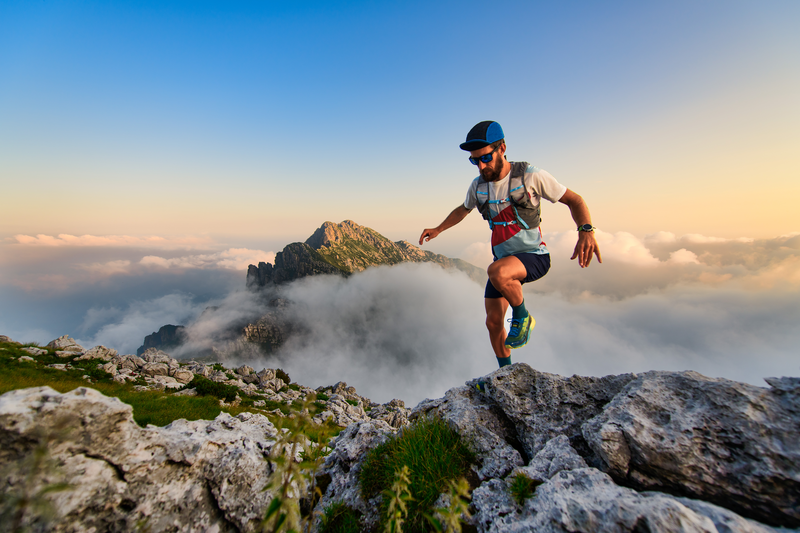
95% of researchers rate our articles as excellent or good
Learn more about the work of our research integrity team to safeguard the quality of each article we publish.
Find out more
ORIGINAL RESEARCH article
Front. Agron. , 10 July 2023
Sec. Weed Management
Volume 5 - 2023 | https://doi.org/10.3389/fagro.2023.1177461
Convolvulus arvensis L. Scop. (field bindweed) is a difficult weed to manage in organic cropping systems. Convolvulus arvensis suppression often requires intensive tillage and cultivation practices that have negative impacts on soil quality. To improve C. arvensis management in organic, small grain cropping systems, we compared ten cropping system treatments in a field trial from the autumn of 2017 until harvest of 2020 in Corvallis, MT, USA. Cropping system treatments varied along a gradient of tillage and crop competition, with tillage intensity and competition inversely related. High soil disturbance and no competition occurred in two treatments that remained in tilled fallow for two consecutive years. Six cropping system treatments had different combinations of tillage and annual or biennial crops, including wheat and forages. Two treatments had minimal soil disturbance and maximum competition from two consecutive years of perennial alfalfa. Convolvulus arvensis ramet density was counted annually to estimate changes in treatments over time. Differences in soil chemical and physical properties between treatments were also assessed in the final years of the study. In the first two years, there was no difference in C. arvensis ramet density among cropping system treatments. In the final two years of the study, C. arvensis density remained below one ramet per m2 in intensively tilled fallow treatments and perennial alfalfa treatments, but was much more variable in treatments with more diverse annual and biennial crop rotations with minimal to moderate tillage. The only differences between treatments in soil physical and chemical properties was aggregate stability. Soil from the most intensively tilled fallow treatments had lower soil aggregate stability than six other treatments, indicating higher erosion potential. Our results suggest that annual cropping systems can lead to increased C. arvensis density. Incorporating a perennial forage or an intensively tilled fallow period can prevent C. arvensis expansion in small grain organic cropping systems, but the perennial forage can protect and enhance soil quality.
Weeds are difficult to manage in organic crop systems and can greatly reduce yields (Gomiero et al., 2011; McBride et al., 2015). Perennial, rhizomatous forbs, including Convolvulus arvensis L. Scop. (field bindweed), have been particularly problematic for organic producers (Tautges et al., 2017b; Orloff et al., 2018). Convolvulus arvensis can reproduce through both seeds and vegetative shoots. The rhizomatous root system of C. arvensis is extensive and allows it to form dense patches in annual small grain cropping systems. Because C. arvensis reestablishes easily from root fragments, cultivation can physically spread the weed across agricultural landscapes (Weaver and Walker, 1982; Sosnoskie et al., 2020). Convolvulus arvensis seeds can also remain viable in soil for up to two decades (Weaver and Walker, 1982). The prolific nature of the root system, long-lived seeds, and competitive growth directly contribute to C. arvensis weediness in both conventional and organic agricultural systems.
Historically, C. arvensis management in agroecosystems involved frequent tillage and soil cultivation practices to disrupt its perennial root system (reviewed by Sosnoskie et al., 2020). In modern conventional cropping systems, C. arvensis seedlings and aboveground shoots are temporarily reduced with chemical herbicides, though long-term reduction of established populations requires repeated herbicide applications (Davis et al., 2018; Bayat and Zargar, 2020). In organic cropping systems, the use of systemic herbicides for suppressing perennial weeds is not allowed. This has led to a reliance on recurrent mechanical cultivation for C. arvensis suppression. Intensive and repeated cultivation has reduced or eradicated C. arvensis in past studies, but cultivation has negative consequences for soil, including increased erosion potential, greater water loss, decreased microbial diversity and abundance, and decreased organic matter (Kasper et al., 2009; Moraru and Teodor, 2010; Soane et al., 2012; Seitz et al., 2019; Carretta et al., 2021).
The negative consequences of intensive soil cultivation and tillage have led to a search for weed management that combines multiple tactics into an integrated weed management plan (Kassam et al., 2010; Lehnhoff et al., 2017; Larson et al., 2021). In organic systems, integrated weed management incorporates biological, cultural, and mechanical practices to suppress weeds (Carr et al., 2012; Benaragama and Shirtliffe, 2013; Lehnhoff et al., 2017). Integrated weed management can provide a more sustainable approach to crop production by reducing the reliance on any single weed management practice. In organic, small grain cropping systems, integrated weed management is often focused on the combination of diverse competitive crop rotations and timely mechanical practices, or sometimes livestock grazing, to remove weed biomass.
Crop rotation is an essential cultural tactic for weed management. Rotations can include a diverse set of crops with different traits such as life cycle or growth habit, which is important for maximizing crop competition. For example, biomass and residue from competitive cover crops has been shown to suppress germination and growth of early-season weeds, specifically annual species, as well as allow for more frequent mechanical management (Teasdale, 1996; Flood and Entz, 2018). However, annual cover crops may be less competitive with rhizomatous perennial weeds. Perennial forage crops, such as alfalfa (Medicago sativa L.), are more competitive with perennial weeds like C. arvensis (Meiss et al., 2010; Tautges et al., 2017a). The deep-reaching root systems allow for perennial crops to compete directly with C. arvensis for resources (e.g., water, soil nutrients).
In water-limited, semi-arid small grain cropping systems, the adoption of cover or forage crops can be challenging due to their soil water use, depression of subsequent cash crop yields, and low biomass production, which inhibits the suppression of weeds (Unger and Vigil, 1998; Carr et al., 2021; DuPre et al., 2022). In organic zero-tillage systems, vegetative mulch from cover crops failed to reduce established perennial weed populations (Carr et al., 2013). Similarly, while integrating livestock grazing into organic cropping systems to remove crop and weed biomass can be an effective way to reduce tillage and provide forage, it has also demonstrated problematic increases in both annual and perennial weed biomass when compared to conventional-no till and organic-tilled systems (Larson et al., 2021). Subsequent increases in weed density can reduce crop yield (Zikeli and Gruber, 2017; Osterholz et al., 2020).
To improve integrated weed management of C. arvensis, we compared ten cropping system treatments for three growing seasons to determine which treatments limited the growth of C. arvensis. Treatments were arranged on a spectrum of both tillage intensity and crop competition. Tillage intensity was inversely related to crop competition. For example, the treatments ranged from a high tillage intensity, low crop competition system with two consecutive years of tilled fallow to a minimal tillage, high crop competition system with two consecutive years of perennial alfalfa. Treatments in the middle of this spectrum had crop rotations with monocot and dicot annual and biennial crops and more moderate tillage intensities. We expected intensively tilled fallow and perennial forage to limit the spread of C. arvensis more than combinations of annual crops and moderate tillage. Repeated and frequent cultivation weakens C. arvensis rhizomes, preventing patch expansion and keeping root density low. However, tillage is expected to lower soil stability and alter soil chemical and physical characteristics compared to treatments with more crop presence. Perennial alfalfa similarly limits C. arvensis density through direct plant competition. Convolvulus arvensis density was expected to be greatest in treatments with annual crops and minimal to moderate tillage because crop competition was weaker and tillage too infrequent to reduce populations.
The field trial was conducted from the autumn of 2017 to the summer of 2020 at the Montana State University Western Agricultural Research Center in Corvallis, MT (46.3274° N, 114.0841° W, 1097 m). The experiment was conducted on a sandy-loam soil in the frigid Typic Haplustoll class, consisting of very deep, well-drained materials formed in alluvium and colluvium. Average annual precipitation for the area is 461 mm, with the majority falling between May and August (Western Regional Climate Center, 2023). A previous crop rotation study comparing crop-weed competition was conducted in the field from the spring of 2015 to the late summer of 2017 and organic certification was maintained for the last two years of the study (Miller, 2017, unpublished).
The study used a randomized complete block design with three replicate blocks. Blocks were separated by 6 m buffer strips. Within each block, ten cropping system treatments were randomly assigned to 6 x 12 m plots. Treatment plots were separated by 1 m buffer strips. In 2015, 48 C. arvensis rhizomes were transplanted into each plot. Rhizomes were between 15 and 20 cm in length.
Each of the ten cropping system treatments combined different crop species and different amounts of mechanical tillage (Tables 1, 2). The cropping system treatments were arranged on a gradient of decreasing tillage intensity and increasing crop competition. Each cropping system treatment lasted three full growing seasons, starting either in 2017 with a fall-planted crop or in 2018 with a spring-planted crop. The maximum amount of soil disturbance occurred in two treatments that were kept in fallow for two years (Tillage 1; Tillage 2). In Tillage 1 and Tillage 2, mechanical cultivation of soil occurred every two to three weeks after initial C. arvensis emergence, usually in late March. Tillage 1 and Tillage 2 also had the lowest amount of crop competition by utilizing two consecutive years of fallow.
Table 1 Crop species planted across ten cropping system treatments, including seeding year and seeding rate (kg ha-1).
Table 2 Timeline of cropping system management for ten cropping system treatments over the four year study period (2017 to 2020), including field operations.
Five cropping system treatments had annual crop species, including mixtures of grain crops (e.g., spring wheat [Triticum turanicum Jakubz.]), green manure cover crops (e.g., field pea [Pisum sativum L.]), and varied tillage intensities. Of this group, the Wheat-CC treatment is a standard farmer practice in the semi-arid wheat-growing areas of North America (Cochran et al., 2006). The Wheat-CC treatment had three to four total tillage events annually, including a pre-plant, a post-harvest, and a final fall disking. Other treatments in this group included Spring Till, Wheat-CC + till, Rye-CC + till, and Reduced Till. The Grazing-CC had increased crop competition by using a biennial sweet clover, and reduction in tillage by using livestock grazing to terminate forage. The remaining two treatments used a two-year perennial alfalfa rotation (Conventional Alfalfa; Organic Alfalfa), which constituted maximum competition and no soil disruption. In 2020, all ten treatments were planted with Khorasan spring wheat (Triticum turanicum Jakubz.; Kamut ®). Seeding rates can be found in Table 1.
In total, four different field implements were used among the treatments for mechanical control of C. arvensis: a moldboard plow, a rototiller, a disc, and a flail mower (for cover crop termination). The moldboard plow and rototiller were used in the most intensively tilled fallow treatment (Tillage 1). The rototiller had a 15 cm maximum depth and was applied during the growing season, starting two weeks after C. arvensis emergence. The moldboard plow had a 25 cm depth and was used in early fall. A disc was used in other treatments requiring tillage at a 15 cm maximum depth. Cover crops were terminated with the flail mower and then incorporated with the disc. Conventional Alfalfa had two initial glyphosate applications (GlyStar® Plus; 2017: 1121 g ai ha-1; 2018: 3365 g ai ha-1). One treatment (Grazing-CC) utilized livestock grazing with sheep to terminate a biennial forage crop in the second year of growth. Further details on field actions can be found in Table 2.
Convolvulus arvensis ramet counts were initiated in the summer of 2017, prior to fall crop planting. Subsequent ramet counts were conducted annually in each plot before crop termination or harvest (either July or August). Convolvulus arvensis ramets were counted inside the core of the plot (4 x 10 m). We excluded a 1 m buffer around the plot to avoid edge effects. In 2017, 2019, and 2020, in addition to density, four 0.25 m2 frames were randomly placed parallel to crop rows in the core of the plot. All biomass was cut, separated into C. arvensis, crop, and other weed biomass. The biomass was then dried and weighed. In the final year of the cropping treatments, wheat grain yield in each plot was estimated from a combine mounted yield monitor. Yield estimates were calculated from a 17 m2 area in the center of the plot.
Soil was sampled from each plot twice: once in summer of 2019 for slake stability testing and once before planting spring wheat in 2020 for soil nutrient analysis. A total of 18 samples were taken on the surface of each plot for slake stability testing. The slake test was performed to measure the stability of soil aggregates when exposed to rapid wetting as a proxy for soil erosion (United States Department of Agriculture, 1999). Stability class was scored based on criteria for soil structural integrity on a scale from 0 to 6, with 0 indicating a soil too unstable to sample and 6 indicating 75-100% of soil remains intact after five wetting events. A stability class of less than 4 is generally considered unstable (United States Department of Agriculture, 1999). In 2020, soil was resampled from all plots and sent to Agvise Laboratories (Northwood, ND, USA) for soil physical and chemical analysis, which included analyses of P, K, Zn, Ca, Mg, N, CEC, organic matter, and pH. In 2020, soils were also analyzed for microaggregate, macroaggregate, and large macroaggregate percentages.
We assessed changes in C. arvensis ramet density in each plot annually over the four-year period, beginning with an initial count in 2017 before treatments started and ending at harvest in 2020. We analyzed density using a linear mixed effects model (`lmer` in R-package ‘lme4’; Bates et al., 2015). The model had the fixed effects of year, cropping system treatment, and the interaction between the two variables. Block and a unique plot identifier were added as random effects to account for grouping of replicate blocks and the repeated measures of the same plots over time. Convolvulus arvensis ramet density was scaled to ramets per m2 and log-transformed to meet assumptions of normality. Type-III ANOVA (`anova` in R-package ‘lmerTest’; Kuznetsova et al., 2017) was used to determine if year, treatment, or their interaction accounted for variation in C. arvensis densities. Estimated marginal means of C. arvensis ramet density were calculated and compared using the Tukey post-hoc method (`emmeans` in the R-package ‘emmeans’; Lenth, 2021). We estimated marginal and conditional R2 using the `r.squaredGLMM` function in ‘MuMIn’ package (Bartoń, 2023). The marginal R2 describes proportionally the variance explained by the fixed effects, whereas the conditional R2 describes the variance explained by both fixed and random effects (Nakagawa & Schielzeth, 2013). We correlated density and biomass of C. arvensis in plots using density and biomass data from 2019 and 2020.
Interannual rates of ramet density change were calculated using the common growth rate equation, [relative growth rate = ln(Nt+1/Nt)], where N is the density of ramets in a plot, and t is an annual time step (Vandermeer and Goldberg, 2013). We fit a linear mixed effects model with transition period (e.g., 2017 to 2018), cropping system treatment, and their interaction as the fixed effects, and block and unique plot identifier as the random effects. Estimated marginal means were calculated and compared using the Tukey post-hoc method (`emmeans` in the R-package ‘emmeans’; Lenth, 2021). To determine if growth rates were positive or negative between transition periods, the estimated marginal means were tested against the default of zero (relative growth rate = 0) using a reference grid and `test` function in the R-package “emmeans” (Lenth, 2021).
To assess if soil chemical characteristics differed among treatments, we tested individual soil parameters using linear mixed effects models with treatment as a fixed effect and block as a random effect. To assess soil stability, slake test data were scored as integer counts and analyzed using a generalized linear model with a Poisson distribution (Carpenter and Chong, 2010). Post-hoc Tukey comparisons using the ‘emmeans’ package (Lenth, 2021) were conducted to assess differences in soil aggregate stability between treatments.
All statistical analyses and graphics were performed and produced in the statistical environment R, version 4.0.2 (R Core Team, 2020; graphics produced using R-packages “ggplot2” (Wickham, 2016) and “cowplot” (Wilke, 2019)). Linear mixed effects models were assessed visually for normality and homoscedasticity. Differences between all explanatory and response variables for all models were analyzed at the P < 0.05 level.
The interaction between year and cropping system treatment affected C. arvensis ramet density (P < 0.001; Table 3; Figure 1). The fixed effects of year, cropping system treatment, and their interaction explained 42% of variation (marginal R2 = 0.42), and the combined fixed and random (block and plot) effects accounted for 71% of variation (conditional R2 = 0.71). There were no differences in C. arvensis ramet density among cropping system treatments in the first (2017) and second year (2018) of the study (Supplemental Table 1.1). In the first two years, mean C. arvensis density for all treatments ranged between 0.3 and 1.3 ramets per m2 (Figure 1; Supplemental Table 1.1). In the third year (2019), C. arvensis ramet density differed among treatments (Figure 1; Supplemental Table 1.1). Six cropping system treatments had C. arvensis densities that remained between 0 and 1 ramet per m2, including Tillage 1 (0.01 ramets/m2 ± 0.01 SE), Tillage 2 (0.1 ± 0.1), Conventional Alfalfa (0.2 ± 0.2), Organic Alfalfa (0.09 ± 0.09), Spring Till (0.4 ± 0.4), and Reduced Till treatments (1.0 ± 1.0). In this year, Tillage 1 had significantly lower C. arvensis ramet density than four other treatments: Wheat-CC + till (P = 0.005; 4.0 ± 4.0), Grazing-CC (P = 0.01; 3.0 ± 3.0), Rye-CC + till (P = 0.01; 2.8 ± 2.8), and Wheat-CC (P = 0.01; 2.5 ± 2.5; Supplemental Table 1.1).
Table 3 Analysis of variance (ANOVA) for a linear mixed effects model of Convolvulus arvensis density on the log scale as effected by year (e.g., 2017), cropping system treatment, and their interaction.
Figure 1 Estimated means of ramet density of Convolvulus arvensis per m2 among ten cropping system treatments over a four-year period (2017-2020).
In the final year of the study (2020), Tillage 1 (0.01 ± 0.01) had lower mean C. arvensis density than the Reduced Till treatment (1.7 ± 1.7), Rye-CC + till treatment (2.8 ± 2.8), the Wheat-CC + till treatment (5.1 ± 5.0), and the Grazing-CC treatment (6.7 ± 6.7; Figure 1; Supplemental Table 1.1). The Conventional Alfalfa treatment (0.04 ± 0.04) also had lower C. arvensis density than the Grazing-CC treatment (P = 0.02) and the Reduced Till treatment (P = 0.03). At the end of the study, treatments with the highest C. arvensis densities (Grazing-CC and Rye-CC + till) had crop rotations with multiple years of either annual or biennial cover crops and minimal to moderate tillage. In contrast, the treatments with the lowest C. arvensis densities had perennial alfalfa or frequent tillage throughout the growing season.
The interannual relative growth rates of C. arvensis varied in response to cropping system treatment (P = 0.04) and the interaction of treatment and transition period (P = 0.005; Table 4). Over the course of the study (2017 to 2020), the relative growth rate of C. arvensis density was negative in the Tillage 1 treatment (P < 0.01; Figure 2A; Supplemental Table 1.2). Over the same period, the relative growth rate of C. arvensis density was positive in the Grazing-CC (P < 0.01; Figure 2A). The average relative growth rates of the remaining eight treatments did not significantly differ from zero over the course of the study (Figure 2A). However, certain cropping system treatments did show significant changes in relative growth rates within years of the study.
Table 4 Analysis of variance (ANOVA) for a linear mixed effects model of Convolvulus arvensis relative population growth rate in response to transition period (e.g., 2017-2018), cropping system treatment, and their interaction.
Figure 2 Convolvulus arvensis mean relative growth rate (change in ramet density over time) with 95% confidence intervals for relative population growth rates by cropping system treatment, as derived from the underlying linear mixed-effects model for the entirety of the study period from 2017 to 2020 (A), 2017 to 2018 (B), 2018 to 2019 (C), and 2019 to 2020 (D). Green lines indicate a significant decrease in relative growth (e.g., significantly less than zero) over the given time interval (P < 0.05). Blue lines indicate a significant increase in relative growth (e.g., significantly greater than zero) over the time interval (P < 0.05). Purple lines indicate relative growth rate does not differ from zero.
The average relative growth rate of C. arvensis in the Reduced Till plots was negative during the first transition period (2017-2018; relative growth rate = -1.64; see Figure 2B) but significantly increased in the second transition (2018-2019; relative growth rate = 2.24; see Figure 2C). In the Reduced Till treatment, the C. arvensis growth rate increase coincided with a reduction in tillage and the second season of an annual cover crop mix. During the same period, C. arvensis relative growth rate in the Tillage 1 plots decreased (relative growth rate = -3.54), in conjunction with the start of intensive, consistent mechanical tillage (Figure 2C). Convolvulus arvensis relative growth rate was also negative (relative growth rate = -1.95) in the Organic Alfalfa treatment during the second transition period (from 2017-2018 to 2018-2019; Figure 2C), which corresponded to the second year of perennial crop growth. Finally, C. arvensis growth rate decreased in the Wheat-CC treatment from the second to third transition period (from 2018-2019 to 2019-2020; Figure 2D), which may be due in part to the planting of a more competitive wheat grain crop instead of a broadleaf annual crop.
Over the course of the study, estimated C. arvensis biomass was significantly correlated with plot density (cor = 0.72). In the final study year (2020), wheat yields did not vary by treatment (P = 0.3; Supplemental Table 1.3). Soil aggregate percentages and most soil chemical properties also did not vary by treatment (Supplemental Tables 1.4 – 1.7). Slake soil stability scores did vary by cropping system treatment (P < 0.001; Figure 3). The Tillage 1 treatment had lower soil stability compared to all other treatments (P < 0.001), except the Tillage 2 treatment (Figure 3). Tillage 1 had a mean score of 3.6, indicating that less than 10% of soil remained on the sieve after five wetting cycles (USDA, 1999). Tillage 2 differed from all treatments (4.0; P = 0.001), except the Spring Till treatment and the Wheat-CC + till treatment. The remaining treatments had mean scores of 5 or higher, indicating that 25% to 75% of soil remained on the testing sieve after five wetting cycles (United States Department of Agriculture, 1999).
Figure 3 Mean slake soil stability scores among ten cropping system treatments with 95% confidence intervals. Letters indicate significant post-hoc differences in soil stability among cropping system treatments (P < 0.05).
Perennial rhizomatous weeds in organic, small grain cropping systems are difficult to manage (OAEC, 2013; DeDecker et al., 2014; Tautges et al., 2017b; Orloff et al., 2018). In this experiment, we recorded increases in mean C. arvensis density in certain annual and biennial crop rotations with minimal to moderate tillage. By the fourth growing season, C. arvensis mean densities were largest in the Grazing-CC treatment, which incorporated livestock grazing and a biennial sweet clover crop. Extensively tilled fallow plots (Tillage 1, Tillage 2) and alfalfa forage (Conventional Alfalfa, Organic Alfalfa) plots had consistently low mean C. arvensis densities (Supplemental Table 1.1). Tilled fallow decreased C. arvensis density over the course of the study. However, tilled treatments also had lower soil aggregate stability than other treatments, and therefore a greater erosion potential. Our research suggests incorporation of periods of perennial crops or extensively tilled fallow may maintain lower C. arvensis populations in organic cropping systems, while prolonged use of annual crops in rotation or large reduction in tillage can allow for expansion for C. arvensis populations.
Annual cropping systems in this study had more varied changes in C. arvensis density than tilled fallow or perennial forage cropping system treatments. Following an initial lag in density changes, our study found C. arvensis densities increased in both annual and biennial cropping systems in years where a broadleaf forage crop was sown (Supplemental Table 1.1). For example, the standard wheat cover crop rotation (Wheat-CC) had low C. arvensis density in the initial wheat phase, which then increased in the field pea phase, and decreased during the final spring wheat phase.
Annual cover crops vary in their ability to suppress weeds in dryland agroecosystems and have produced mixed results depending on crop type (Kumar et al., 2020). Cereals tend to be more competitive with perennial weeds than broadleaf annuals due to increased residue cover (Derksen et al., 2002; Lensenn et al., 2015; Ghimire et al., 2018). Biennial sweet clover (Melilotus officinalis L.), especially when undersown with other crops, has suppressed perennial weeds in dryland cropping systems due to high biomass production (Schoofs and Entz, 2000; Blackshaw et al., 2001). However, research in Montana demonstrated the integration of a sweet clover cover crop and sheep grazing to produce high weed density and low subsequent wheat yields (Larson et al., 2021). In our study, the cropping system treatment with biennial sweet clover had one of the highest densities of C. arvensis. In the initial establishment phase during the first year of the biennial life cycle, the competitive ability of sweet clover is limited, though in both years the density of C. arvensis increased. Because some annual crops have limited competitive ability and tillage can only be used in certain stages, annual cropping systems are likely more suspectable to increases in C. arvensis and overall more variable in their suppression of weeds.
In comparison to the annual crop rotations, C. arvensis density did not increase in perennial alfalfa treatments. In a review of C. arvensis distribution in the United States, Boldt et al. (1998) reported increased C. arvensis infestations after land was removed from the Conservation Reserve Program, suggesting the transition from perennial cover to annual crops allowed for spread of the more competitive perennial C. arvensis. Meiss et al. (2010) similarly reported perennial crop fields to have lower perennial weed populations (specifically C. arvensis) than annual crop fields. Our study demonstrates that perennial crops were associated with lower C. arvensis populations.
The competitive ability of alfalfa with perennial cropland weeds has been well-documented (Meiss et al., 2010; Tautges et al., 2017a; Favrelière et al., 2020), but in this paper we show that it can be used successfully for weed management in organic cropping systems. Integrating an alfalfa phase into conventional small grain cropping system can suppress perennial weeds better than continuous cereal crops (Ominski et al., 1999). In organic systems specifically, Grosse et al. (2021) demonstrated that the choice of a perennial alfalfa-grass crop rotation was the most important factor for managing perennial weeds. Our results suggest that incorporating competitive perennial crops, particularly alfalfa, can effectively prevent the growth of C. arvensis populations.
The success of the intensive tillage-fallow treatment confirms the results of historical studies, which saw reduced or even eradicated C. arvensis populations using thorough and repeated mechanical disturbance (as reviewed by Sosnoskie et al., 2020). In the dryland, organic cropping systems of the Northern Great Plains, soil deterioration due to increased mechanical cultivation is a threat. Though we found similar soil chemical properties among the cropping system treatments, physical soil stability was significantly lower in the intensively tilled fallow compared to the treatments where crops were grown consecutively. While successful in decreasing C. arvensis populations, the adverse effects of such extensive tillage have made it impractical and unsustainable for wide-scale weed management. Alternatively, while no-tillage or reduced tillage is less damaging for soil stability, it has been shown to increase perennial weed presence due to insufficient reduction of underground rhizomes (Hume et al., 1991; Menalled et al., 2001; Buhler, 2002).
Overall, we demonstrated that annual crop-dominated systems can lead to increases in C. arvensis populations, especially in livestock-integrated systems. Intensively tilled fallow reduced C. arvensis populations but was also associated with low soil aggregate stability. Integrating consecutive years of alfalfa into grain production suppressed C. arvensis populations as well as intensive tillage. To improve the sustainability of weed management in dryland organic grain systems, incorporation of a perennial ley phase is recommended to both suppress perennial weeds and reduce dependence on mechanical cultivation.
The raw data supporting the conclusions of this article will be made available by the authors, without undue reservation.
KHe and TS: performed data analysis and wrote the manuscript. ZM and KHu: conceptualized the study, implemented study, and collected data. All authors contributed to the article and approved the submitted version.
This work was supported by a USDA-NIFA-OREI Project Award (2018-51300-28432).
The authors would like to thank the staff at the Western Agricultural Research Center for their contributions to the field experiments. The authors would also like to thank Dr. Lisa Rew, Dr. Patrick Carr, and Christian Larson for their invaluable edits to draft versions of the manuscript.
The authors declare that the research was conducted in the absence of any commercial or financial relationships that could be construed as a potential conflict of interest.
All claims expressed in this article are solely those of the authors and do not necessarily represent those of their affiliated organizations, or those of the publisher, the editors and the reviewers. Any product that may be evaluated in this article, or claim that may be made by its manufacturer, is not guaranteed or endorsed by the publisher.
The Supplementary Material for this article can be found online at: https://www.frontiersin.org/articles/10.3389/fagro.2023.1177461/full#supplementary-material
Bartoń K. (2023) R package ‘MuMIn’. r version 1.47.5. Available at: https://cran.r-project.org/web/packages/MuMIn/MuMIn.pdf.
Bates D., Mächler M., Bolker B., Walker S. (2015). Fitting linear mixed-effects models using lme4. J. Stat. Softw 67, 1–48. doi: 10.48550/arXiv.1406.5823
Bayat M., Zargar M. (2020). Field bindweed (Convolvulus arvensis) control and winter wheat response to post herbicides application. J. Crop Sci. Biotechnol. 23, 149–155. doi: 10.1007/s12892-019-0213-0
Benaragama D., Shirtliffe S. J. (2013). Integrating cultural and mechanical methods for additive weed control in organic systems. Agron. J. 105, 1728–1734. doi: 10.2134/agronj2013.0007
Blackshaw R. E., Moyer J. R., Doram R. C., Boswell A. L. (2001). Yellow sweetclover, green manure, and its residues effectively suppress weeds during fallow. Weed Sci. 49, 406–413. doi: 10.1614/0043-1745(2001)049[0406:YSGMAI]2.0.CO;2
Boldt P. E., Rosenthal S. S., Srinivasan R. (1998). Distribution of field bindweed and hedge bindweed in the USA. J. Prod Agric. 11, 377–381. doi: 10.2134/jpa1998.0377
Buhler D. D. (2002). 50th anniversary – invited article: challenges and opportunities for integrated weed management. Weed Sci. 50, 273–280. doi: 10.1614/0043-1745(2002)050[0273:AIAAOF]2.0.CO;2
Carpenter D. R., Chong G. W. (2010). Patterns in the aggregate stability of mancos shale derived soils. Catena 80, 65–73. doi: 10.1016/j.catena.2009.09.001
Carr P. M., Anderson R. L., Lawley Y. E., Miller P. R., Zwinger S. F. (2012). Organic zero-till in the northern US great plains region: opportunities and obstacles. Renewable Agric. Food Syst. 27, 12–20. doi: 10.1017/S174217051100041X
Carr P. M., Gramig G. G., Liebig M. A. (2013). Impacts of organic zero tillage systems on crops, weeds, and soil quality. Sustainability, 5 (7), 3172–3201. doi: 10.3390/su5073172
Carr P. M., Bell J. M., Boss D. L., DeLaune P., Eberly J. O., Edwards L., et al. (2021). Annual forage impacts on dryland wheat farming in the great plains. Agron. J. 113, 1–25. doi: 10.1002/agj2.20513
Carretta L., Tarolli P., Cardinali A., Nasta P., Romano N., Masin R. (2021). Evaluation of runoff and soil erosion under conventional tillage and no-till management: a case study in northeast Italy. CATENA 197, 104972. doi: 10.1016/j.catena.2020.104972
Cochran V., Danielson J., Kolberg R., Miller P. (2006). “Dryland cropping in the Canadian prairies and the U.S. Northern great plains. 2nd Edn. G. A. Peterson, P. W. Unger, & W. A. Payne (Eds.)” in Dryland agriculture 293–339. doi: 10.2134/agronmonogr23.2ed.c9
Davis S., Mangold J., Menalled F., Orloff N., Miller Z., Lehnhoff E. (2018). A meta-analysis of field bindweed (Convolvulus arvensis) management in annual and perennial systems. Weed Sci. 66, 540–547. doi: 10.1017/wsc.2018.25
DeDecker J. J., Masiunas J. B., Davis A. S., Flint C. G. (2014). Weed management practice selection among Midwest U.S. organic growers. Weed Sci. 62, 520–531. doi: 10.1614/WS-D-13-00138.1
Derksen D. A., Anderson R. L., Blackshaw R. E., Maxwell B. (2002). Weed dynamics and management strategies for cropping systems in the northern great plains. Agron. J. 94, 174–185. doi: 10.2134/agronj2002.1740
DuPre M. E., Seipel T., Bourgault M., Boss D. L., Menalled F. D. (2022). Predicted climate conditions and cover crop composition modify weed communities in semiarid agroecosystems. Weed Res. 62, 38–48. doi: 10.1111/wre.12514
Favrelière E., Ronceux A., Pernel J., Meynard J.-M. (2020). Nonchemical control of a perennial weed, cirsium arvense, in arable cropping systems. a review. Agron. Sustain. Dev. 40, 31. doi: 10.1007/s13593-020-00635-2
Flood H. E., Entz M. H. (2018). Effects of a fall rye cover crop on weeds and productivity of phaseolus beans. Can. J. Plant Sci. 99, 22–33. doi: 10.1139/cjps-2018-0161
Ghimire R., Ghimire B., Mesbah A. O., Idowu O. J., O’Neill M. K., Angadi S. V., et al. (2018). Current status, opportunities, and challenges of cover cropping for sustainable dryland farming in the southern great plains. J. Crop Improvement 32, 579–598. doi: 10.1080/15427528.2018.1471432
Gomiero T., Pimentel D., Paoletti M. G. (2011). Environmental impact of different agricultural management practices: conventional vs. organic agriculture. Crit. Rev. Plant Sci. 30, 95–124. doi: 10.1080/07352689.2011.554355
Grosse M., Haase T., Heß J. (2021). Varying tillage promotes weed diversity, while a perennial alfalfa-grass mixture promotes weed control in an organic tillage system experiment in Germany. Renewable Agric. Food Syst. 36, 465–476. doi: 10.1017/S1742170521000053
Hume L., Tessier S., Dyck F. B. (1991). Tillage and rotation influences on weed community composition in wheat (Triticum aestivum l.) in southwestern Saskatchewan. Can. J. Plant Sci. 71, 783–789. doi: 10.4141/cjps91-112
Kasper M., Buchan G. D., Mentler A., Blum W. E. H. (2009). Influence of soil tillage systems on aggregate stability and the distribution of c and n in different aggregate fractions. Soil Tillage Res. 105, 192–199. doi: 10.1016/j.still.2009.08.002
Kassam A., Friedrich T., Derpsch R. (2010). Conservation agriculture in the 21st century: a paradigm of sustainable agriculture (European Congress on Conservation Agriculture, European Conservation Agriculture Federation (ECAF); Madrid, Spain).
Kumar V., Obour A., Jha P., Liu R., Manuchehri M. R., Dille J. A., et al. (2020). Integrating cover crops for weed management in the semiarid U.S. great plains: opportunities and challenges. Weed Sci. 68, 311–323. doi: 10.1017/wsc.2020.29
Kuznetsova A., Brockhoff P. B., Christensen R. H. B. (2017). lmerTest package: tests in linear mixed effects models. J. Stat. Softw 82, 1–26. doi: 10.18637/jss.v082.i13
Larson C. D., Menalled F. D., Lehnhoff E. A., Seipel T. (2021). Plant community responses to integrating livestock into a reduced-till organic cropping system. Ecosphere 12, e03412. doi: 10.1002/ecs2.3412
Lehnhoff E., Miller Z., Miller P., Johnson S., Scott T., Hatfield P., et al. (2017). Organic agriculture and the quest for the holy grail in water-limited ecosystems: managing weeds and reducing tillage intensity. Agriculture 7, 33. doi: 10.3390/agriculture7040033
Lensenn A. W., Sainju U. M., Jabro J. D., Allen B. L., Evans R. G. (2015). Managment and tillage influence barley forage productivity and water use in dryland cropping systems. Agron. J. 107, 551–557. doi: 10.1002/agj2.20513
Lenth R. (2021) Emmeans: estimated marginal means, aka least-squares means. r package version 1.8.2. Available at: https://CRAN.R-project.org/package=emmeans.
McBride W. D., Greene C., Foreman L., Ali M. (2015). The profit potential of certified organic field crop production (USDA Economic Research Service: Washington, DC, United States). Report Number 188.
Meiss H., Médiène S., Waldhardt R., Caneill J., Muiner-Jolain N. (2010). Contrasting weed species composition in perennial alfalfas and six annual crops: implications for integrated weed management. Agron. Sustain. Dev. 30, 657–666. doi: 10.1051/agro/2009043
Menalled F. D., Gross K. L., Hammond M. (2001). Weed aboveground and seedbank community responses to agricultural management systems. Ecol. Appl. 11, 1586–1601. doi: 10.1890/1051-0761(2001)011[1586:WAASCR]2.0.CO;2
Moraru P., Teodor R. (2010). Soil tillage conservation and its effect on soil organic matter, water management and carbon sequestration. J. Food Agric. Environ. 8, 309–312.
Nakagawa S., Schielzeth H. (2013). Nested by design: model fitting and interpretation in a mixed model era. Methods Ecol. Evol. 4, 14–24. doi: 10.1111/j.2041-210x.2012.00251.x
Ominski P. D., Entz M. H., Kenkel N. (1999). Weed suppression by medicago sativa in subsequent cereal crops: a comparative survey. Weed Sci. 47, 282–290. doi: 10.1017/S0043174500091785
Organic Advisory and Education Council (OAEC) (2013) Report on the survey of research and educational needs of organic grain producers in Montana. Available at: http://www.oaecmt.org/reports.html.
Orloff N., Mangold J., Miller Z., Menalled F. (2018). A meta-analysis of field bindweed (Convolvulus arvensis L.) and Canada thistle (Cirsium arvense L.) management in organic agricultural systems. Agricult Ecosyst. Environ. 254, 264–272. doi: 10.1016/j.agee.2017.11.024
Osterholz W. R., Culman S. W., Herms C., Joaquim de Oliveira F., Robinson A., Doohan D. (2020). Knowledge gaps in organic researcher: understanding interactions of cover crops and tillage for weed control and soil health. Org Agric. 11, 13–25. doi: 10.1007/s13165-020-00313-3
R Core Team (2020). R: a language and environment for statistical computing (Vienna, Austria: R Foundation for Statistical Computing).
Schoofs A., Entz M. H. (2000). Influence of annual forages on weed dynamics in a cropping system. Can. J. Plant Sci. 80, 187–198. doi: 10.4141/P98-098
Seitz S., Goebes P., Puerta V. L., Perira E. I. P., Wittwer R., Six J., et al. (2019). Conservation tillage and organic farming reduce soil erosion. Agron. Sustain. Dev. 39, 4. doi: 10.1007/s13593-018-0545-z
Soane B. D., Ball B. C., Arvidsson J., Basch G., Moreno F., Roger-Estrade J. (2012). No-till in northern, western and south-western Europe: a review of problems and opportunities for crop production and the environment. Soil Tillage Res. 118, 66–87. doi: 10.1016/j.still.2011.10.015
Sosnoskie L. M., Hanson B. D., Steckel L. E. (2020). Field bindweed (Convolvulus arvensis): “all tied up”. Weed Technol. 34, 916–921. doi: 10.1017/wet.2020.61
Tautges N. E., Burke I. C., Borrelli K., Fuerst E. P. (2017a). Competitive ability of rotational crops with weeds in dryland organic wheat production systems. Renewable Agric. Food Syst. 32, 57–68. doi: 10.1017/S1742170516000028
Tautges N., Goldberger J., Burke I. (2017b). A survey of weed management in organic small grains and forage systems in the Northwest united states. Weed Sci. 64, 513–522. doi: 10.1017/S1742170516000028
Teasdale J. R. (1996). Contribution of cover crops to weed management in sustainable agricultural systems. J. Prod Agric. 9, 475–479. doi: 10.2134/jpa1996.0475
Unger P. W., Vigil M. F. (1998). Cover crop effects on soil water relationships. J. Soil Water Conserv. 53, 200–207.
United States Department of Agriculture (1999) Soil quality test kit guide 20-21. Available at: https://www.nrcs.usda.gov/sites/default/files/2022-10/Soil%20Quality%20Test%20Kit%20Guide.pdf.
Vandermeer J. H., Goldberg D. E. (2013). Population ecology: first principles - second edition (United Kingdom: Princeton University Press).
Weaver S. E., Walker R. R. (1982). The biology of Canadian weeds. 53. convolvulus arvensis l. Can. J. Plant Sci. 62, 461–472. doi: 10.4141/cjps82-066
Western Regional Climate Center (2023) Period of record monthly climate summary (Corvallis, MT). Available at: https://wrcc.dri.edu/cgi-bin/cliMAIN.pl?mt8783.
Wickham H. (2016). ggplost2: Elegant Graphics for Data Analysis (New York: Springer). doi: 10.1007/978-3-319-24277-4
Wilke C. O. (2016) Cowplot: streamlined plot theme and plot annotations for ‘ggplot2’. r version 1.1.1. Available at: https://cran.r-project.org/web/packages/cowplot/index.html.
Keywords: Convolvulus arvensis, organic agriculture, field bindweed, perennial weed management, IWM
Citation: Hettinger K, Miller Z, Hubbel K and Seipel T (2023) Crop rotation and cultivation effects on Convolvulus arvensis population dynamics in small grain organic cropping systems. Front. Agron. 5:1177461. doi: 10.3389/fagro.2023.1177461
Received: 01 March 2023; Accepted: 09 June 2023;
Published: 10 July 2023.
Edited by:
Ali Ahsan Bajwa, NSW Government, AustraliaReviewed by:
Eshagh Keshtkar, Tarbiat Modares University, IranCopyright © 2023 Hettinger, Miller, Hubbel and Seipel. This is an open-access article distributed under the terms of the Creative Commons Attribution License (CC BY). The use, distribution or reproduction in other forums is permitted, provided the original author(s) and the copyright owner(s) are credited and that the original publication in this journal is cited, in accordance with accepted academic practice. No use, distribution or reproduction is permitted which does not comply with these terms.
*Correspondence: Kara Hettinger, a2FyYWhldHRpbmdlcjExQGdtYWlsLmNvbQ==
Disclaimer: All claims expressed in this article are solely those of the authors and do not necessarily represent those of their affiliated organizations, or those of the publisher, the editors and the reviewers. Any product that may be evaluated in this article or claim that may be made by its manufacturer is not guaranteed or endorsed by the publisher.
Research integrity at Frontiers
Learn more about the work of our research integrity team to safeguard the quality of each article we publish.