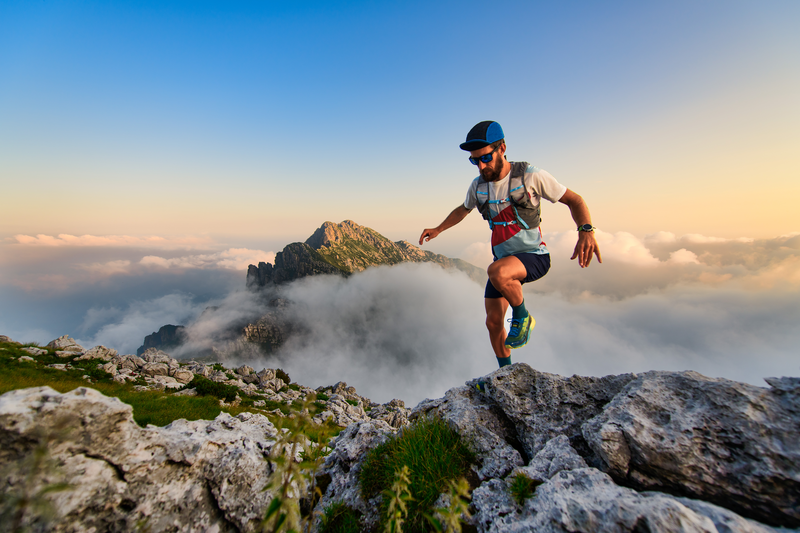
95% of researchers rate our articles as excellent or good
Learn more about the work of our research integrity team to safeguard the quality of each article we publish.
Find out more
ORIGINAL RESEARCH article
Front. Agron. , 18 April 2023
Sec. Plant-Soil Interactions
Volume 5 - 2023 | https://doi.org/10.3389/fagro.2023.1138263
Research efforts to develop alternatives to chemical-based fertilizers for sustainable crop production has led to renewed interest in beneficial soil microbes such as rhizobia and plant growth promoting biostimulants such as the seaweed (Ecklonia maxima). This study assessed the interactive effect of the co-application of seaweed extract with two Bradyrhizobium strains (Inoculant 1 and Inoculant 2) on the growth, symbiotic performance and nutritional composition of three cowpea (Vigna unguiculata (L.) Walp.) genotypes (IT97K-390-2, Songotra and TVU13998) grown under glasshouse conditions. The response of cowpea to the treatments was genotype dependent, such that the combined application of inoculant 2 plus seaweed extract increased nodule dry matter in genotype Songotra, and together with sole inoculant 2 increased the parameter in genotype IT97K-390-2, just as the inoculation plus seaweed extract treatments increased the parameter in genotype TVU13998 when compared to their respective counterparts receiving other treatments. Sole inoculation or inoculation plus seaweed extract treatments increased shoot dry matter in all varieties (2.0 to 7.2 g.plant-1) relative to the control plants receiving sole nitrate (0.5 to 1.2 g.plant-1), sole seaweed extract (0.3 g.plant-1), nitrate plus seaweed extract (1.2 to 1.6 g.plant-1) or the absolute control (0.2 g.plant-1). Due to N2 fixation in the inoculated plants, their leaf δ15N (-2.66‰ to -1.20‰) were markedly lower (p≤0.001) than values recorded by the control plants (+3.30‰ to +510‰) which had no nodules; consequently, leaf N accumulation was greater in the inoculation-based treatments (41.2 to 258.2 mg.plant-1) relative to the uninoculated controls (1.7 to 24.7 mg.plant-1). In most instances, the sole inoculation and inoculation plus seaweed extract treatments increased leaf photosynthetic rates (except for genotype TVU13998 treated with inoculant 1 + seaweed extract), water use efficiency (δ13C) (except in genotype TVU13998) and the concentrations of macro and micronutrients in leaves (except for K in Songotra treated with inoculant 1 or inoculant 1 + seaweed extract as well as Mn in TVU13998 treated with inoculant 1 among others) of the cowpeas relative to the controls. We highlight the potential benefits of the synergistic interactions between rhizobia and seaweed extract for enhancing plant growth and nutrient accumulation in cowpea leaves.
Cowpea (Vigna unguiculata (L.) Walp.) is an indigenous African legume which is widely produced and consumed worldwide. It is extensively grown in Africa, with production dominated in Sub Saharan Africa by Nigeria and Niger, contributing about 80% of global production (Boukar et al., 2019). Cowpea production in Africa is mostly by smallholder farmers, who intercrop it with other grains such as maize, millet, and sorghum (Namatsheve et al., 2020). In the year 2021, the world’s cowpea production was estimated at 8.99 M tonnes from a total land area of 10.8 M hectares, with Africa alone contributing 97% of the quantity produced (FAO, 2023). In South Africa, cowpea is considered an underutilized and under researched crop, resulting in its low adoption and production compared to the rest of the continent. In 2021 for example, South Africa’s cowpea production accounted for just 0.05% of Africa’s production figure in that year (FAO, 2023). Due to the low research focus on cowpea in South Africa, there are inadequate genotypes and production guidelines to promote its widespread cultivation, despite its potential in improving food and nutritional security especially among low-income households. In most areas of cowpea cultivation, both the grain and leaves as well as the green pods are consumed for their nutritional, health and economic benefits (Belane and Dakora, 2011; Alemu et al., 2016), providing affordable and alternative source of protein and minerals to households that consume them relative to other sources of protein such as meat (Das et al., 2012). Thus, improved research on its production in areas where it is lesser known has the potential to promote food and nutritional security.
Economically, many farmers who cultivate the crop are also able to earn an income from its production due to the high demand for human consumption, and for use as animal feed (Singh et al., 2002). Environment-wise, cowpea production can promote soil fertility through symbiotic associations with effective compatible bacteria collectively called rhizobia, making it an ideal crop in rotation and intercropping with cereals (Ndakidemi, 2006). This ability of cowpea and other legumes to fix N2 means that their production does not necessarily require the use of chemical N fertilizers, reducing their harmful effects to the environment and reducing cost for many farmers. Even though some studies have quantified the N2 fixation of cowpea in South African soils (Ayisi et al., 2000; Makoi et al., 2009; Pule-Meulenberg et al., 2010; Belane et al., 2011; Masete et al., 2022), these studies largely assessed exotic commercial inoculants. There is still inadequate information regarding the N2 fixation potential of both commercial and native rhizobia strains.
In recent times, the adoption of bio-stimulants such as seaweed extracts to enhance plant growth offers an environmentally sustainable way of increasing food production as it can improve mineral absorption, reducing excessive fertilizer use (Rathore et al., 2009). The plant growth promoting function of seaweed extracts could be due to the presence of macro and micronutrients and plant growth promoting hormones such as auxins, cytokinins, polysaccharides and amino acids (Craigie, 2011). However, there is lack of knowledge regarding the interaction effect between bio-stimulants such as seaweed extract (Ecklonia maxima) and rhizobia in enhancing cowpea productivity.
In this study, the effect of seaweed extract and cowpea rhizobia inoculation (a commercial inoculant and a test native strain isolated from South Africa) was assessed to determine their effect on cowpea growth, N2 fixation and nutritional composition. We hypothesized that the application of seaweed extract alongside rhizobial inoculation will improve plant growth, photosynthetic parameters, and nutrient accumulation in different cowpea varieties. The findings of the study is expected to contribute useful knowledge to promote cowpea production in South Africa.
The experiment was carried out under glasshouse conditions at the Skills Center of the Department of Crop Sciences, Tshwane University of Technology, using a completely randomized design with four replications. The plants were grown in 2 litre capacity plastic pots with three perforated holes at the bottom and lined with non-absorbent cotton wool to reduce water leakage. The cowpea seeds were sterilized by rinsing in 95% ethanol for 10 seconds and submerged in 0.1% sodium hypochlorite (commercial bleach) for three minutes. The seeds were then rinsed in six changes of sterilized distilled water before sowing. Autoclaved sterile sand (Green’s Sand, Pretoria) was used as a rooting medium for the plants. The rhizobia strain used included Bradyrhizobium sp. strain CB756, which is an exotic commercial inoculant (i.e inoculant 1) and a native Bradyrhizobium sp. strain TUTVUSA28 closely related to Bradyrhizobium daqingense which was earlier isolated from root nodules of cowpea in South Africa (i.e inoculant 2) (Mohammed et al., 2018). Originally, Bradyrhizobium daqingense was isolated from root nodules of soybean in China even though it was later found to also be an effective symbiont of cowpea (Wang et al., 2013). The cowpea variety Songotra was previously obtained from the CSIR-Savanna Agricultural Research Institute (SARI) in Ghana while the genotypes IT97K-390-2 and TVU13998 were obtained from the International Institute of Tropical Agriculture (IITA) in Mozambique. The seaweed extract was applied as a commercial product called Kelpak, a liquid seaweed extract from the giant kelp Ecklonia maxima which contains phytohormones such as auxins and cytokinins as well as amino acids.
The experiment involved three cowpea varieties (namely, IT97K-390-2, Songotra and TVU13998) and eight (8) treatment levels which included sole inoculant1 only, sole inoculant 2 only, seaweed extract + inoculant 1, seaweed extract + inoculant 2, sole seaweed extract, N only (applied as 5 mM KNO3 for positive control), N + seaweed extract, and control (no inoculant, N or seaweed extract). The inoculant treatments were prepared by growing each Bradyrhizobium sp. in yeast mannitol broth to the exponential growth stage which corresponded to 106 – 107 cells/ml.
Three seeds of each variety were planted directly in the pots and thinned to one seedling per pot after germination. Three days after emergence and thinning out, 2 ml each of inoculant 1 and inoculant 2 was applied to the respective inoculated treatments. Seaweed extract was supplied to the plants weekly (0.5-1% solution) as a soil drench with nutrient. The seedlings were supplied with Dilworth’s N-free nutrient solution (Broughton and Dilworth, 1971) and watered when necessary. The glasshouse was naturally lit with daily temperatures between 25 and 30°C during the experimental period.
At the R2 growth stage (when N2 fixation is expected to reach a peak), corresponding to 50 days after planting, the plants were harvested and processed into nodules, roots and shoots. Nodule number per plant was recorded as well as the nodule pigmentation to assess effectiveness of the strains. The shoots and roots were oven-dried (60°C) for 72h and weighed. The shoots were ground, (0.85 mm) for analysis of N concentration using the Kjeldahl digestion at the institute of plant production, Elsenberg, South Africa. Since the plants were supplied with N-free nutrient solution during the growth period and sterile soil was used, shoot N accumulation (the product of %N and plant biomass) was used as a measure of N-fixed.
To assess N2 fixation and carbon accumulation/water use efficiency, cowpea leaves were subjected to 15N/14N and 13C/12C isotopic analysis, respectively at the University of Cape Town using a Carlo Erba NA1500 elemental analyzer coupled to a Finnigan MAT 252 mass spectrometer (Finnigan MAT GmbH, Bremen, Germany) via Conflo II open-split device. For this, 2.00 mg of ground plant sample was weighed into aluminium capsules, loaded onto the Carlo-Erba system, combusted in evacuated quartz tubes in the presence of cupric oxide and metallic copper, and the resultant gases cleaned on-line before being introduced into the mass spectrometer. An internal standard, consisting of material from a Nasturtium spp. was included in every five runs to correct for machine errors during isotopic fractionation. The isotopic composition (δ15N) was measured according to the following relationship (Junk and Svec, 1958; Mariotti et al., 1981).
Where 15N/14Nsample is the δ15N value of cowpea leaf, and 15N/14Natm is the δ15N relative to atmospheric nitrogen.
The 13C natural abundance (δ13C) was calculated using the relation below (Farquhar et al., 1989):
Where, 13C/12Csample is the isotopic ratio of the sample and 13C/12Cstandard is the isotopic ratio of PDB, a universally accepted standard from Belemnite Pee Dee limestone formation (Craig, 1957).
Both the %N and %C of shoot samples were obtained directly from the mass spectrometry analysis and the N and C content computed as the product of either %N or %C x shoot dry matter respectively. The results of only N and C content are reported.
Gas-exchange measurements were carried out on three fully expanded trifoliate leaves per each replicate pot. A reading was taken per trifoliate leaf totalling three readings per pot and nine readings per treatment. The means of the three readings obtained per each pot were used for analysis. The gas exchange parameters measured included Photosynthetic rates (A), stomatal conductance (gs), and transpiration rates (E) using a portable infra-red gas analyser (LI 6400 XT, version 6.2). The prevailing conditions in the chamber included: photosynthetic photon flux density of 1000 µmolm-2s-1, CO2 concentration of 400 µmolmol-1 and flow rate of 500 µmols-1. The measurements were carried out in the mornings between 8 and 10 am. An instantaneous measure of water-use efficiency (WUEi) was computed as the ratio of A to gs (Singh and Reddy, 2011), and the data presented for photosynthesis, stomata conductance and transpiration rates.
Macro (P, K, Ca, Mg and Na) and micronutrient (Fe, Zn and Mn) concentrations in the cowpea leaves were determined using ICP-mass spectrometry at the Soil, Water and Plant Diagnostics laboratory, Elsenburg, Western Cape Provincial Department of Agriculture. One gram of ground plant sample was ashed overnight in a porcelain crucible at 500°C, followed by dissolving the ash in 5 ml 6 M HCl, placing it in an oven at 50°C for 30 minutes after which 35 ml de-ionized water was added. The mixture was then filtered through Whatman No. 1 filter paper, and the mineral element concentrations determined from three replicate samples using inductively coupled plasma mass spectrometry (IRIS/AP HR DUO Thermo Electron Corporation, Franklin, Massachusetts, USA). The quality of the data collected was assessed using standard solutions with certificates of analysis. In place of analyte isotopes to monitor each element, a known sample was used as a standard after every 10 samples.
All quantitative data collected were subjected to a factorial ANOVA analysis using STATISTICA 8.0 program (StatSoft, 2007). The datasets had skewness and kurtosis values ranging from -0.97 to +2.43 and -1.34 to +8.12, respectively, an indication of normal distribution. Kline (2015) indicated that skewness values between -3 to +3 and kurtosis values between -10 to +10 indicate normal distribution. A two-way ANOVA was used considering genotype and treatment as the factors. Where there were significant treatment differences, the Duncan’s Multiple Range Test was used to separate the means at p ≤ 0.05.
There were significant effects of genotype x treatment interaction (p ≤ 0.001) on nodulation and plant growth parameters (Supplementary Table S1). As shown in Figure 1, nodulation was generally higher in plants of genotypes IT97K-390-2 and Songotra, and least in plants of genotype TVU13998. Plants of the genotype IT97K-390-2 treated with inoculant 2 only (131 nodules) and the combination of inoculant 2 and seaweed extract (142 nodules) induced higher nodule numbers when compared to their counterparts receiving only inoculant 1 or inoculant 1 plus seaweed extract. In genotype Songotra, plants treated with inoculant 1 and seaweed extract (133 nodules), as well as those treated with inoculant 2 and seaweed extract (130 nodules) induced higher nodulation than their counterparts receiving sole inoculant treatments. Irrespective of treatment, genotype TVU13998 generally recorded lower nodule numbers (p ≤ 0.001), which ranged between 45 and 82 nodules per plant when compared to values recorded by the other genotypes receiving the same treatments (Figure 1A). The application of inoculant 2 together with seaweed extract significantly increased nodule dry matter (p ≤ 0.001) in genotypes IT97K-390-2 (0.5 g.plant-1) and Songotra (0.5 g.plant-1) when compared to their counterparts receiving inoculant 1 alone or seaweed extract. However, the sole application or inoculant 2 to genotype IT97K-390-2 resulted in similar nodule dry matter (p ≤ 0.001) as plants receiving inoculant 2 plus seaweed extract (Figure 1B). In genotype TUV13998 however, the application of either inoculant 1 or inoculant 2 together with seaweed extract resulted in similarly greater nodule dry matter (p ≤ 0.001) when compared to their counterparts receiving other treatments (p ≤ 0.001) (Figure 1B).
Figure 1 Interactive effect of genotype x treatment on nodule number (A), nodule dry matter (B), root dry matter (C) and shoot dry matter (D) of three cowpea genotypes, planted under glasshouse conditions. Inoculant 1(Inoc 1) = B. yuanmingense strain CB756 (commercial inoculant), Inoculant 2 (Inoc 2) = Bradyrhizobium sp. strain TUTVUSA (closely related to B. daqingense), control = no inoculant and no kelpak, N= 5mM KNO3, control= no inoculation and no kelpak. Vertical lines on bars represent S.E. Bars followed by dissimilar letters are significant at ***p ≤ 0.001.
Root biomass accumulation was highest (p ≤ 0.001) in plants of genotype Songotra that were treated with inoculant 1 plus seaweed extract (2.6 g.plant-1) or inoculant 2 plus seaweed extract (2.1 g.plant-1) (Figure 1C). The interaction effect of genotype x treatment was also significant or shoot dry matter (Supplementary Table S1). In all the genotypes, either sole inoculation or inoculation plus seaweed extract application significantly increased shoot biomass (2.0 – 7.2 g.plant-1) (p ≤ 0.001) when compared to their counterparts treated with 5 mM KNO3, sole seaweed extract or nitrate plus seaweed extract (0.2 – 1.6 g.plant-1). However, shoot biomass was increased in the plants of genotype Songotra that were treated with inoculant 1 plus seaweed extract (6.1 g.plant-1) and inoculant 2 with seaweed extract (7.2 g.plant-1) when compared to their counterparts receiving sole inoculation (Figure 1D). Similarly, dual application of either inoculants plus seaweed extracts increased shoot biomass in genotype TVU13998 when compared to their sole inoculated counterparts (Figure 1D).
The symbiotic performance (measured as δ15N and N content) and carbon nutrition (measured as δ13C and C content) of the three cowpea genotypes tested, revealed significant differences (p ≤ 0.001) (Supplementary Table S1). In all the cowpea genotypes, sole inoculation or inoculation with either inoculant plus seaweed extract resulted in much lower δ15N values (p ≤ 0.001) when compared to all the uninoculated treatments (Figure 2A). For example, the sole application of inoculant 2 led to the lowest δ15N values for plants of genotype IT97K-390-2 (-2.66 ‰) and Songotra (-2.35 ‰), while inoculant 2 plus seaweed extract application reduced δ15N values for TVU13998 (-2.51 ‰). The control plants generally recorded very high δ15N values, with N treated plants recording the highest value of +4.64 ‰ for IT97K-390-2 while sole N and N plus seaweed extract resulted in the highest values (p ≤ 0.001) of +5.09 and +5.10 ‰, respectively for Songotra. Sole N and seaweed extract treatments increased δ15N values (+4.67 and +4.90 ‰) for genotype TVU13998 (Figure 2A).
Figure 2 Interactive effect of genotype x treatment on δ15N (A), leaf N content (B), leaf δ13C (C) and leaf C content (D) of three cowpea genotypes, planted under glasshouse conditions. Inoculant 1(Inoc 1) = B. yuanmingense strain CB756 (commercial inoculant), Inoculant 2 (Inoc 2) = Bradyrhizobium sp. strain TUTVUSA (closely related to B. daqingense), control = no inoculant and no kelpak, N= 5mM KNO3, control= no inoculation and no kelpak. Vertical lines on bars represent S.E. Bars followed by dissimilar letters are significant at ***p ≤ 0.001.
Leaf N content was markedly increased (p ≤ 0.001) in the cowpea genotypes that received sole inoculation or inoculation plus seaweed extract (Figure 2B). Generally, treatment combinations that gave lower δ15N values also resulted in greater leaf N content. For example, the plants of Songotra treated with inoculant 1 plus seaweed extract (25.82 g.plant-1) or inoculant 2 plus seaweed extract (24.37 g.plant-1) as well as plants of genotype TVU13998 treated with inoculant 1 plus seaweed extract, recorded the highest (p ≤ 0.001) leaf N content (Figure 2B). The control treatment generally induced very low N content in the cowpea leaves (Figure 2B).
Leaf δ13C values (used as surrogate for long term water use efficiency) were generally higher for all the inoculated and inoculation plus seaweed extract treatments for all genotypes relative to their control counterparts; although there were instances where the observed differences were not significant (Figure 2C). For example, the plants of genotype IT97K-390-2 treated with inoculant 1 (-24.70‰) or inoculant 1 plus seaweed extract (-24.62‰) recorded the highest δ13C values (p ≤ 0.001) when compared to other genotype/treatment combinations, followed by plants treated with inoculant 2 (-25.16‰) and inoculant 2 plus seaweed extract plants (-25.1 ‰), and then the plants of Songotra treated with inoculant 2 plus seaweed extract (-25.54 ‰) as well as plants of TVU13998 treated with inoculant 2 and 1 plus seaweed extract (-25.82‰ and -26.28‰), respectively (Figure 2C).
There was a significant effect of genotype x treatment interaction on leaf C accumulation (measured as leaf C content) (Supplementary Table S1). As shown in Figure 2D, the trends of leaf C content mirrored the trend observed for leaf N content such that, the sole inoculation or inoculation plus seaweed treatments increased leaf C content in all genotypes, with values ranging from 0.82 g/plant to 3.10 g/plant when compared to values for the uninoculated control treatments (0.06 g/plant to 0.64 g/plant), although with one exception in genotype TVU13998. The highest C content was recorded by applying either inoculant 2 plus seaweed extract in genotype Songotra followed by the application of inoculant 1 plus seaweed extract to the same genotype (Figure 2D). This was followed by plants of genotype TVU13998 that were treated with inoculant 1 plus seaweed extract. The lowest C contents (p ≤ 0.001) were recorded in the control treatments of the test genotypes, with values ranging between 50 and 100 mg.kg-1 (Figure 2D).
The genotype x treatment interaction effect was significant (p ≤ 0.01) for stomata conductance, transpiration, photosynthesis, and instantaneous water use efficiency (Supplementary Table S2). In all the genotypes, there was a general tendency for sole inoculation or inoculation plus seaweed extract treatments increased stomatal conductance relative to the control treatments (Figure 3A). For example, sole inoculant 1 treatment increased stomatal conductance (p ≤ 0.01) in genotype IT97K-390-2 90 when compared to all other treatments (Figure 3A). In genotype Songotra, sole inoculation with either inoculants alone or together with seaweed extract led to significantly higher (p ≤ 0.01) stomatal conductance when compared to the control treatments that had no inoculants. Thus, the uninoculated treatments generally recorded lower stomata conductance across the genotypes (Figure 3A).
Figure 3 Interactive effect of genotype x treatment on stomata conductance (A), transpiration (B), leaf photosynthesis rates (C) and WUEi (D) of three cowpea genotypes, planted under glasshouse conditions. Inoculant 1(Inoc 1) = B. yuanmingense strain CB756 (commercial inoculant), Inoculant 2 (Inoc 2) = Bradyrhizobium sp. strain TUTVUSA (closely related to B. daqingense), control = no inoculant and no kelpak, N= 5mM KNO3, control= no inoculation and no kelpak. Vertical lines on bars represent S.E. Bars followed by dissimilar letters are significant at **p ≤ 0.01 or ***p ≤ 0.001.
Transpiration and photosynthesis rates among the genotypes generally mirrored the trend in stomata conductance, with the sole and inoculation plus seaweed extract treatments generally recording higher transpiration rates when compared to the uninoculated control treatments, although with few exceptions (Figures 3B, C). Sole inoculant 1 treatments of IT97K-390-2 plants generally resulted in increased transpiration and photosynthesis, followed by both sole and inoculated treatments of Songotra plants (Figures 3B, C).
With WUEi, the lowest values (p ≤ 0.001) were recorded in the absolute control plants that did not receive inoculant, nitrate, or seaweed extract (Figure 3). Inoculant 1 plus seaweed extract treatment application resulted in higher WUEi in TVU13998 plants, followed by sole inoculant 2, and then N plus seaweed extract. A similar trend was observed in the plants of IT97K-390-2, while sole inoculant 2, inoculant 2 plus seaweed extract and then sole N and N plus seaweed extract treatments promoted higher WUEi for genotype Songotra plants (Figure 3D).
The genotype x treatment interaction significantly influenced (p ≤ 0.001) the concentration of phosphorus in the cowpea leaves (Supplementary Table S3). As shown in Figure 4A, the level of P in leaves was increased by sole inoculation and inoculation plus seaweed extract treatments, when compared to the control treatments (Figure 4D). However, inoculation plus seaweed extract promoted much higher phosphorus accumulation across the three genotypes. For example, Inoculant 1 plus seaweed extract application promoted the highest P concentration in the plants of genotypes IT97K-390-2 (1.07%) and TVU13998 (1.19%), while inoculant 2 plus seaweed extract promoted the highest P accumulation for genotype Songotra (1.18%). This was followed by sole inoculant 1 and inoculant 2 plus seaweed extract for genotypes TVU13998E and IT97K-390-2, respectively, when compared to the remaining treatments (Figure 4A).
Figure 4 Interactive effect of genotype x treatment on the concentrations of Phosphorus (A), Potassium (B), Calcium (C), Magnesium (D), Sodium (E), and protein (F) in the leaves of three cowpea genotypes, planted under glasshouse conditions. Inoculant 1(Inoc 1) = B. yuanmingense strain CB756 (commercial inoculant), Inoculant 2 (Inoc 2) = Bradyrhizobium sp. strain TUTVUSA (closely related to B. daqingense), control = no inoculant and no kelpak, N= 5mM KNO3, control= no inoculation and no kelpak. vertical lines on bars represent S.E. Bars followed by dissimilar letters are significant at ***p ≤ 0.001.
The application of Inoculant 2 plus seaweed extract (2.37%) and the sole inoculant 2 (2.22%) treatments led to the highest K concentration in leaves (p ≤ 0.01) of IT97K-390-2, while sole N (2.30%) and N plus seaweed extract treatments (1.93%) promoted K accumulation in TVU13998 (Figure 4B). This was followed by the application of inoculant 1 plus seaweed extract (1.94%) on genotype IT97K-390-2, and the application of sole inoculant 2 (1.63%) on genotype TVU13998 (Figure 4B).
Ca concentration in the cowpea leaves varied among the genotypes. Generally, sole inoculation as well as the inoculation plus seaweed extract treatments significantly increased (p ≤ 0.001) the concentration of Ca in the leaves of all genotypes when compared to the controls (Figure 4C). Similarly, the sole inoculation as well as the inoculation plus seaweed extract treatments significantly increased (p ≤ 0.001) the concentration of Magnesium (Mg) in all the genotypes when compared to the control treatments (Figure 4D). Inoculating the cowpea genotypes with sole inoculant 2 or inoculant 2 plus seaweed extract generally improved magnesium concentration in the cowpea leaves of Songotra (0.44 and 0.65% respectively), followed by IT97K-390-2 plants (0.39 and 0.54% respectively). However, inoculant 1 with seaweed extract application resulted in higher Mg accumulation of 0.5% in the plants of genotype TVU13998 when compared to the rest of the treatments (Figure 4D). The concentration of Mg was similar (p>0.05) among all the control treatments irrespective of genotype (Figure 4D).
Sodium concentration varied significantly (p ≤ 0.001) among the three genotypes (Supplementary Table S3). The application of inoculant 1 plus seaweed extract (440 mg/kg) and inoculant 2 plus seaweed extract (377 mg/kg) markedly improved Na concentrations in the plants of TVU13998 when compared to the application of the other treatments on the same genotype (Figure 4E). This was followed by the application of inoculant 2 plus seaweed extract (350.3 mg/kg) and sole inoculant 2 (302.67 mg/kg) treatments to genotype Songotra plants and then the application of sole inoculant 1 (285.3 mg/kg) and inoculant 1 plus seaweed extract (291.6mg/kg) to genotype IT97K-390-2 (Figure 4E).
Protein concentration was significantly higher (p ≤ 0.01) in the plants receiving sole inoculation or inoculation plus seaweed extract treatments when compared to the control treatments (Figure 4F). The plants of genotype TVU13998 treated with sole inoculant 1 (31.14%) and those treated with inoculant 1 plus seaweed extract (32.30%) recorded the highest (p<0.05) protein concentration (Figure 4F). This was followed by inoculant 1 plus seaweed extract for genotype Songotra (26.5%) and inoculant 2 plus seaweed extract (26.42%) for IT97K-390-2. Sole inoculant 1 (25.6%) and inoculant 1 plus seaweed extract (23.1%) similarly improved protein accumulation in genotypes Songotra and IT97K-390-2, respectively (Figure 4F).
The interaction effect of genotype x treatment was significant for iron (Fe), Zinc (Zn) and Manganese (Mn) content of the three cowpea genotypes (Supplementary Table S4). The application of sole inoculant 1 or inoculant 2 treatments on genotype TVU13998 resulted in the highest Fe accumulation of 393.9 and 394.8 mg/kg Fe, respectively in the cowpea leaves (Figure 5A). This was followed by inoculant 1 plus seaweed extract treatment (288 mg/kg) of the same genotype, and sole inoculant 1 treatment for IT97K-390-2, recording 278.1 mg./kg Fe compared to the other treatments (Figure 5A).
Figure 5 Interactive effect of genotype x treatment on the concentrations of Iron (A). Zinc (B) and Manganese (C) in the leaves of three cowpea genotypes, planted under glasshouse conditions. Inoculant 1(Inoc 1) = B. yuanmingense strain CB756 (commercial inoculant), Inoculant 2 (Inoc 2) = Bradyrhizobium sp. strain TUTVUSA (closely related to B. daqingense), control = no inoculant and no kelpak, N= 5mM KNO3, control= no inoculation and no kelpak. Vertical lines on bars represent S.E. Bars followed by dissimilar letters are significant at ***p ≤ 0.001.
With Zn, the concentration was highest (p ≤ 0.001) in plants of IT97K-390-2 treated with sole inoculant 1 and those treated with inoculant 2 plus seaweed extract, followed by the plants of genotype Songotra the received inoculant 1 plus seaweed extract and then the plants of genotype IT97K-390-2 treated with sole inoculant 2 (Figure 5B). Generally, the control plants recorded lower levels of Zn when compared to their counterparts treated with sole inoculants or inoculant plus seaweed extract (Figure 5B).
The highest Mn concentration was recorded in plants of genotype Songotra that were treated with sole inoculant 2 (2182.3 mg/kg) and those treated with inoculant 2 plus seaweed extract (2221.8 mg/kg) (Figure 5C). These were followed by plants of TVU13998 treated with inoculant 2 plus seaweed extract (1796.4 mg/kg) and plants of IT97K-390-2 treated with inoculant 1 plus seaweed extract (1562.1 mg/kg). Among the control plots, the sole N and N plus seaweed extract treatments only increased (p ≤ 0.001) Mn concentration in genotype TVU13998 (Figure 5C).
The results obtained in this study reveal the stimulating effect of seaweed extract application and rhizobia inoculation on the growth, symbiotic nitrogen fixation, photosynthetic rates and nutritional attributes of three cowpea varieties. Generally, the combined application of seaweed extract and rhizobia triggered a varietal response, with variety Songotra and TVU13998 plants responding positively with increased nodulation, root as well as shoot biomass production. In fact, variety Songotra plants exhibited a marked response to the combined application of rhizobia inoculants and seaweed extract, resulting in three-fold root biomass accumulation when seaweed extract was applied with inoculant 1, and two-fold when applied with inoculant 2 when compared to their counterparts receiving sole inoculation (Figure 1). The positive response of these genotypes to the application of seaweed extract plus rhizobia inoculation as observed in this study can be attributed to the presence of the plant hormones auxin and cytokinin in the seaweed extract, as well as the N2-fixing efficiency of the rhizobia. Moreover, plant hormones such as auxin play a vital role in the nodulation process through signaling during lateral roots organogenesis (Mathesius, 2008). In earlier studies, auxin was found to positively regulate lateral root development (Bielach et al., 2012) and play a vital role in nodulation through cell cycle control (Kondorosi et al., 2005) while cytokinins play an important role in leaf development via plant signalling to produce more and larger leaves through cell division (Nadzieja et al., 2018). The high auxin (2.2 mg/l) to cytokinin (0.0062 mg/l) ratio in the seaweed extract used in this study stimulates vigorous root development, enhancing the plants own hormone production, leading to an overall good growth as observed in this study. In contrast, the combined application of seaweed extract and rhizobia to variety IT97K-390-2 did not markedly influence nodulation, root and shoot growth, suggesting possible inherent differences in the ability of cowpea genotypes to effectively utilize the growth promoting compounds in the seaweed extract. For example, the combined application of inoculant 1 and seaweed extract induced 110 nodules on variety IT97K-390-2 versus 117 recorded in their sole inoculated counterparts; moreover, both treatments induced similar root (0.9 g.plant-1), nodule (0.2 g.plant-1) as well as shoot biomass (4.7 g.plant-1). However, whether the test genotypes could respond differently to application method or variable concentrations of seaweed extract were not assessed in this study. In this study, the recommended method of drenching the soil was used and not the foliar spray recommendation. Perhaps, a foliar application on IT97K-390-2 plants could readily facilitate the absorption of cytokinin and other growth promoting compounds as observed by Ferreira and Lourens (2002) in canola and Arthur et al. (2003) in bell peppers. On the other hand, Crouch and Van Staden (1992) reported that, seaweed concentrate applied as drench significantly improved tomato seedling growth, while foliar spray had no effect on the tomato plants. The effect of seaweed extract in promoting plant growth have been reported previously to increase the yield of common bean (Kocira et al., 2018), enhance leaf number, root weight, bulb size and bulb weight of onion (Gupta et al., 2021), improve the growth and yield of bell peppers (Arthur et al., 2003), promote tomato seedling growth (Arthur et al., 2013) and rooting in rose cuttings (Traversari et al., 2022).
In this study, the rhizobial strains exhibited high N2 fixing abilities, and was evidenced by the low δ15N values of the test cowpeas receiving sole inoculation or inoculation treatments when compared to the high δ15N values recorded by the uninoculated control plants. The observed high symbiotic performance in the inoculated plants was likely enhanced by the low to non-existent N in the sand (Niner and Hirsch, 1998) used as the growth medium. The plants treated with inoculants plus seaweed extract exhibited symbiotic superiority and induced greater leaf N accumulation when compared to their counterparts receiving sole inoculation. Even though the interactive mechanism between the seaweed extract and rhizobia is still not clearly understood, it is evident that the combined growth stimulating effects of the active ingredients in seaweed (natural auxins (2.2 mg/l), cytokinins (0.0062 mg/l), macro and micro nutrients), coupled with the contribution of symbiotic N as well as other growth promoting abilities of the rhizobia (Rolland et al., 2002; Stirk et al., 2004) as well as the contribution of symbiotic N and other growth promoting abilities of the rhizobia such as P solubilization, enzyme synthesis and exopolysaccharide production (Tan et al., 2014) synergistically improved the growth of the plants. In South Africa, symbiotic N contribution by cowpea under field conditions is reported to range between 24 – 182 kgN.ha-1 (Makoi et al., 2009; Belane et al., 2011). The increase in symbiotic N likely increased Rubisco and chlorophyll production (Makoi et al., 2010), which resulted in efficient water use (measured as δ13C), high photosynthesis, increased shoot and root production and increased C accumulation, compared to the low fixing controls.
Macro and micromineral accumulation were greatly influenced by variety and inoculant type. In some instances, seaweed extract application with inoculation did not improve the mineral accumulation (example Ca for variety IT97K-390-2 and Songotra), and performed like the sole inoculated plants, while in other instances, its application improved mineral uptake (example P for all varieties and Na for TVU13998) than the sole inoculated plants. Enhanced root growth resulting from the auxins present in seaweed, coupled with the mineral solubilizing ability of rhizobia could have led to the efficient uptake and transport of nutrients such as P and Ca for improved growth (Rathore et al., 2009). Generally, applying seaweed extract plus rhizobia inoculation did not significantly influence protein concentration in the cowpea leaves when compared to sole inoculation, except in variety IT97K-390-2. In this study, the beneficial effects of sole rhizobia inoculation or co-inoculation with seaweed extract on mineral uptake by the three cowpea genotypes has been demonstrated, with food and nutritional security implications. The concentrations of macro and micronutrients observed in this study are either lower (Fe), similar (Mg and Zn) or higher (P, Mn and Ca) than those reported by Belane and Dakora (2011), while the protein content observed following sole inoculant 1 (31.14%) or co-inoculant 1 with seaweed extract (32.30%) are in line with previous reports (Belane and Dakora, 2011). Whereas the present study targeted the effect of inoculants and/or seaweed extract on the nutritional quality of edible cowpea leaves, it will be worthwhile to explore the corresponding effects of those treatments on the nutrient densities in the grains in future works. In this study, a synergistic effect between seaweed extract and rhizobia inoculation in improving cowpea growth was realized and was genotype dependent. The native strain proved highly efficient in growth and N2 fixation comparable to the commercial inoculant and can be further tested in the field to assess its suitability for inoculant development.
In conclusion, this study has shown the beneficial effects of sole inoculation as well as the beneficial interaction between rhizobia and seaweed extract in improving cowpea growth, symbiotic performance, and nutrient uptake. Based on the findings of this study, it has been demonstrated that cowpea production can be improved following either sole inoculation with compatible bacteria or supplemented with seaweed extract to improve production. However, because of the genotypic effect that was realized, more studies are recommended with a wide range of varieties. Also, different concentrations of seaweed extract with rhizobia inoculant should be tested to offer a much broader understanding of the interaction between the rhizobia and seaweed extract.
The raw data supporting the conclusions of this article will be made available by the authors, without undue reservation.
CG contributed to the conceptualization, data collection, analysis and manuscript writing. KM contributed to data collection and article review, MM contributed to photosynthesis data collection and manuscript review and TN contributed to photosynthesis data collection and analysis. All authors contributed to the article and approved the submitted version.
This work is based on the research supported wholly by the National Research Foundation of South Africa (Grant number 129658) and are duly acknowledged.
The University of South Africa and the Tshwane University of Technology are acknowledged.
The authors declare that the research was conducted in the absence of any commercial or financial relationships that could be construed as a potential conflict of interest.
All claims expressed in this article are solely those of the authors and do not necessarily represent those of their affiliated organizations, or those of the publisher, the editors and the reviewers. Any product that may be evaluated in this article, or claim that may be made by its manufacturer, is not guaranteed or endorsed by the publisher.
The Supplementary Material for this article can be found online at: https://www.frontiersin.org/articles/10.3389/fagro.2023.1138263/full#supplementary-material
Alemu M., Asfaw Z., Woldu Z., Fenta B. A., Medvecky B. (2016). Cowpea (Vigna unguiculata (L.) Walp.)(Fabaceae) landrace diversity in northern Ethiopia. Int. J. Biodiversity Conserv. 8 (11), 297–309. doi: 10.5897/IJBC2016.0946
Arthur G., Aremu A., Moyo M., Stirk W., van Staden J. (2013). Growth-promoting effects of a seaweed concentrate at various pH and water hardness conditions. South Afr. J. Sci. 109 (11), 1–6. doi: 10.1590/sajs.2013/20120013
Arthur G., Stirk W., Van Staden J., Scott P. (2003). Effect of a seaweed concentrate on the growth and yield of three varieties of capsicum annuum. South Afr. J. botany. 69 (2), 207–211. doi: 10.1016/S0254-6299(15)30348-3
Ayisi K. K., Nkgapele R. J., Dakora F. D. (2000). Nodule formation and function in six varieties of cowpea (Vigna unguiculata l. walp.) grown in a nitrogen-rich field soil in south Africa. Symbiosis. 28, 17–31.
Belane A. K., Asiwe J., Dakora F. D. (2011). Assessment of N2 fixation in 32 cowpea (Vigna unguiculata l. walp) genotypes grown in the field at taung in south Africa, using 15N natural abundance. Afr. J. Biotechnol. 10 (55), 11450–11458. doi: 10.5897/AJB11.674
Belane A. K., Dakora F. D. (2011). Levels of nutritionally-important trace elements and macronutrients in edible leaves and grain of 27 nodulated cowpea (Vigna unguiculata l. walp.) genotypes grown in the upper West region of Ghana. Food Chem. 125 (1), 99–105. doi: 10.1016/j.foodchem.2010.08.044
Bielach A., Duclercq J., Marhavý P., Benkova E. (2012). Genetic approach towards the identification of auxin–cytokinin crosstalk components involved in root development. Philos. Trans. R. Soc. B: Biol. Sci. 367 (1595), 1469–1478. doi: 10.1098/rstb.2011.0233
Boukar O., Belko N., Chamarthi S., Togola A., Batieno J., Owusu E., et al. (2019). Cowpea (Vigna unguiculata): Genetics, genomics and breeding. Plant Breed. 138 (4), 415–424. doi: 10.1111/pbr.12589
Broughton W., Dilworth M. (1971). Control of leghaemoglobin synthesis in snake beans. Biochem. J. 125, 1075–1080. doi: 10.1042/bj1251075
Craig H. (1957). Isotopic standards for carbon and oxygen and correction factors for mass-spectrometric analysis of carbon dioxide. Geochimica Cosmochimica Acta 12 (1), 133–149. doi: 10.1016/0016-7037(57)90024-8
Craigie J. S. (2011). Seaweed extract stimuli in plant science and agriculture. J. Appl. Phycol. 23 (3), 371–393. doi: 10.1007/s10811-010-9560-4
Crouch I. J., Van Staden J. (1992). Effect of seaweed concentrate on the establishment and yield of greenhouse tomato plants. J. Appl. Phycol. 4 (4), 291–296. doi: 10.1007/BF02185785
Das L., Bhaumik E., Raychaudhuri U., Chakraborty R. (2012). Role of nutraceuticals in human health. J. Food Sci. Technol. 49 (2), 173–183. doi: 10.1007/s13197-011-0269-4
FAO (2023). Available at: https://www.fao.org/faostat/en/#data/QCL/visualize (Accessed 5th February, 2023).
Farquhar G. D., Ehleringer J. R., Hubick K. T. (1989). Carbon isotope discrimination and photosynthesis. Annu. Rev. Plant Biol. 40 (1), 503–537. doi: 10.1146/annurev.pp.40.060189.002443
Ferreira M. I., Lourens A. F. (2002). The efficacy of liquid seaweed extract on the yield of canola plants. South Afr. J. Plant Soil 19 (3), 159–161. doi: 10.1080/02571862.2002.10634457
Gupta S., Stirk W. A., Plačková L., Kulkarni M. G., Doležal K., Van Staden J. (2021). Interactive effects of plant growth-promoting rhizobacteria and a seaweed extract on the growth and physiology of Allium cepa l. (onion). J. Plant Physiol. 262, 153–437. doi: 10.1016/j.jplph.2021.153437
Junk G., Svec H. (1958). The absolute abundance of the nitrogen isotopes in the atmosphere and compressed gas from various sources. Geochimica Cosmochimica Acta 14, 134–243. doi: 10.1016/0016-7037(58)90082-6
Kline R. B. (2015). Principles and practice of structural equation modeling. 4th Edition (New York: Guilford publications).
Kocira A., Świeca M., Kocira S., Złotek U., Jakubczyk A. (2018). Enhancement of yield, nutritional and nutraceutical properties of two common bean cultivars following the application of seaweed extract (Ecklonia maxima). Saudi J. Biol. Sci. 25 (3), 563–571. doi: 10.1016/j.sjbs.2016.01.039
Kondorosi A., Vinardell J. M., Uchiumi T., Mergaert P., Kondorosi E. (2005). “Cell Cycle and Symbiosis”, In: Biological Nitrogen Fixation, Sustainable Agriculture and the Environment. Eds. Wang Y. P., Lin M., Tian Z. X., Elmerich C., Newton W. E. Current Plant Science and Biotechnology in Agriculture, vol 41. Springer, Dordrecht. doi: 10.1007/1-4020-3570-5_35
Makoi J., Chimphango S., Dakora F. (2009). Effect of legume plant density and mixed culture on symbiotic N2 fixation in five cowpea (Vigna unguiculata l. walp.) genotypes in south Africa. Symbiosis 48 (1-3), 57–67. doi: 10.1007/BF03179985
Makoi J., Chimphango S., Dakora F. (2010). Photosynthesis, water-use efficiency and δ13C of five cowpea genotypes grown in mixed culture and at different densities with sorghum. Photosynthetica 48 (1), 143–155. doi: 10.1007/s11099-010-0019-2
Mariotti A., Germon G. C., Hubert P., Kaiser P., Létolle R., Tardieux A., et al. (1981). Experimental determination of nitrogen kinetic isotope fraction: some principle; illustrations for the denitrification and nitrification processes. Plant Soil 62, 413–430. doi: 10.1007/BF02374138
Masete F. M., Munjonji L., Ayisi K. K., Mopape-Mabapa M. P. (2022). Cowpea growth and nitrogen fixation performance under different mulch treatments. Agriculture 12 (8), 1144. doi: 10.3390/agriculture12081144
Mathesius U. (2008). Goldacre paper: Auxin: at the root of nodule development? Funct. Plant Biol. 35 (8), 651–668. doi: 10.1071/FP08177
Mohammed M., Jaiswal S. K., Dakora F. D. (2018). Distribution and correlation between phylogeny and functional traits of cowpea (Vigna unguiculata l. walp.)-nodulating microsymbionts from Ghana and south Africa. Sci. Rep. 8 (18006). doi: 10.1038/s41598-018-36324-0
Nadzieja M., Kelly S., Stougaard J., Reid D. (2018). Epidermal auxin biosynthesis facilitates rhizobial infection in lotus japonicus. Plant J. 95 (1), 101–111. doi: 10.1111/tpj.13934
Namatsheve T., Cardinael R., Corbeels M., Chikowo R. (2020). Productivity and biological N2-fixation in cereal-cowpea intercropping systems in sub-Saharan africa. A review. Agron. Sustain. Dev. 40 (4), 30. doi: 10.1007/s13593-020-00629-0
Ndakidemi P. A. (2006). Manipulating legume/cereal mixtures to optimize the above and below ground interactions in the traditional African cropping systems. Afr. J. Biotechnol. 5 (25), 2526-2533. Available at: http://www.academicjournals.org/AJB. Last accessed on 4th April, 2023.
Niner B., Hirsch A. (1998). How many Rhizobium genes, in addition to nod, niflfix, and exo, are needed for nodule development and function? Symbioses 24, 51–104. Available at: http://hdl.handle.net/10222/77554. Last accessed on 5th April, 2023.
Pule-Meulenberg F., Belane A. K., Krasova-Wade T., Dakora F. D. (2010). Symbiotic functioning and bradyrhizobial biodiversity of cowpea (Vigna unguiculata l. walp.) in Africa. BMC Microbiol. 10 (1), 89. doi: 10.1186/1471-2180-10-89
Rathore S. S., Chaudhary D. R., Boricha G. N., Ghosh A., Bhatt B. P., Zodape S. T., et al. (2009). Effect of seaweed extract on the growth, yield and nutrient uptake of soybean (Glycine max) under rainfed conditions. South Afr. J. Bot. 75 (2), 351–355. doi: 10.1016/j.sajb.2008.10.009
Rolland F., Moore B., Sheen J. (2002). Sugar sensing and signaling in plants. Plant Cell 14 (suppl_1), S185–S205. doi: 10.1105/tpc.010455
Singh B., Ehlers J., Sharma B., Freire Filho F. (2002). “Recent progress in cowpea breeding,” in Challenges and opportunities for enhancing sustainable cowpea production. Eds. Fatokun C. T., Singh S. A., Kormawa B. B. (Ibadan, Nigeria: International Institute of Tropical Agriculture), 22–40.
Singh S. K., Reddy R. K. (2011). Regulation of photosynthesis, fluorescence, stomatal conductance and water-use efficiency of cowpea (Vigna unguiculata [L.] walp.) under drought. J. Photochem. Photobiol. B: Biol. 105 (1), 40–50. doi: 10.1016/j.jphotobiol.2011.07.001
Stirk W. A., Arthur G. D., Lourens A. F., Novák O., Strnad M., van Staden J. (2004). Changes in cytokinin and auxin concentrations in seaweed concentrates when stored at an elevated temperature. J. Appl. Phycol. 16 (1), 31–39. doi: 10.1023/B:JAPH.0000019057.45363.f5
Tan K. Z., Radziah O., Halimi M. S., Khairuddin A. R., Habib S. H., Shamsuddin Z. H. (2014). Isolation and characterization of rhhizobia and plant growth -promoting rhizobacteria and thie effects on growth of rice seedlings. Am. J. Agric. Biol. Sci. 9 (3), 342-360. doi: 10.3844/ajabssp.2014.342.360
Traversari S., Cacini S., Nesi B. (2022). Seaweed extracts as substitutes of synthetic hormones for rooting promotion in rose cuttings. Horticulturae 8 (7), 561. doi: 10.3390/horticulturae8070561
Keywords: biostimulant, cowpea, N2 fixation, seaweed extract, photosynthesis, Rhizobium
Citation: Gyogluu Wardjomto C, Mohammed M, Ngmenzuma TY and Mohale KC (2023) Effect of rhizobia inoculation and seaweed extract (Ecklonia maxima) application on the growth, symbiotic performance and nutritional content of cowpea (Vigna unguiculata (L.) Walp.). Front. Agron. 5:1138263. doi: 10.3389/fagro.2023.1138263
Received: 05 January 2023; Accepted: 30 March 2023;
Published: 18 April 2023.
Edited by:
James T. Tambong, Agriculture and Agri-Food Canada (AAFC), CanadaReviewed by:
Aurelio Scavo, University of Catania, ItalyCopyright © 2023 Gyogluu Wardjomto, Mohammed, Ngmenzuma and Mohale. This is an open-access article distributed under the terms of the Creative Commons Attribution License (CC BY). The use, distribution or reproduction in other forums is permitted, provided the original author(s) and the copyright owner(s) are credited and that the original publication in this journal is cited, in accordance with accepted academic practice. No use, distribution or reproduction is permitted which does not comply with these terms.
*Correspondence: Cynthia Gyogluu Wardjomto, Y3ludGhpYS5neW9nbHV1QGdtYWlsLmNvbQ==
Disclaimer: All claims expressed in this article are solely those of the authors and do not necessarily represent those of their affiliated organizations, or those of the publisher, the editors and the reviewers. Any product that may be evaluated in this article or claim that may be made by its manufacturer is not guaranteed or endorsed by the publisher.
Research integrity at Frontiers
Learn more about the work of our research integrity team to safeguard the quality of each article we publish.