- Department of Soil Science, Federal University of Lavras, Lavras, MG, Brazil
Introduction: The by-product of the intermediate process of tanning (BPIPT) can be used in the manufacturing of environmentally-friendly organo-mineral fertilizers (OMF). However, the presence of potentially toxic elements (e.g., chromium, Cr), can hinder the use of BPIPT in agriculture. This study aimed: i) to evaluate soil chemical and biological properties following the application of fertilizers produced with a BPIPT, in contrast to other OMF produced with traditional organic matrices; and ii) to assess the impacts of such products on wheat growth and nutrition.
Methods: Samples (0-0.2 m) of two Oxisols [Red-Yellow Latosol (LVAd) and Red Latosol (LVd)] were used in this study, consisting of two experiments (with five treatments and five replicates) using different OMF, in a sequence: 1) incubation of fertilizers in soil samples for 30 days, and 2) wheat cultivation (30 days) after the fertilizer incubation. The treatments consisted of an arrangement of mineral fertilizers based on nitrogen, phosphorus, and potassium (NPK fertilizers), associated with OMF based on BPIPT (OM-IPT and OM-IPT+S) or commercial manure (OM-CM and OM-CM+S), with or without sulfur (S), and a control treatment. Elemental availability in soils and microbial attributes were determined after the incubation of the OMF in the soils. After wheat cultivation, plant biomass, nutritional composition, β-glucosidase activity, and fluorescein diacetate hydrolysis (FDA) were measured.
Results and discussion: The application of BPIPT-derived OMF in the present study increased available Cr fraction contents in both soils. However, OM-IPT caused low soil acidification, enhanced wheat growth and nutrition, and stimulated microbial activity in soils (FDA and β-glucosidase), thus evidencing the agronomic and environmental benefits of this OMF and their potential to contribute to a cleaner, more sustainable agriculture.
1 Introduction
Promoting food security and maintaining sustainable production patterns are the primary challenges of worldwide agriculture, which are targeted by the United Nations Sustainable Development Goals (https://sdgs.un.org/goals). Globally, the expansion of agriculture occurs within a scenario of increasing demand for fertilizers and depletion of natural resources, which requires alternative and economically viable sources of nutrients, actions that support the circular economy (Velenturf et al., 2019). Indeed, the European Commission has set new regulations to promote the development and use of organic and waste-based fertilizers (European Commission, 2019), which can reduce up to 30% of the use of non-renewable mineral sources. In this context, the use of industrial by-products in agriculture emerges as a strategy to reduce waste disposal in the environment, while providing an alternative source of nutrients to plants and possibly reducing costs with the application of mineral fertilizers (Lima et al., 2010; Coelho et al., 2015).
The reuse of leather-derived waste is a pressing issue within the circular economy and represents an important step toward the sustainability of the tannery industry (Chojnacka et al., 2021). Raw leather or treated leather waste may contain up to 10.5% protein per dry weight, which can be hydrolyzed to obtain collagen as a raw material to produce organic fertilizers or soil improvers (Nogueira et al., 2011; Oliveira-Longatti et al., 2017; Majee et al., 2021). The organic matrix of fertilizers containing the by-product of the intermediate tanning process (BPIPT) derived from the transformation of collagen from hides and skins is homogeneous. The BPIPT can have high N contents (up to 140 g kg-1 N per dry weight), which in combination with other nutrients, can be used to produce smart fertilizers (Majee et al., 2021; Stefan et al., 2021). Such products can complement or replace the application of mineral N fertilizers (e.g., urea), enhance nutrient use efficiency (NUE) (Nogueira et al., 2011; Ciavatta et al., 2012), increase N mineralization and microbial activity (Oliveira-Longatti et al., 2017), and lead to increased crop biomass and yield (Lima et al., 2010; Coelho et al., 2015; Majee et al., 2021).
One adverse aspect of the use of BPIPT in agriculture is the presence of potentially toxic elements in their composition (Chau et al., 2023). The tanning process applies chromium (Cr-III) salts to hides and skins and forms a Cr-collagen complex – the so-called wet blue leather. Chromium has no biological function in plant metabolism, although is considered a nutrient for animals (Kabata-Pendias, 2011). In increased contents, Cr is toxic to plants, microorganisms, and animals, and can cause damage to human and environmental health. However, studies using tannery by-products have demonstrated so far that the Cr levels in such materials do not impair plant growth or microbial activity (Oliveira-Longatti et al., 2017), and that Cr contents in plants after fertilization with tannery by-products are within safe levels (Nogueira et al., 2011; Coelho et al., 2015). Yet, a proper characterization of such tannery by-products is critical to assess their impacts on environmental contamination.
Waste-based organic fertilizers, which include BPIPT, must meet some requirements in terms of nutrient content and permissible levels of contaminants to be registered and marketed. In Europe, an organic or organo-mineral fertilizer (OMF) is allowed to have up to 2 mg kg-1 Cr (VI) per dry matter (European Commission, 2019). In Brazil, the same maximum permissible value was established for organic fertilizers and soil conditioners (Brasil, Ministério da Agricultura, Pecuária e Abastecimento – MAPA, 2006), along with a limit of 200 mg kg-1 total Cr for inorganic fertilizers containing macronutrients. However, the regulation is inconsistent regarding the limiting values for total Cr in OMF. The same trend is observed in other world regions (Ciavatta et al., 2012; Majee et al., 2021). Thus, evaluations and risk assessment studies of waste-based OMF are needed to support changes or alterations in fertilizers’ regulations. Such knowledge is essential to boost the use and commerce of waste-based fertilizers, which favors cleaner and more sustainable production.
High total Cr contents in BPIPT are concerning if such values extrapolate those recommended by health agencies, which may pose a risk to human and environmental health. In nature, Cr occurs as Cr (III) and Cr (VI). However, the tannery by-products only contain Cr (III), which is non-toxic, immobile, and occurs mainly as insoluble inorganic compounds (Ertani et al., 2017). Conversely, Cr (VI) usually occurs as oxyanions, is very mobile, and forms soluble inorganic compounds, which are highly bioavailable and toxic (Alloway, 2012). Thus, the oxidation of Cr (III) to Cr (VI) can be a concerning aspect related to the use of BPIPT-based fertilizers, due to the mutagenic and carcinogenic potential of Cr oxidized form (Ciavatta et al., 2012). In soils, Cr dynamics in soils rely on its oxidation state and mobility (Kožuh et al., 2000; Ertani et al., 2017; Choppala et al., 2018; Xu et al., 2020), which in turn is affected by soil composition and soil conditions (i.e., the content of organic matter, Fe (II) and Mn (IV) oxides, the soil texture, moisture, pH, and temperature) (Kožuh et al., 2000). Although the reduction of Cr (VI) predominates over the possible oxidation of Cr (III) in soils, the application of OMF in soils may require constant monitoring of soil attributes.
The BPIPT has multiple environmental and economic benefits, yet the presence of Cr is a potential drawback that can impair its use in agriculture. Additionally, information on the content of nutrients and contaminants in such products is needed to support changes in fertilizers’ regulations and, or, to boost innovation with waste-based OMF. Thus, this study aimed: i) to evaluate soil chemical and biological properties following the application of fertilizers produced with a BPIPT, in contrast to other OMF produced with traditional organic matrices; and ii) to assess the impacts of such products on wheat growth and nutrition. We hypothesized that i) the organic material (collagen) in the BPIPT increases soil microbial activity, and nutrient availability to plants; and ii) Cr contents in the OMF-containing BPTIP are not toxic to wheat plants and soil microbes.
2 Materials and methods
2.1 Characterization of pristine soils
Soil samples were collected in the 0-0.2 m layer of a native area (native vegetation of the Cerrado) located in the region of Campo das Vertentes, State of Minas Gerais, Brazil. According to the Brazilian Soil Classification System (Santos et al., 2018), soils were classified as typical dystrophic Red-Yellow Latosol (LVAd), and typical dystrophic Red Latosol (LVd), corresponding to Oxisol in the USDA Soil Taxonomy (Soil Survey Staff, 2014). The physical and chemical properties were determined in the air-dried fine earth (ADFE; < 2mm) (Table 1).
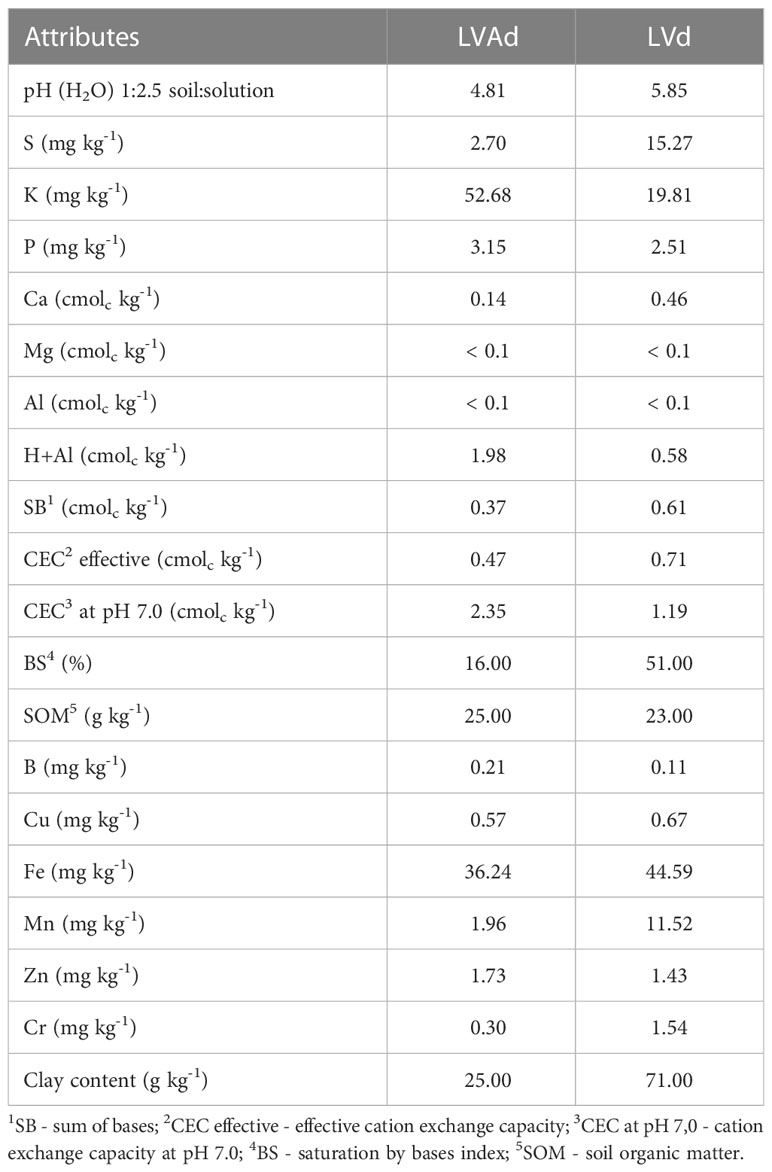
Table 1 Chemical and physical characterization of the typical dystrophic Red-Yellow Latosol (LVAd) and the typical dystrophic Red Latosol (LVd) used in the experiments.
Soil samples were dried, crushed, and passed through a 4-mm sieve. Then, the samples were homogenized and stored in plastic bags. A mixture of calcium (Ca) and magnesium (Mg) carbonates (p.a.) in a 3:1 molar ratio (Ca : Mg) was used to increase soil pH and increase base saturation to 60%, according to previous soil analyses (Alvarez and Ribeiro, 1999). The soil was incubated at room temperature for 60 days with soil moisture maintained at field capacity. Soil samples were homogenized once a week during the incubation period. At the end of the 60 days, the average pH values for LVAd and LVd were 6.15 ± 0.08 and 6.23 ± 0.06, respectively.
Soil pH in H2O was measured using a 1:2.5 (v/v) soil:solution ratio (TEC-11 model from Tecnal). The content of soil organic matter (SOM) was determined by oxidation with a potassium dichromate solution in the presence of sulfuric acid (Carter and Gregorich, 2006).Calcium (Ca2+), magnesium (Mg2+), and aluminum (Al3+) were obtained with the 1 mol L-1 KCl extractant (1:10 v/v soil: solution ratio), whereas available phosphorus (P), potassium (K), copper (Cu), iron (Fe), manganese (Mn), zinc (Zn), and chromium (Cr) were obtained by Mehlich (1953) (0.05 mol L-1 HCl + 0.0125 mol L-1 H2SO4, in ratio 1:10 v/v soil: solution); sulfur (S) was extracted as sulfate by monocalcium phosphate with acetic acid by using 1:2.5 soil: solution ratio and activated charcoal (Hoeft et al., 1973). Exchangeable Al was determined by titration with NaOH (0.025 mol L-1); P and S were determined colorimetrically using a UV/VIS spectrophotometer (B582 model from Micronal®); K by flame emission photometry (DM-62 model from Digimed); and Ca, Mg, Zn, Fe, Mn, and Cu were determined by atomic absorption spectrometry (AAnalyst 400 model from PerkinElmer). Cr was determined by inductively coupled plasma optical emission spectrometry (ICP-OES) (Blue model from Spectro). Attributes related to soil fertility (SB - sum of bases; CEC effective - effective cation exchange capacity; CEC at pH 7.0 - cation exchange capacity at pH 7,0; BS - base saturation index) were calculated according to Teixeira et al. (2017).
2.2 Experimental design
The experiments were carried out in two sequential steps: 1) incubation of fertilizers in soil samples for 30 days; and 2) growing wheat (Triticum aestivum L.) after incubating the fertilizers for 30 days. Two experiments were carried out, one in each soil (LVAd and LVd), with the same completely randomized experimental design, with five treatments and five replications. The treatments consisted of the composition of two types of OMF, which contained mineral fertilizers based on nitrogen (N), phosphorus, and potassium (NPK fertilizer), with and without the addition of S. The organic matrix of the OMF was BPIPT and organic compost commercial. The treatments were identified as OM-IPT (OMF based on BPIPT), OM-IPT+S (OMF based on BPIPT plus S), OM-CM (OMF based on organic compound), OM-CM+S (OMF based on organic compound plus S). In Table 2 describes the composition and nutrient content of each OMF.
All fertilizers were oven-dried (40°C) and crushed in a ball mill before their characterization and application to the soil. Then, they were passed in a 1-mm sieve to standardize the fertilizers’ granulometry and quartered in a stainless-steel sample splitter. The OMF were characterized according to the Manual of Official Analytical Methods for Fertilizers and Liming Materials (Brasil, Ministério da Agricultura, Pecuária e Abastecimento – MAPA, 2017) (Table 2).
Chromium (Cr) contents were evaluated in the OMF according to the USEPA 3051A method (USEPA, 2007a). Five grams of each OMF were ground with an agate mortar and sieved through a 0.15 mm mesh. Then, 0.1 g aliquots plus 5 mL of concentrated HNO3 were placed in Teflon® vessels. The extract stood overnight at room temperature, and the digestion was performed the following day. The containers were sealed and heated in a Mars-5 microwave digestion oven (CEM Corp, Matthews, NC) to 175°C under controlled pressure conditions (0.76 MPa for 15 minutes). The sealed vessels were then removed from the oven and cooled to room temperature under a fume hood. Finally, the vessels were opened, and the resulting solution was filtered through Whatman #40 filter paper. The volume of the filtered extract was adjusted to 50 mL with ultrapure water. Each sample was digested in triplicate. Chromium contents were determined by ICP-OES (Blue model from Spectro) (Table 2). Reference samples of a Natural Moroccan Phosphate Rock BCR® 32 and a Trace Elements in Multi-Nutrient Fertilizer NIST 695, as well as a blank sample, were analyzed in triplicates. The analysis of the reference material for Cr had the following recoveries (%): 85 and 43 for BCR® 32 and NIST 695, respectively.
The application of macronutrients (N, P, K, and S) was based on the fertilizer recommendation proposed by Malavolta (1980), with modifications to meet the objectives of this study, i.e., no micronutrients were added, which were already in the OMF source, and the doses of S changed with the treatments. Thus, the amount of fertilizers applied to the soil supplied 300 mg kg-1 of N, 200 mg kg-1 of P, 200 mg kg-1 of K, and 50 mg kg-1 of S. The sources of inorganic fertilizers (commercial sources) used to supply N, P and K were, respectively, urea, monoammonium phosphate (MAP) and potassium chloride (KCl). Micronutrients were not applied. The fertilizers were applied to the dry soil samples, which were stored in plastic bags, and stirred until complete homogenization. Then, 1 kg of the soil samples were placed in pots, weighed, and kept on benches in a greenhouse under controlled temperature conditions.
2.3 Incubation of OMF in the soils
The first stage of the experiment was the incubation of the OMF in the soils for a period of 30 days. The purpose of this stage was to evaluate the interaction between the OMF and the soils, evaluating the levels of nutrients available after incubation and the effect on the soil microbiota. Soil samples were mixed and incubated with the dosage of each OMF. Daily irrigations maintained the soil moisture at field capacity. Eventual agitations ensured the reaction of the fertilizers with soil samples. After the incubation period, 200 g of soil were collected to determine the availability of Cr, Cu, lead (Pb), Zn, P, and K by the Mehlich-1 method (Mehlich, 1953). In addition, the following microbiological attributes were assessed: microbial biomass carbon (MBC), basal respiration (SBR), activity of β-glucosidase, arylsulfatase enzymes, and total soil enzymatic activity performed by hydrolysis of fluorescein diacetate (FDA).
2.3.1 Incubation of OMF: available elements in the soils
The availability of Cr, Cu, Pb, Zn, P, and K after the incubation of OMF in soils was determined by the Mehlich-1 method (Mehlich, 1953). Briefly, 10 g of soil were placed in Erlenmeyer flasks (125 mL capacity) with 100 mL of a Mehlich-1 solution (0.05 mol L-1 HCl + 0.0125 mol L-1 H2SO4). Then, the flasks were shaken for 5 min on a horizontal shaker at 220 RPM. The suspension was decanted for 16 hours, and the supernatant was collected and analyzed by ICP-OES (Blue model from Spectro).
2.3.2 Incubation of OMF: microbiological attributes in the soils
Microbial biomass carbon (MBC, µg C g-1) was determined from 20 g of soil irradiated in a microwave oven [120 V (60 Hz), frequency of 2,450 MHz, and energy concentration of 1.35 KW], for four minutes, to kill the microorganisms and release their cellular components (Islam and Weil, 1998). Then, carbon was extracted with potassium sulfate (0.5 mol L-1) followed by oxidation with potassium dichromate (0.066 mol L-1), in the presence of an acid solution (H2SO4 and H3PO4) on a hot plate for five minutes after reaching the boiling temperature. After cooling, the solution was titrated with ferrous ammonium sulfate (0.033 mol L-1) and diphenylamine (1%) as an indicator (Vance et al., 1987).
The metabolic activity of soil microbiota at the community level was measured by basal respiration (SBR, µg CO2 g-1 72 h-1) (Alef, 1995). A 20 g subsample of soil was incubated in a hermetically sealed pot for three days at 28°C. The CO2 released from the sample was captured by a NaOH solution (0.5 mol L-1) and then titrated with HCl (0.5 mol L-1), using phenolphthalein (1%) as an indicator. The basal respiration was divided by the microbial biomass to obtain the biomass-specific respiration or metabolic quotient (qCO2, µg C-CO2 µg-1 MBC h-1). This variable is a proxy for microbial C use and demand for cellular energy (Anderson and Domsch, 1993).
The activities of the β-glucosidase (µg ρ-nitrophenol g-1 h-1) (EC 3.2.1.21) (Eivazi and Tabatabai, 1988) and arylsulfatase enzymes (µg ρ-nitrophenol g-1 h-1) (EC 3.1.6.1) (Tabatabai and Bremner, 1970) were measured by the difference in optical density (O.D.) using a spectrophotometer (B582 model from Micronal®) at 410 nm. Briefly, samples of 1 g of soil containing 1 mL of substrate (ρ-nitrophenyl-β-D-glucoside for β-glucosidase and ρ-nitrophenyl-sulfate for arylsulfatase) were incubated for one hour at 37°C, in the presence of toluene and a buffer solution at specific pH for each enzyme (pH 6.0 for β-glucosidase, and 5.8 for arylsulfatase). After the incubation period, the reaction was discontinued by adding CaCl2 (0.5 mol L-1) and NaOH (0.5 mol L-1). Then, the supernatant was filtered (Whatman #40 filter paper) and analyzed in a spectrophotometer.
The total enzymatic activity of the soil was estimated through the hydrolysis of fluorescein diacetate (FDA, mg fluorescein g-1 24 h-1) (Dick, 2011). Subsamples of 2.5 g of soil were incubated at 37°C with a fluorescein solution (C20H12O5 10 mg L-1) in a sodium phosphate buffer (60 mmol L-1 at pH 7.0) for 24 hours and stirred at 50 RPM. Then, the reaction was discontinued using acetone (50%), and the suspension was centrifuged for 5 minutes at 3000 RPM. The supernatant was filtered, and the O.D. was measured in a spectrophotometer (B582 model from Micronal®) at 490 nm.
2.4 Incubation of OMF and wheat cultivation
The second stage of the experiment was to evaluate plant growth in the soil after OMF incubation. Ten wheat (Triticum aestivum L. cultivar TBIO Aton) seeds were sown in pots with 800 g of soil after the OMF incubation. Daily irrigations were carried out to maintain soil moisture at field capacity. After seven days, the seedlings were thinned to have only seven plants per pot. The experiment was conducted for 30 days under greenhouse conditions, with controlled temperature and air humidity. The plant material was oven-dried (60°C) until constant weight (after ± 72 h), and the dry mass of roots and shoots was weighed and recorded.
2.4.1 Incubation of OMF and wheat cultivation: Cr contents and plant nutrition
The shoot dry mass (SDM) was finely ground in an electric crusher. Then, the nutrients and other elements in the SDM were determined by the USEPA 3051A acid digestion method (USEPA, 2007a).
Briefly, 0.5 g of finely ground SDM were transferred to Teflon® digestion tubes and treated with 5 mL of concentrated HNO3. The extract stood overnight at room temperature, and the digestion was performed the following day. The containers were sealed and heated in a Mars-5 microwave digestion oven (CEM Corp, Matthews, NC) to 175°C under controlled pressure conditions (0.76 MPa for 15 minutes). After, the digestion tubes were removed from the oven and cooled to room temperature. The tubes were then uncapped, and the volume was completed to 5 mL with double-distilled water.
Each batch of analyses contained a White Clover BCR® 402 reference sample and a blank sample for quality assurance/quality control protocols. This procedure ensured quality control and allowed the calculation of the method detection limit (Penha et al., 2017). White Clover® is certified for Mo and has reported values for Cr, Ni, Zn, and Fe.
The determinations were performed in ICP-OES (Blue model from Spectro). The analysis of the reference material had the following recoveries (%): 97, 72, 91, 97, and 105 for molybdenum (Mo), Cr, nickel (Ni), Zn, and Fe, respectively. Such values demonstrate the quality of the analytical procedures used to determine the content of these elements in the plant material.
2.4.2 Incubation of OMF and wheat cultivation: FDA and soil β-glycosidase activities in the soils
The activity of the enzyme β-glucosidase (EC 3.2.1.21) (Eivazi and Tabatabai, 1988) and the estimation of the total enzymatic activity of the soil through the hydrolysis of fluorescein diacetate (FDA) (Dick, 2011) were determined, based on the methods listed in section 2.3.2.
2.4.3 Incubation of OMF and wheat cultivation: soil pH
The pH in H2O and CaCl2 was measured using a 1:2.5 (v/v) soil:solution ratio (TEC-11 pH meter from Tecnal) in soil samples after incubation of OMF and wheat cultivation for 30 days.
2.4.4 Incubation of OMF and wheat cultivation: total elemental composition of soils
The total elemental composition of the soil samples at the end of the two experiments (incubation and agronomic efficiency) was obtained by portable X-ray fluorescence spectrometry (pXRF) following the recommendations described by Weindorf and Chakraborty (2020) and the USEPA 6200 method (USEPA, 2007b). Approximately 10 g of soil were used, ensuring at least 10 mm of thickness.
For quality control of the pXRF analysis, the internal calibration was performed using the Bruker® S1 Titan 800 instrument with the Geoexploration calibration curve. In addition, a blank sample (pure SiO2) and NIST 2711a certified reference material (Montana Soil II) were analyzed. The recovery percentage for the identified elements was (NIST 2711a): Cr (100.4), Ni (108.3), Cu (91.7), Zn (91.1), and arsenic (As) (111.4). The limits of detection (LOD) reported by the manufacturer were (mg kg-1): Cr (45), Ni (4), and 3 for Cu, Zn, and As.
2.5 Statistical analysis
Statistical analysis was performed in the R programming language (R Development Core Team, 2020), version 4.0.3. Data normality and homoscedasticity were evaluated. Analysis of variance (p ≤ 0.05) was used to verify the significance of the treatments. Then, the treatments were compared using the Tukey HSD test with the emmeans v1.4 package (Length, 2020). Principal component analysis (PCA) was performed using the Vegan package v2.5-7 (Oksanen et al., 2016) to demonstrate the importance of variables in explaining nutrient contents and biomass increase of wheat plants.
3 Results
3.1 Incubation of OMF: available elements in the soils
The available contents of Cr, P, and K in soils after OMF incubation are shown in Figure 1 and Cu, Pb and Zn in Figure S1. The application of OM-IPT in the LVAd and OM-IPT+S in the LVd caused the highest contents of Cr. However, the available contents of Cr in these soils were below 3 mg kg-1 (Figure 1A). Contents of P and K increased in both soils following the application of OMF (Figure 1). In the LVAd, K contents did not differ between the OMF tested. On the other hand, P contents were reduced in the treatments OM-CM+S, OM-IPT, and OM-IPT+S in the LVAd. In the LVd, OM-CM and OM-IPT+S increased the P and K contents.
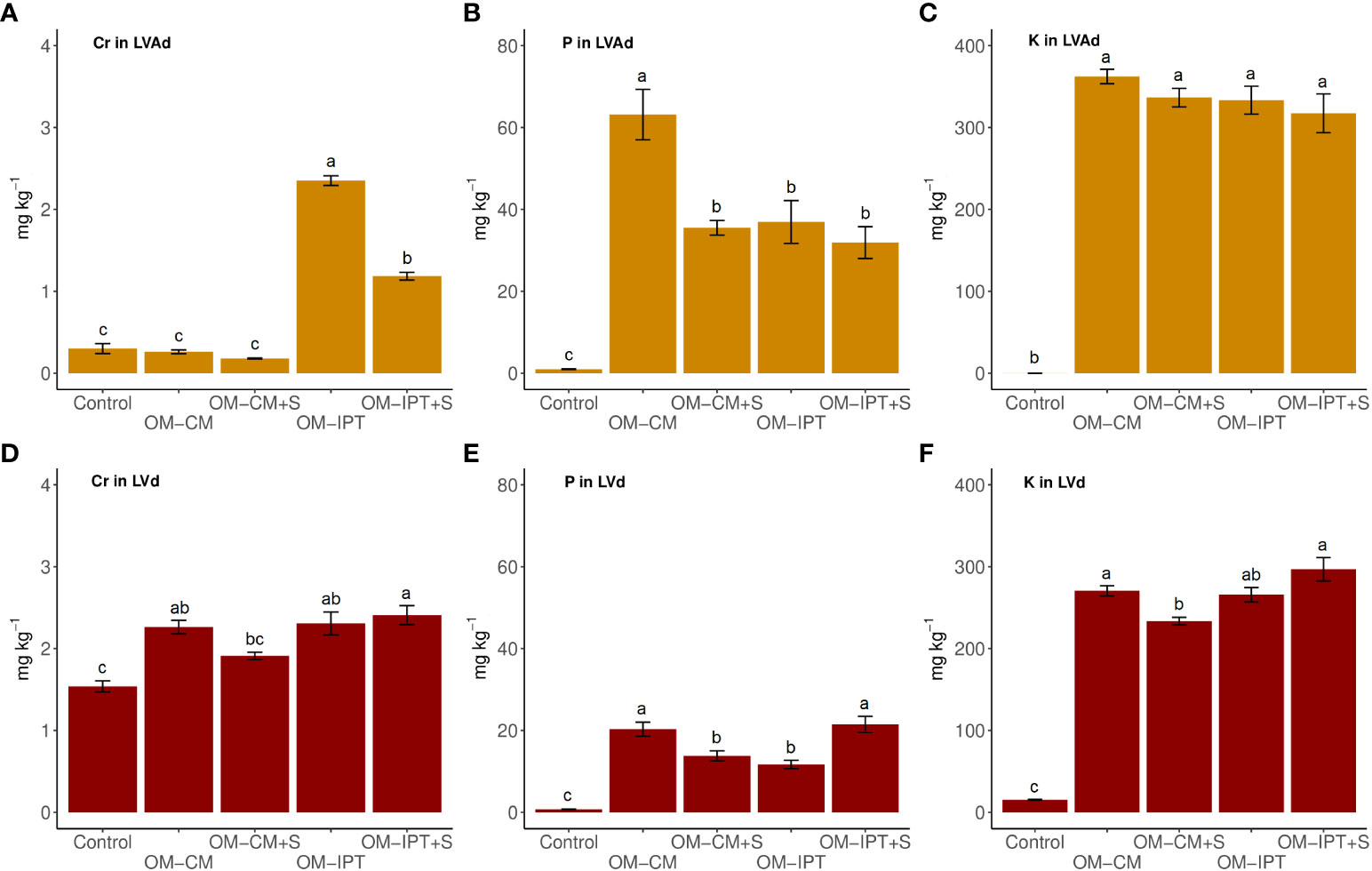
Figure 1 Cr, P and K contents extracted by Mehlich-1 in a typical dystrophic Red-Yellow Latosol (LVAd - A, B and C) and a typical dystrophic Red Latosol (LVd - D, E and F) after incubation of different organo-mineral fertilizers (mean ± SE, n = 5). The averages followed by the same letter do not differ statistically by the ANOVA and Tukey test results (p ≤ 0.05). The description of the treatments are: Control (without application), OM-CM (organo-mineral fertilizer formulated with a commercial manure, containing NPK), OM-CM+S (organo-mineral fertilizer formulated with a commercial manure, containing NPK+S), OM-IPT (organo-mineral fertilizer formulated with a by-product of the intermediate processes of tanning (BPIPT), containing NPK), OM-IPT+S (organo-mineral fertilizer formulated with a BPIPT containing NPK+S).
3.2 Incubation of OMF: microbiological attributes in the soils
The MBC increased significantly after the application of OM-CM, OM-CM+S, and OM-IPT+S in the LVAd (Figures 2A, D). The SBR had a varying behavior in the soils, and the effect of OMF on the metabolic capacity of the microbiota was unclear (Figures 2B, E). The qCO2 was higher in the control treatment (Figures 2C, F), indicating that the application of OMF enhanced the metabolic efficiency of the microbial community. In the LVAd, the FDA increased significantly with the application of all OMF compared with the control (Figure 3A). As for the LVd, only the OM-IPT and OM-IPT+S treatments increased the FDA activity (Figure 3D). The β-glucosidase activity increased significantly with the OM-IPT+S treatment in the LVAd (Figure 3B), and after the application of OM-IPT in the LVd (Figure 3E). The arylsulfatase activity significantly decreased after the application of OMF in the LVd relatively to the control (Figures 3C, F).
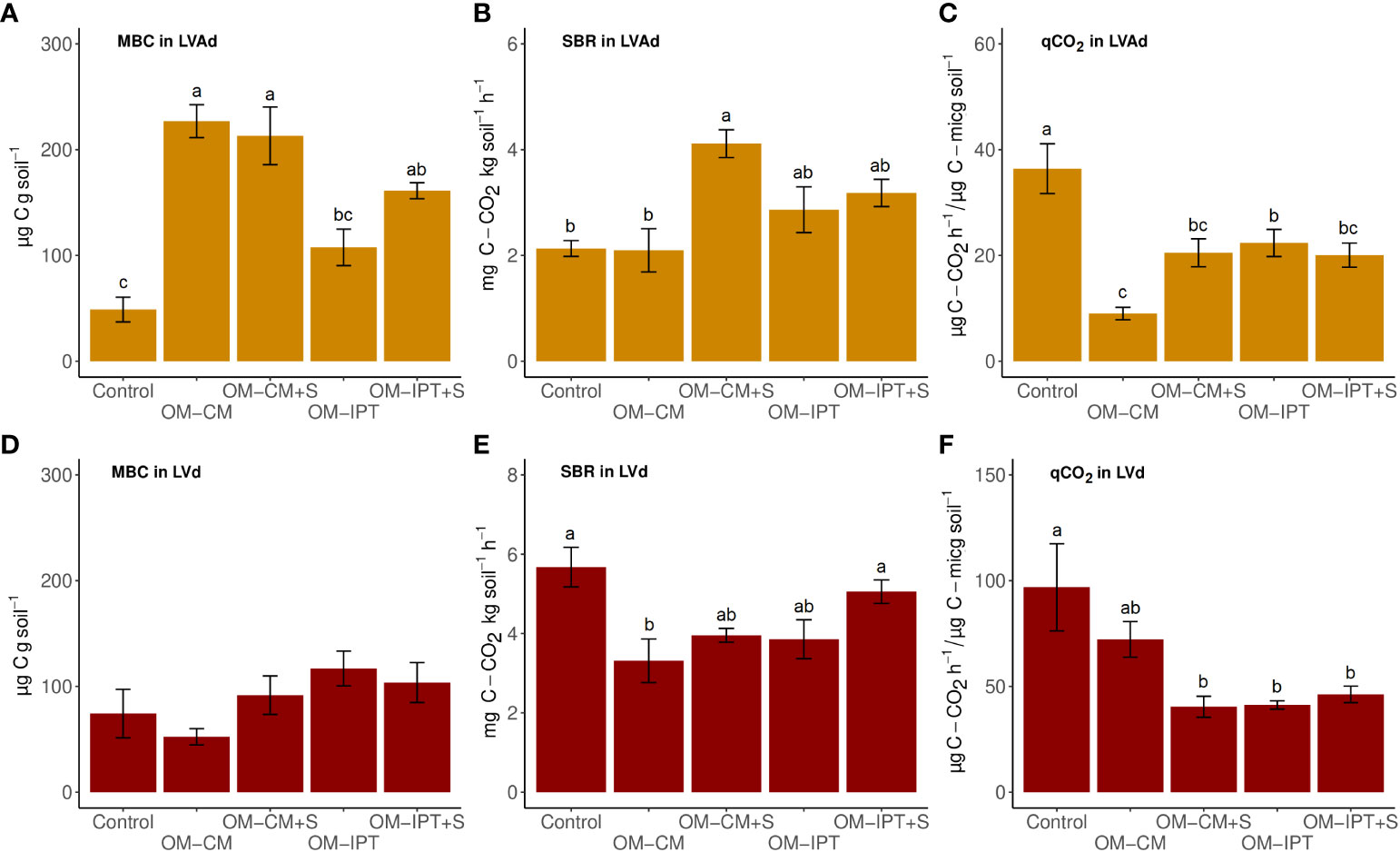
Figure 2 Microbial biomass carbon (MBC), soil basal respiration (SBR) and metabolic quotient (qCO2) in a typical dystrophic Red-Yellow Latosol (LVAd - A, B and C) and a typical dystrophic Red Latosol (LVd - D, E and F) after incubation of different organo-mineral fertilizers (mean ± SE, n = 5). The averages followed by the same letter do not differ statistically by the ANOVA and Tukey test results (p ≤ 0.05). The description of the treatments are: Control (without application), OM-CM (organo-mineral fertilizer formulated with a commercial manure, containing NPK), OM-CM+S (organo-mineral fertilizer formulated with a commercial manure, containing NPK+S), OM-IPT (organo-mineral fertilizer formulated with a by-product of the intermediate processes of tanning (BPIPT), containing NPK), OM-IPT+S (organo-mineral fertilizer formulated with a BPIPT containing NPK+S).
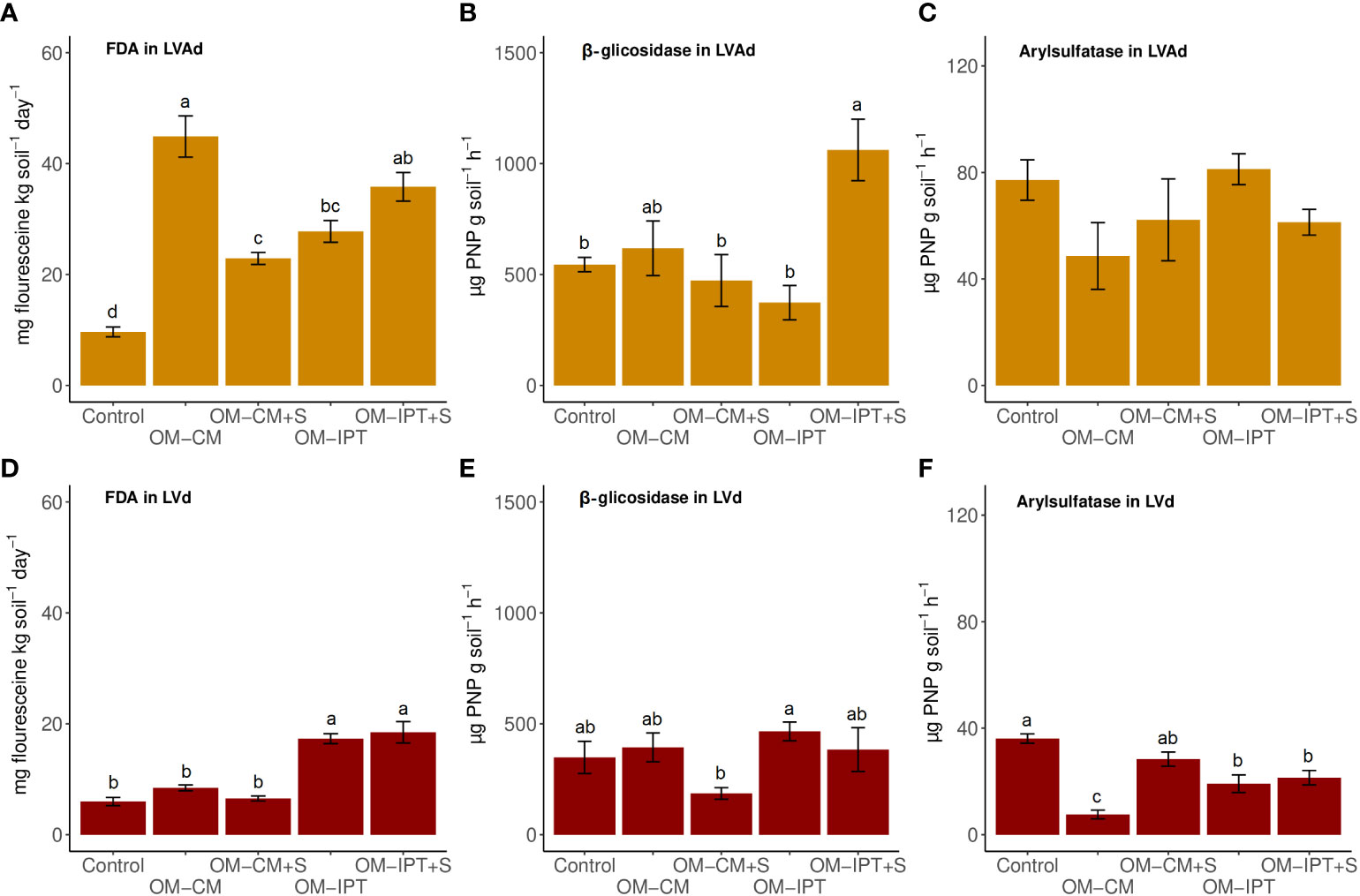
Figure 3 General soil enzymatic activity (fluorescein diacetate hydrolysis - FDA), arylsulfatase and β-glucosidase in a typical dystrophic Red-Yellow Latosol (LVAd - A, B and C) and a typical dystrophic Red Latosol (LVd – D, E an F) after incubation of different organo-mineral fertilizers (mean ± SE, n = 5). The averages followed by the same letter do not differ statistically by the ANOVA and Tukey test results (p ≤ 0.05). The description of the treatments are: Control (without application), OM-CM (organo-mineral fertilizer formulated with a commercial manure, containing NPK), OM-CM+S (organo-mineral fertilizer formulated with a commercial manure, containing NPK+S), OM-IPT (organo-mineral fertilizer formulated with a by-product of the intermediate processes of tanning (BPIPT), containing NPK), OM-IPT+S (organo-mineral fertilizer formulated with a BPIPT containing NPK+S).
3.3 Incubation of OMF and wheat cultivation: total Cr and available Cr fraction in the soils
The elemental composition of the soil determined via Mehlich-1 (plant-available fraction) via pXRF is shown in Table S1. The contents of total Cr (pXRF) and the relationship between total Cr and the available Cr fraction are found in Figure 4. The LVd has the highest contents of Fe2O3 and the lowest contents of SiO2 (Table S1), and the highest levels of total Cr (Figure 4D), as well as other elements (Ni, Cu, Zn, and As) (Table S1).
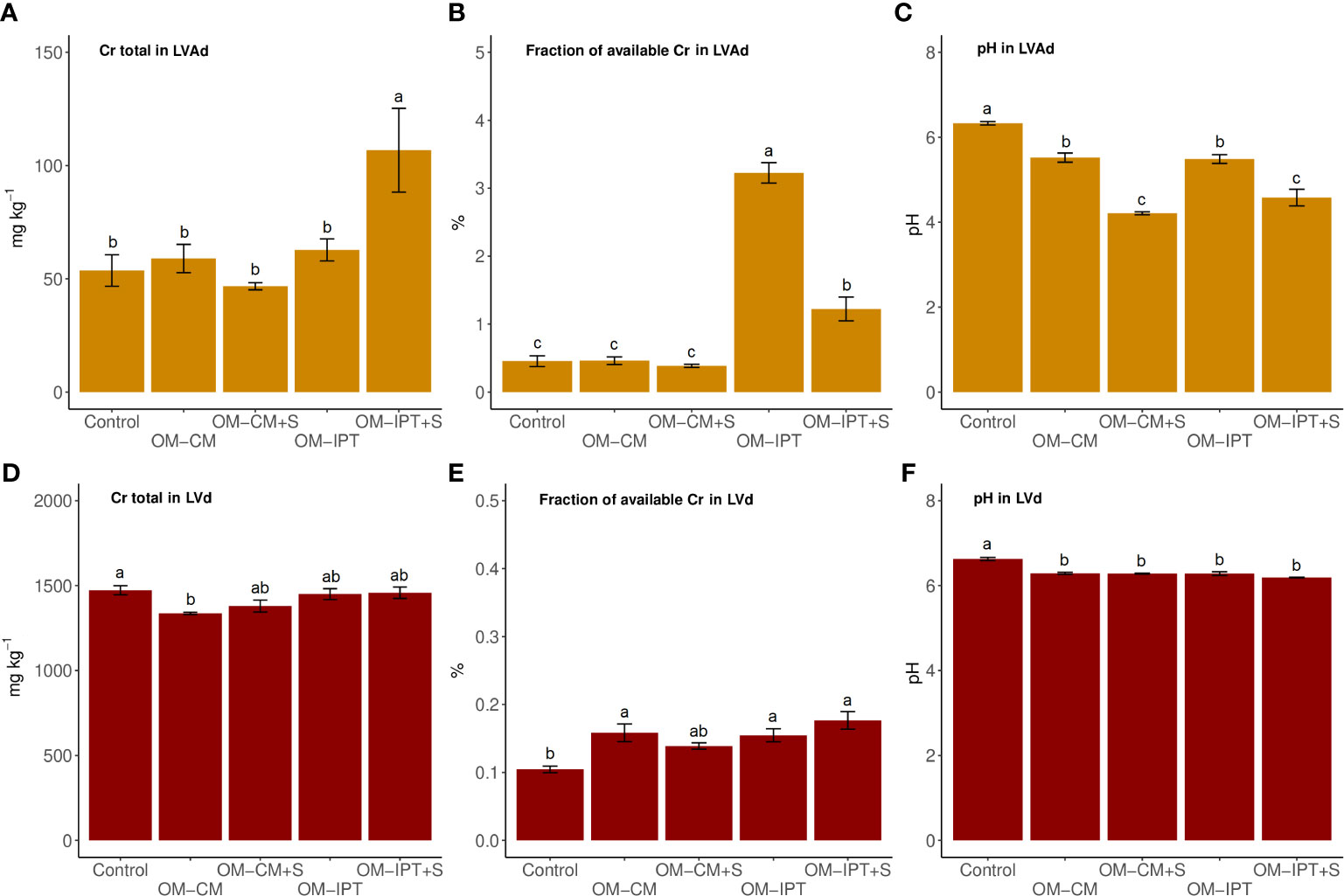
Figure 4 Total Cr contents (portable X-ray fluorescence - pXRF), available Cr fraction (Cr by Mehlich-1/Cr by pXRF) and soil pH values determined in CaCl2 in a typical dystrophic Red-Yellow Latosol (LVAd - A, B and C) and a typical dystrophic Red Latosol (LVd - D, E and F) after wheat cultivation with different organo-mineral fertilizers (mean ± SE, n = 5). The averages followed by the same letter do not differ statistically by the ANOVA and Tukey test results (p ≤ 0.05). The description of the treatments are: Control (without application), OM-CM (organo-mineral fertilizer formulated with a commercial manure, containing NPK), OM-CM+S (organo-mineral fertilizer formulated with a commercial manure, containing NPK+S), OM-IPT (organo-mineral fertilizer formulated with a by-product of the intermediate processes of tanning (BPIPT), containing NPK), OM-IPT+S (organo-mineral fertilizer formulated with a BPIPT containing NPK+S).
In the LVd, the average Cr content in the control treatment is 1472 mg kg-1. However, the available Cr fraction corresponds to less than 1% of the total Cr (Figure 4E), regardless of the treatment. As for the LVAd, the highest Cr available fraction occurred after the application of OM-IPT, but it did not exceed 5% of the total Cr (Figure 4B). In the OM-IPT+S treatment, the available Cr fraction is approximately 2% lower than in the OM-IPT treatment. This available fraction (5%) is relative to a total Cr content of 63 mg kg-1 in the OM-IPT treatment and to a total Cr content of 54 mg kg-1 in the control treatment.
3.4 Incubation of OMF and wheat cultivation: Cr contents and plant nutrition
The Cr contents in wheat SDM were higher in the control treatments of LVAd (6.6 mg kg-1) and LVd (5.4 mg kg-1) (Figures 5A, D). The lowest Cr content in wheat SDM was found in the OM-IPT treatment (2.4 mg kg-1) in the LVd (Table 3).
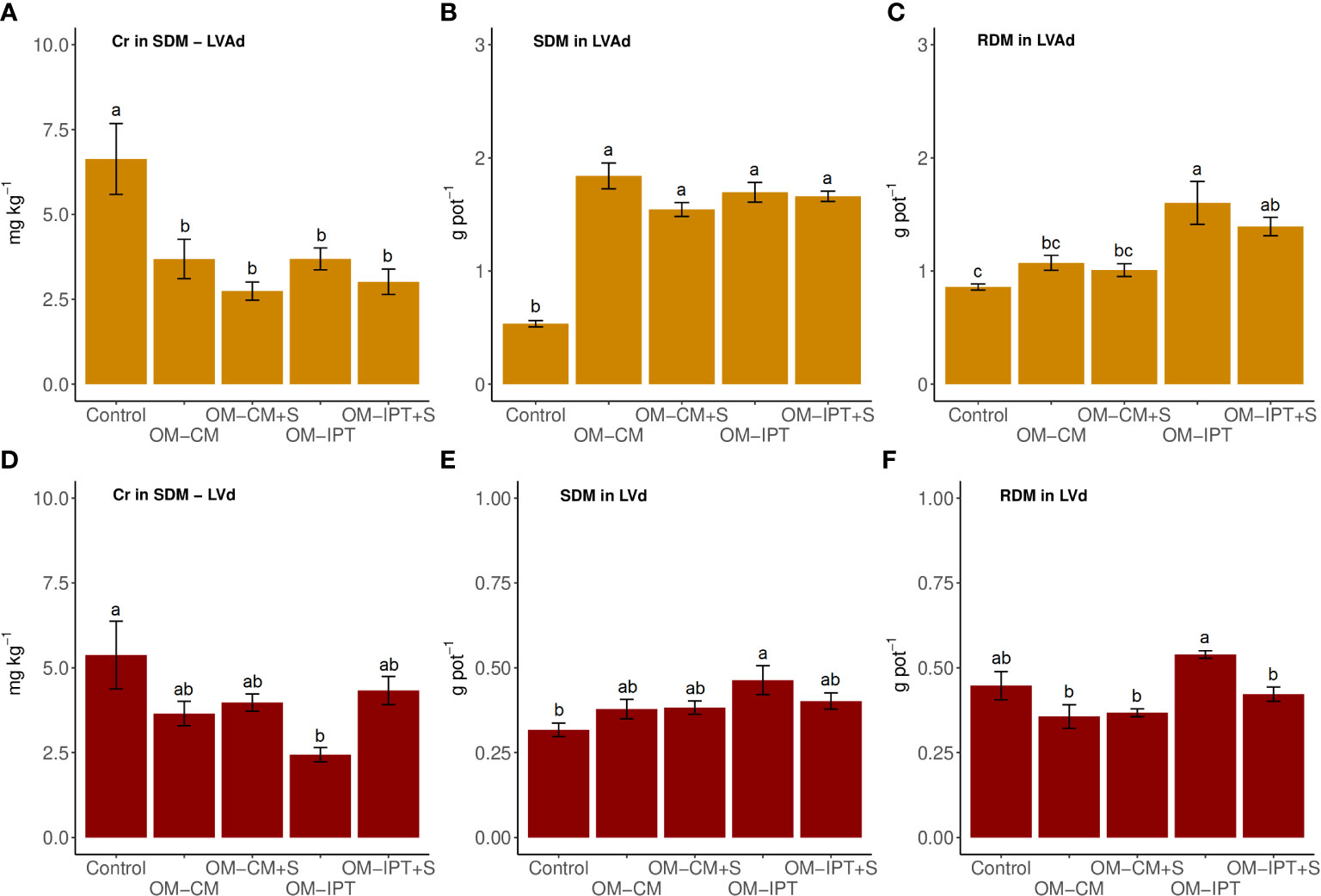
Figure 5 Cr contents in shoot dry mass (SDM), SDM and root dry mass (RDM) of wheat in a typical dystrophic Red-Yellow Latosol (LVAd - A, B and C) and a typical dystrophic Red Latosol (LVd - D, E and F) after wheat cultivation with different organo-mineral fertilizers (mean ± SE, n = 5). The averages followed by the same letter do not differ statistically by the ANOVA and Tukey test results (p ≤ 0.05). The description of the treatments are: Control (without application), OM-CM (organo-mineral fertilizer formulated with a commercial manure, containing NPK), OM-CM+S (organo-mineral fertilizer formulated with a commercial manure, containing NPK+S), OM-IPT (organo-mineral fertilizer formulated with a by-product of the intermediate processes of tanning (BPIPT), containing NPK), OM-IPT+S (organo-mineral fertilizer formulated with a BPIPT containing NPK+S).
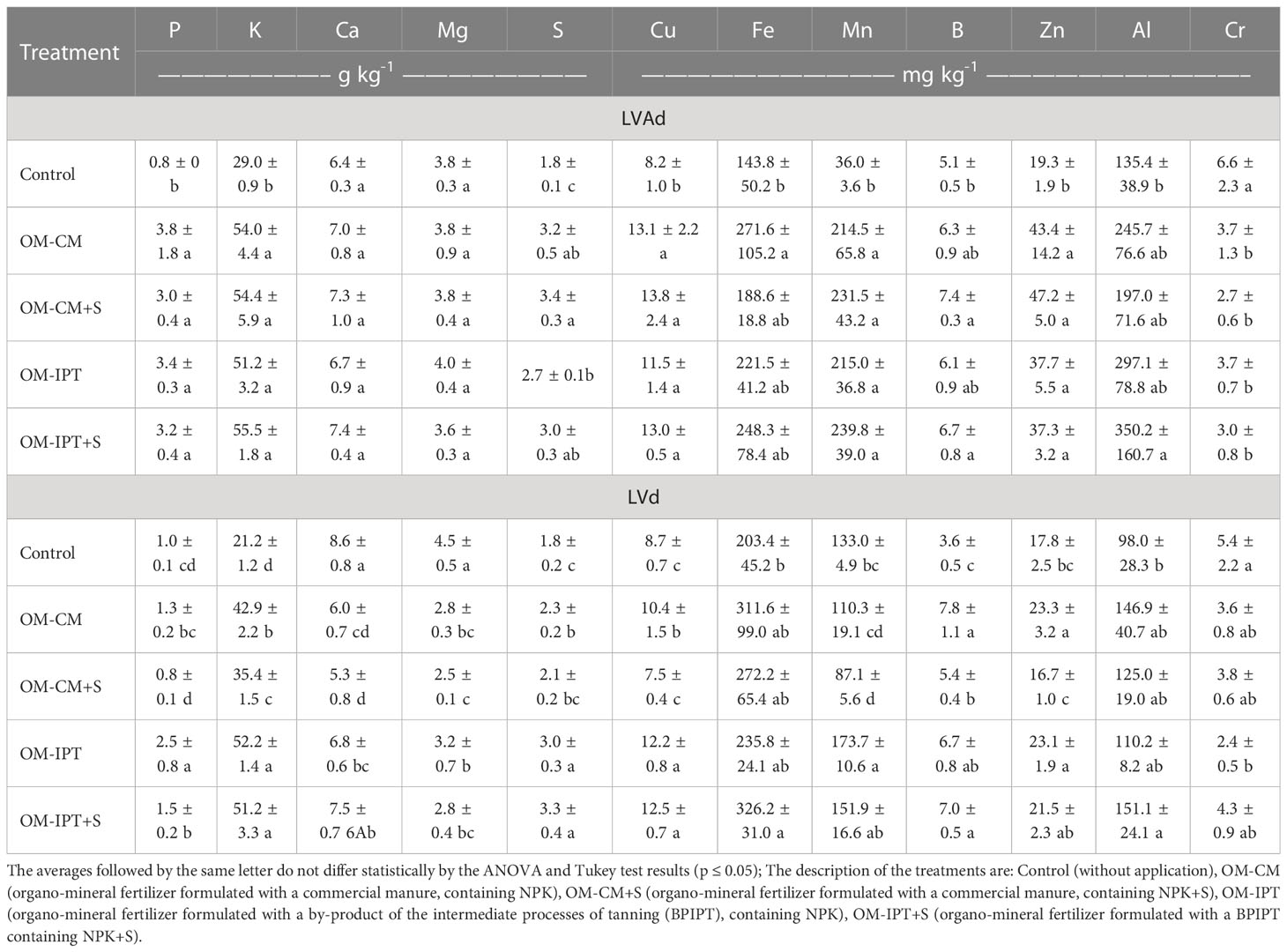
Table 3 Shoot nutrient content of wheat grown in a typical dystrophic Red-Yellow Latosol (LVAd) and a typical dystrophic Red Latosol (LVd) with different organo-mineral fertilizers (mean ± SE, n = 5).
The application of OMF increased Al contents in wheat SDM. The highest Al levels were found in the OM-IPT+S treatment in the LVAd (350 mg kg-1) and LVd (151 mg kg-1) (Table 3).
The application of OMF increased wheat SDM (Figures 5B, E). In the LVAd, higher SDM values were observed after the application of OMF, with an average of 1.7 g pot-1. However, within OMF treatments, no differences in SDM were observed (Figure 5B). In the LVd, the SDM values in the OMF treatments did not exceed 0.5 g pot-1 (Figure 5E). In this soil, OM-IPT increased SDM relatively to the control. The OM-IPT treatment caused the highest RDM in the LVAd, and a high RDM in the LVd, which did not differ from the control.
In wheat grown in the LVAd, P and K contents in SDM increased significantly in all treatments compared with the control. However, no difference was observed among OMF treatments. The Ca and Mg contents did not differ from the control. The S content in SDM was the highest for OM-CM+S (3.4 g kg-1) and the lowest for the control (1.8 g kg-1; Table 3). Overall, the application of OMF in the LVAd increased the contents of micronutrients (Table 3). Specifically, Mn had an average content of 36 mg kg-1 in the control, and an average content of 225 mg kg-1 for the OMF treatments in the LVAd.
For the LVd, the OM-IPT treatment increased the P content in SDM (2.5 g kg-1). The OM-IPT and OM-IPT+S treatments had the highest K and S contents. Lastly, Ca and Mg contents decreased after the application of OMF in the LVd (Table 3), except for Ca content in the OM-IPT+S treatment, which did not differ from the control. Overall, the contents of micronutrients in SDM increased after the OMF addition. However, probably due to the buffering effect of the higher clay content of this soil, the increases in Mn and Zn contents were less pronounced when compared with LVAd.
3.5 Incubation of OMF and wheat cultivation: FDA and soil β-glycosidase activities in the soils
The FDA activity increased after wheat cultivation in the LVAd, with the highest value after the application of OM-IPT (Figure 6A). On the other hand, FDA activity in the LVd was unaffected by OMF application (Figure 6C). β-glucosidase activity varied in both soils (Figures 6B, D). In the LVAd, all OMF treatments had higher β-glucosidase activity than the control (Figure 6B). As for the LVd, the application of OM-IPT (Figure 6D) increased the β-glucosidase activity compared with the control.
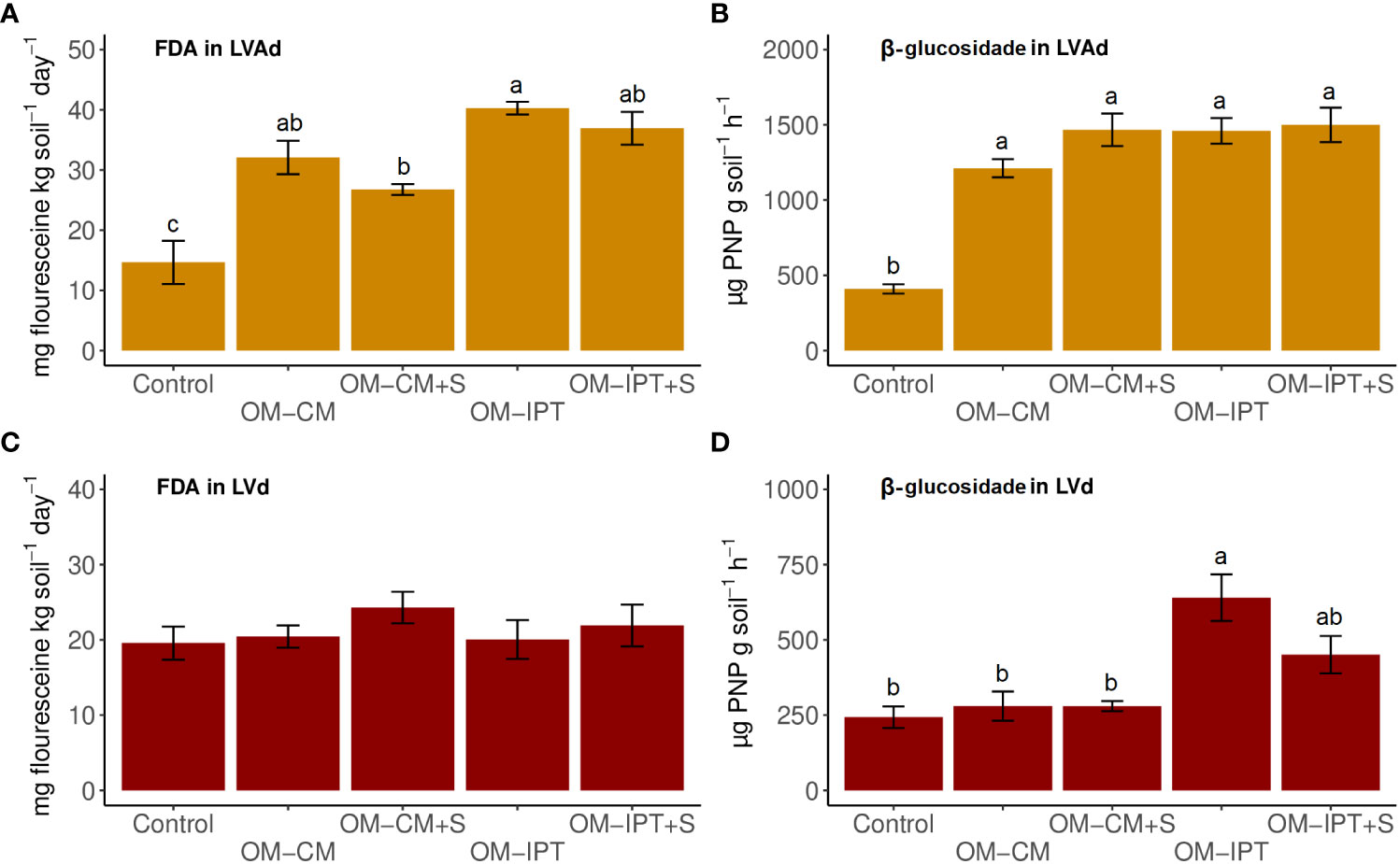
Figure 6 General soil enzymatic activity (fluorescein diacetate hydrolysis - FDA) and β-glucosidase in soils in a typical dystrophic Red-Yellow Latosol (LVAd – A, B) and a typical dystrophic Red Latosol (LVd – C, D) after wheat cultivation with different organo-mineral fertilizers (mean ± SE, n = 5). The averages followed by the same letter do not differ statistically by the ANOVA and Tukey test results (p ≤ 0.05). The description of the treatments are: Control (without application), OM-CM (organo-mineral fertilizer formulated with a commercial manure, containing NPK), OM-CM+S (organo-mineral fertilizer formulated with a commercial manure, containing NPK+S), OM-IPT (organo-mineral fertilizer formulated with a by-product of the intermediate processes of tanning (BPIPT), containing NPK), OM-IPT+S (organo-mineral fertilizer formulated with a BPIPT containing NPK+S).
3.6 Incubation of OMF and wheat cultivation: soil pH
The pH values in CaCl2 (Figures 4C, F) and in H2O (Figure S2) at the end of cultivation decreased after the application of OMF in the soils.
In the LVAd, the application of OM-CM+S and OM-IPT+S caused the highest acidification (Figures 4C, F). As for the LVd, the application of OMF reduced the pH compared with the control treatment, but no differences were observed among OMF treatments.
3.7 Principal component analysis of soil chemical and microbial attributes
The PCA shows the clusters between the OMF applied in both soils (Figure 7). Variables related to plant productivity, SDM, and soil microbial activities were in the same group, suggesting that OMF applications led to increased SDM production and improved the microbiological quality of both soils (Figure 7). In the LVAd, the treatments had a similar response, with close clustering, influenced by the increased contents of elements in SDM and soil microbiological activity (Figure 7A). In the LVd, the group of OM-IPT was directly related to SDM, nutrient content in SDM, and β-glucosidase activity. Such pattern suggests that this product positively influenced SDM and plant nutrition in this soil (Figure 7B).
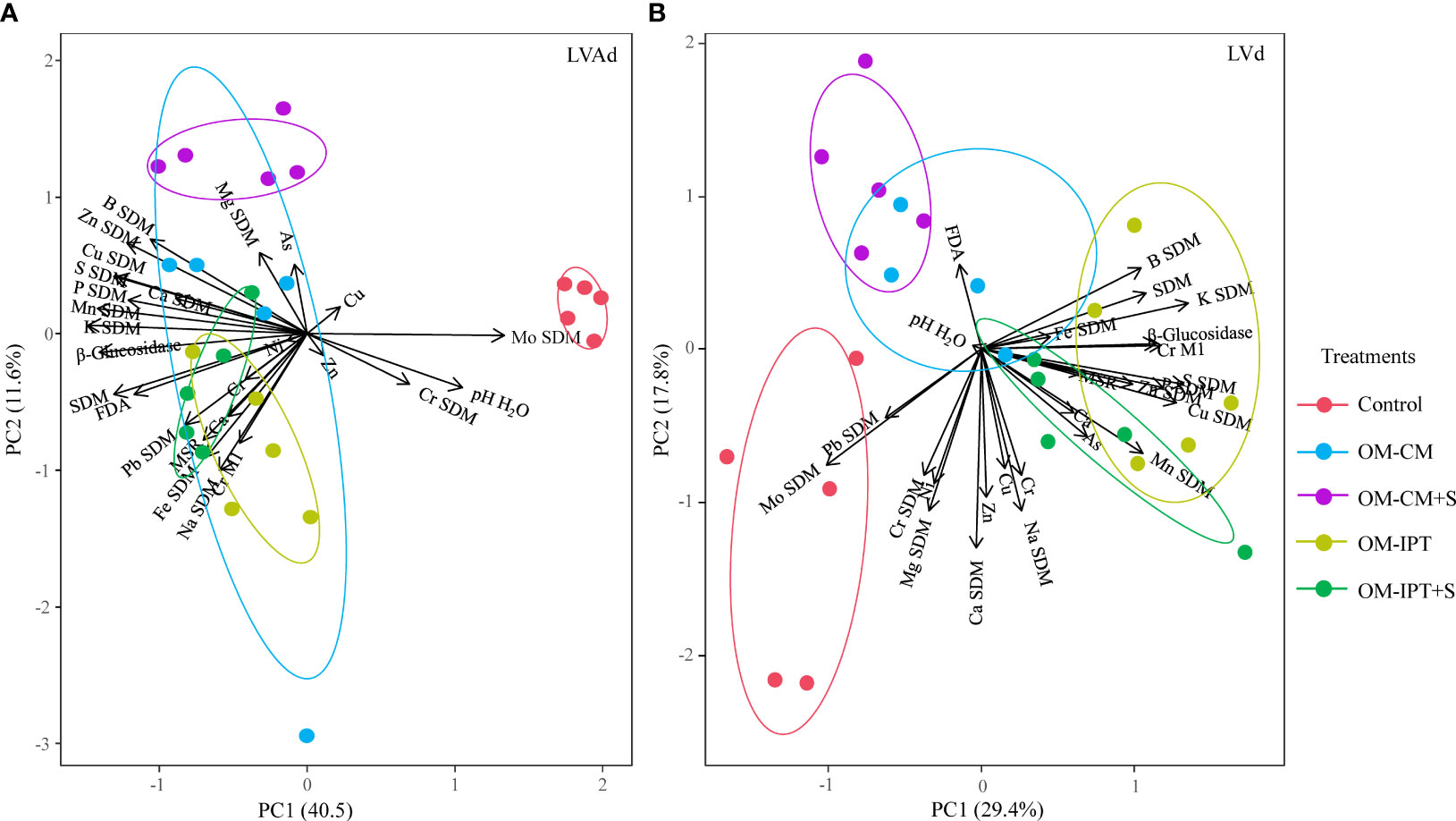
Figure 7 Principal component analysis (PCA) for typical dystrophic Red-Yellow Latosol (LVAd - A) and typical dystrophic Red Latosol (LVd - B) and variables shoot dry mass (SDM), root dry mass (RDM), content of nutrients and other elements in SDM, pH in H2O, general soil enzyme activity (FDA) and β-glucosidase, Cr contents in soils by Melhich-1 (Cr_M1) and Cr, As, Cu, Zn, and Ni contents in soils via portable X-ray fluorescence. The description of the treatments are: Control (without application), OM-CM (organo-mineral fertilizer formulated with a commercial manure, containing NPK), OM-CM+S (organo-mineral fertilizer formulated with a commercial manure, containing NPK+S), OM-IPT (organo-mineral fertilizer formulated with a by-product of the intermediate processes of tanning (BPIPT), containing NPK), OM-IPT+S (organo-mineral fertilizer formulated with a BPIPT containing NPK+S).
4 Discussion
4.1 Impacts of OMF on Cr contents in the soil and wheat plants
The contents of Cr, P, and K in the soil were modified after the incubation of OMF in both soils (Figure 1). Although the responses were diverse concerning the effects of treatments in the different soils (Figure 1), it was possible to note that this greater availability of elements - plant nutrients or not - was beneficial for the development of wheat and for the biological activity of the soil (Figures 2; 3; 5).
The total Cr contents varied within the soils (Figure 4), with a much higher total Cr content in the LVd (1472 mg kg-1) than in the LVAd (54 mg kg-1). Such increased Cr levels in the LVd are likely a result of the wreathing of the parent mafic rocks, which can contain up to 3400 mg kg-1 of Cr (Kabata-Pendias and Mukherjee, 2007). Nonetheless, the Cr available fraction in the LVd was lower than 0.2%. Even at such low levels, the Cr available fraction increased after the OMF application in the LVd, thus evidencing the potential of these fertilizers to increase Cr availability in this soil. The LVAd had lower levels of Cr, clay, and Fe2O3, suggesting a lower buffering capacity and possibly greater responses to management practices. Indeed, the application of OM-IPT and OM-IPT+S in this soil increased the Cr available fraction, whereas the S-containing treatments (OM-CM+S and OM- IPT+S) caused soil acidification compared with the control (Figure 4).
Adding organic and inorganic amendments can increase the sorption of Cr (III) in soils. These products release hydroxyl ions and increase the negative charges in the soil surface layers, thus favoring the retention of Cr (III) and decreasing Cr (VI) sorption (Choppala et al., 2018). The increase in Cr (VI) sorption is possible by adding elemental S (S0), as the S0 oxidation causes soil acidification and thus results in the opposite effect of liming on Cr (VI) and Cr (III) species (Choppala et al., 2018). Besides, the sorption of Cr in the soil also relates to the type of SOM and clay (Covelo et al., 2007). Chromium - Cr (III) - can form inner-sphere complexes with SOM, with varying solubilities, or remain in the insoluble humin fraction of SOM (Ratke et al., 2019). In the present study, the addition of OMF likely increased the SOM levels in the soils, contributing to higher sorption of Cr compared with the control. Thus, Cr was less available to plant uptake, which explains the overall lower Cr contents in SDM after the OMF application in both soils, particularly the LVAd (Figures 5A, D). Organic matter additions can also stimulate microbial activity and the biotic reduction of Cr (VI) to Cr (III) in soils. Accordingly, organic sources (e.g., animal manure, compost, biosolids, or plant biomass) are commonly used as a recovery strategy in Cr-contaminated sites (Shahid et al., 2017; Choppala et al., 2018).
The OMF produced from BPIPT increased the content of available Cr in the soils (Figure 1). However, such an increase did not seem to affect the development of wheat plants (Figure 5). Indeed, the OMF produced with BPIPT provided a high level of nutrients for the plants, comparable to the other fertilizer sources (Table 3). For plants grown in the LVAd, the highest SDM yields occurred after the application of OMF produced from BPIPT. Tannery by-products-based fertilizers have shown many benefits to soil attributes and plant production. They have increased C and N contents in their composition (Ciavatta et al., 2012; Majee et al., 2021) and have a gradual N release (Lima et al., 2010), which optimizes the use of N fertilizers by the crops. Such enhanced N use efficiency can result in increased yields (Castilhos et al., 2002; Coelho et al., 2015) and lower soil acidity (Ferreira et al., 2003; Teixeira et al., 2006). As such, using the BPIPT to produce OMF favors a circular economy (Chojnacka et al., 2021), as the by-product is incorporated into a different production sector, that is, from the tannery industry to agriculture.
The application of OMF in soils reduced the Cr uptake by wheat plants (Figure 5). Plants can capture both Cr (III) and Cr (VI). Cr (III) uptake by plants is a passive mechanism and does not require energy. As for Cr (VI), its uptake by plants is an active process (generally via phosphate or sulfate transport), due to the similarity of CrO42- with these anions. The presence of sulfate in soils can even inhibit Cr (VI) uptake by plants (Shahid et al., 2017). The Cr available contents in soils (total Cr divided by Cr determined via Mehlich-1 extractor) below 3 mg kg-1 (Figure 1) were not toxic to wheat plants in both soils. The Cr contents in SDM ranged from 6.6 to 2.4 mg kg-1, with the highest level in the LVAd control treatment (Table 3). Toxicity symptoms were not observed, even at the highest Cr contents in SDM. Toxic effects of Cr for most plants occur at foliar contents higher than 18.0 mg kg-1 (Losi et al., 1994). Coelho et al. (2015) and Oliveira et al. (2008), after using the tannery by-product as an N source, also did not report toxic levels of Cr in wheat and elephant grass, respectively. Wheat germination can be affected by Cr contents ≥ 4,000 mg kg-1 and ≥ 2,000 mg kg-1 for oats and sorghum that received the application of tannery by-products (López-Luna et al., 2009).
Liming was performed to simulate the process of soil acidity correction in tropical soils and to maintain the appropriate pH range for the cultivation of plants. It is known that the use of fertilizers with elemental sulfur (S°) may cause soil acidification, from the increasing content of hydrogen ions (H+) formed during its microbiological oxidation (Lisowska et al., 2022). Soil acidification with the presence of S was observed in the LVAd, which has lower buffer capacity, yet this did not cause a higher availability of Cr.
4.2 Impacts of OMF on soil microbiological attributes
The OMF application promoted increases in MBC and in the biological and enzymatic activities of these soils (Figures 2, 3), except for arylsulfatase in the LVd (Figure 3F), thus evidencing the beneficial effects of these fertilizers. This effect was probably due to the increased availability of nutrients and energy for the microbial community in this soil. The tannery by-products are rich in proteins and lipids, which represent a promising source of nutrients, they can stimulate the decomposing and mineralizing activities of the heterotrophic community of the soil, providing nutrients to plants and microorganisms (Majee et al., 2021). What makes the reuse of this waste an eco-friendly activity, is because in addition to disseminating the concept of circular economy in the industry (Velenturf et al., 2019), it is also a source of multi-element fertilizers for plants and microorganisms.
The Cr contents present in the OMF do not seem to have an impact on the microbial activities in the soil (Figures 2, 6). In fact, the application of OMF led to higher wheat yields and increases in enzymatic activities in the soil. However, the activity of the enzyme arylsulfatase decreased after the application of OMF in the LVd (Figure 3F), which may be related to a higher S availability after applying the products. This enzyme acts in some stages of the mineralization of organic S, and reductions in arylsulfatase activity may occur if inorganic S is present in these fertilizers. Oliveira-Longatti et al. (2017) also observed increases in microbial biomass and the activities of β-glucosidase and urease enzymes after the application of tannery by-products in two tropical soils. The authors demonstrated that the trace levels of Cr in the by-product were not harmful to the biological activity in the soil.
With the addition of OMF, it was possible to observe that there were increases in the availability of resources, carbon, and nutrients for the microbiota, which favored the increase in MBC and microbial activities, even in a short experimental period (Figures 2E, 6). Microbial ecophysiological characteristics, such as MBC and SBR, are known to control the renewal, accumulation, and losses of C in soils (Schimel, 2013; Liang et al., 2017). MBC stabilizes C in soils through the formation of microbial biomass. Such function is crucial for efficient nutrient cycling, given that microorganisms are the primary agents in the decomposition process (Bradford et al., 2013; Liang et al., 2017; Majee et al., 2021). At the same time, microorganisms rely on the availability of organic matter as a source of energy and nutrients, particularly N, to build their biomass and release and activate their enzymes (Moorhead et al., 2013; Sokol and Bradford, 2019).
The metabolic quotient (qCO2) was more efficient than SBR in showing a decrease in the metabolic stress of the microbial community after the addition of OMF in both soils (Figures 2C, F). Soil microbiota was more efficient in storing C in the biomass in treatments that received OMF, considering the reduced qCO2 in the OMF treatments (Figures 2C, F). Such reduced qCO2 is a positive aspect of these materials on the soil microbiota. The qCO2 is the ratio of basal respiration rates to microbial biomass and represents a simplified indicator of energy demands for cellular maintenance and microbial C use (Anderson and Domsch, 1993; Joergensen and Wichern, 2018). As a result, higher qCO2 rates can potentially enhance C losses from soil microorganisms, thus decreasing C accumulation in soils and ecosystem functioning (Schimel, 2013; Malik et al., 2020).
The activities of β-glucosidase and FDA were evaluated before and after wheat cultivation (Figures 3, 6). These enzymes were very sensitive in evaluating soil microbiological activity in the incubation stage and the plant development after the OMF application. Both soils presented a trend for increasing β-glucosidase and FDA activities after the OMF application and higher wheat yields (Figures 3, 6). Thus, our results corroborate the use of enzymatic activities of soil microbiota as a sensitive indicator of management changes in the soil (Wahsha et al., 2017; Anghinoni et al., 2021). Besides, we evidenced the positive relationship between enzymatic activities and crop yield (Lopes et al., 2013; Mendes et al., 2019), and demonstrated that tannery by-products containing trace levels of Cr were not detrimental to the microbiological activity in the soil (Oliveira-Longatti et al., 2017).
4.3 OMF and current Brazilian regulations concerning Cr in soils and fertilizers
The total Cr contents determined by pXRF in the LVAd (average value of 54 mg kg-1) are below the prevention value for Cr in soils in Brazil (75 mg kg-1; Brasil, Conselho Nacional do Meio Ambiente – CONAMA, 2009). The application of OM-IPT+S led to a total Cr content of 106.8 mg kg-1, higher than the other treatments, but below the intervention value for agricultural use (150 mg kg-1). The LVd had a much higher total Cr content (1472 mg kg-1). Such increased value reflects the composition of the parent material and strengthens the premise that threshold values must be determined with respect to different assumptions and modeling. Some countries or regions emphasize that threshold values must vary according to soil types (clay content), extractors, pH values and soil depth (Carlon, 2007; Vácha et al., 2016). Most regulations have threshold values for Cr (VI) in soils, due to its deleterious effects to human and environmental health.
The Cr contents in the OMF were 187.2, 94.1, 10,061.2 and 10,307.7 mg kg-1 for OM-CM, OM-CM+S, OM-IPT, and OM-IPT+S, or 0.02, 0.01, 1.01 and 1.03%, respectively. Most threshold values for fertilizing products do not consider total Cr contents, which complicates our attempt to establish safe levels for the use of tannery by-products-based fertilizers. The threshold value for organic fertilizers and soil conditioners in Brazil is 2 mg kg-1 of Cr (VI) per dry matter and 200 mg kg-1 of total Cr for inorganic fertilizers containing macronutrients (Brasil, Ministério da Agricultura, Pecuária e Abastecimento – MAPA, 2006). In Europe, organic or organo-mineral fertilizer can have up to 2 mg kg-1 Cr (VI) per dry matter (European Commission, 2019). Future studies of Cr in soils following the OMF application should include direct speciation analyses (e.g., synchrotron-based spectroscopies) to investigate the chemical forms of Cr in soils, tannery by-products, and organo-mineral fertilizers. This knowledge would demonstrate the suitability of these products within the current regulations and evidence the impacts of long-term OMF applications on Cr (VI) levels in soils.
5 Conclusions
The application of organo-mineral fertilizer (OMF) in the soils of the present study increased growth, macro (P, K, Ca, Mg and S) and micronutrients (Cu, Fe, Mn, B, Zn) contents in wheat plants. Such trend was more evident in the dystrophic Red-Yellow Latosol (LVAd), which had lower contents of clay and Fe oxides than the dystrophic Red Latosol (LVd), and thus, can be more responsive to changes in management practices. After wheat cultivation, the presence of S in the OMF led to soil acidification.
The application of OMF formulated with by-product of the intermediate processes of tanning (BPIPT) increased available Cr fraction contents in both soils. Overall, the OM-IPT treatment (formulated with BPIPT, containing NPK and without S) caused the lowest acidification and highest biomass production, and highest soil enzymatic activity. However, OM-IPT treatment presented the highest available Cr fraction (3.6%) in the LVAd, but it did not lead to increased Cr contents in shoot dry mass. When compared to control, the Cr in shoot dry mass was lower in OMF treatments for both soils, which was attributed to the complexation of Cr by organic matter added via OMF.
The BPIPT-derived OMF tested were very beneficial to soils and plants, and the presence of Cr in these materials was harmless to plants and soil microbiota. Lastly, our findings have shown that the Brazilian regulation regarding Cr contents in organo-mineral fertilizers needs revision and, possibly, the inclusion of thresholds for Cr (VI) rather than total Cr contents. Such threshold values would facilitate the inclusion of these products in the fertilizer market and stimulate the reuse of tannery by-products, thus favoring a sustainable production and circular economy.
Data availability statement
The raw data supporting the conclusions of this article will be made available by the authors, without undue reservation.
Author contributions
The experiment was mainly planned and designed by FL and LG, and all authors were involved in the designing of the study. FL: conceptualization, methodology, investigation, data analysis, writing (original draft preparation). AS: collecting all the data, methodology, writing (review and editing). HA: collecting all the data, methodology, writing (review and editing). EF: collecting all the data, methodology, writing (review and editing). RC: collecting all the data, methodology, writing (review and editing). MJ: collecting the data, methodology, writing (review and editing). MC: conceptualization, methodology, supervision, writing (review and editing). LG: conceptualization, methodology, investigation, analysis of the data, project administration, supervision, writing (review and editing). All authors contributed to the article and approved the submitted version.
Funding
This research was financed by grants from the Minas Gerais State Research Foundation (FAPEMIG), National Council for Scientific and Technological Development (CNPq), and Coordination for the Improvement of Higher Education Personnel (CAPES).
Acknowledgments
The authors would like to thank the funding agencies Minas Gerais State Research Foundation (FAPEMIG), National Council for Scientific and Technological Development (CNPq), and Coordination for the Improvement of Higher Education Personnel (CAPES) for the financial support of this study and the scholarships provided. Also, the authors thank the National Institute of Science and Technology on Soil and Food Security (CNPq Grant #406577/2022-6) and ILSA Brazil Fertilizers.
Conflict of interest
The authors declare that the research was conducted in the absence of any commercial or financial relationships that could be construed as a potential conflict of interest.
Publisher’s note
All claims expressed in this article are solely those of the authors and do not necessarily represent those of their affiliated organizations, or those of the publisher, the editors and the reviewers. Any product that may be evaluated in this article, or claim that may be made by its manufacturer, is not guaranteed or endorsed by the publisher.
Supplementary material
The Supplementary Material for this article can be found online at: https://www.frontiersin.org/articles/10.3389/fagro.2023.1215448/full#supplementary-material
References
Alef K. (1995). ““Field methods,” in Methods in Applied Soil Microbiology and Biochemistry. Eds. K. Alef and P. Nannipieri (Cambridge: Academic Press), 463–490. doi: 10.1016/B978-012513840-6/50025-2
Alloway B. J. (2012). Heavy metals in soils: trace metals and metalloids in soils and their bioavailability (Reading: Springer).
Alvarez V. H., Ribeiro A. C. (1999). “Calagem,” in Recomendações Para o Uso de Corretivos e Fertilizantes em Minas Gerais: 5A Aproximação (Viçosa: CFSEMG).
Anderson T. H., Domsch K. H. (1993). The metabolic quotient for CO2 (qCO2) as a specific activity parameter to assess the effects of environmental conditions, such as pH, on the microbial biomass of forest soils. Soil Biol. Biochem. 25, 393–395. doi: 10.1016/0038-0717(93)90140-7
Anghinoni G., Anghinoni F. B. G., Tormena C. A., Braccini A. L., Mendes I. C., Zancanaro L., et al. (2021). Conservation agriculture strengthen sustainability of Brazilian grain production and food security. Land Use Policy. 108, 105591. doi: 10.1016/j.landusepol.2021.105591
Bradford M. A., Keiser A. D., Davies C. A., Mersmann C. A., Strickland M. S. (2013). Empirical evidence that soil carbon formation from plant inputs is positively related to microbial growth. Biogeochemistry. 113, 271–281. doi: 10.1007/s10533-012-9822-0
Brasil, Conselho Nacional do Meio Ambiente – CONAMA (2009). Resolução CONAMA n° 420, de 28 de dezembro de 2009: Dispõe sobre critérios e valores orientadores de qualidade do solo quanto à presença de substâncias quı́micas e estabelece diretrizes para o gerenciamento ambiental de áreas contaminadas por essas substâncias em decorrência de atividades antrópicas (Brası́lia, DF: Diário Oficial da União). Available at: http://www.mma.gov.br/port/conama/res/res09/res42%20009.pdf.
Brasil, Ministério da Agricultura, Pecuária e Abastecimento – MAPA (2006). Instrução Normativa SDA N° 27, de 05 de junho de 2006 (Alterada pela IN SDA n° 7, de 12/04/2016, republicada em 02/05/2016). Dispõe sobre fertilizantes, corretivos, inoculantes e biofertilizantes para serem produzidos, importados e comercializados, deverão atender aos limites estabelecidos no que se refere às concentrações máximas admitidas para agentes fitotóxicos, patogênicos ao homem, animais e plantas, metais pesados tóxicos, pragas e ervas daninhas (Brasília, DF: Diário Oficial da União). Available at: https://www.gov.br/agricultura/pt-br/assuntos/insumos-agropecuarios/insumos-agricolas/fertilizantes/legislacao/in-sda-27-de-05-06-2006-alterada-pela-in-sda-07-de-12-4-16-republicada-em-2-5-16.pdf.
Brasil, Ministério da Agricultura, Pecuária e Abastecimento – MAPA (2017). Manual de métodos Analíticos Oficiais para Fertilizantes e Corretivos (Brasília, DF: Secretaria de Defesa Agropecuária). Available at: https://www.gov.br/agricultura/pt-br/assuntos/insumos-agropecuarios/insumos-agricolas/fertilizantes/legislacao/manual-de-metodos_2017_isbn-978-85-7991-109-5.pdf.
Carlon C. (2007). Derivation methods of soil screening values in Europe. A review and evaluation of national procedures towards harmonisation (Ispra: European Commission). Available at: https://esdac.jrc.ec.europa.eu/ESDB_Archive/eusoils_docs/other/EUR22805.pdf
Carter M. R., Gregorich E. G. (2006). Soil Sampling and Methods of Analysis (Boca Raton: CRC press).
Castilhos D. D., Tedesco M. J., Vidor C. (2002). Rendimentos de culturas e alterações químicas do solo tratado com resíduos de curtume e crômio hexavalente. Rev. Bras. Cienc. Solo. 26, 1083–1092. doi: 10.1590/S0100-06832002000400026
Chau T. P., Bulgariu L., Saravanan M., Rajkumar R., Chinnathambi A., Salmen S. H., et al. (2023). Bioremediation efficiency of free and immobilized form of Aspergillus niger and Aspergillus tubigenesis biomass on tannery effluent. Environ. Res. 231, 116275. doi: 10.1016/j.envres.2023.116275
Chojnacka K., Skrzypczak D., Mikula K., Witek-Krowiak A., Izydorczyk G., Kuligowski K., et al. (2021). Progress in sustainable technologies of leather wastes valorization as solutions for the circular economy. J. Clean. Prod. 313, 127902. doi: 10.1016/j.jclepro.2021.127902
Choppala G., Kunhikrishnan A., Seshadri B., Hee J., Bush R., Bolan N. (2018). Comparative sorption of chromium species as influenced by pH, surface charge and organic matter content in contaminated soils. J. Geochemical Explor. 184, 255–260. doi: 10.1016/j.gexplo.2016.07.012
Ciavatta C., Manoli C., Cavani L., Franceschi C., Sequi P. (2012). Chromium-containing organic fertilizers from tanned hides and skins: A review on chemical, environmental, agronomical and legislative aspects. J. Environ. Prot. 03, 1532–1541. doi: 10.4236/jep.2012.311169
Coelho L. C., Ferreira M. M., Bastos A. R. R., Oliveira L. C. A. D., Ferreira E. D. (2015). Resíduo de curtumes como fonte de nitrogênio para trigo e arroz em sucessão. Rev. Bras. Cienc. Solo. 39, 1445–1455. doi: 10.1590/01000683rbcs20140608
Covelo E. F., Vega F. A., Andrade M. L. (2007). Simultaneous sorption and desorption of Cd, Cr, Cu, Ni, Pb, and Zn in Acid Soils. J. Hazard. Mater. 147, 862–870. doi: 10.1016/j.jhazmat.2007.01.123
Dick R. P. (2011). Methods of Soil Enzymology, Methods of Soil Enzymology (Madison: American Society of Agronomy, Crop Science Society of America, and Soil Science Society of America). doi: 10.2136/sssabookser9
Eivazi F., Tabatabai M. A. (1988). Glucosidases and galactosidases in soils. Soil Biol. Biochem. 20, 601–606. doi: 10.1016/0038-0717(88)90141-1
Ertani A., Mietto A., Borin M. (2017). Chromium in agricultural soils and crops: A review. Water Air Soil pollut. 228, 190. doi: 10.1007/s11270-017-3356-y
European Commission (2019). 1009 of the European parliament and of the council of 5 june 2019 laying down rules on the making available on the market of EU fertilising products and amending regulations (EC) no 1069/2009 and (EC) no 1107/2009 and repealing regulation (EC) no 2003/2003. 2019. J. Eur. Union 170, 1–114.
Ferreira A. S., Camargo F. A. O., Tedesco M. J., Bissani C. A. (2003). Alterações de atributos químicos e biológicos de solo e rendimento de milho e soja pela utilização de resíduos de curtume e carbonífero. Rev. Bras. Cienc. Solo. 27, 755–763. doi: 10.1590/S0100-06832003000400020
Hoeft R. G., Walsh L. M., Keeney D. R. (1973). Evaluation of various extractants for available sulfur. Soil Sci. Soc Am. Proc. 37, 401–404. doi: 10.2136/sssaj1973.03615995003700030027x
Islam K. R., Weil R. R. (1998). Microwave irradiation of soil for routine measurement of microbial biomass carbon. Biol. Fert. Soils. 27, 408–416. doi: 10.1007/s003740050
Joergensen R. G., Wichern F. (2018). Alive and kicking: Why dormant soil microorganisms matter. Soil Biol. Biochem. 116, 419–430. doi: 10.1016/j.soilbio.2017.10.022
Kabata-Pendias A., Mukherjee A. B. (2007). Trace Elements from Soil to Human (Berlin: Springer-Verlag).
Kožuh N., Štupar J., Gorenc B. (2000). Reduction and oxidation processes of chromium in soils. Environ. Sci. Technol. 34, 112–119. doi: 10.1021/es981162m
Length R. (2020). “emmeans: estimated marginal means, aka least-squares means,” in R package version 1.4. Available at: https://CRAN.R-project.org/package=emmeans.
Liang C., Schimel J. P., Jastrow J. D. (2017). The importance of anabolism in microbial control over soil carbon storage. Nat. Microbiol. 2, 1–6. doi: 10.1038/nmicrobiol.2017.105
Lima D. Q., Oliveira L. C. A., Bastos A. R. R., Carvalho G. S., Marques J. J. G. S. M., Carvalho J. G., et al. (2010). Leather industry solid waste as nitrogen source for growth of common bean plants. Appl. Environ. Soil Sci., 2010, 703842. doi: 10.1155/2010/703842
Lisowska A., Filipek-Mazur B., Komorowska M., Niemiec M., Bar-Michalczyk D., Kuboń M., et al. (2022). Environmental and production aspects of using fertilizers based on waste elemental sulfur and organic materials. Materials 15, 3387. doi: 10.3390/ma15093387
Lopes A. A. C., Sousa D. M. G., Chaer G. M., Reis Junior F. B., Goedert W. J., Mendes I. C. (2013). Interpretation of microbial soil indicators as a function of crop yield and organic carbon. Soil Sci. Soc Am. J. 77, 461–472. doi: 10.2136/sssaj2012.0191
López-Luna J., González-Chávez M. C., Esparza-García F. J., Rodríguez-Vázquez R. (2009). Toxicity assessment of soil amended with tannery sludge, trivalent chromium and hexavalent chromium, using wheat, oat and sorghum plants. J. Hazard. Mater. 163, 829–834. doi: 10.1016/j.jhazmat.2008.07.034
Losi M. E., Amrhein C., Frankenberger W. T. (1994). Environmental biochemistry of chromium. Rev. Environ. Contam. Toxicol. 91-121. doi: 10.1007/978-1-4612-2656-7_3
Majee S., Halder G., Mandal D. D., Tiwari O. N., Mandal T. (2021). Transforming wet blue leather and potato peel into an eco-friendly bio-organic NPK fertilizer for intensifying crop productivity and retrieving value-added recyclable chromium salts. J. Hazard. Mater. 411, 125046. doi: 10.1016/j.jhazmat.2021.125046
Malik A. A., Martiny J. B. H., Brodie E. L., Martiny A. C., Treseder K. K., Allison S. D. (2020). Defining trait-based microbial strategies with consequences for soil carbon cycling under climate change. ISME J. 14, 1–9. doi: 10.1038/s41396-019-0510-0
Mehlich A. (1953). Determination of P, ca, mg, K, na, and NH4 (1953) (Raleigh, North Carolina: North Carolina Soil Test Division).
Mendes I. C., Souza L. M., Sousa D. M. G., Lopes A. A. C., Reis Junior F. B., Lacerda M. P. C., et al. (2019). Critical limits for microbial indicators in tropical Oxisols at post-harvest: The FERTBIO soil sample concept. Appl. Soil Ecol. 139, 85–93. doi: 10.1016/j.apsoil.2019.02.025
Moorhead D.L., Rinkes Z.L., Sinsabaugh R.L., Weintraub M. N., 2013. Dynamic relationships between microbial biomass, respiration, inorganic nutrients and enzyme activities: informing enzyme-based decomposition models. Front. Microbiol. 4, 1–12. https://doi.org/10.3389/fmicb.2013.00223.
Nogueira F. G., Castro I. A., Bastos A. R., Souza G. A., de Carvalho J. G., Oliveira L. C. (2011). Recycling of solid waste rich in organic nitrogen from leather industry: Mineral nutrition of rice plants. J. Hazard. Mater. 186, 1064–1069. doi: 10.1016/j.jhazmat.2010.11.111
Oksanen J., Blanchet F. G., Friendly M., Kindt R., Legendre P., McGlinn D., et al. (2016). Vegan: Community Ecology Package. Ordination methods, diversity analysis and other functions for community and vegetation ecologists. Available at: https://cran.r-project.org/web/packages/vegan/vegan.pdf
Oliveira D. Q. L. D., Carvalho K. T. G., Bastos A. R. R., Oliveira L. C. A. D., Marques J. J. G. D. S., Nascimento R. S. M. P. D. (2008). Utilização de resíduos da indústria de couro como fonte nitrogenada para o capim-elefante. Rev. Bras. Cienc. Solo. 32, 417–424. doi: 10.1590/S0100-06832008000100039
Oliveira-Longatti S. M., Cannata M. G., Ferrazani J. C., Carvalho T. S., Ciavatta C., Guilherme L. R. G. (2017). Agronomic and environmental implications of using a by-product of the intermediate tanning processes as nitrogen fertilizer. Sci. Agric. 743, 250–257. doi: 10.1590/1678-992X-2016-000
Penha J. G., Carvalho G. S., de Abreu L. B., Ribeiro B. T., de Souza Costa E. T., Marques J. J. (2017). Boletim Técnico - Procedimentos para quantificação de elementos-traço por espectrofotometria de absorção atômica em matrizes de interesse ambiental (Lavras: Editora UFLA). doi: 10.13140/RG.2.2.24948.55685
Ratke R. F., Lemke-de-Castro M. L., De-Campos A. B., Rocha C., Barbosa J. M., Verginassi A., et al. (2019). Sorption and desorption of chromium from applied tannery sludge in soils under pastures and Cerrado vegetation. Rev. Ciências Agrárias. 42, 358–368. doi: 10.19084/rca.17177
R Development Core Team (2020). R: A Language and Environment for Statistical Computing (Vienna, Austria: R Foundation for Statistical Computing).
Santos H. G., Jacomine P. K. T., Anjos L. H. C., Oliveira V. A., Lumbreras J. F., Coelho M. R., et al. (2018). Sistema Brasileiro de Classificação de Solos (Rio de Janeiro: Embrapa Solos).
Schimel J. P., Schaeffer S. M. (2013). Microbes and global carbon. Nat. Clim. Chang. 3, 867–868. doi: https://doi.org/10.1038/nclimate2015
Shahid M., Shamshad S., Rafiq M., Khalid S., Bibi I., Niazi N. K., et al. (2017). Chromium speciation, bioavailability, uptake, toxicity and detoxification in soil-plant system: A review. Chemosphere. 178, 513–533. doi: 10.1016/j.chemosphere.2017.03.074
Sokol N. W., Bradford M. A. (2019). Microbial formation of stable soil carbon is more efficient from belowground than aboveground input. Nat. Geosci. 12, 46–53. doi: 10.1038/s41561-018-0258-6
Stefan D. S., Bosomoiu M., Constantinescu R. R., Ignat M. (2021). Composite polymers from leather waste to produce smart fertilizers. Polymers 13, 4351. doi: 10.3390/polym13244351
Tabatabai M. A., Bremner J. M. (1970). Arylsulfatase activity of soils. Soil Sci. Soc Am. Proc. 34, 225–229. doi: 10.2136/sssaj1970.03615995003400020016x
Teixeira P. C., Donagemma G. K., Wenceslau A. F., Teixeira G. (2017). Manual de Métodos de Análise de Solo (Rio de Janeiro: Embrapa Solos).
Teixeira K. R. G., Gonçalves Filho E. M. S., Carvalho A. S. F., Araújo L., Santos V. B. (2006). Efeito da adição de lodo de curtume na fertilidade do solo, nodulação e rendimento de matéria seca do caupi. Cienc. e Agrotecnologia. 30, 1071–1076. doi: 10.1590/s1413-70542006000600004
USEPA (2007a). Method 3051A (SW-846): Microwave Assisted Acid Digestion of Sediments, Sludges, and Oils, Revision. 1. ed (Washington: USEPA).
USEPA (2007b). Method 6200 - Field Portable X-ray Fluorescence Spectrometry for the Determination of Elemental Concentrations in Soil and Sediment (Washington: USEPA).
Vácha R., Sáňka M., Skála J., Čechmánková J., Horváthová V. (2016). “Soil contamination health risks in Czech proposal of soil protection legislation,” in Environmental Health Risk, 1st ed. Ed. Larramendy M., 57–75.
Vance E. D., Brookes P. C., Jenkinson D. S. (1987). An extraction method for measuring soil microbial biomass C. Soil Biol. Biochem. 19, 703–707. doi: 10.1016/0038-0717(87)90052-6
Velenturf A. P. M., Archer S. A., Gomes H. I., Christgen B., Lag-Brotons A. J., Purnell P. (2019). Circular economy and the matter of integrated resources. Sci. Total Environ. 689, 963–969. doi: 10.1016/j.scitotenv.2019.06.449
Wahsha M., Nadimi-Goki M., Fornasier F., Al-Jawasreh R., Hussein E. I., Bini C. (2017). Microbial enzymes as an early warning management tool for monitoring mining site soils. Catena. 148, 40–45. doi: 10.1016/j.catena.2016.02.021
Weindorf D. C., Chakraborty S. (2020). Portable X-ray fluorescence spectrometry analysis of soils. Soil Sci. Soc. Am. J. 84, 1384–1392. doi: 10.1002/saj2.20151
Keywords: tannery waste, circular economy, innovative fertilizer, microbial attributes, chromium
Citation: Lima FRDd, Silva AO, Amorim HCS, Figueredo ESS, Carneiro RMC, Jara MS, Carneiro MAC and Guilherme LRG (2023) Agronomic and environmental aspects of organo-mineral fertilizers produced with a by-product of the intermediate process of tanning. Front. Agron. 5:1215448. doi: 10.3389/fagro.2023.1215448
Received: 02 May 2023; Accepted: 18 July 2023;
Published: 04 August 2023.
Edited by:
Thiago Nogueira, São Paulo State University, BrazilReviewed by:
Xiaoping Xin, University of Florida, United StatesBulgariu Laura, Gheorghe Asachi Technical University of Iași, Romania
Copyright © 2023 Lima, Silva, Amorim, Figueredo, Carneiro, Jara, Carneiro and Guilherme. This is an open-access article distributed under the terms of the Creative Commons Attribution License (CC BY). The use, distribution or reproduction in other forums is permitted, provided the original author(s) and the copyright owner(s) are credited and that the original publication in this journal is cited, in accordance with accepted academic practice. No use, distribution or reproduction is permitted which does not comply with these terms.
*Correspondence: Luiz Roberto Guimarães Guilherme, guilherm@ufla.br