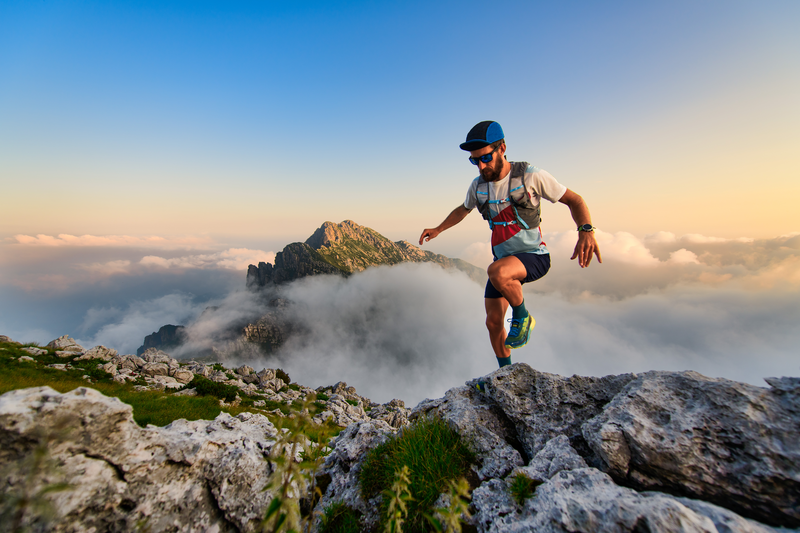
94% of researchers rate our articles as excellent or good
Learn more about the work of our research integrity team to safeguard the quality of each article we publish.
Find out more
SYSTEMATIC REVIEW article
Front. Agron. , 05 July 2023
Sec. Agroecological Cropping Systems
Volume 5 - 2023 | https://doi.org/10.3389/fagro.2023.1215441
This article is part of the Research Topic Sustainable Cropping Systems for Small Grain Crops for Smallholder Farmers View all 5 articles
Quinoa (Chenopodium quinoa Wild.) is a drought and salinity-tolerant crop that originated in the Andes over 7000 years ago. It is adapted to different agroecological areas and can be grown from sea level to an altitude of 4000 m. The outstanding nutritional status of quinoa, with its high content of proteins, vitamins, and minerals, makes it a promising crop able to combat hunger and malnutrition in different countries in the 21st century. Quinoa cultivation has expanded from South America to Africa, Europe, Asia, and North America. Reviewing quinoa cropping practices will provide farmers with adequate recommendations for improving the agronomic and environmental sustainability of quinoa cultivation worldwide. For this reason, we conducted a systematic review of agronomic management practices in 148 field experiments conducted worldwide from 2000 to 2022. The collected data from the literature were analyzed and presented by location to determine high-performing genotypes, optimal planting dates, and other adequate cropping practices affecting quinoa performance and yield. Results showed that quinoa could be successfully cultivated in the new farming areas. Quinoa yields were higher than those reported in its place of origin, ranging from 108 kg ha-1, obtained by KU-2 in Washington State, to 9667 kg ha-1, obtained by Longli in China. Although quinoa is considered a crop with low input requirements, positive grain yield response was observed following increasing fertilization rates. Quinoa needs 2 to 4.6 kg of nitrogen to produce 1q of grain yield. In terms of phosphorus and potassium, quinoa needs 3.7 kg P2O5 and 4.3 kg K2O to produce 1 ton of total biomass. Quinoa has low water requirements (300-400 mm). However, a positive response was recorded with water quantities up to 866 mm. During our investigation, weed control in quinoa crop is still undeveloped and usually done manually. Research addressing this issue can increase quinoa yields and decrease the production cost. Downey mildew and birds’ attack are the major phytosanitary problems affecting quinoa grain yield. Other pests such as miners and aphids can also affect the health of quinoa, but their injury is not a serious problem. After the harvest, saponins found in the out layer of the seed can be removed through washing and mechanical pearling process, but the latter technic was found to be efficient and cost effective to reduce the saponin content. Our results constitute the first recommendation base for the adequate worldwide agronomic practices of quinoa crop.
Quinoa is originated from the Andean region of South America (Adolf et al., 2013). This crop has received much attention because of its high nutritional value and its high tolerance to frost, drought, and salinity (Jensen et al., 2000; Jacobsen, 2003; Jacobsen et al., 2005; Adolf et al., 2013; Hlásná Cepková et al., 2022; Shafiq et al., 2022; Terletskaya et al., 2022). Besides the human consumption, quinoa could be used as a fodder crop of high quality in adverse environments (Liu et al., 2020). Recently, quinoa cultivation has expanded beyond the Andes to several countries across the world (Bazile et al., 2016). However, Bolivia and Peru are still the largest quinoa producers with a share of 80% (Bazile et al., 2015; Alandia et al., 2020). Knowledge on quinoa production techniques and its response to different environments are necessary to adapt this crop to new farming areas.
The diversified genetic pool of quinoa allowed its cultivation possible from sea level to more than 4000 m above sea level, from 2° North latitude to 40° South latitude, in alkaline soils up to pH 9 as well as in acidic soils up to pH 4.5 (Mujica et al., 2001; Jacobsen, 2003). Quinoa thrives when the temperature is between 10-25°C with an optimum growth when temperature ranges from 15-20°C (Mujica et al., 2001). However, quinoa withstands temperatures up to -8°C, especially at branching stage, but temperatures above 38°C cause flower abortion and pollen infertility (Mujica et al., 2001). In Australia, quinoa yield decrease was associated with temperatures above 17°C during the grain filling as well as with temperatures below 15°C during the flowering stage (Snowball et al., 2022). Regarding the relative humidity, quinoa grows normally from 40% to 100% relative humidity (Tapia, 2001).
Until the new millennium, most of the scientific studies on quinoa were carried out in the countries of origin and reported as written materials (Sellami et al., 2020). To our knowledge, review papers of the response of quinoa to different agronomic practices and environmental conditions are unavailable. With the expansion of quinoa cultivation to Africa, Europe, Asia, and North America (Alandia et al., 2020), there was a need to a systematic review on the cropping practices and strategies to increase yields in the new production areas. After 2000, the scientific production of agronomic techniques started to increase with a peak during 2019 (Sellami et al., 2020). Most of these studies were concentrated in Europe, followed by Africa, and Asia whereas few studies has been reported from the Australian continent. Quinoa yield ranged from 11 kg ha-1 in North America to 11,000 kg ha-1 in Asia (Sellami et al., 2020). The low yields recorded in North America reflect the poor adaptation of quinoa crop and the limited experiments and knowledge about the management practices in this continent.
To increase the productivity of quinoa, it is important to identify suitable cultivation guidelines for each region. In general, crop yields are the result of genetic and environment interactions (Kaya and Akcura, 2014). In some cases, genotypes screened for high yields and quality at one site occasionally don’t perform well at another (Murphy and Matanguihan, 2015). Therefore, it is important to identify adapted quinoa genotypes that give high yields in various environments. Optimal planting date is also an important agronomic practice because it helps adjusting quinoa phenology to optimal environmental conditions, and enhances plant development and yields (Taaime et al., 2022). For this reason, adequate planting dates must be determined for each region, considering the rainy season, the plant growing cycle length, and frost and heat periods (Murphy and Matanguihan, 2015). In the context of climate change, sustainable irrigation strategies are also crucial to optimize water use, especially in arid and semi-arid regions. Other agronomic aspects of high importance, such as quinoa fertilization, planting density, pests and diseases, and postharvest techniques can highly affect quinoa production.
Quinoa’s ability to produce grains with high protein content and quality under extreme environmental conditions makes it a promising crop for the diversification of agricultural systems in different countries worldwide (Bhargava et al., 2007). Reviewing appropriate agronomic practices for each region will help disseminate the information among farmers and industries and increase quinoa production to meet the increasing demand in the market. For this reason, we conducted a systematic review of field studies available in the literature to identify, gather, and optimize quinoa agronomic practices worldwide.
A systematic review was conducted across Scopus Database to identify research related to the agronomic management practices of quinoa production worldwide. The studies selected were published between 2000 and 2022 in peer-reviewed journals written in English, Chinese and Spanish. The identification of scientific published papers was performed on 7 August 2022. Quinoa was the key word used to search the scientific papers with the limitation to “Agricultural and Biological Sciences” subject area. PRISMA was the methodology followed to screen the papers reviewed in this study (Figure 1). This methodology consisted of evaluating the title of the article. After judging that the title was related to agronomic practices of quinoa, we studied the abstract then the full article to screen only experiments conducted under field conditions. The process of article selection resulted in 148 chosen papers from which the data (quinoa yield variation according to cultivars, planting dates, planting structure, irrigation quantities, fertilization, and organic amendment) was extracted, analyzed, and represented in graphs and tables. Literature on soil tillage, planting methods, weed control, quinoa pests, diseases, and harvest and post-harvest techniques was selected for traditional literature review.
Quantitative data were collected from the selected papers and organized in Excel table. Table lines corresponded to the total screened articles. From each article, quinoa grain yield and corresponding cultivar, planting date, plant and row spacing, irrigation quantities, and fertilizers and organic amendments’ rates were represented in the table columns. To reduce the effect of environmental variability, we calculated the yield means of quinoa cultivars in each country and represented them in histograms. Box plots of quinoa yield variation in response to planting dates in each country were performed using R software. The other agronomic practices: soil tillage, planting methods, weed control, pests and diseases, harvest and postharvest were reviewed, summarized, and discussed.
The high genetic diversity of quinoa makes its cultivation possible under different environmental conditions. Quinoa genotypes are adapted to a wide range of soils with different pH varying from 4.7 to 9.7. This crop tolerates extreme temperatures from -8°C to 38°C in certain phenological stages and it is planted from sea level to about 4000 m above sea level (Mujica et al., 2001). Our evaluation focused on screening genotypes from the reviewed papers and assessing their performance in different parts of the world. In order to reduce the variability, we analyzed quinoa yields according to cultivars and locations and results were presented by country and continent. Quinoa grain yields ranged from 108 kg ha-1, obtained with KU-2 cultivar in Washington State, North America to 9667 kg ha-1, obtained with Longli cultivar in China, Asia. It was found that during the last twenty years, quinoa gained more interest in the African continent where high genetic diversity was evaluated, especially in Morocco and Egypt (Figure 2). Titicaca, Puno, Q5, and DO708 were the cultivars most evaluated in Morocco with 14, 13, 20 and 20 replications, respectively. SW2 and Atlas were also well adapted to Moroccan conditions, with 7830 and 6370 kg ha-1 of grain yield. However, these two cultivars were represented once in the literature, and we cannot rely on the obtained high yields for further recommendations. Evaluation of these cultivars in other regions of Morocco is important to test their yield stability in different environments. Pasankalla cultivar was less adapted to Moroccan conditions but performed very well in Burkina Faso reaching 1070 kg ha-1 of grain yield. However, quinoa yields in Burkina Faso are still low compared to Morocco and more research are needed to explore the genetic variability and find adapted cultivars to this country. Quinoa seemed to be well adapted to Egypt conditions and yields varied from 932 kg ha-1 with Q1 to 3287 kg ha-1 with CICA. Publications on quinoa in Zimbabwe and Kenya were scarce and only one cultivar, Cherry vanilla, was evaluated in Zimbabwe with an average yield of 533 kg ha-1, and two quinoa cultivars were evaluated in Kenya, Nairobi L and Nairobi S, with an average yield of 2097 and 1583 kg ha-1, respectively. Yields in Rwanda were low reaching only 960 kg ha-1 with the high performing cultivar Kaslaea. Brightest B.R. was the cultivar most adapted to Malawi environment and produced an average yield of 2214 kg ha-1.
Figure 2 Performance of quinoa cultivars in Africa (A) Morocco, (B) Burkina Faso, (C) Egypt, (D) Zimbabwe and Kenya, (E) Rwanda, (F) Malawi.
Quinoa was well adapted to the environmental conditions of different countries in Europe (Figure 3). Most of the research were conducted in Denmark and Turkey and quinoa yields were above the average found in its area of origin. Titicaca, Puno, and Pasto were adapted to the diverse environmental conditions in Belgium, Germany, Greece, Italy, Spain, Turkey, and Denmark. The highest and lowest quinoa yields, 3200 and 200 kg ha-1, were obtained in Italy by Regalona and DISPAA-Q47-CB, respectively.
According to the reviewed literature, quinoa cultivation was restricted to some parts of North America such as Washington and Utah states and Canada (Figure 4). Five genotypes were reported in the published literature and yields were low than those obtained in the place of origin. The highest and lowest yields, 563 kg ha-1 and 108 kg ha-1, were obtained in Washington State with Cherry Vanilla and KU-2, respectively.
In South America, only local varieties were cultivated, and the highest yield was recorded in Argentina by 2-Want cultivar (Figure 5). Narino cultivar showed high performance in Colombia with a grain yield of 4317 kg ha-1. On the other hand, Real Blanca seemed to be less adapted to the environmental conditions of Bolivia with a grain yield of 380 kg ha-1.
The cultivation of quinoa has extended to different countries in Asia (Figure 6). In China, the evaluation of the studied genotypes showed that Longli was highly adapted to the country conditions and recorded a high average yield of 9667 kg ha-1. Yields in India and U.A.E were also high with IHBT/Ac-1, IHBT/Ac-2, Q5 reaching 5750, 5950, and 5384 kg ha-1, respectively. However, yields were lower in Pakistan, Syria, Uzbekistan and Vietnam ranging from 944 kg ha-1 to 2538 kg ha-1. Drought, heat stress, and salt-affected soils in these regions may have negatively affected quinoa grain yield (Abbas et al., 2023).
Planting date is an important agronomic practice that helps adjusting the favorable environment for the cultivation of quinoa. The first climatic factor that affects quinoa yield and quality is temperature (Gómez et al., 2021; Stoleru et al., 2022). This parameter is estimated to increase from between 1.5°C to 6°C to the end of the XXI century (IPCC, 2018). Thus, optimal planting date should be identified to escape the heat stress, especially during the most sensitive development stages of quinoa (Flowering and seed filling) (Dao et al., 2020; Garcia-Parra et al., 2020). This was recorded in Argentina where delay in planting date from October to November exposed quinoa to higher temperature and affected the grain yield. In a study conducted by Gómez et al. (2021) in Argentina, 20°C was considered the threshold above which seed weight was negatively affected.
Optimal planting date should also allow enough time to the plant for its growth and development (Taaime et al., 2022). Under the agro-climatic conditions of Pakistan, planting in mid-November allowed long development period and gave higher biological and grain yield than planting earlier or later (Hamza et al., 2021). During this planting date, favorable temperature coincided with the grain filling stage and resulted in high yield.
Great attention should also be paid to the quinoa cultivar growing cycle length. It was found that quinoa long cycle cultivars are more susceptible to heat stress because of their long cycle. Planting these cultivars between November-December and October-December is suitable for quinoa short cycle varieties in the Soudano-Sahelian and Soudanian zones, respectively (Dao et al., 2020).
High temperature also affects quinoa at the early development stages. It was found that the suitable planting date of quinoa in Greece is early March and late sowing coincides with high temperature, which dried the soil after each irrigation and affected the germination rate (Noulas et al., 2017). In Brazil, autumn–winter was optimal for planting quinoa, due to optimal temperature and range between night and day values, which increased grain yield and large seed size (Spehar and da Silva Rocha, 2010).
In addition, water is another important factor that conditions quinoa growth and yield. For instance, April planting of quinoa when grown as a leafy green in Poland produced greater biomass and chlorophyll a + b than August planting, due to the availability of water (Adamczewska-Sowińska et al., 2021). In another study, planting during the first week of April month received the highest precipitations and represented the appropriate time for a successful quinoa cultivation in Turkey-Mardin ecology condition (Altuner, 2019).
In other regions prone to frost, planting quinoa should be done in order to avoid the cold conditions occurring at late growing stages of quinoa. In fact, early planting of quinoa (before August) enhanced plant height and total biomass and helped escaping the frost damage in Midwestern China (Liu et al., 2020). In another study carried out in the northern foothills of Yinshan Mountains, China, the optimum sowing time of quinoa was from mid-April to early May (Ren et al., 2018). Similarly in Canada, mid-May to mid-June planting was suitable for the cultivation of quinoa (Nurse et al., 2016).
Besides the environmental factors, weed infestation is an important biotic stress to take into consideration while planting quinoa. Under Mediterranean climate conditions (Turkey), the adequate time to sow quinoa is April. The increasing temperature of late sowing exposed quinoa to high infestation of weed during the plant establishment, decreased the crop population and subsequently the grain yield (Zulkadir, 2020). In Morocco, seed and dry matter yields were highest when quinoa was sown in November and early December (Hirich et al., 2014a). Planting in these dates helped avoiding weed infestation and downy mildew occurring with late quinoa planting dates. Similar results were found in England whereas late sowing results in high weed competition and sowing in March was adapted to this region (Risi and Galwey, 1991).
Quinoa sensitivity to photoperiod has been studied by several authors. It is well knowledge that quinoa genotypes cultivated in the tropics have longer vegetative phases and are more photoperiod sensitive than genotypes grown by the sea and on the Andean altiplano (Jacobsen, 2003).
We analyzed the collected quinoa yields from the selected literature according to planting dates and locations (Figure 7). Switching from spring to winter cultivation can be an essential criterion for the success of quinoa cultivation (Maamri et al., 2022). In Egypt, quinoa was sown in the rainy season between October and December, with optimal date occurring in November. In Kenya, two planting dates were evaluated and results showed that March planting gave higher yield than October planting with 2097 kg ha-1. Planting during the cool dry season in Malawi (May) gave higher yield than planting during the hot rainy season (December), probably due to the effect of temperature on quinoa development and yield. In Morocco, quinoa was planted in different dates from October to April. The highest average grain yield was November planting with 3050 kg ha-1. In Zimbabwe, planting in May produced a grain yield three time higher than planting in January.
Figure 7 Worldwide quinoa grain yield variation according to sowing dates (A) Africa; (B) North America; (C) Asia; (D) Europe; (E) South America.
There was no big difference between planting dates in Europe with planting in April gave the highest grain yield in Belgium, Denmark, Germany, Greece, Italy, Spain, and Turkey. In North America, the analysis of yields showed that planting in May was suitable for the cultivation of quinoa in Canada and Utah State whereas planting quinoa during April in Washington State gave higher yields.
In the South America, planting in October was suitable for the cultivation of quinoa with a grain yield of 2786, 1416, 1883 and 1850 kg ha-1 in Argentina, Bolivia, Chile, and Peru, respectively. However, Planting in December gave the highest yield in Brazil with an average of 1750 kg ha-1. Analysis of quinoa yields in Asia showed that January was the optimal date for planting this crop in India, Iraq, Israel, and Syria. Regarding other countries, quinoa gave high yields when planted during October in Iran and Oman, during November in Pakistan, and U.A.E, and during April in China, Japan, and Uzbekistan.
Quinoa grows well in loamy soils, with good drainage and high content of organic matter (Mujica et al., 2001). Quinoa can also adapt to different soils, but it is very susceptible to water logging and to excess humidity, especially in the first development stages (Mujica et al., 2001). In general, deep ploughing using moldboard or disc plow was used to prepare the soil for deep root system development. Before planting, a second tillage is recommended to prepare the seedbed.
Recent work comparing two tillage systems showed that the minimum tillage, chiseling at 25 cm depth followed by chiseling at 10-15 cm, gave lower grain yield than the conventional tillage where moldboard plowing at 20-25 cm followed by one rotary hoeing at 10-15 cm were used. This yield difference was essentially due to enhanced crop growth parameters (Kakabouki et al., 2019).
Table 1 represents the different soil tillage techniques used by researchers according to soil texture.
Row and plant spacing of quinoa crop were collected from the selected published literature and represented in Table 2. Results showed that quinoa was cultivated around the world in different crop structures. Row spacing varied between 15 and 100 cm and plant spacing varied between 2 and 60 cm. High row spacing was more suitable for mechanical weeding. The highest average quinoa yield, 5122 kg ha-1, was obtained with 35 row to row spacing and 20 cm plant to plant spacing. However, 38% of the studied cases have evaluated quinoa cultivation with 50 cm row to row spacing.
Optimal planting rate varies according to climatic conditions and soil fertility (Asik et al., 2020). In China, quinoa grain yield increased by 27% with a planting density varying from 20 to 40 plants m-2 (Wang et al., 2021). Plant densities in Colombia were lower, with quinoa grain yield decreasing by 38% from 7 to 16 plant m-2 (Diaz et al., 2021). In another study conducted in Zimbabwe, quinoa grain yield increased from a plant density of 3 plants m-2 to 6 plants m-2 then decreased with higher densities (Parwada et al., 2020). The plant density of 8 plants m-2 seemed to be the optimal density for quinoa cultivation under Vietnam conditions (Minh et al., 2020).
Quinoa was planted in the peer-reviewed papers by different methods. Hand sowing in holes of 3 to 10 seeds per hole was the first reported method (Deng et al., 2021; González et al., 2021; Hirich et al., 2021). Mechanical sowing was also practiced for large areas in order to reduce the amount of labor and time needed for this operation (Matías et al., 2021). Quinoa was also planted in ridges, probably to increase the water infiltration, reduce runoff and hasten the plant growth (Hamza et al., 2021; Mansouri et al., 2021). Another method is to grow quinoa plants in the nursery during the first development stages and transplant it in the field when the climatic and soil conditions become suitable for its cultivation (Ludvigson et al., 2019).
Water is one of the major limiting factors for crop production, especially in arid and semi-arid regions. Cultivating drought tolerant crops, such as quinoa is important to valorize marginal soils and increase agricultural productivity. Quinoa water needs for optimal development and yield are low and estimated to 300-400 mm (Fghire et al., 2012). The response of quinoa to deficit irrigation was evaluated in Egypt using 100% ETc, 85% ETc and 65% ETc (Badran, 2022). Results showed that the highest yields, 1680, 1846, 1691, 1811 and 1800 kg ha-1 were obtained under full irrigation (303 mm) with the cultivars L12, L14, Q3, Q5 and Chipaya, respectively. Applying 65% of ETc (197mm) resulted in a yield decrease of the same cultivars by 45, 36, 43, 40 and 33%, respectively. Quinoa gave higher yield (3993 kg ha-1) in Morocco when receiving 356 mm of irrigation water (Fghire et al., 2021). Applying 50% ETc (140 mm) and 33% ETc (93 mm) reduced quinoa yields to 2500 and 1138 kg ha-1, respectively. In Chile, quinoa grain yield increased with higher water application levels recording 583, 1356, 1375, 1919, 2294, 2250, 3055, 3112 and 3286 kg ha-1 when receiving 25, 222, 280, 345, 369, 447, 505 and 537 mm, respectively (Pinto et al., 2021). In Iran, the average grain yield increased from 903 kg ha-1 with the application of 354 mm to 1142 kg ha-1 with 530 mm (Nadali et al., 2021). Higher water level (707 mm) resulted in a grain yield of 1409 kg ha-1. When quinoa was conducted under rainfed conditions in India, there was no big difference between receiving 530 or 658 mm of rainwater, with yields reaching 5928 and 5814 kg ha-1, respectively (Rathore and Kumar, 2021). In Israel, quinoa yielded 2893 and 4521 kg ha-1 with 364 and 420 mm of irrigation water, respectively (Asher et al., 2020).
Quinoa has high tolerance to water salinity up to 30 dS m-1 (Peyghan et al., 2020). In this experiment, quinoa reached 3293, 3515 and 5360 kg ha-1 with the application of 139, 208, and 278 mm. In Vietnam, quinoa recorded 1761 kg ha-1 when the crop has received 866 mm (Minh et al., 2020). According to Salim and Hadeethi (2020), the average quinoa water requirement was estimated to be 231 mm. In Burkina Faso, quinoa grain yields were low and application of 246 and 410 mm resulted in similar yields, 635 and 631 kg ha-1, respectively (Alvar-Beltrán et al., 2019). In addition, application of 250 mm of water in Greece resulted in a grain yield of 2283 kg ha-1 (Kakabouki et al., 2019). Quinoa received higher amounts of water in an experiment conducted in Egypt with grain yields of 1480, 1855 and 2071 kg ha-1 under 237, 550 and 820 mm irrigation water (Al-Naggar et al., 2017).
Quinoa exhibits high tolerance to water stress during the vegetative growth, followed by flowering stage but a substantial loss of yield was noticed if the stress occurs during the seed filling (Hirich et al., 2012; Lavini et al., 2014). Water stress in the initial period can significantly increase the tolerance to drought during later phases (Oudou et al., 2019).
The drought tolerance of quinoa is attributed to morphological adaptations such as reducing leaf area and increasing root growth (Hirich et al., 2012; Lavini et al., 2014). Physiological adaptations are also noticed. Quinoa increases its antioxidant activity to cope with the reactive oxygen species (ROS) generated by water stress. The activity of the antioxidant enzyme superoxide dismutase (SOD) increased by 90% and 322.42% at 33% ETc and under rainfed conditions, respectively. The catalase (CAT) activity increased significantly by 87.4% under rainfed conditions when compared to full irrigation (Fghire et al., 2013). The plant also decreased its Leaf water potential, closed its stomata, increased the chlorophyll a and b content and had a great ability to repair them for rapid restoration of photosynthetic functions during post stress recovery (Oudou et al., 2019; Maestro-Gaitán et al., 2022). Anatomically, the plant increased the number of its stomata under stressed conditions in order to allow better control of transpiration (Oudou et al., 2019).
Nitrogen (N) deficient quinoa plants exhibit pale green or yellow leaf color, small inflorescence, and grains with low protein content (Pando and Castellanos, 2016). Because of its structural role in the chlorophyll as well as in nucleic and amino acids composition, insufficient quantities of N result in very slow growth, stunted plant, and light green to yellow foliage color (Benton Jones, 2012).
For an optimum yield, quinoa internal N requirement, based on N content of total biomass at harvest, was estimated to 1.015% (Berti et al., 2000). In another study, quinoa absorbed approximately 2 kg N to produce 1 q of grain (Deza, 2018). In Germany, Schulte auf’m Erley et al. (2005) declared that quinoa absorbed 4.6 kg N ha-1 to produce 1 q of grain.
Quinoa can grow in soils with low fertility and is considered as a crop with low input requirement. Under moderate temperate condition of Denmark, a slight increase of quinoa yield of 24.1% was achieved when the N supply increased from 40 to 160 kg N ha-1 and an increase of only 2.7% from 120 to 160 kg N ha-1 (Jacobsen et al., 1994). In a silty-clay loam soil under Mediterranean climatic conditions, N at 150 kg ha-1 was proved to be the best level for N supplementation for grain yield of 2950 kg ha-1, being increased by 357% compared to the control (Geren, 2015). Related results were obtained by Almadini et al. (2019) when evaluating the response of quinoa to N fertilization levels from 0 to 160 kg N ha-1. N at 160 kg ha-1 enhanced vegetative growth and increased yield by 759.6% compared to the control. Schulte auf’m Erley et al. (2005) indicated that grain yield of quinoa (3495 kg ha-1) was enhanced by 194% at the highest N rate (120 kg ha-1). In Burkina Faso, application of 100 kg N ha-1 increased quinoa grain yield by 37%, reaching 1380 kg ha-1 (Abdolahpour et al., 2021). In Iran, 100 kg N ha-1 seemed to be the optimal N rate producing 2790 kg ha-1 (Keshtkar et al., 2022). Under arid conditions in Syria, N fertilization at 90 kg N ha-1 resulted in the highest grain yield (5320 kg ha-1) whereas higher N rates decreased quinoa grain yield. In Iraq, the grain yield of quinoa increased with N application rates up 120 kg N ha-1 and decreased with higher N rates (Salim et al., 2019).
High fertilization rates are recommended with high potential cultivars and optimal environmental conditions. In fact, water availability increases quinoa nitrogen uptake (Wang et al., 2020). According to Berti et al. (2000), quinoa grain yield increased with N fertilization up to 225 kg ha-1 producing 2268 and 3555 kg ha-1 of grain yield with the cultivars FARO and UDEC10, respectively. Shams (2011) also tested the response of quinoa to higher N rates in sandy soils. He found that maximum economic yield 1145 kg ha-1, was achieved by application of 360 kg ha-1, being almost eleven time over control. In Greece, 200 kg N ha-1 increased quinoa grain yield with only 14% (Kakabouki et al., 2019). In Denmark, quinoa responded positively to the highest N application rate (180 kg N ha-1) with a yield increase of 25%.
Because of its high mobility in the soil, N should be split to ensure high utilization efficiency. Partitioning of nitrogen fertilization depends mainly on soil type and climatic conditions. N application time differed in the reviewed papers. In Argentina, 20% of N rate was applied before planting and 80% at 30-35 days after emergence (Mez MB et al., 2011; Curti et al., 2014). Similarly in Baghdad, N was split into two equal rates and applied before planting and at branching stage (Salim et al., 2019). In Brazil, 40% of total N was applied before planting and 60% at 40 days after emergence (Spehar and da Silva Rocha, 2010).
Generally, N fertilization affected negatively the N use efficiency (NUE) and the application of N rates higher than 100 kg N ha-1 did not increase the NUE (Kakabouki et al., 2019). The highest NUE of 13.92 kg kg-1 was recorded with the lowest N rate 75 kg ha-1 (Berti et al., 2000). In agreement with that, Almadini et al. (2019) reported that N fertilization and NUE have an inverse relationship, with highest value recorded at 80 kg N ha-1. Related results were found by Shams (2011) where application of 90 kg N ha-1 corresponded to maximum NUE. This parameter also differs among quinoa cultivars. Deza (2018) recorded that LM 89-77 genotype had the highest NUE with 46.02 kg of grain per kg of applied N.
Unlike N, studies evaluating phosphorus (P) nutrition of quinoa were scarce. Phosphorus deficiency was manifested by dark green or red tips of leaves (Pando and Castellanos, 2016). It reduces plant height, delayed flowering and ripening with small and twisted inflorescences and very small or poorly developed grains (Pando and Castellanos, 2016). P deficient quinoa plants display necrosis of the lower leaves, and the upper leaves became pale green (Sales et al., 2021).
The evaluation of quinoa P requirements showed that quinoa needs 3.7 kg P2O5 to produce 1 ton of biomass (Alvar-Beltrán et al., 2019). The high demand of this crop in P requires an adequate fertilization to attain high yields. Llaca Ninaja (2014) studied the effect of four P rates 0, 40, 80 and 120 kg P2O5 ha-1 and found that 88 kg P2O5 ha-1 was the optimal level to obtain 2890 kg ha-1. Higher P rates reduced quinoa yield. In another study, increasing P rate from 90 to 180 kg P2O5 ha-1 did not significantly affect growth characteristics and yield of quinoa, with an average of 4101 kg ha-1 (Quispe and León, 2018).
Plants need P in early stages of development to build good root system. For this reason, P was applied at sowing in all studied cases, except when the P was applied with water in the irrigation system.
Potassium (K) deficiency is manifested by poor root system and weak stems (Pando and Castellanos, 2016). It is also found that this deficiency is observed with chlorosis at the margins of older leaves followed by necrosis (Sales et al., 2021).
In terms of K requirements, quinoa needs 4.3 kg K2O to produce 1 q of quinoa biomass (Alvar-Beltrán et al., 2019). This indicated how much quinoa is demanding in K and adequate fertilization is necessary to fulfill the crop needs and enhance quinoa yields. Despite the importance of this nutrient, research studying the response of quinoa to K was scarce and optimal fertilization program should be established for this crop.
Quinoa strongly responded to organic fertilizers. The amendment did not only improve yields, but also enhanced soil structure and fertility (Mujica et al., 2001). The combination of three types of biofertilizers (Manure at 36 t ha-1; biol at 600 l ha-1 and nitrifying bacteria at 108 UFC) ameliorated vegetative growth of quinoa and resulted in high yield of 2445 kg ha-1, being 7 times over the control (Neyra, 2014).
Organic matter also acts as water retaining agent, facilitates the dissolution and the absorption of other applied minerals and thus enhances the quality of the seeds (proteins, carbohydrates, and fats content) (Garcia-Parra et al., 2018). The application of organic fertilizers (4 q ha-1) along with mineral fertilizers (45-45-45 kg ha-1 NPK) resulted in the highest yield (2280 kg ha-1) (García-Parra et al., 2017). This is due to maintaining the balance between organic and chemical fertilizers and increasing the availability of nutrients throughout the productive cycle of the plant.
Organic matter enhances the water holding capacity of the soil, improves its structure, facilitates the soil aeration and favor the development of microbial flora for a prompt humification (Mujica and Jacobsen, 1999). Osco Limachi (2009) points out that increasing levels of sheep manure up to 12 t ha-1 favored the growth and development of the plant and improved the physical characteristics of the soil by avoiding its compaction and maintaining its humidity. However, 8 t ha-1 of sheep manure was considered the optimal rate for obtaining an economic yield of 2300 kg ha-1.
We summarized in Table 3 all the reviewed research papers evaluating the effect of organic amendment on quinoa grain yield, and we presented them according to locations. In Iran, quinoa responded positively to the application of organic manure with yield increasing by 23% compared to the control. Some organic amendments slightly decreased quinoa grain yield. This may be due to field heterogeneity or nitrogen immobilization by soil organisms, which make nitrogen unavailable to crops. Thus, adequate amendment management is important for enhancing soil quality and increasing crop yields. In addition, the phosphogypsum was 7tested as an amendment and its effect on quinoa grain yield was highly significant, with an increase from 1940 kg ha-1 with no application to 2670 kg ha-1 with 9 t ha-1 of phosphogypsum. Different studies evaluating manure and compost effect on quinoa growth and yield were found. The highest yield was recorded with the application of 10 t ha-1 of compost. In addition, the vermicompost highly affected quinoa grain yield in India and applying 5 t ha-1 increased yield by 28%.
Weed management in quinoa cultivation is the most difficult operation, because at present, it is almost done manually. The absence of chemical treatments makes the weeding operation expensive and represents a large part of quinoa production costs. The development of weed control techniques and the identification of adequate herbicides is a necessity for the development of the cultivation techniques of this crop. In the reviewed literature, weeds were controlled by hand or using manual tools. The frequency of these operations differed from once a week to once during the growing season (Table 4).
Applying herbicides during the previous cropping season to the cultivation of quinoa may also cause harmful effects on the seedling. Results showed that applying Imazaquin had negative effect on quinoa seedling even after 206 days of its application, followed by clomazone applied before 15-38 days. However, trifluralin and pendimethalin had no residual effect (Santos et al., 2003).
Several insects were reported to be associated with quinoa crop in the Andes, some of them can highly reduce the yield and result in considerable economic losses. On the other hand, several natural enemies were also observed in quinoa, and they can be used in the biological control of this crop (Yabar et al., 2002). Quinoa shoots were subjected to sapsuckers (Aphididae, Cicadellidae), leaf miners (Agromyzidae), and chewing insects attack (Yabar et al., 2002). Studies showed that the insect attacks were abundant in the early season of quinoa cultivation (Yabar et al., 2002). In the Andes, the temporal dynamic of Aphididae population recorded two peaks, at the beginning and at the end of the growing season (Yabar et al., 2002). The low abundance of this enemy in the mid-season was probably associated with the peak of Coccinellidae population, which resemble the traditional predator–prey dynamics (Hassell, 1978). Braconidae and chrysopid larvae were also reported to be the enemy of aphids (Mackauer and Volkl, 1993; Cruces et al., 2020). Chemical control of aphids using methomyl + dimethoate was efficient to control the Aphididae (Cruces et al., 2020).
During the process of quinoa adaptation to the environmental conditions of Europe, it was attacked by several insects native to this continent (Sigsgaard et al., 2008). Heavy attacks of Scrobipalpa atriplicella in the maturing inflorescence of quinoa and Cassida nebulosa in Denmark were reported as serious problems (Sigsgaard et al., 2008). In the Mediterranean basin, Nysius cymoides constitutes a polyphagous specie that sucks plant sap and may cause high economic losses (Rivnay, 1962). This bug attacks the inflorescence and pods when seeds are in the milky stage (Rivnay, 1962).
Quinoa moth, Eurysacca melanocampta, was detected in Argentina, Bolivia and Peru (Rasmussen et al., 2003; Cruces et al., 2020). The economic threshold level was found to be three to 15 larvae per plant (Villanueva, 1978; Blanco, 1994). In higher infestation levels, this insect was controlled with emamectin benzoate + methomyl, leading to its suppression in the field. The biological management using sprays of Bacillus thuringiensis var. kurstaki to control low population of this insect was less effective (Cruces et al., 2020). The caterpillars infested the plants 43 days after planting.
Birds attack is a serious problem in quinoa cultivation and can cause potential damage to the crop with losses up to 60% (Rasmussen et al., 2003). In the Peruvian highlands, Patagioenas maculosa, Zenaida auriculata, Sicalis uropygialis, and Zonorrichia capensis are the major observed bird species and they attack quinoa seeds from the milky stage to physiological maturity (Loza et al., 2016). Birds attack quinoa in the early morning, in the mid-day and in the afternoon (Loza et al., 2016).
Concerning quinoa diseases, downy mildew, caused by Peronospora farinose, is the most damaging disease of quinoa and may reduce yield by 20 to 99% (Alandia et al., 1979; Danielsen and Munk, 2004). This disease has been reported in all areas of quinoa cultivation, and it causes chlorotic lesions on the upper leaf surface and grays spores’ development on the lower leaf surface, an often leading to early defoliation (Danielsen et al., 2002; Choi et al., 2014). These lesions may turn to reddish and purple in some cultivars and may develop to small necrotic flecks in hypersensitive cultivars (Danielsen et al., 2003). Downey mildew is seed transmitted and the presence of free water and RH>85% are optimal conditions for the development of the causal pathogen of downy mildew (Danielsen et al., 2003; Danielsen et al., 2004). To control this disease, metalaxyl fungicide are used as the traditional control method. However, attention should be paid to the environmental and human risks and to the possibility of resistance development by this pathogen (Danielsen et al., 2003).
Quinoa Anthracnose caused by Colletotrichum nigrum and C. truncatum were observed in the United State in 2009. This disease causes stem bleach to dark lesions, oval to linear with slightly tapered tips and may cause stem lodging (Pal and Testen, 2021). Other quinoa diseases, probably of minor importance as quinoa production constraints, were reported around the world. These reports include: Rhizoctonia damping off and Fusarium wilt in Peru and Italy, seed rot and damping off in the United State, damping off in Japan and leaf spot and brown stalk rot in the Andean region of South America (Bechman and Finch, 1980; Barboza et al., 2000; Ikeda and Ichitani, 1985; Boerema et al., 1977; Otazu and Salas, 1977; Beccari et al., 2021).
Leaf spot of quinoa, caused by Ascochyta hyalospora, is transmitted by the seed (Danielsen et al., 2003). The symptoms of this disease include circular spots with light color and light brown edges. The spots may develop to necrotic black pycnidia visible to the naked eyes (Boerema et al., 1977; Alandia et al., 1979). Quinoa may lose its foliage in high infestation cases (Danielsen et al., 2003). The geographic distribution of this disease is not known, and it is not considered a major constraint to quinoa production.
Brown Stalk Rot is another quinoa disease caused by Phoma exigua var. foveata and it was reported for the first time in Peru (Otazu and Salas, 1977). The symptoms of this disease include lesions in the stem and inflorescence, chlorosis in the leaves and the stem bends downward and become easily fracturable (Danielsen et al., 2003). This disease is transmitted to the crop from the soil when the plant is injured and it develops rapidly with low temperature and high humidity (Danielsen et al., 2003).
Seed Rot and Damping Off of quinoa caused by Sclerotium rolfsii and Pythium were reported for the first time in California. Plants affected by this disease display stem girdling, and collapse appeared in erratic areas in the field (Danielsen et al., 2003).
Leaf spot caused by Pseudomonas syringae, a pathogenic bacterium, was observed in Colombia in 2020 (Fonseca−Guerra et al., 2021). This disease causes considerable yield losses and induces the formation of apical necrosis and dark brown spots (Fonseca−Guerra et al., 2021). Lesions in the stem giving a vitreous appearance and smooth consistency were also reported (Fonseca−Guerra et al., 2021)
Nacobbus aberrans and Thecavermiculatus andinus are two parasitoid nematode that attack quinoa in the Andean region (Franco, 2003). The first enemy, N. aberrans, also referred to as False Root Knot nematode causes quinoa roots nodules that contained the females along with protruding gelatinous matrix containing the eggs (Franco, 2003). This pathogen can cause considerable yield loss of quinoa (Franco, 2003). The second nematode, T. andinus is commonly known as the “nematode of the oca” (Astocaza and Franco, 1983). It was observed surrounding Lake Titicaca and negatively affected vegetative development and quinoa grain yield (Franco, 2003).
Various postharvest operations, and the combination of several, have been developed to act on the quinoa seeds, to prepare them for processing procedures taking place at a later stage. This section aims to describe the main harvest and post-harvest operations and their influence potential on the quinoa quality as referred to universal standards. This assessment is based on the comparison of composition profile before and after the concerned operation procedure.
At harvest time, the leaves of quinoa plants turn to yellow or red depending on the variety (Garcia et al., 2015). It is crucial to determine the optimal harvest time of quinoa crop, because quinoa grains start to drop from the panicle when quinoa plants exceed maturity (Garcia et al., 2015). It is assumed that physiological maturity is attained when the seeds can be seen in the panicle through the opening of the perigonium (Aroni, 2005), or when the grains fall out when taping the panicle with the hand. Harvest can be done manually using a sickle and cutting the plant 10–15 cm above the soil (Garcia et al., 2015). However, it was found difficult to cut the quinoa plants because of their thick stems and pulling out the plants is preferred and commonly used by farmers. This method is labor consuming and high harvest losses are expected.
After the harvest, natural drying of quinoa plants can be done in the field and threshing can be done manually or mechanically (Salas, 2003). From a commercial requirement, additional ventilation can be carried out to reduce the grain moisture under 10% (Garcia et al., 2015).
Quinoa contains antinutritional compounds such as saponins (Mora-Ocación et al., 2022). They present a wide group of glycosides found in different plants and play an important role in protecting seeds from insect and fungal attack (Simmonds, 1965). They are normally found in a range of 0.1% to 5% (Chaudhary et al., 2023) and are located in out layer of the seed. These compounds confer to quinoa bitter taste but are easily removable through washing process as they are hydrosoluble. The procedure of washing quinoa grains hold not only hygienic importance, but also further ensures the reduction of saponin content and elimination of bitterness associated with saponins. Traditional process of washing involves the soaking of the quinoa seeds, which permits the water-soluble saponin to be washed away (Shi et al., 2009; Quispe-Fuentes et al., 2012). However, this procedure demands the use of significant amounts of water that make disposal of wastewater an issue, and may raise pollution concerns (Taylor and Parker, 2002; Repo-Carrasco et al., 2003; Rafik et al., 2021a). There is also high cost associated with subsequent drying of the product (Repo-Carrasco et al., 2003). Additionally, inefficiency in saponin removal by washing has also been mentioned (Quispe-Fuentes et al., 2012; Carrasco et al., 2021). However, improper technologies can cause poor grain germination, fungi and mold damage, and color and odor change. The combined method of abrasive dehulling and washing together has been suggested as an effective way to address the issues listed above while achieving efficient saponin removal, during which the quinoa grains are quickly burnished and briefly washed, to minimize the loss of nutrients, lower the cost of drying, and lower the saponin content in the wastewater (Repo-Carrasco et al., 2003; Rafik et al., 2021a).
The saponin of quinoa grains can be removed by mechanical pearling (Rafik et al., 2021b). Pearling, which is also referred to as debranning, has yet to be properly and specifically characterized for quinoa, but the technology has been developed to wheat kernels and other cereal grains in order to remove the outer layer through a frictional and abrasion force (D'Amico et al., 2019). It has been suggested that unsignificant change has been detected in the macronutrient content of the pearled grains, micronutrient alongside antioxidant capacity are altered significantly in quinoa grains after the pearling process depending on the extent of pearling (D'Amico et al., 2019; Jiang et al., 2021; Rafik et al., 2021a). One of which such change is the reduction of the bitter antinutritional compound saponin to an acceptable level of palatability and digestibility, that is achieved through mechanical pearling as compared to manual abrasion (Mhada et al., 2020; Jiang et al., 2021; Rafik et al., 2021a). It has been found that pearling quinoa at a degree of 30% reduced the saponin by 79% compared with the original whole quinoa (Gómez-Caravaca et al., 2014). Another study has stated that depending on the quinoa variety, the total saponin content has been reduced from a starting range of 0.37% to 0.57% to merely 0.07 to 0.1%, with a pearling duration of two minutes (Rafik et al., 2021b).
Quinoa is a promising alternative crop to cereals in arid and saline environments. It has gained considerable interest, especially from health-conscious consumers, because of its high nutritional value. The increasing demand for quinoa along with limited agricultural resources urges developing technologies to increase yield and productivity. The Andean region, especially Bolivia and Peru, is the center of origin of quinoa. However, quinoa has high genetic variability and plasticity, which make its cultivation possible in a large range of environments. Making quinoa more popular would require dissemination of information about the crop among farmers as well as consumers. The present study helps identifying adapted quinoa genotypes and assesses their performances in different countries around the world. Results showed that quinoa can be successfully cultivated in Africa, Europe, Asia, North American and Australia. Quinoa production techniques differed according to the area of its introduction. The sowing times markedly differ from one location to another, and the optimal date was determined in each region in order to escape harsh environmental conditions, especially drought, heat, and frost. Additionally, plant thinning is required to avoid intraspecific competition. If planted in rows, it is recommended that quinoa plants should be spaced by 20 cm with a row to row spacing of 35 cm. Early weeding (± 30 days after sowing) is important in quinoa fields in order to reduce plants competition for limited nutrients. Additional weeding associated with high level of weed infestation is recommended before quinoa canopy closure. Weeding is almost done manually, and further research is needed to develop adapted chemical techniques in order to optimize quinoa production. To ensure an adequate nutrient supply for quinoa, a good balance of nutrients in the soil should be maintained. Quinoa needs 2 to 4.6 kg of N to produce 1q of grain yield. In terms of P and K, quinoa needs 3.7 kg P2O5 and 4.3 kg K2O to produce 1 ton of total biomass. Quinoa has low water requirements, and it is a suitable crop for arid and semi-arid regions. However, drought tolerance is often translated to low yields because quinoa sacrifices yield in order to survive and adapt. Under this consideration, several research studied the impact of irrigation on quinoa performance and deficit irrigation is shown to be highly beneficial in various experimental location. Although quinoa can withstand harsh growth conditions, pests such as birds, insects, and several diseases caused by fungi, bacteria, nematodes, and viruses can cause significant yield losses. Quinoa is an interesting cash crop and had a great potential to enhance the livelihood of smallholder farmers in marginal areas. Our work represents the first review paper identifying the adequate agronomic practices for quinoa cultivation worldwide.
The raw data supporting the conclusions of this article will be made available by the authors, without undue reservation.
NT, MG, KM, RC-A, AO, and RB contributed to the conception and design of the manuscript. NT, SR, and RC-A collected and organized the data. NT and SR wrote the first draft of the manuscript. All authors contributed to the manuscript revision, read, and approved the submitted version.
The authors declare that the research was conducted in the absence of any commercial or financial relationships that could be construed as a potential conflict of interest.
All claims expressed in this article are solely those of the authors and do not necessarily represent those of their affiliated organizations, or those of the publisher, the editors and the reviewers. Any product that may be evaluated in this article, or claim that may be made by its manufacturer, is not guaranteed or endorsed by the publisher.
Abbas G., Areej F., Asad S. A., Saqib M., Anwar-ul-Haq M., Afzal S., et al. (2023). Differential effect of heat stress on drought and salt tolerance potential of quinoa genotypes: a physiological and biochemical investigation. Plants 12, 774. doi: 10.3390/plants12040774
Abdolahpour H., Nejad E. T., Pour A. P. (2021). Effect of nitrogen, phosphorus and potassium fertilizers on morpho-physiological characteristics and seed yield of quinoa (Chenopodium quinoa willd.). Res. Crop ecophysiol. 15 (1), 57–72. doi: 10.30495/jcep.2021.681006
Adamczewska-Sowińska K., Sowiński J., Jama-Rodzeńska A. (2021). The effect of sowing date and harvest time on leafy greens of quinoa (chenopodium quinoa willd.) yield and selected nutritional parameters. Agriculture 11, 405. doi: 10.3390/agriculture11050405
Adolf V. I., Jacobsen S.-E., Shabala S. (2013). Salt tolerance mechanisms in quinoa (chenopodium quinoa willd.). Environ. Exp. Bot. 92, 43–54. doi: 10.1016/j.envexpbot.2012.07.004
Alandia S., Otazu V., Salas B. (1979). “Enfermedades,” in Quinua y kaniwa. Eds. Tapia M., Gandarillas H., Alandia S., Cardozo A., Mujica A., Ortiz R. (Bogota Colombia: IICA).
Alandia G., Rodriguez J. P., Jacobsen S.-E., Bazile D., Condori B. (2020). Global expansion of quinoa and challenges for the Andean region. Glob. Food Sec. 26, 100429. doi: 10.1016/j.gfs.2020.100429
Almadini A. M., Badran A. E., Algosaibi A. M. (2019). Evaluation of efficiency and response of quinoa plant to nitrogen fertilization levels. Middle East J. Appl. Sci. 9 (4), 839–849. doi: 10.36632/mejas/2019.9.4.1
Al-Naggar A. M. M., Abd El-Salam R. M., El-Sayed Badran A. E., El-Moghazi M. M. A. F. (2017). Effects of genotype and drought stress on some agronomic and yield traits of quinoa (Chenopodium quinoa willd.). Biomed. Res. 14 (4), 1080–1090.
Altuner F. (2019). The impact of different sowing-times of the quinoa (chenopodium quinoa willd.) and its varieties on the yield and yield components in Turkey-mardin ecology condition. Appl. Ecol. Environ. Res. 17, 10105–10117. doi: 10.15666/aeer/1704_1010510117
Alvar-Beltrán J., Dalla Marta A., Vivoli R., Verdi L., Orlandini S. (2022). Greenhouse gas emissions and yield production from an organic and conventional fertilization on quinoa. Agronomy 12, 1015. doi: 10.3390/agronomy12051015
Alvar-Beltrán J., Dao A., Marta A. D., Saturnin C, Casini P., Sanou J., et al. (2019). Effect of drought, nitrogen fertilization, temperature, and photoperiodicity on quinoa plant growth and development in the sahel. Agronomy 9, 607. doi: 10.3390/agronomy9100607
Alvar-Beltrán J., Napoli M., Dao A., Ouattara A., Verdi L., Orlandini S., et al. (2021). Nitrogen, phosphorus and potassium mass balances in an irrigated quinoa field. Ital. J. Agron. 16, 1788. doi: 10.4081/ija.2021.1788
Aroni J. C. (2005). “Cosecha y poscosecha,” in Serie de módulos publicados en sistemas de producción sostenible en el cultivo de la quinoa. Eds. Proinpa, Fautapo (La Paz, Bolivia: Fundación Proinpa, Fundación Autapo, Embajada Real de los Países Bajos), 87–102.
Asher A., Galili S., Whitney T., Rubinovich L. (2020). The potential of quinoa (chenopodium quinoa) cultivation in Israel as a dual-purpose crop for grain production and livestock feed. Sci. Hortic. 272, 109534. doi: 10.1016/j.scienta.2020.109534
Asik B. B., Uzun A., Acikgöz E. (2020). Seeding rate and cultivar impacts on nutrient uptake of field pea under fertile soil condition. Chil. J. Agric. Res. 80, 11–20. doi: 10.4067/s0718-58392020000100011
Astocaza E., Franco J. (1983). El “nematodo de la oca” (Thecavermiculatus andinus sp.n.) en el altiplano peruano. Fitopatologıa 18, 39–47.
Badran A. E. (2022). Assessment of variation and stability parameters of five quinoa genotypes under drought stress conditions. Egypt. J. Bot. 62 (1), 21–30. doi: 10.21608/EJBO.2021.25026.1463
Barboza J., Ames T., Jacobsen S. E. (2000). “Reduccion de la germinacion y muerte de plantas de chenopodium quinoa causadas por rhizoctonia y fusarium,” in Memorias del primer taller internacional sobre quinua. Eds. Acobsen S. E., Mujica A., Portillo Z. (Peru: Centro Internacional de la Papa y Universidad Nacional Agraria), 457.
Bazile D., Bertero H. D., Nieto C. (2015). State of the art report on quinoa around the world in 2013 (Roma: FAO & CIRAD), 589.
Bazile D., Jacobsen S.-E., Verniau A. (2016). The global expansion of quinoa: trends and limits. front. Plant Sci. 7, 1–6. doi: 10.3389/fpls.2016.00622
Beccari G., Quaglia M., Tini F., Pannacci E., Covarelli L. (2021). Phytopathological threats associated with quinoa (chenopodium quinoa willd.) cultivation and seed production in an area of central Italy. Plants 10, 1933. doi: 10.3390/plants10091933
Bechman P. M., Finch H. C. (1980). Seed rot and damping-off of chenopodium quinoa caused by sclerotium rolfsii. Plant Dis. 64, 497–498. doi: 10.1094/PD-64-497
Benton Jones J. (2012). Plant nutrition and soil fertility manual (Boca Raton: Taylor & Francis Group).
Berti M., Wilckens R., Hevia F., Serri H., Vidal and C. Mendez I. (2000). Nitrogen fertilization in quinoan(Chenopodium quinoa willd.). Cienc. Investig. Agrar. 27 (2), 81–90. doi: 10.7764/rcia.v27i2.999
Bhargava A., Shukla S., Ohri D. (2007). Effect of sowing dates and row spacings on yield and quality components of quinoa (Chenopodium quinoa) leaves. Indian J. Agric. Sci. 77, 748–751.
Bilalis D., Kakabouki I., Karkanis A., Travlos I., Triantafyllidis V., H Berti D. (2012). Seed and saponin production of organic quinoa (Chenopodium quinoa willd.) for different tillage and fertilization. Not. Bot. Horti. Agrobot. Cluj. Napoca. 40, 42. doi: 10.15835/nbha4017400
Blanco A. (1994). Umbral economico de kcona kcona eurysacca melanocampta (Lepidoptera, gelechiidae) en quinua (Chenopodium quinoa willd.) (Peru: Universidad Nacional del Altiplano).
Boerema G. H., Mathur S. B., Neergaard P. (1977). Ascochyta hyalospora (Cooke & ell.) comb. nov. in seeds of chenopodium quinoa. Neth. J. Plant Pathol. 83, 153–159. doi: 10.1007/bf01981382
Buckland K. R., Reeve J. R., Creech J. E., Durham S. L. (2018). Managing soil fertility and health for quinoa production and weed control in organic systems. Soil tillage Res. 184, 52–61. doi: 10.1016/j.still.2018.07.001
Carrasco C., Jönsson L. J., Martín C. (2021). Hydrothermal pretreatment of water-extracted and aqueous ethanol-extracted quinoa stalks for enzymatic saccharification of cellulose. Energies 14, 4102. doi: 10.3390/en14144102
Casini P. (2019). Seed yield of two new quinoa (Chenopodium quinoa willd.) breeding lines as affected by sowing date in central Italy. Acta Agric. Slov. 113, 51–62. doi: 10.14720/aas.2019.113.1.05
Chaudhary N., Walia S., Kumar R. (2023). Functional composition, physiological effect and agronomy of future food quinoa (chenopodium quinoa willd.): a review. J. Food Compost. Anal. 118, 105192. doi: 10.1016/j.jfca.2023.105192
Choi Y. J., Choi I. Y., Kim J. S., Shin H. D. (2014). First report of quinoa downy mildew caused by peronospora variabilis in republic of Korea. Plant Dis. 98, 1003–1003. doi: 10.1094/pdis-12-13-1209-pdn
Cruces L., de Peña E., De Clercq P. (2020). Seasonal phenology of the major insect pests of quinoa (chenopodium quinoa willd.) and their natural enemies in a traditional zone and two new production zones of Peru. Agriculture 10, 644. doi: 10.3390/agriculture10120644
Curti R. N., de la Vega A. J., Andrade A. J., Bramardi S. J., Bertero H. D. (2014). Multi-environmental evaluation for grain yield and its physiological determinants of quinoa genotypes across Northwest Argentina. Field Crops Res. 166, 46–57. doi: 10.1016/j.fcr.2014.06.011
D'Amico S., Jungkunz S., Balasz G., Foeste M., Jekle M., Tömösköszi S., et al. (2019). Abrasive milling of quinoa: study on the distribution of selected nutrients and proteins within the quinoa seed kernel. J. Cereal Sci. 86, 132–138. doi: 10.1016/j.jcs.2019.01.007
Danielsen S., Bonifacio A., Ames T. (2003). Diseases of quinoa (chenopodium quinoa). Food Rev. Int. 19, 43–59. doi: 10.1081/fri-120018867
Danielsen S., Jacobsen S.-E., Hockenhull J. (2002). First report of downy mildew of quinoa caused by peronospora farinosa f. sp. chenopodii in Denmark. Plant Dis 86, 1175–1175. doi: 10.1094/pdis.2002.86.10.1175b
Danielsen S., Mercado V. H., Ames T., Munk L. (2004). Seed transmission of downy mildew (Peronospora farinosa f. sp. chenopodii) in quinoa and effect of relative humidity on seedling infection. Seed Sci. Technol. 32, 91–98. doi: 10.15258/sst.2004.32.1.10
Danielsen S., Munk L. (2004). Evaluation of disease assessment methods in quinoa for their ability to predict yield loss caused by downy mildew. Crop Prot. 23, 219–228. doi: 10.1016/j.cropro.2003.08.010
Dao A., Guira A., Alvar-Beltrán J., Gnan-Da A., Nebie L., Sanou J. (2020). Quinoa’s response to different sowing periods in two agro-ecological zones of Burkina Faso. Ital. J. Agrometeorol. 1, 63–72. doi: 10.13128/ijam-731
Deng Y., Wang J. L., Wang C. Y., Zhao L., Zhang L. G., Guo H. X., et al. (2021). Effects of combined application of bio-bacterial fertilizer and inorganic fertilizer on agronomic characters, yield, and quality in quinoa. Zuo. Wu. Xue. Bao. 47 (7), 1383–1390. doi: 10.3724/SP.J.1006.2021.04171
Deza M. D. P. (2018). Rendimiento y calidad de la quinua (Chenopodium quinoa willd) con dos densidades de siembra y dos sistemas de fertilización en condiciones de la Molina (Peru: Universidad Nacional Agraria La Molina).
Diaz I. C., Hans N. C., Linda I. D., Gladys A. R. (2021). Effect of sowing density on the agronomic performance of quinoa naro cultivar and the transmissivity of photosynthetically active radiation in the high tropics of Colombia. Rev. Fac. Nac. Agron. Medellín 74 (2), 9491–9497. doi: 10.15446/rfnam.v74n2.90040
Ebrahimikia M., Jami Moeini M., Marvi H., Hasheminejhad Y., Ghasemzadeh Ganjehie M. (2021). Agro-physiological response of quinoa (chenopodium quinoa willd.) to the nitrogen application rate and split application method. J. Soil Sci. Plant Nutr. 21, 3437–3450. doi: 10.1007/s42729-021-00618-6
El-Shamy M. A., Alshaal T., Mohamed H. H., Rady A. M., Hafez E. M., Alsohim A. S., et al. (2022). Quinoa response to application of phosphogypsum and plant growth-promoting rhizobacteria under water stress associated with salt-affected soil. Plants 11, 872. doi: 10.3390/plants11070872
Fatemi Kiyan H., Tatari M., Tokalo M. R., Salehi M., Hajmohammadnia Ghalibaf K. (2022). The effect of deficit irrigation and fertilizer on quantitative and qualitative yield of quinoa (chenopodium quinoa). Ital. J. Agrometeorol. 1, 83–99. doi: 10.36253/ijam-1136
Fghire R., Anaya F., Issa Ali O., Lamnai K., Foughali B., Faghire M., et al. (2021). Effects of deficit irrigation and fertilization on quinoa (chenopodium quinoa willd.) water status and yield productions. Gesunde Pflanzen 74, 97–110. doi: 10.1007/s10343-021-00591-1
Fghire R., Chraibi A., Wahbi S. (2012). Does deficit irrigation be a sustainable approach for optimized quinoa production in arid and semi-arid regions? J. Hydrocarb. Mines Environ. Res. 3 (2), 202. Abstract retrieved from Abstracts in First International Colloquium REZAS'12.
Fghire R., Oudou I., Anaya F., Benlhabib O., Jacobsen S. E., Wahbi S. (2013). Protective antioxidant enzyme activities are affected by drought in quinoa (Chenopodium quinoa willd.). J. Biol. Agric. Healthc. 3, 62–68.
Fonseca-Guerra I., Chiquillo C., Padilla M. J., Benavides-Rozo M. (2021). First report of bacterial leaf spot on chenopodium quinoa caused by pseudomonas syringae in Colombia. J. Plant Dis. Prot. 128, 871–874. doi: 10.1007/s41348-021-00435-0
Franco J. (2003). Parasitic nematodes of quinoa in the Andean region of Bolivia. Food Rev. Int. 19, 77–85. doi: 10.1081/fri-120018869
Garcia M., Condori B., Castillo C. (2015). “Agroecological and agronomic cultural practices of quinoa in south America,” in Quinoa improvement and sustainable production. Eds. Murphy K., Matanguihan J. (Hoboken, New Jersey, Canada: John Wiley & Sons).
Garcia-Parra M., García-Molano J., Carvajal-R D. (2018). Evaluación del efecto de la fertilización química y orgánica en la composición bromatológica de semillas de quinua (Chenopodium quinoa willd) en boyacá – Colombia. Rev. Investig. Agrar. Ambient. 9 (2), 1–10. doi: 10.22490/21456453.2282
García-Parra M., García-Molano J., Melo-Ortiz D., Deaquiz-Oyola Y. (2017). Respuesta agronómica de la quinua (Chenopodium quinoa willd.) variedad dulce de soracá a la fertilización en ventaquemada - boyacá. Cuad. Cult. 15, 66–77. doi: 10.38017/issn.1657-463X
Garcia-Parra M., Roa D. F., Stechauner R., García-Molano F., Bazile D., Leguizamon Plazas N. Z. (2020). Effect of temperature on the growth and development of quinoa plants (Chenopodium quinoa willd.): a review on a global scale. Sylwan 164, 411–433.
Garrido M., Silva P., Silva H., Muñoz R., Baginsky C., Acevedo E. (2013). Evaluación del rendimiento de nueve genotipos de quinua (chenopodium quinoa willd.j bajo diferentes disponibilidades hídricas en ambiente mediterráneo. Idesia (Arica) 31, 69–76. doi: 10.4067/s0718-34292013000200010
Geren H. (2015). Effects of different nitrogen levels on the grain yield and some yield components of quinoa (chenopodium quinoa willd.) under Mediterranean climatic conditions. Turk. J. Field Crops 20, 59–64. doi: 10.17557/.39586
Gómez M. B., Curti R. N., Bertero H. D. (2021). Seed weight determination in quinoa ( chenopodium quinoa willd.). J. Agron. Crop Sci. 208, 243–254. doi: 10.1111/jac.12532
Gómez-Caravaca A. M., Iafelice G., Verardo V., Marconi E., Caboni M. F. (2014). Influence of pearling process on phenolic and saponin content in quinoa (chenopodium quinoa willd). Food Chem. 157, 174–178. doi: 10.1016/j.foodchem.2014.02.023
González J. A., Hinojosa L., Mercado M. I., Fernández-Turiel J.-L., Bazile D., Ponessa G. I., et al. (2021). A long journey of CICA-17 quinoa variety to salinity conditions in Egypt: mineral concentration in the seeds. Plants 10, 407. doi: 10.3390/plants10020407
Hamza A., Usman K., Malik M. W., Saad M., Ghulam S., Khan Z., et al. (2021). Response of quinoa genotypes to sowing dates and sowing methods under agroclimatic condition of dera ismail khan, KP Pakistan. Arch. Agron. Soil Sci. 68, 1903–1913. doi: 10.1080/03650340.2021.1939018
Hassell M. P. (1978). The dynamics of arthropod predator–prey systems (Princeton, NJ, USA: Princeton University Press).
Hirich A., Choukr-Allah R., Jacobsen S.-E. (2014a). Quinoa in Morocco - effect of sowing dates on development and yield. J. Agron. Crop Sci. 200, 371–377. doi: 10.1111/jac.12071
Hirich A., Choukr-Allah R., Jacobsen S.-E. (2014b). Deficit irrigation and organic compost improve growth and yield of quinoa and pea. J. Agron. Crop Sci. 200, 390–398. doi: 10.1111/jac.12073
Hirich A., Choukr-Allah R., Jacobsen S. E., El Yousfi L., El Omari H. (2012). Using deficit irrigation with treated wastewater in the production of quinoa (Chenopodium quinoa willd.) in Morocco. Rev. Cient. UDO Agric. 12, 570–583. doi: 10.28546/s00271-013-0422-0
Hirich A., Rafik S., Rahmani M., Fetouab A., Azaykou F., Filali K., et al. (2021). Development of quinoa value chain to improve food and nutritional security in rural communities in rehamna, morocco: lessons learned and perspectives. Plants 10, 301. doi: 10.3390/plants10020301
Hlásná Cepková P., Dostalíková L., Viehmannová I., Jágr M., Janovská D. (2022). Diversity of quinoa genetic resources for sustainable production: a survey on nutritive characteristics as influenced by environmental conditions. Front. Sustain. Food Syst. 6. doi: 10.3389/fsufs.2022.960159
Hussain M. I., Al- Dakheel A. J., Reigosa M. J. (2018). Genotypic differences in agro-physiological, biochemical and isotopic responses to salinity stress in quinoa (Chenopodium quinoa willd.) plants: prospects for salinity tolerance and yield stability. Plant Physiol. Biochem. 129, 411–420. doi: 10.1016/j.plaphy.2018.06.023
Hussain M. I., Muscolo A., Ahmed M., Asghar M. A., Al-Dakheel A. J. (2020). Agro-morphological, yield and quality traits and interrelationship with yield stability in quinoa (chenopodium quinoa willd.) genotypes under saline marginal environment. Plants 9, 1763. doi: 10.3390/plants9121763
Ikeda A., Ichitani T. (1985). Density of pythium zingiberum oospores in relation to infection of chenopodium quinoa seedlings. Bull. Univ. Osaka Prefect. 37, 13–18. doi: 10.24729/00009338
IPCC. (2018). “Summary for policymakers,” in Global warming of 1.5°C. Eds. Masson-Delmotte V., Zhai P., Pörtner H.-O., Roberts D., Skea J., Shukla P. R. (UK and New York: Cambridge University Press), 3–24.
Iqbal S., Basra S. M., Afzal I., Wahid A., Saddiq M. S., Hafeez M. B., et al. (2018). Yield potential and salt tolerance of quinoa on salt-degraded soils of Pakistan. J. Agron. Crop Sci. 205, 13–21. doi: 10.1111/jac.12290
Isobe K., Koide S., Yamazaki R., Kawamura Y., Higo M., Ujiie K. (2021). Study on yield determinants of valley type-quinoa, amarilla de maranganivalley. Japanese J. Crop Sci. 90 (2), 206–210. doi: 10.1626/jcs.90.206
Jacobsen S.-E. (2003). The worldwide potential for quinoa (chenopodium quinoawilld.). Food Rev. Int. 19, 167–177. doi: 10.1081/fri-120018883
Jacobsen S.-E., Jørgensen I., Stølen O. (1994). Cultivation of quinoa (chenopodium quinoa) under temperate climatic conditions in Denmark. J. Agric. Sci. 122, 47–52. doi: 10.1017/s0021859600065783
Jacobsen S.-E., Monteros C., Christiansen J. L., Bravo L. A., Corcuera L. J., Mujica A. (2005). Plant responses of quinoa (chenopodium quinoa willd.) to frost at various phenological stages. Eur. J. Agron. 22, 131–139. doi: 10.1016/j.eja.2004.01.003
Jensen C. R., Jacobsen S.-E., Andersen M. N., Núñez N., Andersen S. D., Rasmussen L., et al. (2000). Leaf gas exchange and water relation characteristics of field quinoa (Chenopodium quinoa willd.) during soil drying. Eur. J. Agron. 13, 11–25. doi: 10.1016/s1161-0301(00)00055-1
Jiang F., Ren Y., Du C., Nie G., Liang J., Yu X., et al. (2021). Effect of pearling on the physicochemical properties and antioxidant capacity of quinoa (chenopodium quinoa willd.) flour. J. Cereal Sci. 102, 103330. doi: 10.1016/j.jcs.2021.103330
Ji-kai L., Jian-li W., Chen S., Hai-ling Z., Jie-lin L., Ji-shan C., et al. (2019). Adaptability of different quinoa germplasm lines in Harbin. Cao. Ye. Xue. Bao. 28 (9), 202–208. doi: 10.11686/cyxb2019190
Kakabouki I., Roussis I. E., Papastylianou P., Kanatas P., Hela D., Katsenios N., et al. (2019). Growth analysis of quinoa (chenopodium quinoa willd.) in response to fertilization and soil tillage. Not. Bot. Horti. Agrobot. Cluj. Napoca. 47, 1025–1036. doi: 10.15835/nbha47411657
Kaya Y., Akcura M. (2014). Effects of genotype and environment on grain yield and quality traits in bread wheat (T. aestivum l.). J. Food Sci. Technol. (Campinas) 34, 386–393. doi: 10.1590/fst.2014.0041
Keshtkar A., Aien A., Naghavi H., Najafinezhad H., And Shirzadi M. H. (2022). Effect of the application of foliar jasmonic acid and drought stress on grain yield and some physiological and biochemical characteristics of chenopodium quinoa cultivars. Tarim Bilim. Derg. 28 (2), 171–180. doi: 10.15832/ankutbd.714568
Lavini A., Pulvento C., d'Andria R., Riccardi M., Choukr-Allah R., Belhabib O., et al. (2014). Quinoa's potential in the Mediterranean region. J. Agron. Crop Sci. 200, 344–360. doi: 10.1111/jac.12069
Lesjak J., Calderini D. F. (2017). Increased night temperature negatively affects grain yield, biomass and grain number in Chilean quinoa. Front. Plant Sci. 8. doi: 10.3389/fpls.2017.00352
Liu M., Yang M., Yang H. (2020). Biomass production and nutritional characteristics of quinoa subjected to cutting and sowing date in the Midwestern China. Grassl. Sci. 67, 215–224. doi: 10.1111/grs.12307
Llaca Ninaja B. G. C. (2014). Influencia de la fertilización nitrogenada y fosfórica en el rendimiento de quinua (Chenopodium quinoa willd) en el proter sama, región tacna. (Panama: Universidad de Panama).
Loza A., Clavitea J., Delgado P. (2016). Incidencia de aves granívoras y su importancia como plagas en el cultivo de quinua (Chenopodium quinoa willd.) en el altiplano peruano. Bioagro 28 (3), 139–150.
Ludvigson K., Reganold J. P., Murphy K. M. (2019). Sustainable intensification of quinoa production in peri-urban environments in Western Washington state utilizing transplant vs. direct-seed methods. Cienc. Investig. Agrar. 46 (2), 100–112. doi: 10.7764/rcia.v46i2.2169
Maamri K., Zidane O. D., Chaabena A., Fiene G., Bazile D. (2022). Adaptation of some quinoa genotypes (chenopodium quinoa willd.), grown in a Saharan climate in Algeria. Life 12, 1854. doi: 10.3390/life12111854
Mackauer M., Volkl W. (1993). Regulation of aphid populations by aphidiid wasps: does parasitoid foraging behaviour or hyperparasitism limit impact? Oecologia 94, 339–350. doi: 10.1007/BF00317107
Maestro-Gaitán I., Granado-Rodríguez S., Orús M. I., Matías J., Cruz V., Bolaños L., et al. (2022). Genotype-dependent responses to long-term water stress reveal different water-saving strategies in chenopodium quinoa willd. Environ. Exp. Bot. 201, 104976. doi: 10.1016/j.envexpbot.2022.104976
Mansouri M., Alavi Fazel M., Gilani A., Lak S., Mojddam M. (2021). Effect of nitrogen fertilization on efficiency and remobilization in quinoa cultivars. Ann. di Bot. 11, 155–170. doi: 10.13133/2239-3129/17108
Martínez E. A., Veas E., Jorquera C., San Martín R., Jara P. (2009). Re-introduction of quínoa into arid Chile: cultivation of two lowland races under extremely low irrigation. J. Agron. Crop Sci. 195, 1–10. doi: 10.1111/j.1439-037x.2008.00332.x
Matías J., Rodríguez M. J., Cruz V., Calvo P., Reguera M. (2021). Heat stress lowers yields, alters nutrient uptake and changes seed quality in quinoa grown under Mediterranean field conditions. J. Agron. Crop Sci. 207, 481–491. doi: 10.1111/jac.12495
Mez MB G., Castro P. A., Mignone C., Bertero H. D. (2011). Can yield potential be increased by manipulation of reproductive partitioning in quinoa (Chenopodium quinoa)? evidence from gibberellic acid synthesis inhibition using paclobutrazol. Funct. Plant Biol. 38 (5), 420–430. doi: 10.1071/FP10168
Mhada M., Metougui M. L., El Hazzam K., El Kacimi K., Yasri A. (2020). Variations of saponins, minerals and total phenolic compounds due to processing and cooking of quinoa (chenopodium quinoa willd.) seeds. Foods 9, 660. doi: 10.3390/foods9050660
Minh N. V., Hoang D. T., Loc N. V., Long N. V. (2020). Effects of plant density on growth, yield and seed quality of quinoa genotypes under rain-fed conditions on red basalt soil regions. Aust. J. Crop Sci. 14 (12), 1977–1982. doi: 10.21475/ajcs.20.14.12.2849
Mora-Ocación M. S., Morillo-Coronado A. C., Manjarres-Hernández E. H. (2022). Extraction and quantification of saponins in quinoa (Chenopodium quinoa willd.) genotypes from Colombia. Int. J. Food Sci. 2022, 1–7. doi: 10.1155/2022/7287487
Mujica A., Canahua A., Saravia R. (2001). “Capitulo II. agronomía del cultivo de la quinua,” in Quinua (chenopodium quinoa willd.): ancestral cultivo andino, alimento del presente y futuro. Eds. Mujica A., Jacobsen S. :. E. :., Izquierdo J., Marathee J. P. (Santiago, Chile: Santiago, FAO), 197.
Mujica A., Jacobsen S.-E. (1999). “Resistencia a sequı´a de la quinua (Chenopodium quinoa willd.) y su mejoramiento,” in Pertubaciones climaticas de la corriente El nin˜o en la produccio´n agropecuaria de la region de puno. Eds. Larico J., Lopez D., Solano M. A., Suca A. (Peru: Universidad Nacional del Altiplano), 59–80.
Murphy K., Matanguihan J. (2015). Quinoa improvement and sustainable production (New Jersey: John Wiley and Sons).
Nadali F., Asghari H. R., Abbasdokht H., Dorostkar V., Bagheri M. (2021). Improved quinoa growth, physiological response, and yield by hydropriming under drought stress conditions. Gesunde Pflanzen 73, 53–66. doi: 10.1007/s10343-020-00527-1
Neyra J. (2014). Efecto de la fertilización bioorgánica en el rendimiento del cultivo de quinua (Chenopodium quinoa willd.) (Peru: Universidad Nacional Jorge Basadre Grohmann).
Noulas C., Tziouvalekas M., Vlachostergios D., Baxevanos D., Karyotis T., Iliadis C. (2017). Adaptation, agronomic potential, and current perspectives of quinoa under Mediterranean conditions: case studies from the lowlands of central Greece. Commun. Soil Sci. Plant Anal. 48 (22), 2612–2629. doi: 10.1080/00103624.2017.1416129
Nurse R. E., Obeid K., Page E. R. (2016). Optimal planting date, row width, and critical weed-free period for grain amaranth and quinoa grown in Ontario, Canada. Can. J. Plant Sci. 96, 360–366. doi: 10.1139/cjps-2015-0160
Osco Limachi S. V. (2009). Productividad de variedades de quinua (Chenopodium quinoa willd.) con la aplicación de diferentes niveles de fertilizacion organica en la localidad de tiwanacub. (Bolivia: Universidad Mayor de San Andres).
Otazu V., Salas B. (1977). Brown stalk of chenopodium quinoa caused by phoma exigua var. foveata. Fitopatologıa 12, 54–58.
Oudou I. A., Fghire R., Anaya F., Benlhabib O., Wahbi S. (2019). Physiological and morphological responses of two quinoa cultivars (Chenopodium quinoa willd.) to drought stress. Gesunde Pflanz. 71, 123–133. doi: 10.1007/s10343-019-00460-y
Pal N., Testen A. L. (2021). First report of quinoa anthracnose caused by colletotrichum nigrum and c. truncatum in the united states. Plant Dis. 105, 705–705. doi: 10.1094/pdis-07-20-1568-pdn
Parwada C., Mandumbu R., Tibugari H., Badze D., Mhungu S. (2020). Effect of soil fertility amendment, planting density and growing season on chenopodium quinoa willd (quinoa) in Zimbabwe. Cogent Food Agric. 6, 1792668. doi: 10.1080/23311932.2020.1792668
Peyghan K., Golabi M., Albaji M. (2020). Simulation of quinoa (Chenopodium quinoa) yield and soil salinity under salinity and water stress using the SALTMED model. Commun. Soil Sci. Plant Anal. 51 (18), 2361–2376. doi: 10.1080/00103624.2020.1836203
Pinto A. A., Fischer S., Wilckens R., Bustamante L., Berti M. T. (2021). Production efficiency and total protein yield in quinoa grown under water stress. Agriculture 11, 1089. doi: 10.3390/agriculture11111089
Pulvento C., Riccardi M., Lavini A., Iafelice G., Marconi E., d’Andria R. (2012). Yield and quality characteristics of quinoa grown in open field under different saline and non-saline irrigation regimes. J. Agron. Crop Sci. 198, 254–263. doi: 10.1111/j.1439-037x.2012.00509.x
Quispe H., León A. A. (2018). Fertilización nitrogenada y fosfórica en quinua (chenopodium quinoa willd.) CV. ´Salcedo INIA´ bajo riego a goteo en zona árida (Peru: Universidad Nacional de San Agustín de Arequipa).
Quispe-Fuentes I., Vega-Gálvez A., Miranda M., Lemus-Mondaca R., Lozano M., And Ah-Hen K. (2012). A kinetic approach to saponin extraction during washing of quinoa (chenopodium quinoawilld.) seeds. J. Food Process. Eng. 36, 202–210. doi: 10.1111/j.1745-4530.2012.00673.x
Rafik S., Rahmani M., Choukr-Allah R., El Gharous M., Calle J. P., Filali K., et al. (2021a). Techno-economic assessment of quinoa production and transformation in Morocco. Environ. Sci. pollut. Res. 28, 46781–46796. doi: 10.1007/s11356-021-12665-8
Rafik S., Rahmani M., Rodriguez J. P., Andam S., Ezzariai A., El Gharous M., et al. (2021b). How does mechanical pearling affect quinoa nutrients and saponin contents? Plants 10, 1133. doi: 10.3390/plants10061133
Rasmussen C., Lagnaoui A., Esbjerg P. (2003). Advances in the knowledge of quinoa pests. Food Rev. Int. 19, 61–75. doi: 10.1081/fri-120018868
Rathore S., Kumar R. (2021). Vermicompost fertilization and pinching improves the growth, yield, and quality of super food (chenopodium quinoa willd.) in the western himalaya. Acta Physiol. Plant 43, 1–16. doi: 10.1007/s11738-020-03184-z
Razzaghi F., Bahadori-Ghasroldashti M. R., Henriksen S., Sepaskhah A. R., Jacobsen S. E. (2020). Physiological characteristics and irrigation water productivity of quinoa ( chenopodium quinoa willd.) in response to deficit irrigation imposed at different growing stages–a field study from southern Iran. J. Agron. Crop Sci. 206, 390–404. doi: 10.1111/jac.12392
Ren Y. F., Mei L., Yang Y. D., Wang Z. M., Zhao P. Y., Gao Y. (2018). Effects of sowing time on agronomic characteristics and yield of quinoa. Chin. J. Eco-Agric. 26 (5), 643–656. doi: 10.13930/j.cnki.cjea.170852
Repo-Carrasco R., Espinoza C., Jacobsen S.-E. (2003). Nutritional value and use of the Andean crops quinoa (chenopodium quinoa) and kañiwa (chenopodium pallidicaule). Food Rev. Int. 19, 179–189. doi: 10.1081/fri-120018884
Risi J., Galwey N. W. (1991). Effects of sowing date and sowing rate on plant development and grain yield of quinoa (chenopodium quinoa) in a temperate environment. J. Agric. Sci. 117, 325–332. doi: 10.1017/s002185960006706x
Salas S. (2003). Quinoa - postharvest and commercialization. Food Rev. Int. 19, 191–201. doi: 10.1081/FRI-120018885
Sales A. C., Campos C. N., de Souza Junior J. P., da Silva D. L., Oliveira K. S., de Mello Prado R., et al. (2021). Silicon mitigates nutritional stress in quinoa (Chenopodium quinoa willd.). Sci. Rep. 11, 1–16. doi: 10.1038/s41598-021-94287-1
Salim S. A., Al-Hadeethi I. K., Alobaydi S. A. J. (2019). Role of irrigation scheduling and potassium fertilization on soil moisture depletion and distributino of quinoa root (irrigation schedulling fertilization and their effect on moisture depletion and yield). Plant Arch. 19 (2), 3844–3852.
Salim S. A., Hadeethi I. K. H. (2020). Water stress on different growing stages for quinoa (chenopodium quinoa willd) and its infleunce on water requiriments and yield. Iraqi J. Agric. Sci. 51, 953–966. doi: 10.36103/ijas.v51i3.1051. G. M. , A.
Santos R. L. B., Spehar C. R., Vivaldi L. (2003). Quinoa (Chenopodium quinoa) reaction to herbicide residue in a Brazilian savannah soil. Pesq. agropec. Bras. 38, (6). doi: 10.1590/S0100-204X2003000600014
Schulte auf’m Erley G., Kaul H.-P., Kruse M., Aufhammer W. (2005). Yield and nitrogen utilization efficiency of the pseudocereals amaranth, quinoa, and buckwheat under differing nitrogen fertilization. Eur. J. Agron. 22, 95–100. doi: 10.1016/j.eja.2003.11.002
Sellami M. H., Pulvento C., Lavini A. (2020). Agronomic practices and performances of quinoa under field conditions: a systematic review. Plants 10, 72. doi: 10.3390/plants10010072
Shafiq M., Khalid M. Z., Latif M. M., Saleem F. S., Sabir A., Ayyoub M. Z., et al. (2022). Botanical description, cultivation, and nutritional profile of ancients’ grains (Quinoa, chia, and buckwheat) and their importance in 21st century: a review. J. pure Appl. Agric. 7 (4), 1–13.
Shams A. S. (2011). Response of quinoa to nitrogen fertilizer rates under sandy soil conditions. Int. J. Water Resour. arid Environ. 1 (5), 318–325.
Shi J., Xue S. J., Ma Y., Li D., Kakuda Y., Lan Y. (2009). Kinetic study of saponins b stability in navy beans under different processing conditions. J. Food Eng. 93, 59–65. doi: 10.1016/j.jfoodeng.2008.12.035
Sigsgaard L., Jacobsen S. E., Christiansen J. L. (2008). Quinoa,chenopodium quinoa, provides a new host for native herbivores in northern Europe: case studies of the moth,scrobipalpa atriplicella, and the tortoise beetle,Cassida nebulosa. J. Insect Sci. 8, 1–4. doi: 10.1673/031.008.5001
Simmonds N. W. (1965). The grain chenopods of the tropical American highlands. Econ. Bot. 19, 223–235. doi: 10.1007/bf02914309
Snowball R., Dhammu H. S., D’Antuono M. F., Troldahl D., Biggs I., Thompson C., et al. (2022). Adaptation of quinoa (chenopodium quinoa willd.) to Australian environments. Agronomy 12, 2026. doi: 10.3390/agronomy12092026
Spehar C. R., da Silva Rocha J. E. (2010). Exploiting genotypic variability from low-altitude Brazilian savannah-adapted chenopodium quinoa. Euphytica 175, 13–21. doi: 10.1007/s10681-010-0154-7
Stoleru V., Jacobsen S.-E., Vitanescu M., Jitareanu G., Butnariu M., Munteanu N., et al. (2022). Nutritional and antinutritional compounds in leaves of quinoa. Food Biosci. 45, 101494. doi: 10.1016/j.fbio.2021.101494
Taaime N., El Mejahed K., Moussafir M., Bouabid R., Oukarroum A., Choukr-Allah R., et al. (2022). Early sowing of quinoa cultivars, benefits from rainy season and enhances quinoa development, growth, and yield under arid condition in Morocco. Sustainability 14, 4010. doi: 10.3390/su14074010
Tapia M. E. (2001). “Zonificación agro ecológica del cultivo de quinua (Chenopodium quinoa willd),” in Primer taller internacional sobre quinua : recursos genéticos y sistemas de producción (Lima, Peru: International Potato Center), 17–27.
Taylor J. R., Parker M. L. (2002). “Quinoa,” in Pseudocereals and less common cereals. (Berlin: Springer), 93–122. doi: 10.1007/978-3-662-09544-7_3
Terletskaya N. V., Erbay M., Zorbekova A. N., Prokofieva M. Y., Saidova L. T., Mamirova A. (2022). Influence of osmotic, salt, and combined stress on morphophysiological parameters of chenopodium quinoa photosynthetic organs. Agriculture 13, 1. doi: 10.3390/agriculture13010001
Thiam E., Allaoui A., Benlhabib O. (2021). Quinoa productivity and stability evaluation through varietal and environmental interaction. Plants 10, 714. doi: 10.3390/plants10040714
Toubali S., Ait-El-Mokhtar M., Boutasknit A., Anli M., Ait-Rahou Y., Benaffari W., et al. (2022). Root reinforcement improved performance, productivity, and grain bioactive quality of field-droughted quinoa (chenopodium quinoa). Front. Plant Sci. 13. doi: 10.3389/fpls.2022.860484
Valdivia-Cea W., Bustamante L., Jara J., Fischer S., Holzapfel E., Wilckens R. (2021). Effect of soil water availability on physiological parameters, yield, and seed quality in four quinoa genotypes (chenopodium quinoa willd.). Agronomy 11, 1012. doi: 10.3390/agronomy11051012
Villanueva S. (1978). Determinacio´n del “Umbral econo´mico” y “nivel critico” de “Kcona kcona” (Scrobipalpula sp.) en quinua (Chenopodium quinua willd.) (Peru: Universidad Nacional del Altiplano).
Wang N., Wang F.-X., Shock C. C., Meng C.-B., Huang Z.-J., Gao L., et al. (2021). Evaluating quinoa stem lodging susceptibility by a mathematical model and the finite element method under different agronomic practices. Field Crops Res. 271, 108241. doi: 10.1016/j.fcr.2021.108241
Wang N., Wang F., Shock C. C., Meng C., Qiao L. (2020). Effects of management practices on quinoa growth, seed yield, and quality. Agronomy 10, 445. doi: 10.3390/agronomy10030445
Yabar E., Gianoli E., Echegaray E. R. (2002). Insect pests and natural enemies in two varieties of quinua (chenopodium quinoa) at cusco, Peru. J. Appl. Entomol. 126, 275–280. doi: 10.1046/j.1439-0418.2002.00664.x
Keywords: quinoa, systematic review, cultivar, planting date, fertilization, irrigation, postharvest
Citation: Taaime N, Rafik S, El Mejahed K, Oukarroum A, Choukr-Allah R, Bouabid R and El Gharous M (2023) Worldwide development of agronomic management practices for quinoa cultivation: a systematic review. Front. Agron. 5:1215441. doi: 10.3389/fagro.2023.1215441
Received: 02 May 2023; Accepted: 16 June 2023;
Published: 05 July 2023.
Edited by:
Cosmas Parwada, Midlands State University, ZimbabweReviewed by:
Liboster Mwadzingeni, University of KwaZulu-Natal, South AfricaCopyright © 2023 Taaime, Rafik, El Mejahed, Oukarroum, Choukr-Allah, Bouabid and El Gharous. This is an open-access article distributed under the terms of the Creative Commons Attribution License (CC BY). The use, distribution or reproduction in other forums is permitted, provided the original author(s) and the copyright owner(s) are credited and that the original publication in this journal is cited, in accordance with accepted academic practice. No use, distribution or reproduction is permitted which does not comply with these terms.
*Correspondence: Nawal Taaime, bmF3YWwudGFhaW1lQHVtNnAubWE=
Disclaimer: All claims expressed in this article are solely those of the authors and do not necessarily represent those of their affiliated organizations, or those of the publisher, the editors and the reviewers. Any product that may be evaluated in this article or claim that may be made by its manufacturer is not guaranteed or endorsed by the publisher.
Research integrity at Frontiers
Learn more about the work of our research integrity team to safeguard the quality of each article we publish.