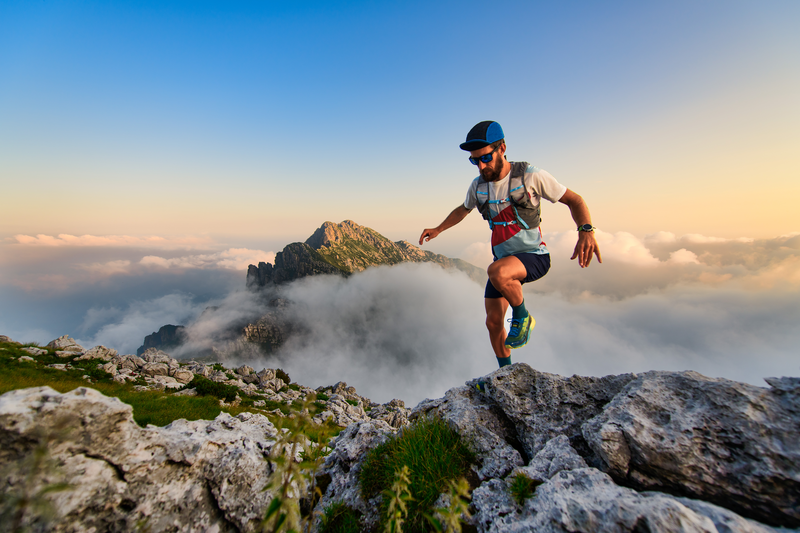
94% of researchers rate our articles as excellent or good
Learn more about the work of our research integrity team to safeguard the quality of each article we publish.
Find out more
ORIGINAL RESEARCH article
Front. Agron. , 28 November 2023
Sec. Climate-Smart Agronomy
Volume 5 - 2023 | https://doi.org/10.3389/fagro.2023.1284173
This article is part of the Research Topic Cropping Systems Adaptation in the Context of Global Change: Current Trends and Future Directions View all articles
Faced with the challenges of extreme climatic events and increasing food demand, cowpea farming offers a viable option for generating grains and foliage while aiding soil preservation. This research aimed to evaluate the impact of diverse organic fertilizers and plastic mulches on cowpea yields and soil macrofauna. A completely randomized block design was employed, with a factorial arrangement of 3 × 3 + 1, encompassing plastic mulch (3.5 mm) color (white, black, and blue), organic fertilizer (compost of chicken manure-agricultural soil-dolomite, island guano, and cattle manure compost), as well as a control (without synthetic mulch and organic fertilization). The study evaluated soil temperature, plant height, area biomass, foliar macronutrients, yield, and soil macrofauna. The findings demonstrate that soil temperature varies based on the color of the plastic mulch. It was observed that using organic fertilizers resulted in significantly taller plants than the control group, with fresh and dry biomass weight also being significantly greater (p<0.05) for cattle manure compost compared to the other treatments. Furthermore, the use of plastic mulches had an impact on soil macrofauna. Cowpea displayed resistance to low soil fertility and fluctuations in soil temperature ranging from <35°C to >40°C. However, high precipitation during the fruiting and harvesting seasons significantly impacted grain yields. Organic fertilizers produced noticeable variances (p<0.05) in the aerial biomass weight of cowpeas, particularly cattle manure compost. The data, indicates that soil macrofauna, like the earthworm Pontoscolex corethrurus, improved the availability of N, P, and K to the plant during the reproductive stage. Plastic mulches and organic fertilizers were linked to an increase in earthworm weights, specifically P. corethrurus. Additionally, plastic mulches served as a physical barrier against ants and bugs.
Cowpea (Vigna unguiculata) is a legume predominantly grown in warm climates within the tropics of Africa, South Asia, and Latin America (Boukar et al., 2018). Peru, in particular, produces 17,588 tonnes of cowpea mulch an area of 12,779 hectares with an average yield of 1,376 kg/ha (FAOSTAT, 2017). An annual production increase of 131.9% has been observed (INEI—Instituto Nacional de Estadística e Informática, 2020). Cowpea is predominantly cultivated in the Peruvian Amazon for grain, foliage production, and soil reclamation on sloped terrain. Its cultivation improves erosion control while enhancing physical, chemical, and biological soil characteristics.
The cowpea is a important source of calories and protein for many people worldwide, as nearly every part of the plant is consumed (Herniter et al., 2020). Due to their nutritional and nutraceutical benefits, its green and dry grains, green pods, and foliage are used in the human diet, it is also included in the diet of domestic animals, especially ruminants and poultry (Avanza et al., 2013; Herniter et al., 2020; Abebe and Alemayehu, 2022). Cowpea grains contain between 20 to 25%, and the foliage can reach up to 18.6% protein per dry weight (Boukar et al., 2018).
Although it is well known that cowpea does not require particularly fertile soil, their production is constrained by soils with low phosphorus (P) levels. (Boukar et al., 2018). Organic fertilizers enhance soil fertility and biology in cowpea production, which contains mineralized and non-mineralized organic matter. The mineralized portion of organic matter plays a crucial role in the physical, chemical, and biological fertility of the soil (Six et al., 2002; Ferreira et al., 2013; IPCC—Intergovernmental Panel on Climate Change, 2019; Murga-Orrillo et al., 2019), while the non-mineralized portion of organic matter provides energy and nutrients for soil organisms, including macrofauna, as well as for the crop (Sofo et al., 2020). As a result, studies focusing on the interaction between macrofauna and organic fertilization are especially important.
In recent decades, extreme climatic events, such as high temperatures, droughts, and heavy precipitation, have occurred (AghaKouchak et al., 2020; Fischer et al., 2021). These phenomena are frequently pronounced in tropical regions, with severe socio-economic impacts in densely populated and agricultural areas (Zhou et al., 2023). Cowpea is subjected to these extreme climatic conditions, which affect its yield due to high temperatures and intense precipitation that further erode the soil in sloped agricultural areas.
Permeable plastic mulches on the soil could mitigate these extreme climatic conditions by stabilizing soil temperature, conserving soil, and promoting cowpea biomass production. Plastic mulches improve the hydrothermal conditions of the soil, enhance nutrient mineralization rates, and facilitate the transport of N and P (Mao et al., 2020; Shah and Wu, 2020). They conserve water, suppress weeds, and increase agricultural productivity (Shah and Wu, 2020), enhancing the efficiency of N and water resource utilization, thereby increasing yields (Yu et al., 2018).
Black and silver-colored plastic mulches can reflect up to 33% of sunlight (Rumahorbo et al., 2020). Therefore, light-colored plastic mulches reflect more sunlight, causing variations in soil temperature and impacting soil micro- and macrofauna. Plastic mulches have different effects on soil macrofauna due to the complexity of interactions between species and the availability of organic substrates (Zhang et al., 2021).
Soil macrofauna represents most soil animal biomass and is considered ecosystem engineering (Gongalsky, 2021). They play a crucial role in organic matter decomposition, the formation of biogenic structures, and nutrient recycling (Lavelle and Spain, 2001; Lavelle et al., 2006; Gongalsky, 2021). However, they are susceptible to changes in land use, types of organic substrates, and climatic variations (Edwards and Bohlen, 1996; Kozlov et al., 2022).
The hypothesis proposes that various colors of permeable plastic mulches influence soil temperature, consequently affecting soil macrofauna, organic matter mineralization, and nutrient availability for the crop. Similarly, organic fertilizers influence soil macrofauna, serving as a substrate that these species will mineralize, and the physiology of cowpea by providing specific nutrients such as N, P, and K, which are essential for the growth and production of this crop. This research assessed the effects of different organic fertilizers and permeable plastic mulches on cowpea production and soil macrofauna.
The experiment was carried out at “Munichis” University Campus of the School of Agronomy, National Autonomous University of Alto Amazonas (UNAAA), between December 01, 2022, and April 25, 2023. The experimental area is geolocated at a longitude of 76.2313W, latitude 5.8983S, and an altitude of 145.3 meters.
The weather data during the study period included an average temperature of 26.4°C, a relative humidity of 81.7%, and a rainfall of 1439.6 mm. The highest precipitation occurred in March, 557.0 mm, and in April, 344.2 mm (SENAMHI—Servicio Nacional de Meteorología e Hidrología del Perú, 2023) (Figure 1A). Cowpea thrives best in average temperatures ranging from 25 to 35°C, with annual rainfall between 750 and 1100 mm and various soil types (Drahansky et al., 2016).
Figure 1 Weekly climate conditions for the study period. (A) Rainfall (bars), maximum temperature (red line), average temperature (blue line), and minimum temperature (green line), and (B) experimental cowpea plots.
The total area of the experiment was 255 m2, with each experimental unit measuring 2.5 m × 3.4 m (8.5 m2). There were a total of 30 experimental units. On December 02, 2022, the cowpea “Chiclayo blanco,” with code “PER-1005853,” was planted at a plant density of 166,666.7 plants/ha. The plants were spaced at 30 cm × 20 cm, but only 30 cowpea plants were evaluated per experimental unit (1.8 m2), excluding the plants in the border area (6.7 m2).
Physico-chemical properties of soil were determined using composite sample, which was collected using an auger at a depth of 0–20 cm. This sample was air-dried, ground, and sieved through a 2 mm mesh. Subsequently, organic matter was determined following the method of Walkley and Black (1934), phosphorus (P) was determined according to Olsen et al. (1954), and exchangeable cations (K+, Ca+2, Mg+2, and Na+) were extracted using a 1N KCl solution and determined by atomic absorption spectroscopy, following the methodology proposed by Hunter (1986). Nitrogen (N) content was calculated based on the soil’s organic matter content. Similarly, in the case of organic fertilizers (compost of chicken manure-agricultural soil-dolomite, island guano, and cattle manure compost), chemical analyses were carried out following the soil chemical analysis methodology. In Table 1, the physical and chemical properties of the soil are presented, corresponding to a loam-silty clay-textured soil (Sand: 65.24%, Silt: 12.84%, Clay: 21.92%) with low nutrient content and high acidity due to Al+3 (5.90 cmolc/kg).
Table 1 Physical-chemical properties of the soil in the experimental area of cowpea subjected to plastic mulches and organic fertilizers. Yurimaguas, Munichis 2023.
Before plowing and neutralizing soil acidity, 7.5 t/ha of CaO was applied. The CaO was homogeneously mixed by preparing the soil at a depth of 30 cm using a disc plow attached to an agricultural tractor (Kubota, 120 HP, Model: M108S, Japan). Subsequently, plot demarcation was conducted, with each plot receiving 10 t/ha of compost of chicken manure-agricultural soil-dolomite (from wood residues, rice husk biochar, and agricultural soil), island guano (decomposed organic remains of seabirds), and cattle manure compost (decomposed cow manure). Besides the control, 3.5 mm thick black, blue, and white plastic mulches (100 g/m2 Polypropylene Burlap Fabrics) were applied to each plot (Figure 1B).
A completely randomized block design was employed with a 3 × 3 + 1 factorial arrangement. The plastic mulch factor included three levels: white (C1), black (C2), and blue (C3), while the organic fertilizer factor consisted of composed of a ratio of 3:5:1 of chicken manure-agricultural soil-dolomite (A1), island guano (A2), and cattle manure compost (A3). A total of nine treatments resulted, each with an additional control represented by one plot (01) without plastic mulch (C0) and without organic fertilizer (A0). The treatments and the control were distributed in three replicates (three blocks), resulting in 30 experimental units.
Plant height was measured using a millimeter tape at 16, 30, 42, and 50 days after planting (DAP), with the final assessment conducted when more than 50% of the plants were in flower. When the pods reached physiological maturity, weekly harvests were performed during the last month to prevent moisture-related rot, as this period experienced frequent and intense rainfall (March). The pods were air-dried, and subsequently, the grains were extracted to determine crop yield (kg/ha). Following the final harvest on April 05, 2023, the above-ground biomass of the plant (FB) was collected and weighed using a 15 kg clock-type balance (Kambor Brand). Lastly, in the UNAAA Chemistry Laboratory, the above-ground biomass was oven-dried (Kert Lab Brand, Model: Heating Drying Oven Odhg-9070A, China) at 70°C for 36 hours until a constant weight was achieved. Subsequently, the dry biomass weight (DB) was determined using a precision scale (OHAUS Brand, Model V11P3T, USA).
The content of N, P, and K in the physiologically active leaves of cowpea was determined as follows: 10 leaves per experimental unit were collected (from the middle third of the plant). These leaves were dried in an oven (Kert Lab Brand, Model: Heating Drying Oven Odhg-9070A, China) at 70°C for 36 hours, after which they were ground (Retsch Brand mill, Model: GRINDOMIX GM 300, Germany). Nitrogen was then determined using the Kjeldahl method (Oesper, 1934), while phosphorus (P) and potassium (K) were determined through HNO3 digestion. For P, visible light spectrophotometry was employed, and for K, atomic absorption spectroscopy was used (Embarapa—Empresa Brasileira de Pesquisa Agropecuária, 2009).
Soil temperature was measured using soil thermometers (VIVOSUN Brand, Model B09CGLXTQ5, USA). Temperature readings were taken at 0 to 10 cm depth within each treatment. Measurements were recorded between 12:00 PM and 1:00 PM on sunny and cloudy days. Temperature data were recorded in degrees Fahrenheit and converted to degrees Celsius for analysis.
After the cowpea harvest, monoliths measuring 30 cm in depth within a 25 cm × 25 cm were considered in the experimental plots, following the methodology adapted from Moreira et al. (2012). The macrofauna collected from each monolith was preserved in 5% formalin-dampened samples for subsequent taxonomic identification and weighing in a precision analytical balance with a 0.01 g sensitivity (Model: EHB3000, Brand: TSCALE) at the UNAAA Chemistry Laboratory.
The data underwent normality and homogeneity of variance tests to analyze variance using the F-test, followed by Tukey’s post hoc test. All these analyses were conducted at a significance level of 95% and were presented using boxplots. Similarly, data without a normal distribution for soil macrofauna, soil temperature, and N, P, and K content in the leaves were analyzed and presented using boxplots. All analyses were performed using the statistical software Rstudio (R Core Team, 2023).
In Table 2 displays the chemical properties of the organic fertilizers. Island guano has a higher availability of N, P, and K, while cattle manure compost has an alkaline pH and lower electrical conductivity than island guano and compost of chicken manure-agricultural soil-dolomite.
Table 2 Chemical properties of three organic fertilizers used in cowpea production. Yurimaguas, Munichis 2023.
In Table 3, the mean squares of the ANOVA in the F-test show a significant difference (p<0.05) in the block, possibly due to the experimental area having a 9% slope. On the other hand, when analyzing the factors, they did not show a significant interaction; however, the organic fertilizer independent of the plastic mulches showed a significant difference (p<0.05) for the variables plant height, fresh biomass, and dry biomass.
The F-test (Table 3) and Tukey’s mean test (Figure 2) determined that there was no initial effect of the organic fertilizer on plant height (Figures 2A, B). Subsequently, in evaluations when the foliage covered the entire space between plants (42 DAP) and during cowpea full flowering (50 DAP), all three organic fertilizers showed significant differences (p<0.05), resulting in greater plant height compared to the control (Figures 2C, D).
Figure 2 Height of cowpea plants at 16 (A), 30 (B), 42 (C), and 50 (D) days after planting, grown under four treatments (Control: A0, compost of chicken manure-agricultural soil-dolomite: A1, island guano: A2, and cattle manure compost: A3). The same lowercase letters on the boxplot indicate no significant difference in means according to Tukey’s test (p<0.05).
Fresh biomass and dry matter of cowpea exhibited significant differences (p<0.05) in Tukey’s test, with better performance for cattle manure compost and moderate performance for compost of chicken manure-agricultural soil-dolomite and island guano when compared to the control (Figures 3A, B). However, the crop yield grain was very low, with no significant differences obtained (Figure 3C). Additionally, the treatment with cattle manure compost showed higher %N, %P, and %K content in cowpea leaves (Figures 4B, D, F). Simultaneously, the white plastic mulch had a higher %P content in cowpea leaves compared to the other treatments (Figures 4A, C, E).
Figure 3 Fresh biomass (A), dry biomass (B), and yield (C) of cowpea, planted with organic fertilization (control: A0, compost of chicken manure-agricultural soil-dolomite: A1, island guano: A2, and cattle manure compost: A3). The same lowercase letters on the boxplot indicate no significant difference according to Tukey’s test (p<0.05).
Figure 4 N, P, and K (%) content in cowpea leaves: (A, C, E) plastic mulch (no mulch: C0, white: C1, black: C2, and blue: C3) and (B, D, F) organic fertilization (control: A0, compost of chicken manure-agricultural soil-dolomite: A1, island guano: A2, and cattle manure compost: A3).
The soil temperature in the cowpea experimental area varied depending on the color of the plastic mulch and the control (Figure 5). On sunny days, the soil temperature in the control recorded maximum values above 40°C, while on cloudy days, the soil temperature fluctuated between 35 to 36°C. On sunny and cloudy days, the white synthetic mulch maintained the soil temperature below 35°C. Conversely, on sunny and cloudy days, the black and blue synthetic mulches kept the temperature between 36 to 37°C (Figures 5A, B).
Figure 5 Soil temperature (A) at midday on sunny days, and (B) at midday on cloudy/rainy days in cowpea, planted with plastic mulch (no mulch: C0, white: C1, black: C2, and blue: C3).
Earthworms and other arthropods including ants, termites, Glomeridae, Myriapods, Elateridae, Scarabidae, Crickets, Arachnidae, Diptera, and Bugs were recorded and identified among the soil macrofauna (Table 4). Among this macrofauna, the earthworm P. corethrurus had a higher live weight under white plastic mulch. It also obtained a higher live weight when treated with cattle manure compost than other treatments (Figures 6A, B). It is noteworthy that the white plastic mulch maintained the soil temperature below 35°C (Figures 5A, B), and cattle manure compost showed significant differences (p<0.05) with higher fresh and dry cowpea biomass weights (Figure 3A). On the other hand, the “cuello blanco” earthworm (Figures 6C, D) exhibited higher weights across all synthetic mulches and with island guano and cattle manure compost compared to the control.
Table 4 Soil macrofauna under organic fertilization (control: A0, compost of chicken manure-agricultural soil-dolomite: A1, island guano: A2, and cattle manure compost: A3) and plastic mulch (no mulch: C0, white: C1, black: C2, and blue: C3) associated with cowpea.
Figure 6 Weight of P. corethrurus (A, B) and earthworm “cuello blanco” (C, D) under plastic mulch conditions (no mulch: C0, white: C1, black: C2, and blue: C3) and (A) organic fertilization (Control: A0, compost of chicken manure-agricultural soil-dolomite: A1, guano island: A2, and cattle manure compost: A3) associated with cowpea.
Among the soil macrofauna, particularly noteworthy were arachnids (Order: Araneae), bugs (Order: Hemiptera), and beetles (Order: Coleoptera, Scarabidae) (Figure 7). Only spiders (Figures 7A, B) and bugs (Figures 7C, D) were found in the control; in these plots, the soil temperature varied from 40°C on sunny days to 35°C on cloudy days (Figures 5A, B). However, the highest body weight for beetles were found under the black mulch plastic (Figure 7E).
Figure 7 Weight of spiders (A, B), bed bugs (C, D) and beetles (E, F) in soil under plastic mulch conditions (no mulch: C0, white: C1, black: C2, and blue: C3) and organic fertilization (control: A0, composed of chicken manure-agricultural soil-dolomite: A1, guano island: A2, and cattle manure compost: A3) associated with cowpea.
The favorable effect of organic fertilizers became evident in cowpea growth at 42 DAP (Figures 2C, D), suggesting that cowpea thrives well on low soil fertility levels during the vegetative phase. However, at the onset of reproductive phase, nutritional demands increase, the organic treatments proved better sources for nutrient supply which resulted in greater plant height than the control. In the case of another legume, such as alfalfa (Medicago sativa), it was determined that treatments with organic fertilizers (compost) achieved greater plant height, fresh biomass, and yield compared to the control group (Fu et al., 2012).
On sunny and cloudy days, white plastic mulches maintained soil temperatures below 35°C, while blue and black mulches kept temperatures between 36 to 37°C. In contrast, the control exhibited temperature variations ranging from 35°C to over 40°C (Figures 5A, B). The varying soil temperatures did not significantly affect cowpea plant height, as indicated by the F-test (Table 3). In other words, cowpea exhibits resistance to soil temperature fluctuations. Other studies support cowpea’s resistance to abiotic factors such as high temperatures and drought stress (Kang et al., 2023; Boukar et al., 2018).
Moreover, the intense rainfall in March (557.0 mm) (Figure 1A) did not lead to excess moisture stress in the plants, but it did result in low grain yields ranging from 50 to 80 kg/ha. The average global cowpea yield is 450 kg/ha (Kebede and Bekeko, 2020). However, improved cowpea varieties with proper agronomic management can achieve yields of 2,200 to 3,200 kg/ha (Ashinie et al., 2020).
Organic fertilizers enhance cowpea’s fresh and dry biomass production compared to the control (Figures 3A, B), with cattle manure compost standing out. Cattle manure compost, with its alkaline pH (Table 2), complements soil CaO, resulting in increased biological activity of P. corethrurus and “cuello blanco” earthworms (Figures 6B, D). These chemical and biological soil conditions likely promote greater availability of N, P, and K for cowpea. Indeed, cattle manure compost plots exhibited higher N, P, and K contents in the leaves (Figures 4B, D, F). It is known that soil P is more available to legumes under alkaline pH conditions (Ditta et al., 2018). Additionally, the biodegradation of organic matter by P. corethrurus and other earthworms increases the availability of N and P, optimizing biological nitrogen fixation and P solubilization, resulting in significant yield increase in cowpea and other crops (Padmavathiamma et al., 2008)
The availability of earthworms was higher in plots with plastic mulches (Figures 6A, C), likely favored by the organic matter from the fertilizers and the reduction in soil temperature (Figures 5A, B). However, ants and bugs were found exclusively in the control, indicating that these insects are exclusive to open spaces (Figures 7A–D), where soil temperatures can exceed 40°C on sunny days and drop below 35°C on cloudy days (Figures 5A, B). Plastic mulches act as a physical control for potential pests of these insects. In contrast, beetles were generally found in soil with black synthetic mulches (Figure 7E). Plastic mulches of different colors on the ground either control certain insects or attract them (Rumahorbo et al., 2020). Therefore, it is possible that the black color of the plastic mulch has an affinity for ground beetles, possibly as a means of mimicry to evade their natural enemies. Ground beetles are attracted to traps of black or dark color (Cavaletto et al., 2021).
Cowpea is resilient to low soil fertility and soil temperature fluctuations between <35°C and >40°C, but high rainfall during the fruiting and harvesting period significantly impacted negative grain yields. Organic fertilizers showed significant differences (p<0.05) in cowpea biomass, especially the cattle manure compost fertilizer, suggesting that soil macrofauna such as Pontoscolex corethrurus and others influenced greater availability of N, P, and K for the plant during the reproductive phase. Plastic mulches and organic fertilizers were associated with higher weights of earthworms like P. corethrurus, and the plastic mulches also served as a physical control for ants and bugs.
The original contributions presented in the study are included in the article/supplementary material. Further inquiries can be directed to the corresponding author.
The manuscript presents research on animals that do not require ethical approval for their study.
HM-O: Conceptualization, Formal Analysis, Investigation, Methodology, Supervision, Validation, Visualization, Writing – original draft, Writing – review & editing. JC: Data curation, Investigation, Resources, Writing – original draft. BP: Funding acquisition, Investigation, Resources, Writing – original draft. LA: Funding acquisition, Investigation, Methodology, Project administration, Resources, Validation, Writing – original draft.
The author(s) declare that no financial support was received for the research, authorship, and/or publication of this article.
The authors are deeply grateful for donating seeds to INIA through Johnny Carlos Campos Cedano. To the Agronomy Engineering student, Katterinne Jhadira Sánchez Torres, for her collaboration in the evaluations. To the Research Institute of the UNAAA for financing the research.
The authors declare that the research was conducted in the absence of any commercial or financial relationships that could be construed as a potential conflict of interest.
All claims expressed in this article are solely those of the authors and do not necessarily represent those of their affiliated organizations, or those of the publisher, the editors and the reviewers. Any product that may be evaluated in this article, or claim that may be made by its manufacturer, is not guaranteed or endorsed by the publisher.
Abebe B. K., Alemayehu M. T. (2022). A review of the nutritional use of cowpea (Vigna unguiculata L. Walp) for human and animal diets. J. Agric. Food Res. 10, 100383. doi: 10.1016/j.jafr.2022.100383
AghaKouchak A., Chiang F., Huning L. S., Love C. A., Mallakpour I., Mazdiyasni O., et al. (2020). Climate extremes and compound hazards in a warming world. Annu. Rev. Earth Planet. Sci. 48, 519–548. doi: 10.1146/annurev-earth-071719-055228
Ashinie S. K., Tesfaye B., Wakeyo G. K., Fenta B. A. (2020). Genetic diversity for immature pod traits in Ethiopian cowpea [Vigna unguiculata (L.) Walp.] landrace collections. Afr. J. Biotechnol. 19 (4), 171–182. doi: 10.5897/AJB2020.17097
Avanza M., Acevedo B., Chaves M., Añón M. (2013). Nutritional and anti-nutritional components of four cowpea varieties under thermal treatments: principal component analysis. Food Sci. Technol. 51 pp, 148–157. doi: 10.1016/j.lwt.2012.09.010
Boukar O., Belko N., Chamarthi S., Togola A., Batieno J., Owusu E., et al. (2018). Cowpea (Vigna unguiculata): Genetics, genomics and breeding. Plant Breed. 138 (4), 415–424. doi: 10.1111/pbr.12589
Cavaletto G., Faccoli M., Marini L., Spaethe J., Giannone F., Moino S., et al. (2021). Exploiting trap color to improve surveys of longhorn beetles. J. Pest Sci. 94, 871–883. doi: 10.1007/s10340-020-01303-w
Ditta A., Imtiaz M., Mehmood S., Rizwan M. S., Mubeen F., Aziz O., et al. (2018). Rock phosphate-enriched organic fertilizer with phosphate-solubilizing microorganisms improves nodulation, growth, and yield of legumes. Commun. Soil Sci. Plant Anal. 49 (21), 2715–2725. doi: 10.1080/00103624.2018.1538374
Drahansky M., Paridah M., Moradbak A., Mohamed A., Owolabi F. A. T., Asniza M., et al. (2016). Cowpea: a strategic legume species for food security and health. Legume Seed Nutraceutical Res. Dietary pp, 47–65. doi: 10.5772/intechopen.79006
Edwards C. A., Bohlen P. J. (1996). “Biology and ecology of earthworms. Chapman and Hall, London,” in Biological indicators of soil health. Eds. Pankhurst. C., Doube B. M., Gupta V. V. S. R. (Oxon, UK: Cab International), 266.
Embarapa—Empresa Brasileira de Pesquisa Agropecuária. (2009). Manual de análises químicas de solos, plantas e fertilizantes. Brasília, DF: Embrapa Informação Tecnológica; Rio de Janeiro: Embrapa Solos. P, 627. Available at: https://www.infoteca.cnptia.embrapa.br/infoteca/handle/doc/330496.
FAOSTAT (2017). FAOSTAT, Statistical data base (Rome: Food and Agricultural Organizations of the United Nations).
Ferreira P. V. I. C., Araujo V. A., Nascimento L. A., Cavalcanti F. M. T., Santos T. L. D. (2013). Cobertura morta e adubação orgânica na produção de alface e supressão de plantas daninhas. Rev. Ceres Viçosa 60 (4), 582–588. doi: 10.1590/S0034-737X2013000400019
Fischer E. M., Sippel S., Knutti R. (2021). Increasing probability of record-shattering climate extremes. Nat. Clim. Change 11, 689–695. doi: 10.1038/s41558-021-01092-9
Fu D., Teng Y., Luo Y., Tu C., Li S., Li Z., et al. (2012). Effects of alfalfa and organic fertilizer on benzo [a] pyrene dissipation in an aged contaminated soil. Environ. Sci. pollut. Res. 19, 1605–1611. doi: 10.1007/s11356-011-0672-4
Gongalsky K. B. (2021). Soil macrofauna: Study problems and perspectives. Soil Biol. Biochem. 159, 108281. doi: 10.1016/j.soilbio.2021.108281
Herniter I. A., Muñoz-Amatriaín M., Close T. J. (2020). Genetic, textual, and archeological evidence of the historical global spread of cowpea (Vigna unguiculata [L.] Walp.). Legume Sci. 2 (4), e57. doi: 10.1002/leg3.57
Hunter H. A. (1986). Soil analysis methods for P, K, Cu, Fe, Mn, and Zn, using ASI extracting solution. Technical notes. Florida: ASI-Agro Services International.
INEI—Instituto Nacional de Estadística e Informática. (2020). Informe técnico N° 2, febrero 2020. Produccion Nacional. P, 3. Available at: https://www.inei.gob.pe/media/MenuRecursivo/boletines/02-informe-tecnico-n02_produccion-nacional-dic-2019.pdf.
IPCC—Intergovernmental Panel on Climate Change (2019) Climate change and land (Geneva, Switzerland: IPCC). Available at: https://www.ipcc.ch/srccl-report-download-page/ (Accessed 07/08/2023).
Kang B. H., Kim W. J., Chowdhury S., Moon C. Y., Kang S., Kim S. H., et al. (2023). Transcriptome analysis of differentially expressed genes associated with salt stress in cowpea (Vigna unguiculata L.) during the early vegetative stage. Int. J. Mol. Sci. 24 (5), 4762. doi: 10.3390/ijms24054762
Kebede E., Bekeko Z. (2020). Expounding the production and importance of cowpea (Vigna unguiculata (L.) Walp.) in Ethiopia. Cogent Food Agric. 6 (1), 1769805. doi: 10.1080/23311932.2020.1769805
Kozlov M. V., Zverev V., Gusarov V. I., Korobushkin D. I., Krivosheina N. P., Mattila J., et al. (2022). Changes in biomass and diversity of soil macrofauna along a climatic gradient in european boreal forests. Insects 13 (1), 94. doi: 10.3390/insects13010094
Lavelle P., Decaens T., Aubert B., Barot S., Blouin M., Bureau F., et al. (2006). Soil invertebrates and ecosystem services. Eur. J.Soil Biol. 42, 3–15. doi: 10.1016/j.ejsobi.2006.10.002
Lavelle P., Spain A. (2001). Soil ecology. Kluwer scientific publications, amsterdam, the Netherlands. Library of Congress Cataloging in Publication Data, 651. doi: 10.1007/978-94-017-5279-4
Mao R., Lang M., Yu X., Wu R., Yang X., Guo X. (2020). Aging mechanism of microplastics with UV irradiation and its effects on the adsorption of heavy metals. J. Hazard. Mater. 393, 122515. doi: 10.1016/j.jhazmat.2020.122515
Moreira F. M. S., Huising E. J., Bignell D. E. (2012). Manual de biología de suelos tropicales: Muestreo y caracterización de la biodiversidad bajo suelo. Primera edición, 360.
Murga-Orrillo H., Irigoín Aguilar J. M., Hilares Vargas S., Bardales Lozano R. M., De Almeida Lobo F. (2019). Adubos e coberturas orgânicas, fontes de nutrientes de liberação lenta na produção de acelga de colheitas múltiplas. Revista de Ciências Agroveterinárias 18 (3), 380–384. doi: 10.5965/223811711832019380
Oesper R. E. (1934). Kjeldahl and the determination of nitrogen. J. Chem. Educ. 11 (8), 457. doi: 10.1021/ed011p457
Olsen S. R., Cole C. V., Watanabe F. S., Dean L. A. (1954). Estimation of available phosphorus in soils by extraction with sodium bicarbonate (Washington, D.C: U.S. Government Printing Office).
Padmavathiamma P. K., Li L. Y., Kumari U. R. (2008). An experimental study of vermi-biowaste composting for agricultural soil improvement. Bioresour. Technol. 99 (6), 1672–1681. doi: 10.1016/j.biortech.2007.04.028
R Core Team (2023) R: A language and environment for statistical computing (Vienna, Austria: R Foundation for Statistical Computing). Available at: https://www.R-project.org/ (Accessed May 12 2023).
Rumahorbo D. P. R., Marheni M., Bakti D. (2020). “Macrofauna soil diversity in chili plants (Capsicum annum L.) with the use of organic and inorganic mulch,” in The 3rd International Conference Community Research and Service Engagements, IC2RSE 2019, North Sumatra, Indonesia, December 04 2019. doi: 10.4108/eai.4-12-2019.2293815
SENAMHI—Servicio Nacional de Meteorología e Hidrología del Perú. (2023). Available at: https://www.senamhi.gob.pe/?p=pronostico-meteorologico.
Shah F., Wu W. (2020). Use of plastic mulch in agriculture and strategies to mitigate the associated environmental concerns. Adv. Agron. 164, 231–287. doi: 10.1016/bs.agron.2020.06.005
Six J., Conant R. T., Paul E. A., Paustian K. (2002). Stabilization mechanisms of soil organic matter: implications for C-saturation of soils. Plant Soil 241, 155–176. doi: 10.1023/A:1016125726789
Sofo A., Mininni A. N., Ricciuti P. (2020). Soil macrofauna: A key factor for increasing soil fertility and promoting sustainable soil use in fruit orchard agrosystems. Agronomy 10 (4), 456. doi: 10.3390/agronomy10040456
Walkley A., Black I. A. (1934). An examination of the detjareff method for determining soil organic matter and a proposed modification to the chronic acid titration method. Soil Sci. 37, 29–38. doi: 10.1097/00010694-193401000-00003
Yu Y. Y., Turner N. C., Gong Y. H., Li F. M., Fang C., Ge L. J., et al. (2018). Benefits and limitations to straw-and plastic-film mulch on maize yield and water use efficiency: A meta-analysis across hydrothermal gradients. Eur. J. Agron. 99, 138–147. doi: 10.1016/j.eja.2018.07.005
Zhang H., Miles C., Gerdeman B., LaHue D. G., DeVetter L. (2021). Plastic mulch use in perennial fruit cropping systems–A review. Scientia Hortic. 281, 109975. doi: 10.1016/j.scienta.2021.109975
Keywords: soil macrofauna, soil temperature, plastic mulch, organic fertilizers, climate extremes
Citation: Murga-Orrillo H, Chuquimez Gonzales JK, Pashanasi Amasifuén B and Arévalo López LA (2023) Vigna unguiculata: a productive option in the face of climate change? Front. Agron. 5:1284173. doi: 10.3389/fagro.2023.1284173
Received: 28 August 2023; Accepted: 02 November 2023;
Published: 28 November 2023.
Edited by:
Tafadzwanashe Mabhaudhi, University of KwaZulu-Natal, South AfricaReviewed by:
Tahir Sheikh, Sher-e-Kashmir University of Agricultural Sciences and Technology of Kashmir, IndiaCopyright © 2023 Murga-Orrillo, Chuquimez Gonzales, Pashanasi Amasifuén and Arévalo López. This is an open-access article distributed under the terms of the Creative Commons Attribution License (CC BY). The use, distribution or reproduction in other forums is permitted, provided the original author(s) and the copyright owner(s) are credited and that the original publication in this journal is cited, in accordance with accepted academic practice. No use, distribution or reproduction is permitted which does not comply with these terms.
*Correspondence: Hipolito Murga-Orrillo, aG11cmdhQHVuYWFhLmVkdS5wZQ==
†ORCID: Hipolito Murga-Orrillo, orcid.org/0000-0001-5039-5838
Disclaimer: All claims expressed in this article are solely those of the authors and do not necessarily represent those of their affiliated organizations, or those of the publisher, the editors and the reviewers. Any product that may be evaluated in this article or claim that may be made by its manufacturer is not guaranteed or endorsed by the publisher.
Research integrity at Frontiers
Learn more about the work of our research integrity team to safeguard the quality of each article we publish.