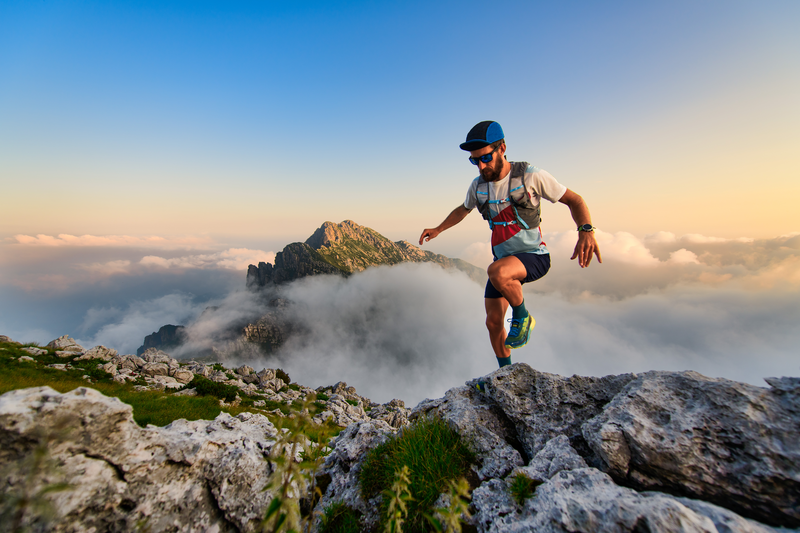
95% of researchers rate our articles as excellent or good
Learn more about the work of our research integrity team to safeguard the quality of each article we publish.
Find out more
METHODS article
Front. Agron. , 29 August 2023
Sec. Agroecological Cropping Systems
Volume 5 - 2023 | https://doi.org/10.3389/fagro.2023.1223639
This article is part of the Research Topic Cover Crops and Green Manures: Providing Services to Agroecosystems View all 10 articles
Cover crops are often mentioned as a way to decrease nutrient losses during the fallow period. Species mixtures of crucifer-legume have been shown to effectively take up nitrogen (N) and sulphur (S) to decrease losses due to leaching, but their ability to simultaneously take up other key macro- and micronutrients remains unknown. Our study assessed the performances of a wide variety of bispecific crucifer-legume mixtures to provide synergetic uptake of multiple nutrients to target nutrient-recycling ecosystem services. Cultivars from eight crucifer and seven legume species were tested as sole and bispecific cover crops at an experimental site near Toulouse, France. Seven macronutrients (C, N, P, K, S, Ca and Mg) and six micronutrients (B, Cu, Mn, Zn, Fe and Na) were measured in both species. Crucifer-legume mixtures showed synergetic uptake of nutrients per ha compared to sole cover crops for some nutrients through niche complementarity and facilitation processes and net competition for other nutrients. Species mixtures induced both i) higher nutrient concentrations for Mn and Fe in crucifers and Ca and B in legumes) and ii) lower concentrations for P, K, Ca and B in crucifers and Cu and Fe in legumes, indicating that the interactions differed among the species mixtures. Nevertheless, the nutrient uptake measured in shoots were always higher in species mixtures than in sole crops (LER > 1) demonstrating the compatibility and complementarity of crucifer and legume mixtures in providing multiple-nutrient catch-crop and recycling related ecosystem services. Despite overall positive interactions and synergetic complementarities, the results also highlight that some net negative competition occurred for some nutrients. Thus, further investigation is still necessary to completely understand the processes behind multiple-nutrient acquisition in species mixtures.
Nutrient availability is one of the main factors that influence soil fertility and plant growth in natural and agricultural systems (Halvin et al., 2005). Soil erosion and leaching are major causes of nutrient loss in agroecosystems that are often increased in intensive agricultural systems due to over-fertilisation, tillage and/or leaving soils bare during periods of high rainfall (Drinkwater and Snapp, 2007; Justes, 2017). The most important nutrients for plant growth are not equally sensitive to leaching and erosion. Macronutrient anions such as nitrate (NO3-) and sulphate (SO42-) are highly mobile and leachable in soils (Thorup-Kristensen et al., 2003). Cations such as ammonium (NH4+), calcium (Ca2+), magnesium (Mg2+) and potassium (K+) can also be leached (Lehmann and Schroth, 2003), but in smaller quantities than NO3- (Halvin et al., 2005). While Mg2+ is more leachable than Ca2+, loss of Mg can sometimes exceed the subsequent plant requirement (Mengel et al., 2001; van der Heijden et al., 2014). Phosphorus (P) is mostly immobile in the soil, so its leaching is negligible, but it is sensitive to erosion by runoff (Torstensson et al., 2006; Aronsson et al., 2007). Among the micronutrients, boron (B) and manganese (Mn) are susceptible to leaching, while zinc (Zn) and copper (Cu) are more tightly bound to organic matter than other micronutrients and are thus less mobile and susceptible to leaching (Halvin et al., 2005).
Under temperate conditions, heavy rainfall during the fallow period exposes bare soil to runoff and leaching between two main cash crops; this period thus has a high risk of nutrient losses (Carranca, 2012; Justes, 2017). Growing cover crops during the fallow period can provide multiple ecosystem services (Justes, 2017; Couëdel et al., 2019; Lavergne et al., 2021; Scavo et al., 2022; Van Eerd et al., 2023). For example, it is an effective solution for 1) reducing potentially leachable nutrient concentrations in the soil solution (Thorup-Kristensen et al., 2003; Constantin et al., 2010; De Notaris et al., 2018), 2) reducing the amount of water drained (Meyer et al., 2019) 3) reducing soil erosion by water and wind (Blanco-Canqui et al., 2015; Basche et al., 2016; Blanco-Canqui, 2018; Mohammed et al., 2021) and 4) improving soil physical properties (Blanco-Canqui and Ruis, 2020; Haruna et al., 2020). Besides their ability to take up nutrients from the soil, cover crops can also make nutrients available for the next crop through mineralisation of crop residues following termination (Thorup-Kristensen et al., 2003). Surprisingly, besides the well-known nitrogen (N) cycle, the potential of cover crops to take up and recycle other nutrients has rarely been quantified. The few studies that have assessed the ability of cover crops to simultaneously take up multiple nutrients revealed variability in performances among families and species (Wang et al., 2008; Wendling et al., 2016). An effective option to encourage synergetic uptake of multiple nutrients by cover crops combines a variety of families that have different characteristics and performances. Thus, species mixtures of cover crops could be a solution to increase nutrient uptake over that of sole cover crops due to niche complementarity and facilitation processes.
The review of Xue et al. (2016) highlighted that bispecific cereal-legume cash-crop mixtures grown for grains could increase the uptake of several soil nutrients through root-architecture complementary and differences in requirements between species. Studies of cash crops have shown that Zn and Fe concentrations in legumes increase when mixed with a cereal, through complementarity for resources and interspecific facilitation (Zuo and Zhang, 2008; Zuo and Zhang, 2009). However, antagonism in nutrient acquisition was also observed, e.g when mixed with maize, peanut had higher Fe, Zn, P and K concentrations, but lower Ca concentrations and no change in Mn or N concentrations (Inal et al., 2007). Similarly, Xia et al. (2013) observed that N, P, K, Ca and Mn concentrations in cucumber shoots and roots were significantly higher when grown in a species mixture with green garlic, but Mg concentrations were lower than those in sole crops. Such trade-offs in simultaneous nutrient acquisition require assessing cover crop mixtures to determine their potential to provide nutrient ecosystem services for leaching mitigation and recycling to the next cash crop.
Among families used in cover cropping, crucifers (Brassicaceae) are the most effective catch crops for nitrogen (N) and sulphur (S) uptake (Couëdel et al., 2018a; Couëdel et al., 2018b) while legume (Fabaceae) cover crops are especially useful for providing exogenous N to the system thanks to biological symbiotic fixation (Jensen, 1986; Tribouillois et al., 2016). Crucifer-legume mixtures grown as cover crops showed complementarity in providing ecosystem services related to N and S cycles (Couëdel et al., 2018a; Couëdel et al., 2018b). However, the only study we identified in the scientific literature that focussed on multiple-nutrient acquisition by crucifer-legume cover crop mixtures was published by Koefender et al. (2016), who showed that a mixture of radish and vetch took up more nutrients than when in sole crops and more than oat-vetch mixtures. Despite their benefits in mixtures, crucifers may be a poor companion crop for nutrient acquisition, as they cannot host arbuscular mycorrhizal fungi (AMF) and thus may negatively (or at least not positively) impact legume nutrient acquisition. Indeed, AMF facilitate acquisition of nutrients such as P, Mn, Ca, Fe and B in many mixtures of plant species (Jin et al., 2014; Teste et al., 2014). Moreover, potential negative effects of a crucifer companion crop on the acquisition of nutrients involved in symbiotic N fixation by legumes (e.g. P, Fe, Mo, Cu) need to be assessed (O’Hara et al., 1988; Brear et al., 2013). Legumes can be good companion crops as they facilitate the uptake of certain nutrients (e.g. P, K, Ca, Mg) by crucifers. Indeed, symbiotic N fixation by legumes liberates protons that acidify the rhizosphere, increasing the solubilisation of nutrients potentially available to the crucifer (Hauggaard-Nielsen and Jensen, 2005; Hinsinger et al., 2011; Tang et al., 2021).
The goal of our study was to analyse the potential of multiple-nutrient acquisition to provide multiple ecosystem services in bispecific crucifer-legume cover crop mixtures. Our specific aim is to propose a methodological analysis on a first dataset in order to illustrate an ecological theory using agronomic data, never demonstrated for crop multi-elements uptake. We believe that this novelty would be very useful in the literature as a first step to understand competition between cover crop mixtures for multi-nutrients. To this end, we tested a wide variety of species, including several cultivars. The objectives of our methodological analysis were to 1) analyse all essential macronutrients (carbon (C), P, K, Mg and Ca) and the most important micronutrients (Mn, Cu, Fe, B, Zn and Na) and 2) assess the complementarity and facilitation performances of bispecific crucifer-legume cover crops in acquiring these nutrients. The original method used is the “4C” approach developed by Justes et al. (2021), which assesses effects of competition, complementarity, cooperation (facilitation) and compensation in species mixtures grown in agroecosystems for providing ecosystem services.
We tested two hypotheses for using cover crop sole crops and species mixtures in short (summer to mid-autumn) fallow periods:
1) The relation (complementarity or competition) between crucifers and legumes depends on the nutrient considered and the species mixed.
2) Bispecific mixtures mutualise their acquisition of multiple nutrients, unlike when the species are in sole crops, mainly due to the niche complementarity of nutrient uptake capacity and root systems when exploring the soil.
A field experiment was conducted in 2014 at the Lamothe experimental farm of INP Purpan, which is located at Seysses, in south-western France (43.506° N, 1.237° E), and has a silty clay loam soil. According to the Köppen climate classification, Lamothe has an oceanic climate (temperate without a dry season but with warm summers). Cover crops were sown on 19 August and terminated on 4 November. Cumulative rainfall during the growing period was 140 mm, and the mean temperature was 19°C (see Table S1 for monthly climate averages). The soil mineral N content at sowing was 93 kg N ha−1 (see Table S2 for more detailed soil chemical characteristics). The experiment followed a completely randomised design with three replicates in blocks (See Figure S1 for detailed field scheme). Each elementary plot, which contained 10 rows per treatment, measured 18 m2 (12 x 1.5 m). To avoid confounding effects of plant-plant interactions between adjacent treatments, only the six rows in the middle of each plot were harvested and used for soil measurements.
Cover crop species were selected for their ability to grow rapidly during a short fallow period in the autumn and to maximise the services related to N and S (Couëdel et al., 2018a; Couëdel et al., 2018b). Crucifer and legume species and cultivars were selected for their diversity in shoot and root architecture, sensitivity to photoperiod and precocity. All bispecific mixtures (Table S3) contained one crucifer and one legume and were designed to minimise competition according to expert knowledge and recently published information (Tribouillois et al., 2016). Therefore, only mixtures of species that had already been identified as compatible and having good growth performance when intercropped were tested, to keep the experiment within the human and financial resources available. The following five crucifer species were used: rape (Brassica napus L.), white mustard (Sinapis alba L.), Ethiopian mustard (Brassica carinata A. Braun.), turnip rape (Brassica rapa L. subsp. oleifera) and radish (Raphanus sativus L.). The following seven legume species were used: Egyptian clover (Trifolium alexandrium L.), crimson clover (Trifolium incarnatum L.), common vetch (Vicia sativa L.), purple vetch (Vicia benghalensis L.), hairy vetch (Vicia villosa Roth.), Narbonne vetch (Vicia narbonensis L.), soya bean (Glycine max (L.) Merr.) and faba bean (Vicia faba L.). Three additional crucifer species (Indian mustard (Brassica juncea L. Czern.), turnip (Brassica rapa L. subsp. rapa) and rocket (Eruca sativa Mill.)), along with more cultivars of the five original crucifer species, were tested only in sole crops to assess shoots and roots nutrient concentrations and uptake in a wider range of species and cultivars (Table S3). Supplementary information displaying results on this larger range of crucifer sole crops are available in Figures S1, S2 and Table S4.
Species grown as sole cover crops were sown at densities recommended for cover crops to obtain plant cover with similar densities and rapid growth and soil coverage. Sowing densities for sole cover crops were 800 seeds m-2 for all clover species; 150 plants m-2 for Ethiopian mustard; 100 plants m-2 for white mustard and all vetch species; 80 plants m-2 for rape, radish and turnip rape; 70 plants m-2 for soya bean and 40 plants m-2 for faba bean. Sowing densities for bispecific mixtures were half of the corresponding sole cover crop density of each species (50% crucifers and 50% legumes) to create a substitutive design of species mixtures. Seeds of both species were mixed in the row and sown with an experimental seeding machine, similar to a seed drill, to obtain a homogenous mixed-plant cover crop in the row to maximise plant-plant interactions.
After shallow tillage, seeds were sown 1.5-2.0 cm deep in rows 15 cm apart. The percentage of each sown species was monitored during crop emergence. Irrigation (30 mm) was applied after sowing to ensure homogeneous emergence and establishment of cover crops. Irrigation and fertilisers were not applied during the cover crop growing period to mimic “normal farming conditions”. The experimental field had previously hosted crop rotations with rape, and neither the rotation in general nor the rape in particular had experienced N or S deficiency. In addition, just before the experiment, no soil-nutrient deficiencies were detected according to French soil-analysis standards (Table S2). We thus assumed that nutrients in the soil were not a limiting factor in our experiments, in particular for the cover crops, which have a shorter growing period and thus produce less biomass, which decreases nutrient requirements. An anti-grass herbicide (Axéo®) was sprayed at 1.2 litre per hectare to control a strong emergence of weeds (mainly annual grasses) in September to decrease the influence of extraneous factors.
Cover crops were sampled 2.5 months after sowing, which is consistent with the usual practice of incorporating cover crops into the soil before sowing the next winter crop, as autumn is warm and rainy in this region, which promotes rapid growth of cover crops. All above-ground biomass and, for crucifers alone, root biomass, was collected from 1 m2 per replicate (more precisely, two 0.5 m2 quadrats were randomly put in each 12 m plot length). Roots were collected to a depth of ca. 30 cm by digging with a fork. The roots of legume species were not considered in the results due to technical difficulties in sampling fine roots in the silty clay loam soil of the study site. Most of the root biomass of legumes is composed of fine roots, while the roots of crucifers are generally tuberous, which makes them easier to collect and measure more accurately. Shoots and roots were separated using a sharp knife and secateurs, and the root systems were carefully washed with cold water to remove the remaining soil. Samples were washed, dried at 80°C for 48 h, weighed and ground to measure total C, N, S, P, K, Ca, Mg, Na, B, Cu, Fe, Mn, and Zn via elemental analyses based on the Dumas method (Elementar MicroVario Cube, Germany) for C and N, and by inductively coupled plasma - atomic emission spectroscopy (ICP-AES) for the other nutrients (at the SADEF-accredited laboratory, Aspach-le-Bas, France).
The land equivalent ratio (LER), defined as the sole crop area required to reach the same biomass as a multi-species mixture or intercrop (Willey, 1979), equals the sum of the partial LER (pLER) of each mixed species. We used the LER index to assess nutrient uptake as Couëdel et al., 2018a; Couëdel et al., 2018b did for N and S acquisition, and applied here for all nutrients analysed:
where pLER_C and pLER_L are the pLER of mixed crucifers and legumes, respectively, NutrientupIC is the nutrient uptake of the given nutrient for a given species (crucifer or legume) in the mixture, and NutrientupSC is the nutrient uptake of the same species as a sole cover crop.
LER > 1 indicates that the bispecific mixture takes up resources more efficiently than the species grown as a sole cover crop (i.e. complementarity exceeds competition). In contrast, LER< 1 indicates that competition exceeds complementarity for resource uptake, while LER = 1 indicates that the balance of plant-plant interactions is null. In a substitutive design (50% of each sole cover crop species), pLER > 0.5 indicates that, at the plant level, the species takes up more nutrients in a mixture than as a sole cover crop, while pLER< 0.5 indicates that interactions in the mixture negatively influence the species’ nutrient uptake. Overall, when the two pLERs are significantly greater than 0.5, which yields a LER significantly greater than 1, complementary and facilitation processes are stronger than competition in plant-plant interactions. Inversely, when the two pLERs are less than 0.5, competition processes are stronger than complementarity for nutrient uptake (Justes et al., 2021).
Analysis of variance (ANOVA) was used to assess effects of cover crop species and cover crop type (i.e. sole crucifer, sole legume or mixture) on nutrient acquisition per unit area (kg ha-1). Tukey’s post-hoc test was used to distinguish differences among cover crop types for each nutrient. Normality was tested using the Shapiro-Wilk test while homoscedasticity was tested using the Bartlett’s test. Percentage data were non-normal after arcsine-square root transformation, then nonparametric Wilcoxon tests were performed to test 1) differences in nutrient concentration between sole and mixed crucifers or legumes, and 2) the significance of differences in LER between cover crops and their relative difference from 1.0 (i.e. the net neutral effect of the 4C processes), as well as those between pLER_C and pLER_L and 0.5 (i.e. the net neutral effect at half density). Statistical analysis was performed using R software (R core team, 2023), and differences among treatments were considered significant at P< 0.05. Cultivar data were pooled as no significant differences in nutrient concentrations or acquisition were observed between cultivars. Results were then analysed by crop family and species to increase the robustness of the results and to highlight key points.
Nutrient concentrations of mixed crucifers and legumes differed from those in their respective sole crops (Figure 1). For crucifers, Fe (+350% in shoots, +228% in roots in mixtures) and Mn (+40% in shoots, +200% in roots in mixtures) were the only nutrients with significantly higher concentrations in both mixed shoots and roots compared to sole cover crops (Figure 1) especially for radish and turnip rape (Table 1). Mg (+15%) and Cu (+20%) concentrations were significantly higher in crucifer mixed roots only (Figure 1; Table 1). In comparison, concentrations were significantly lower in crucifer mixture shoots than those in sole cover crops for P (-8%, especially for radish), K (-13%), Ca (-21%, except for Ethiopian mustard) and B (-25%). C (-8%) and Na (-24%) concentrations were significantly lower in crucifer mixture roots than those in sole cover crops (Figure 1; Table 1). Zn was the only nutrient with higher concentrations in crucifer mixture roots (+21%), while it was lower in mixture shoots (-10%). The concentrations of other macronutrients, such as N and S, did not differ significantly between crucifers in mixtures and sole cover crops (Figure 1; Table 1).
Figure 1 Macronutrient (%) and micronutrient (ppm) concentrations in shoots and roots of crucifers and shoots of legumes. Values correspond to the mean of sole (SC) and mixed (Mix) crucifers or legumes. Asterisks indicate a significant difference in macro- and micronutrient concentrations between a given family in a sole crop and in mixtures (P< 0.05). Error bars represent standard errors.
Table 1 Macronutrient (%) and micronutrient (ppm) concentrations in shoots of crucifer and legume sole crops (SC) and species mixtures (Mix).
For legume shoots, Ca (+26%) and B (+27%) were the only nutrients with significantly higher concentrations in mixtures compared to sole cover crops (Figure 1). This pattern was observed for all legume species except for Egyptian clover and soya bean, whose Ca and B concentrations did not differ significantly between mixtures and sole cover crops (Table 1). In contrast, Cu (-12%) and Fe (-34%) were the only nutrients with significantly lower concentrations in mixed legume shoots compared to sole legume cover crops (Figure 1). For Fe, this pattern was observed in all species except faba bean, crimson clover and soya bean, for which the pattern was the opposite (Table 2). All other nutrient concentrations (C, N, S, P, K, Mg, Na, Mn and Zn) did not differ significantly between legume shoots in mixtures and in sole cover crops (Figure 1; Table 1).
Table 2 Macronutrients (kg ha-1) and micronutrients (g ha-1) uptake in shoots of crucifer and legume sole crop (SC) and species mixtures (Mix).
Interestingly, some differences in nutrient concentrations in mixtures compared to sole cover crops showed opposite patterns for crucifer shoots compared to legume shoots (Figure 1). Fe was the only nutrient that had significantly higher concentrations in crucifers and lower concentrations in legumes than those in the respective sole crops (Figure 1), suggesting that the crucifers may have outcompeted the legumes for Fe. In contrast, Ca and B were the only nutrients that had significantly higher concentrations in legumes and lower concentrations in crucifers than those in the respective sole crops (Figure 1), suggesting that the legumes may have outcompeted the crucifers for Ca and B.
Nutrient uptake by cover crop mixtures was significantly higher or the same as that of the best sole cover crop family, except for Cu and Fe, for which the legume sole crop took up more nutrients than the mixtures (Figure 2). Shoot biomass, expressed as C content, was the only component that mixtures produced significantly more of (1263 kg C ha-1) than crucifer sole crops (1014 kg C ha-1) or legume sole crops (941 kg C ha-1), especially for mixtures with turnip rape, white mustard, soya bean and Egyptian clover (Figure 2; Table 2).
Figure 2 Macronutrients (kg ha−1) and micronutrients (g ha−1) acquired in shoots of crucifers and legumes in sole crops and species mixtures. Values correspond to the mean of crucifer (Cr) and legume (Le) sole crops or the mean of both species included in a mixture (Mix). Different letters indicate treatments with significant differences at P< 0.05. Only shoots of crucifers and legumes were included in the analysis.
Compared to crucifer sole cover crops, species mixtures (legume and crucifer combined) acquired significantly more N (+40%) and took up significantly more P (+16%), Cu (+81%), Fe (+763%), Mn (+122%) and Zn (+32%) (Figure 2). In contrast species mixtures did not acquired significantly more of these nutrients compared to legume sole cover crops (Figure 2). The N acquisition and P and Cu uptake were particularly higher in mixtures including turnip rape and white mustard compared to their corresponding sole cover crops (Table 2).
In contrast, compared to legume sole cover crops, species mixtures (legume and crucifer combined) took up significantly more S (+180%), K (+137%), Ca (+84%), Mg (+22%) and B (+26%, except for faba bean and hairy vetch), while these nutrients did not differ significantly in crucifer sole cover crops (Figure 2; Table 2). In mixtures, soya bean and crimson clover had higher S, K, Ca and B uptake; common vetch had higher K and Ca uptake; and Egyptian clover had higher K and Ca uptake than those in their respective sole crops (Figure 2; Table 2). Mg and Na were the only nutrients with no significant difference in uptake between the mixture and crucifer and legume sole cover crops (Figure 2; Table 2).
The LER was significantly greater than 1 for all nutrients, indicating that the bispecific mixtures had significantly greater nutrient-acquisition capacity than sole cover crops (Figure 3). The LER of C, N, S, P, K, Ca, Mg, B, Cu and Zn was ca. 1.25, while the LER of Mn, Na and Fe was 1.53, 1.74 and 3.93, respectively. The crucifer partial LER (pLER_C) was significantly greater than 0.5 for all nutrients except Na and B, indicating that crucifers took up more nutrients in mixtures than as sole cover crops, which explained most of the high LERs (Figure 3). The legume partial LER (pLER_L) was significantly greater than 0.5 for Ca, Na and B, indicating that legumes took up more of these nutrients per plant in mixtures than as sole cover crops (Figure 3). The pLER_L did not differ significantly from 0.5 for all other nutrients indicating that mixture had no effect on legume acquisition per plant for these nutrients.
Figure 3 Partial land equivalent ratio (pLER) of crucifers (red) and legumes (blue). The land equivalent ratio (LER) equals the sum of the two partial LERs. Each value represents the mean of species mixtures. Asterisks indicate that pLER and LER were significantly greater than 0.5 and 1.0, respectively. Error bars represent standard errors.
At the species level, all crucifers had a pLER_C > 0.5, except for rape for N, P, K, Ca, B, and Na (y axis, Figure 4). Crucifer species competed differently with the associated legume in mixture as shown by different pLER_L according to the crucifer included in mixture (x axis, Figure 4). For example, legumes had pLER_L< 0.5 for most nutrient when mixed with white mustard and radish while they had pLER_L > 0.5 for all nutrients when mixed with rape (x axis, Figure 4).
Figure 4 Partial land equivalent ratios (LER) for nutrient acquisition of crucifers (LERp_C) as a function of that for legumes (LERp_L). Each point represents the mean of species included in species mixtures. The solid line represents y = x (corresponding to LER = 1). Dashed lines represent pLER_C = 0.5 and pLER_L = 0.5. In area (j), both species grow better in mixtures than as sole crops, indicating that effects of species complementary and cooperation are stronger than those of competition (and vice versa in area (k)). Areas (i-1) and (i-2) indicate that the legume dominates the crucifer and vice versa in (l-1) and (l-2). Plant-plant interactions lead to an advantage of mixture in (i-2) and (l-2), but a poorly balanced mixture due to too much competition between the two species in (i-1) and (l-1). See Justes et al. (2021) for more details. mt, mustard; Ethi, Ethiopian; V, vetch; C, clover.
Among legumes, some species had a pLER_L< 0.5 such as hairy vetch (for nearly all nutrients), purple vetch (for S and Cu), faba bean (for S, P, K and Cu) and common vetch (for Mn and Fe), while other legume × nutrient combinations had a pLER_L > 0.5 (Figure 4).
None of species mixtures had both pLER_C and pLER_L< 0.5, indicating that overall competition in mixtures was never stronger than complementarity and compensation. In comparison, approximately half of the species mixtures had a pLER_L and pLER_C > 0.5, indicating that complementarity and facilitation were stronger than competition (Figure 4).
Our study indicated that cover crop mixtures influenced nutrient acquisition of all species tested as cover crops, but the influence depended on the nutrient considered. We used the “4C concept” developed by Justes et al. (2021) to understand the main species interactions in mixtures. LER exceeded 1 for all cover crop family × nutrient combinations, indicating that the overall performances of species mixtures were driven by plant-plant complementarity and cooperation (facilitation) more than competition. This validates our hypothesis that interspecific interactions between crucifers and legumes are synergistic due to niche complementary and/or facilitation processes, even if the degree of synergy depends on the nutrient. For most nutrients, an LER > 1 was due mainly to higher biomass in mixtures, with relatively constant nutrient concentrations in shoots. For a few nutrients, however, the difference in concentration was larger than the difference in plant biomass, and because biomass was higher in most mixtures, some nutrient concentrations (Mn, Fe and Na) may have driven the higher biomass in mixtures. Indeed, Fe and Mn concentrations in crucifers were much higher when grown in mixtures than as sole cover crops, which suggests 1) stronger intraspecific competition among crucifer plants in sole crops than interspecific competition with legume plants in mixtures, and/or 2) facilitation by legumes of Fe and Mn acquisition by crucifers. This agrees with results of Inal et al. (2007), who observed higher Fe concentrations in maize intercropped with a legume (peanut). To our knowledge, our study is the first to observe such a large increase in Fe and Mn in crucifers intercropped with legume cover crops. Surprisingly, crucifers in mixtures had significantly lower P, K, and Ca concentrations but the same Mg concentrations, while other authors have suggested that legumes facilitate acquisition of these nutrients, such as in cereal-legumes mixtures (Hauggaard-Nielsen and Jensen, 2005; Hinsinger et al., 2011; Tang et al., 2021). Instead, legumes seemed to compete strongly with the crucifer for Ca, as their Ca concentrations increased in mixtures. Although crucifers cannot host AMF that could facilitate acquisition of nutrients such as P, Mn, Ca, Fe and B in many types of mixtures (Jin et al., 2014; Teste et al., 2014), legumes in mixtures did not have lower P, Mn or B concentrations than those of sole legumes. Nevertheless, crucifers competed slightly or strongly with the legume for Cu and Fe, respectively, which could decrease the legume’s ability to fix N (O’Hara et al., 1988; Brear et al., 2013). Interestingly, Fe concentrations in mixtures were lower in vetches and crimson clover but higher in Egyptian clover, soybean and faba bean. Unlike cereals, which exude phytosiderophores that can increase Fe and Zn bioavailability for the companion legume or crucifer (Zuo and Zhang, 2009; Xue et al., 2016; Jiao et al., 2021; Sadeghzadeh et al., 2021), crucifers do not have the same capacity to increase Fe availability in the soil. Nevertheless, when intercropped with a cereal, crucifers do not decrease Fe, Mn, Cu or Zn concentrations in the grain (e.g. Xia et al., 2013 for maize/turnip intercropping). Thus, the increase or decrease in Fe concentrations in mixed crucifers and legumes, respectively, could be due to strong competition of the crucifer with the legume more than a change in the degree of facilitation. Moreover, in basic soils (not present in our study) and unlike crucifers, most legumes release carboxylic acids that dissolve phosphate ions from bound forms, such as calcium and iron phosphates that are otherwise unavailable to plants and immobile in the soil. Consequently, legumes can then make Fe more available for the companion crucifer (Mikić et al., 2015).
Cross-analysis of nutrient concentrations in plants could help detect antagonisms or facilitation between species for a given nutrient that would decrease acquisition of other nutrients and thus the performance of the entire mixture (i.e. Liebig’s Law). Rietra et al. (2017) found synergy between P and K acquisition and antagonism between K and Mg acquisition. Our study confirms these results in crucifers, as lower K concentrations in mixtures than those in sole crops were associated with lower P concentrations but higher Mg concentrations. Nevertheless, cross-analysis of nutrient acquisition requires further studies at more sites and for additional years to definitively validate the net effect of nutrient-acquisition processes by cover crops as synergy or antagonism.
Multiple-nutrient acquisition per unit area by cover crops is a good proxy of the performance of their catch-crop function and ecosystem service for decreasing nutrient losses through leaching and/or runoff and increasing nutrient recycling for the next cash crop. Previous research has provided limited insights into the acquisition of multiple nutrients by sole cover crops (Wang et al., 2008; Wendling et al., 2016) and even fewer studies have focused on cover crop mixtures (Koefender et al., 2016). We found that mixed crucifer and legume cover crops exhibit a synergistic effect on nutrients acquisition. Cover crop mixtures composed with legumes and non-legumes acquired as much or even more of each nutrient compared to the best-performing sole cover crops (Figure S4), as observed in previous studies of N and S elements (Couëdel et al., 2018a; Couëdel et al., 2018b). In other words, adding a legume to crucifer cover crops can increase the uptake of C, N, Fe, Cu and Mn, while adding a crucifer to legume cover crops can increase the uptake of C, S, K, Ca, Mg and B without decreasing those for other nutrients. Besides nutrients uptake synergy, biomass and C accumulated were the only components for which species mixtures performed better, indicating more photosynthesis per unit area than both sole crucifers and legumes, confirming results that highlighted higher biomass and C accumulated in mixtures than in sole crops (Wortman et al., 2012; Mortensen et al., 2021). P, Mg, B and Zn uptake were also slightly higher in mixtures, albeit not statistically significant, than in both crucifer and legume sole cover crops, suggesting potential complementarity of mixtures in providing catch-crop effects for these nutrients as well.
In addition to the multiple-nutrient catch-crop effects, multiple-nutrient recycling effects (for availability for the next crop) of cover crops are another set of ecosystem services that needs to be evaluated. Nutrient recycling have been extensively studied only for P (Takeda et al., 2009; Maltais-Landry et al., 2016; Teles et al., 2017) and a few other nutrients, such as N, S, K, Ca and Mg (Eriksen et al., 2004; Brunetto et al., 2011; Tiecher et al., 2017). Interestingly, recycling of N and S showed different patterns (Tribouillois et al., 2016; Couëdel et al., 2018a; Couëdel et al., 2018b), such as the C:N ratio was not the only driver of nutrient recycling, as supported by the key influence of the C:S ratio on S recycling. Therefore, we advocate the need for further studies to address multiple macro- and micronutrient recycling processes from cover crop decomposition and species mixtures in general. Such studies would help assessing the multi-nutrient ecosystem service effects of cover crops. Indeed, among the growing literature that assesses multiple services provided by cover crops (Schipanski et al., 2014; Finney et al., 2017; Chapagain et al., 2020), such as biocontrol, biomass production and soil C storage, nutrient ecosystem services are assessed mainly under the prism of the N cycle, while other macro- and micronutrients also generate key ecosystem services (Daryanto et al., 2018). By assessing the multi-nutrient uptake capabilities of species mixtures composed of crucifers and legumes, our study contributes valuable insights for assessing their performance across a broader spectrum of ecosystem services.
Overall, crucifer-legume cover crop mixtures provide synergetic multiple-nutrient catch-crop services by acquiring as much or more of each nutrient than the best sole cover crop. In species mixtures, crucifers acquired certain nutrients in much larger quantities, such as Fe (ca. four-fold) and Mn (ca. two-fold), than in sole crops. Crop nutrition status revealed strong niche complementarity and/or facilitation in crucifer-legume mixtures, in which crucifers had higher Mn and Fe concentrations, and legumes had higher Ca and B concentrations. Net competition and/or dilution effects of nutrient concentration were also identified in the cover crop mixtures tested, as legumes tended to have a negative impact on crucifer concentrations of Ca, P, K and B, while crucifers had a negative impact on legume concentrations of Cu and Fe. Our work validated the potential of species complementarity for multi-nutrient uptake using a wide range of crucifer-legume species and cultivars. However further studies should include additional sites with a variety of soil and climate conditions to further validate these first results and help design more efficient cover crop mixtures to achieve high levels of multiple nutrient-related ecosystem services under different growing conditions.
The raw data supporting the conclusions of this article will be made available by the authors, without undue reservation.
LA and EJ, designed and funded the study. AC prepared the data and performed all preliminary and final analyses. All authors were involved in the interpretation of the results and contributed to writing the original version of the manuscript and improving the subsequent ones. All authors contributed to the article and approved the submitted version.
This study was part of the CRUCIAL project, which was financially supported by the French Ministry of Agriculture (CASDAR project no. C-2013-05) and the Occitanie Region (CLE project no. 13053068). This project has received funding from the European Union’s Horizon Europe research and innovation programme under grant agreement No 101081973 - IntercropValuES programme.
The authors thank Annick Basset from Jouffray Drillaud and Cedric Monprofit from RAGT Semences for their expert advice in selecting cover crops for the project. We also thank François Perdrieux and Gaël Rametti for their effective technical help at the Domaine de Lamothe site of INP-EI Purpan, and Eric Lecloux and Didier Raffaillac for their support in sampling plants and laboratory analysis. We also thank Simon Giuliano, Gaëlle van Frank and Antoine Parisot for their helpful discussions. We thank Michelle and Michael Corson for reviewing the English language.
The authors declare that the research was conducted in the absence of any commercial or financial relationships that could be construed as a potential conflict of interest.
All claims expressed in this article are solely those of the authors and do not necessarily represent those of their affiliated organizations, or those of the publisher, the editors and the reviewers. Any product that may be evaluated in this article, or claim that may be made by its manufacturer, is not guaranteed or endorsed by the publisher.
The Supplementary Material for this article can be found online at: https://www.frontiersin.org/articles/10.3389/fagro.2023.1223639/full#supplementary-material
Aronsson H., Torstensson G., Bergström L. (2007). Leaching and crop uptake of N, P and K from organic and conventional cropping systems on a clay soil. Soil Use Manag 23, 71–81. doi: 10.1111/j.1475-2743.2006.00067.x
Basche A. D., Kaspar T. C., Archontoulis S. V., Jaynes D. B., Sauer T. J., Parkin T. B., et al. (2016). Soil water improvements with the long-term use of a winter rye cover crop. Agric. Water Manag. 172, 40–50. doi: 10.1016/j.agwat.2016.04.006
Blanco-Canqui H. (2018). Cover crops and water quality. Agron. J. 110, 1633–1647. doi: 10.2134/agronj2018.02.0077
Blanco-Canqui H., Ruis S. J. (2020). Cover crop impacts on soil physical properties: A review. Soil Sci. Soc. Am. J. 84, 1527–1576. doi: 10.1002/saj2.20129
Blanco-Canqui H., Shaver T. M., Lindquist J. L., Shapiro C. A., Elmore R. W., Francis C. A., et al. (2015). Cover crops and ecosystem services: Insights from studies in temperate soils. Agron. J. 107, 2449–2474. doi: 10.2134/agronj15.0086
Brear E. M., Day D. A., Smith P. M. C. (2013). Iron : an essential micronutrient for the legume – rhizobium symbiosis. Front. Plant Sci. 4. doi: 10.3389/fpls.2013.00359
Brunetto G., Ventura M., Scandellari F., Alberto C., Kaminski J., de Melo G. W., et al. (2011). Nutrient release during the decomposition of mowed perennial ryegrass and white clover and its contribution to nitrogen nutrition of grapevine. Nutr. Cycl Agroecosyst. 90, 299–308. doi: 10.1007/s10705-011-9430-8
Carranca C. (2012). “Nitrogen use efficiency by annual and perennial crops,” in Farming for food and water security. Ed. Lichtfouse E. (Dordrecht: Springer Netherlands), 57–82.
Chapagain T., Lee E. A., Raizada M. N. (2020). The potential of multi-species mixtures to diversify cover crop benefits. Sustain 12, 1–16. doi: 10.3390/su12052058
Constantin J., Mary B., Laurent F., Aubrion G., Fontaine A., Kerveillant P., et al. (2010). Effects of catch crops, no till and reduced nitrogen fertilization on nitrogen leaching and balance in three long-term experiments. Agric. Ecosyst. Environ. 135, 268–278. doi: 10.1016/j.agee.2009.10.005
Couëdel A., Alletto L., Justes É (2018a). Crucifer-legume cover crop mixtures provide effective sulphate catch crop and sulphur green manure services. Plant Soil 426, 61–76. doi: 10.1007/s11104-018-3615-8
Couëdel A., Alletto L., Tribouillois H., Justes E. (2018b). Cover crop crucifer-legume mixtures provide effective nitrate catch crop and nitrogen green manure ecosystem services. Agric. Ecosyst. Environ. 254, 50–59. doi: 10.1016/j.agee.2017.11.017
Couëdel A., Kirkegaard JÆ, Alletto L., Justes E. (2019). Crucifer-legume cover crop mixtures for biocontrol : Toward a new multi-service paradigm. Adv. Agron. 157, 55–139. doi: 10.1016/bs.agron.2019.05.003
Daryanto S., Fu B., Wang L., Jacinthe P., Zhao W. (2018). Earth-Science Reviews Quantitative synthesis on the ecosystem services of cover crops. Earth-Sci. Rev. 185, 357–373. doi: 10.1016/j.earscirev.2018.06.013
De Notaris C., Rasmussen J., Sørensen P., Olesen J. E. (2018). Nitrogen leaching: A crop rotation perspective on the effect of N surplus, field management and use of catch crops. Agric. Ecosyst. Environ. 255, 1–11. doi: 10.1016/j.agee.2017.12.009
Drinkwater L. E., Snapp S. S. (2007). Nutrients in agroecosystems: rethinking the management paradigm. Adv. Agron. 92, 163–186. doi: 10.1016/S0065-2113(04)92003-2
Eriksen J., Thorup-Kristensen K., Askegaard M. (2004). Plant availability of catch crop sulfur following spring incorporation. J. Plant Nutr. Soil Sci. 167, 609–615. doi: 10.1002/jpln.200420415
Finney D. M., Murrell E. G., White C. M., Baraibar B., Barbercheck M. E., Bradley B. A., et al. (2017). Ecosystem services and disservices are bundled in simple and diverse cover cropping systems. Agric. Environ. Lett. 2, 1–5. doi: 10.2134/ael2017.09.0033
Halvin J., Beaton J., Tisdale S., Nelson W. (2005). Soil fertility and fertilizers: An introduction to nutrient management, 7th ed (Upper Saddle River, NJ: Pearson Prentice Hall).
Haruna S. I., Anderson S. H., Udawatta R. P., Gantzer C. J., Phillips N. C., Cui S., et al. (2020). Improving soil physical properties through the use of cover crops: A review. Agrosystems Geosci. Environ. 3, 1–18. doi: 10.1002/agg2.20105
Hauggaard-Nielsen H., Jensen E. S. (2005). Facilitative root interactions in intercrops. Plant Soil 274, 237–250. doi: 10.1007/s11104-004-1305-1
Hinsinger P., Betencourt E., Bernard L., Brauman A., Plassard C., Shen J., et al. (2011). P for two, sharing a scarce resource: soil phosphorus acquisition in the rhizosphere of intercropped species. Plant Physiol. 156, 1078–1086. doi: 10.1104/pp.111.175331
Inal A., Gunes A., Zhang F., Cakmak I. (2007). Peanut/maize intercropping induced changes in rhizosphere and nutrient concentrations in shoots. Plant Physiol. Biochem. 45, 350–356. doi: 10.1016/j.plaphy.2007.03.016
Jensen E. S. (1986). The influence of rate and time of nitrate supply on nitrogen fixation and yield in pea (Pisum sativum L.). Fertil Res. 10, 193–202. doi: 10.1007/BF01049349
Jiao N., Wang F., Ma C., Zhang F., Jensen E. S. (2021). Interspecific interactions of iron and nitrogen use in peanut (Arachis hypogaea L.)-maize (Zea mays L.) intercropping on a calcareous soil. Eur. J. Agron. 128, 126303. doi: 10.1016/j.eja.2021.126303
Jin C. W., Ye Y. Q., Zheng S. J. (2014). An underground tale : contribution of microbial activity to plant iron acquisition via ecological processes. Ann. Bot. 113, 7–18. doi: 10.1093/aob/mct249
Justes E., Bedoussac L., Dordas C., Frak E., Louarn G., Boudsocq S., et al. (2021). The 4C approach as a way to understand species interactions determining intercropping productivity. Front. Agric. Sci. Eng. 8, 1–13. doi: 10.15302/J-FASE-2021414
Koefender J., Schoffel A., Manfio C. E., Golle D. P. (2016). Biomass and nutrient cycling by winter cover crops. Rev. Ceres 63, 816–821. doi: 10.1590/0034-737X201663060010
Lavergne S., Vanasse A., Thivierge M. N., Halde C. (2021). Using fall-seeded cover crop mixtures to enhance agroecosystem services: A review. Agrosystems Geosci Environ. 4, 1–18. doi: 10.1002/agg2.20161
Lehmann J., Schroth G. (2003). "Nutrient leaching," in Trees, crops and soil fertility: concepts and research methods., 151–166. doi: 10.1079/9780851995939.0000
Maltais-Landry G., Scow K., Brennan E., Torbert E., Vitousek P. (2016). Higher flexibility in input N: P ratios results in more balanced phosphorus budgets in two long-term experimental agroecosystems. Agric. Ecosyst. Environ. 223, 197–210. doi: 10.1016/j.agee.2016.03.007
Mengel K., Kirkby E., Kosegarten H., Appel T. (2001). Principles of plant nutrition. 5th edn (Dordrecht: Kluwer Academic Publishers).
Meyer N., Bergez J. E., Constantin J., Justes É (2019). Cover crops reduce water drainage in temperate climates : A meta-analysis. Agron. Sustain Dev. 39 (3), 1–11. doi: 10.1007/s13593-018-0546-y
Mikić A., Ćupina B., Rubiales D., Mihailović V., Šarunaite L., Fustec J., et al. (2015). Models, developments, and perspectives of mutual legume intercropping. Adv. Agron. 130, 337–419. doi: 10.1016/bs.agron.2014.10.004
Mohammed S., Hassan E., Abdo H. G., Szabo S., Mokhtar A., Alsafadi K., et al. (2021). Impacts of rainstorms on soil erosion and organic matter for different cover crop systems in the western coast agricultural region of Syria. Soil Use Manag. 37, 196–213. doi: 10.1111/sum.12683
Mortensen E. Ø., De Notaris C., Peixoto L., Olesen J. E., Rasmussen J. (2021). ) Short-term cover crop carbon inputs to soil as affected by long-term cropping system management and soil fertility. Agric. Ecosyst. Environ. 311, 1–10. doi: 10.1016/j.agee.2021.107339
O’Hara G. W., Boonkerd N., Dilworth M. J. (1988). Mineral constraints to nitrogen fixation. Plant Soil 110, 93–110. doi: 10.1007/BF02370104
R core team (2023). R: a language and environment for statistical computing (Vienna, Austria: R Foundation 656 for Statistical Computing). Available at: https://www.R-project.org/.
Rietra R. P. J. J., Heinen M., Dimkpa C. O., Bindraban P. S. (2017). Effects of nutrient antagonism and synergism on yield and fertilizer use efficiency. Commun. Soil Sci. Plant Anal. 48, 1895–1920. doi: 10.1080/00103624.2017.1407429
Sadeghzadeh N., Hajiboland R., Moradtalab N., Poschenrieder C. (2021). Growth enhancement of Brassica napus under both deficient and adequate iron supply by intercropping with Hordeum vulgare: a hydroponic study. Plant Biosyst. 155, 632–646. doi: 10.1080/11263504.2020.1769215
Scavo A., Fontanazza S., Restuccia A., Pesce G. R., Abbate C., Mauromicale G. (2022). The role of cover crops in improving soil fertility and plant nutritional status in temperate climates. A review. Agron. Sustain Dev. 42, 42–93. doi: 10.1007/s13593-022-00825-0
Schipanski M. E., Barbercheck M., Douglas M. R., Finney D. M., Haider K., Kaye J. P., et al. (2014). A framework for evaluating ecosystem services provided by cover crops in agroecosystems. Agric. Syst. 125, 12–22. doi: 10.1016/j.agsy.2013.11.004
Takeda M., Nakamoto T., Miyazawa K., Murayama T. (2009). Phosphorus transformation in a soybean-cropping system in Andosol : effects of winter cover cropping and compost application. Nutr. Cycl Agroecosyst. 85, 287–297. doi: 10.1007/s10705-009-9267-6
Tang X., Zhang C., Yu Y., Shen J., van der Werf W., Zhang F. (2021). Intercropping legumes and cereals increases phosphorus use efficiency; a meta-analysis. Plant Soil 460, 89–104. doi: 10.1007/s11104-020-04768-x
Teles A. P. B., Rodrigues M., Bejarano Herrera W. F., Soltangheisi A., Sartor L. R., Withers P. J. A., et al. (2017). Do cover crops change the lability of phosphorus in a clayey subtropical soil under different phosphate fertilizers. Soil Use Manag. 33, 34–44. doi: 10.1111/sum.12327
Teste F. P., Veneklaas E. J., Dixon K. W., Lambers H. (2014). Complementary plant nutrient-acquisition strategies promote growth of neighbour species. Funct. Ecol. 28, 819–828. doi: 10.1111/1365-2435.12270
Thorup-Kristensen K., Magid J., Jensen L. S. (2003). Catch crops and green manures as biological tools in nitrogen management in temperate zones. Adv. Agron. 79, 227–302. doi: 10.1016/S0065-2113(03)81005-2
Tiecher T., Calegari A., Caner L., Rheinheimer D dos S. (2017). Soil fertility and nutrient budget after 23-years of different soil tillage systems and winter cover crops in a subtropical Oxisol. Geoderma 308, 78–85. doi: 10.1016/j.geoderma.2017.08.028
Torstensson G., Aronsson H., Bergström L. (2006). Nutrient use efficiencies and leaching of organic and conventional cropping systems in Sweden. Agron. J. 98, 603–615. doi: 10.2134/agronj2005.0224
Tribouillois H., Cohan J.-P., Justes E. (2016). Cover crop mixtures including legume produce ecosystem services of nitrate capture and green manuring: assessment combining experimentation and modelling. Plant Soil 401, 347–364. doi: 10.1007/s11104-015-2734-8
van der Heijden G., Legout A., Pollier B., Ranger J., Dambrine E. (2014). The dynamics of calcium and magnesium inputs by throughfall in a forest ecosystem on base poor soil are very slow and conservative : Evidence from an isotopic tracing experiment (26Mg and 44Ca). Biogeochemistry 118, 413–442. doi: 10.1007/s10533-013-9941-2
Van Eerd L. L., Chahal I., Peng Y., Awrey J. C. (2023). Influence of cover crops at the four spheres: A review of ecosystem services, potential barriers, and future directions for North America. Sci. Total Environ. 858, 159990. doi: 10.1016/j.scitotenv.2022.159990
Wang G., Ngouajio M., Warncke D. D. (2008). Nutrient cycling, weed suppression, and onion yield following Brassica and Sorghum Sudangrass cover crops. Horttechnology 18, 68–74. doi: 10.21273/HORTTECH.18.1.68
Wendling M., Büchi L., Amossé C., Sinaj S., Walter A., Charles R. (2016). Influence of root and leaf traits on the uptake of nutrients in cover crops. Plant Soil 409, 419–434. doi: 10.1007/s11104-016-2974-2
Willey R. W. (1979). ) Intercropping - Its importance and research need. Part 1. Competition and yield advantages. F Crop Abstr 32, 1–10.
Wortman S. E., Francis C. A., Lindquist J. L. (2012). Cover crop mixtures for the western Corn Belt: Opportunities for increased productivity and stability. Agron. J. 104, 699–705. doi: 10.2134/agronj2011.0422
Xia H. Y., Zhao J. H., Sun J. H., Xue Y. F., Eagling T., Bao X. G., et al. (2013). Maize grain concentrations and above-ground shoot acquisition of micronutrients as affected by intercropping with turnip, faba bean, chickpea, and soybean. Sci. China Life Sci. 56, 823–834. doi: 10.1007/s11427-013-4524-y
Xue Y., Xia H., Christie P., Zhang Z., Li L., Tang C. (2016). Crop acquisition of phosphorus, iron and zinc from soil in cereal/legume intercropping systems: a critical review. Ann. Bot. 117, 363–377. doi: 10.1093/aob/mcv182
Zuo Y., Zhang F. (2008). Effect of peanut mixed cropping with gramineous species on micronutrient concentrations and iron chlorosis of peanut plants grown in a calcareous soil. Plant Soil 306, 23–36. doi: 10.1007/s11104-007-9484-1
Keywords: intercropping, cover crop, macronutrient, micronutrient, interspecific interactions, complementarity, competition, Brassicaceae-Fabaceae intercrop
Citation: Couëdel A, Alletto L and Justes É (2023) The acquisition of macro- and micronutrients is synergistic in species mixtures: example of mixed crucifer-legume cover crops. Front. Agron. 5:1223639. doi: 10.3389/fagro.2023.1223639
Received: 16 May 2023; Accepted: 09 August 2023;
Published: 29 August 2023.
Edited by:
Walter Daniel Carciochi, National University of Mar del Plata, ArgentinaReviewed by:
Corina Carranca, Instituto Nacional Investigaciao Agraria e Veterinaria (INIAV), PortugalCopyright © 2023 Couëdel, Alletto and Justes. This is an open-access article distributed under the terms of the Creative Commons Attribution License (CC BY). The use, distribution or reproduction in other forums is permitted, provided the original author(s) and the copyright owner(s) are credited and that the original publication in this journal is cited, in accordance with accepted academic practice. No use, distribution or reproduction is permitted which does not comply with these terms.
*Correspondence: Antoine Couëdel, YW50b2luZS5jb3VlZGVsQGNpcmFkLmZy
†ORCID: Antoine Couëdel, orcid.org/0000-0002-5786-0939
Lionel Alletto, orcid.org/0000-0003-0933-9476
Eric Justes, orcid.org/0000-0001-7390-7058
Disclaimer: All claims expressed in this article are solely those of the authors and do not necessarily represent those of their affiliated organizations, or those of the publisher, the editors and the reviewers. Any product that may be evaluated in this article or claim that may be made by its manufacturer is not guaranteed or endorsed by the publisher.
Research integrity at Frontiers
Learn more about the work of our research integrity team to safeguard the quality of each article we publish.