- 1Department of Plant Science, The Pennsylvania State University, University Park, PA, United States
- 2Department of Horticulture and Crop Science, The Ohio State University, Columbus, OH, United States
- 3United States Department of Agriculture – Agricultural Research Service, Morris, MN, United States
- 4Department of Agriculture, Illinois State University, Normal, IL, United States
- 5Department of Agronomy and Plant Genetics, University of Minnesota, St. Paul, MN, United States
- 6School of Agriculture, Western Illinois University, Macomb, IL, United States
- 7Electrical Engineering-Sustainability and Renewable Energy Systems, University of Wisconsin-Platteville, Platteville, WI, United States
Introduction: Oilseed pennycress (Thlaspi arvense L.) is an emerging biofuel crop for use in the aviation industry that has potential as a rotational crop in corn (Zea mays L.)–soybean [Glycine max (L.) Merr.] cropping sequences. Ensuring autumn emergence of pennycress after early broadcast seeding is key because this practice may result in uneven spatial distribution due to the small seed size and variable germination, soil contact, and moisture availability. The objective of this research was to evaluate the impact of five seed treatments and enhancements on autumn establishment in two pennycress lines (MN106NS and tt8-t/ARV1) in broadcast seeding compared with no treatment.
Methods: Tested treatments were (i) gibberellic acid (GA) soak, (ii) fludioxonil fungicide, (iii) pelleting with diatomaceous earth and a commercial binder, (iv) fungicide plus pelleting, or (v) fungicide plus pelleting with GA added to the binder. Seeds were planted at nine sites in four U.S. states to assess establishment (stand counts and percentage canopy cover) in the autumn and spring and seed yield after maturity.
Results: The MN106NS line had greater plants m−2 and percent green cover with treatments that included GA compared to the control. Line tt8-t/ARV1 had reduced stands when pelleted compared to the untreated, and establishment was unchanged when treated with GA compared to the untreated. Pelleting treatments without GA were not beneficial for stand establishment of either line. Seed yield for MN106NS was 20% greater than the untreated when treated with GA only or pelleted. Seed yield for tt8-t/ARV1 did not increase over the untreated with any treatment and was reduced by 20%–40% when pelleted.
Discussion: These results suggest minimal benefit of seed treatment and pelleting for the tested lines under broadcast seeding, though black-seeded lines may still benefit from GA treatment. Plant establishment and yield were negatively correlated with total precipitation post-planting, suggesting that excessive rainfall post-planting (>60 mm) may impede establishment when broadcast seeded.
1 Introduction
Corn and soybean production dominate the U.S. Midwest agricultural landscape, which limits crop diversification. Crop diversification, while being challenged by climate and management practices (Mohammed et al., 2020), can increase agricultural productivity and sustainability (Brummer, 1998; Davis et al., 2012; Gaba et al., 2015). Increasing the bioeconomy of the agriculture sector through biofuel production can promote its sustainability (National bioeconomy blueprint, 2012). Oilseed pennycress grown as a winter annual crop for sustainable aviation fuel could fit into temperate climate regions such as the upper Midwest (McGinn et al., 2019). However, adoption of pennycress may be limited due to its poor germination and establishment (Hazebroek & Metzger, 1990; Sedbrook et al., 2014; Mohammed et al., 2020).
Recent studies that continue to demonstrate pennycress seed yields of 2,400 kg ha—1 (Cubins et al., 2019) and oil content of 270–390 g kg—1 (McGinn et al., 2019) highlight the suitability for biofuel generation (Moser et al., 2009b; Moser, 2012; Fan et al., 2013; Mousavi-Avval & Shah, 2020). Pennycress offers a suite of ecosystem services by providing food for pollinators (Eberle et al., 2015; Chopra et al., 2020), promoting the diversity of beneficial arthropods (Groeneveld & Klein, 2015; Groeneveld et al., 2015), suppressing weeds (Johnson et al., 2015), and scavenging residual N from the field (Weyers et al., 2019; Moore et al., 2020). Pennycress also has higher biofuel production potential and lower negative environmental impact compared to other existing oil-producing crops (Moser et al., 2009a; Mousavi-Avval & Shah, 2021).
Successful establishment and subsequent survivability are key requirements for crop production, and without achieving these, a crop is very unlikely to be adopted. Pennycress naturally has a higher degree of seed dormancy, though seed treatments with gas (especially GA4 + 7) have been found to be effective in breaking dormancy and increasing germination in seeds with a black seed coat (Metzger, 1983; Saini et al., 1987; Koirala et al., 2022). Koirala et al. (2022) also reported that seed pelleting increased germination in a black-seeded pennycress line under controlled conditions. Similarly, recent efforts in domestication have led to low-fiber golden pennycress lines that exhibit high germination potential in the absence of exogenous treatment (Ott et al., 2021; Koirala et al., 2023). Improved germination under ideal conditions does not always guarantee better establishment in field conditions (Finch-Savage & Bassel, 2016; Reed et al., 2022), but seed pelleting might be effective to improve field establishment of pennycress as has been demonstrated in other crops (Burns et al., 2002; Gesch et al., 2012; Javed & Afzal, 2020).
Improved establishment under broadcast seeding of pennycress in autumn is desired to facilitate easy intercropping and shortening the time between annual crop harvest and pennycress emergence. Being an emerging crop, pennycress lacks optimized crop management practices (Cubins et al., 2019; Zanetti et al., 2019; Verhoff et al., 2022). Previous studies on pennycress have reported using seeding rates ranging from 1.1 to 16.8 kg ha−1 (Cubins et al., 2019), which may have been an artifact of high seed dormancy and poor establishment. Planting depth and method are also reported to influence pennycress establishment. Phippen et al. (2010) reported drill seeding having a better crop stand than broadcast seeding while Moore and Mirsky (2020) did not observe a benefit of drill seeding over broadcast seeding. Similar studies comparing these two establishment methods did not find a significant difference in seed and biomass yield in pennycress (Phippen et al., 2010; Noland et al., 2018; Moore et al., 2020). CoverCress, Inc (2022) recommends farmers use a 5.6 kg ha−1 seeding rate with surface seeding or seeding <0.6 cm below soil surface during early September through mid-October for golden pennycress “CoverCress™” lines. Soil water availability is identified as the most limiting factor for field emergence (Hazebroek & Metzger, 1990), and precipitation of approximately 13 mm within 3 days of planting is stated as adequate to facilitate establishment (CoverCress, Inc, 2022). The integrated use of seed treatment such as fungicide, pelleting, and GA may increase pennycress establishment in autumn and may lead to increased seed yield. The objective of this study was to assess how different seed treatments (including seed pelleting) affect establishment and yield of different pennycress lines in a range of environmental conditions after broadcast seeding. The hypothesis was that treatments would vary in their efficacy of improving establishment, though gains in germination and establishment would be seen for all over the untreated.
2 Materials and methods
2.1 Study sites and experimental design
A field experiment was conducted in 2021–2022 at nine sites across the U.S. Midwest (Table 1, Figure 1). The design at each site was a randomized complete block with three replicates per site. The treatments included two varieties with six seed treatments: untreated control (Unt.); GA4 + 7 soak (Gibberellic acid A4+A7 90%, ThermoFisher Scientific Chemicals, Inc., Ward Hill, MA) at 0.01% w/w for 12 h (GA only); fludioxonil [4-(2,2-Difluoro-2H-1,3-benzodioxol-4-yl)-1H-pyrrole-3-carbonitrile] fungicide (Maxim 4FS, Syngenta Crop Protection Canada Inc., Guelph, ON) at 50 μg ai per g of seed (Fung only); pelleting with diatomaceous earth (Spectrum Chemical Mfg. Corp., Gardena, CA) and a commercial binder (Seedworx Lite, AgInnovation, Walnut Grove, CA) (Pellet); and two pelleting treatments with the addition of fungicide alone (Pellet + Fung) or fungicide and GA at 0.01% w/v added to the pelleting binder solution (Pellet + GA + Fung). Fludioxonil as the fungicide was chosen due to its availability in other agronomic crops, and to combat potential fungal infection that could influence seedling survival and crown integrity. The first line was an improved, black-seeded pennycress from a public source (“MN106NS”; USDA-ARS, St. Paul, MN), and the second was a golden-seeded pennycress from a private source (“tt8-t/ARV1”; CoverCress, Inc., St. Louis, MO). Both lines are improved beyond the wild type, though the golden-seeded line is a transparent testa type that has a modified seed coat and may be less responsive to seed treatments to stimulate germination (Ott et al., 2021; Koirala et al., 2023). The seed of both tested lines was harvested in June 2021. The seed treatments were applied to a 40-g sample of both lines using the same materials and methods described in Koirala et al. (2022). Each batch of seed was subdivided into envelopes containing 1,000 seeds counted using a seed counter (Seedburo 801, Seedburo Equipment Company, Des Plains, IL) and were distributed in replicate for planting at each field location.
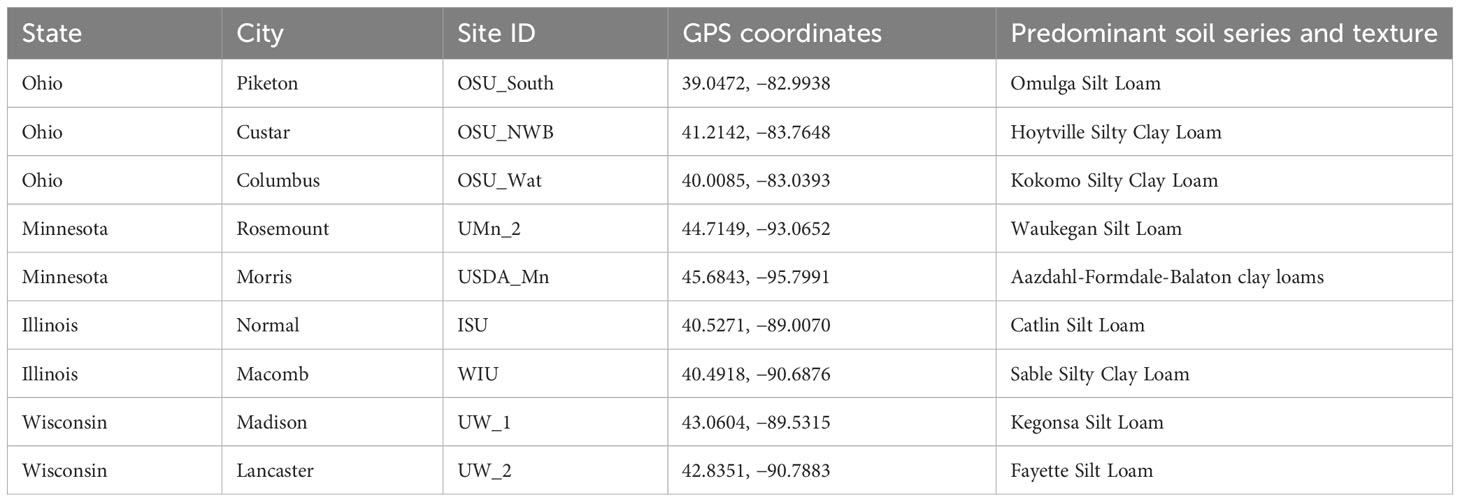
Table 1 State, city, site abbreviation (ID), GPS coordinates, and predominant soil series and texture for each site as identified from USDA-NRCS Web Soil Survey.
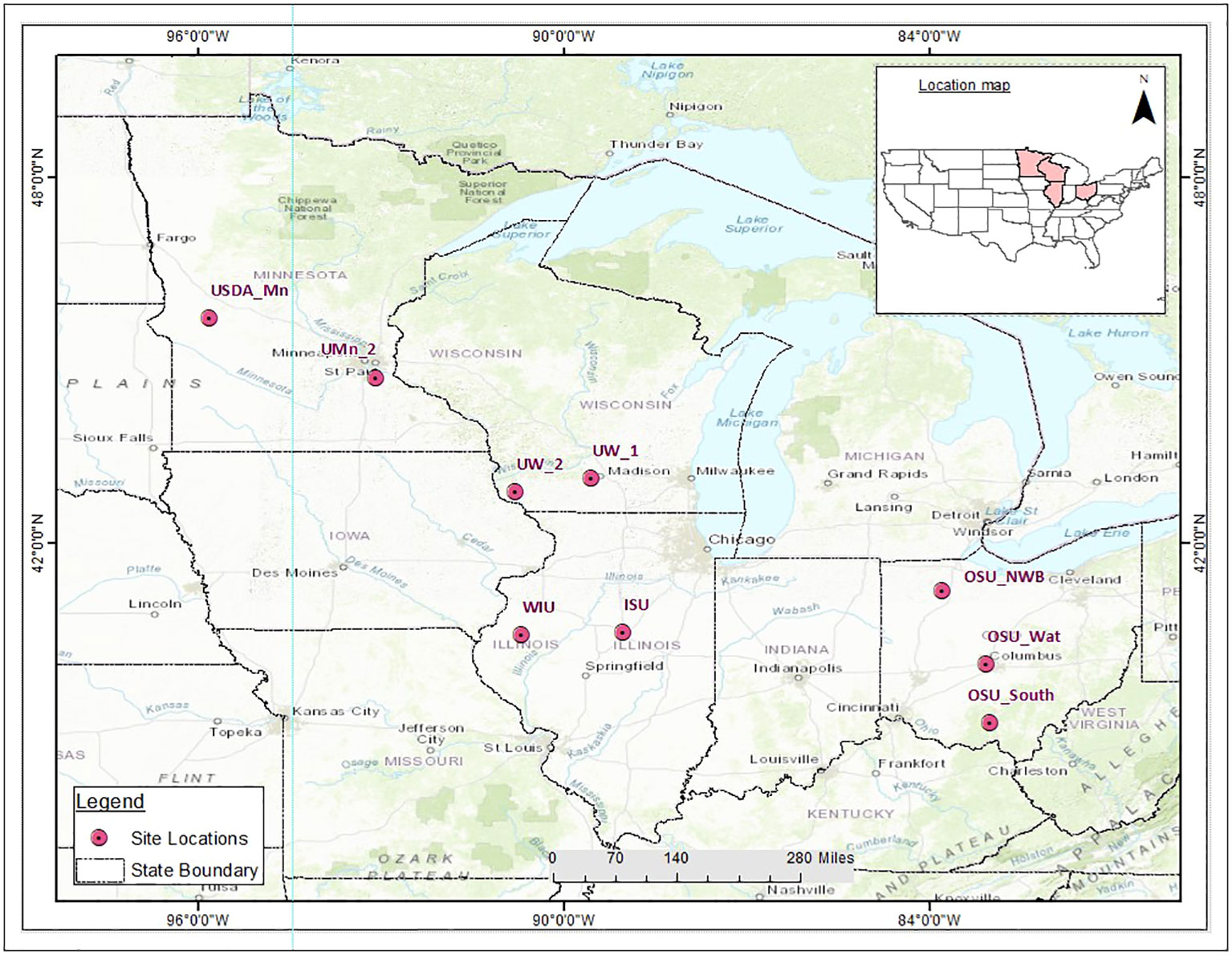
Figure 1 Map illustrating state and location for study sites. Abbreviations for sites are described in Table 1.
2.2 Cultivation practices
The plots were 1.2 × 1.2 m with a 0.6-m border between them. Planting dates ranged from 13 September to 12 October 2021 (Table 2). The previous crop and tillage practice used before sowing for each site are also listed in Table 2. The percentage residue cover from the previous crop at planting and soil surface moisture condition (rated on a scale of 1–5: 1 = dry surface, 2 = 25% wet, 3 = 50% wet, 4 = 75% wet, and 5 = 100% wet) for each site was noted using visual assessment (Table 2).
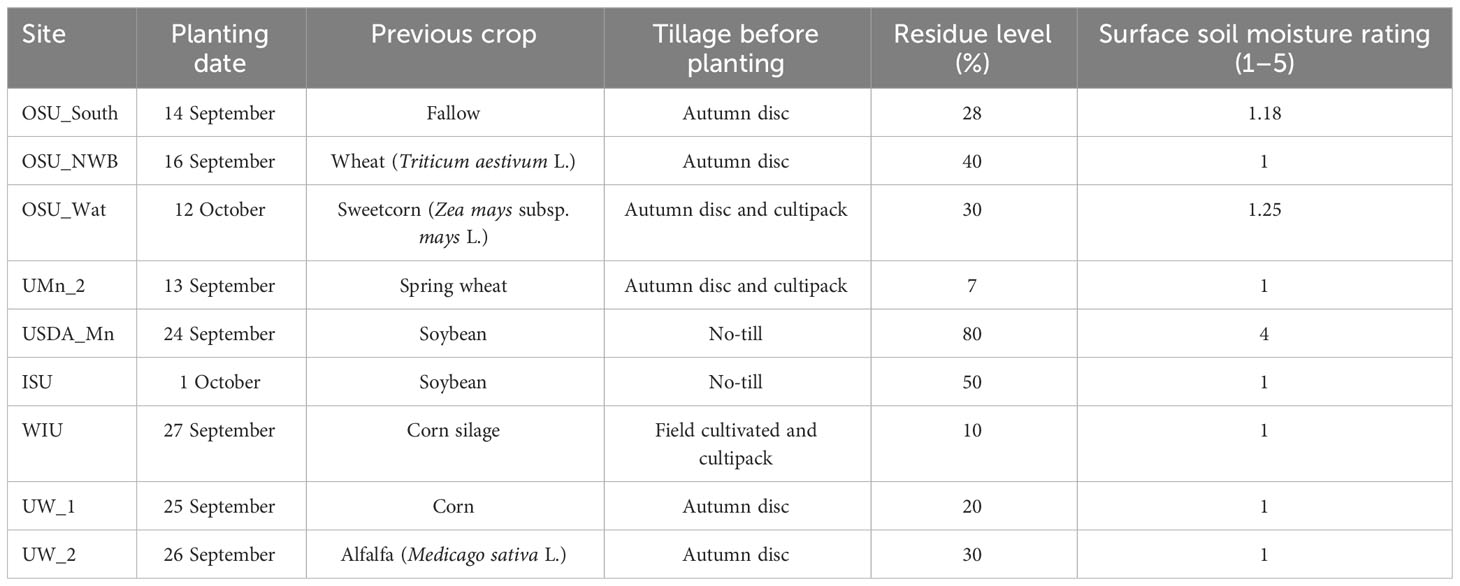
Table 2 Planting date, previous crop, tillage before planting, percentage residue cover, and surface soil moisture rating on a scale of 1–5 (1 = dry surface, 2 = 25% wet, 3 = 50% wet, 4 = 75% wet, and 5 = 100% wet) for each site (site abbreviations in Table 1).
Plots were seeded with 1,000 seeds each as a consistent seed number was desired for treatment evaluation. Seeding rate was not calculated on weight basis because of the difference in weight between pelleted and non-pelleted seeds, and MN106NS naturally had a greater 1,000 seed weight than tt8-t/ARV1. Assuming the non-pelleted seeds had an approximate weight of 0.9 mg (Koirala et al., unpublished data), the seeding rate would equate to 6 kg ha−1, which is similar to the rates used in previous studies on pennycress (Phippen et al., 2010; Johnson et al., 2015; Dose et al., 2017; Cubins et al., 2019; Zanetti et al., 2019). Owing to the small area of each plot and low volume of seed, pennycress seeds were blended with 5 g of inert teff [Eragrostis tef (Zucc.) Trotter] seed prior to hand-broadcasting to increase uniformity of seed distribution.
2.3 Measurements collected
A composite soil sample to 20-cm depth was collected from each site for baseline analysis of cation exchange capacity (CEC), organic matter (OM) (measured as loss on ignition), pH (in water), P (Bray P1), and Mehlich-3 extraction of K, Ca, and Mg (Table 3). Daily total precipitation and mean air temperature data were recorded from 7 days prior to seeding up to 14 days after seeding. The rainfall data were extracted from the respective research station’s weather records from each site. Temperature data were obtained from the records available at the Parameter-elevation Regressions on Independent Slopes Model (PRISM) Climate Group’s website maintained by Northwest Alliance for Computational Science and Engineering (NACSE), based at Oregon State University using the coordinates for each site (NACSE, 2022). Stand count was assessed early to mid-November (after the first frost) using a 0.25-m2 area quadrat in each plot. One image per plot was taken from a consistent height and was processed using the “Canopeo” mobile application (Oklahoma State University, Stillwater, OK) to obtain green cover area percentage for each quadrat. Spring stand counts and canopy coverage using the same procedures were conducted in late March to mid-April at each site. Following spring stand assessment, plots were top-dressed with 45 kg N ha−1 of urea (46-0-0).
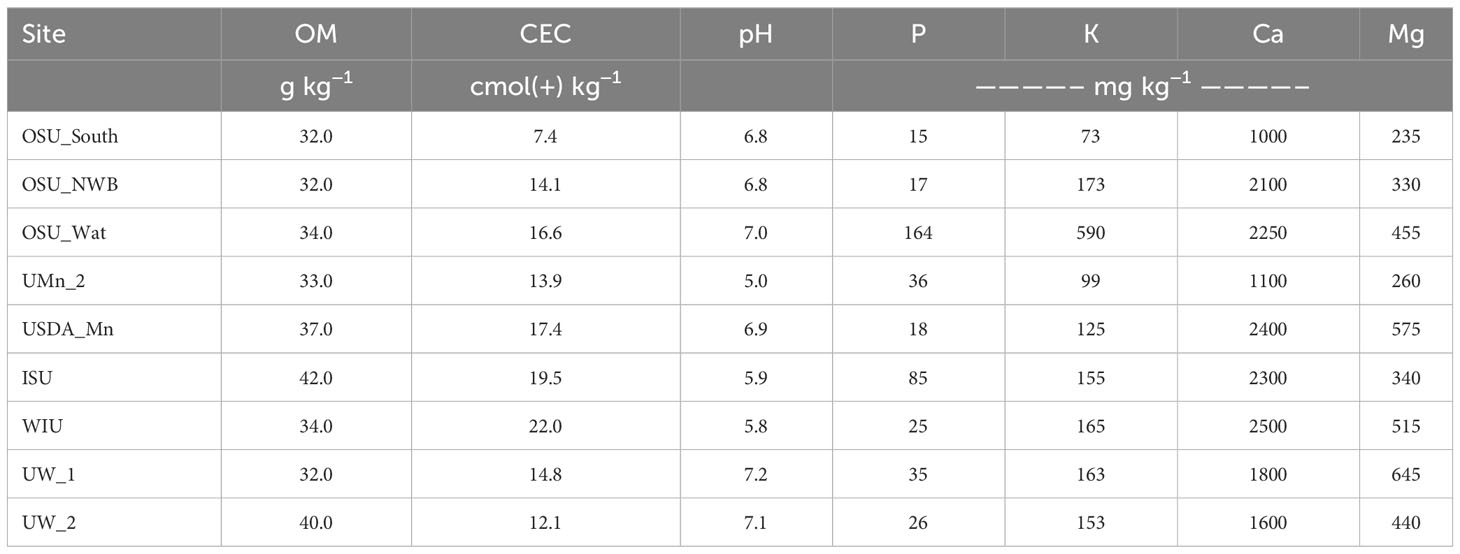
Table 3 Soil test results including organic matter (OM) measured as loss on ignition, cation exchange capacity (CEC), pH (in water), P (Bray P1), and Mehlich-3 extraction of K, Ca, and Mg for each site (site description in Tables 1 and 2).
Because of excessive spring weed competition after spring stand assessment, OSU_South and UW_2 were unable to be harvested. Prior to harvest, each plot was visually assessed for stem lodging (0–100%) where higher percentages indicated greater lodging in each plot. Each plot was harvested between 9 and 30 June 2022. Seed yields were determined following hand-harvest and threshing using a small batch thresher (i.e., SBT Thresher, Almaco, Nevada, IA) or by mechanically harvesting using a Wintersteiger plot combine (Quantum model, Salt Lake City, Utah). The weight of 1,000 dried seeds was also measured from each plot.
2.4 Statistical analysis
Data analysis was conducted using a general linear mixed model (GLIMMIX procedure) in SAS 9.4 (SAS Institute, Cary, NC, USA). To address issues with assumptions of normality, the data for autumn stand count and canopy coverage were processed using a negative binomial distribution and a log link function. Spring stand count data were processed using a Poisson distribution with a log link function, and spring canopy coverage and lodging at harvest were analyzed using a beta distribution and logit link function.
Seed treatment and pennycress line and their interaction were considered as fixed factors, with site and replication nested within site being set as random factors. Site was treated initially as a fixed factor, but majority of interactions with site and other fixed effects were of magnitude (rather than direction). Site was treated as a random effect to assess the overarching ability of seed treatment influence on germination of the two lines when broadcast seeded. When the Global F-test was found to be significant (α = 0.05), means separation was conducted using LSMEANS statement in SAS after applying the ilink adjustment. Correlation analysis (CORR procedure) was conducted to determine potential relationships between percentage green area cover and number of plants m−2 and other measured variables (surface moisture level, residue cover percentage, precipitation after planting, precipitation 7 days before planting to 14 days after planting, day of year, and latitude).
3 Results
3.1 Weather at different sites
During the daily total precipitation measurement period (7 days prior to planting to 14 days after planting), there was one site with a single-day precipitation value of 40 mm (OSU_NWB), three sites with a single-day total between 20 and 40 mm (UMn_2, UW_1, and UW_2), and five sites with a single-day precipitation value below 20 mm (OSU_South, OSU_Wat, USDA_Mn, ISU, and WIU) (Table 4). Of the 84 mm precipitation received at OSU_NWB after planting, 71 mm of that total occurred in a single day. All other sites received some precipitation in the first 2 weeks after planting though distribution of precipitation in each site varied. Average daily temperature at each site ranged between 15 and 20°C, suggesting temperatures were adequate for pennycress germination.
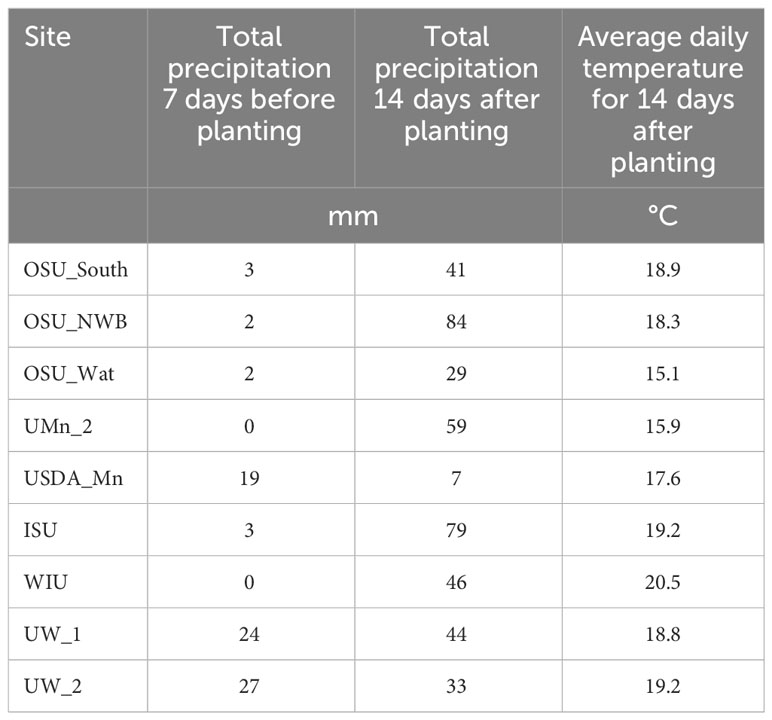
Table 4 Precipitation total 7 days prior to planting and 14 days post-planting, as well as average daily temperature for the first 14 days post-planting.
3.2 Autumn and spring establishment
Data from OSU_NWB are not included in the analyzed results as autumn establishment was 0 plants m−2 for all treatments likely due to the excessive rainfall event (71 mm) that occurred after planting. Across all other sites, a significant treatment by line interaction was evident for autumn and spring stands (Table 5). The MN106NS line was more responsive to GA treatment, exhibiting the greatest number of plants and greatest canopy cover of all treatments when soaked or pelleted with GA included in the binder solution (55%–70% more plants m−2 and 16%–37% greater canopy coverage compared to untreated MN106NS). Pelleting MN106NS without GA application and treatment with fungicide alone improved establishment compared to the untreated check, though they were statistically similar in most cases (12%–40% more plants m−2, 0%–25% greater canopy coverage). In contrast, tt8-t/ARV1 establishment was negatively affected by all pelleting treatments (Table 5) reducing plant numbers by 60%–75% and canopy coverage by 31%–61% compared to untreated tt8-t/ARV1. Treatment of tt8-t/ARV1 with fungicide or GA alone had marginal effects on establishment compared to the untreated (−15% to 20% compared to untreated). These results indicate that seed treatment improved establishment for MN106NS, whereas establishment of tt8-t/ARV1 was impeded by pelleting.
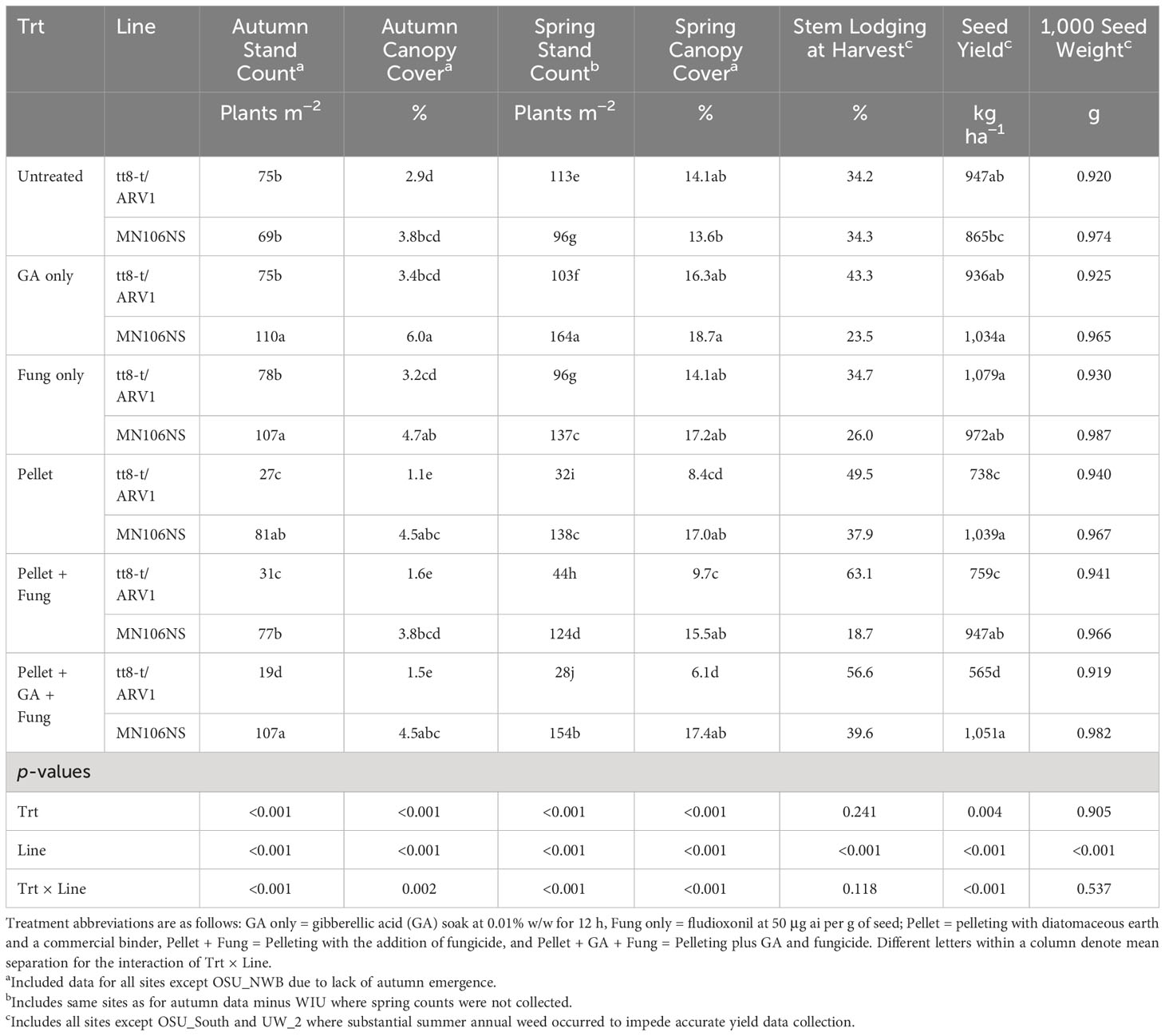
Table 5 Effects of seed treatment (Trt) and pennycress line on autumn and spring stand count, percentage canopy coverage, percentage stem lodging at harvest, seed yield, and 1,000 seed weight after harvest.
3.3 Stem lodging, yield, and 1,000 seed weight
Visual rating of stem lodging at harvest was unaffected by seed treatment, but was greater for tt8-t/ARV1 (46.8%) than for MN106NS (29.4%) across seed treatments. Seed treatment effects on seed yield for MN106NS mirrored the effects observed for autumn and spring establishment values (Table 5) in that all treatments were similar or greater yielding than the untreated by 9% to 21%. Conversely, tt8-t/ARV1 treated with fungicide produced the greatest yield but was statistically similar to untreated tt8-t/ARV1. All treatments where pelleting was used reduced yield by 20%–40% compared to untreated tt8-t/ARV1. Seed treatment did not affect 1,000 seed weight after harvest, though MN106NS seeds were heavier than tt8-t/ARV1 seeds (0.974 vs. 0.929 g, respectively).
3.4 Correlation results
Different correlations were observed between the dependent variables and other variables independent to our treatments (Table 6). Plant number (at both autumn and spring) was positively correlated to precipitation before planting and negatively correlated to precipitation after planting, possibly due to heavy precipitation post-planting reducing establishment (Figure 2). Percentage canopy coverage correlations were less impacted by precipitation patterns, though they were correlated to their respective plant numbers within each measurement period. Canopy coverage was negatively correlated to residue percentage, suggesting that emerged plants may have been less visible in sites with heavier surface residue. Spring plant number exhibited the strongest correlation to yield. Drier soil at planting and greater precipitation post-planting were correlated to greater 1,000 seed weights, though further investigation into these relationships may be warranted to draw further conclusions. Stems with fewer or smaller seeds in their silicles may have been less prone to lodging at the time of harvest, which may explain the significant correlation between lodging and 1,000 seed weight.
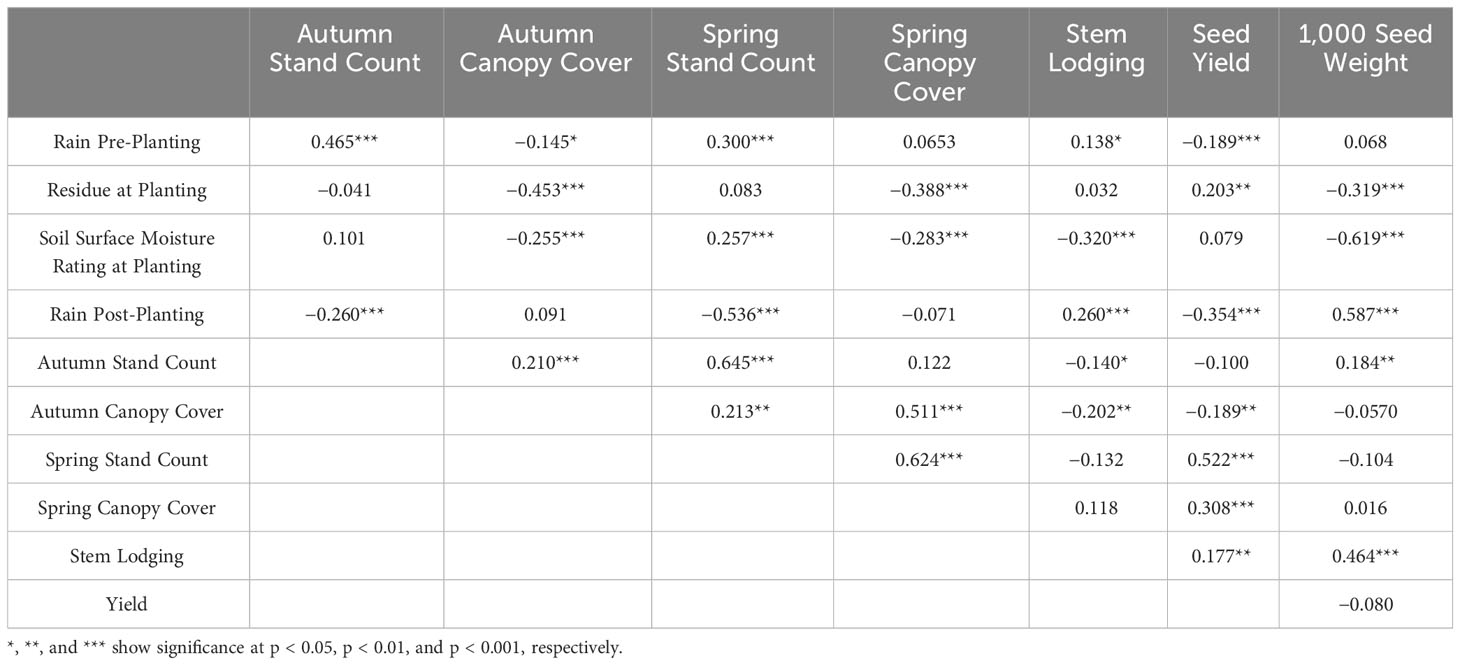
Table 6 Pearson correlation coefficient (r) for precipitation from 7 days before planting (rain pre-planting), surface residue coverage at planting, soil surface moisture rating at planting, precipitation up to 14 days after planting (rain post-planting), autumn and spring stand counts, percentage green area cover during autumn and spring, and other parameters: percentage stem lodging, seed yield, and 1,000 seed weight across locations, lines, and seed treatments.
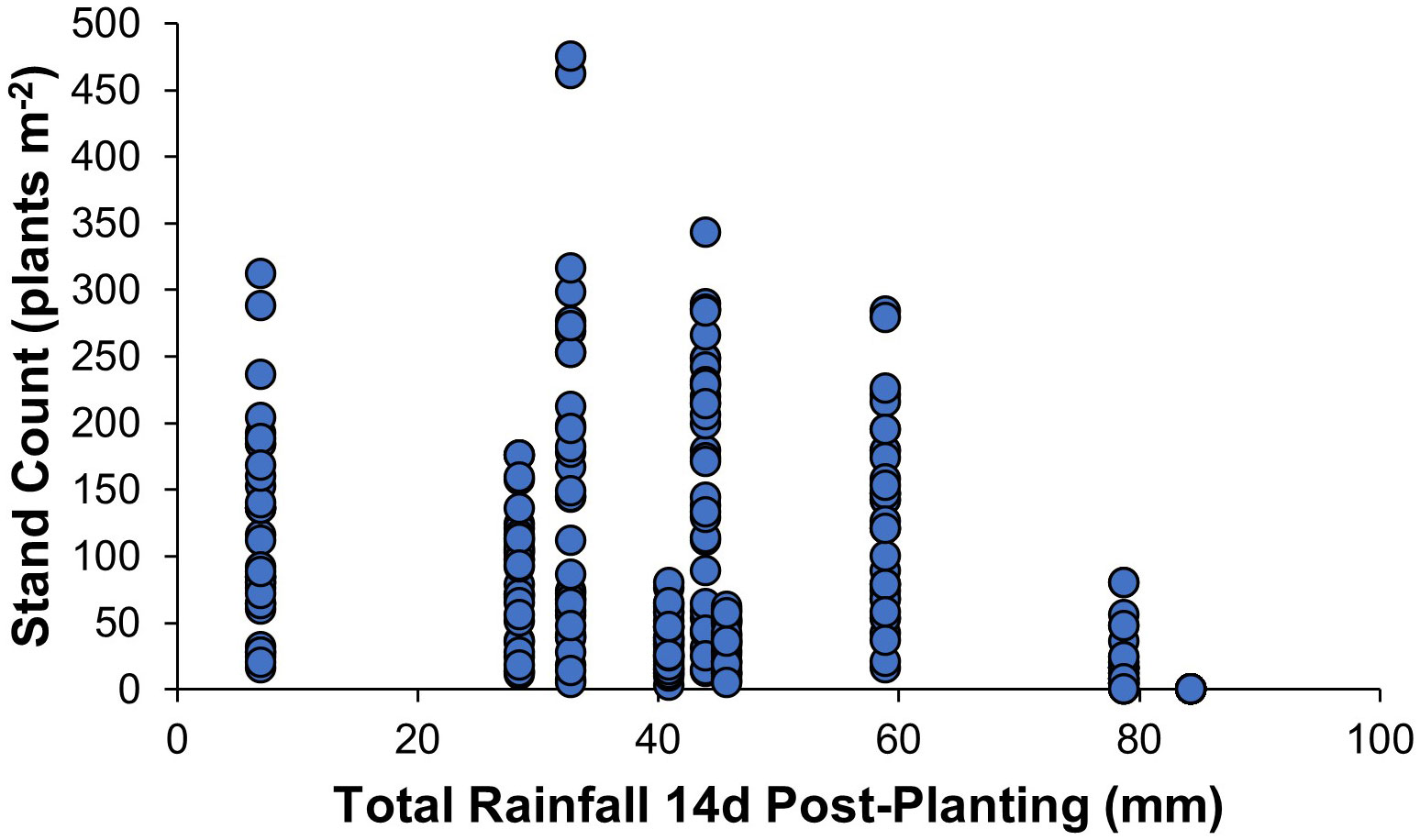
Figure 2 Autumn stand count (y-axis) as affected by total precipitation during the first 14 days post-planting (x-axis).
4 Discussion
Similarities in pennycress seedling establishment occurred across all sites, suggesting that its potential for production exists over a wide area of the upper U.S. Midwest. Establishment was demonstrated over a wide range of soil pH from 5.0 to 7.2 (Table 3) under broadcast seeding conditions, though autumn precipitation played an important role in establishment. The OSU_NWB site experienced heavy rainfall (71 mm) on the 6th day after sowing, which likely washed out or killed any emerged seedlings. With the failure of plant establishment in all seed treatments of both lines at OSU_NWB, forecasted rainfall may be key in ensuring autumn establishment. Industry recommendations are for 13-mm precipitation within 3 days of planting to facilitate establishment (CoverCress, Inc, 2022); the current study suggests between 10 and 60 mm in the 14 days following seeding may be sufficient for the establishment of surface broadcast seeds. Both OSU_South and UW_2 were not harvested due to excessive weed competition, and both sites were planted after a non-grain crop (Table 2). This indicates that annual crop herbicide programs or past management practices may be more conducive to pennycress establishment compared to other cropping systems. The inability to harvest in these two sites due to high competition supported the idea of low water use efficiency and competing ability of pennycress as discussed by Johnson et al. (2015).
There was an overall increase in plant stand count (averaged across all sites counted) in spring compared to autumn stand count across all seed treatments and both varieties (Table 5). This indicated the presence of spring emergence even with the GA seed treatments that were applied to break dormancy and accelerate germination shortly after planting. A similar increase was reported by Wohrley (2022), though Mohammed et al. (2020) reported a reduction in stand count in spring compared to autumn. All pelleted treatments with or without GA improved number of established plants in spring for black-seeded MN106NS. This increase supported the idea presented by Koirala et al. (2023) of improved seed vigor with these treatments in MN106NS. Conversely, pelleted seed treatments on golden-seeded tt8-t/ARV1 had a negative impact in plant stand count. These results were supported by Koirala et al. (2023) that showed that seed pelleting of golden-seeded decreased seed vigor.
The positive correlation observed between number of plants and green canopy cover (Table 6) was similar to a study reported by Wohrley (2022). However, a negative relationship between these two variables was observed and discussed in Koirala (2022) and was reported in another study of Wohrley (2022) possibly due to plant thinning along with growth and rosette development. Canopy cover of at least 30% of the soil surface is expected in a cover crop to protect soil from erosion (Allmaras & Dowdy, 1985); the maximum observed spring canopy cover averaged across sites (18.7%) was lower than the desired cover of more than 30% to fully realize erosion prevention benefits. This might be due to the use of broadcast seeding in this study, which was reported to have a poor establishment than drill seeding (Phippen et al., 2010). However, the number of plants m−2 even with drill seeding in the study by Phippen et al. (2010) was less than what was observed for most treatments in the current study.
Despite the positive correlation observed between spring stand count and canopy cover with seed yield, non-pelleted tt8-t/ARV1 had a similar yield to that of MN106NS. The observed yields were similar and within the range of the studies reported by Cubins et al. (2019) in their review of pennycress agronomics. The same article mentioned that a pennycress seed yield of 1,684 kg ha−1 is required to be an economically viable crop, while Trejo-Pech et al. (2019) estimated that a seed yield of 1,335 kg ha−1 was needed to cover production costs. The seed yields observed in the current study across sites were 25% or more below these desired estimates, indicating that the efforts to improve establishment and yield through seed treatments under broadcast seeding were inadequate. Further advances in both genetics and other management practices (i.e., seeding method and seeding rate) will be needed to ensure critical yield levels are achieved.
The golden-seeded line tt8-t/ARV1 experienced a reduction in establishment and yield following most treatments in this study, suggesting that treatment using these methods was not necessary or was detrimental (e.g., pelleting). Even though seed pelleting with GA improved establishment and yield for MN106NS, there was no clear advantage of pelleting over the GA-only treatment as suggested in literature (Scott, 1989; Gesch et al., 2012; Vinod Kumar et al., 2015; Su et al., 2017; Javed & Afzal, 2020). Pelleting could result in improved flow rate in mechanical planters (Burns et al., 2002), though mechanized planting was not assessed in the current study. The treatments from the current study may be used for MN106NS depending on the availability of seed treatment facility, cost of treatment, and goals for treating the seed.
In conclusion, the seed treatments applied in the current study were not enough to fully address the challenge of establishing pennycress as a viable winter annual cash cover crop in the Midwestern United States with broadcast seeding. Rainfall accumulation of more than 60 mm within 14 days of planting can reduce plant establishment; thus, a weather forecast should be considered when deciding a planting period to avoid heavy rain events. Similarly, as rainfall within 7 days prior to planting improved plant stand, it is recommended to broadcast pennycress after a rainfall during autumn for better establishment. It is not recommended to treat the golden-seeded line with the treatments used in the present study, while the black-seeded line had better establishment and yield from seed treatment with GA and the novel seed treatment—seed pelleting with added GA. Pennycress had lower competing ability with other crops and weeds; therefore, a herbicide program needs to be developed for pennycress especially if it is broadcasted following non-grain crops or fallow conditions.
Data availability statement
The raw data supporting the conclusions of this article will be made available by the authors, without undue reservation.
Author contributions
NK: study design, treatment implementation, data collection, and drafted original manuscript; DB: study design and edited manuscript draft; RG: data collection and edited manuscript draft; YM: data collection and edited manuscript draft; NH: data collection and edited manuscript draft; AH: data collection and edited manuscript draft; SW: data collection and edited manuscript draft; WP: data collection and edited manuscript draft; PT: data collection and edited manuscript draft; AL: study design, treatment implementation, data collection, drafted original manuscript, and incorporated revisions/corresponding author. All authors contributed to the article and approved the submitted version.
Funding
Funding support was provided through the Sustainable Agricultural Systems grant program from USDA-NIFA, award number 2019-69012-29851. The authors acknowledge support of these projects through the Integrated Pennycress Research Enabling Farm and Energy Resilience (IPREFER) group. Salary and research support provided in part by state and federal funds appropriated by the Ohio Agricultural Research and Development Center (OARDC) and The Ohio State University, manuscript number HCS23-12.
Acknowledgments
Some of the data included in this publication are also a component of NK’s MSc thesis entitled Effects of Seed Treatment on Germination and Establishment of Oilseed Pennycress (Thlaspi arvense L.), The Ohio State University, Columbus, OH. We thank Chris Aulbach for his suggestions of seed treatment types to consider. We are also grateful for the field and technical support provided by Allison Pohlmann, Tad Wesley, Kevin Schrock, Glenn Mills, Wayne Lewis, and Matt Davis.
Conflict of interest
The authors declare that the research was conducted in the absence of any commercial or financial relationships that could be construed as a potential conflict of interest.
Publisher’s note
All claims expressed in this article are solely those of the authors and do not necessarily represent those of their affiliated organizations, or those of the publisher, the editors and the reviewers. Any product that may be evaluated in this article, or claim that may be made by its manufacturer, is not guaranteed or endorsed by the publisher.
References
Allmaras R. R., Dowdy R. H. (1985). Conservation tillage systems and their adoption in the United States. Soil Tillage Res. 5, 197–222. doi: 10.1016/0167-1987(85)90030-3
Brummer E. C. (1998). Diversity, stability, and sustainable American agriculture. Agron. J. 90 (1), 1–2. doi: 10.2134/agronj1998.00021962009000010001x
Burns J., Bennet B., Rooney K., Walsh J., Hensley J. (2002). “Coatings for legume and grass seed,” in 57th Southern Pasture and Forage Crop Improvement Conference, Athens, GA, USA. Southern Pasture & Forage Crop Improvement Conference: Texas A&M AgriLife Extension, College Station, TX
Chopra R., Johnson E. B., Emenecker R., Cahoon E. B., Lyons J., Kliebenstein D. J., et al. (2020). Identification and stacking of crucial traits required for the domestication of pennycress. Nat. Food 1 (1), 84–91. doi: 10.1038/s43016-019-0007-z
CoverCress, Inc (2022) Agronomic recommendations. Available at: https://www.covercress.com/agronomic-recommendations.cfm.
Cubins J. A., Wells M. S., Frels K., Ott M. A., Forcella F., Johnson G. A., et al. (2019). Management of pennycress as a winter annual cash cover crop. A review. Agron. Sustain. Dev. 39 (5), 46–57. doi: 10.1007/s13593-019-0592-0
Davis A. S., Hill J. D., Chase C. A., Johanns A. M., Liebman M. (2012). Increasing cropping system diversity balances productivity, profitability and environmental health. PloS One 7 (10):e47149. doi: 10.1371/journal.pone.0047149
Dose H. L., Eberle C. A., Forcella F., Gesch R. W. (2017). Early planting dates maximize winter annual field pennycress (Thlaspi arvense L.) yield and oil content. Ind. Crops Prod 97, 477–483. doi: 10.1016/j.indcrop.2016.12.039
Eberle C. A., Thom M. D., Nemec K. T., Forcella F., Lundgren J. G., Gesch R. W., et al. (2015). Using pennycress, camelina, and canola cash cover crops to provision pollinators. Ind. Crops Prod 75, 20–25. doi: 10.1016/j.indcrop.2015.06.026
Fan J., Shonnard D. R., Kalnes T. N., Johnsen P. B., Rao S. (2013). A life cycle assessment of pennycress (Thlaspi arvense L.) -derived jet fuel and diesel. Biomass Bioenergy 55, 87–100. doi: 10.1016/j.biombioe.2012.12.040
Finch-Savage W. E., Bassel G. W. (2016). Seed vigour and crop establishment: Extending performance beyond adaptation. J. Exp. Bot. 67 (3), 567–591. doi: 10.1093/jxb/erv490
Gaba S., Lescourret F., Boudsocq S., Enjalbert J., Hinsinger P., Journet E. P., et al. (2015). Multiple cropping systems as drivers for providing multiple ecosystem services: from concepts to design. Agron. Sustain. Dev. 35 (2), 607–623. doi: 10.1007/s13593-014-0272-z
Gesch R. W., Archer D. W., Spokas K. (2012). Can using polymer-coated seed reduce the risk of poor soybean emergence in no-tillage soil? Field Crops Res. 125, 109–116. doi: 10.1016/j.fcr.2011.09.005
Groeneveld J. H., Klein A. M. (2015). Pennycress-corn double-cropping increases ground beetle diversity. Biomass Bioenergy 77, 16–25. doi: 10.1016/j.biombioe.2015.03.018
Groeneveld J. H., Lührs H. P., Klein A. M. (2015). Pennycress double-cropping does not negatively impact spider diversity. Agric. For. Entomol 17 (3), 247–257. doi: 10.1111/afe.12100
Hazebroek J. P., Metzger J. D. (1990). Environmental control of seed germination in Thlaspi arvense (Cruciferae). Am. J. Bot. 77 (7), 945–953. doi: 10.1002/j.1537-2197.1990.tb15189.x
Javed T., Afzal I. (2020). Impact of seed pelleting on germination potential, seedling growth and storage of tomato seed. Acta Hortic. 1273, 417–423. doi: 10.17660/ActaHortic.2020.1273.54
Johnson G. A., Kantar M. B., Betts K. J., Wyse D. L. (2015). Field pennycress production and weed control in a double crop system with soybean in minnesota. Agron. J. 107 (2), 532–540. doi: 10.2134/agronj14.0292
Koirala N. (2022). Effects of Seed Treatment on Germination and Establishment of Oilseed Pennycress (Thlaspi arvense L.). Masters of Science Thesis. (Columbus, OH, United States: Ohio State University). Available at: http://rave.ohiolink.edu/etdc/view?acc_num=osu1658311918285856.
Koirala N., Barker D., Gesch R. W., Heller N. J., Hard A. W., Wells S. S., et al. (2023). Seed pelleting and storage effects on germination of pennycress (Thlaspi arvense L.). Crop Sci 63(5):3025–3036. doi: 10.1002/csc2.21077
Koirala N.. (2022). Effects of Seed Treatment on Germination and Establishment of Oilseed Pennycress (Thlaspi arvense L.). Masters of Science Thesis. Ohio State University. Available at: http://rave.ohiolink.edu/etdc/view?acc_num=osu1658311918285856
McGinn M., Phippen W. B., Chopra R., Bansal S., Jarvis B. A., Phippen M. E., et al. (2019). Molecular tools enabling pennycress (Thlaspi arvense) as a model plant and oilseed cash cover crop. Plant Biotechnol. J. 17 (4), 776–788. doi: 10.1111/pbi.13014
Metzger J. D. (1983). Promotion of germination of dormant weed seeds by substituted phthalimides and gibberellic acid. Weed Sci. 31 (3), 285–289. doi: 10.1017/S0043174500069010
Mohammed Y. A., Matthees H. L., Gesch R. W., Patel S., Forcella F., Aasand K., et al. (2020). Establishing winter annual cover crops by interseeding into maize and soybean. Agron. J. 112 (2), 719–732. doi: 10.1002/agj2.20062
Moore V. M., Mirsky S. B. (2020). Cover crop biomass production across establishment methods in mid-Atlantic corn. Agron. J. 112 (6), 4765–4774. doi: 10.1002/agj2.20414
Moore S. A., Scott Wells M., Gesch R. W., Becker R. L., Rosen C. J., Wilson M. L. (2020). Pennycress as a cash cover-crop: Improving the sustainability of sweet corn production systems. Agronomy 10 (5). doi: 10.3390/agronomy10050614
Moser B. R. (2012). Biodiesel from alternative oilseed feedstocks: Camelina and field pennycress. Biofuels 3 (2), 193–209. doi: 10.4155/BFS.12.6
Moser B. R., Knothe G., Vaughn S. F., Isbell T. A. (2009a). Production and evaluation of biodiesel from field pennycress (Thlaspi arvense L.) oil. Energy Fuels 23 (8), 4149–4155. doi: 10.1021/ef900337g
Moser B. R., Shah S. N., Winkler-Moser J. K., Vaughn S. F., Evangelista R. L. (2009b). Composition and physical properties of cress (Lepidium sativum L.) and field pennycress (Thlaspi arvense L.) oils. Ind. Crops Prod 30 (2), 199–205. doi: 10.1016/j.indcrop.2009.03.007
Mousavi-Avval S. H., Shah A. (2020). Techno-economic analysis of pennycress production, harvest and post-harvest logistics for renewable jet fuel. Renewable Sustain. Energy Rev. 123, 109764. doi: 10.1016/j.rser.2020.109764
Mousavi-Avval S. H., Shah A. (2021). Life cycle energy and environmental impacts of hydroprocessed renewable jet fuel production from pennycress. Appl. Energy 297, 117098. doi: 10.1016/j.apenergy.2021.117098
NACSE, O.S.U (2022) PRISM Climate Group. Available at: https://www.prism.oregonstate.edu/explorer/.
National bioeconomy blueprint (2012). Available at: https://obamawhitehouse.archives.gov/sites/default/files/microsites/ostp/national_bioeconomy_blueprint_april_2012.pdf.
Noland R. L., Wells M. S., Sheaffer C. C., Baker J. M., Martinson K. L., Coulter J. A. (2018). Establishment and function of cover crops interseeded into corn. Crop Sci. 58 (2), 863–873. doi: 10.2135/cropsci2017.06.0375
Ott M. A., Gardner G., Rai K. M., Wyse D. L., Marks M. D., Chopra R. (2021). TRANSPARENT TESTA 2 allele confers major reduction in pennycress (Thlaspi arvense L:) seed dormancy. Ind. Crops Prod 174, 114216. doi: 10.1016/j.indcrop.2021.114216
Phippen W., Gallant J., Phippen M. (2010). Evaluation of Planting Method and Seeding Rates with Field Pennycress (Thlaspi arvense L.) (Western Illinois University, Macomb IL: Western Illinois University). Available at: http://www.wiu.edu/pennycress/current-experiments/PlantDensity2010.pdf.
Reed R. C., Bradford K. J., Khanday I. (2022). Seed germination and vigor: ensuring crop sustainability in a changing climate. Heredity 128 (6), 450–459. doi: 10.1038/s41437-022-00497-2
Saini H. S., Bassi P. K., Goudey J. S., Spencer M. S. (1987). Breakage of seed dormancy of field pennycress (Thlaspi arvense) by growth regulators , nitrate , and environmental factors. Weed Sci. 35 (6), 802–806. doi: 10.1017/S0043174500079376
Scott J. M. (1989). Seed coatings and treatments and their effects on plant establishment. Adv. Agron. 42, 43–83. doi: 10.1016/S0065-2113(08)60523-4
Sedbrook J. C., Phippen W. B., Marks M. D. (2014). New approaches to facilitate rapid domestication of a wild plant to an oilseed crop: Example pennycress (Thlaspi arvense L.). Plant Sci. 227, 122–132. doi: 10.1016/j.plantsci.2014.07.008
Su L. Q., Li J. G., Xue H., Wang X. F. (2017). Super absorbent polymer seed coatings promote seed germination and seedling growth of Caragana korshinskii in drought. J. Zhejiang University: Sci. B 18 (8), 696–706. doi: 10.1631/jzus.B1600350
Trejo-Pech C. O., Larson J. A., English B. C., Edward Yu T. (2019). Cost and profitability analysis of a prospective pennycress to sustainable aviation fuel supply chain in southern USA. Energies 12 (16). doi: 10.3390/en12163055
Verhoff K., Phippen W., Heller N., Lindsey A. (2022). Winter-type oilseed pennycress crop staging guide. Crop Forage Turfgrass Manage. 8 (1), 1–9. doi: 10.1002/cft2.20165
Vinod Kumar S., Vyakaranahal B., Deshpande V., Raikar S., Nadaf H., Aravinda Kumar B. (2015). Effect of seed polymer coating on growth and yield of pigeonpea. J. Farm Sci. 27 (4), 19–22.
Weyers S., Thom M., Forcella F., Eberle C., Matthees H., Gesch R., et al. (2019). Reduced potential for nitrogen loss in cover crop-soybean relay systems in a cold climate. J. Enviornmental Qual. 48 (3), 660–669. doi: 10.2134/jeq2018.09.0350
Wohrley B. (2022). Pennycress establishment in response to management of corn residue and harvest date. Masters of Science Thesis. (Normal, IL, United States: Illinois State University), 1583. Available at: https://ir.library.illinoisstate.edu/etd/1583.
Keywords: pennycress, emergence, yield, oilseed, sustainable aviation fuel, crop rotation, seed pelleting, seed treatment
Citation: Koirala N, Barker DJ, Gesch RW, Mohammed YA, Heller NJ, Hard AW, Wells SS, Phippen WB, Tas P and Lindsey AJ (2023) Seed treatment affected establishment and yield in two pennycress lines. Front. Agron. 5:1205259. doi: 10.3389/fagro.2023.1205259
Received: 13 April 2023; Accepted: 18 September 2023;
Published: 12 October 2023.
Edited by:
Senthil-Nathan, Sengottayan, Manonmaniam Sundaranar University, IndiaReviewed by:
Nicole Fiorellino, University of Maryland, College Park, United StatesDavey Jones, Bangor University, United Kingdom
Copyright © 2023 Koirala, Barker, Gesch, Mohammed, Heller, Hard, Wells, Phippen, Tas and Lindsey. This is an open-access article distributed under the terms of the Creative Commons Attribution License (CC BY). The use, distribution or reproduction in other forums is permitted, provided the original author(s) and the copyright owner(s) are credited and that the original publication in this journal is cited, in accordance with accepted academic practice. No use, distribution or reproduction is permitted which does not comply with these terms.
*Correspondence: Alexander J. Lindsey, lindsey.227@osu.edu