- 1Department of Crop Production Ecology, Swedish University of Agricultural Sciences, Uppsala, Sweden
- 2Department of Soil and Environment, Swedish University of Agricultural Sciences, Uppsala, Sweden
- 3Department of Plant Biology, Uppsala BioCenter, Swedish University of Agricultural Sciences, Uppsala, Sweden
- 4Linnean Center for Plant Biology in Uppsala, Swedish University of Agricultural Sciences, Uppsala, Sweden
Wheat is considered an established crop with a long breeding history. Its varieties are being developed with differential breeding targets, e.g. high grain yield or high grain protein content. The different breeding targets strongly influence performance in the targeted traits, but may also influence non-target traits related to grain quality, biofortification potential, and nutrient accumulation. This influence of non-targeted traits may, in turn, affect the environmental performance and ecological sustainability of the crop. The aim of this study was to evaluate the relationships between breeding target traits such as grain yield and grain quality, and non-target traits for three groups of spring wheat varieties differing in breeding targets, i.e. high yield (I), organic high protein (II), and intermediate (III) wheat types. Data from a field trial with nine spring wheat varieties grown under two soil compaction treatments over two years with contrasting weather were used. We found that wheat type affected most target and non-target traits with partly large effect sizes , but not nitrogen (N) uptake efficiency ( =0.006), which reflects the potential N resource requirement of the crop. Associations shown between target and non-target traits will be advantageous for wheat breeding programs. Wheat type and environment had similarly sized effects on grain yield and quality traits. Grain concentrations of various macro- and micro-nutrients were frequently, but not always, correlated, indicating that the biofortification potential varied between wheat types and was affected by environmental conditions. The grain and starch yields per accumulated plant N were higher in the wheat varieties bred for high grain yields than those bred for high grain protein content; whilst the protein yield per accumulated whole-plant N was similar across all wheat types despite much higher grain N concentrations in the high-protein varieties. Additionally, most of the evaluated traits tended to preserve their static stability across environments. The results link grain yield and grain quality traits to crop nutrient accumulation aspects relevant for the environmental performance and ecological sustainability of the crop. Future breeding strategies should consider the non-target effects in traits that influence the environmental performance of the crop.
1 Introduction
One of the main challenges of contemporary agriculture is to fulfil the nutrition requirements of growing populations by producing safe, nutritional, and high-quality food without harming biodiversity or depleting limited resources (Dwivedi et al., 2017). Wheat is recognized as one of the big three cereal crops globally (Baye et al., 2020) and plays a key role as a human food source. Compared to maize and rice, wheat has a greater amount of protein, turning it into one of the most important staple foods worldwide (Mecha et al., 2017).
During the domestication process, selection of desirable traits such as the loss of seed shattering, and the free-threshing naked seed form, were selected because these characteristics increased the chance of achieving higher grain yield (Shewry, 2009). Since then, wheat breeding targets have been focused mostly on the improvement of grain yield and thousand-kernel weight (i.e., two traits that here are considered as “yield traits”), disease resistance, and seed maturity traits. However, the attention has shifted to include grain quality traits, with those enhancing end-use quality becoming vital to wheat breeders (Table 1). Nevertheless, the genetic bottlenecks due to strong directed selection in the breeding of target traits have negatively affected some non-target traits that have potential in tackling challenges such as climate change and food security. Biotic and abiotic stress related traits have been considered main target traits due to economic impact on the yield, while morphological traits have not been extensively identified and exploited yet (Yong et al., 2004; Charmet, 2011; Dempewolf et al., 2017; Amiri et al., 2018; Pourazari et al., 2018).
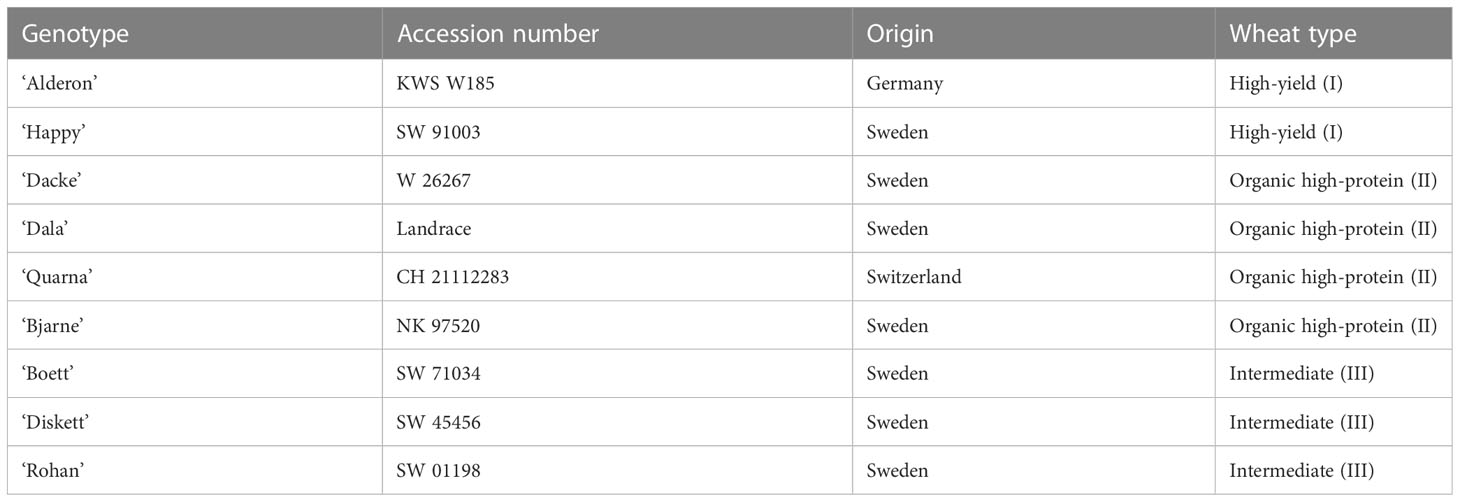
Table 1 List of the nine-spring wheat varieties used in this study, their origin, and the classification to their breeding target.
The definition of wheat quality is a dynamic and complex concept to describe, mostly because it changes depending on the intended end product. Common quality traits of wheat are often based on morphological properties, such as grain size, hectoliter weight, and the absence of grain damage. Wheat intended for human consumption target organoleptic properties, gluten quality and quantity, and grain hardness; with food manufactures including targets such as grain uniformity. In the case of millers, wheat quality is mainly focused on grain morphology traits, grain density and grain hardness (Guzman et al., 2016). The quantity of starch and protein stored in the wheat grain are here considered as quality traits, although they are closely linked to grain yield (Xurun et al., 2015). Moreover, consumers often link quality to end-use traits such as baking properties or nutritional values. Both traits are essential breeding targets in wheat (Koppel and Ingver, 2008; Mecha et al., 2017). However, maximizing quality and yield traits in the same plant is challenging because of a genetic and inverse correlation between them (Fowler, 2003; Amiri et al., 2018). Grain yield establishment is a complex process in which breeders are interested to explore the primary and secondary connections between related traits. Additionally, a high genetic variability should be present in the material for achieve an improvement (Iqbal et al., 2022). Other important grain quality traits are related to the possibility of grain biofortification, for which micronutrients such as zinc (Zn) and iron (Fe) are of major concern (Cakmak, 2008; Gupta et al., 2021). Breeding for biofortification has become increasingly popular and could aid in providing essential nutrients to vulnerable populations that depend on staple crops as their main dietary intake, ensuring food and nutritional security (Guzman et al., 2016; Velu et al., 2017). It has been shown that high-yielding wheat cultivars usually produce grains with low concentrations of Zn and Fe, due to a “dilution effect” (Ortiz-Monasterio et al., 2007; Gupta et al., 2021), which is another example for links between traits with a high relevance for breeding. Due to these links between traits, breeders face a difficult task to deliver varieties that satisfy the demands of different stakeholders and end-users (Guzman et al., 2016). Thus, many grain yield and quality traits might be correlated, either through physiological or genetic links between traits (Weih, 2003); and targeting one of the traits could imply a high likelihood to also modify other trait(s) in the same breeding process. However, little is known about trait correlations among yield and different grain quality traits, and this poor knowledge reduces the possibility to target suites of traits rather than single traits in the breeding.
Previous studies have pointed out that quality traits such as grain protein content in wheat is strongly influenced by the genotype (Simons et al., 2012; Makawi et al., 2013; Taheri et al., 2021), but also by environmental factors (Amiri et al., 2018). The grain starch content is usually correlated to grain yield, and seems to be mainly influenced by the environmental conditions (Labuschagne et al., 2007). Taken together, the previous results suggest that grain quality traits can be expected to be mainly affected by the genotype, while grain yield should be more affected by environmental factors.
Frequent genotype by environment interaction (G x E) is observed for both grain yield and quality traits (Nagarajan et al., 2007), although a desirable prerequisite for viable wheat production is the availability of genotypes with high and stable performance among diverse environmental conditions (Nagarajan et al., 2007; Tayyar, 2010). Trait stability can be either static or dynamic. Static stability is when performance remains similar in different environments (low G x E), while dynamic stability is when performance follows the potential of an environment (high G x E) (Knapp et al., 2017). Static stability of quality traits, reflected by low G x E, favors stable end products and thus ensures that a quality standard can be performed by a given genotype, allowing efficient processing and decreased waste (Mut et al., 2010; Knapp et al., 2017). However, little is known about (static) stability of grain yield and quality traits in wheat varieties that where bred for different breeding targets.
Many grain yield and quality traits are connected to crop nutrition issues, because nutrient elements are important for the generation of crop growth and yield on the one hand, and grain quality traits (e.g., protein content and micronutrient biofortification) on the other hand. Crop nutrition is, in turn, linked to crop requirements for nutrient resources. Higher crop nutrient requirements imply a greater depletion of nutrient resources and thus potentially a negative impact on the ecological sustainability of the crop (Weih et al., 2014). Because of these links, it is possible that wheat varieties bred for high protein contents (i.e., high grain N concentrations) have greater N requirement and a stronger impact on ecological sustainability than varieties bred for high grain yields. In this study, we use the N accumulation efficiency concept (Weih et al., 2011; Weih et al., 2018), in which the N uptake efficiency (U) reflects the mean N amount during the entire growth period (N’) divided by the N amount in initial seed; where all variations regarding U were caused by N’ changes in this study. In the same conceptual approach (Weih et al., 2011; Weih et al., 2018), grain-specific N efficiency (EN) quantifies the efficiency of utilizing N to produce grain. As proposed in a previous paper (Pourazari et al., 2018), this concept is here supplemented by the efficiency of utilizing N to produce specific end products; i.e., the protein-specific N efficiency (EN,yp) and starch-specific N efficiency (EN,ys), quantifying the efficiencies of utilizing N to generate protein and starch yield, respectively.
This paper complements a series of previous papers in which data from the same field trial have been used. Thus, the effects of soil compaction on growth and grain yield as modulated by the weather conditions were investigated by (Liu et al., 2022a); whilst other works addressed the N economy and co-limitation by nutrients other than N especially in relation to root traits (Weih et al., 2021; Liu et al., 2022b). In contrast to our previous papers using the same field trial, the main objective of this study is to evaluate the relationships between target and non-target traits related to grain yield, various grain quality traits, biofortification potential, and N accumulation aspects (indicating N requirement and use) for three groups of spring wheat varieties. The three wheat groups where classified based on their breeding targets: high-yield (type I), organic high-protein (type II), and intermediate (in terms of both grain yield and protein content) (type III) wheats. The nine varieties were grown in a field trial with different soil compaction treatments over two years with contrasting weather. They were assessed for grain yield and quality traits, including the grain macro and micronutrient concentrations, and N accumulation aspects. We explored the following hypotheses: (H1) The relationship between target and not-target traits is specific for a given wheat type and stable across different environments. (H2) Grain quality traits are expected to be mainly affected by the genotype and more stable across contrasting environmental conditions, while grain yield should be more affected by environmental factors. (H3) Wheat varieties bred for high grain protein concentration (type II) have greater N demand and thus greater N uptake efficiency than varieties bred for high grain (starch) yields (type I).
2 Materials and methods
2.1 Study location
Field studies were conducted in Uppsala, Central Sweden (59° 45’ N, 17° 42’ E) during the 2018 and 2019 growing seasons. Uppsala has a boreal-temperate climate and the growing season lasts from April to October. During the summer months of 2018 the weather was drier and warmer, compared to the cooler and wetter weather of 2019 (Weih et al., 2021). Because of the lack of precipitation in 2018, artificial irrigation of ca. 10 mm of water was required 28 and 34 days after sowing in order to ensure survival of the plants during an extreme drought period. The soil has a silt loam texture (16% clay, 70% silt, 14% sand) and an organic matter content of 4% in the top 0.3 m. A commercial nutrient fertilizer with 140 kg ha-1 N, 24 kg ha-1 P, and 46 kg ha-1 K was added to the soil after sowing in both years. The pH was 5.8, and the particle density was 2.61 Mg m-3.
2.2 Experimental design
The field trial was established in spring 2018 in a randomized split plot design with four replications; the size of each plot was 12 x 2 m (soil treatment, compaction vs. non-compaction). The soil compaction treatment started in April 2018 by double track-by-track passing using a front loader with four wheels and an average wheel load of 42 kN. To ensure crop establishment, the surface of the compacted soil was loosened to a depth of approximately 50 mm with a surface cultivator before sowing. During the field trial in 2019, the same soil compaction areas were used as in 2018 using the same protocol, while the positions of the genotype plots were re-randomized. Further details of the experimental treatments and soil conditions during the two experimental years are presented by Liu et al. (2022a). Nine spring-wheat varieties were selected and grown for the two years: ‘KWS Alderon’, ‘Bjarne’, ‘Boett’, ‘Dacke’, ‘Diskett’, ‘Happy’, ‘Quarna’, ‘Rohan’, and the landrace ‘Dala’. The varieties were classified at three different sets based on their breeding targets. The groups were high–yield type (type I): ‘Alderon’ and ‘Happy’, organic high–protein type (type II): ‘Quarna’, ‘Dacke’, ‘Dala’, and ‘Bjarne’, and intermediate type (type III): ‘Diskett’, ‘Rohan’, and ‘Boett’ (Table 1).
2.3 Plant sampling and assessments
Five plants were selected in homogeneous areas of each plot at the crop developmental stages tillering (BBCH29), flowering (BBCH65), and spike maturity (BBCH89) (Lancashire et al., 1991) in both 2018 and 2019. The designated plants were cut with scissors at approximately 15 mm above ground, oven-dried at 65°C for 48 h and weighed. The central plot area (6 m * 2 m) was harvested with a combine harvester to assess grain yields, and a sub-sample was taken to assess grain protein, gluten, starch, TKW, HLW and falling number. The spikes of the selected plants were then manually threshed using a Smooth Chopper 6948A-2 (Tupperware, USA), and the dried grains were weighed. These samples (straw and grains separated at maturity) were ground in a stainless steel grinder to pass a 1 – mm mesh before nutrient element analysis. Eleven grain parameters were measured: grain yield and thousand-kernel weight (TKW) (these two traits are here considered as “yield traits”), protein content, gluten, starch, hectoliter weight (HLW), falling number; and the grain concentrations of N, P, Zn and Fe (Table 2), related to biofortification potential. In addition, the N accumulation traits U, EN, EN,yp, and EN,ys were also assessed as indicators of the characteristics affecting environmental performance and sustainability (Table 2). The N concentrations of planted seeds, shots and grains of five representative plants were sampled in the 0.5 m x 0.5 m plots and were analyzed on a LECO CNS72000 analyzer using standard method (SS-ISO13878) in order to determine the N use efficiency and related traits. The contents of P, Fe, Zn were extracted using 32.5% Nitric acid on a heat block and concentrations were determined using ICP-AES technique (Spectro Blue FMS 26, Spectro Analytical Instruments, Kleve, Germany) by applying internal standardization protocols (Protocol number SS028311).
Protein, starch, hectoliter weight and gluten content were determined using FOSS Infratec NOVA grain analyser (Foss Analytical A/S. Infratec™nova), thousand kernel weight was determined on an Opto-Agri Machine (Opto Machines,TSW & Seed Biometry), and falling number was determined on a FOSS Alphatec FN° (Method AACC 56-81B).
2.4 Calculations of nitrogen accumulation efficiency and related traits
The calculations were based on the N pool of five sampled plants and the method presented by Weih et al. (2018). The N uptake efficiency (U; Eq. 1), the mean N amount during the entire growth period (N’) and the grain-specific N efficiency (EN; Eq. 2) were calculated separately for each replicate. For the calculations of protein-specific N efficiency (EN,yp; Eq. 3) and starch-specific N efficiency (EN,ys; Eq. 4) the EN equation was adapted.
2.5 Statistical analysis
All the statistical analyses were performed using SPSS version 26. Analyses of Variance (ANOVA) were used for the calculation of the probabilities of significant differences in each of the fifteen plant traits and element concentrations, using fixed effects of year, treatment, wheat type, genotype nested within wheat type and block. This was also used to assess the interactions between year, treatment, and wheat type, as well as their effect sizes (partial eta squared; ). Correlations between the eleven traits were analyzed using Spearman’s rank coefficient. Linear regression analysis was performed to evaluate the relationships between the concentrations of various macro- and microelements. In addition, a Principal Component Analysis (PCA) following CATPCA procedure was performed in order to relate and group traits and wheat types for each year using SPSS version 26. Coefficient of variation (CV) was used to test the environmental static stability of the traits for the three wheat types.
3 Results
3.1 Effects of wheat type, variety and environment on grain yield and quality traits
Significant differences between years, wheat types, genotype nested within wheat type, and the year*wheat type interactions were observed for the grain yield, starch, and TKW (Table 3). In this study, environmental variation is represented by the experimental compaction treatments and the two years with contrasting weather. No significant effect of compaction treatment on grain yield was found, whilst year significantly affected grain yield (P<0.001). The effect sizes of year were large for TKW, starch, and yield . Additionally, the effect sizes of wheat type for the same traits were large as well . The year*wheat type interaction displayed large effect sizes for starch and TKW ( = 0.418 and 0.205, respectively) and medium effects for yield ( = 0.105).
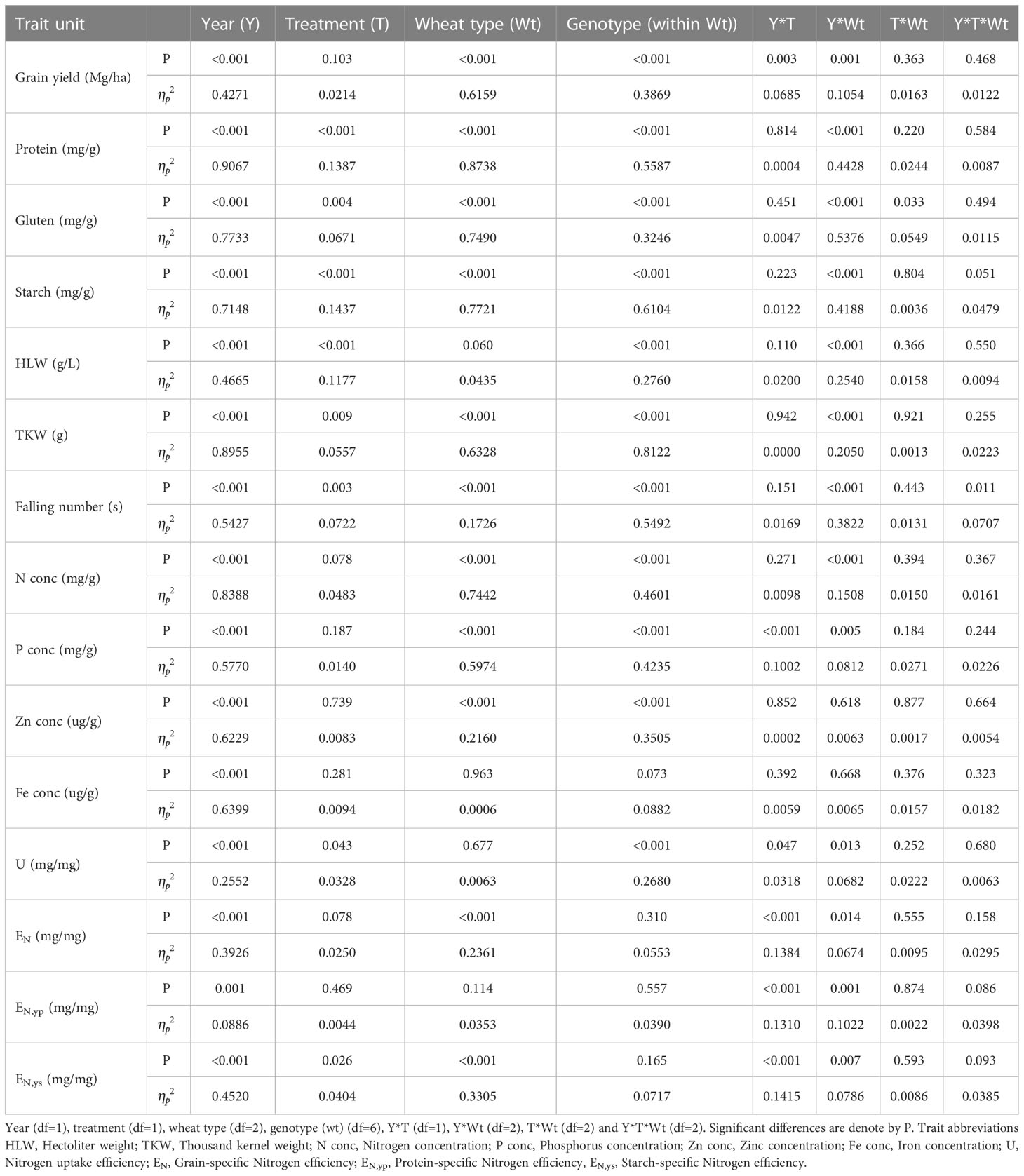
Table 3 Analysis of variance (ANOVA) p-value (P) result for grain yield and quality traits and their equivalent effect sizes ( ) for fixed effects.
Protein, gluten, HLW, and falling number were significantly affected by year (P<0.001), treatment (P<0.001, P=0.004, P<0.001, and P=0.003, respectively), wheat type (except HLW) (P<0.001), and the interaction between year and wheat type (P<0.001) (Table 3). Among these traits, the effect sizes of year and wheat type were largest for protein ( = 0.907 and 0.874, respectively). Similarly, large effect sizes of year and wheat type were found for gluten ( = 0.773 and 0.749, respectively). Falling number and HLW showed large effect sizes for year ( = 0.543 and 0.466, respectively) and large and small effect sizes for wheat type ( = 0.173 and 0.043, respectively).
Grain concentrations of N, P, Zn, and Fe varied significantly between years, with large effect sizes . For wheat type, the data showed large effect sizes in grain N, P, and Zn (Table 3).
Significant differences between years were reported for U, EN, EN,yp and EN,ys (P<0.001, P<0.001, P=0.001, P<0.001, respectively), wheat type (except U and EN,yp), and year*wheat type interaction (P=0.013, P=0.014, P=0.001 and P=0.007, respectively). Medium effect sizes of year *wheat interaction were shown by these traits . Whereas, large effect sizes of year and wheat type were registered for EN ( = 0.393 and 0.236, respectively) and ENy,s ( = 0.452 and 0.331, respectively).
3.2 Trait correlations and the influence of genotype and environment on trait stability
Due to the year effect, that had a strong influenced on all traits the correlation analysis was performed separately for each year. In this way, the identification of links between traits across varieties and the wheat types was more effective (Table 4). Most traits showed consistent correlation patterns between years, with the notable exception of the correlations involving falling number and HLW, for which trait correlations were not consistent and changed from negative to positive from one year to the other (Table 4).
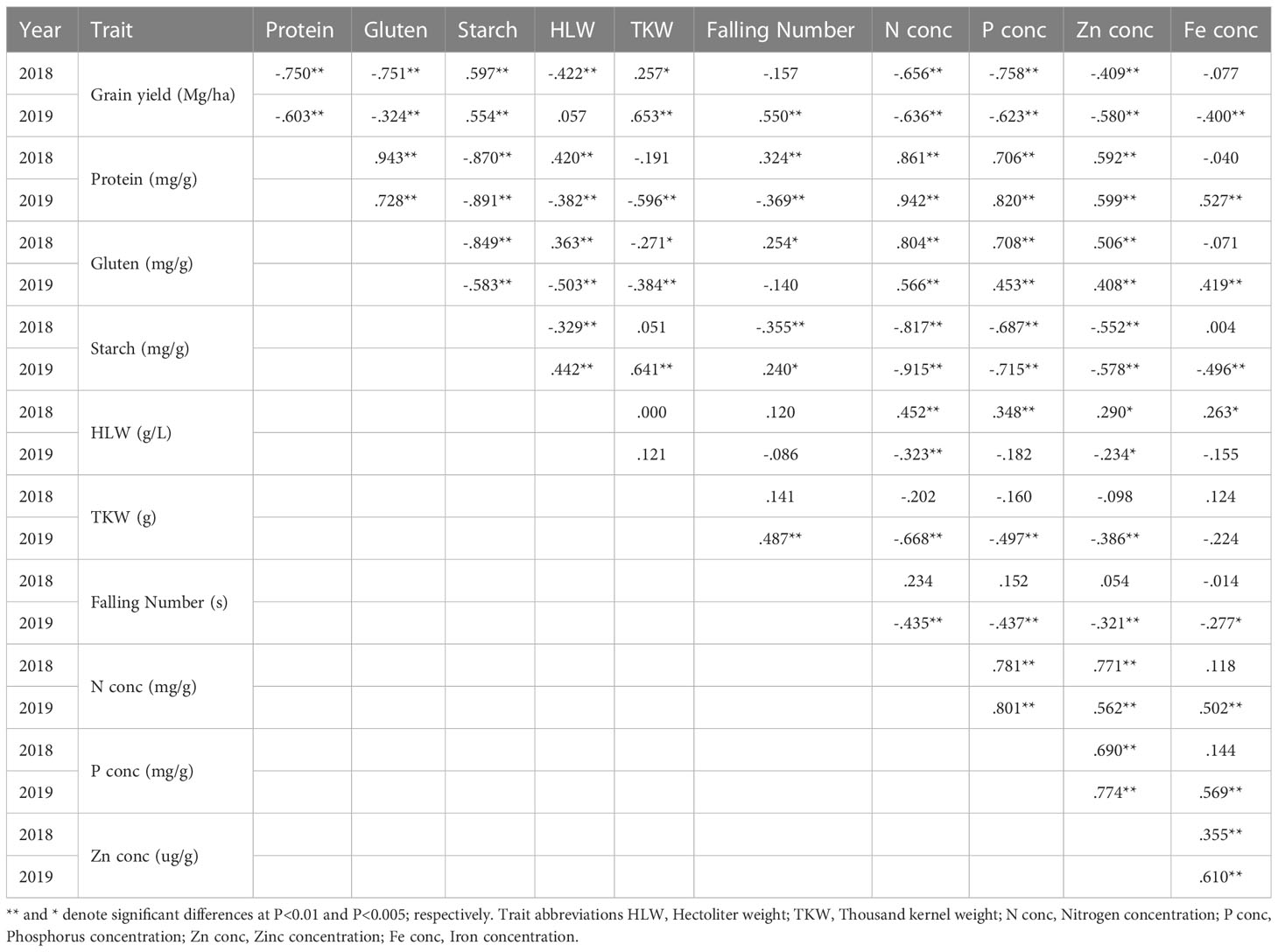
Table 4 Spearman’s rank correlation coefficients between grain yield and quality parameters per year.
To visualize correlation patterns and specifically evaluate the associations between wheat type and traits for each year, a Principal component analysis (PCA) was performed. The total Eigenvalue was 5.7 for dimension 1 (explanatory power 57%) and 1.5 for dimension 2 (explanatory power 15%) for 2018. For 2019 the total Eigenvalue was 6.2 for dimension1 (explanatory power 62%) and 1.5 for dimension 2 (explanatory power 15%). In 2018 grain yield and starch were part of the same cluster, and the wheat types related to both traits were High yield (I) and intermediate (III) type (Figure 1A). In a different cluster, protein, gluten, HLW, Zn, N, P, and falling number were associated with the high protein (II) type. In 2019, protein, gluten, Zn, N, and P remained together as part of the same cluster, and the wheat type most related to these traits was the high protein (II) type, while grain yield, starch, TKW, falling number, and HLW were related to the high yield (I) type (Figure 1B).
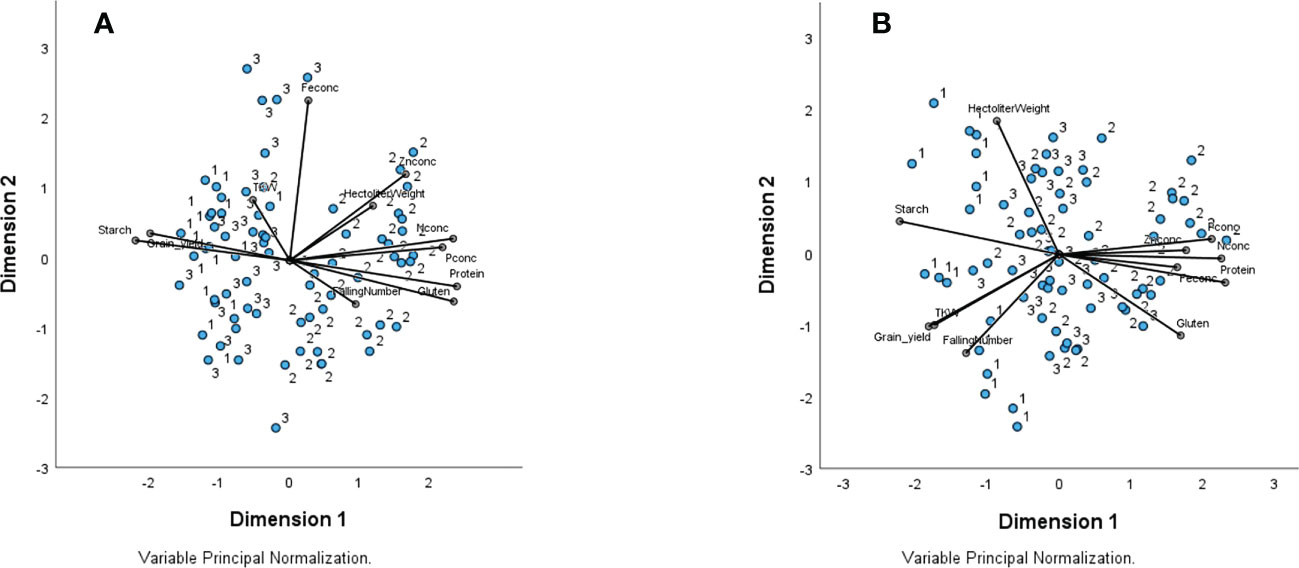
Figure 1 Biplot containing grouping of variables according to Principal component analysis (PCA) for 2018 (A) and 2019 (B) datasets. The variables are the trait values measured on three different wheat type groups: 1 - High yield, 2 - Organic high protein, and 3 – Intermediate for nine wheat varieties field-trial in Sweden. In 2018 the total Eigenvalue was 5.7 for dimension 1 (explanatory power 57%) and 1.5 for dimension 2 (explanatory power 15%). For 2019 the total Eigenvalue was 6.2 for dimension 1 (explanatory power 62%) and 1.5 for dimension 2 (explanatory power 15%). Traits Grain yield, protein, starch, gluten, hectoliter weight, TKW thousand-kernel weight, falling number, Nconc Nitrogen concentration, Pconc Phosphorus concentration, Znconc Zinc concentration, and Feconc Iron concentration.
High yielders (type I) were associated to high grain yield, starch content, and TKW in both years, while high protein types (II) were related to high concentrations of protein, gluten, and nutrients. The Intermediate wheat types (III) were more related to High yield type (I) during both years. Similar to the results from the correlation analysis, HLW and falling number also showed opposite patterns between the two years in the PCA where HLW and falling number were associated to different wheat types in 2018 and 2019 (Figure 1).
Coefficient of variation (CV), based on five individual plants for each variety, indicated that most of the traits were stable for the three wheat groups for both years (Figure 2). Hectoliter weight (CV 2.1% for type (I), 0.91% for type (II), and 1.3% for type (III)) and starch (CV 2.1%, 1.9%, and 3.7%, respectively) were the most stable traits across the three wheat types, while Fe was the least stable trait with a CV above 60% (CV 69%, 64%, and 78%, respectively) follow by Zn (CV 23%, 19% and 24%, respectively). Macro nutrients N and P showed similar values for type (I) 11%, 12%, for type (II) 9%, 11%, and for type (III) 13%, 15% respectively. Results of high yielders (I), high protein type (II), and intermediate ones (type III) were similar, except for gluten and falling number in high protein wheat (type II). For these two traits, the percentage value in the high-protein wheat was doubled in comparison to the other two wheat types (Figure 2).
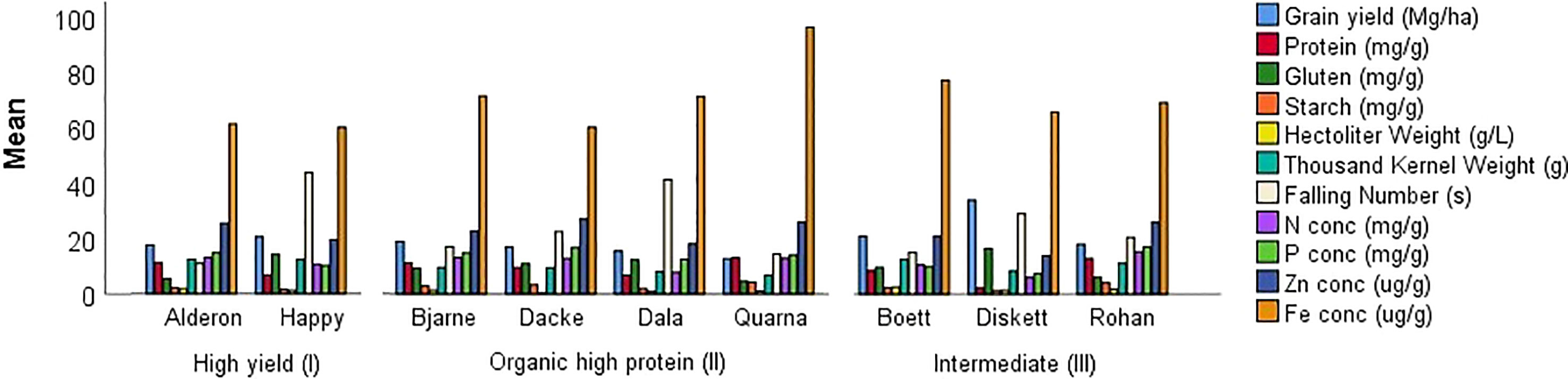
Figure 2 Coefficient of variation analysis to determine the static stability of quality traits of nine-spring wheat varieties, grouped in three wheat types due to their main breeding target, grown over two years (2018 and 2019) in a field experiment in Uppsala, Sweden. Genotypes Alderon and Happy are categorized as High yield wheat type (I); Bjarne, Dacke, Dala, and Quarna are categorized as Organic high protein wheat type (II), and Boett, Diskett, and Rohan are categorized as Intermediate wheat type (III).
3.3 Biofortification potential
Significant positive correlations between grain N and Zn were found for the high yielders (I) and high protein types (II) (Figures 3A, B), while the Intermediate type (III) showed a positive correlation between N and Zn only in 2018. The high yield wheat varieties showed a poor correlation between Zn and Fe in 2018 (R2 = 0.004), but a higher correlation in 2019 (R2 = 0.385) (Figures 3C, D). The correlation between Zn and P during 2018 and 2019 (Figures 3E, F) was positive across all wheat types; however, the strongest correlation was observed during 2019 in the high protein wheats (II). A positive correlation was found between N and Fe in the high protein (II) and intermediate (III) wheat type during 2018, while in 2019 the positive correlation was found in the high yield (I) and high protein (II) wheat groups (Figures 3G, H). Finally, a low correlation between P and Fe in 2018 was registered among all wheat types. During 2019, the correlation between P and Fe was positive, however, the highest correlation was noticed in high protein wheat type (R2 = 0.304) (Figures 3I, J).
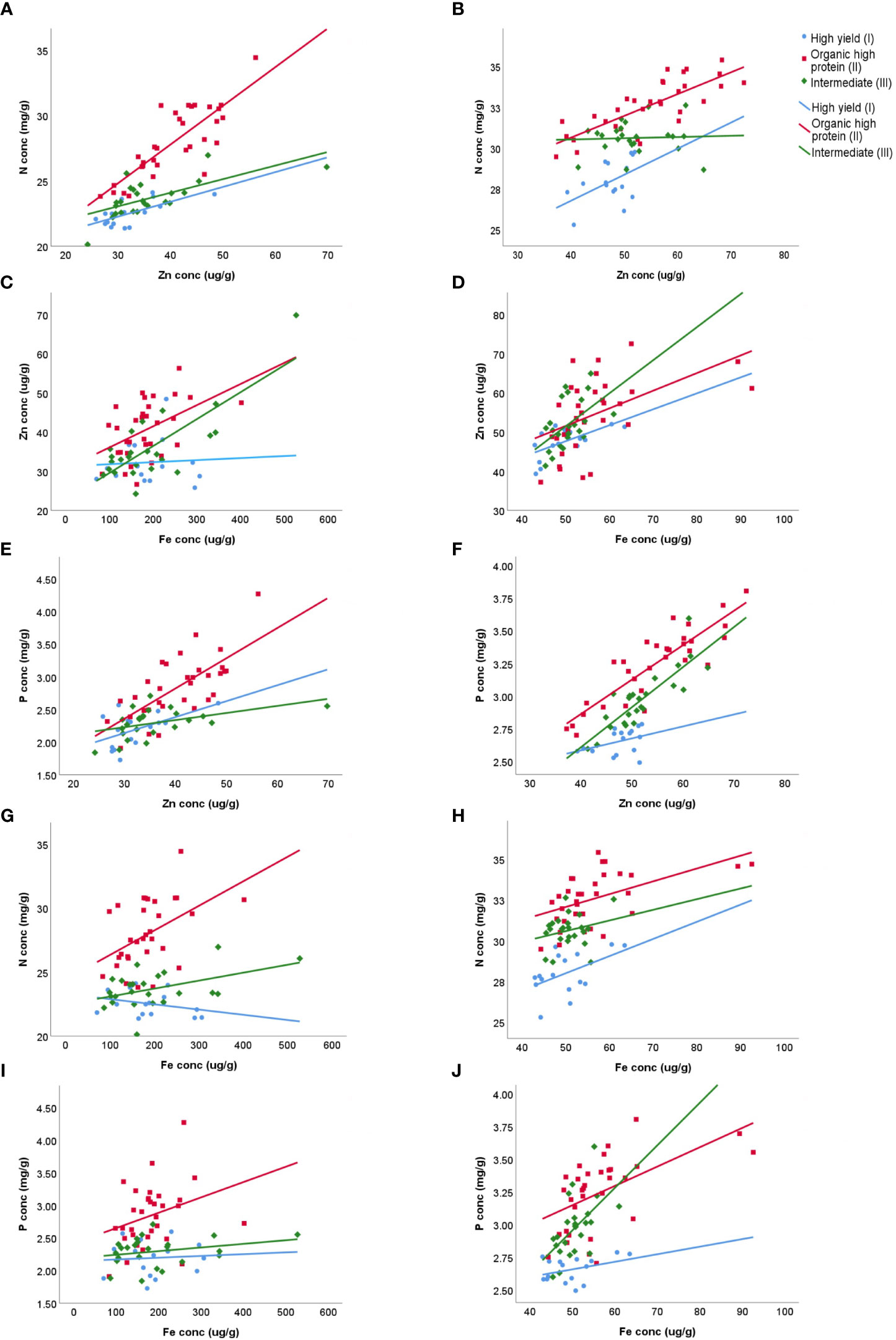
Figure 3 Relationships between grain concentrations of various macro- and micro-elements relevant for biofortification potential, plotted for 2018 and 2019 data sets and grouped into three wheat types (of nine spring wheats) grown in Uppsala, Sweden. Shown are the relationships between N and Zn concentrations: (A) 2018 and (B) 2019, Zn and Fe concentrations (C) 2018 and (D) 2019, Zn and P concentrations (E) 2018 and (F) 2019, N and Fe concentrations: (G) 2018 and (H) 2019, and P and Fe concentrations: (I) 2018 and (J) 2019Linear regressions: (A) High yield R2 = 0.467, Organic high protein R2 = 0.663, Intermediate R2 = 0.451; (B) High yield R2 = 0.238, Organic high protein R2 = 0.614, Intermediate R2 = 0.002; (C) High yield R2 = 0.004, Organic high protein R2 = 0.220, Intermediate R2 = 0.619; (D) High yield R2 = 0.385, Organic high protein R2 = 0.252, Intermediate R2 = 0.269; (E) High yield R2 = 0.276, Organic high protein R2 = 0.495, Intermediate R2 = 0.205; (F) High yield R2 = 0.148, Organic high protein R2 = 0.771, Intermediate R2 = 0.715; (G) High yield R2 = 0.089, Organic high protein R2 = 0.209, Intermediate R2 = 0.211; (H) High yield R2 = 0.241, Organic high protein R2 = 0.267, Intermediate R2 = 0.060; (I) High yield R2 = 0.005, Organic high protein R2 = 0.097, Intermediate R2 = 0.071; (J) High yield R2 = 0.138, Organic high protein R2 = 0.304, Intermediate R2 = 0.306 Traits N conc Nitrogen concentration, Zn conc Zinc concentration, Fe conc Iron concentration and P conc Phosphorus concentration. Wheat varieties High yield wheat type (I) (blue), Organic high protein wheat type (II) (red) and Intermediate wheat type (III) (green).
Greater mean values for N, P and Zn concentrations in the grain were recorded for the high protein wheat type (II) for both years combined (Figure 4).
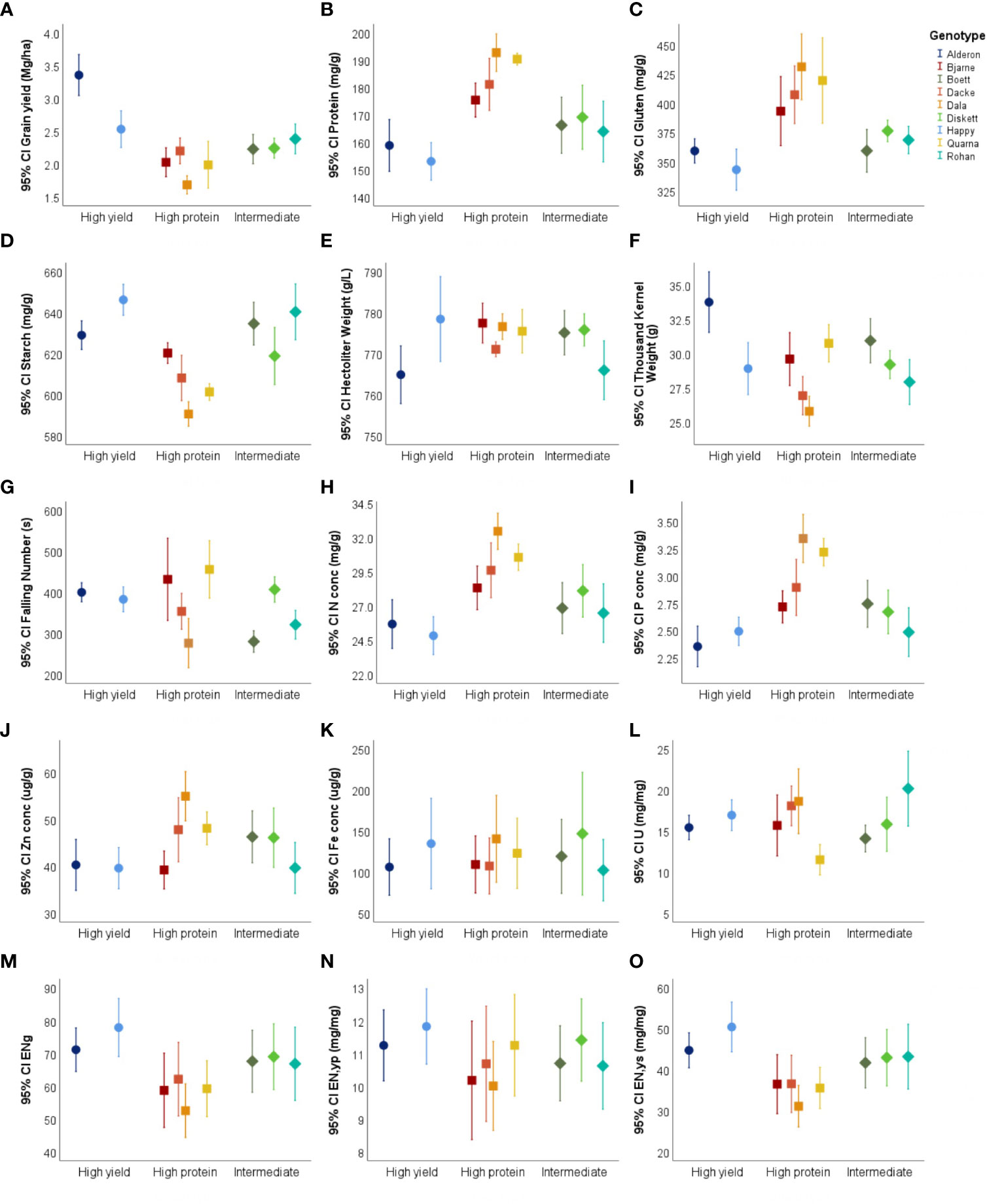
Figure 4 Mean values and confidence interval at 95% of fifteen traits (A) Grain yield, (B) Protein, (C) Gluten, (D) Starch, (E) Hectoliter Weight, (F) Thousand Kernel Weight, (G) Falling Number, (H) N conc, (I) P conc, (J) Zn conc, (K) Fe conc, (L) U, (M) EN, (N) EN,yp, and (O) EN,ys of nine spring heat varieties divided by their breeding target in Uppsala, Sweden for combined years (2018 & 2019). Wheat groups High yield wheat type (I), Organic high protein wheat type (II), and Intermediate wheat type (III). Trait abbreviations N conc Nitrogen concentration, P conc Phosphorus concentration, Zn conc Zinc concentration, Fe conc Iron concentration, U Nitrogen uptake efficiency, EN Grain-specific Nitrogen efficiency, EN,yp Protein-specific Nitrogen efficiency, EN,ys Starch-specific Nitrogen efficiency.
Due to the positive correlations between N and Zn in the high protein group, the higher mean values and the high environmental effect in N, Zn and Fe; an ANOVA analysis was performed for each variety of the high protein wheat type (II) regarding environmental factors. The four varieties: Bjarne, Dacke, Dala and Quarna, did not present significant differences for N regarding environmental effects, as year (P=0.139, P=0.153, P=0.198 and P=0.302 respectively), treatments (P=0.981, P=0.810, P=0.852 and P=0.664 respectively) and the interaction of Y*T (P=0.985, P=0.847, P=0.894 and P=0.972 respectively). Additionally, all four showed significant differences regarding year for Fe (P=0.002, P=0.012, P=0.007 and P=0.007 respectively). Finally, varieties Dala and Quarna did not present significant differences for year (P=0.199), treatment (P=0.966 and P=0.367) and Y*T interaction (P=0.724 and P=0.365) regarding Zn (Table 5).
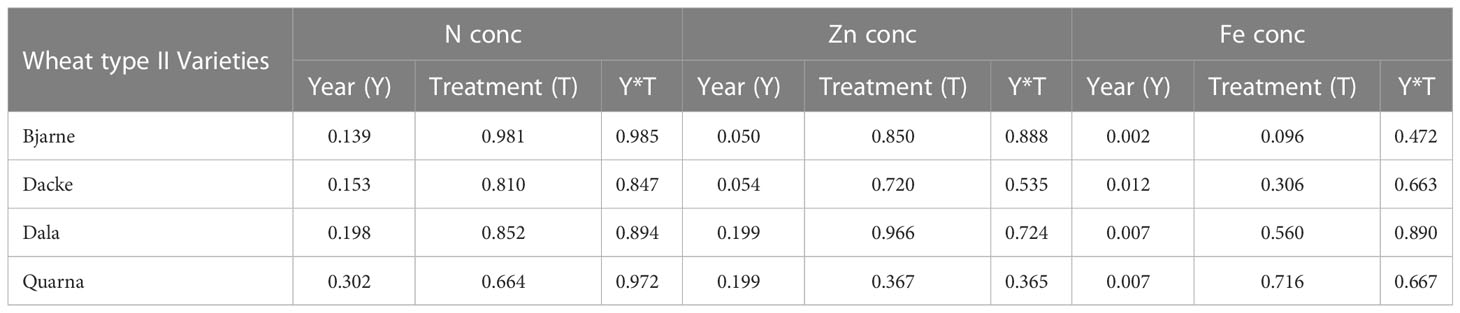
Table 5 Analysis of variance (ANOVA) p-value for N, Zn and Fe for fixed effects related to environmental variation of the four varieties in high protein wheat type (II).
3.4 Nitrogen accumulation efficiency and its components
For this study, the values of U and N’ during the growing season were similar, and therefore, in this study, the variation in U was caused mostly by N’ and not by the initial amount of N in the seed. Among all the N accumulation and N efficiency traits assessed, only EN and EN,ys were significantly different between wheat types (Table 3). Year and the year*wheat type interaction significantly affected the N uptake efficiency (U), EN, EN,yp, and EN,ys. Thus, under the drier conditions in 2018, the high yield wheat type (I) accumulated more N, shown by higher U than the other wheat types, while in 2019, the intermediate (III) and high protein (II) types showed the highest U values (Table 6). For EN and EN,ys, high-yield varieties achieved the highest values in 2019 and intermediate varieties had the highest values in 2018. During 2018, no significant differences were found between the three wheat types regarding EN,yp. However, in 2019, the high yield type (I) showed a significantly higher EN,yp (P=0.001) (Tables 3, 6).
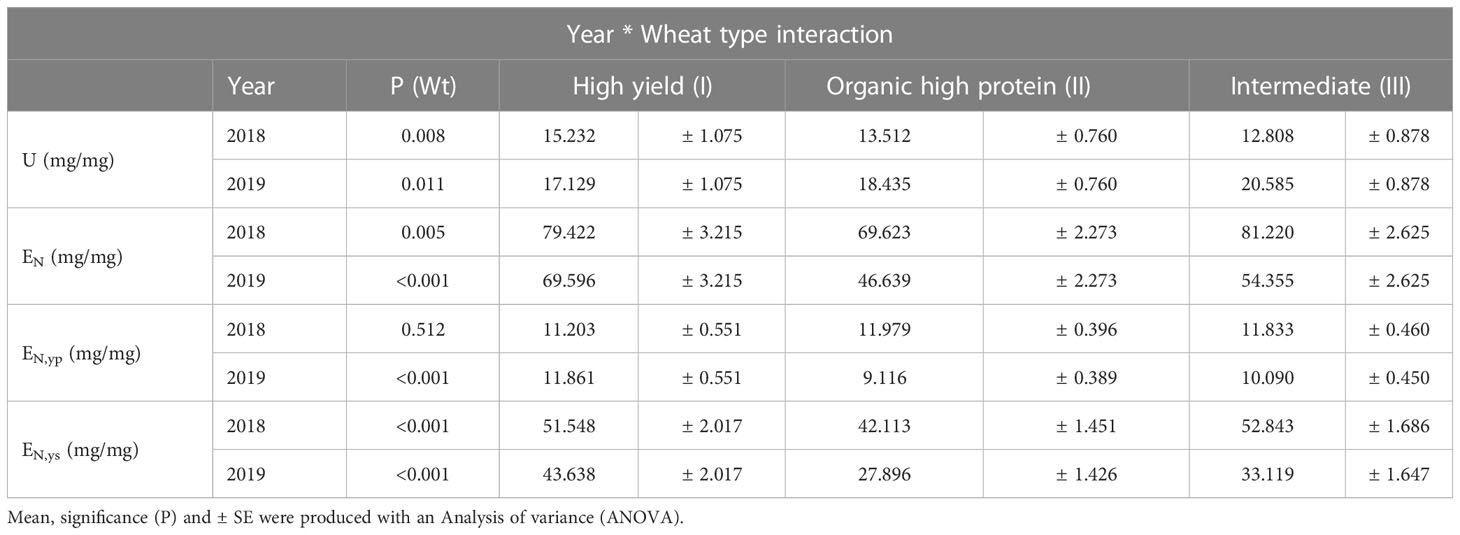
Table 6 Interaction between Year (2018 & 2019) and Wheat type (Wt; High yield (I), Organic high protein (II), and Intermediate (III)) of nitrogen (N) accumulation efficiency components: U N use efficiency, EN Grain-specific N efficiency, EN,yp Protein-specific N efficiency and EN,ys Starch-specific N efficiency.
The effect sizes for year regarding U, EN, EN,yp, and EN,ys were between medium and large . For the year*wheat interaction, the effect sizes were medium for the four traits (Table 3).
Mean values of U, EN, EN,ys, and EN,yp were plotted separately for 2018, 2019, and both years combined (Figures 4L–O and 5) (Supplementary Table S1). The N uptake efficiency (U) varied significantly between the wheat types in both years, and was either lower (2018) or similar (2019) in the high-protein varieties compared to the high-yield varieties (Table 6). The grain-specific and yield-specific N efficiencies (EN and EN,ys, respectively) were always much higher in the high-yielding varieties than the high-protein varieties. In contrast, the protein-specific N efficiency (EN,yp) was similar between the wheat types in the dry year (2018), but lower in the high-protein varieties than the high-yielding varieties in 2019.
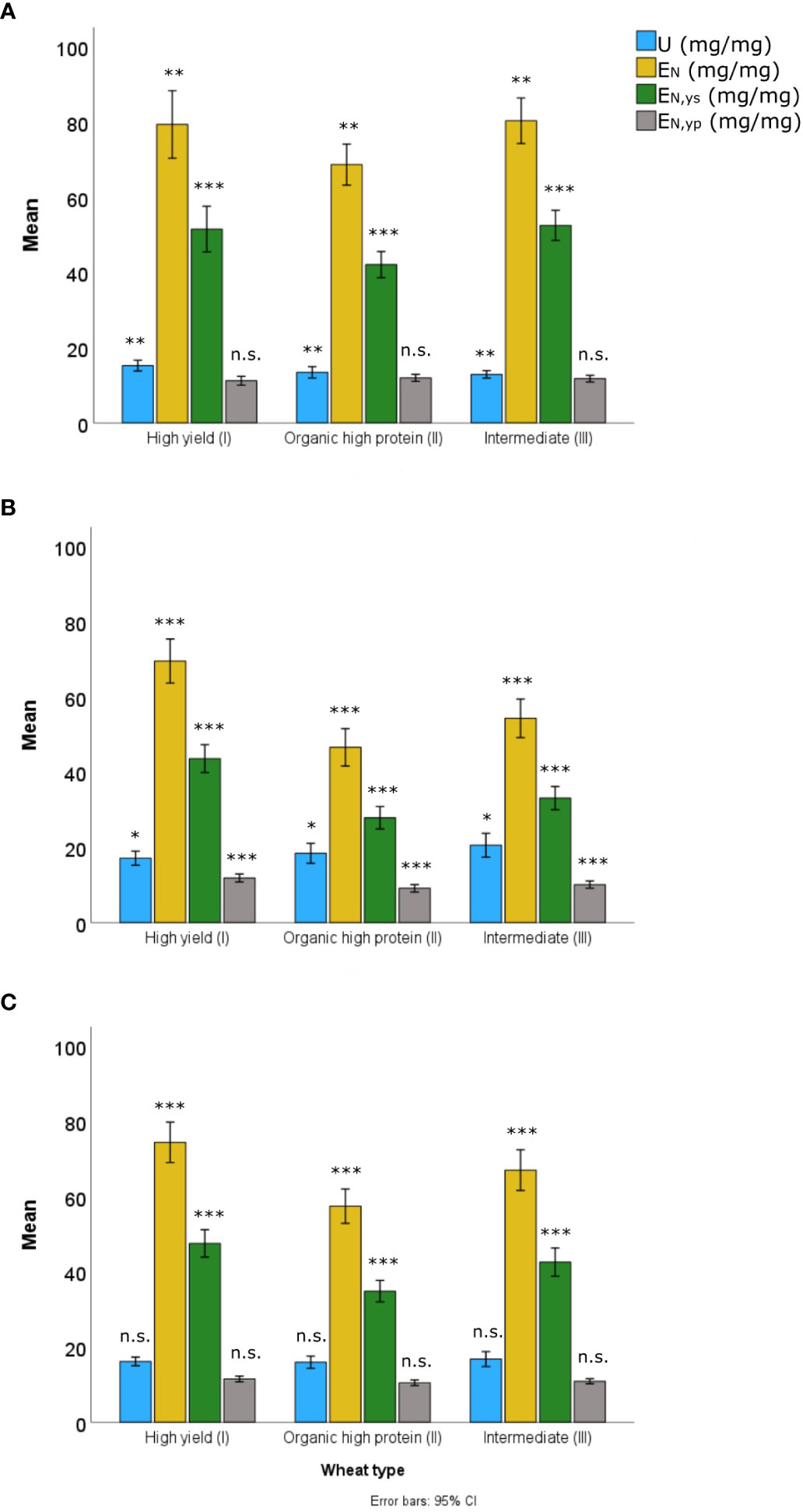
Figure 5 Comparison between U Nitrogen uptake efficiency, EN Grain-specific Nitrogen efficiency, EN,yp Protein-specific Nitrogen efficiency, and EN,ys Starch-specific Nitrogen efficiency for three wheat types corresponding to nine spring wheat varieties grown in central Sweden in 2018 (A), 2019 (B) and the combined years (C). Bars represent the mean values of each parameter. Wheat varieties High yield wheat type (I), Organic high protein wheat type (II), and Intermediate wheat type (III). ***, ** and * denote significant differences at P<0.001, P<0.01 and P<0.05, respectively, n.s. denotes non-significant effects.
4 Discussion
Based on the analysis of very different spring wheat materials over two years under contrasting weather conditions, this paper evaluated the relationships between grain yield and quality traits, as well as crop nutrient accumulation aspects relevant for the evaluation of environmental performance and ecological sustainability of the crop. Additionally, it incorporated a large number of grain quality traits including four elements relevant to the potential for biofortification. Some of the results are not surprising and have been known for long time, such as the negative relationship between grain yield and grain N or protein concentration (Bogard et al., 2010). Our study goes beyond the established knowledge and investigated the relationships between the two target traits grain yield and grain protein concentration on the one hand, and various non-target traits including grain quality traits, grain nutrient (cf. biofortification) and crop nitrogen use efficiency traits (cf. environmental performance of the crop) on the other hand. Our results have implications for crop breeding, especially in wheat. The limitations of this study are the small number of wheat varieties we analysed and the lack of additional locations, the latter of which could have contributed to a better analysis of the G x E interactions.
Due to the large differences between sowing years, all correlation, regression and PCA analyses were applied separately for the two years. Not surprisingly, this study illustrated that grain yield and quality traits are partly strongly affected by the underlying breeding targets, represented here as wheat types, but also by the contrasting environmental conditions in the two study years. Supporting our first hypothesis (H1), the results indicate that most of the grain quality traits were positively associated with the high protein wheat type (II), and the grain yield was positively correlated with the high yield wheat type (I); confirming the differential breeding targets (Figure 1). The effect size analysis revealed only small differences in stability between grain quality and yield traits. Contrary to our second hypothesis (H2), both trait groups were affected by genetic differences and environmental conditions (Table 3), which partly contradicts the literature (Makawi et al., 2013; Labuschagne et al., 2016). We also found no evidence for high-protein varieties (type II) to have greater N uptake efficiency than varieties bred for high grain (starch) yields (type I), not supporting our third hypothesis (H3).
4.1 Associations among target and non-target traits favor breeding selection
The relationship between target and non-target traits was specific for the three wheat types investigated here, and stable for both years (H1). Whilst starch and TKW were in the same cluster with grain yield, and associated to the high-yield wheat type during both years, macro- and microelements, gluten, and protein content were associated to the high-protein varieties (Figures 1A, B). Our findings suggest that some non-target and target traits may have been inherited together during the breeding process, although the large quantity of significant genotype (within wheat type) effects in our study indicates that most of the traits also have a strong individual genotype-specific component which is discussed elsewhere (Weih et al., 2021; Liu et al., 2022b). Similar to other studies (Koppel and Ingver, 2008; Mut et al., 2010), our study showed that HLW and falling number were strongly affected by the environmental conditions, and the low coefficient of variation (CV) for these two traits (Figure 2) is probably a result of the high values of these traits (high means result in low CV) and can here not be interpreted as indicators of static stability. In 2018, both traits also showed a positive correlation with protein and gluten instead of the positive correlation with yield and starch that was expected and seen in 2019 (Figures 1A, B) (Table 4).
4.2 Positive correlation between Zn and P could jeopardize wheat biofortification
A positive correlation between grain N content (consider as a target trait for the high-protein varieties) and Zn and Fe grain content (non-target traits) was found in our results. This result partially supports our first hypothesis (H1), because it implies that target and non-target traits can be positively correlated due to pleiotropic effects or genetic linkage, facilitating the breeding process (Tabbita et al., 2017). Therefore, breeding for high grain N (e.g. high protein wheat) results most likely in high grain concentrations of Zn and Fe, and a high potential for biofortification with these nutrients (Cakmak, 2008). However, we also found a positive correlation between Zn, Fe and N in cultivars bred for higher yields as was suggested by others (Welch and Graham, 2004; Cakmak et al., 2010; Kutman et al., 2011). Additionally, we found that Zn and Fe was positively correlated with P (which is mainly accumulated as phytic acid in the grain) which could lead into problems of Zn and Fe bioavailability in the grain. Phytic acid acts as an antinutrient hindering Zn bioavailability, which can impact public health by reducing Zn and Fe absorption in the human body (Svečnjak et al., 2007; Shi et al., 2008; Gupta et al., 2015; Xue et al., 2015). Thus, breeding programs need to consider how to prevent high grain concentrations of phytic acid when breeding for high Zn and Fe concentrations in an attempt to achieve biofortification, as 49-80% of P in the grain is accumulated as phytic acid (Svečnjak et al., 2007).
4.3 Environmental and genetic differences influence grain yield and quality traits nearly equally
The relationship between wheat types, quality traits, and their interaction with different weather conditions has been studied before (Koppel and Ingver, 2008). Although wheat varieties with a persistent performance across diverse environments are desirable (Knapp et al., 2017), our results showed significant and large variation in yield and quality traits not only between wheat types, but also between years. Contrary to our second hypothesis (H2) and to other investigations (Labuschagne et al., 2007; Simons et al., 2012; Makawi et al., 2013; Taheri et al., 2021), grain yield was more affected by wheat type than by environment in our study (Table 3), whilst protein and grain N content were more affected by the environment (here weather) than the breeding target (Table 3). This suggests that both genetic and environmental variation influenced grain quality and yield similarly. The effect sizes for differences between years and between wheat types were greatest for grain yield, protein, gluten, starch, N, and P, implying that environment and genotype explained more of the variation than for the other studied traits, a finding that was also reported by others (Amiri et al., 2018).
4.4 High yield wheat type is the most efficient regarding EN, EN,ys in a sustainability perspective
Pronounced effects of wheat genotype and year on the various N use efficiency traits have been reported and discussed previously for the same material (Liu et al., 2022b). Here we evaluate differences in N uptake and use in relation to the breeding targets of the different wheat varieties (i.e., the wheat groups) and various grain quality traits. The efficiency of converting plant-internal N to grain biomass (EN) and starch yields (EN,ys) was clearly highest in the group of high-yielding varieties, irrespective of the year. This implies that the high-yielding varieties generate higher amounts of the targeted end product per whole-plant N than the other wheat types, which is positive in an environmental performance perspective, because lower amounts of resources (e.g., N fertilizer) are needed to produce the desired end product (Pourazari et al., 2018). If high protein contents are desired, the protein-specific N efficiency (EN,yp) is a more relevant measure. Our results indicate that the high-protein types were not more efficient in converting whole-plant N into protein yield than the other wheat types, because EN,yp was similar between the wheat types in the dry year (2018), and lower in the high-protein types in the year with a more normal weather (2019). An interesting measure for evaluating environmental performance is the total amount of N accumulated in the plant representing plant N requirement, here reflected by the N uptake efficiency (U). We hypothesized (H3) that the high-protein varieties should have greater N demand by means of higher U than the high-yield varieties, but surprisingly our results showed that U was either lower (2018) or similar (2019) in the high-protein varieties compared to the high-yield varieties. Breeding for high grain protein concentrations therefore is not necessarily associated with a high N uptake and N fertilizer requirement, although breeding target significantly influenced the U in both years (Table 6).
4.5 Near future breeding perspective
This study adopted an integrated perspective on grain yield and various quality traits in spring wheat. As wheat is one of the most important staple foods globally, the breeding programs have been focusing mostly on two major breeding targets, high yield and high protein content. In addition, the strong selection during wheat domestication and breeding have reduced the allelic diversity of both target and non-target traits so that a commercial cultivar consists of a single genotype. However, neglecting quality traits in breeding could prove crucial in the development of better elite varieties.
Although plant domestication and breeding have reduced the genetic diversity in target and non-target traits, the target traits seem to be linked to some non-target traits either by pleiotropy or linkage disequilibrium, where traits are inherited together as part of the same QTL contributing positively to the phenotypic expression of the target trait. Therefore, it is beneficial to plant breeding if a linkage block is discovered that has for breeders favorable effects on target and non-target traits (Charmet et al., 2005; Wang et al., 2012).
However, conventional and non-conventional plant breeding techniques might have a negative impact on some non-target traits, affecting some crop quality traits. Additionally, both target and non-target traits can be significantly affected by environmental factors.
Based on our results, we suggest the inclusion of biofortification as a regular quality trait in wheat breeding if the varieties show a high content of N, Zn, Fe, do not show a high influence of environmental effects and if do not show a strong correlation with P. Reaching bioavailability of Zn through genetic breeding has been the focus of many studies (Cakmak, 2008; Kutman et al., 2011; Guzmán et al., 2014; Tabbita et al., 2017), as it seems to be the most sustainable and cost-effective approach. Our results confirm a positive correlation between Zn and P across the spring wheat varieties in this study (Shi et al., 2008). If this result is a general pattern, it will cause problems in developing varieties with high Zn and low phytic acid concentrations. However, the development of a variety with this characteristic will be a beneficial long-term breeding goal. There are different strategies to implement biofortification. An approach proposed to cope with phytic acid accumulation in the seed was by increasing the level of the enzyme phytase (Nielsen et al., 2013). It has been reported that phytases are able to increase iron absorption in human intestine. Therefore, breeding for biofortified crops will require low phytic acid and high phytase content (Nielsen et al., 2013). Another method is to interfere in the Phytic acid biosynthesis pathway. Wheat inositol pentakiphosphate kinase (TalPK1) takes part in Phytic acid synthesis. After silencing IPK1 gene, a higher quantity of free phosphate in mature kernels was obtained. This finding was complemented by the rise of Zn and Fe in the grain content. Once more, the reduction of the Phytic acid content in cereal grains is a key step to achieve a greater accumulation and biovailability of Zn and Fe (Aggarwal et al., 2018). Furthermore, breeding efforts can be accompanied by agronomic biofortification. Plants required adequate amount of micronutrients from the soil and many of agricultural lands present complications that decrease availability of them to roots. Therefore, applications of Zn-fertilizers, Zn-NPK fertilizers and foliar sprays of ZnSO4 and FeSO4 suggest a complementary strategy to on-going breeding programs by enriching agricultural soil and by enhancing the re-translocation of Zn from flag leaves to the grain (Cakmak, 2008; Xue et al., 2015). Finally, the positive correlation between N and Zn showed in our results suggest that both traits could share genetic factors influencing the accumulation of Zn and N in the grain, even indicating that genes controlling protein and Zn accumulation in the grain are possibly co-segregated.
Our integrated perspective also considered environmental performance aspects related to crop resource (N) requirement and resource depletion of the production system. Genetic variation in traits related to nutrient use efficiency have been found in different cultivars, and include total N uptake, translocation, and assimilation (Xu et al., 2012). Additionally, total N uptake from soil is affected by the developmental growing stage of the plant, thus with a suitable N fertilization, will not increase N depletion. Moreover, based on the reviewed literature, N uptake and remobilization seem to be independently inherited traits, thus a combination of both alleles will be a positive addition to a cultivar for N uptake efficiency, and it will also allow the combination between low N uptake with high grain protein concentration (Xu et al., 2012).
5 Conclusions
High yield and high protein content are two of the most significant traits in modern wheat breeding. However, non-target traits are also required to breed new varieties, especially because some are related to quality concerns. Our results indicate that grain yield and quality parameters are affected by environmental conditions and wheat type in similar proportions. In addition, our results suggest that correlations between target and non-target traits tend to remain stable within the wheat types depending on their breeding target across different weather conditions, and the majority of the traits generally conserve their static stability (except for Fe). Furthermore, the inclusion of macro- and micro-elements in wheat varieties pose a new perception for quality, and should be further investigated. Moreover, in the case of varieties with a high protein content, biofortification may be considered in future breeding programs as a viable trait especially for varieties in which these traits are not strongly affected by the environmental conditions. Finally, the high-yielding varieties generate higher amounts of the targeted end product per whole-plant N than the other wheat types, and breeding for high grain protein concentrations is not necessarily associated with a high N fertilizer requirement, which has implications for the environmental performance of the crop.
Data availability statement
The raw data supporting the conclusions of this article will be made available by the authors, without undue reservation.
Author contributions
The field experiment was mainly planned and designed by MW and AW, and all authors were involved in the designing of the study; LG-V.: conceptualization, methodology, investigation, data analysis, writing (original draft preparation); HL: collecting all the data, methodology, writing (review and editing); JC: conceptualization, methodology, supervision, writing (review and editing); AW: conceptualization, methodology, supervision, writing (review and editing); MW: conceptualization, methodology, investigation, analysis of the data, project administration, supervision, writing (review and editing). All authors have read and agreed to the published version of the manuscript.
Funding
This research was financed by grants from the Swedish research council Formas (grants number 2016-00491 and 2019-00314 to MW).
Conflict of interest
The authors declare that the research was conducted in the absence of any commercial or financial relationships that could be construed as a potential conflict of interest.
Publisher’s note
All claims expressed in this article are solely those of the authors and do not necessarily represent those of their affiliated organizations, or those of the publisher, the editors and the reviewers. Any product that may be evaluated in this article, or claim that may be made by its manufacturer, is not guaranteed or endorsed by the publisher.
Supplementary material
The Supplementary Material for this article can be found online at: https://www.frontiersin.org/articles/10.3389/fagro.2023.1151015/full#supplementary-material
References
Aggarwal S., Kumar A., Bhati K. K., Kaur G., Shukla V., Tiwari S., et al. (2018). RNAi-mediated downregulation of inositol pentakisphosphate kinase (IPK1) in wheat grains decreases phytic acid levels and increases fe and zn accumulation. Front. Plant Sci. 9, 259. doi: 10.3389/fpls.2018.00259
Amiri R., Sasani S., Jalali-Honarmand S., Rasaei A., Seifolahpour B., Bahraminejad S. (2018). Genetic diversity of bread wheat genotypes in Iran for some nutritional value and baking quality traits. Physiol. Mol. Biol. Plants 24, 147–157. doi: 10.1007/s12298-017-0481-4
Baye A., Berihun B., Bantayehu M., Derebe B. (2020). Genotypic and phenotypic correlation and path coefficient analysis for yield and yield-related traits in advanced bread wheat (Triticum aestivum l.) lines. Cogent. Food Agric. 6, 1752603. doi: 10.1080/23311932.2020.1752603
Bogard M., Allard V., Brancourt-Hulmel M., Heumez E., Machet J.-M., Jeuffroy M.-H., et al. (2010). Deviation from the grain protein concentration–grain yield negative relationship is highly correlated to post-anthesis n uptake in winter wheat. J. Exp. Bot. 61, 4303–4312. doi: 10.1093/jxb/erq238
Cakmak I. (2008). Enrichment of cereal grains with zinc: agronomic or genetic biofortification? Plant Soil 302, 1–17. doi: 10.1007/s11104-007-9466-3
Cakmak I., Pfeiffer W. H., Mcclafferty B. (2010). Biofortification of durum wheat with zinc and iron. Cereal Chem. 87, 10–20. doi: 10.1094/CCHEM-87-1-0010
Charmet G. (2011). Wheat domestication: lessons for the future. Comptes. Rendus. Biol. 334, 212–220. doi: 10.1016/j.crvi.2010.12.013
Charmet G., Robert N., Branlard G., Linossier L., Martre P., Triboi E. (2005). Genetic analysis of dry matter and nitrogen accumulation and protein composition in wheat kernels. Theor. Appl. Genet. 111, 540–550. doi: 10.1007/s00122-005-2045-1
Dempewolf H., Baute G., Anderson J., Kilian B., Smith C., Guarino L. (2017). Past and future use of wild relatives in crop breeding. Crop Sci. 57, 1070–1082. doi: 10.2135/cropsci2016.10.0885
Dwivedi S. L., Van Bueren E. T. L., Ceccarelli S., Grando S., Upadhyaya H. D., Ortiz R. (2017). Diversifying food systems in the pursuit of sustainable food production and healthy diets. Trends Plant Sci. 22, 842–856. doi: 10.1016/j.tplants.2017.06.011
Fowler D. B. (2003). Crop nitrogen demand and grain protein concentration of spring and winter wheat. Agron. J. 95, 260–265. doi: 10.2134/agronj2003.2600
Gupta P., Balyan H., Sharma S., Kumar R. (2021). Biofortification and bioavailability of zn, fe and Se in wheat: Present status and future prospects. Theor. Appl. Genet. 134, 1–35. doi: 10.1007/s00122-020-03709-7
Gupta R., Gangoliya S., Singh N. (2015). Screening and characterization of wheat germplasms for phytic acid and iron content. J. Agric. Sci. Technol. 17, 747–756.
Guzmán C., Medina-Larqué A. S., Velu G., González-Santoyo H., Singh R. P., Huerta-Espino J., et al. (2014). Use of wheat genetic resources to develop biofortified wheat with enhanced grain zinc and iron concentrations and desirable processing quality. J. Cereal Sci. 60, 617–622. doi: 10.1016/j.jcs.2014.07.006
Guzman C., Peña R. J., Singh R., Autrique E., Dreisigacker S., Crossa J., et al. (2016). Wheat quality improvement at CIMMYT and the use of genomic selection on it. Appl. Trans. Genomics 11, 3–8. doi: 10.1016/j.atg.2016.10.004
Iqbal M. S., Kamran M., Akbar M. (2022). Agromorphological traits and comparative performance for boosting yields in wheat (Triticum aestivum l.) germplasm and cluster analysis. Bangladesh. J. Bot. 51, 23–28. doi: 10.3329/bjb.v51i1.58816
Knapp S., Brabant C., Oberforster M., Grausgruber H., Hiltbrunner J. (2017). Quality traits in winter wheat: Comparison of stability parameters and correlations between traits regarding their stability. J. Cereal Sci. 77, 186–193. doi: 10.1016/j.jcs.2017.08.011
Koppel R., Ingver A. (2008). A comparison of the yield and quality traits of winter and spring wheat. Latvian. J. Agron. 11, 83–89.
Kutman U. B., Yildiz B., Cakmak I. (2011). Improved nitrogen status enhances zinc and iron concentrations both in the whole grain and the endosperm fraction of wheat. J. Cereal Sci. 53, 118–125. doi: 10.1016/j.jcs.2010.10.006
Labuschagne M. T., Geleta N., Osthoff G. (2007). The influence of environment on starch content and amylose to amylopectin ratio in wheat. Starch-Stärke 59, 234–238. doi: 10.1002/star.200600542
Labuschagne M. T., Moloi J., Van Biljon A. (2016). Abiotic stress induced changes in protein quality and quantity of two bread wheat cultivars. J. Cereal Sci. 69, 259–263. doi: 10.1016/j.jcs.2016.03.018
Lancashire P. D., Bleiholder H., Boom T. V. D., Langelüddeke P., Stauss R., Weber E., et al. (1991). A uniform decimal code for growth stages of crops and weeds. Ann. Appl. Biol. 119, 561–601. doi: 10.1111/j.1744-7348.1991.tb04895.x
Liu H., Colombi T., Jäck O., Keller T., Weih M. (2022a). Effects of soil compaction on grain yield of wheat depend on weather conditions. Sci. Total. Environ. 807, 150763. doi: 10.1016/j.scitotenv.2021.150763
Liu H., Colombi T., Jäck O., Westerbergh A., Weih M. (2022b). Linking wheat nitrogen use to root traits: Shallow and thin embryonic roots enhance uptake but reduce conversion efficiency of nitrogen. Field Crops Res. 285, 108603. doi: 10.1016/j.fcr.2022.108603
Makawi A. B., Mahmood M. I., Hassan H. A. R., Ahmed I. A. M. (2013). Grains quality characteristics of local wheat (Triticum aestivum) cultivars grown at Khartoum state, Sudan. Int. J. Life Sci. 7, 12–16. doi: 10.3126/ijls.v7i1.8061
Mecha B., Alamerew S., Assefa A., Dutamo D., Assefa E. (2017). Correlation and path coefficient studies of yield and yield associated traits in bread wheat (Triticum aestivum l.) genotypes. Adv. Plants Agric. Res. 6, 1–10.
Mut Z., Aydin N., Orhan Bayramoglu H., Ozcan H. (2010). Stability of some quality traits in bread wheat (Triticum aestivum) genotypes. J. Environ. Biol. 31, 489.
Nagarajan S., Tripathi S., Singh G., Chaudhary H. (2007). Effect of genotype and environment on quality traits and grain yield of wheat. Indian J. Genet. Plant Breed. 27, 149–152.
Nielsen A. V., Tetens I., Meyer A. S. (2013). Potential of phytase-mediated iron release from cereal-based foods: a quantitative view. Nutrients 5, 3074–3098. doi: 10.3390/nu5083074
Ortiz-Monasterio J. I., Palacios-Rojas N., Meng E., Pixley K., Trethowan R., Pena R. (2007). Enhancing the mineral and vitamin content of wheat and maize through plant breeding. J. Cereal Sci. 46, 293–307. doi: 10.1016/j.jcs.2007.06.005
Pourazari F., Andersson M., Weih M. (2018). Altered tuber yield in genetically modified high-amylose and oil potato lines is associated with changed whole-plant nitrogen economy. Front. Plant Sci. 9, 342. doi: 10.3389/fpls.2018.00342
Shi R., Li H., Tong Y., Jing R., Zhang F., Zou C. (2008). Identification of quantitative trait locus of zinc and phosphorus density in wheat (Triticum aestivum l.) grain. Plant Soil 306, 95–104. doi: 10.1007/s11104-007-9483-2
Simons K., Anderson J. A., Mergoum M., Faris J. D., Klindworth D. L., Xu S. S., et al. (2012). Genetic mapping analysis of bread-making quality traits in spring wheat. Crop Sci. 52, 2182–2197. doi: 10.2135/cropsci2012.03.0175
Svečnjak Z., Bujan M., Vedrina Dragojević I., Vitali D., Čebuškin A., Jenel M. (2007). Nitrogen and phosphorus content, hectoliter weight and yield variations of wheat grain as affected by cropping intensity. Agricult. Conspectus. Sci. 72, 251–255.
Tabbita F., Pearce S., Barneix A. J. (2017). Breeding for increased grain protein and micronutrient content in wheat: Ten years of the GPC-B1 gene. J. Cereal Sci. 73, 183–191. doi: 10.1016/j.jcs.2017.01.003
Taheri A., Heidari Sharif Abad H., Nourmohammadi G., Seyedain Ardabili M. (2021). Investigating quantitative and qualitative performance of bread wheat genotypes under different climatic conditions. Gesunde. Pflanzen. 73, 229–238. doi: 10.1007/s10343-021-00547-5
Tayyar S. (2010). Variation in grain yield and quality of Romanian bread wheat varieties compared to local varieties in northwestern Turkey. Romanian. Biotechnol. Lett. 15, 5189–5196.
Velu G., Singh R. P., Huerta J., Guzmán C. (2017). Genetic impact of rht dwarfing genes on grain micronutrients concentration in wheat. Field Crops Res. 214, 373–377. doi: 10.1016/j.fcr.2017.09.030
Wang L., Cui F., Wang J., Jun L., Ding A., Zhao C., et al. (2012). Conditional QTL mapping of protein content in wheat with respect to grain yield and its components. J. Genet. 91, 303–312. doi: 10.1007/s12041-012-0190-2
Weih M. (2003). Trade-offs in plants and the prospects for breeding using modern biotechnology. New Phytol. 158, 7–9. doi: 10.1046/j.1469-8137.2003.00716.x
Weih M., Asplund L., Bergkvist G. (2011). Assessment of nutrient use in annual and perennial crops: A functional concept for analyzing nitrogen use efficiency. Plant Soil 339, 513–520. doi: 10.1007/s11104-010-0599-4
Weih M., Hamnér K., Pourazari F. (2018). Analyzing plant nutrient uptake and utilization efficiencies: comparison between crops and approaches. Plant Soil 430, 7–21. doi: 10.1007/s11104-018-3738-y
Weih M., Hoeber S., Beyer F., Fransson P. (2014). Traits to ecosystems: the ecological sustainability challenge when developing future energy crops. Front. Energy Res. 2, 17. doi: 10.3389/fenrg.2014.00017
Weih M., Liu H., Colombi T., Keller T., Jäck O., Vallenback P., et al. (2021). Evidence for magnesium–phosphorus synergism and co-limitation of grain yield in wheat agriculture. Sci. Rep. 11, 1–13. doi: 10.1038/s41598-021-88588-8
Welch R. M., Graham R. D. (2004). Breeding for micronutrients in staple food crops from a human nutrition perspective. J. Exp. Bot. 55, 353–364. doi: 10.1093/jxb/erh064
Xu G., Fan X., Miller A. J. (2012). Plant nitrogen assimilation and use efficiency. Annu. Rev. Plant Biol. 63, 153–182. doi: 10.1146/annurev-arplant-042811-105532
Xue Y.-F., Xia H.-Y., Mcgrath S. P., Shewry P. R., Zhao F.-J. (2015). Distribution of the stable isotopes 57 fe and 68 zn in grain tissues of various wheat lines differing in their phytate content. Plant Soil 396, 73–83. doi: 10.1007/s11104-015-2582-6
Xurun Y., Xinyu C., Liang Z., Jing Z., Heng Y., Shanshan S., et al. (2015). Structural development of wheat nutrient transfer tissues and their relationships with filial tissues development. Protoplasma 252, 605–617. doi: 10.1007/s00709-014-0706-0
Keywords: grain yield, grain quality, target traits, non-target traits, nitrogen uptake efficiency, breeding targets, spring wheat
Citation: Guardia-Velarde L, Liu H, Cope JE, Westerbergh A and Weih M (2023) Differential breeding targets in wheat influence non-target traits related to grain quality, but not crop nitrogen requirement. Front. Agron. 5:1151015. doi: 10.3389/fagro.2023.1151015
Received: 25 January 2023; Accepted: 16 March 2023;
Published: 04 April 2023.
Edited by:
Nasim Ahmad Yasin, University of the Punjab, PakistanReviewed by:
Rehana Sardar, University of the Punjab, PakistanMuhammad Akbar, University of Gujrat, Pakistan
Copyright © 2023 Guardia-Velarde, Liu, Cope, Westerbergh and Weih. This is an open-access article distributed under the terms of the Creative Commons Attribution License (CC BY). The use, distribution or reproduction in other forums is permitted, provided the original author(s) and the copyright owner(s) are credited and that the original publication in this journal is cited, in accordance with accepted academic practice. No use, distribution or reproduction is permitted which does not comply with these terms.
*Correspondence: Lorena Guardia-Velarde, bG9yZW5hLmd1YXJkaWFAc2x1LnNl