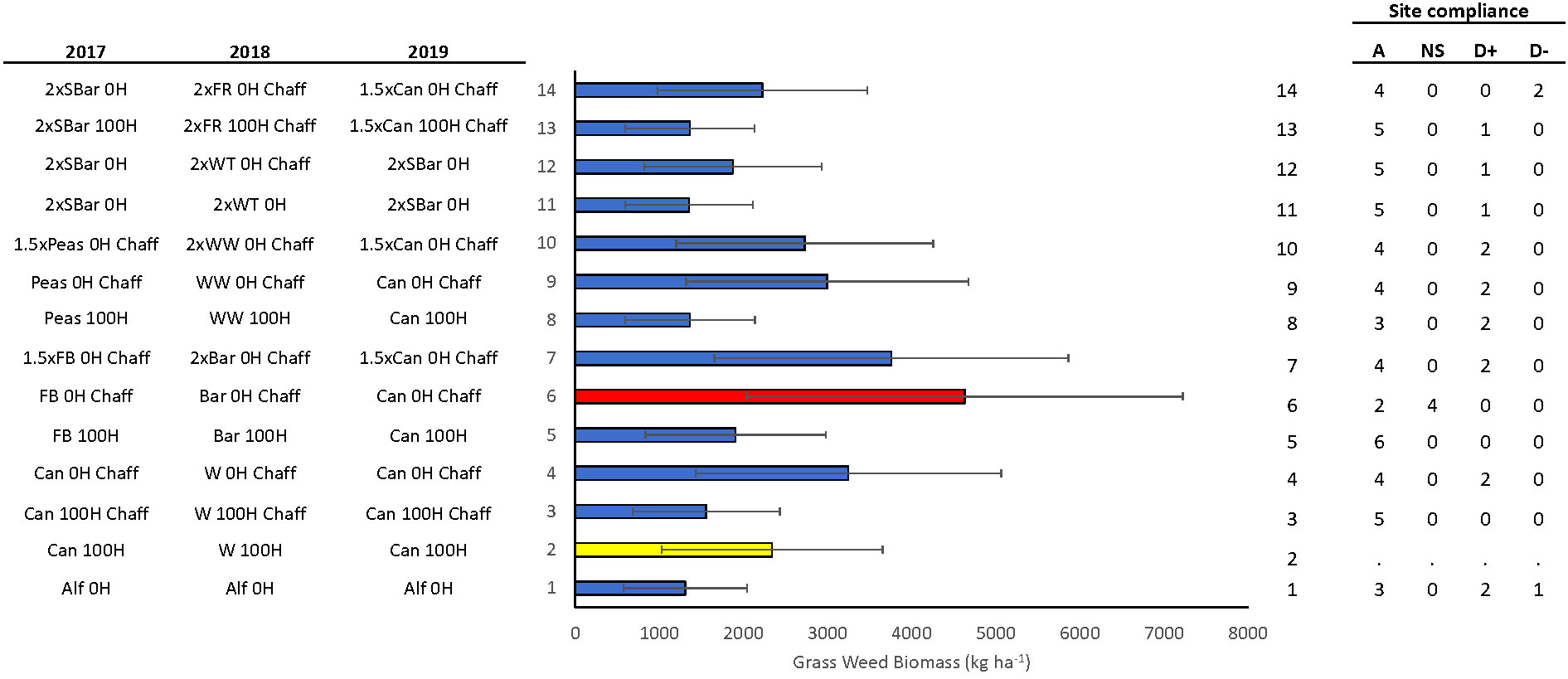
Figure 4 Grass weed biomass across locations by treatment in the final year of the rotational study. The yellow-highlighted treatment is the canola–wheat rotation with standard seeding rate, full herbicide, and no harvest weed seed control, to which all the other treatments are contrasted. Significant increases in grass weed biomass are highlighted in red, whereas significant reductions in grass weed biomass are highlighted in green, based on a Dunnett’s comparison of means using an α = 0.05. Error bars indicate standard errors. Site compliance indicates the number of individual locations with Dunnett’s comparison of means that are in agreement with across-location comparisons to the yellow-highlighted treatment: A, agreement; NS, not significant when across-location comparison is significant; D+, significant difference (same pattern) when across-location comparison is not significant; D−, significant difference (opposite pattern) when across-location comparison is not significant. Alf, alfalfa; Can, canola; W, wheat; FB, fababean; Bar, barley (grain); WW, winter wheat; SBar, silage barley; WT, winter triticale; FR, fall rye. If the numbers are present in front of the crop, then they indicate an increased seeding rate. 0H and 100H indicate no or full herbicide regimes, respectively, and if the word “chaff” is present, then it indicates that chaff was collected.
Broadleaf weed biomass, while affected by treatment (p = 0.0203), showed no differences from the standard canola–wheat treatment when analyzed across locations (Figure 5). Within locations, however, biomass was reduced in treatment 4 (canola–wheat, baseline seeding rate, no herbicide, chaff collection) at three locations and reduced in treatment 6 (diversified spring annual, no in-crop herbicide, chaff collection), treatment 9 (diversified life cycle, no in-crop herbicide, chaff collection), and treatment 10 (same as previous but with increased seeding rate) at one location. At one location, biomass was also reduced in the perennial alfalfa treatment.
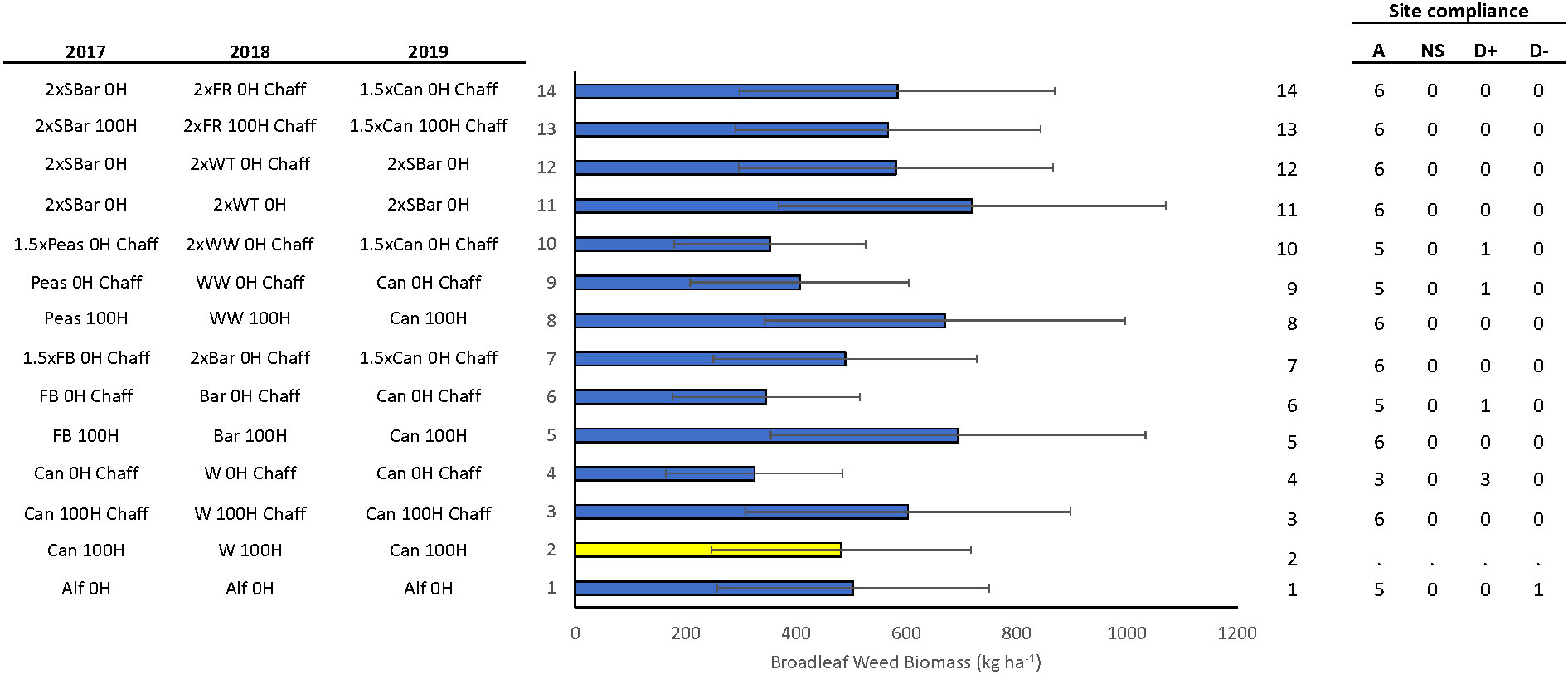
Figure 5 Broadleaf weed biomass across locations by treatment in the final year of the rotational study. The yellow-highlighted treatment is the canola–wheat rotation with standard seeding rate, full herbicide, and no harvest weed seed control, to which all the other treatments are contrasted. Significant increases in broadleaf weed biomass are red, whereas significant reductions in biomass are green, based on a Dunnett’s comparison of means using an α = 0.05. Error bars indicate standard errors. Site compliance indicates the number of individual locations with Dunnett’s comparison of means that are in agreement with across-location comparisons to the yellow-highlighted treatment: A, agreement; NS, not significant when across-location comparison is significant; D+, significant difference (same pattern) when across-location comparison is not significant; D−, significant difference (opposite pattern) when across-location comparison is not significant. Alf, alfalfa; Can, canola; W, wheat; FB, fababean; Bar, barley (grain); WW, winter wheat; SBar, silage barley; WT, winter triticale; FR, fall rye. If the numbers are present in front of the crop, then they indicate an increased seeding rate. 0H and 100H indicate no or full herbicide regimes, respectively, and if the word “chaff” is present, then it indicates that chaff was collected.
Wheat biomass in 2020 was affected by cropping system treatment (p < 0.0001). When compared with the standard treatment among locations, wheat biomass was reduced in treatment 4 (canola wheat, no in-crop herbicides, chaff collection), treatment 6 (diversified spring annual, no in-crop herbicide, chaff collection), treatment 7 (same as previous but with increased seeding rate), treatment 9 (diversified life cycle, no in-crop herbicide, chaff collection), and treatment 10 (same as previous but with increased seeding rate) (Figure 6). However, treatment 4 was similar to the standard treatment at 50% of the locations when analyzed separately, and treatment 6 was not different at 33% of the locations, treatment 7 at 50% of the locations, treatment 9 at 66% of the locations, and treatment 10 at 83% of the locations. At a single location, a greater crop biomass was observed in the perennial alfalfa treatment; in treatment 3, which was the standard system plus chaff collection; and in treatment 8, which was a diversified annual crop rotation with full herbicide applications.
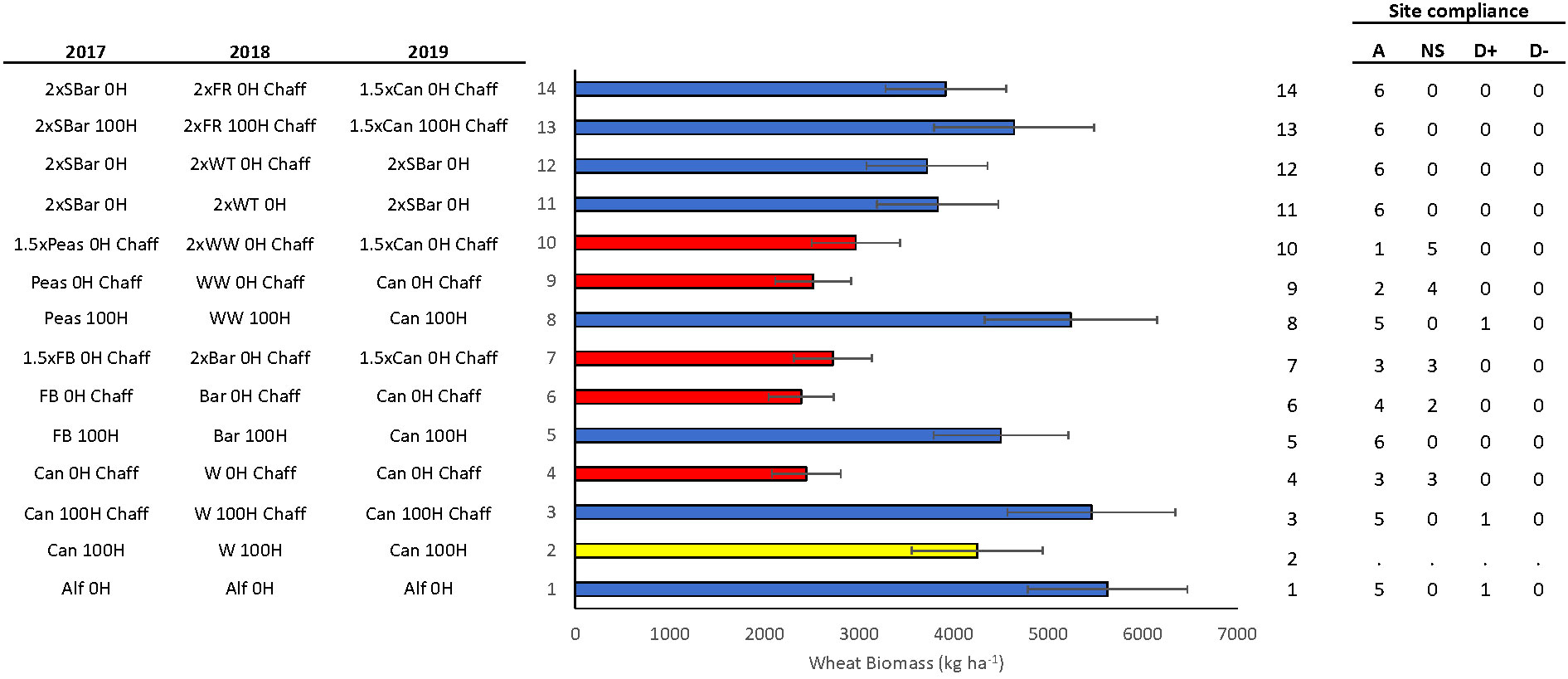
Figure 6 Wheat crop biomass across locations by treatment in the final year of the rotational study. The yellow-highlighted treatment is the canola–wheat rotation with standard seeding rate, full herbicide, and no harvest weed seed control, to which all the other treatments are contrasted. Significant biomass reductions are in red, whereas significant biomass increases are in green, based on a Dunnett’s comparison of means using an α = 0.05. Error bars indicate standard errors. Site compliance indicates the number of individual locations with Dunnett’s comparison of means that are in agreement with across-location comparisons to the yellow-highlighted treatment: A, agreement; NS, not significant when across-location comparison is significant; D+, significant difference (same pattern) when across-location comparison is not significant; D−, significant difference (opposite pattern) when across-location comparison is not significant. Alf, alfalfa; Can, canola; W, wheat; FB, fababean; Bar, barley (grain); WW, winter wheat; SBar, silage barley; WT, winter triticale; FR, fall rye. If the numbers are present in front of the crop, then they indicate an increased seeding rate. 0H and 100H indicate no or full herbicide regimes, respectively, and if the word “chaff” is present, then it indicates chaff was collected.
3.4 Crop yield and quality
Wheat yield in the final year of the experiment was affected by treatment (p < 0.0001). When compared with the standard canola–wheat rotation, a final-year yield was reduced in treatment 4 (canola wheat, no in-crop herbicides, chaff collection), treatment 6 (diversified spring annual, no in-crop herbicide, chaff collection), treatment 7 (same as previous but with increased seeding rate), treatment 9 (diversified life cycle, no in-crop herbicide, chaff collection), and treatment 10 (same as previous but with increased seeding rate) (Figure 7), all of which did not receive an in-crop herbicide. Again, with each of these treatments, there were individual locations where there were no differences in wheat yield. Yield was greater at one location in the standard canola–wheat rotation where chaff collection was added (treatment 3). Treatments with no in-crop herbicide that included silage barley and a competitive winter cereal produced yields similar to the standard canola–wheat rotation.
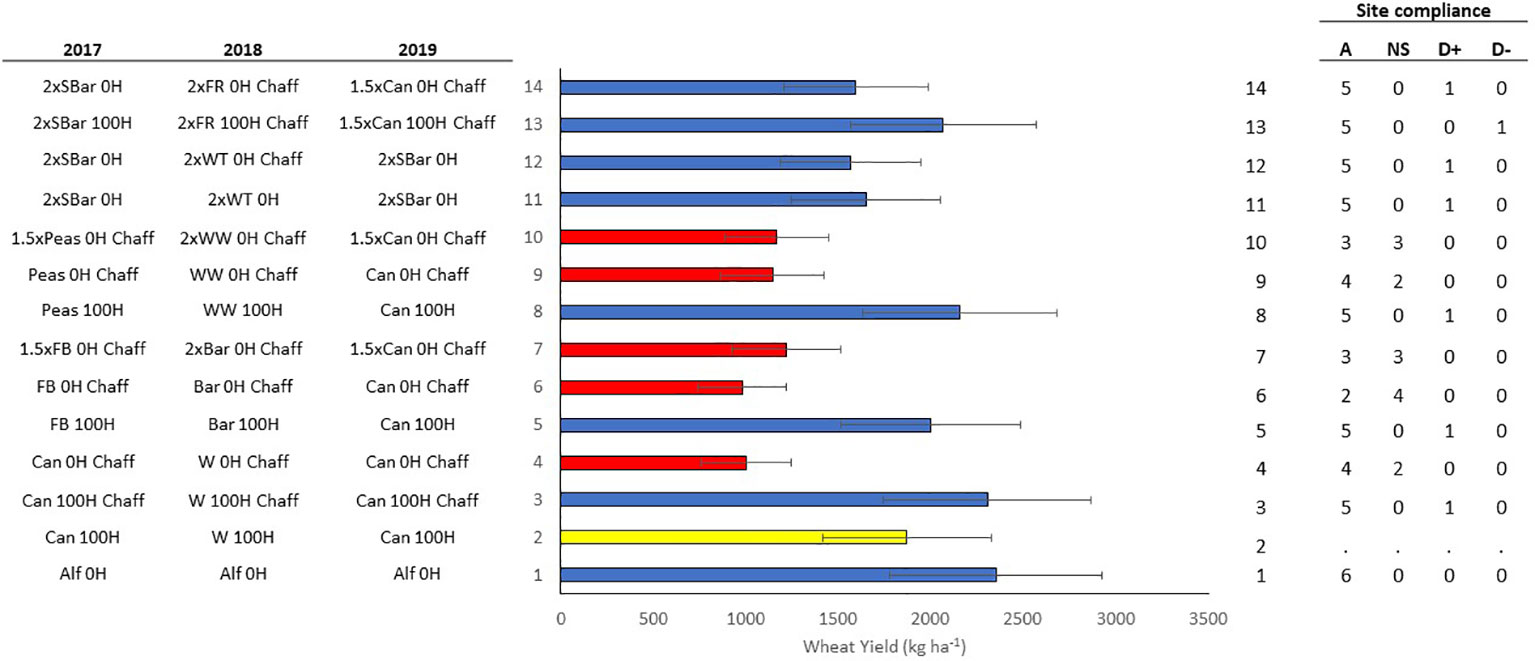
Figure 7 Wheat yield across locations by treatment in the final year of the rotational study. The yellow-highlighted treatment is the canola–wheat rotation with standard seeding rate, full herbicide, and no harvest weed seed control, to which all the other treatments are contrasted. Significant yield reductions are in red, whereas significant yield increases are in green, based on a Dunnett’s comparison of means using an α = 0.05. Error bars indicate standard errors. Site compliance indicates the number of individual locations with Dunnett’s comparison of means that are in agreement with across-location comparisons to the yellow-highlighted treatment: A, agreement; NS, not significant when across-location comparison is significant; D+, significant difference (same pattern) when across-location comparison is not significant; D−, significant difference (opposite pattern) when across-location comparison is not significant. Alf, alfalfa; Can, canola; W, wheat; FB, fababean; Bar, barley (grain); WW, winter wheat; SBar, silage barley; WT, winter triticale; FR, fall rye. If the numbers are present in front of the crop, then they indicate an increased seeding rate. 0H and 100H indicate no or full herbicide regimes, respectively, and if the word “chaff” is present, it indicates that chaff was collected.
Dockage was also affected by cropping system treatment (p < 0.0001). In comparison with the standard canola–wheat rotation, dockage was reduced among locations in the perennial alfalfa treatment and in treatment 3, which has the same treatments as the standard rotation but includes chaff collection (Figure 8). Density differences in these treatments were significant at half and one-third of locations, respectively, in the by-location analyses. Treatment 13, which included chaff collection in 2 years, also had lower dockage in 33% of locations, although the across-location comparison was not significant.
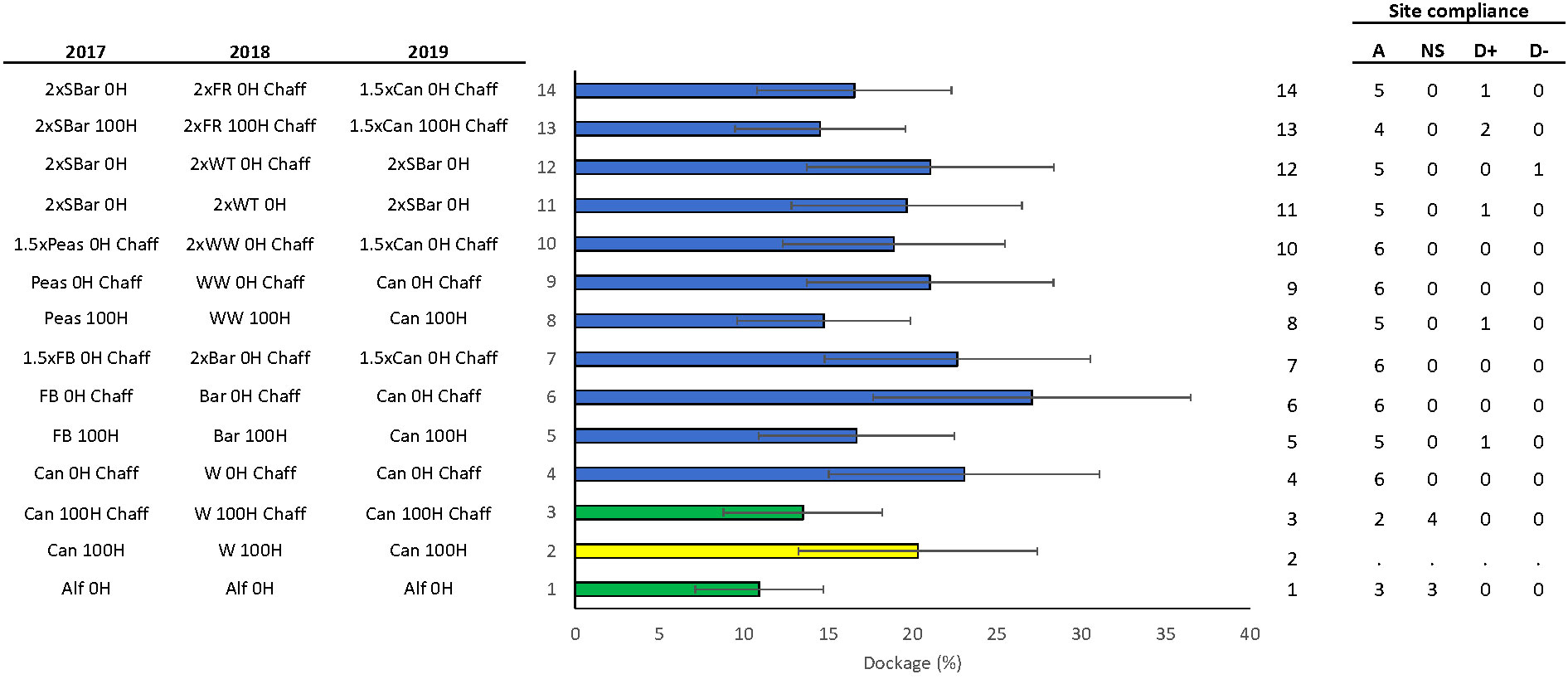
Figure 8 Dockage at wheat harvest across locations by treatment in the final year of the rotational study. The yellow-highlighted treatment is the canola–wheat rotation with standard seeding rate, full herbicide, and no harvest weed seed control, to which all the other treatments are contrasted. Significant increases in dockage are red, whereas significant decreases in dockage are green, based on a Dunnett’s comparison of means using an α = 0.05. Error bars indicate standard errors. Site compliance indicates the number of individual locations with Dunnett’s comparison of means that are in agreement with across-location comparisons to the yellow-highlighted treatment: A, agreement; NS, not significant when across-location comparison is significant; D+, significant difference (same pattern) when across-location comparison is not significant; D−, significant difference (opposite pattern) when across-location comparison is not significant. Alf, alfalfa; Can, canola; W, wheat; FB, fababean; Bar, barley (grain); WW, winter wheat; SBar, silage barley; WT, winter triticale; FR, fall rye. If the numbers are present in front of the crop, then they indicate an increased seeding rate. 0H and 100H indicate no or full herbicide regimes, respectively, and if the word “chaff” is present, it indicates that chaff was collected.
3.5 Weed seed-bank densities
Several IWM treatments reduced wild oat seed-bank densities compared with the standard canola–wheat rotation (p < 0.0001) (Figure 9). Across locations, reductions in the wild oat seed-bank density were observed in treatment 3 (chaff collection added to the standard treatment), treatment 5 (diversified spring annual rotation with full herbicide regime), treatment 8 (addition of winter wheat to spring annual rotation with full herbicide regime), and treatments 11 and 12 (2 years of 2× silage barley plus 2× winter triticale both with and without chaff collection) (Figure 9). Density differences in these treatments were significant across locations, but not at the majority of individual locations when analyzed separately. Conversely, wild oat seed-bank densities were greater than in the standard in treatment 4 (canola–wheat standard with no in-crop herbicide but with chaff collection), treatments 6 and 7 (diversified spring annual rotation with no in-crop herbicide regardless of seeding rate), and treatments 9 and 10 (addition of winter wheat to a diversified annual crop rotation with no in-crop herbicide regardless of seeding rate) (Figure 9). The perennial alfalfa rotation and the silage barley, fall rye, and canola rotations, both with and without in-crop herbicide, had similar wild oat seed-bank densities to the standard treatment.
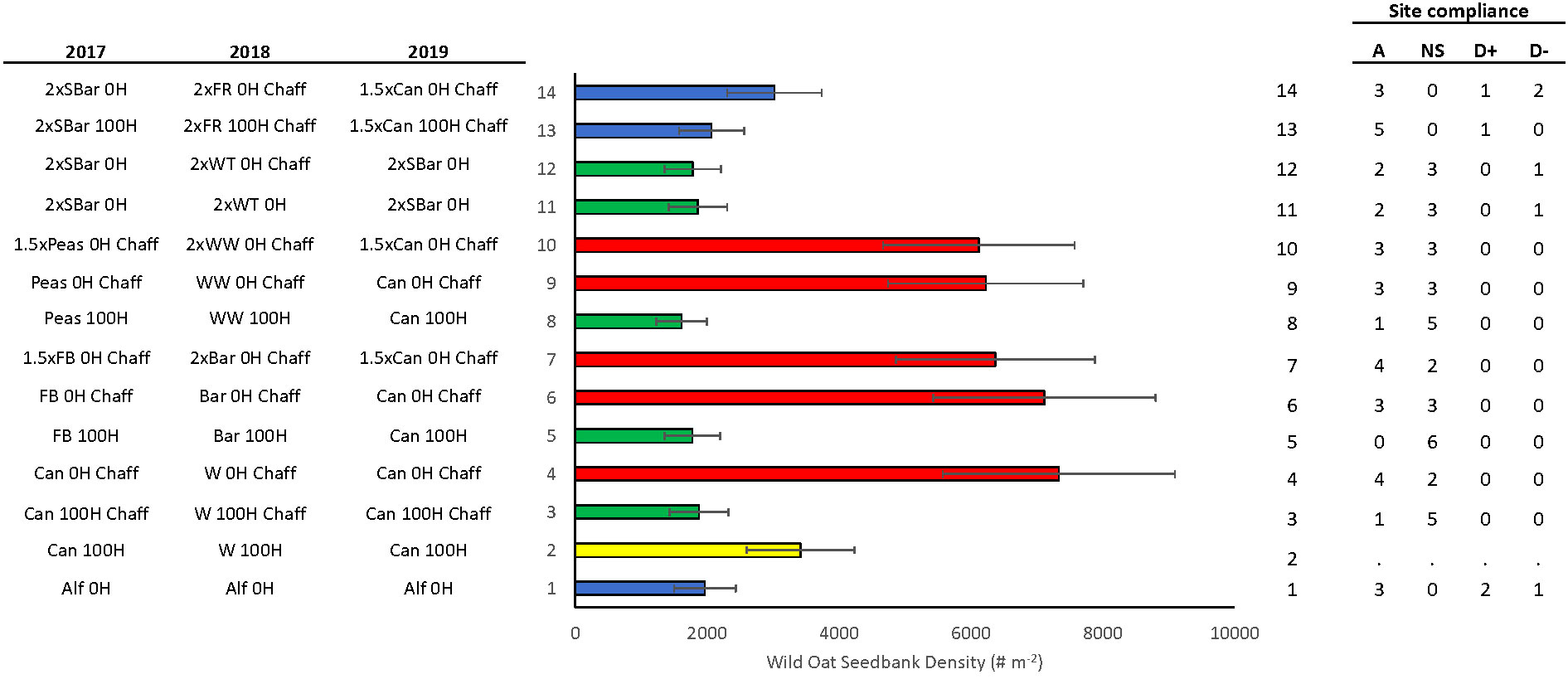
Figure 9 Wild oat seed-bank density across locations by treatment in the final year of the rotational study. The yellow-highlighted treatment is the canola–wheat rotation with standard seeding rate, full herbicide, and no harvest weed seed control, to which all the other treatments are contrasted. Significant increases in the wild oat seed bank are red, whereas significant reductions in the wild oat seed bank are green, based on a Dunnett’s comparison of means using an α = 0.05. Error bars indicate standard errors. Site compliance indicates the number of individual locations with Dunnett’s comparison of means that are in agreement with across-location comparisons to the yellow-highlighted treatment: A, agreement; NS, not significant when across-location comparison is significant; D+, significant difference (same pattern) when across-location comparison is not significant; D−, significant difference (opposite pattern) when across-location comparison is not significant. Alf, alfalfa; Can, canola; W, wheat; FB, fababean; Bar, barley (grain); WW, winter wheat; SBar, silage barley; WT, winter triticale; FR, fall rye. If the numbers are present in front of the crop, then they indicate an increased seeding rate. 0H and 100H indicate no or full herbicide regimes, respectively, and if the word “chaff” is present, then it indicates that chaff was collected.
Wild buckwheat seed-bank densities were also affected by treatment (p = 0.0006) but differed from the standard canola–wheat rotation only in the perennial alfalfa treatment where wild buckwheat densities were higher (Figure 10). However, this was only the case at one-third of the locations when analyzed separately. Other locations showed no differences compared with that in the standard treatment.
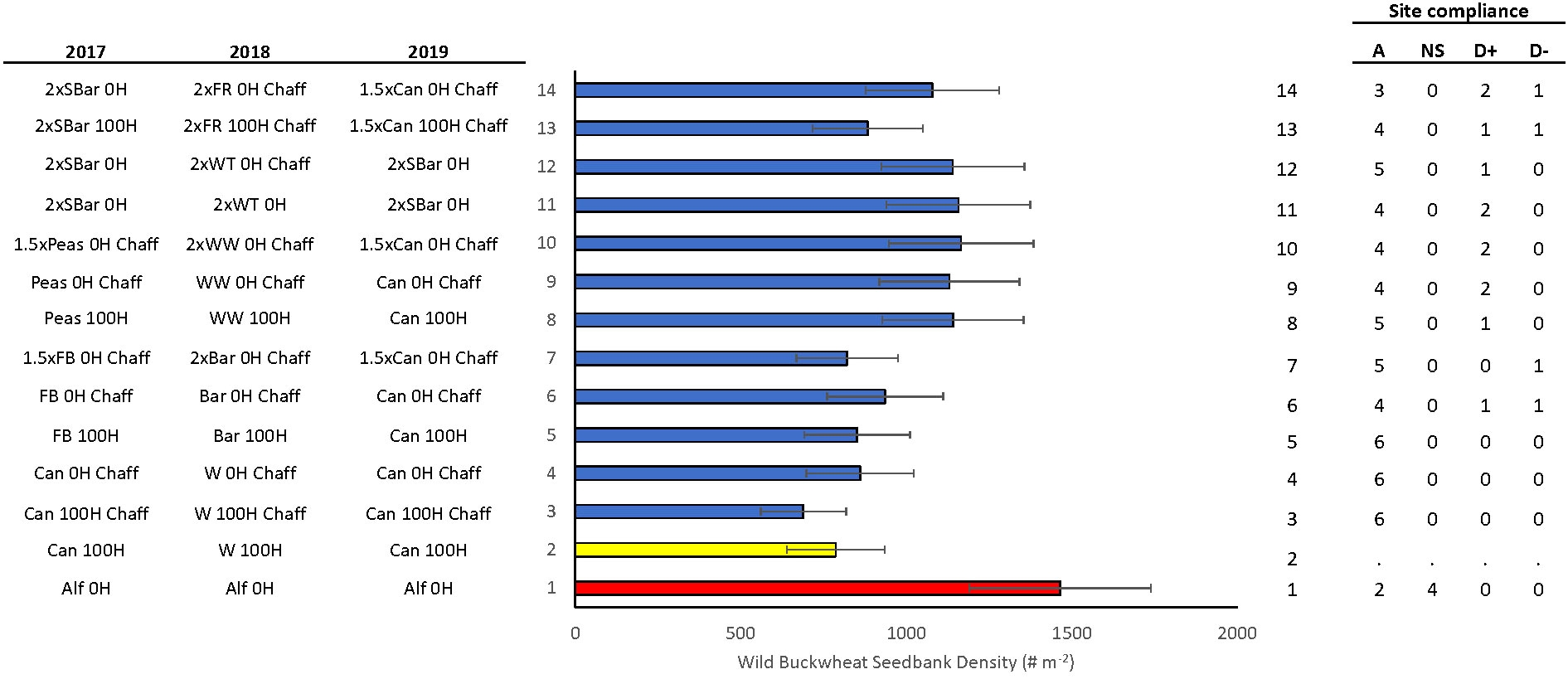
Figure 10 Wild buckwheat seed-bank density across locations by treatment in the final year of the rotational study. The yellow-highlighted treatment is the canola–wheat rotation with standard seeding rate, full herbicide, and no harvest weed seed control, to which all the other treatments are contrasted. Significant increases in wild buckwheat seed-bank densities are red, whereas significant decreases in seed-bank densities are green, based on a Dunnett’s comparison of means using an α = 0.05. Error bars indicate standard errors. Site compliance indicates the number of individual locations with Dunnett’s comparison of means that are in agreement with across-location comparisons to the yellow-highlighted treatment: A, agreement; NS, not significant when across-location comparison is significant; D+, significant difference (same pattern) when across-location comparison is not significant; D−, significant difference (opposite pattern) when across-location comparison is not significant. Alf, alfalfa; Can, canola; W, wheat; FB, fababean; Bar, barley (grain); WW, winter wheat; SBar, silage barley; WT, winter triticale; FR, fall rye. If the numbers are present in front of the crop, then they indicate an increased seeding rate. 0H and 100H indicate no or full herbicide regimes, respectively, and if the word “chaff” is present, then it indicates chaff was collected.
At individual locations, weed seed-bank density was often similar among treatments or compared with the standard treatment. However, a few individual species and location combinations did have effects that warrant discussion. Lambsquarters (Chenopodium album L.) seed-bank densities at Beaverlodge were reduced in treatment 8 (winter wheat in a diversified annual system with full herbicide regime) and increased in treatments 12 and 14 (silage barley and winter triticale, and silage barley, fall rye, and canola at increased seeding rates with no in-crop herbicide and chaff collection where possible) compared with the standard (Supplementary Figure 4A). Redroot pigweed (Amaranthus retroflexus L.) seed-bank densities in Carman were reduced in treatment 1 (alfalfa), treatment 4 (no in-crop herbicide but chaff collection in canola–wheat), treatment 5 (diversified spring annual, full herbicide regime), treatment 6 (diversified spring annual, no herbicide regime), treatment 7 (diversified spring annual, increased seeding rate, no in-crop herbicide), treatment 9 (winter wheat with spring annuals, no in-crop herbicide, chaff collection), and treatment 10 (winter wheat rotation with increased seeding rates, no in-crop herbicide, chaff collection) (Supplementary Figure 4B). Green and yellow foxtail (Setaria viridis (L.) P. Beauv and Setaria pumila (Poir.) Roem. & Schult, respectively) seed-bank densities at Carman were combined due to challenges differentiating the species at the one-leaf stage and analyzed as foxtail species. Foxtail species densities were reduced in treatment 1 (alfalfa), treatment 4 (no in-crop herbicide but chaff collection in canola–wheat), treatment 5 (diversified spring annual, full herbicide regime), treatment 6 (diversified spring annual, no herbicide regime), treatment 7 (diversified spring annual, increased seeding rate no in-crop herbicide), treatment 9 (winter wheat with spring annuals, no in-crop herbicide, chaff collection), treatment 10 (winter wheat rotation with increased seeding rates, no in-crop herbicide, chaff collection), and treatment 11 (increased seeding rates of silage barley and winter triticale with no in-crop herbicide applications) (Supplementary Figure 4C) compared with the standard. Cleaver seed-bank density was affected by the treatments at both Lacombe (Supplementary Figure 5A) and Saskatoon (Supplementary Figure 5B). In Lacombe, densities were reduced compared with that in the standard in the alfalfa rotation, in the diversified spring annual rotation with no herbicide but with chaff collection (treatment 6), in the winter wheat treatment with full herbicide application (treatment 8), and in the silage barley-fall rye-canola rotation with full in-crop herbicide regime (treatment 13). In Saskatoon, cleaver seed-bank densities were greater than the standard in the diversified spring annual rotation with no in-crop herbicide and chaff collection (treatment 6). Seed-bank densities were substantially higher in Lacombe than in Saskatoon. Finally, wild mustard seed-bank densities were over double the standard in Saskatoon in treatment 13, which is the silage barley-fall rye-canola rotation with full in-crop herbicide regime (Supplementary Figure 5C).
4 Discussion
4.1 Wild oat and grassy weeds
Management of wild oat densities was possible, even without in-crop herbicides, but it was generally less effective than in previous studies on the Canadian prairies (Harker et al., 2016). In previous studies, treatments of 2 years of increased seeding rate and early cut silage in rotation with a winter cereal or through the use of a spring annual crop with full in-crop herbicide application, regardless of which winter cereal was utilized, maintained relatively low wild oat densities (Harker et al., 2016). In the current study, however, wild oat densities were maintained at an acceptable level when a competitive winter cereal was utilized or when herbicides were used in-crop in all 3 years. Previous research also showed that diversifying rotations and utilizing increased crop seeding rates were beneficial for managing wild oat (O'Donovan et al., 2000; Harker et al., 2009). In this study, diversifying rotations to spring annuals or including a winter cereal was not enough to forego in-crop herbicides while maintaining or reducing wild oat seed densities. This result agrees with recent results from Benaragama et al. (2022), where crop diversification strategies in which spring annual crops were included in rotation did not improve management of wild oat.
Treatments were compared with the standard canola–wheat rotation, but not to all other treatments. Treatments 6 and 7 and treatments 9 and 10 differed between each other by an increased seeding rate. The higher seeding rate treatments had numerically fewer wild oat; however, all of these treatments still had greater densities than the standard treatment. Similarly, using Lacombe as an example, while wild oat densities were maintained compared with that in the standard rotation, densities around 200 wild oat m−2 (160 m−2 in the standard treatment) (data not shown) would not be considered a successfully managed population by most producers. Densities were lower in the Harker et al. (2016) study than in the current study; differences between the studies include the inclusion of a weed establishment year with no herbicides in the current study and the utilization of canola in the final year with a full herbicide regime. The herbicide application in canola in 2014 in the study by Harker et al. (2016), in combination with the competitiveness of the canola crop itself, likely restricted wild oat growth in the final year of their study. In addition, the establishment year allowed nearly full seed production for the wild oat in the current study, increasing the densities in comparison with that in the study by Harker et al. (2016). It is worth noting, however, the greater success of the IWM strategies at lower wild oat densities reported by Harker et al. (2016). Most farmers are likely to begin incorporating IWM strategies when they are out of other options; this is typically when weed densities have already risen, in some cases, due to resistance or due to other failures in previous control strategies. Our study results and on-location observations suggests that the adoption of IWM strategies at that time may reduce the impact or reliability of those strategies in reducing the population; farmers are more likely to find success with these strategies if they start incorporating them when weed densities are lower. In the Canadian Prairies, a substantial rise in herbicide-resistant wild oat to the herbicide groups available for selective in-crop applications in many crops has been observed (Beckie et al., 2020). This study contrasts with canola–wheat rotations with full in-crop herbicides in regions where populations can be found where the in-crop herbicides would have limited to no efficacy (Beckie et al., 2020). This result may improve the perception and acceptability of some of the less effective IWM strategies.
It is known that winter cereals generally suppress wild oat better than spring cereals (Brown, 1953; Thurston, 1962; Beres et al., 2010); however, our results suggest the importance of successful crop establishment. Establishment has also been shown to impact competitiveness in spring cereal crops (O’Donovan et al., 2005). Poor stand establishment of winter wheat has been one of the impediments to the increased adoption of the crop in Western Canada (Beres et al., 2016) and, when poorly established, also allows weeds the opportunity to flourish. More winter hardy (and therefore more competitive) winter crops such as fall rye or winter triticale provide alternatives; however, their acreage and market demand are lower (Statistics Canada, 2023).
Grass weed biomass was composed predominantly of wild oat when averaged across locations, based on weed densities and researcher observations. Foxtail species made up a substantial proportion of grass weed biomass at the Manitoba location. Grass weed biomass was notably higher in this study compared with recent IWM studies on wild oat (Harker et al., 2016). Fewer treatments in the current study showed an increase or decrease in wild oat biomass compared with that in the standard canola–wheat rotation; however, biomass and variability in biomass was also larger, possibly impacting the number of significant comparisons. Grass weed biomass across locations increased when diversification of the spring annual crop rotation was utilized alone; however, the combination of increased seeding rates and diversification of rotation did help. It was possible to maintain grass weed biomass with 3 years of no herbicides, by utilizing winter cereals, early cut silage barley chaff collection, and increased seeding rates, similar to other recent studies (Harker et al., 2016). This provides opportunity to reduce reliance on in-crop grass herbicides, of which there are few options for rotating herbicide modes of action. This, in turn, reduces selection for herbicide resistance (Powles and Yu, 2010) to those in-crop herbicides as they are being utilized less. However, the level of observed control at some locations in these treatments that would allow elimination of in-crop herbicides may not have been considered sufficient by producers.
Several IWM strategies in the current study maintained the wild oat seed bank at similar levels to the standard canola–wheat rotation with full in-crop herbicides. A reduction in the seed bank was observed through chaff collection alone, which is unexpecoted as wild oat was identified as a poor target for HWSC due to low levels of seed retention at harvest (Tidemann et al., 2016; Tidemann et al., 2017). In the seed bank, however, in contrast to biomass and seed densities, we observed the benefits of rotation diversification when herbicides were applied, similar to that reported by O'Donovan et al. (2013) and Harker et al. (2009), although the latter reported the effects on weed seed production rather than the seed bank. We also observed a benefit of 2 years of early cut silage barley similar to what has been previously reported (Harker et al., 2003), particularly in combination with increased seeding rates of a competitive winter cereal (Harker et al., 2016). Interestingly, the wild oat seed-bank densities were only maintained at the same level as the standard in the treatments where there was 1 year of silage barley, compared with 2 years of silage barley (treatments 13 and 14 vs. treatments 11 and 12; Figure 9). This is consistent with Benaragama et al. (2022) where, in a flax-based rotation where a single year of silage barley was employed, there was no obvious benefit when compared with that in the spring annual grain crop. The alfalfa treatment also provided 3 years of no grass herbicides while maintaining the wild oat seed bank, even reducing the seed bank at two of the five locations. The wild oat seed-bank results are in agreement with those of Harker et al. (2016): diverse crop life cycles, and strategic employment of early-cut silage barley, increased seeding rates, and, in our case, incorporation of HWSC, can reduce wild oat growth and seed production enough to effectively manage wild oat seed banks. Seed dormancy and the persistent nature of wild oat seed banks mean that effects of this management may not be immediately apparent in seedling densities, even when the treatment effect is present (Harker et al., 2016; Selig et al., 2022). Long-term studies are needed to fully elucidate the long-term impact of IWM strategies.
4.2 Wild buckwheat
Wild buckwheat was selected as a common broadleaf weed due to its prevalence across the Canadian Prairies (Leeson, 2016; Leeson et al., 2017; Leeson et al., 2019). However, across locations, populations were low. With none of the treatments showing a density difference to the standard canola–wheat rotation, it becomes a question of whether all the treatments are equally effective or equally ineffective for wild buckwheat management. Blackshaw and Lindwall (1995) showed that, in a fallow system, tillage could be effective at managing wild buckwheat, but herbicides alone often did not, and that control was typically optimized in systems where tillage and herbicides were combined. This agrees with the idea of “many little hammers” being incorporated into an IWM system (Liebman and Gallandt, 1997). However, in Western Canada, there has been a substantial shift to no-till or minimum tillage production systems. Some of the strategies that we employed including rotational diversity, silage barley, increased seeding rates, and HWSC did not appear to be overly effective additions to wild buckwheat management. Previous research has shown benefits of increased seeding rates and narrowing row spacing on weed communities that included wild buckwheat in dry bean (Blackshaw et al., 2000), but the benefit of increased planting density was not apparent in the crops utilized in our study. Burton et al. (2017) also identified wild buckwheat as a good target for HWSC, yet clear benefits of chaff collection were not apparent in the current study. Low population densities may have reduced our ability to measure differences between treatments. From the seed-bank perspective, wild buckwheat densities were increased in the perennial alfalfa treatment. This was unexpected as the repeated cuts of alfalfa were expected to reduce seed production in the species. Why this treatment showed increased seed-bank densities is unclear. As only two of the locations had higher wild buckwheat seed-bank densities and the four others had similar densities to the comparison treatment, perhaps the two locations had weaker alfalfa stands that did not compete or establish as well. This is an area warranting further investigation.
4.3 Broadleaf weeds
Few treatment effects were observed for broadleaf weed biomass. However, in some locations, broadleaf weed biomass was reduced in the treatment where no herbicides were applied, contrary to what would be expected (Figure 5). However, in those locations, grass weed biomass was often quite high. The authors expect that the extreme competition from the grass weeds, and particularly wild oat, resulted in decreased broadleaf weed biomass. Therefore, the reduction in broadleaf weed biomass was likely due to the ineffectiveness of the treatment at managing grass weeds, rather than the effectiveness of the treatment on broadleaf weed management. The broadleaf weed community was generally maintained compared with that in the standard canola–wheat treatment; however, high levels of variability raise questions on success of the treatments on different broadleaf weed growth habits. For example, there were substantial differences in the response of cleaver density at the three locations where the species was present (Supplementary Figure 1). Differences in the overall density of cleavers between locations at the start of treatment differentiation may have played a role; however, the species also seems less responsive to strategies such as increased seeding rates. We hypothesize that twining–growth-habit species like wild buckwheat and cleavers may not be as responsive to increased crop competition as other non-twining species like wild oat, due to their ability to climb into the crop canopy to acquire light. This is an area that requires further investigation. Benaragama et al. (2022) noted benefits from winter cereals when two consecutive winter crops were incorporated into a rotation, a cropping system rotation that was not included in the current study. However, this only occurred at locations with good winter crop establishment (Benaragama et al., 2022) as successful establishment and overwinter survival of winter-annual crops were required for competition with weeds, similar to the observations in this study. Perennial alfalfa has been shown to successfully manage cleavers (Benaragama et al., 2022); however, this benefit was not apparent in the current study for seedling densities although seed-bank densities were reduced in Lacombe.
Volunteer canola densities were greater in wheat crops preceded by canola in rotation. This highlights not only the importance of crop sequencing to allow for management of preceding crop volunteers but also the need for optimizing harvest settings and minimizing harvest losses of preceding crops. Canola harvest losses of up to 6,100 seeds m−2 have been recorded on commercial farms in the Prairies (Cavalieri et al., 2016); thus, harvest management can have a clear effect on the weed densities and the weed seed bank. Early cut silage was an effective addition to managing volunteer canola, as the silage process would occur before seed set and seed loss (Supplementary Figure 2) (Harker et al., 2003; Tidemann et al., 2017). It is likely that low canola densities in some of the treatments (i.e., treatments 4 and 6) are a result of the ineffectiveness of the treatment on wild oat management.
Kochia, while one of the predominant weed issues in the southern Prairies, particularly due to rapid emergence of herbicide resistance to multiple herbicide groups (Geddes et al., 2021a; Geddes et al., 2021b; Geddes et al., 2022; Sharpe et al., 2023) did not show obvious impacts of increased seeding rate, crop rotation, or early cut silage. In Lethbridge, only the rotation with increased seeding rates of peas, winter wheat and canola in rotation, with chaff collection reduced kochia densities (Supplementary Figure 3A), which may have been a result of their lack of efficacy on grass weed management, rather than efficacy on kochia. However, winter wheat in crop rotations or other IWM strategies including increased seeding rate, crop rotation, and narrow row spacing have been effective in other studies for kochia management (Geddes, unpublished data).
Wild mustard densities were reduced in the alfalfa treatments, as well as in treatments that tended to correspond with high grass weed biomass (Supplementary Figure 3B). The seed-bank densities of wild mustard were quite variable; however, treatment 13 showed an increase in seed-bank densities (Supplementary Figure 5C). This was intriguing as this treatment utilized full herbicide rates in comparison with treatment 14, which was the same treatment but without herbicides, where seed-bank densities were lower. Another western Canadian study has shown improvement in management of wild mustard with narrower row spacing and increased crop densities (Kirkland, 1993). Our study did not show as much responsiveness of wild mustard to seeding rate; however, the authors of the previous study measured wild mustard biomass specifically, whereas we focused on density. Wild mustard may warrant additional studies to determine effects of strategies such as winter cereals and early cut silage on management.
Shepherd’s purse densities were quite variable and were greater in some of the winter cereal treatments in Scott (Supplementary Figure 3C), particularly those where no herbicides were utilized. Shepherd’s purse as a facultative winter annual species is expected to be less affected by incorporation of winter cereals in the rotation for early competition. This species has not previously been the focus of many IWM studies as it is relatively easy to control with available herbicides. However, it is possible that facultative winter annual broadleaves such as shepherd’s purse may require additional research to determine how they may be affected by recommended IWM strategies for other weeds. Their ability to emerge in the fall alongside the fall seeded crop may eliminate the competitive advantage of diversifying crop rotations with winter cereals.
Lambsquarters’ seed-bank density also responded poorly to the winter cereal treatments where no herbicides were used (Supplementary Figure 4A). Lambsquarters is not a facultative winter annual so the reason for the lack of control in these treatments is unclear and perhaps warrants additional study. The exception is the winter wheat treatment where herbicides were applied and densities were reduced. The overall impact of winter cereals in rotation, without herbicide application, needs to be dissected further. In contrast, redroot pigweed seed-bank density (Supplementary Figure 4B) was reduced by a number of treatments including perennial alfalfa and a number of other treatments where herbicides were not applied. The reductions in the treatments without herbicides are likely where grass weed competition became dominant.
Overall, it is clear that broadleaf weed species do not all respond the same to IWM strategies (Table 3). In particular, research should investigate further the impact of IWM strategies on twining–growth-habit weeds such as cleavers and wild buckwheat, and facultative winter annuals, as their biology gives them opportunity to avoid the impact of these competition-based strategies. In addition, it is important to identify those species that are not affected by strategies being recommended for a dominant problem weed such as wild oat (Table 3). It is not ideal to recommend a weed management strategy for one species that results in another becoming more abundant or problematic if those strategies allow that species to flourish.
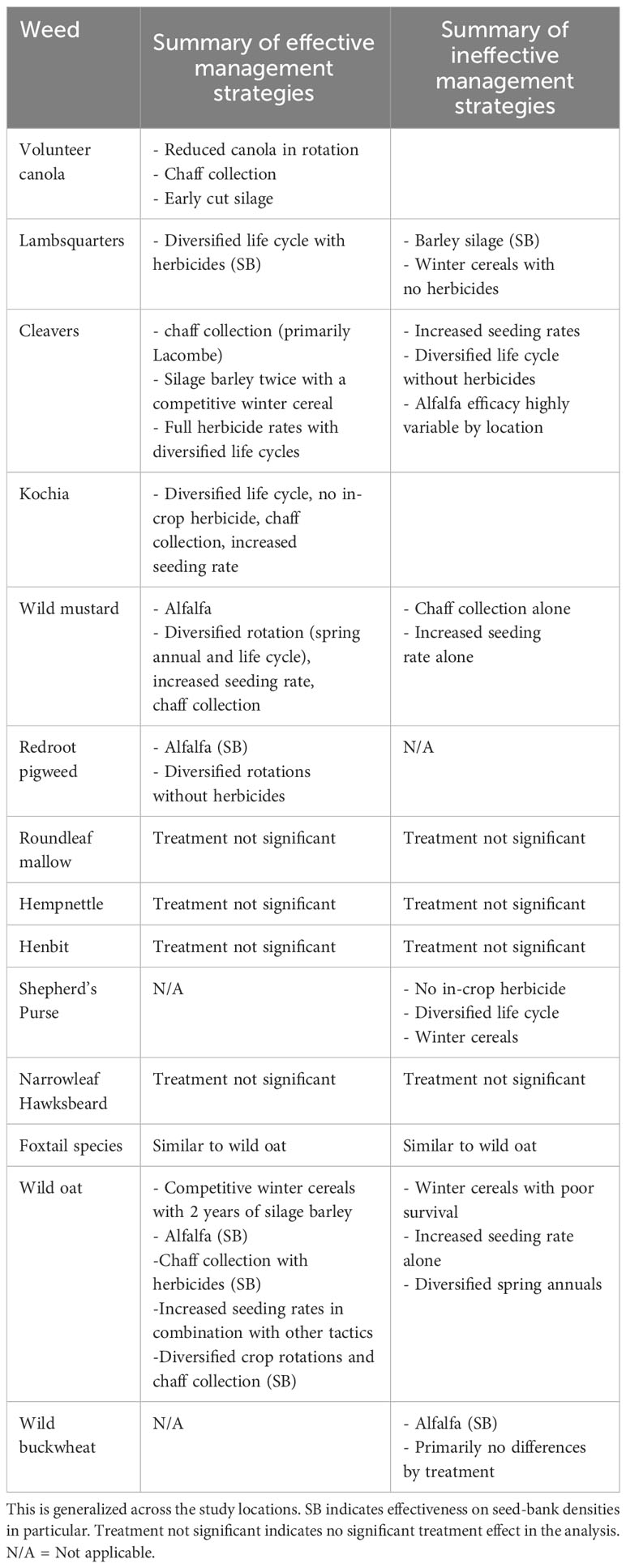
Table 3 A summary of effective and ineffective management strategies on the various weed species considered in the study.
4.4 Crop biomass, yield, and dockage
Crop biomass and yield consistently showed similar treatment effects. Treatments with no herbicides that did not include a competitive winter cereal and/or 2 years of silage barley resulted in decreased final-year wheat crop yields (Figures 6, 7). Although not different in the across location analysis, one location showed increases in crop biomass in the perennial alfalfa treatment and in the canola–wheat rotation where chaff collection was added and an increase in yield where the chaff collection was added. Similarly, reductions in dockage were observed at three locations for the alfalfa treatment and two locations for the canola wheat rotation with chaff collection (Figure 8). The benefit of alfalfa could result from weed control (Benaragama et al., 2022) or from nitrogen fixation; however, our weed control results were not as consistent as that in the work by Benaragama et al. (2022), meaning the benefit did not carry through to the wheat yield. It was interesting to see the benefit of chaff collection at one location and a numerically higher albeit statistically similar yield across locations. Although incorporation of HWSC into cropping systems has been shown to reduce weed populations (Walsh et al., 2018; Shergill et al., 2020; Akhter et al., 2023), its benefit in this study was unexpected, given that weed populations were dominated by wild oat that has been reported as a poor target for HWSC (Burton et al., 2016; Burton et al., 2017; Tidemann et al., 2017). This study also demonstrates that HWSC is not a replacement for herbicides but is intended and is most effective as an additional, incremental tool for weed management strategies (Walsh and Powles, 2014). Longer-term studies, where the seed banks are impacted over a longer period, particularly for weeds such as wild oat with a dormant seed bank, may show additional benefits of HWSC to weed densities and, as a result, to crop biomass and yield.
4.5 Practical implications
This study concurs with previous research showing the potential to manage wild oat without herbicides for 3 years (Harker et al., 2016; Benaragama et al., 2022). However, it also highlights challenges associated with managing entire weed communities utilizing the same IWM tactics for each weed species and reducing or removing herbicide applications for 3 years. There were some tactics that can be beneficial for the majority of the weed community, whereas others may not be effective based on weed life cycles (facultative winter annuals) or growth habit (twining weeds) (Table 3). However, although tactics such as silage barley and winter cereals show efficacy in managing weed communities or species, it will be important to investigate the economics and marketability of these crops. Silage barley can effectively reduce wild oat (Harker et al., 2003; Harker et al., 2016), but, if a farmer does not have livestock or neighbors in need of livestock feed, then their product does not have a market. This highlights the need for continued investigation into IWM strategies such as HWSC that can impact weed management without changing the product or marketability of the producer’s rotation. However, as shown in this study, HWSC is not an effective replacement for herbicides or as a stand-alone weed management strategy. Developing IWM strategies that provide the desired level of weed management and economic and environmental sustainability and that can be practically incorporated into farming operations is an on-going challenge, made even more difficult by regionality and differences in farm values, equipment, and specific problem pests. In addition, higher weed densities can limit the ability of IWM strategies to successfully manage weeds, emphasizing that success will be achieved most easily by early adoption of the strategies. This contrasts with the typical contemporary approach where new tactics are adopted only when current strategies are no longer effective. This study not only shows the ability to reduce reliance on herbicides with strategies that can be effective in Western Canada but also highlights the need for further understanding of our different weed species and their responses to IWM strategies, as well as the complexity of managing weed communities with IWM.
Data availability statement
The raw data supporting the conclusions of this article will be made available by the authors, without undue reservation.
Author contributions
BT: Conceptualization, Data curation, Formal Analysis, Investigation, Methodology, Project administration, Supervision, Writing – original draft, Writing – review & editing. KH: Conceptualization, Data curation, Investigation, Methodology, Writing – review & editing. SS: Conceptualization, Investigation, Project administration, Supervision, Validation, Writing – review & editing. CW: Conceptualization, Project administration, Supervision, Writing – review & editing. EJ: Conceptualization, Investigation, Writing – review & editing. RG: Conceptualization, Investigation, Project administration, Supervision, Writing – review & editing. NL: Conceptualization, Investigation, Writing – review & editing. TT: Conceptualization, Investigation, Writing – review & editing. ES: Conceptualization, Investigation, Writing – review & editing. RB: Conceptualization, Investigation, Project administration, Supervision, Writing – review & editing. CG: Conceptualization, Formal Analysis, Investigation, Project administration, Supervision, Writing – review & editing. HK: Formal Analysis, Investigation, Project administration, Supervision, Writing – review & editing. GS: Conceptualization, Investigation, Project administration, Supervision, Writing – review & editing. AM: Conceptualization, Investigation, Project administration, Supervision, Writing – review & editing. CG: Conceptualization, Investigation, Project administration, Supervision, Writing – review & editing. LM: Data curation, Investigation, Methodology, Writing – review & editing. PR: Data curation, Investigation, Methodology, Writing – review & editing. ES: Data curation, Investigation, Methodology, Writing – review & editing. JZ: Data curation, Investigation, Methodology, Writing – review & editing.
Funding
The author(s) declare financial support was received for the research, authorship, and/or publication of this article. Funding for this project was provided by Western Grains Research Foundation, Alberta Barley, Alberta Canola, and Sask Wheat Development Commission through project number 2016F017R.
Conflict of interest
The authors declare that the research was conducted in the absence of any commercial or financial relationships that could be construed as a potential conflict of interest.
Publisher’s note
All claims expressed in this article are solely those of the authors and do not necessarily represent those of their affiliated organizations, or those of the publisher, the editors and the reviewers. Any product that may be evaluated in this article, or claim that may be made by its manufacturer, is not guaranteed or endorsed by the publisher.
Supplementary material
The Supplementary Material for this article can be found online at: https://www.frontiersin.org/articles/10.3389/fagro.2023.1304741/full#supplementary-material
Supplementary Figure 1 | Cleavers seedling density at (A) Beaverlodge; (B) Lacombe and (C) Saskatoon in the final year of the rotational study. The yellow highlighted treatment is the standard seeding rate, full herbicide, no harvest weed seed control canola-wheat rotation to which all the other treatments are contrasted. Significant increases in density are red, while significant decreases in density are green, based on a Dunnett’s comparison of means using an α=0.05. Error bars indicate standard errors.
Supplementary Figure 2 | Canola seedling density at (A) Beaverlodge; (B) Lacombe and (C) Scott in the final year of the rotational study. The yellow highlighted treatment is the standard seeding rate, full herbicide, no harvest weed seed control canola-wheat rotation to which all the other treatments are contrasted. Significant increases in density are red, while significant decreases in density are green, based on a Dunnett’s comparison of means using an α=0.05. Error bars indicate standard errors.
Supplementary Figure 3 | Seedling densities for (A) Lethbridge kochia; (B) Saskatoon wild mustard and (C) Scott shepherd’s purse in the final year of the rotational study. The yellow highlighted treatment is the standard seeding rate, full herbicide, no harvest weed seed control canola-wheat rotation to which all the other treatments are contrasted. Significant increases in density are red, while significant decreases in density are green, based on a Dunnett’s comparison of means using an α=0.05. Error bars indicate standard errors.
Supplementary Figure 4 | Seedbank densities for (A) Beaverlodge lambsquarters; (B) Carman redroot pigweed and (C) Carman foxtail species in the final year of the rotational study. The yellow highlighted treatment is the standard seeding rate, full herbicide, no harvest weed seed control canola-wheat rotation to which all the other treatments are contrasted. Significant increases in seedbank density are red, while significant decreases in seedbank density are green, based on a Dunnett’s comparison of means using an α=0.05. Error bars indicate standard errors.
Supplementary Figure 5 | Seedbank densities for (A) Lacombe cleavers (B) Saskatoon cleavers and (C) Saskatoon wild mustard in the final year of the rotational study. The yellow highlighted treatment is the standard seeding rate, full herbicide, no harvest weed seed control canola-wheat rotation to which all the other treatments are contrasted. Significant increases in seedbank density are red, while significant decreases in seedbank density are green, based on a Dunnett’s comparison of means using an α=0.05. Error bars indicate standard errors.
References
Akhter M. J., Sønderskov M., Loddo D., Ulber L., Hull R., Kudsk P. (2023). Opportunities and challenges for harvest weed seed control in European cropping systems. Eur. J. Agron. 142, 126639. doi: 10.1016/j.eja.2022.126639
Anderson R. L. (2005). A multi-tactic approach to manage weed population dynamics in crop rotations. Agron. J. 97, 1579–1583. doi: 10.2134/agronj2005.0194
Beckie H. J., Blackshaw R. E., Harker K. N., Tidemann B. D. (2018). Weed seed shatter in spring wheat in Alberta. Can. J. Plant Sci. 98, 107–114. doi: 10.1139/cjps-2017-0103
Beckie H. J., Harker K. N. (2017). Our top 10 herbicide-resistant weed management practices. Pest Manage. Sci. 73, 1045–1052. doi: 10.1002/ps.4543
Beckie H. J., Shirriff S. W., Leeson J. Y., Hall L. M., Harker K. N., Dokken-Bouchard F., et al. (2020). Herbicide-resistant weeds in the Canadian prairies: 2012 to 2017. Weed Technol. 34, 461–474. doi: 10.1017/wet.2019.128
Benaragama D. I., May W. E., Gulden R. H., Willenborg C. J. (2022). Functionally diverse flax-based rotations improve wild oat (Avena fatua) and cleavers (Galium spurium) management. Weed Sci. 70, 220–234. doi: 10.1017/wsc.2021.79
Beres B. L., Harker K. N., Clayton G. W., Bremer E., Blackshaw R. E., Graf R. J. (2010). Weed-competitive ability of spring and winter cereals in the Northern Great Plains. Weed Technol. 24, 108–116. doi: 10.1614/WT-D-09-00036.1
Beres B. L., Turkington T. K., Kutcher H. R., Irvine B., Johnson E. N., O'Donovan J. T., et al. (2016). Winter wheat cropping system response to seed treatments, seed size, and sowing density. Agron. J. 108, 1101–1111. doi: 10.2134/agronj2015.0497
Blackshaw R., Lindwall C. (1995). Management systems for conservation fallow on the southern Canadian prairies. Can. J. Soil Sci. 75, 93–99. doi: 10.4141/cjss95-012
Blackshaw R. E., Harker K. N., O'Donovan J. T., Beckie H. J., Smith E. (2008). Ongoing development of integrated weed management systems on the Canadian prairies. Weed Sci. 56, 146–150. doi: 10.1614/WS-07-038.1
Blackshaw R. E., Molnar L. J., Muendel H.-H., Saindon G., Li X. (2000). Integration of cropping practices and herbicides improves weed management in dry bean (Phaseolus vulgaris). Weed Technol. 14, 327–336. doi: 10.1614/0890-037X(2000)014[0327:IOCPAH]2.0.CO;2
Burton N. R., Beckie H. J., Willenborg C. J., Shirtliffe S. J., Schoenau J. J., Johnson E. N. (2016). Evaluating seed shatter of economically important weed species. Weed Sci. 64, 673–682. doi: 10.1614/WS-D-16-00081.1
Burton N. R., Beckie H. J., Willenborg C. J., Shirtliffe S. J., Schoenau J. J., Johnson E. N. (2017). Seed shatter of six economically important weed species in producer fields in Saskatchewan. Can. J. Plant Sci. 97, 266–276. doi: 10.1139/cjps-2016-0183
Cavalieri A., Harker K. N., Hall L. M., Willenborg C. J., Haile T. A., Shirtliffe S. J., et al. (2016). Evaluation of the causes of on-farm harvest losses in canola in the northern great plains. Crop Sci. 56, 2005–2015. doi: 10.2135/cropsci2016.01.0014
Ervin D. E., Frisvold G. B. (2016). Community-based approaches to herbicide-resistant weed management: lessons from science and practice. Weed Sci. 64, 609–626. doi: 10.1614/WS-D-15-00122.1
Geddes C. M., Ostendorf T. E., Owen M. L., Leeson J. Y., Sharpe S. M., Shirriff S. W., et al. (2021a). Fluroxypyr-resistant kochia [Bassia scoparia (L.) AJ Scott] confirmed in Alberta. Can. J. Plant Sci. 102, 437–441. doi: 10.1139/cjps-2021-0111
Geddes C. M., Pittman M. M., Gulden R. H., Jones T., Leeson J. Y., Sharpe S. M., et al. (2021b). Rapid increase in glyphosate resistance and confirmation of dicamba-resistant kochia (Bassia scoparia) in Manitoba. Can. J. Plant Sci. 102, 459–464. doi: 10.1139/cjps-2021-0169
Geddes C. M., Pittman M. M., Hall L. M., Topinka A. K., Sharpe S. M., Leeson J. Y., et al. (2022). Increasing frequency of multiple herbicide-resistant kochia (Bassia scoparia) in Alberta. Can. J. Plant Sci. 103, 233–237. doi: 10.1139/cjps-2022-0224
Harker K. N., Kirkland K. J., Baron V. S., Clayton G. W. (2003). Early-harvest barley (Hordeum vulgare) silage reduces wild oat (Avena fatua) densities under zero tillage. Weed Technol. 17, 102–110. doi: 10.1614/0890-037X(2003)017[0102:EHBHVS]2.0.CO;2
Harker K. N., O'Donovan J. T. (2013). Recent weed control, weed management, and integrated weed management. Weed Technol. 27, 1–11. doi: 10.1614/WT-D-12-00109.1
Harker K. N., O'Donovan J. T., Irvine R. B., Turkington T. K., Clayton G. W. (2009). Integrating cropping systems with cultural techniques augments wild oat (Avena fatua) management in barley. Weed Sci. 57, 326–337. doi: 10.1614/WS-08-165.1
Harker K. N., O'Donovan J. T., Turkington T. K., Blackshaw R. E., Lupwayi N. Z., Smith E. G., et al. (2016). Diverse rotations and optimal cultural practices control wild oat (Avena fatua). Weed Sci. 64, 170–180. doi: 10.1614/WS-D-15-00133.1
Hurvich C. M., Tsai C. L. (1989). Regression and time series model selection in small samples. Biometrika 76, 297–307. doi: 10.1093/biomet/76.2.297
Kirkland K. J. (1993). Weed management in spring barley (Hordeum vulgare) in the absence of herbicides. J. Sustain. Agric. 3 (3-4), 95–104. doi: 10.1300/J064v03n03
Leeson J. Y. (2016). Saskatchewan Weed Survey of cereal, oilseed and pulse crops in 2014 and 2015 (Saskatoon, SK: Agriculture and Agri-Food Canada 371).
Leeson J. Y., Gaultier J., Grenkow L. (2017). Manitoba weed survey of annual crops in 2016 (Saskatoon, SK: Agriculture and Agri-Food Canada), 213.
Leeson J. Y., Hall L. M., Neeser C., Tidemann B., Harker K. N. (2019). Alberta weed survey of annual crops in 2017 (Saskatoon, SK: Agriculture and Agri-Food Canada), 285.
Liebman M., Gallandt E. R. (1997). “Many little hammers: Ecological management of crop-weed interactions,” in Ecology in agriculture, 1st edn. Ed. Jackson L. (San Diego, California: Academic Press), 291–343.
Littell R. C., Milliken G. A., Stroup W. W., Wolfinger R. D. (2006). SAS System for mixed models. 2nd edn (Cary, NC: SAS Institute), 814.
Norsworthy J. K., Ward S. M., Shaw D. R., Llewellyn R. S., Nichols R. L., Webster T. M., et al. (2012). Reducing the risks of herbicide resistance: best management practices and recommendations. Weed Sci. 60, 31–62. doi: 10.1614/WS-D-11-00155.1
O'Donovan J. T., Harker K. N., Clayton G. W., Hall L. M. (2000). Wild oat (Avena fatua) interference in barley (Hordeum vulgare) is influenced by barley variety and seeding rate. Weed Technol. 14, 624–629. doi: 10.1614/0890-037X(2000)014[0624:WOAFII]2.0.CO;2
O'Donovan J. T., Harker K. N., Turkington T. K., Clayton G. W. (2013). Combining cultural practices with herbicides reduces wild oat (Avena fatua) seed in the soil seed bank and improves barley yield. Weed Sci. 61, 328–333. doi: 10.1614/WS-D-12-00168.1
O'Donovan J., Newman J., Harker K., Blackshaw R., McAndrew D. (1999). Effect of barley plant density on wild oat interference, shoot biomass and seed yield under zero tillage. Can. J. Plant Sci. 79, 655–662. doi: 10.4141/P98-132
O’Donovan J., Blackshaw R., Harker K., Clayton G., Moyer J., Dosdall L., et al. (2007). Integrated approaches to managing weeds in spring-sown crops in western Canada. Crop Prot. 26, 390–398. doi: 10.1016/j.cropro.2005.09.018
O’Donovan J. T., Blackshaw R. E., Neil Harker K., Clayton G. W., McKenzie R. (2005). Variable crop plant establishment contributes to differences in competitiveness with wild oat among cereal varieties. Can. J. Plant Sci. 85, 771–776. doi: 10.4141/P04-190
Owen M. D. K. (2016). Diverse approaches to herbicide-resistant weed management. Weed Sci. 64, 570–584. doi: 10.1614/WS-D-15-00117.1
Powles S. B., Yu Q. (2010). Evolution in action: plants resistant to herbicides. Annu. Rev. Plant Biol. 61, 317–347. doi: 10.1146/annurev-arplant-042809-112119
Selig C., De Mol F., Westerman P. R., Gerowitt B. (2022). Quantifying seed and establishment limitation to seedling recruitment of arable weeds: An example of barnyardgrass (Echinochloa crus-galli). Weed Sci. 70, 87–94. doi: 10.1017/wsc.2021.68
Sharpe S. M., Leeson J. Y., Geddes C. M., Willenborg C. J., Beckie H. J. (2023). Survey of glyphosate-and dicamba-resistant kochia (Bassia scoparia) in Saskatchewan. Can. J. Plant Sci. 103, 472–480. doi: 10.1139/cjps-2023-0016
Shergill L. S., Schwartz-Lazaro L. M., Leon R., Ackroyd V. J., Flessner M. L., Bagavathiannan M., et al. (2020). Current outlook and future research needs for harvest weed seed control in North American cropping systems. Pest Manage. Sci. 76, 3887–3895. doi: 10.1002/ps.5986
Statistics Canada. (2023). Table 32-10-0359-01 Estimated areas, yield, production, average farm price and total farm value of principal field crops, in metric and imperial units (Accessed Sept. 6, 2023).
Thurston J. M. (1962). The effect of competition from cereal crops on the germination and growth of Avena fatua L. in a naturally‐infested field. Weed Res. 2, 192–207. doi: 10.1111/j.1365-3180.1962.tb00199.x
Tidemann B. D., Hall L. M., Harker K. N., Alexander B. C. (2016). Identifying critical control points in the wild oat (Avena fatua) life cycle and the potential effects of harvest weed-seed control. Weed Sci. 64, 463–473. doi: 10.1614/WS-D-15-00200.1
Tidemann B. D., Hall L. M., Harker K. N., Beckie H. J., Johnson E. N., Stevenson F. C. (2017). Suitability of wild oat (Avena fatua), false cleavers (Galium spurium), and volunteer canola (Brassica napus) for harvest weed seed control in western Canada. Weed Sci. 65, 769–777. doi: 10.1017/wsc.2017.58
Walsh M. J., Aves C., Powles S. B. (2017). Harvest weed seed control systems are similarly effective on rigid ryegrass. Weed Technol. 31, 178–183. doi: 10.1017/wet.2017.6
Walsh M. J., Broster J. C., Schwartz-Lazaro L. M., Norsworthy J. K., Davis A. S., Tidemann B. D., et al. (2018). Opportunities and challenges for harvest weed seed control in global cropping systems. Pest Manage. Sci. 74, 2235–2245. doi: 10.1002/ps.4802
Walsh M. J., Powles S. B. (2014). Management of herbicide resistance in wheat cropping systems: learning from the Australian experience. Pest Manage. Sci. 70, 1324–1328. doi: 10.1002/ps.3704
Yang R.-C. (2010). Towards understanding and use of mixed-model analysis of agricultural experiments. Can. J. Plant Sci. 90, 605–627. doi: 10.4141/CJPS10049
Keywords: integrated weed management, crop rotation, cultural control, harvest weed seed control, weed community dynamics
Citation: Tidemann BD, Harker KN, Shirtliffe S, Willenborg C, Johnson E, Gulden R, Lupwayi NZ, Turkington TK, Stephens EC, Blackshaw RE, Geddes CM, Kubota H, Semach G, Mulenga A, Gampe C, Michielsen L, Reid P, Sroka E and Zuidhof J (2023) Using integrated weed management systems to manage herbicide-resistant weeds in the Canadian Prairies. Front. Agron. 5:1304741. doi: 10.3389/fagro.2023.1304741
Received: 29 September 2023; Accepted: 16 November 2023;
Published: 15 December 2023.
Edited by:
Carol Mallory-Smith, Oregon State University, United StatesReviewed by:
Stephen Christopher Marble, University of Florida, United StatesAhmet Uludag, Çanakkale Onsekiz Mart University, Türkiye
Copyright © 2023 S. Shirtliffe, C. Willenborg, E. Johnson, R. Gulden, and His Majesty the King in Right of Canada, as represented by the Minister of Agriculture and Agri-Food Canada for the contribution of B.D. Tidemann, K.N. Harker, N.Z. Lupwayi, T.K. Turkington, E.C. Stephens, R.E. Blackshaw, C.M. Geddes, H. Kubota, G. Semach, A. Mulenga, C. Gampe, L. Michielsen, P. Reid, E. Sroka, and J. Zuidhof. This is an open-access article distributed under the terms of the Creative Commons Attribution License (CC BY). The use, distribution or reproduction in other forums is permitted, provided the original author(s) and the copyright owner(s) are credited and that the original publication in this journal is cited, in accordance with accepted academic practice. No use, distribution or reproduction is permitted which does not comply with these terms.
*Correspondence: Breanne Darlene Tidemann, breanne.tidemann@agr.gc.ca