- 1Department of Soil Science, School of Agriculture, University of Ghana, Accra, Ghana
- 2Department of Agro Enterprise Development, Ho Technical University, Ho, Ghana
- 3Department of Crop Science, School of Agriculture, University of Ghana, Accra, Ghana
Introduction: The main constraint to cowpea, Vigna unguiculata, production in West Africa is unavailability of applied phosphorus due to ligand exchange with clay minerals and precipitation reactions facilitated by low pH and low organic matter.
Materials and methods: To overcome this challenge, cow dung biochar (CB) and poultry litter biochar (PB) were applied as P sources for cowpea cultivation in Plinthustult and Kandiustalf soil in Ghana. The biochar types and triple super phosphate (TSP) were applied to meet the standard phosphorus requirement (SPR) and ½ SPR of the soils. Basal K from KCl was added to the TSP treatment. In addition to a non-amended soil, the treatments were triplicated and arranged in a completely randomized design in a screen house for an efficacy trial using cowpea as the test crop. Moisture content was maintained at 80% field capacity.
Results and discussion: Hundred cowpea seed weight was 20.3 g and 19.6 g for the TSP-amended Plinthustult at SPR and ½ SPR, respectively. This increased to 25.1 g and approximately 27 g at SPR and ½ SPR in the CB- and PB-amended Plinthustult, respectively. Hundred seed weight for TSP at both rates was similar in the two soils. The PB-amended Kandiustalf at SPR had seed with a weight of 27.02 g, 1.1 g heavier than the ½ SPR. The CB-amended Kandiustalf at both rates had 100 seeds weighing 25 g. Residual available P was 334.2 mg/kg and 213.2 mg/kg at SPR and ½ SPR, respectively, in the Plinthustult as opposed to a paltry 2.5 mg/kg at SPR in the TSP counterpart. The study recommends for the two biochar types to be applied at ½ SPR.
Introduction
Cowpea, Vigna unguiculata [L. Walp], is the most popular grain legume produced by smallholder farmers in Africa. It is consumed by about 200 million people in Africa (Oyewale et al., 2013). It offers food, animal feed, fertilizer, and income to resource-poor small-holder farmers in both the Transition and Savannah Agro-ecological Zones (Sheahan, 2012). Increased productivity of cowpea is, thus, key to the attainment of food security (FAOSTAT, 2011).
Low soil fertility is among the most important abiotic factors that cause severe setbacks to cowpea production. Several studies have concluded that in the West African savannah region, yield declines due to poor soil fertility surpass rainfall (Souleymane, 2018). Good soil health is the basis of a vibrant and sustainable food system (Souleymane, 2018). It is, therefore, imperative to adopt sustainable means of improving soil fertility to ensure improved yields of the crop.
Soils of West Africa are highly weathered, mainly dominated by kaolinite and poor in P-bearing parent material (Nye and Bertheux, 1957). There is fixation of phosphorus through ligand exchange and precipitation (Schoumans and Chardon, 2015), making plant-available phosphorus deficiency a major constraint in crop production. Thus, phosphorus availability in these soils is very low. Co-incidentally, phosphorus is one of the main nutrients controlling growth and development of cowpea. This nutrient element is vital in root initiation and growth. It also helps in nodule formation and efficiency of rhizobium-legume symbiosis (Haruna, 2011).
It is estimated that up to 90% of the soluble phosphorus applied to tropical soils is quickly rendered insoluble due to high fixation (Broggi et al., 2010). This has culminated in an agricultural practice where more phosphorus is usually applied than is required by the crop (Zoboli et al., 2016). The poor phosphorus use efficiency of plants grown in tropical soils and the high cost of inorganic fertilizers in recent times, coupled with the fast depletion of the phosphate rock deposits in the world, have made the search for a more viable and sustainable alternative source of the nutrient element a necessity. Amendment of tropical soils with plant and animal residues have not yielded the desired results with regard to P availability due to high haulage and application cost as a result of the low level of P in such residues. Composting to increase P availability has the drawback of long composting time to attain maturity. Composting and application of poultry litter and cow dung to soils are also prone to ammonia and carbon dioxide emissions and runoff of dissolved phosphates (Shakya and Agarwal, 2017). Application of cow dung has also been implicated in the spread of weed seeds. The high temperatures in tropical Africa have led to high organic matter turnover, such that applications would have to be done annually with their attendant high costs.
Transformation of cow dung and poultry litter into biochar by pyrolysis provides a safer, more efficient, and more stable compound (Subedi et al., 2016) for use as P sources. The effective P in carbonized animal manure has been found to be 2.5 times higher than in the feedstock from which it was charred (Chan et al., 2008; Steiner et al., 2010). Biochar is particularly ideal for the tropics where high temperatures lead to the accelerated mineralization of organic materials in the soil. Poultry manure and cow dung abound in tropical Africa, and are usually applied to soil as decomposed or composted amendments as N sources (Ogundare et al., 2015). Use of biochar as a P source for crop production has been limited to plant-based feedstocks (Akumah et al., 2021; Nartey et al., 2021; Sulemana et al., 2021). Transformation of cow dung and poultry manure into biochar for P availability in Africa has received very little attention.
This work, therefore, sought to evaluate the efficacy of cow dung and poultry litter biochar types for use as P sources in the cultivation of cowpea in two inherently P-deficient Ghanaian soils viz. a Kandiustalf (Toje Series) and a Plinthustult (Kokofu Series) soil. Should these two biochar types be as efficacious as inorganic P from triple superphosphate (TSP), then they could be used as P sources for crop cultivation in tropical Africa.
Materials and methods
Soil and sampling
Two inherently P-deficient soils viz. Kokofu Series and Toje Series from two different agro-ecological zones of Ghana were used for the study. The Kokofu Series (a Plinthustult) was sampled from a moist semi-deciduous forest reserve while the Toje series (a Kandiustalf) was sampled from a fallow field within the Coastal Savannah belt of Ghana. Both soils had no known history of legume production.
Kokofu Series is located at the middle slope of the catena of the Forest and Horticulture Research Centre (FOHCREC) at Okumaning, near Kade in the Eastern Region of Ghana. The area has a mean temperature of 30-35 °C during the main rainy season between April and July, with mean annual rainfall between 800-1200 mm. Toje series, on the other hand, is on the upper slope of the Legon Hill catena at the University of Ghana Botanical Gardens in Accra. The site is about 77.0 m above sea level, with a monthly average highest temperature of 29.0°C and lowest temperature of 24.0°C. The annual rainfall amount is 725.0 mm (GMS, 2010).
Vegetation cover was removed from each sampling site, and disturbed soil samples were randomly taken from the plough layer (0-20 cm), homogenized, and composited. A portion was subsampled, air-dried, ground, and passed through a 2 mm sieve to obtain the fine earth fraction for soil physico-chemical characterization. The remaining unprocessed soil was used for a pot experiment in the screen house. Undisturbed soil samples were used for bulk density determination.
The two biochar types, cow dung biochar (CB) and poultry litter biochar (PB), used for the study were respectively prepared from poultry litter and cow dung feed stocks. The cow dung was obtained from the Soil and Irrigation Research Centre of the University of Ghana and the poultry litter from a deep litter system of a commercial farm. These feedstocks were charred using a retort stove, the Kuntan kiln. A fire was lit and the metallic base of the Kuntan kiln placed over the fire to facilitate heating of the base. The chimney of the kiln was then inserted onto the base after which the biomass was heaped around the base. The heat emanating from the hot base then charred the biomass. The average charring temperature during the charring process was taken by an Extech Dual Laser infrared thermometer (Model 42570). The prepared biochar was air-dried, ground, and passed through a 2 mm sieve for chemical characterization.
Characterization of soil and biochar
Physical properties of the soils such as bulk density and particle size distribution were respectively determined by the methods of (Blake and Hartge, 1986) and (Day, 1965). The pH of both soils was measured in water and KCl at 1:1 solution:soil. A pot with drainage holes plugged with glass wool was filled with one kilogram of bulk (un-sieved) soil. The soil in the pot was then saturated with water and covered with polyethylene to avoid evaporation. This was allowed to stand for two days to lose gravitational water. Thereafter, sub samples of the soil were taken into moisture cans, weighed, and oven-dried at a temperature of 105 0C until constant weights were attained to determine moisture-holding capacity.
The pH of the two biochar types were also determined in biochar:water (1:10) suspensions. Total C and N contents of the two soils and two biochar types were determined by dry combustion using a LECO Trumac CNS analyzer (LECO Corporation, Michigan, US). Total P of the samples was determined by wet digestion using HNO3 and 60% HClO4 in the ratio of 1:1.5 of the two reagents. The method of Mehlich-3 was used to extract available P for both soil and biochar samples and the concentration of the extracted P determined using the molybdate-ascorbic acid color development method of Murphy and Riley (1962). Cation exchange capacity and exchangeable bases of the soils were determined using the ammonium acetate method. The exchangeable bases in the soil extract were then read on a Perkin Elmer Analyst 800 Atomic Absorption Spectrophotometer. The standard P requirement (SPR) of the two soils was determined using the method described by Fox and Kamprath (1970).
Screen house studies
In order to evaluate the efficacy of poultry litter and cow dung biochar types as P sources for cowpea growth, a pot experiment was conducted under screen house conditions in the Research Farm at the University of Ghana, Legon. Temperature in the screen house throughout the experiment was between 25 °C and 32 °C. The experiment was conducted in the screen house to control environmental conditions.
The unprocessed Kokofu and Toje Series were packed into cylindrical PVC pipes, 16 cm in diameter, to attain their respective bulk densities of 1.29 g/cm3 and 1.30 g/cm3. The two biochar amendments, poultry litter biochar (PB) and cow dung biochar (CB), and an inorganic amendment of triple superphosphate (TSP) were applied to meet the full standard P requirement (F) and half (½) standard phosphorus requirement (H) of the two soils. The inorganic amendments were supplemented with basal K from muriate of potash with the rate of application estimated from the average soluble and exchangeable K contents in the two biochar types. There were soil treatments with no amendments to serve as control (C). Thus, with two soils, Kokofu Series (K) and Toje Series (T), three amendments (TSP, CB, and PB), three rates (zero P (C), P at half SPR (H), and P at full SPR (F)), and four replicates for each treatment, there were 72 experimental units arranged in a completely randomized design (CRD) in the screen house.
Table 1 shows the standard phosphorus requirement of the two soils, Kokofu Series (K) and Toje Series (T), and the respective rates of the three amendments viz. CB, PB, and TSP applied to attain the full SPR (F) and half SPR (H) of the two soils. From the table, Kokofu Series amended with poultry litter biochar at half SPR (KPBH) received 10.7 mg of the amendment per pot, with the Toje Series counterpart (TPBH) receiving 11.0 mg of the amendment per pot. Kokofu Series amended with TSP at full SPR (KTSPF) received 0.69 mg TSP per pot with its Toje Series counterpart (TTSPF) receiving 0.70 mg TSP per pot.
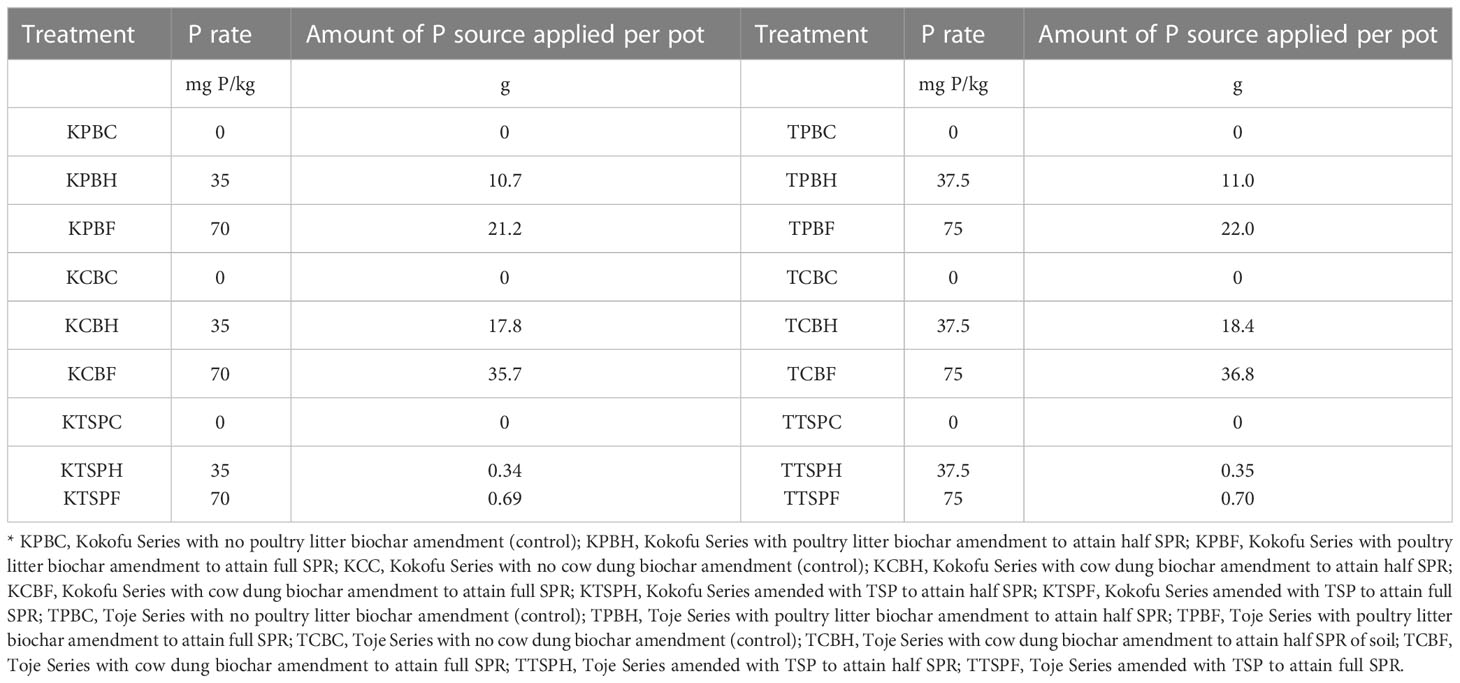
Table 1 Requirements of the two soils at the P application rates from the three amendments to the soils*.
Upon amendment of the soils with the two biochar types, the soils were moistened to 80% water-holding capacity and the pH of the biochar-amended soils was monitored weekly until equilibration was achieved by the end of the fourth week. Three cowpea seeds of variety California Black Eye and of 85% germination percentage were then sown per pot. Moisture content of all the treatment units were kept at 80% throughout the growth of the plant. Eight (8) days after sowing (DAP), the seedlings were thinned to one plant per pot. Average plant heights at harvest for each of the treatments were taken as height for the crop. Other agronomic parameters taken were number of days to 50% flowering, average number of days to 50% maturity, average number of pods per plant, average number of seeds per pod, and the average 100 seed weight per plant for each treatment. There was no need to control insect pest and disease outbreak as the trial was under screen house conditions.
The soil samples from each pot after harvesting were homogenized, subsampled, air-dried, sieved through a 2 mm sieve, and labeled appropriately. The soils were then analyzed for residual pH, EC, total C, and total and available P as outlined in the previous sections.
Statistical analysis
The data were subjected to analysis of variance (ANOVA) using Genstat 12th edition to establish, if any, significant treatment effects using Duncan Multiple Range Test. Means separations were done at a 5% level of significance.
Results
Characterization of soils
The physicochemical properties of the plough layer (0-20 cm) of the two soils used for the study are shown in Table 2. The bulk densities of the soils were 1.30 Mg/m3 and 1.29 Mg/m3 for Kokofu Series and Toje Series, respectively. The two soils had similar sand content, between 66.7 and 66.2%. Kokofu Series had 5.4% more silt content than its Toje Series counterpart with the clay content being 5.9% less than its coastal Savanna counterpart. The two soils according to the USDA (2003) system of classification are sandy clay loam.
Kokofu and Toje Series were very strongly acidic and moderately acidic respectively, with respective pH in water values of 4.8 and 5.7 (Table 2). The two soils had negative ΔpH (pH salt – pH H2O) values of 0.7 and 0.5, respectively for Kokofu and Toje Series. The EC of the soils were very low and below 0.2 dS/m. The Kokofu Series had an organic carbon content of 17.3 g/kg, 7.1 g/kg more than the counterpart from Coastal Savanna (10.2 g/kg). Consequently, Kokofu Series had a higher total P content of 408 mg/kg, 1.65 times more than in the Toje Series (247 mg/kg), and over twice as much total N (1.5 g/kg) than in the Toje Series (0.7 g/kg). Correspondingly, Kokofu Series had more than twice as much available N as in the Toje Series, albeit at very low levels. The two soils had very low available P concentrations of 1.65 mg/kg and 1.25 mg/kg for the Kokofu and Toje Series, respectively.
The exchangeable bases in the two soil types were mainly Ca ranging from 1.21 cmolc/kg in Toje Series to 1.52 cmolc/kg in Kokofu Series. Exchangeable Mg was less than 50% of exchangeable Ca in both soils. Exchangeable K and Na were very low and below 0.2 cmolc/kg in both soils. The CEC of the soils were low, and very low particularly for the Toje Series (5.12 cmolc/kg).
Chemical characterization of biochar
Some chemical properties of poultry litter biochar (PB) and cow dung biochar (CB) are shown in Table 3. The pH of PB and CB in water were alkaline, with values of 8.7 and 8.9, respectively. The alkaline pH of the two biochar types agrees with Lehmann and Joseph (2009), who reported that anaerobic charring of feedstock leads to biochar with neutral to strongly alkaline pH regimes. The EC of the poultry litter biochar and cow dung biochar were quite high, with values of 4.1 and 3.2 dS/m, respectively.
Cow dung biochar had a carbon concentration of 310 g/kg as opposed to 406 g/kg of PLB. The total N content of the CB and PB were quite high and of concentrations 16.2 g/kg and 22.6 g/kg, respectively, resulting in respective C:N ratios of 19.1 and almost 18. The total P content of both biochar types were very high. Total P in CB was 23,600 mg/kg, almost 68% that of PB. Available P in the two biochar types were also correspondingly very high, with PB being approximately 1.67 times more than the concentration in CB. It is worth noting that, despite the high available P contents in the two biochar types, this fraction formed a paltry respective 37.5% and 42.4% of total P in CB and PB. The exchangeable bases in the amendments are presented in Table 3. Generally, the PB is richer in bases than CB. Potassium and calcium formed approximately 83% and 87%, respectively, of the total soluble and exchangeable bases in the two biochar types.
Effects of P source on growth parameters of cowpea grown in Kokofu series
Table 4 shows the effects of the different P amendment sources on the growth parameters of cowpea in the Kokofu Series. Average plant height of cowpea at harvest in the un-amended soils (KPBC, KCBC, and KTSPC) were generally the same, at approximately 45 cm. This increased by almost 6 cm to 51 cm when the Kokofu Series was amended with TSP at both half (KTSPH) and full SPR (KTSPF). Heights of the cowpea plants at harvest increased significantly to 55 cm when cow dung biochar was amended at both half (KCBH) and full SPR (KCBF). The tallest plants with heights of 57 cm were recorded when poultry litter biochar was amended to Kokofu Series at both half SPR (KPBH) and full SPR (KPBF).
It took, on average, 41 days for the cowpea plant in the un-amended Kokofu Series to get to 50% flowering (Table 4). This reduced significantly by five days when the crop was grown in the TSP-amended Kokofu Series (KTSPF and KTSPH). In the cow dung biochar-amended soils at half and full SPR, days to 50% flowering decreased significantly to 30 days. The least number of days to 50% flowering, which was 29, was recorded in the poultry litter biochar-amended soils (KPBF and KPBH). It took 52 days for 50% of the crops in the un-amended Kokofu Series to reach maturity (Table 4). This was shortened to 48 and 50 days, respectively, when the soil was amended with TSP at full and half SPR. In the cow dung biochar-amended soil, irrespective of the rate of application, the cowpea plant took 41 days on average for 50% of the crops to get to maturity. At half and full SPR, 50% of the cowpea grown in the PB-amended Kokofu Series took 38 and 39 days, correspondingly, to mature.
The number of pods harvested per cowpea plant grown in the un-amended Kokofu Series were 15 on average. This number increased significantly to approximately 24 and 26, respectively, when the soil was amended with TSP at half SPR (KTSPH) and full SPR KTSPF). In the cow dung biochar-amended Kokofu Series at the two P application rates, number of pods per cowpea plant harvested were between 42 and 43. This increased significantly to 46 and 47 pods in plants grown in the PB-amended soil at full SPR (KPBF) and half SPR (KPBH), respectively. The number of seeds per pod from plants in the un-amended Kokofu Series (KPBC, KCBC and KTSPC) were 9 on average, which increased significantly to 10 and 11, respectively, in TSP at half (KTSPH) and full SPR (KTSPF). In the KCBH and KCBF soils, the number of seeds per pod were 14. In the KPBH and KPBF soils, number of seeds per pod were 16 and 15, respectively. The average 100 seed weight from plants grown in the un-amended soil (KPBC, KCBC and KTSPC) was 17 g, increasing to 19.6 and 20.3 g, respectively, in the TSP-amended soil at half and full SPR. The CB-amended soils had 100 seeds weighing 25 g at both P application rates. The heaviest 100 seed weights were recorded in the PB-amended soils at half SPR (27 g) and full SPR (26.6 g).
It can be deduced from Table 4 that the half SPR rates of the poultry litter biochar (KPBH), which received about 35 mg/kg equivalent of P, recorded higher values for all the cowpea growth parameters. In general, the growth parameters of cowpea grown in the Kokofu Series were in the order of KPBH > KPBF > KCBH = KCBF > KTSPH = KTSPF > un-amended soil.
Effects of P source on growth parameters of cowpea grown in Toje Series
Table 5 shows the effects of the diverse P amendment sources on the growth parameters of cowpea grown in Toje Series. In the un-amended Toje Series (TPBC, TCBC, and TTSPC), plants were the shortest at time of harvesting with an average height of 45 cm. When the cowpea in the Toje Series were fertilized with TSP at half (TSPH) and full SPR (TSPF), plant height increased significantly by approximately 6 cm. When the Kandiustalf was amended with the cow dung biochar at both half SPR and full SPR, plant height at harvest increased significantly to 55.49 and 55.07 cm, respectively. Cowpea plants were the tallest in the poultry litter biochar-amended Toje Series with average height of 56.7 cm at full SPR rate of P application.
About 50% of the cowpea plants in the un-amended Toje Series took approximately 43 days to flower and 54 days to reach physiologic maturity (Table 5). When amended with TSP, 50% of the plants took 38.12 and 50.25 days to flower and mature, respectively, at half SPR (TTSPH), which further reduced to 37.4 and 49.50 days at full SPR (TTSPF). In the cow dung biochar-amended soils, irrespective of the P application rate, 50% of the plants took approximately 31 and 42 days to flower and mature, respectively. The number of days for 50% of the plants to flower and mature were shortest in the PB-amended soils. The plants took 30 days to flower and 39 and 41 days to mature, respectively, at both half SPR and full SPR. Number of pods per plant from the un-amended Toje (TPBC, TCBC, and TTSPC) were 12, with about nine seeds per pod. Cowpea from the TSP-amended soil at half and full SPR had 21 and 23 pods per plant, respectively, which produced, respectively, 10 and 11 seeds per pod. Number of pods per plant in the cow dung biochar-amended soil were 43 and 42, correspondingly for half and full SPR application rates, with respective average seeds per pod of 14 and 13. The poultry litter-amended soils produced plants bearing almost 45 and 43 pods, respectively, at half and full SPR. These pods bore on average 15 and 14 seeds, respectively, at half and full SPR. Hundred seed weight from cowpea plants in the un-amended soil was 16.92 g, which increased significantly to 19.38 and 20.15 g, respectively, at half and full SPR of TSP application. The cow dung biochar-amended soil produced cowpea seeds with 100 seed weight of 25 g, irrespective of application rate. The poultry litter biochar-amended soil at half SPR produced the heaviest 100 seeds weight of 27.02 g. Just as was observed in the Kokofu Series, the organically amended soils produced plants with superior growth and yield attributes to the TSP-amended soils, and the poultry litter biochar applied at half SPR produced plants with superior growth and yield parameters.
Residual chemical properties of Kokofu Series
Results of the effect of the amendments on residual pH, EC, total C, total phosphorus, and available phosphorus in the Kokofu Series after harvesting cowpea at maturity are presented in Table 6. The pH of the un-amended soil (KPBC, KCBC, and KTSPC) remained strongly acidic at 4.8 -5.0 like the initial pH (4.8) of the soil at the onset of the experiment. The pH of the residual soil amended with the inorganic fertilizer, KTSPH (5.0) and KTSPF (4.8), also remained unchanged, and similar to the soil pH before the application of the amendment. All the biochar amendments raised the pH of the residual soil to values between 6.0 and 6.2 (Table 6). The increase in pH was between 1.2 and 1.4 pH units, with the amendments at the full SPR of cow dung biochar (KCF) giving the highest increase in pH (6.2).
The EC of the un-amended and the TSP-amended soils were similar, with values of about 0.009 dS/m, 0.008 dS/m higher than the EC at the onset of the experiment. The residual EC in the biochar-amended soils increased from that of the un-amended at harvest, with values of approximately 0.2 to 0.3 dS/m. It is noteworthy that despite the increase in EC upon amendment with the two biochar types, it was lower than the threshold of 4 dS/m to cause salt injury to subsequent crops.
The total P at the onset of the screen house experiment was 408 mg/kg (Table 2) and this did not change in the un-amended soil, as the residual P was between 401.8 and 403.1 mg/kg. The residual P in the TSP-amended soil at half (KTSPH) and full SPR (KTSPF) was 424.9 and 422.6 mg/kg, respectively, which were statistically the same (p<0.001) as that of the un-amended soil. All the biochar-amended soils were significantly higher in total P than in the inorganic amended soil at SPR (Table 6). These residual P contents of the biochar-amended soils increased more than between 1.8 and 3 folds over the total P contents of the soil at the onset of the screen house experiment. The residual total P accumulations in the biochar amended soils were in the order of KPBF > KCBF > KPBH > KCBH with respective concentrations of 1234.1, 966.8, and 854.1, 733.6 mg/kg. Residual available P contents of 1.81 mg/kg and 2.45 mg/kg for both KTSPF and KTSPH were recorded, which were statistically (p<0.001) similar to the concentration of available P in the un-amended soil. The biochar-amended soils recorded significantly higher residual available P than the inorganic amended soil. Among the biochar-amended soils, residual available P was in the same order as in the total P fraction i.e. KPBF (334.16 mg/kg) > KCBF (223.79 mg/kg) > KPBH (213.2 mg/kg) > KCBH (117.17 mg/kg)
Residual total carbon in the un-amended soil (21.88 -22.30 g/kg) and the TSP-amended soil at half SPR, KTSPH, (21.88 mg/kg) did not differ significantly. The soils that were TSP-amended to the full SPR had higher residual total carbon contents than their counterparts that were amended to half the SPR of the soil. All the biochar-amended residual soils recorded a significantly higher total C (p<0.001) than the inorganic and un-amended soils. The order of total carbon accumulation in the soils organically amended was KPBF > KPBH > KCBF>KCBH. It is worthy of note that total carbon accumulations in the biochar-amended soils were more than twice the contents in the inorganically amended soils (Table 6).
Residual chemical properties of Toje series
Table 7 illustrates the effects of the various P amendment sources on residual pH, EC, total C, and total and available and phosphorus of Toje Series. The pH of the un-amended (TPBC, TCBC, TTSPC) and inorganically amended Toje series (TTSPF, TTSPH) remained unchanged after cultivation, with a value of 5.8, similar to that of the soil before cultivation. The EC, which was 0.0012 dS/m in the initial soil, increased to 0.007 ds/m in the un-amended and inorganically amended soils. Electrical conductivity increments in the biochar-amended soils were much higher, attaining values of between 0.02 and 0.07 dS/m.
The residual total carbon content of the un-amended and the two TSP-amended Toje Series were approximately 16 g/kg. The total carbon contents were, however, very high in the biochar-amended Toje Series. The residual total carbon contents were almost 3.6 and 4 times, respectively, higher in the poultry biochar-amended soil at half SPR and SPR than in the residual un-amended soil. The cow dung biochar-amended soil at full SPR and half SPR were three times and 2.6 times, respectively, higher in total carbon than their un-amended soil counterpart.
Total P in the original Toje Series was 247 mg/kg, which decreased marginally after cultivation to 240.5 mg/kg in the un-amended and TSP-amended Toje Series at half SPR (TTSPH). Residual total P in the poultry litter biochar-amended Toje Series increased 2.83 times at half SPR and 4.3 times at SPR over the concentration in the soil at the onset of the trial. In the cow dung biochar-amended Toje Series at half SPR (TCBH), accumulation of total P was 1.5 times higher than total P fraction in the original soil. When cow dung biochar was applied at SPR, total P accumulation was almost 3.4 times above the original soil. Residual available P (1.06 mg/kg) of the un-amended soils also did not change significantly from the concentration in the original soil. Available P in the residual Toje Series of the un-amended and the inorganically amended soil at half and full SPR were similar to the concentration of the nutrient in the soil at the onset of the trial. In the poultry litter biochar-amended soils, available P increased tremendously to 169 and 286 mg/kg at half SPR and SPR, respectively, after harvest. Similarly, available P concentration in the cow dung biochar-amended soils after harvest also increased substantially with levels of 112.5 and 185 mg/kg for application rates at half SPR and SPR, respectively. It is clear from Table 7 that residual P from poultry litter biochar at half SPR was far higher in concentration than the counterpart at SPR for the cow dung biochar in the Toje Series.
Growth effects of cowpea and trends for all the treatments under Toje Series (Table 7) were similar to those observed under Kokofu Series (Table 6). It is key to note that the residuals of all individual treatments under Kokofu series were higher as compared to those under Toje series.
Discussion
The medium bulk densities of both soils, Kokofu (1.30 Mg m-3) and Toje (1.29 Mg m-3), could be attributed to their sandy clay loam texture. These physical attributes of the two soils would enable them to support the cultivation of many arable crops. The Δ pH (pH salt –pH H2O) of both Kokofu and Toje is an indication that the two soils have a net negative charge (Nartey et al., 1997) as evident in their CEC, albeit low capacities. The negative charge of pH can be attributed to the highly weathered nature of the soils (Abekoe and Sahrawat, 2001). The lower pH value reported for Kokofu Series from the semi-deciduous zone than Toje Series (Coastal Savanna) is due to the higher rainfall in the former leading to leaching of basic cations. These pH values are corroborated by their lower base saturation of 25.5% and 35.1%, respectively.
The forest vegetation in the Kokofu Series contributes to higher litter fall and hence higher organic carbon than the Toje series, which has sparse vegetation. The faster decomposition rates of organic matter in the warmer Toje Series may have also led to its lower organic carbon content. In line with the higher level of OC in both soils, CEC, N, and available P were expectedly higher in Kokofu Series than Toje Series.
Electrical conductivity are indications of the presence of dissolved ions and salinity (Ackah et al., 2011). The low EC values of the two soils suggest that the soils are not saline, which is corroborated by the low exchangeable bases in the two soils. The poor fertility status of the two soils with the particularly low available P contents of less than 2 mg/kg make them ideal for use as media for the efficacy trial as there is likely to be a response to P amendment.
The two biochar types are alkaline in pH, making them ideal for use as liming material in the two acidic soils. These two biochar types upon amendment to the two acidic soils are likely to raise soil pH as evident in their respective residual pHs to make P more available and promote root proliferation. Poultry feed is formulated with high levels of salt. The higher EC of the PB than the CB could be due to the inherently higher salt contents of the former’s feedstock. The nutritional contents of the cow dung biochar (CD) and poultry litter biochar (PB) are a reflection of the feeding regime of the animals. Cattle in Ghana generally feed on highly cellulosic grass, compared to the highly proteinaceous feed formulations of poultry. It, therefore, stands to reason that poultry litter biochar is far richer in C, N, and especially available P than its cow dung biochar counterpart. Similarly, the highly proteinaceous feed on which the chickens are fed led to biochar with higher nitrogen concentration than CB. The higher available P in the PB than CB and the relatively lower C:N ratio of the former implies that availability of P would be more enhanced in PB-amended soils than its CB counterpart.
The higher increases in height and yield of cowpea from the biochar-amended soils than from inorganic applications can be related to the fact that biochar improved the fertility of the soils by increasing the pH, total carbon, and available P contents as reflected in the residual soil properties. The 1.67 times higher available P in the poultry litter biochar (PB) than its cow dung biochar type (CB), coupled with the latter’s lower C:N and higher total P contents, translated into superior cowpea growth parameters and ultimately higher yields at each of the two P application rates. For example, at half SPR P application rate on Kokofu and Toje Series, cowpea plants grown in the poultry-amended soils matured 3 days earlier than those from the cow dung biochar counterparts. It is, thus, clear that poultry litter biochar is better in quality as a P fertilizer source than its cow dung biochar type.
Accessibility, availability, and ease of charring are factors that will have to be considered when deciding on which of the two biochar types to use. In the northern parts and Savannah Zones of West Africa, where cattle rearing is popular, confining the animals in kraals at night after grazing during the day would help make cow dung more accessible and available as a feedstock for charring. Drying of this feedstock and ultimate conversion into biochar could become a source of livelihood for the indigenes in the Savannah Zones of West Africa. In the more humid southern parts of West Africa, where poultry rearing is more common, the litter will be more available. Considering the fact that poultry feed is formulated from cereals, soya bean, and fish, conversion of poultry litter manure into biochar for use as a P source would sequester more P and carbon into the soil to close the nutrient loop.
The Kokofu and Toje Series, which had pH in water of 4.8 and 5.7, respectively, increased to near neutral of between 6.0 and 6.2 upon amendment with the biochar types. Organic carbon content also increased in the biochar-amended soil. Thus, with increased pH and organic carbon contents, it is expected that deprotonation of the carboxylic groups in the amended PB and CB soils will increase. Acetic, citric, and oxalic acids, the main components of the biochar added, have pKa of about 4.8 (Evangelou, 1998). Toje Series is an Alfisol, with Kokofu Series being an Ultitisol. Kaolinite will, therefore, be the dominant clay mineral in the two soils. This clay mineral has an aluminol group with a pKa of 5.4 (Evangelou, 1998). The pKa values of the carboxylic groups from the biochar and aluminol group of kaolinite are, respectively, 1.2 and 0.6 pH units below the least residual pH (6.0) of the biochar-amended Kokofu Series soils. The pKa of the two functional groups are 2 and 1.4 pH units lower than the Toje Series biochar-amended soil that produced the least residual pH (6.8). Thus, most of the carboxylic and aluminol groups in the biochar-amended soils will be deprotonated. With increased negative charges in the soil and increased available P from the CB and PB, it stands to reason that there would be higher repulsion of added P and the negatively charged organic and inorganic colloids in the soil, leading to increased P availability culminating in better uptake by plants and hence higher yields.
In the un-amended and TSP-amended soils, residual pH was similar to that at the onset of the experiment. There was also no increase in organic carbon content. Consequently, it is not expected that CEC of the soil will increase. Therefore, the availability of P in the TSP-amended soil will be lower than that in the biochar-amended soil, as corroborated by the lower available P concentration in the residual soils of the former. This may partly explain the relatively lower growth parameters measured in TSP-amended soils than in biochar-amended soils. Sole fertilization with inorganic P may, therefore, not be the panacea to cowpea production, especially on acid soils. According to Lehmann and Joseph (2009) and Sulemana et al. (2021), biochar provides better access to phosphorus in soils. The high residual available P of between 117 mg/kg and 334 mg/kg in the biochar-amended soils should be enough to sustain subsequent cowpea production, decreasing the need for fertilization since biochar operates as a slow-release fertilizer (Struhs et al., 2020). The inorganically amended soil with a residual available P of less than 3 mg/kg will certainly have to be fertilized again for subsequent crop production. The similar growth parameters of cowpea in the half SPR-amended soils than the full SPR counterpart implies that the former should be the preferred application rate for farmers. This is because it will be cost-saving in terms of amount of amendment to be incorporated into the soil.
Kokofu Series had an inherently lower pH than Toje series but, after amendment, both soils had near neutral pH. Kokofu Series had higher residual available P than Toje Series, obviously due to its sharper response to the liming effects of the two biochar types. Thus, the higher yield of cowpea grown in Kokofu Series compared to Toje when amended with the two biochar types could be attributed to the better conditions created after amendment. It is, thus, clear that cowpea production is sensitive to pH change and increases in P availability. The negative impact of low soil pH on cowpea productivity is corroborated by the relatively poor yields in the TSP-amended and un-amended soils.
The high EC of the cow dung and poultry biochar types did not influence the respective EC of the Kokofu and Toje Series negatively as the increment in the property was still less than 0.1 dS/m in the residual soils, making the soils safe for the cultivation of most arable crops with no threat to salt injury. The higher residual EC in the biochar-amended soils could be the result of the salt concentration and exchangeable basic cations in the poultry and cow dung biochar types, which tends to increase EC of the amended soils. A similar rise in EC with biochar addition to soils has also been observed in other related works (Chintala et al., 2014; Meena and Prakasha, 2020). Increase in residual available P, especially in the biochar-amended soils, is as a result of the inherently high total and available P in the amendment added.
There were higher residual total carbon contents in the PB-amended soils than the CB counterparts due to the inherently higher carbon contents in the poultry litter biochar type, implying that soils amended with poultry litter biochar would sequester more carbon. Biochar contains more recalcitrant carbon than the feedstock from which it is prepared (Lehmann and Joseph, 2009). Thus, for the highly weathered and degraded soils of tropical Africa where temperatures are warmer, addition of the two biochar types, especially poultry litter biochar, would sequester more carbon and hence improve the fertility status of the soils.
Conclusions
Poultry litter biochar (PB) and cow dung biochar (CB) are efficacious P sources for growth and development of cowpea and could be used as P fertilizers, especially in acid soils. Poultry litter and cow dung biochar could also be used as liming materials on acid soils to improve P availability. The two biochar types also have the ability of sequestering carbon and phosphorus into soils for subsequent crop production. Application of the two biochar types at half the standard phosphorus requirement of the soils should be the preferred application rate, as yield parameters are as good as those from the standard P requirement application. Poultry litter and cow dung biochar types are better used as P sources and liming agents instead of for carbon sequestration since they have a C:N ratio below 20. Poultry litter biochar is a better P source than cow dung biochar. Application of poultry litter biochar at ½ SPR in the two soils culminated in cowpea maturing 3 days earlier than crops from the cow dung biochar-amended soils and seven days earlier than crops from the TSP-amended soils at SPR.
Data availability statement
The original contributions presented in the study are included in the article/Supplementary Material. Further inquiries can be directed to the corresponding author.
Author contributions
EN conceptualized the study, supervised student, and drafted the original manuscript. AR carried out the screen house study as part of his MPhil thesis research work at the University of Ghana, Legon. NS was involved in original draft preparation, curation of data, and proofreading. TA, AA, and CA proofread the article and were involved in data curation. MA supervised the student, proofread the article, and was involved in data curation. All authors contributed to the article and approved the submitted version.
Conflict of interest
The authors declare that the research was conducted in the absence of any commercial or financial relationships that could be construed as a potential conflict of interest.
Publisher’s note
All claims expressed in this article are solely those of the authors and do not necessarily represent those of their affiliated organizations, or those of the publisher, the editors and the reviewers. Any product that may be evaluated in this article, or claim that may be made by its manufacturer, is not guaranteed or endorsed by the publisher.
References
Abekoe M. K., Sahrawat K. L. (2001). Phosphate retention and extractability in soils of the humid zone in West Africa. Geoderma 102 (1-2), 175–187. doi: 10.1016/S0016-7061(00)00110-5
Ackah M., Agyemang O., Anim A. K., Osei J., Bentil N. O., Kpattah L., et al. (2011). Assessment of groundwater quality for drinking and irrigation: the case study of Teiman-Oyarifa Community, Ga East Municipality, Ghana. Proc. Int. Acad. Ecol. Environ. Sci. 1 (3-4), 186.
Akumah A. M., Nartey E., Ofosu-Budu G., Ewusie E., Offei B., Adamtey N. (2021). Innovations in market crop waste compost production: use of black soldier fly larvae and biochar. Int. J. Recycl. Organ. Waste Agric. 10, 185–202. doi: 10.30486/ijrowa.2021.1899111.1071
Blake G. R., Hartge K. H. (1986). “Bulk density,” in In: Klute, A., Ed., Methods of Soil Analysis, Part 1—Physical and Mineralogical Methods, 2nd Edition, Agronomy Monograph 9, (Madison: American Society of Agronomy—Soil Science Society of America) 363–382
Broggi F., Oliveira A. C. D., Freire F. J., Freire M. B. D. S., do Nascimento C. W. (2010). Adsorption and chemical extraction of phosphorus as a function of soil incubation time. Rev. Bras. Engenharia Agrícola e Ambiental 14 (1), 32–38. doi: 10.1590/S1415-43662010000100005
Chan K. Y., Van Zwieten L., Meszaros I., Downie A., Joseph S. (2008). Using poultry litter biochars as soil amendments. Soil Res. 46 (5), 437–444. doi: 10.1071/SR08036
Chintala R., Mollinedo J., Schumacher T. E., Malo D. D., Julson J. L. (2014). Effect of biochar on chemical properties of acidic soil. Arch. Agron. Soil Sci. 60 (3), 393–404. doi: 10.1080/03650340.2013.789870
Day P. R. (1965). Particle fractionation and particle-size analysis. Methods Soil Analysis: Part 1 Phys. Mineralogical Properties Including Stat Measurement Sampling 9, 545–567. doi: 10.2134/agronmonogr9.1.c43
Evangelou V. P. (1998). Environmental Soil and Water Chemistry: Principles and Applications (New York: John Wiley and Sons), 564.
FAOSTAT (2011) Bulletin of Tropical Legumes. Available at: http://www.icrisat.org/tropical.
Fox R. L., Kamprath E. J. (1970). Phosphate sorption isotherms for evaluating the phosphate requirements of soils. Soil Sci. Soc. America J. 34 (6), 902–907. doi: 10.2136/sssaj1970.03615995003400060025x
Ghana Meteorological Service (2010). Raw Data on Climate 2010 Annual Report (Accra, Ghana Ghana Meteorological Service).
Haruna I. M. (2011). Dry matter partitioning and grain yield potential in Sesame (Sesamum indicum L.) as influence by Poultry manure, nitrogen and phosphorus at Samaru, Nigeria. Elixir Agric. 39, 4884–4887.
Lehmann J., Joseph S. (Eds.) (2009). Biochar for environmental management Vol. Vol. 1 (London: Earthscan).
Meena H. M., Prakasha H. C. (2020). Effect of biochar, lime and soil test value based fertilizer application on soil fertility, nutrient uptake and yield of rice-cowpea cropping system in an acid soil of Karnataka. J. Plant Nutr. 43 (17), 2664–2679. doi: 10.1080/01904167.2020.1793188
Murphy J. A. M. E. S., Riley J. P. (1962). A modified single solution method for the determination of phosphate in natural waters. Analytica chimica Acta 27, 31–36. doi: 10.1016/S0003-2670(00)88444-5
Nartey E. K., Darko D. A., Sulemana N. (2021). Integrated bioplant and groundnut husk biochar compost application on yield of lettuce in a Rhodic Kandiustalf. Front. Sustain. Food Syst. 5. doi: 10.3389/fsufs.2021.676776
Nartey E., Dowuona G. N., Ahenkorah Y., Mermut A. R., Tiessen H. (1997). Amounts of distribution of some forms of phosphorus in ferruginous soils of the interior savanna zone of Ghana l. Ghana J. Agric. Sci. 30 (2), 135–143. doi: 10.4314/gjas.v30i2.1965
Nye P. H., Bertheux M. H. (1957). The distribution of phosphorus in forest and savannah soils of the Gold Coast and its agricultural significance. J. Agric. Sci. 49 (2), 141–159. doi: 10.1017/S0021859600036121
Ogundare S. K., Owa F. D., Etukudo O. O., Ibitoye-Ayeni N. K. (2015). Influence of different nitrogen sources on the growth and yield of three varieties of okra (Abelmoschus esculentus) in Kabba, Kogi State, Nigeria. Agric. Sci. 6 (10), 1141. doi: 10.4236/as.2015.610109
Oyewale O. R., Acad S., Biosci J., Cobley S. (2013). Introduction to the Botany of Tropical Crops (Harlow: Longman Group Limited-Essex), 79–98.
Schoumans O. F., Chardon W. J. (2015). Phosphate saturation degree and accumulation of phosphate in various soil types in The Netherlands. Geoderma 237, 325–335. doi: 10.1016/j.geoderma.2014.08.015
Shakya A., Agarwal T. (2017). Poultry litter biochar: an approach towards poultry litter management–a review. Int. J. Curr. Microbiol. App Sci. 6 (10), 2657–2668. doi: 10.20546/ijcmas.2017.610.314
Sheahan C. M. (2012). Plant Guide for Cowpea (Vigna unguiculata) (Cape May, NJ: USDA-Natural Resources Conservation Service, Cape May Plant Materials Center).
Souleymane A. (2018). Selection of Cowpea (vigna unguiculata (l). walp) for High Yield Under Low Soil Phosphorus Conditions (Doctoral Thesis) (University of Ghana).
Steiner C., Das K. C., Melear N., Lakly D. (2010). Reducing nitrogen loss during poultry litter composting using biochar. J. Environ. Qual. 39 (4), 1236–1242. doi: 10.2134/jeq2009.0337
Struhs E., Mirkouei A., You Y., Mohajeri A. (2020). Techno-economic and environmental assessments for nutrient-rich biochar production from cattle manure: a case study in Idaho, USA. Appl. Energy 279, 115782. doi: 10.1016/j.apenergy.2020.115782
Subedi R., Taupe N., Ikoyi I., Bertora C., Zavattaro L., Schmalenberger A., et al. (2016). Chemically and biologically-mediated fertilizing value of manure-derived biochar. Sci. Total Environ. 550, 924–933. doi: 10.1016/j.scitotenv.2016.01.160
Sulemana N., Nartey E. K., Abekoe M. K., Adjadeh T. A., Darko D. A. (2021). Use of biochar-compost for phosphorus availability to Maize in a concretionary ferric lixisol in Northern GHANA. Agronomy 11 (2), 359. doi: 10.3390/agronomy11020359
Keywords: Plinthustult, Kandiustalf, precipitation, ligand exchange, standard phosphorus requirement
Citation: Nartey EK, Sulemana N, Razak A, Adjadeh TA, Akumah AM, Amoatey C and Abekoe MK (2023) Poultry litter and cow dung biochar as P sources for cowpea cultivation in two Ghanaian soils. Front. Agron. 5:1233255. doi: 10.3389/fagro.2023.1233255
Received: 01 June 2023; Accepted: 03 July 2023;
Published: 20 July 2023.
Edited by:
Muhammad Bilal Khan, Ayub Agriculture Research Institute, PakistanReviewed by:
Amit Anil Shahane, Central Agricultural University, IndiaAbdul-Halim Abubakari, University for Development Studies, Ghana
Copyright © 2023 Nartey, Sulemana, Razak, Adjadeh, Akumah, Amoatey and Abekoe. This is an open-access article distributed under the terms of the Creative Commons Attribution License (CC BY). The use, distribution or reproduction in other forums is permitted, provided the original author(s) and the copyright owner(s) are credited and that the original publication in this journal is cited, in accordance with accepted academic practice. No use, distribution or reproduction is permitted which does not comply with these terms.
*Correspondence: Eric K. Nartey, ZW5hcnRleUB1Zy5lZHUuZ2g=