- 1Postgraduate Institute of Agriculture, University of Peradeniya, Peradeniya, Sri Lanka
- 2Department of Plant Sciences, Faculty of Agriculture, Rajarata University of Sri Lanka, Anuradhapura, Sri Lanka
- 3Department of Crop Science, Faculty of Agriculture, University of Peradeniya, Peradeniya, Sri Lanka
- 4Department of Plant Sciences, Faculty of Agricultural and Food Sciences, University of Manitoba, Winnipeg, MB, Canada
Integrated weed control strategies are essential for organic and integrated nutrient management, where both systems are progressing with a fundamental of zero or minimum synthetic chemical cultivations. For optimizing the outcome of weed management, a better understanding of the weed dynamic is needed. Especially, with the absence of herbicides, weeds are expected to be controlled by the system itself, during the transition period under rice-based crop rotation systems. This study was conducted to estimate the weed abundance, growth, and composition during the transitional period with conventional (CONV), integrated (INT), and organic (ORG) nutrient management under four crop diversification intensities in a dry zone of Sri Lanka. Monocrop rice and a rice-maize rotation were the starting point. After 1 year, the diversification intensity was increased by adding interseason sunnhemp (rice-sunnhemp-rice and rice-sunnhemp-maize). Weed density and weed biomass were measured at 20 DAS and 60 DAS intervals. Weed density was higher in ORG during the early growth stages of monocrop rice rotation in the 1st cycle, and monocrop rice and rice-sunnhemp-rice rotation in the 2nd cycle while didn’t show any changes during the later growth stage of all systems in both cycles. The total weed biomass in ORG increased with increasing crop diversification. Overall, crop rotation in INT reported the lowest weed density and biomass after two cycles. In the CONV with rice-sunnhemp-maize rotation, weed biomass had declined, while in ORG grass biomass decreased only in sunnhemp cultivated rotations. Overall, INT was the best for weed suppression irrespective of crop rotation intensities. Monoculture with rice in the INT was able to suppress weed more effectively than rice-maize rotation.
1 Introduction
Increasing biotic and abiotic threats due to climate change (Teshome et al., 2020) and the global rise in input prices, and the unavailability of farm inputs further calls for alternative sustainable crop production systems globally as well as locally. Hence, current cropping systems require development of more sustainable practices which are less dependent on anthropogenic and petroleum inputs, efficient resource use, and low environmental impact with protecting human and animal health (Pretty, 2008). Low-input or organic production systems can be an alternative to conventional systems to overcome the present challenges in crop production in Sri Lanka. These systems less rely on external synthetic inputs but rather rely on ecological and natural processes to maintain soil fertility and provide crop protection (Reganold and Wachter, 2016). Even though these systems may look like an attractive alternative to conventional systems, low crop yields and managing pests, disease, and weeds particularly during the transition from conventional to alternative systems are hindering the wide adoption by farmers (Zinati, 2002).
Weeds are considered the most detrimental and persistent challenge to agricultural production (Scavo and Mauromicale, 2020) with around 34% yield loss worldwide (Oerke, 2006; Gharde et al., 2018; IRRI, 2018). Managing weeds in both conventional and alternative crop production systems was found to be a persistent challenge. Although herbicides are a predominant tool to manage weeds in arable land (Jansen and Dubois, 2014), non-chemical/ecological weed management strategies are gaining attention as an alternative weed management strategy after realizing many negative impacts such as cost of production, enhanced environmental pollution, and accelerated evolution of herbicide-resistant weeds (Qasem, 2011; Pingali, 2012; Herath et al., 2017; Sharma and Singhvi, 2017).
Integrated weed management using ecological, cultural, and mechanical weed management strategies has become essential for alternative cropping systems, especially in organic and low external nutrient management systems (Liebman et al., 1997; Shirtliffe and Benaragama, 2014; Reganold and Wachter, 2016). In the absence/reduction of herbicide usage, weed dynamics particularly in alternative cropping systems are determined due to the long-term interaction of diverse crop rotations (Smith and Gross, 2006; Anderson, 2015), tillage (Armengot et al., 2016), altered nutrient dynamics due to different rates and sources of nutrients (Little et al., 2021) and the short-term integration of many cultural and mechanical weed control strategies (Liebman et al., 1997). Therefore, understanding the interactions of all these factors on weed dynamics is essential for devising sustainable weed management strategies for most alternative cropping systems where herbicides are excluded or minimally used.
In most tropical alternative cropping systems, the most significant difference occurs among systems due to differences in nutrient management strategies. Nutrient management practices can have a direct and indirect effect on weed dynamics either by weed seed immigration via nutrient sources such as compost or due to altered competition dynamics due to differences in the source and rates of nutrients applied (Cordeau et al., 2021). Both organic and low-input systems rely mainly on manure and compost as nutrient sources that can harbor weed seeds thus increasing weed seed bank density and diversity (Pleasant and Schlather, 1994). Even though composting of manure and other materials can reduce weed seed viability, this can highly vary depending on the species and the process (Larney and Blackshaw, 2003). Therefore, with manure treatments, new difficult-to-control weeds might be introduced in some situations (Cordeau et al., 2021). Organic fertilizers and amendments typically release nutrients (especially N) at a slower rate compared with mineral fertilizers (Magdoff, 1995). Faster nutrient release from mineral fertilizer is often advantageous to weeds, which are usually able to take up nutrients in earlier growth stages more quickly and efficiently than crops (Jørnsgård et al., 1996; Liebman and Davis, 2000). Although slower nutrient release from organic sources suppresses the weed’s competitive ability to a certain degree (Paul and Beauchamp, 1993; Liebman and Davis, 2000), the occurrence of late season weed emergence flushes that contribute to seed bank replenishment and consequently to higher weed seedling recruitment in subsequent years can be aided by organic fertilizers. Further, appropriate timing of N fertilizer application is vital in integrated cropping nutrient management systems to unbalance nutrient competition between crops and weeds to the benefit of the former.
Increasing the functional diversity of crop rotation found to be an effective strategy in weed management (Weisberger et al., 2019; Benaragama et al., 2022), thus can be an essential strategy to manage weeds from a long-term perspective in both conventional and organic systems. Increasing crop rotational diversity exerts stresses and mortality factors at different stages of a weed life cycle (Liebman and Staver, 2001; Teasdale, 2018). During a rotation, cropping sequences impose different degrees of resource competition, allelopathic interference, soil disturbance, and mechanical damages that destabilize the environment for the proliferation and dominance of a particular weed (Liebman and Davis, 2000). Long-term crop rotations with functional diversity among crops are not common in rice-based cropping systems in Sri Lanka. However, crop rotation effects on weed dynamics can be more profound in low-land rice-based cropping systems due to alternate wetting and drying cycles. In irrigated or rainfed lowlands, maintaining submerged conditions suppresses weed germination resulting in low weed abundance in inundated rice fields (Moody et al., 1986). However, aquatic, and semiaquatic weeds can take the advantage of moisture-inundated conditions. Upland crops, which are strictly defined as no surface water accumulations, hence generate aerobic conditions that favor weed seed germination and growth (Jayawardane and Weerasena, 2001). A rotation between upland and lowland conditions benefits from changing environmental conditions and utilizing existing conditions and resources in harmony (Berzsenyi et al., 2000). On top of direct effects on weed germination, emergence and growth, alternate upland and low land crop rotation can influence nutrient dynamics which either can favor, or disfavor weed proliferation.
In Sri Lanka, low-land rice-rice rotation is the most predominant while upland crops such as maize (Zea mays L), and pulses are grown typically in certain regions in the minor dry (Yala) season due to low water availability thus can contribute to differential weed dynamics. Understanding the cropping systems perspective of weed dynamics is vital as crop rotations, nutrient management, crop management practices and seasonal weather collectively determine weed dynamics. Yet, the interaction of different nutrient management and upland and lowland crop rotations in rice-based systems on weed dynamics has seldom been studied. Understanding this interaction is vital to devise an optimized weed management strategy for organic and integrated nutrient management systems. Such understanding would be essential for effective weed management, particularly in the transition period from conventional to organic or integrated systems. Therefore, we hypothesize that weed dynamics (abundance, growth, and composition) are different under contrasting nutrient management strategies (conventional, integrated, and organic nutrient management systems) and with different crop rotation strategies under rice-based cropping systems in Sri Lanka. The main objective of this study was to assess the weed abundance, growth, and composition during the transitional period from conventional to integrated and organic systems and to evaluate how crop rotation diversity can interactively affect weed dynamics during this transition period.
2 Materials and methods
2.1 Experimental location and treatments
A field experiment was carried out within the farm premises of the Faculty of Agriculture, Rajarata University of Sri Lanka which is located on the agroecological region DL1b at 8°25’18.12” of latitude and 80°24’9.37” of longitude. This area consists of an undulated catenary landscape (Thenabadu, 1988). The study area consists of imperfectly drained Reddish-Brown Earth soils (Soil taxonomic Order-Alfisols, Suborder-Ustalfs, Great Group (Hapludalfs) (Mapa et al., 2010). The study was conducted for two crop rotational cycles, cycle 1; 2018/19 wet (September to February) and 2019 dry (March to August), and cycle 2; 2019/20 wet, and 2020 dry. Seasonal rainfalls across four seasons were 364.6 mm, 181.6 mm, 962 mm, and 621.7 mm; the mean monthly maximum and minimum temperatures were 35.7 °C and 21.6 °C, respectively (Figure 1).
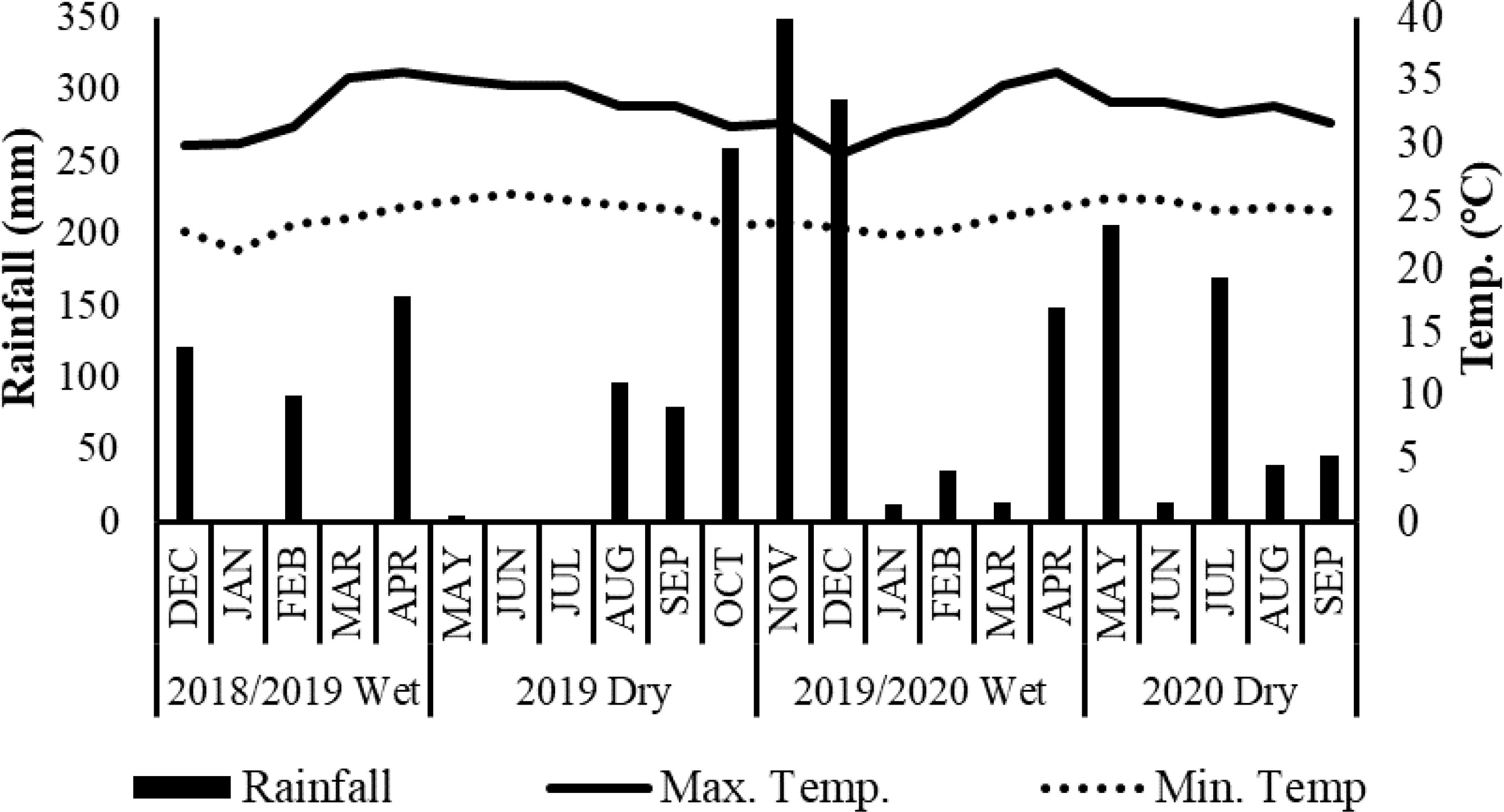
Figure 1 Monthly cumulative rainfall, monthly mean maximum and minimum temperature in the experiment site during 2018/2019 wet, 2019 dry, 2019/2020 wet and 2020 dry seasons.
The experiment consisted of factorially arranged two main treatments 1. Nutrient management system (3 levels) and 2. Crop rotation diversity (4 levels) that gives twelve diverse combinations of cropping systems). The three main nutrient management strategies were, CONV- Conventional nutrient management system-100% N applied as mineral fertilizer application based on recommended by the Department of Agriculture (DOA) 2013, INT-Integrated nutrient management system- 50% N supply with mineral fertilizer and 25% N supply with organic fertilizer, ORG- Organic nutrient management system- no mineral fertilizer was added and organic fertilizer was applied to satisfy the 50% N amount of the conventional system. Organic fertilizers, which N content was calculated to determine the relevant rate to deliver the required N content were added as the main source of nutrients. Nutrient management was defined based on the elemental N supply and the sources. These rates were decided considering the losses of N from urea and organic matter while aiming to provide adequate N for crop growth. The phosphorus and potassium rates were not adjusted based on the DOA 2013 recommendation. The amount of these two elements depended on the amount of organic source used to supply N to both integrated and organic systems (Table 1). Four crop rotation diversity treatments were established covering both wet and dry seasons and inter seasons between wet and dry seasons. The four crop rotations were 1. Monocrop rice (rice-rice), 2. Rice-sunnhemp (Crotalaria juncea)-rice rotation 3. Rice-maize rotation and 4. Rice-sunnhemp-maize rotation. During the study period the crop rotations were carried out for two cycles. In the first cycle, monocrop rice system and rice-maize rotation were established. In the second cycle, those systems were intensified in subsequent seasons as additional experimental units to rice-sunnhemp-rice system and rice-sunnhemp-maize systems (Table 2).
The twelve treatments were established on a split-plot design with three replicates. The three-nutrient managements were allocated in a non-random manner into three blocks due to the need for the physical isolation for irrigation management of the organic system from the two other systems. The four crop rotation diversities (the sub-plot factor) were randomly allocated to each main plot and its replicates. Each main plot was 15 m × 24 m, while the subplot was 15 m × 6 m in size. The total land area of the study was 3240 m2.
The total N content of organic fertilizer was analyzed using the Kjeldahl procedure (Bremner and Mulvaney, 1982). Exchangeable K was determined by the ammonium acetate extraction method (Jackson, 1958). Available P was extracted by 0.5 M sodium bicarbonate solution while the colorimetric method was used to quantify P after Molybdate blue color development in the extraction using UV Visible Spectrophotometer (Model UVD-2960) (Watanabe and Olsen, 1965).
2.2 Crop establishment and management
Land preparation for rice crop during both seasons was done by impounding water after plowing and harrowing with a moldboard plow and disk harrow followed by fine leveling with the help of a wooden leveler. Pre-germinated seeds of rice variety Bg300 (3-month-old variety) were broadcasted at a rate of 120 kg/ha on the prepared plots. For maize cultivation, the land was deeply plowed and prepared flatbed with four sub-plots. The pre-soaked seeds of hybrid Pacific (duration of 110 days) were manually planted at 45 cm x 30 cm spacing, as two seeds per hill at the rate of 12 kg ha-1 following the crop management guidelines (DOA, 2015). Tables 3, 4 show the varied mineral and organic fertilizer applications for rice and maize crops under different nutrient management systems.
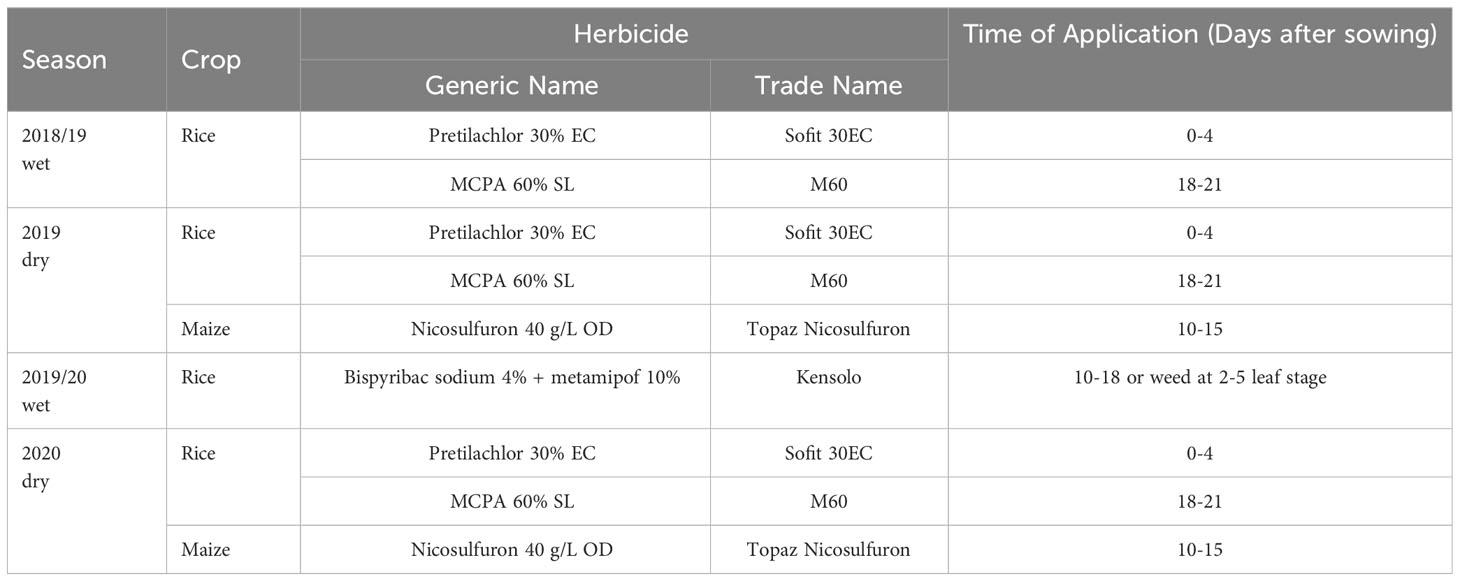
Table 3 Herbicide applications in CONV and INT during 2018/2019 wet, 2019 dry, 2019/2020 wet, and 2020 dry.
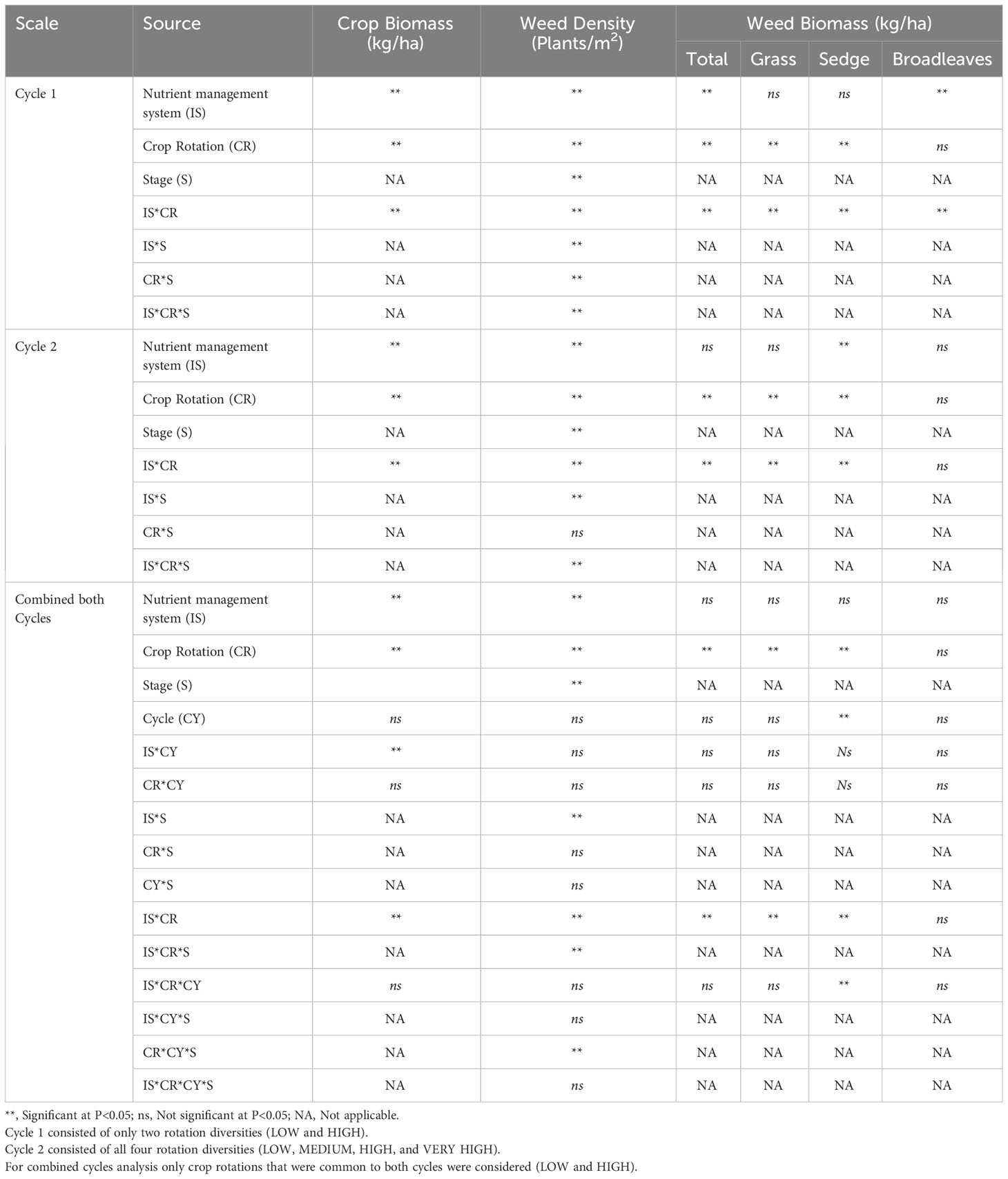
Table 4 Effect of nutrient management systems, crop rotation diversity, cropping cycle, growth stages, and their interactions on crop biomass, weed density weed biomass.
In low-land rice cultivation inundation of land with water (flood irrigation) has considered one of the main weed control strategies besides herbicides. In addition to this, post-emergence weed management was carried out in CONV and INT using post-emergence herbicide application rates recommended by the Sri Lanka Department of Agriculture, avoiding high rainfall and windy conditions (Table 3). An adjustable single-nozzle knapsack sprayer was used for herbicide application, and the herbicide was carefully administrated ensuring no difference between CONV and INT (Table 3). Weed control of maize crops was mainly carried out using post-emergence herbicides for the CONV and INT systems (Table 3). Weeds were maintained in organic systems by practicing manual weeding concurrently with chemical weed management. The irrigation was done one week after the seed sowing and impounded with a 5 cm depth of water to keep soil sufficiently moist throughout the rice cultivation period. The flood irrigation method was followed to keep the soil moist for rice at regular intervals for all three systems. Irrigation was done for two weeks for maize cultivation using flooded irrigation. The drains were opened about 1–2 hours after the flooding of the plot. Deep drains were placed around and in the middle of the plot to minimize flood damage due to heavy rain.
2.3 Data collection
To assess total weed density, four randomly selected locations within a single plot were chosen using a 0.5 m by 0.5 m quadrate at the seedling stage of rice and the five-leaf stage of maize [20 Days after sowing (DAS)]. The weed dry weight was measured separately for grasses, sedges, and broad leaves at the heading stage of rice and tasseling stage of maize (60 DAS). All weeds within each quadrate were uprooted, cleaned, and identified by type. The above-ground parts were air-dried for two days, followed by oven-drying at 60°C until a constant weight was achieved.
To collect rice yield samples during wet and dry seasons, as well as sunnhemp samples during interseason, four random sampling points within each plot were selected at the harvesting stage using a 0.5 m by 0.5 m quadrate. All rice and sunnhemp plants within each quadrate were used to measure crop biomass. For maize, twelve crops per plot were randomly selected at the harvesting stage to measure crop biomass. All crop plants were air-dried at room temperature for two days and then individually placed into paper bags for oven-drying at 60°C until a constant weight was achieved. The shoot dry weight of each sample was recorded by an electric balance and expressed as kg ha-1.
2.4 Statistical analysis
Data from four seasons were analyzed to identify the impact of different nutrient management, crop phase, cropping season, and growth stages on weed dynamics during the crop period. Due to the difference in crop rotation diversities in the two cycles, the data were analyzed at different temporal scales of the experiment. First the data from each cycle were analyzed separately and secondly an additional analysis was carried out by combining the data of the two cycles for the two rotations (monocrop rice and Rice-maize) since it was common to both cycles. Data were statistically analyzed using the SAS computer program version 9.0 (SAS Institute, 2011). Seasonal weed data were tested for normality and heteroscedasticity. The data were log-transformed to maintain the homogeneity and normality of residuals. Analysis of variance (ANOVA) was carried out using the repeated measures MIXED model using Proc MIXED (SAS Institute, 2011). Fixed variables were nutrient management system, crop rotation, and growth stage. The random factor was the block and subplot by block interaction, while the season was considered the repeated factor. The appropriate covariance structure for the model was selected using AIC (Akaike’s Information Criterion). The means were separated using the least significant difference (LSD) method at the 5% probability level. The means were compared within each growth stage using the slice option in the MIXED procedure in SAS.
3 Results
3.1 Weather
The two major growing seasons had different weather conditions. The wet (major) season received approximately 65% more rainfall than the dry (minor) season. The highest rainfall was received during the 2019/2020 wet, and the lowest was recorded during the 2019 dry season. The monthly mean maximum temperature for all four seasons was 35–36°C. The monthly mean minimum temperature was recorded as 25°C in the dry season and around 22°C in the wet season (Figure 1).
3.2 Crop biomass
The two-way interaction of the nutrient management system and crop rotation showed a significant effect on total crop biomass (crop biomass of all the crop phases in the rotation) in both cycles 1 and 2 (Table 4). During the first crop rotation cycle, The CONV and INT nutrient management systems under the monocrop rice diversity rotation had 62% and 76% greater total crop biomass compared with the ORG system, respectively. All three nutrient management systems in rice-maize rotation resulted in significantly lower crop biomasses compared with monocrop rice rotation, while the reduction was 6% (CONV), 28% (INT), and 25% (ORG). The ORG-rice-maize cropping system recorded the lowest crop biomass of 8411 kg ha-1 (Figure 2A) indicating lower productivity.
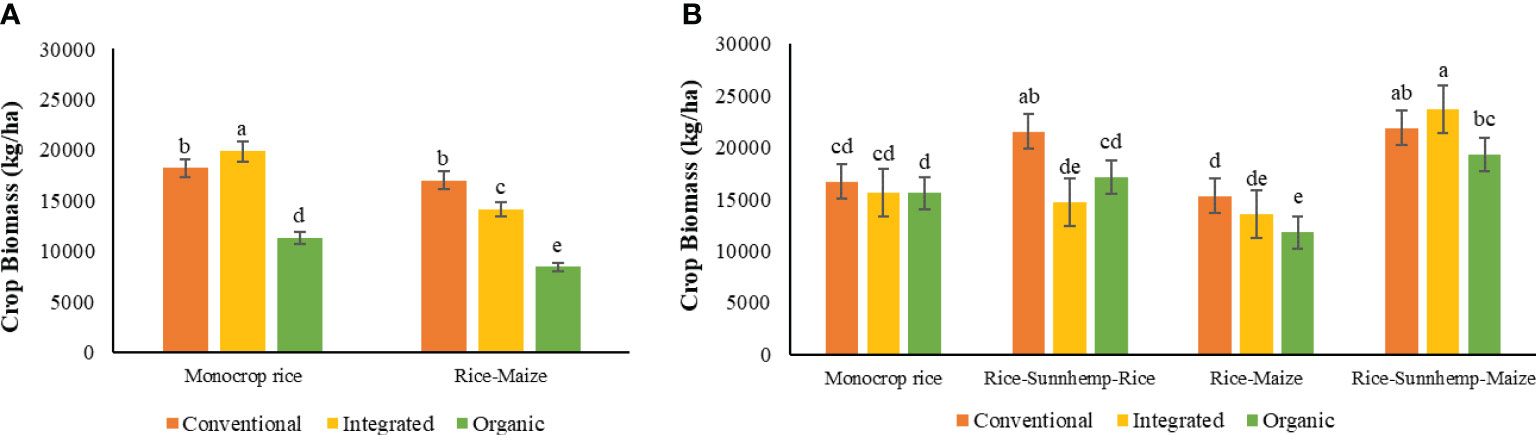
Figure 2 Effect of nutrient management systems, crop rotation diversity and their interaction on crop biomass at harvesting stage of (A) 1st cropping cycle and (B) 2nd cropping cycle [The same letters in the graph are not significantly different at P<0.05 (LSD)].
During the second rotation cycle, there were no differences in total crop biomass among nutrient management systems in the monocrop rice diversity rotation. In the rice-sunnhemp-rice and rice-maize diversity rotations, the CONV nutrient management system had a greater crop biomass (26% and 30%, respectively) compared to the ORG. But ORG had similar biomass productivity to that of the INT system. Interestingly, in the most diverse crop rotation (rice-sunnhemp-maize), ORG had similar total crop biomass to that of CONV, but the INT system had 23% greater crop biomass productivity than the ORG system) (Figure 2B).
Data analysis from the combined cycles revealed nutrient management system by cycle interaction (Table 4). In the 1st cycle, CONV and INT nutrient management systems (average of both monocrop rice and rice-maize diversity) had similar crop biomass and were 69% and 76% higher compared with ORG. During the 2nd cycle, ORG had similar biomass to INT, but 15% lower compared to the CONV system. The biomass of the ORG system was increased by 39% in the ORG nutrient management system from 1st cycle to 2nd, while in contrast, the biomass of the INT nutrient management system from cycle 1 to 2 was decreased by 16% (Figure 3). Furthermore, there was a significant nutrient management system by crop rotation diversity interaction for total crop biomass when the data of two rotations (monocrop rice and rice-maize) in the two cycles were combined for analysis. The monocrop rice rotation of the CONV (26%) and INT (34%) system recorded higher total biomass than ORG, while the rice-maize rotation of the CONV recorded higher total biomass than INT (8.5%) and ORG (35%). Despite similar biomasses in CONV input system in monocrop rice and rice-maize rotations, both INT and ORG input systems reported significantly lower biomasses in rice-maize rotation compared with the monocrop rice rotation (Figure 4).
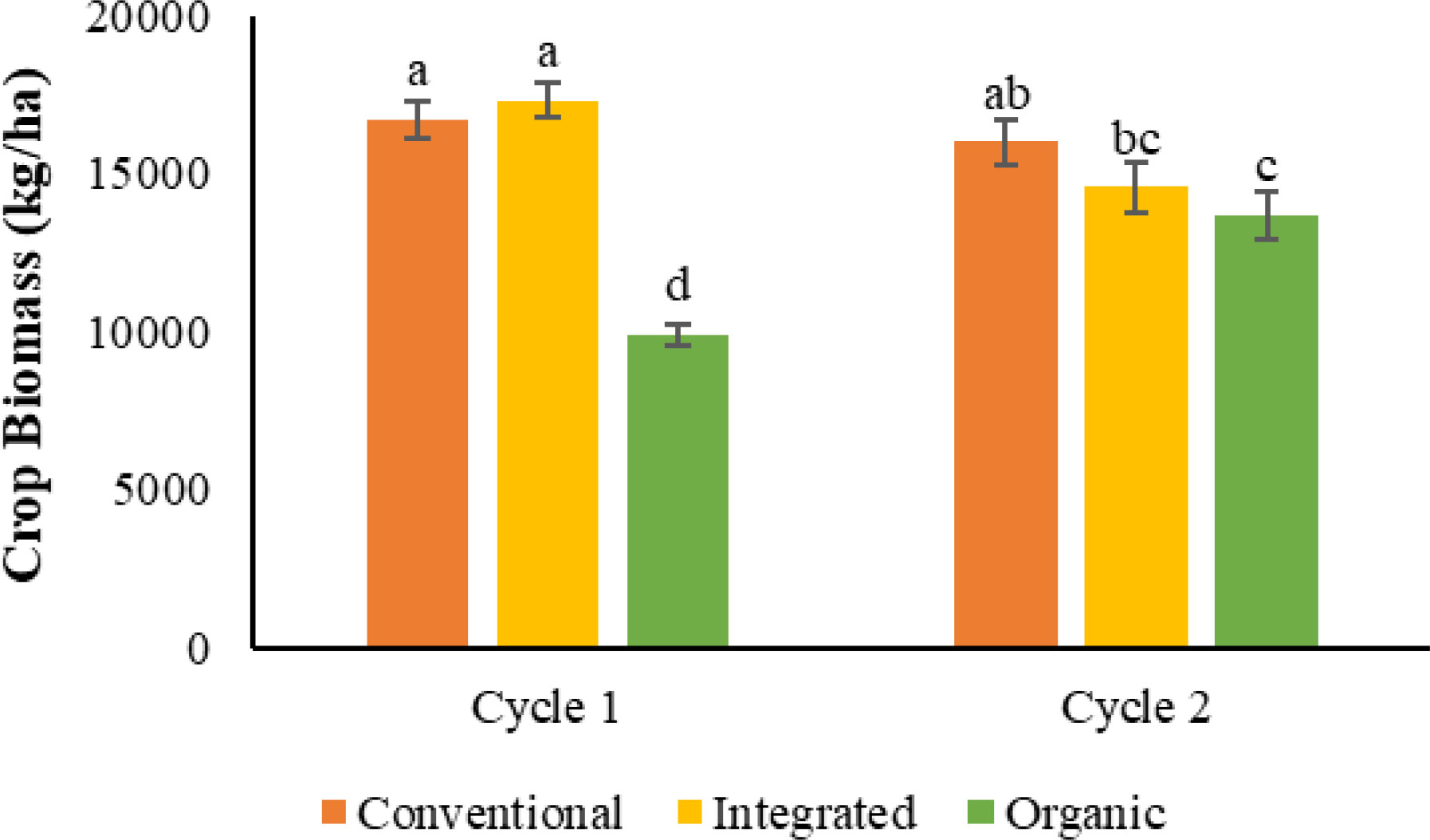
Figure 3 Effect of nutrient management system and cycle on crop biomass at the harvesting stage of both cycles [The same letters in the graph are not significantly different at P<0.05 (LSD)].
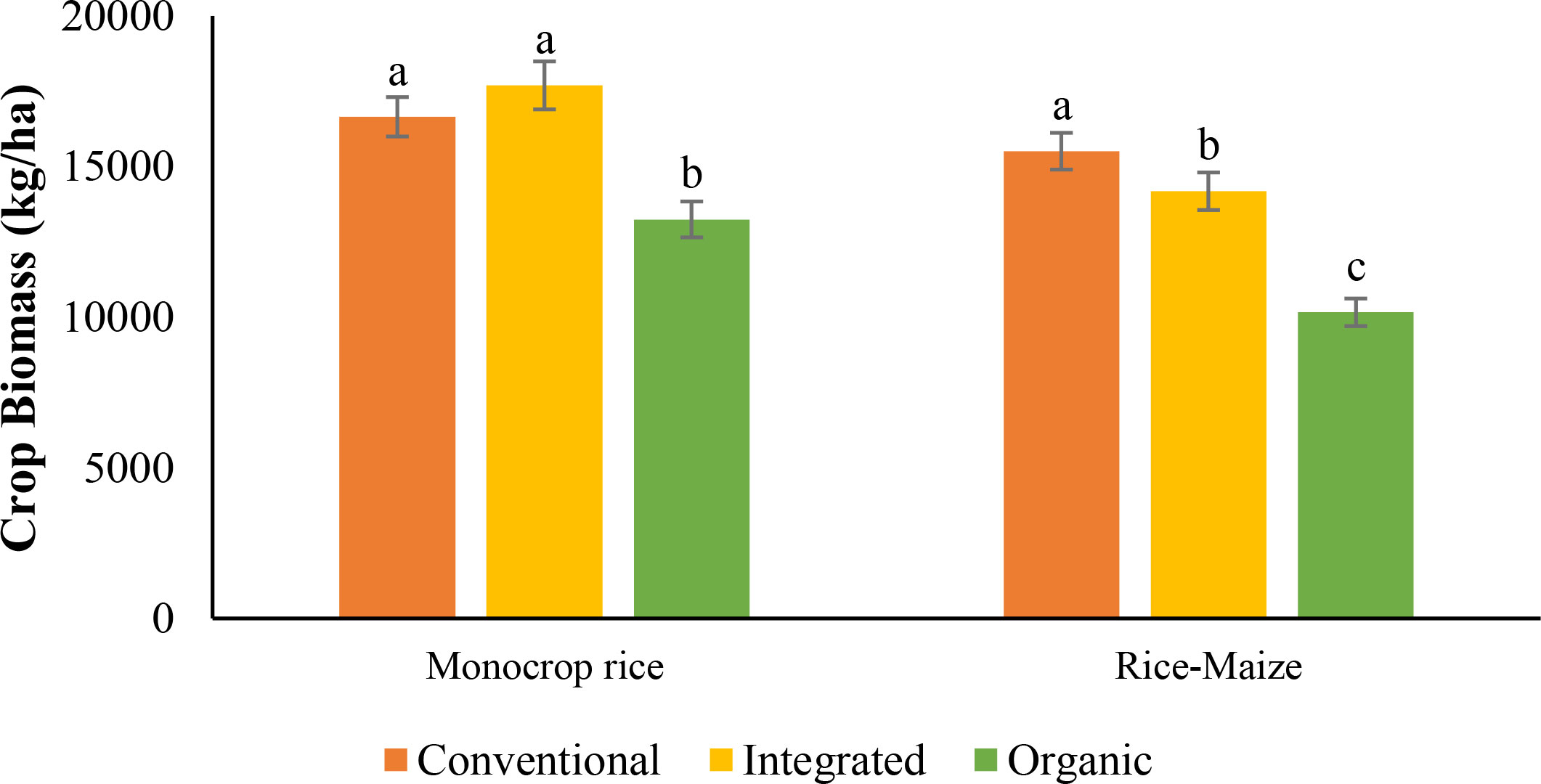
Figure 4 Effect of nutrient management system and crop rotation on crop biomass at the harvesting stage of both cycles [The same letters in the graph are not significantly different at P<0.05 (LSD)].
3.3 Total weed density
The three-way interaction of the nutrient management system, crop rotation, and crop growth stage significantly affected the total weed density of cycles 1 and 2 (Table 4). During the 1st cycle, ORG-monocrop rice system recorded substantially higher weed density which was two-fold higher than the ORG-rice-maize system at 20 DAS. In contrast, no difference in weed density was identified among CONV and INT rotations systems at 20 DAS. At 60 DAS, a significantly higher weed density was recorded in rice-maize rotation than in monocrop rice rotation irrespective of the nutrient management system (Figure 5A). Sedges weeds were very high in other nutrient management systems with monocrop rice and rice-maize crop rotation, except for the ORG system with rice-maize crop rotation in the first crop cycle. That system recorded a relatively higher grass density.
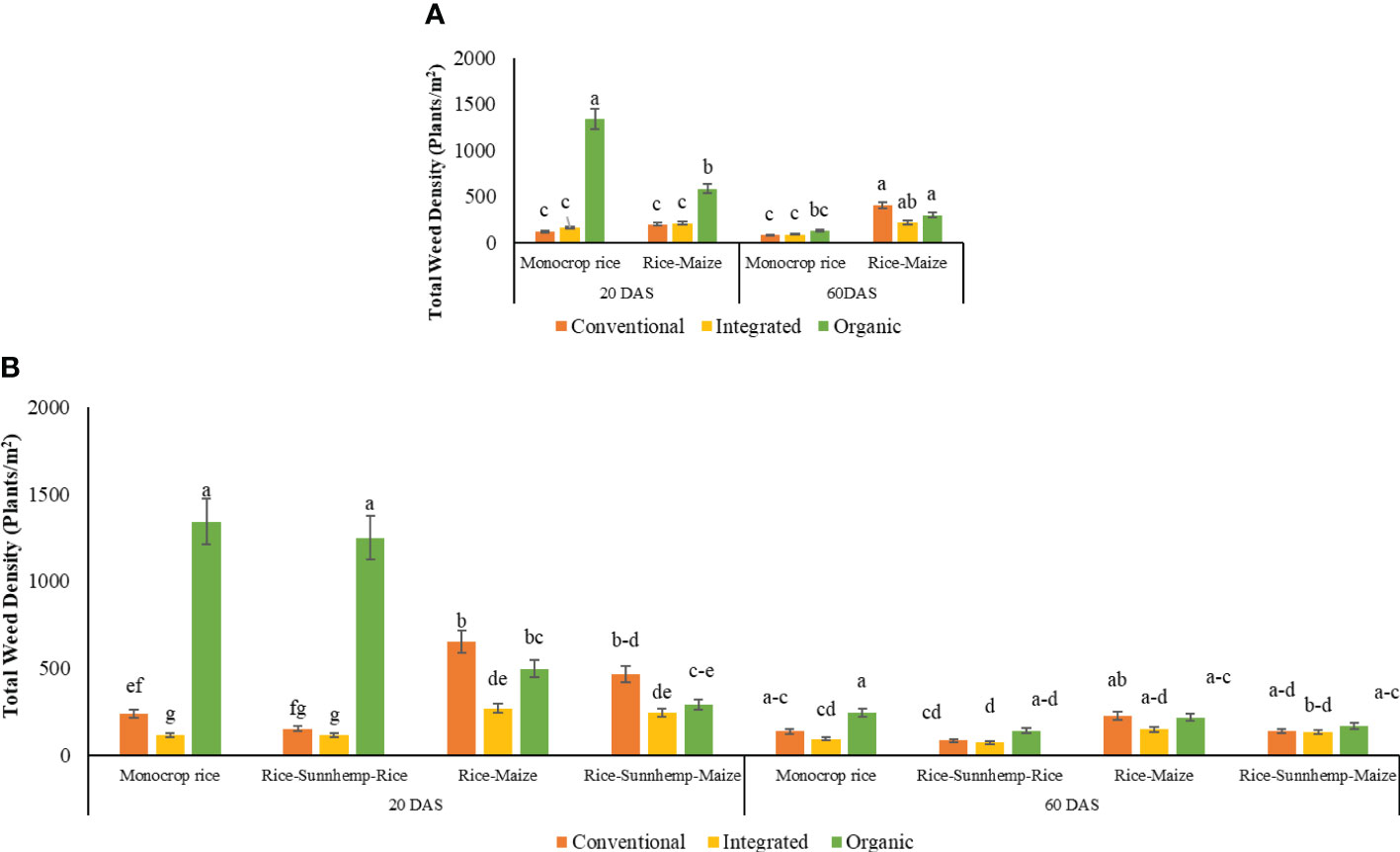
Figure 5 Effect of growth stages, nutrient management, and crop rotation diversity on total weed density under (A) 1st cropping cycle and (B) 2nd cropping cycle [The same letters within each DAS are not significantly different at P<0.05 (LSD), mean separation was sliced by DAS].
During the 2nd cropping cycle, the total weed density at 20 DAS was the highest in the ORG-monocrop rice system and ORG-rice-sunnhemp-rice system, which was 1344 weeds m-2 and 1251 weeds m-2, respectively. The CONV-rice-maize system recorded the second-highest weed density, which was similar to the weed density of the ORG-rice-maize and CONV-rice-sunnhemp-maize cropping systems. Weed densities were lowest in INT-monocrop rice, CONV-rice-sunnhemp-rice, and INT-rice-sunnhemp-rice cropping systems with a density ranging from 115-150 weeds m-2. Interestingly, ORG systems had low weed density in rice-maize and rice-sunnhemp-maize rotations compared to monocrop rice and rice-sunnhemp-rice rotation diversity. There were no differences in weed density between rice-maize and rice-sunnhemp-maize rotations for all three nutrient management systems. At 60 DAS, total weed densities were lower than 20 DAS with a range of 72–245 weeds m-2. The total weed density at 60 DAS did not show any differences among nutrient management systems in the rice-sunnhemp-rice, rice-maize, and rice-sunnhemp-maize rotations. However, at monocrop rice crop rotation diversity, weed density in ORG was higher compared with the INT system (Figure 5B). The INT system with monocrop rice system, the CONV and INT system with rice-sunnhemp-rice crop rotation, and the ORG system with rice-maize crop rotation diversity recorded more than 50% of the grass density from the total weed density. Other systems reported more sedges than other weeds.
When the data of the two rotations (monocrop rice and rice-maize) were combined across the two cycles (combine analysis, Table 4) ORG-monocrop rice and ORG-rice-maize systems had greater weed density at 20 DAS compared to all other cropping systems. Increasing the rotation diversity from monocrop rice to rice-maize substantially decreases weed density in ORG system. In contrast, it increased the weed density in CONV and no change in INT system. The total weed densities at 60 DAS were low compared to 20 DAS irrespective of the nutrient management systems. At 60 DAS, ORG-monocrop rice cropping system had 48% higher density compared to INT-monocrop rice cropping system but was similar to CONV-monocrop rice cropping system. Interestingly, there were no differences in weed densities at 60 DAS, among nutrient management systems at rice-maize rotation diversity (Figure 6A).
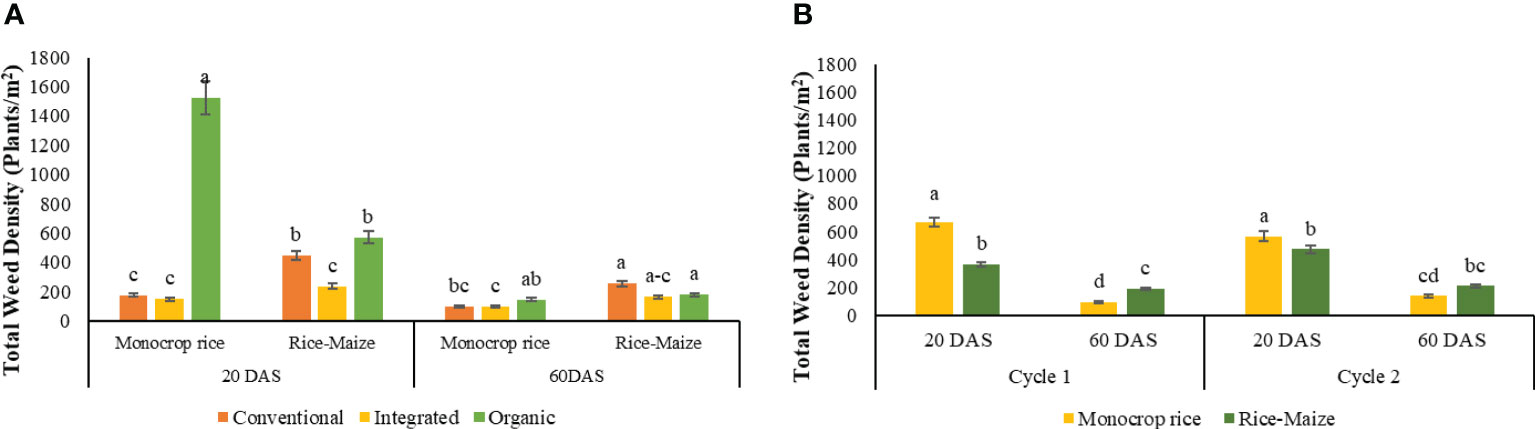
Figure 6 Total weed density of combined cycles as the effect of (A) nutrient management, crop rotation, and growth stages [The same letters within each DAS are not significantly different at P<0.05 (LSD), mean separation was sliced by DAS] (B) crop rotation, cycle and growth stage.
There was a cycle by crop rotation by stage interaction for weed density (Table 4). The highest weed density was observed in the monocrop rice diversity rotations, in both cycles at 20 DAS. Again, the densities declined from 20 DAS to 60 DAS in both cycles. Despite a significant difference between monocrop rice and rice-maize rotations in the 1st cycle at 60 DAS, no significant difference was observed between monocrop rice and rice-maize rotations in the 2nd cycle (Figure 6B).
3.4 Total weed biomass
During the 1st cycle, weed biomass was significantly influenced by the two-way interaction of nutrient management and crop rotation (Table 4). Within monocrop rice rotation, weed biomass was significantly low (31%) in the ORG compared with CONV while the INT had intermediate weed biomass. rice-maize rotation in all nutrient management systems recorded noticeably higher weed biomass than monocrop rice diversity rotation (Figure 7A) Under rice-maize rotation, the INT system had 25% and 40% low weed biomass compared with CONV and ORG nutrient management systems. Interestingly, weed biomass in the ORG system was similar to the CONV system under rice-maize crop rotation diversity (Figure 7A). Grass biomass had the highest effect on total weed biomass in all systems.
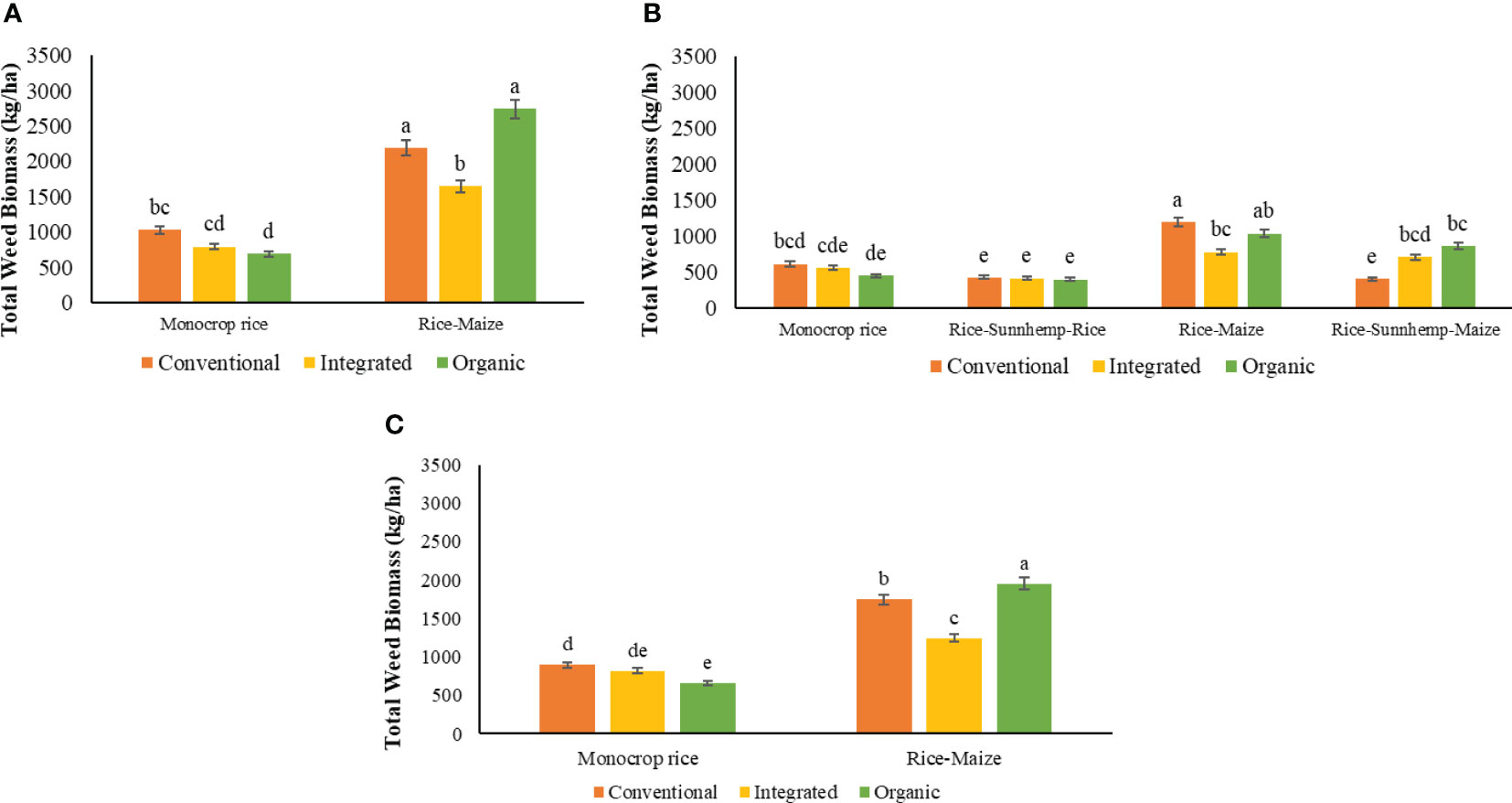
Figure 7 Effect of nutrient management systems, and crop rotation diversity on total weed biomass assessed at 60 DAS under (A) 1st cropping cycle, (B) 2nd cropping cycle, and (C) combined both cycles [The same letters in each graph are not significantly different at P<0.05 (LSD)].
During the 2nd cycle, there were no differences in weed biomass among nutrient management systems under both monocrop rice and rice-sunnhemp-rice rotations. Under rice-maize rotation, INT nutrient management system had 35% low weed biomass compared with CONV. The ORG nutrient management system had intermediate weed biomass. Under rice-sunnhemp-maize diversity, the CONV nutrient management system had the least weed biomass and was 43% and 53% compared with INT and ORG respectively (Figure 7B). The INT system with monocrop rice system, the CONV and INT system with rice-sunnhemp-rice crop rotation, and the ORG system with rice-maize crop rotation diversity recorded more grass biomass from the total weed density. Other systems reported more sedges biomass than other weeds biomass.
The combined analysis of the data from two cycles for monocrop rice and rice-maize diversity rotations revealed that there is an interaction between nutrient management systems by crop diversity (Table 4). The monocrop rice rotation was found to have lower total weed biomass compared to rice-maize rotation in all three nutrient management systems. The ORG nutrient management system had 27% low weed biomass compared to CONV nutrient management system under the monocrop rice rotation, however, under the rice-maize diversity rotation, ORG showed 12% and 57% greater weed biomass compared to both CONV and INT systems. The INT nutrient management system had the lowest weed biomass under the rice-maize diversity rotation (Figure 7C).
3.5 Weed composition
3.5.1 Grass biomass
A significant crop rotation and nutrient management system interaction was identified in grass biomass in both cycles (Table 4). Isachne globose was the most commonly identified and highly contributed grass type in both rice and maize fields. Echinochloa glabrescens, Ischaemum rugosum, and Echinochloa colonum were also identified throughout the cropping period.
There was no difference in grass biomass among nutrient management systems under the monocrop rice rotation in 1st cycle. In the rice-maize rotation diversity, grass biomass was greater in CONV (124%) and ORG (93%) systems compared with INT system (Figure 8A). As observed in the 1st cycle, there was no difference in grass weed biomass among nutrient management systems in the monocrop rice diversity rotation in the 2nd cycle. The ORG-rice-sunnhemp-rice system had 71% low grass biomass compared to CONV-rice-sunnhemp-rice system. However, the grass biomass in the ORG system was similar to the CONV system in the rice-maize and rice-sunnhemp-maize rotations. INT system always found to have similar grass biomass to the CONV system in all rotations (Figure 8B). There was no difference identified among nutrient management systems for grass biomass under the rice-sunnhemp-maize rotation.
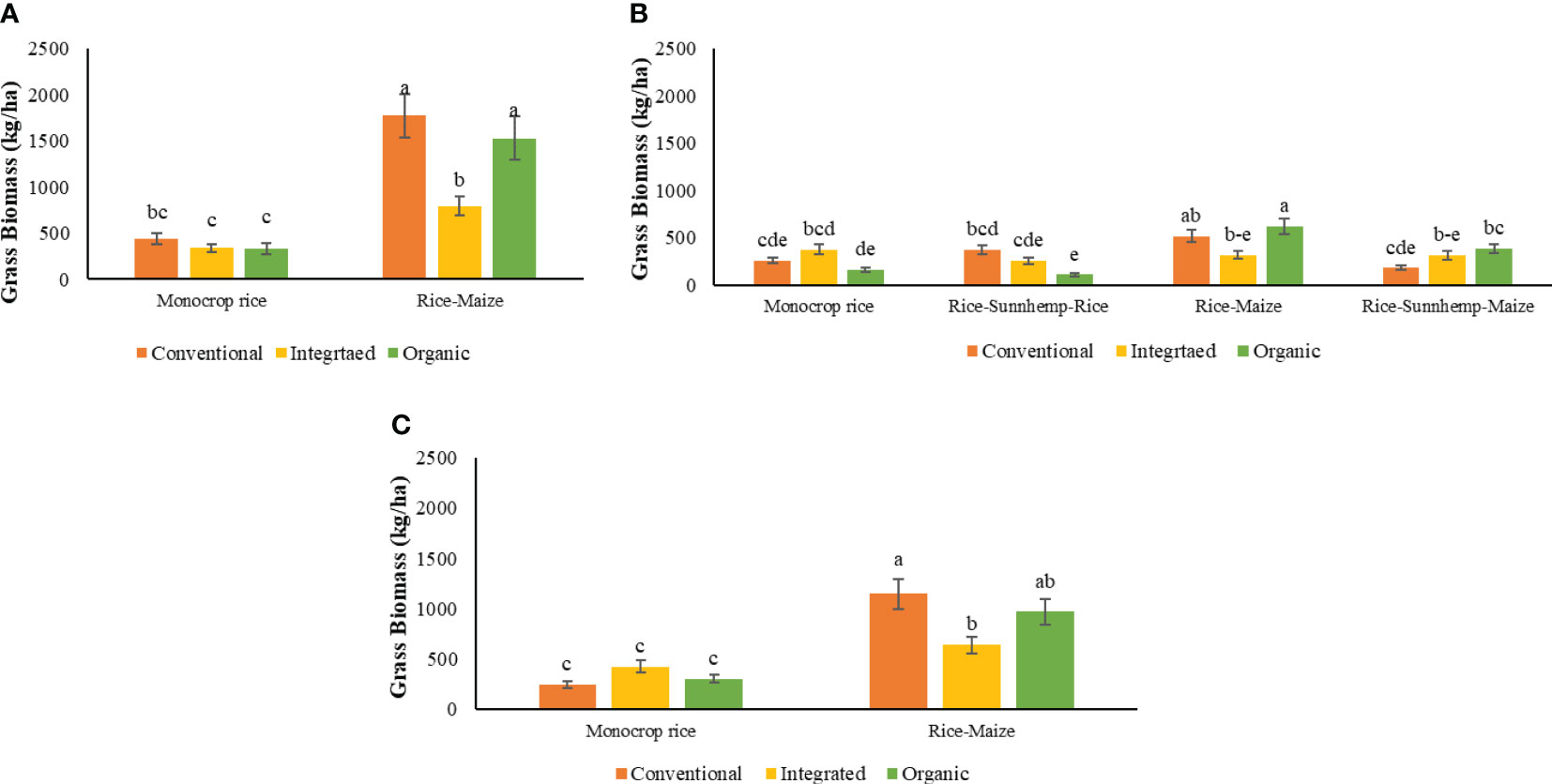
Figure 8 Effect of nutrient management systems, and crop rotation diversity on grass biomass under (A) 1st cropping cycle, (B) 2nd cropping cycle, and (C) combined both cycles [The same letters in each graph are not significantly different at P<0.05 (LSD)].
When monocrop rice and rice-maize diversity rotations in both cycles were combined for the analysis, the grass biomass was influenced by the interaction of the nutrient management system and crop rotation (Table 4). There was no difference in grass biomass among nutrient management systems under the monocrop rice rotation. All nutrient management systems had greater grass biomass in the rice-maize rotation compared with monocrop rice rotation with the 5-fold (CONV), 1.5-fold (INT), and 3-fold (ORG) growth increase compared with the monocrop rice rotation. Within rice-maize rotation, the INT system had 44% lower grass biomass compared to CONV but similar grass biomass to ORG systems which had intermediate grass biomass (Figure 8C).
3.5.2 Sedge biomass
The sedges biomass in crop rotation cycles 1 and 2 varied significantly due to the two-way interaction of crop rotation and nutrient management systems (Table 4). Cyperus rotundus was the most prominent sedge in both crops. Cyperus iria, Fimbristylis dichotoma, and Fimbristylis miliaceae were sedges observed during the research period. Even if herbicides were applied, the regenerative part of the underground tuber of Cyperus rotundus was not killed and grew back under favorable conditions (Data not shown).
The sedge biomass in the three nutrient management systems in the monocrop rice rotation were statistically similar in both cycles (Figures 9). In the monocrop rice diversity rotation, sedge biomass was different among nutrient management systems where ORG had the highest and CONV had the lowest biomass. INT-rice-maize and ORG-rice-maize systems resulted in 80% and 270% greater sedge biomass than the INT-monocrop rice and ORG-monocrop rice systems. (Figure 10A).
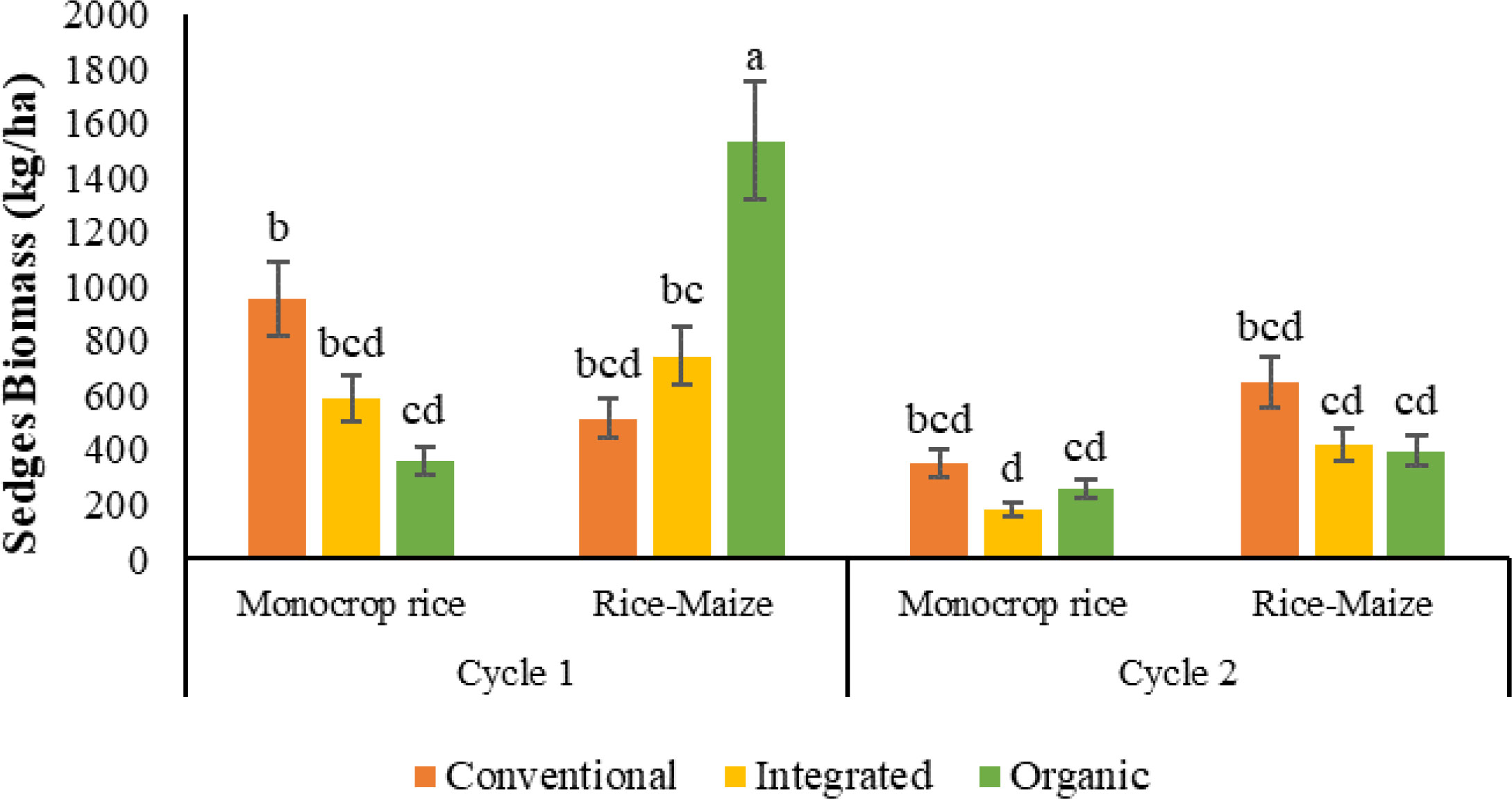
Figure 9 Sedge biomass of combined cycles as the effect of the nutrient management system, crop rotation, and cycle [The same letters in the graph are not significantly different at P<0.05 (LSD)].
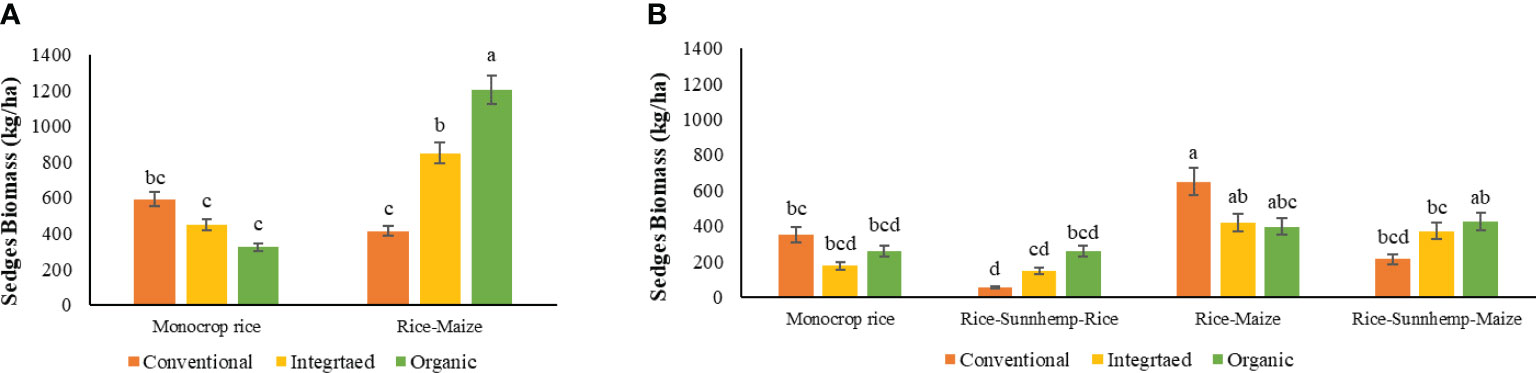
Figure 10 Effect of nutrient management systems, and crop rotation diversity on sedges biomass under (A) 1st cropping cycle, (B) 2nd cropping cycle [The same letters in each graph are not significantly different at P<0.05 (LSD)].
The sedge biomasses were similar among nutrient management systems within all four rotation diversities during the 2nd cycle. When compared within nutrient management systems across different crop rotation diversities, there were no changes in sedge biomass from monocrop rice to rice-sunnhemp-maize rotations for both ORG and INT systems. However, in CONV, it was found that in the rice-maize rotation, sedge biomass increased substantially compared with all other three rotations (Figure 10B).
When combined in both cycles, the cropping cycle, nutrient management system, and crop rotation interaction significantly affected the sedge biomass (Table 4). In the monocrop rice diversity rotation, ORG system had the least sedge biomass during the 1st cycle, and the rice-maize rotation ORG recorded the significantly highest sedges biomass, indicating an increased abundance of sedges with alternate upland and lowland rotation. No such changes were observed for INT and CONV systems. However, during the 2nd cycle, all nutrient management systems in each crop rotation reported similar sedge biomass (Figure 9).
3.5.3 Broadleaf biomass
There was a nutrient management system by crop rotation interaction for broadleaf weed biomass in the 1st cycle (Table 4). The most common broadleaves weed was Marsilea crenata. Other than that, Scoparia dulcis and Aeschynomene indica were also identified in a minute density (Data not shown). The highest biomass was reported in the ORG-monocrop rice cropping system with a biomass of 34 kg/ha and was reduced to 16 kg/ha in cycle 2. The lowest was recorded in monocrop rice rotation in the CONV system with 2 kg/ha, which was similar to the CONV and INT system with both crop rotations (Figure 11). However, variations of broadleaves biomass were not fixed when compared to grass and sedges density and biomass.
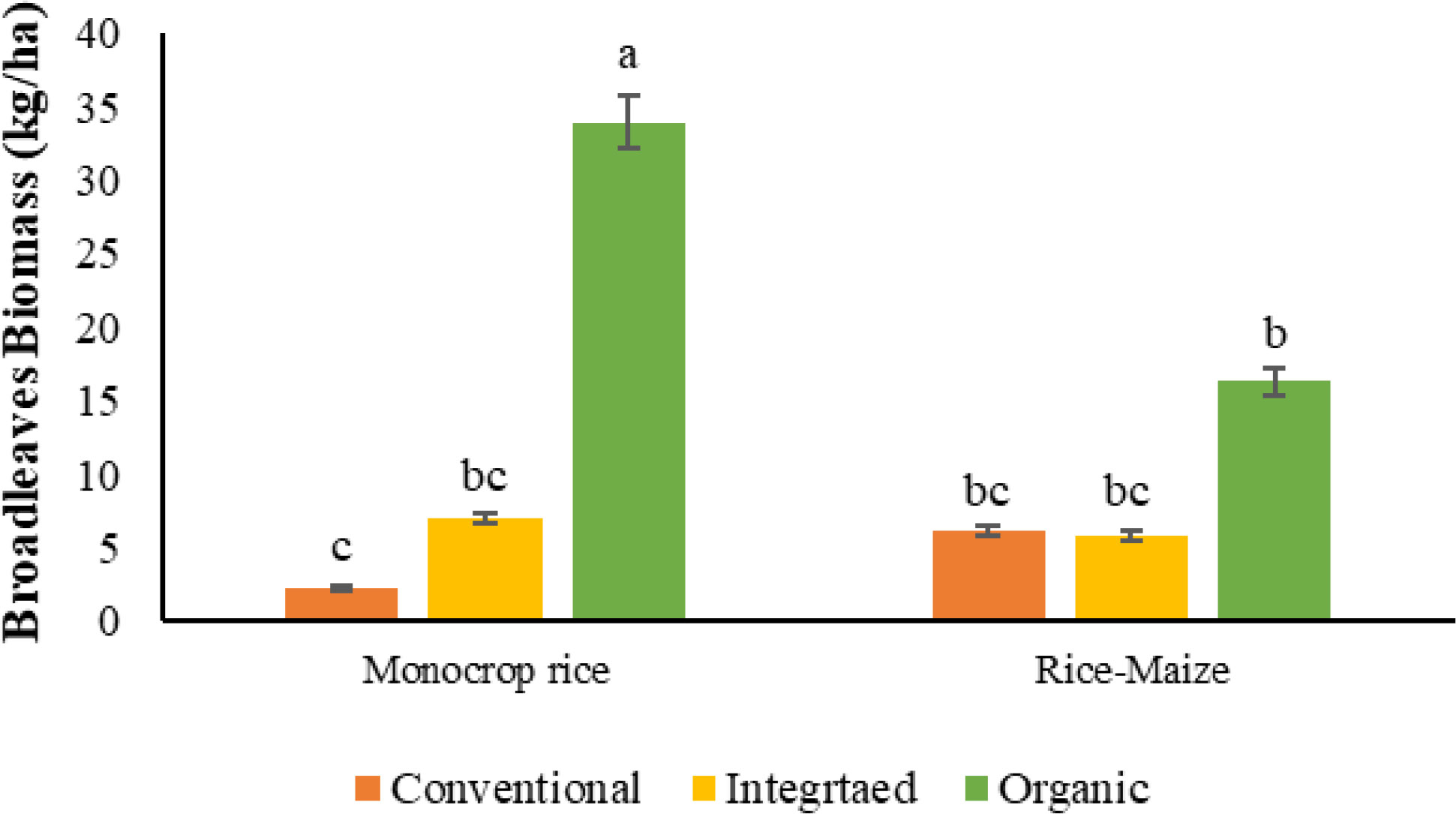
Figure 11 Broadleaves biomas of 1st cycle as effect of nutrient management system, and crop rotation [The same letters in each graph are not significantly different at P<0.05 (LSD)].
4 Discussion
This study revealed that the nutrient management system and crop rotation diversity interactively determined the weed dynamics (weed abundance, biomass, and composition) in rice-based cropping systems. Further, these effects changed from the first cycle to the second cycle indicating the temporal effects of the cropping systems on weeds. During the early stage of the transition, weed density tends to be high in ORG-monocrop rice cropping system at 20 DAS. This is typically related to the lack of effective weed control after the seeding of the rice crop in ORG (Verschwele and Zwerger, 2005) compared with the other two systems, which used post-emergence herbicides to control weeds. This agrees with Barberi et al. (1998), who observed almost four times more weeds in an organic maize system than in a conventionally managed system. Increasing the rotation diversity from monocrop rice to rice-maize (having an upland maize crop in the second season) significantly reduced weed density in ORG systems during 20 DAS in both cycles and overall (combined cycles). This indicates that the changes from rice-rice to rice-maize had created a different environmental condition for weeds. Monocropping of rice with sufficient moisture drove to higher weed density in the ORG than rice-maize crop rotation, which alters the anaerobic to aerobic conditions with rice-maize rotation. The study by Opeña et al. (2014) also revealed that the rice-maize crop rotation created lower weediness than the long-lasting monoculture pattern due to alteration in favorable conditions for weeds in the preceding season. Furthermore, increasing the crop rotation diversity from monocrop rice to rice-sunnhemp-maize substantially reduced the weed density in ORG system. The reduction in weed densities in rice-sunnhemp-maize diversity could be due to sunnhemp cultivation in the previous interseason replacing the fallow period which can contribute to the reduction of weed density by reducing the weed seed bank (Tatenda et al., 2021).
In contrast to ORG system, increasing the crop rotation diversity from monocrop rice to rice-maize and monocrop rice to rice-sunnhemp-maize increased weed density in both CONV and INT systems. This could be due to the reduction in the use of water management as a weed control strategy as an additive weed control tool in rice-rice rotation compared with rice-maize rotation. Even with herbicides, flooding with water at an early stage is a highly effective weed control tool (Williams et al., 1990; Rao et al., 2017). The lack of difference identified between ORG and the other two systems in weed densities in the latter growth stages of the crop (60 DAS) is due to overall low weed densities in all three nutrient management systems. The total weed densities of all nutrient management systems in 60 DAS were lower compared to the weed densities in 20 DAS due to the canopy development and increased crop competitiveness when plants reach maturity at 60 DAS. During this stage, crops are greater competitors for nutrients and space. Both rice and maize crops were able to suppress weed growth by restricting radiation availability with canopy development of the crop and being able to outcompete weeds for growth requirements (Mhlanga et al., 2016). The rice-maize diversity rotation in all nutrient management systems recorded higher total weed density compared with the monocrop rice rotation at 60 DAS in the 1st cycle and combined cycle. The changes in water management (Jayawardane and Weerasena, 2001) and other agronomic differences, especially the high inter-row spacing in maize than in rice might have increased the weed density in rice-maize rotation than the monocrop rice rotation.
In accordance with what was observed in weed density, weed biomass (at 60 DAS) was greater in the rice-maize diversity rotation compared with monocrop rice diversity rotation for all three nutrient management systems. This can be due to two reasons. First, the overall crop biomass productivity tends to be lower in the rice-maize diversity compared to monocrop rice diversity rotation. Secondly, the difference in water management as a weed control tool could have been a key factor in better weed management in monocrop rice diversity rotation. However, further diversifying crop rotation to include a sunnhemp crop was found to have a substantial benefit for weed management particularly to CONV system. Weed suppression by sunnhemp has been noted by several research with conventional fertilizer application (Taylor et al., 1985; Leather and Forrence, 1990; McSorley et al., 1994; Wang et al., 2001; Adler and Chase, 2007; Collins et al., 2007; Price et al., 2008). The reduction in weed biomass due to sunnhemp in the rotation was more pronounced in the CONV system. This could be probably due to the temporary immobilization of residual N from mineral fertilizers by growing sunnhemp which reduces the availability of N for weeds to thrive in the proceeding season. The impact of sunnhemp on ORG system could be mainly due to a reduction in weed seed bank addition in contrast to having a fallow phase in the previous interseason. Overall, this study concludes that for all nutrient management systems, rice-rice rotation is the most effective in managing weeds. When upland crops are needed for the rotation, it was found that incorporating a sunnhemp crop as an interseason crop can benefit weed management in such rotations.
In comparing nutrient management systems, this study revealed that ORG system had low weed biomass compared with CONV and INT in the monocrop rice rotation and similar weed biomass in most other rotations. Low weed biomass is in accordance with low or similar weed densities observed in ORG at 60 DAS. Other than differences in weed management among these systems, the low crop biomass productivity identified could be a major reason for lower weed control.
Generally, organic sources release nutrients more slowly than conventional nutrient management, and the instant nutrient releasing ability of conventional nutrient management with minerals often favors the accumulation of biomass in weeds (Jørnsgård et al., 1996; Liebman and Davis, 2000). Despite having similar weed control strategy and similar crop biomass productivity a similar mechanism could explain the reason for low weed biomass in INT system compared with CONV system. The application of mineral fertilizers in relatively large quantities may benefit weeds to a greater extent than crops with more efficient and rapid nutrient absorption mechanisms of weeds compared with crop plants (Balasubramaniyan and Palaniappan, 2003). Furthermore, the organic manures, which were added as a supplement in INT, may have released allelopathic phytochemicals that have the potential to reduce weed emergence and increase weed seed mortality (Hoagland et al., 2008; Abdulla and Kumar, 2014). The results of Ghosh et al. (2020), depicted the application of 50% of the recommended fertilizer through mineral sources and the remaining 50% through organic manure, effectively reducing the weed density and growth, which is almost analog to the current study.
The diverse rice-based cropping systems not only influenced weed abundance and weed growth but had some impact on weed composition as well. Moving from rice-rice rotation to rice-maize rotation was found to increase grass biomass during the first and combined cycles for all three nutrient management systems. This implies that inundation in rice-rice rotation favored grass weed control. The efficacy of management of water depth in managing grass weed density and biomass has also been shown by Williams et al. (1990). Other than the rotation effect, crop nutrient management systems were also found to influence weed composition as grass weeds were greater in CONV and ORG systems compared with INT in the rice-maize rotations. The abundance of grass species in ORG could be due to overall inadequate weed control and manure and plant materials used for compost preparation which can serve as vectors for weed seeds (Liebman and Davis, 2000). However, the INT system reported lower grass biomass due to the lower nutrient availability with the high plant performances (Kruidhof et al., 2008) and the effect of herbicides at initial cropping stages. Changes in crop rotation from rice-rice to rice-maize also increased sedge biomass but it was only prominent for CONV and INT systems.
5 Conclusions
This study revealed that eliminating the use of herbicides for organic crops allows the weeds to thrive well with high weed densities and weed biomasses in the early crop growth stages. Weed densities were high in monocropping rice systems than in rice-maize rotation, particularly at the initial growth stages in all input systems. Weed densities in organic systems during later crop growth stages were approximately similar to the conventional and integrated systems. Generally, crop rotation and integrated nutrient management approach were effective in suppressing weeds, both densities, and biomass, with several effective mechanisms within the system itself. Having an interseason green manure crop in the rotation was effective in suppressing the weeds, particularly in the conventional system. Advantageously, the weed compositional changes with the presence of sunnhemp in organic nutrient management systems effectively reduced the grass biomass. Overall, this study reveals that weed dynamics of rice based cropping systems are dictated by crop rotations and particularly the rotation of upland vs. low land crop. The more pronounced differences identified among nutrient management systems during the first rotation cycle was not prominent during the second cycle where organic systems found to have reduced weed infestation (density and biomass) in the second cycle and this could be attributed to better crop growth in the organic in the second cycle than in the first cycle. The Integrated nutrient management system found to be the best system for managing weeds irrespective of crop rotation diversity.
Data availability statement
The original contributions presented in the study are included in the article/supplementary material. Further inquiries can be directed to the corresponding author.
Author contributions
DW: carried out the experiment, laboratory analysis, data analysis and wrote the manuscript. UD: Financial Handling and reviewing: LS: Supervision and review: CE: conceptualization, designed the experiment supervision, review and editing: DB: conceptualization, designed the experiment, review and editing. All authors contributed to the article and approved the submitted version.
Funding
The research was funded (Grant number PS/SD/N/FPU/MA1/36II) by Presidential Secretariat which is greatly appreciated.
Conflict of interest
The authors declare that the research was conducted in the absence of any commercial or financial relationships that could be construed as a potential conflict of interest.
Publisher’s note
All claims expressed in this article are solely those of the authors and do not necessarily represent those of their affiliated organizations, or those of the publisher, the editors and the reviewers. Any product that may be evaluated in this article, or claim that may be made by its manufacturer, is not guaranteed or endorsed by the publisher.
References
Abdulla M. K., Kumar S. (2014). Phytotoxic effect of mustard cake on seed germination and seedling growth of crop and weeds. Nat. Environ. pollut. Technol. 19, 132–136.
Adler M. J., Chase C. A. (2007). Comparison of the allelopathic potential of leguminous summer cover crops: cowpea, sunnhemp, and velvet bean. HortScience 42 (2), 289–293. doi: 10.21273/HORTSCI.42.2.289
Anderson R. L. (2015). Integrating a complex rotation with no-till improves weed management in organic farming. A review. Agron. Sustain. Dev. 35 (3), 967–974. doi: 10.1007/s13593-015-0292-3
Armengot L., Blanco-Moreno J. M., Bàrberi P., Bocci G., Carlesi S., Aendekerk R., et al. (2016). Tillage as a driver of change in weed communities: a functional perspective. Agriculture Ecosyst. Environ. 222, 276–285. doi: 10.1016/j.agee.2016.02.021
Balasubramaniyan P., Palaniappan S. P. (2003). “Principles and practices of agronomy, Agrobies Jodhpur India. In: Oyewole CI and Oyewole AN. (2010). Crop production and the livestock industry, the interplay: a case study of poultry manure and crop production,” in Research and value addition: key to transformation of the Nigerian livestock industry. Proceeding of the 16th Annual Conference of ASAN. Eds. Adukwu O. A., Oluwagbemi T.O., Aribido S.O., Daikwo S.I., Saliu O.J.. 124–127.
Barberi P., Cozzani A., Macchia M., Bonari E. (1998). Size and composition of the weed seedbank under different management systems for continuous maize cropping. Weed Res. (Oxford) 38 (5), 319–334. doi: 10.1046/j.1365-3180.1998.00098.x
Benaragama D. I., May W. E., Gulden R. H., Willenborg C. J. (2022). Functionally diverse flax-based rotations improve wild oat (Avena fatua) and cleavers (Galium spurium) management. Weed Sci. 70 (2), 220–234. doi: 10.1017/wsc.2021.79
Berzsenyi Z., Győrffy B., Lap D. (2000). Effect of crop rotation and fertilisation on maize and wheat yields and yield stability in a long-term experiment. Eur. J. Agron. 13 (2-3), 225–244. doi: 10.1016/S1161-0301(00)00076-9
Bremner J. M., Mulvaney C. S. (1982). “Total nitrogen,” in Methods of Soil Analysis, vol. 10. (Madison, Wisconsin: American Society of Agronomy, Soil Science Society of America), 594–624.
Collins A. S., Chase C. A., Stall W. M., Hutchinson C. M. (2007). Competitiveness of three leguminous cover crops with yellow nutsedge (Cyperus esculentus) and smooth pigweed (Amaranthus hybridus). Weed Sci. 55 (6), 613–618. doi: 10.1614/WS-07-044.1
Cordeau S., Wayman S., Ketterings Q. M., Pelzer C. J., Sadeghpour A., Ryan M. R. (2021). Long-term soil nutrient management affects taxonomic and functional weed community composition and structure. Front. Agron. 3, 636179. doi: 10.3389/fagro.2021.636179
Department of Agriculture. (2015). Agri Techno Guide (Peradeniya, Sri Lanka: Agriculture Publication Unit, Department of Agriculture).
Gharde Y., Singh P. K., Dubey R. P., Gupta P. K. (2018). Assessment of yield and economic losses in agriculture due to weeds in India. Crop Prot. 107, 12–18. doi: 10.1016/j.cropro.2018.01.007
Ghosh D., Brahmachari K., Brestic M., Ondrisik P., Hossain A., Skalicky M., et al. (2020). Integrated weed and nutrient management improve yield, nutrient uptake and economics of maize in the rice-maize cropping system of Eastern India. Agronomy 10 (12), 1906. doi: 10.3390/agronomy10121906
Herath H. M. S., Hafeel R. F., Bentota A. P., Chandrasiri W. A. C. K. (2017). Farmers’ practices of herbicide usage: reflection on importance of weed management practices. Ann. Sri Lanka Department Agric. 19, 60–71.
Hoagland L., Carpenter-Boggs L., Reganold J. P., Mazzola M. (2008). Role of native soil biology in Brassicaceous seed meal-induced weed suppression. Soil Biol. Biochem. 40 (7), 1689–1697. doi: 10.1016/j.soilbio.2008.02.003
Jackson M. L. (1958). Soil chemical analysis prentice Hall Vol. 498 (Englewood Cliffs, NJ: Inc.), 183–204.
Jansen K., Dubois M. (2014). Global pesticide governance by disclosure: Prior informed consent and the Rotterdam convention. Transparency Global Environ. Governance: Crit. Perspect., 107–131. doi: 10.7551/mitpress/9780262027410.003.0005
Jayawardane S.S.B.D.G., Weerasena L. A. (2001). Crop diversification in Sri Lanka. Crop diversification Asia-Pacific region, 112. Available at: https://www.fao.org/3/X6906E/x6906e0b.htm
Jørnsgård B., Rasmussen K., Hill J., et al. (1996). Influence of nitrogen on competition between cereals and their natural weed populations. Weed Res. 36 (6), 461–470. doi: 10.1111/j.1365-3180.1996.tb01675.x
Kruidhof H. M., Bastiaans L., Kropff M. J. (2008). Ecological weed management by cover cropping: effects on weed growth in autumn and weed establishment in spring. Weed Res. 48 (6), 492–502. doi: 10.1111/j.1365-3180.2008.00665.x
Larney F. J., Blackshaw R. E. (2003). Weed seed viability in composted beef cattle feedlot manure. J. Environ. Qual. 32, 1105–1113. doi: 10.2134/jeq2003.1105
Leather G. R., Forrence L. E. (1990). Sunn hemp is allelopathic to leafy spurge. Proceedings and Progress Reports of the Leafy Spurge Symposium. Great Plains Agricultural Council: Leafy Spurge Symposium. (Gillette, WY), 10–12.
Liebman M., Davis A. S. (2000). Integration of soil, crop and weed management in low-external-input farming systems. Weed research-oxford 40 (1), 27–48. doi: 10.1046/j.1365-3180.2000.00164.x
Liebman M., Gallandt E. R., Jackson L. (1997). Many little hammers: ecological management of crop-weed interactions. Agric. Ecol. J. 1, 291–343. doi: 10.1016/B978-012378260-1/50010-5
Liebman M., Staver C. P. (2001). “Crop diversification for weed management,” in Ecological management of agricultural weeds. Eds. Liebman M., Mohler C. L., Staver C. P. (Cambridge: Cambridge University Press), 322–363.
Little N. G., DiTommaso A., Westbrook A. S., Ketterings Q. M., Mohler C. L. (2021). Effects of fertility amendments on weed growth and weed–crop competition: a review. Weed Sci. 69 (2), 132–146. doi: 10.1017/wsc.2021.1
Magdoff F. (1995). “Soil quality and management,” in Agroecology. The Science of Sustainable Agriculture. Ed. Altieri M. A. (Boulder, CO, USA: Westview Press), 349–364.
Mapa R. B., Somasiri S., Dassanayake A. R. (2010). Soils of dry zone of Sri Lanka: Morphology, characterization, and classification. Soil Sci. Soc. Sri Lanka 51, 126–147.
McSorley R., Dickson D. W., De Brito J. A. (1994). Host status of selected tropical rotation crops to four populations of root-knot nematodes. Nematropica 24, 45–53.
Mhlanga B., Chauhan B. S., Thierfelder C. (2016). Weed management in maize using crop competition: A review. Crop Prot. 88, 28–36. doi: 10.1016/j.cropro.2016.05.008
Moody K., De Datta S. K., Bhan V. M., Manna G. B. (1986). “Weed control in rainfed lowland rice,” in Progress in rainfed lowland rice (Los Baños Philippines: International Rice Research Institute), 371–383.
Oerke E. C. (2006). Crop losses to pests. J. Agric. Sci. 144 (1), 31–43. doi: 10.1017/S0021859605005708
Opeña J. L., Quilty J. R., Correa T. Q. Jr., Chauhan B. S. (2014). Weed population dynamics, herbicide efficacies, and crop performance in a sprinkler-irrigated maize-rice cropping system. Field Crops Res. 167, 119–130. doi: 10.1016/j.fcr.2014.07.014
Paul J. W., Beauchamp E. G. (1993). Nitrogen availability for corn in soils amended with urea, cattle slurry, and solid and composted manures. Can. J. Soil Sci. 73 (2), 253–266. doi: 10.4141/cjss93-027
Pingali P. L. (2012). Green revolution: impacts, limits, and the path ahead. Proc. Natl. Acad. Sci. 109 (31), 12302–12308. doi: 10.1073/pnas.0912953109
Pleasant J. M., Schlather K. J. (1994). Incidence of weed seed in cow (Bos sp.) manure and its importance as a weed source for cropland. Weed Technol. 8, 304–310. doi: 10.1017/S0890037X00038823
Pretty J. (2008). Agricultural sustainability: concepts, principles and evidence. Philos. Trans. R. Soc. B: Biol. Sci. 363 (1491), 447–465. doi: 10.1098/rstb.2007.2163
Price A. J., Stoll M. E., Bergtold J. S., Arriaga F. J., Balkcom K. S., Kornecki T. S., et al. (2008). Effect of cover crop extracts on cotton and radish radicle elongation. Commun. Biometry Crop Sci. 3, 60–66.
Qasem J. R. (2011). “Herbicides applications: problems and considerations,” in Herbicides and environment (London, UK: IntechOpen), 643–662.
Rao A. N., Wani S. P., Ahmed S., Haider Ali H., Marambe B. (2017). An overview of weeds and weed management in rice of South Asia. In: Weed management in rice in the Asian-Pacific region. Hyderabad: Asian-Pacific Weed Science Society (APWSS). pp. 247–281. Available at: https://oar.icrisat.org/10211.
Reganold J. P., Wachter J. M. (2016). Organic agriculture in the twenty-first century. Nat. Plants 2 (2), 1–8. doi: 10.1038/nplants.2015.221
Scavo A., Mauromicale G. (2020). Integrated weed management in herbaceous field crops. Agronomy 10 (4), 466. doi: 10.3390/agronomy10040466
Sharma N., Singhvi R. (2017). Effects of chemical fertilizers and pesticides on human health and environment: a review. Int. J. agriculture Environ. Biotechnol. 10 (6), 675–680. doi: 10.5958/2230-732X.2017.00083.3
Shirtliffe S. J., Benaragama D. (2014). “Sometimes you need a big hammer: evaluating and appraising selected nonherbicidal weed control methods in an integrated weed management system,” in Managing energy, nutrients and pests in organic field crops. Eds. Martin R. C., MacRae R. (New York: CRC Press), 149–174.
Smith R. G., Gross K. L. (2006). Rapid change in the germinable fraction of the weed seed bank in crop rotations. Weed Sci. 54 (6), 1094–1100. doi: 10.1614/WS-06-090R.1
Tatenda R. J., Jacobus P. P., Stanford M. (2021). Influence of short-term maize–green manure cover crop rotations on germinable weed seed bank under conservation agriculture. Asian J. Agric. Rural Dev. 11 (1), 139–155. doi: 10.18488/journal.ajard.2021.111.139.155
Taylor S. G., Baltensperger D. D., Dunn R. A. (1985). Interactions between six warm-season legumes and three species of root-knot nematodes. J. Nematol. 17 (3), 367. Available at: https://www.ncbi.nlm.nih.gov/pmc/articles/PMC2618461.
Teasdale J. R. (2018). “The use of rotations and cover crops to manage weeds,” in Integrated Weed Management for Sustainable Agriculture (Cambridge, UK: Burleigh Dodds Science Publishing), 227–260.
Teshome D. T., Zharare G. E., Naidoo S. (2020). The threat of the combined effect of biotic and abiotic stress factors in forestry under a changing climate. Front. Plant Sci. 11, 1874. doi: 10.3389/fpls.2020.601009
Thenabadu M. W. (1988). “Soil and soil conservation in Sri Lanka,” in National Resources (Colombo: Energy and Science Authority of Sri Lanka), 4–8.
Verschwele A., Zwerger P. (2005). “Effects of organic farming on weed abundance-long-term results from a site in Northern Germany,” in Proceedings of the 13th EWRS Symposium (Bari, Italy: EWRS).
Wang K. H., Sipes B. S., Schmitt D. P. (2001). Suppression of Rotylenchulus reniformis by Crotalaria juncea, Brassica napus, and Tagetes erecta. Nematropica 31, 235–250.
Watanabe F. S., Olsen S. R. (1965). Test of an ascorbic acid method for determining phosphorus in water and NaHCO3 extracts from soil. Soil Sci. Soc. America J. 29 (6), 677–678. doi: 10.2136/sssaj1965.03615995002900060025x
Weisberger D., Nichols V., Liebman M. (2019). Does diversifying crop rotations suppress weeds? A meta-analysis. PLoS One 14 (7), 0219847. doi: 10.1371/journal.pone.0219847
Williams J., Roberts S., Hill J., Scardaci S., Tibbits G. (1990). IPM: managing water for weed control in rice. California Agric. 44 (5), 7–10. doi: 10.3733/ca.v044n05p7
Keywords: conventional, integrated, organic, weed biomass, weed density
Citation: Wickramasinghe D, Devasinghe U, Suriyagoda LDB, Egodawatta C and Benaragama DI (2023) Weed dynamics under diverse nutrient management and crop rotation practices in the dry zone of Sri Lanka. Front. Agron. 5:1211755. doi: 10.3389/fagro.2023.1211755
Received: 25 April 2023; Accepted: 05 September 2023;
Published: 25 September 2023.
Edited by:
Simerjeet Kaur, Punjab Agricultural University, IndiaReviewed by:
Kassio Ferreira Mendes, Federal University of Viçosa, BrazilSanjay Singh Rathore, Indian Agricultural Research Institute (ICAR), India
Arnold Mashingaidze, Chinhoyi University of Technology, Zimbabwe
Copyright © 2023 Wickramasinghe, Devasinghe, Suriyagoda, Egodawatta and Benaragama. This is an open-access article distributed under the terms of the Creative Commons Attribution License (CC BY). The use, distribution or reproduction in other forums is permitted, provided the original author(s) and the copyright owner(s) are credited and that the original publication in this journal is cited, in accordance with accepted academic practice. No use, distribution or reproduction is permitted which does not comply with these terms.
*Correspondence: Chamnida Egodawatta, egowcp@agri.rjt.ac.lk