- 1International Maize and Wheat Improvement Center (CIMMYT), Texcoco, Mexico
- 2Faculty of Veterinary Medicine and Zootechnics, Autonomous University of Yucatan (UADY), Mérida, Yucatán, Mexico
- 3Unión Rural de Productores de Cuautempan y Tetela, Tetela, Puebla, Mexico
- 4Instituto Nacional de Investigaciones Forestales Agrícolas y Pecuarias (INIFAP), Oaxaca, Oaxaca, Mexico
- 5Universidad Autonoma Chapingo, Zimatlan, Oaxaca, Mexico
The milpa system is the basis of traditional agriculture in Mesoamerica. It is based on a polyculture of maize (Zea mays L.), bean (Phaseolus spp.) and squash (Cucurbita spp.) and is associated with a great diversity of crops and wild species. The milpa has great cultural and historical importance but can also be highly productive and provide sufficient and healthy diets for smallholder farmers. The milpa system is practiced less and less however, mainly due to socioeconomic changes, but also due to a lack of agronomic knowledge adapted to current conditions. To provide a starting point for new agronomic research, we reviewed the scientific literature to identify the current knowledge on milpa agronomy and determine research priorities to further improve the system. Given the wide diversity of conditions under which milpa is practiced, agronomic research is lacking, but indicates that improvements to the milpa can be made in all aspects of the milpa. 176 research articles on the milpa system were found in databases, of which 61 treated agronomic research. The main agronomic topics described in literature are crop variety, soil fertility management, weed management and productivity. Most research has focused only on maize and studies reporting on the associated crops are scarce. More research on all aspects of the milpa is needed to understand and improve the agronomy of the system under the changing conditions of modern agriculture. Reducing the workload associated with the milpa, as well as soil fertility and weed management can be identified as research priorities.
1 Introduction
The milpa is the basis of traditional agriculture in Mesoamerica. It is a production system based on the cultivation of the “Mesoamerican triad” of maize (Zea mays L.), bean (Phaseolus spp.) and squash (Cucurbita spp.), but also associated with a great diversity of crops and wild species (Linares and Bye, 2011) (Figures 1–3). The term “milpa” comes from the Nahuatl language (“milli,” sown field, and “pan,” on top of) and is the common term in Spanish and Mexico. The system has other names in other languages or regions (for example, in the USA it is known as “the three sisters”), but, for simplicity, we will refer to all forms as milpa. The milpa helps to safeguard the enormous biodiversity of the aforementioned crops and is a pillar of food security in traditional rural communities, in addition to having great cultural and historical importance. Milpa has been cultivated under a wide range of conditions. Depending on local climate, soil and culture, many different types of milpa exist, which differ in plant species cultivated, land preparation, use of fire, water regime and the length of the fallow cycle. Broadly, milpa systems can be divided into extensive rainfed systems with long fallow cycles, intensive rainfed systems with a short fallow and intensive rainfed or irrigated systems without fallow (Aguilar et al., 2003). Milpa area is decreasing rapidly due to the system’s low productivity and profitability, cultural rejection and the migration of youth from rural areas, and soil degradation due to reduced fallow periods (Isakson, 2009; Novotny et al., 2021; Orozco Ramírez and Astier, 2022).
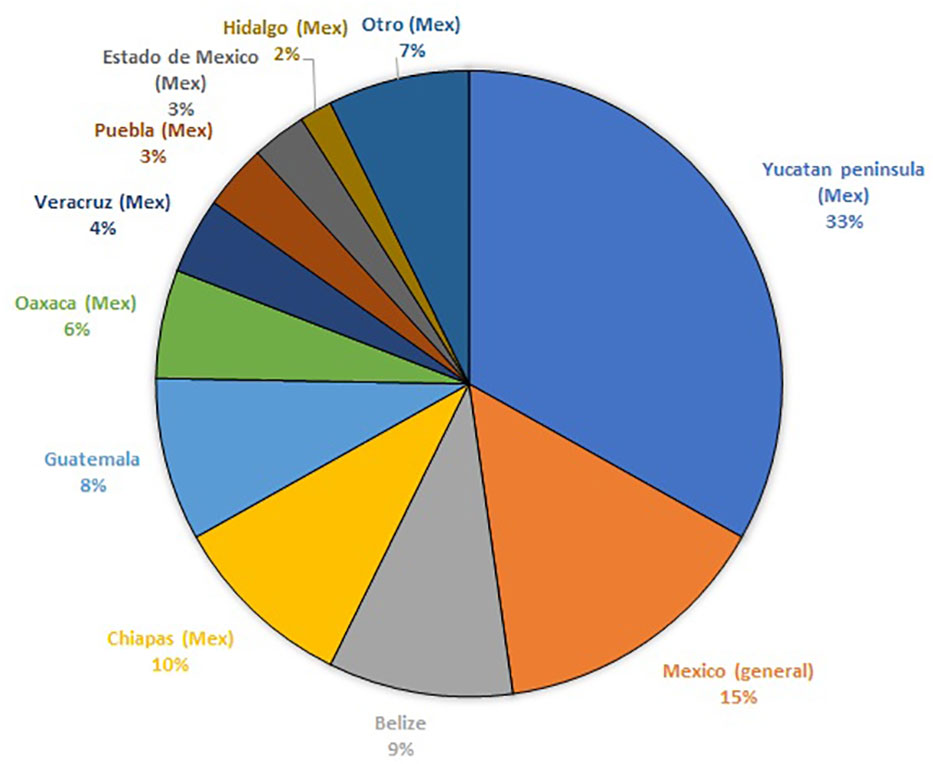
Figure 3 Distribution of scientific articles by location (country or state in Mexico, with states in Mexico indicated by Mex).
Crop associations generate agronomic benefits that increase system productivity (Vandermeer, 1989) and polyculture systems have higher productivity if the associated crops are complementary, meaning that (1) the crops occupy different niches and use different resources (light, water, nutrients, space), (2) the action of one species provides resources for the others, and/or (3) the crops modify the microclimate favorably for the other crops (Bybee-Finley and Ryan, 2018). Milpa crops are highly complementary in that 1) the plants receive light at different heights, maize being the tallest, while squash grows at ground level and beans climb the maize stalks; 2) beans fix nitrogen that the other plants can use (Tsai et al., 1993); 3) squash leaves shade the ground, reducing weeds and conserving soil moisture (Trujillo-Arriaga and Altieri, 1990; Pérez-Hernández et al., 2020); 4) the flowers of beans, squash, and other milpa plants attract beneficial insects, helping to reduce pest damage (Altieri et al., 1978); and 5) the greater volume of milpa crop roots and resulting soil channels, over those in monocultures, increases system water and nutrient availability (Zhang et al., 2014; Albino-Garduño et al., 2015).
Climate change, rural labor shortages, and social and economic changes are making milpa agriculture less attractive and reduce farmers’ food security (Haas, 2021; Novotny et al., 2021). Modern commercial agriculture, with its specialized and intensive cultivation, has gained ground over milpa systems, partly due to decades of support from public and private agronomic science that raises the yield and profitability of modern agriculture over milpa systems (Appendini, 2014). However, the practices and technologies developed to support commercial agriculture, for example, hybrid maize cropping, do not necessarily work well in traditional systems such as the milpa, which is generally sown by hand. In Chiapas, Mexico, a region where many farmers still grow local maize varieties using traditional, non-technified approaches, Wies et al. (2022) found that fertilization, insecticides or herbicides had little measurable effect on maize productivity.
Since milpa agriculture was developed and maintained over many generations and has provided livelihoods for many indigenous people throughout the Americas, studying the production methods of milpa farmers can provide learnings to increase productivity, food security and nutrition in other regions where maize is the basis of the diet of subsistence farmers, such as many regions in sub-Saharan Africa. The scientific community can also address agronomic challenges faced by milpa farmers and develop more effective extension programs for milpa systems, thus helping to preserve this productive, agroecologically interesting system and associated traditional knowledge. Related research should focus on the potential of milpa systems, offering solutions for agronomic constraints and socioeconomic and environmental challenges and, where possible, encouraging use of the milpa as a sustainable solution for subsistence or small-scale commercial production (Ebel et al., 2017).
This review summarizes current agronomic research on the milpa published in scientific peer reviewed articles, identifies research gaps, and offers recommendations for future research. The latter will focus on applied research to understand and improve milpa systems and processes (Porter, 2020), including practices that promote system productivity through increased yields and reduced workload, production costs, and dependence on external inputs, as well as those that conserve genetic diversity, reduce land and environmental degradation, or combinations of these factors. We do not describe the diversity of milpa systems or cultural or socioeconomic circumstances in rural households or communities, which are already extensively documented (Hernández, 1959; Pérez-Toro, 1981; García-Marín and May, 1992; Terán and y Rassmusen, 1994; Terán and Rasmussen, 2009). Nor do we cover agronomic management of polycultures in general, limiting our focus to milpa production systems.
2 Methodology
Using Scopus (www.scopus.com) and “milpa” as the search term, we conducted a search on 15 June 2021 for scientific articles reporting research on milpas. We found 283 articles published during 1955- 2021, 107 of which we discarded because they referred to the municipality of Milpa Alta in Mexico City, the prickly pear variety “Milpa Alta,” archaeological or historical studies, or issues unrelated to milpa cropping systems. Of the 176 remaining articles relating to milpa crop production systems, 44 addressed sociocultural aspects, 17 related to wild species biodiversity, 25 focused on the diversity of milpa crops, and 29 involved research on environmental issues, leaving only 61 studies (35%) focusing on agronomic issues.
To gather additional studies published in national journals and which might not be indexed in Scopus, we reviewed the repositories of SciELO (Scientific Electronic Library Online) and Redalyc (Network of Scientific Journals of América Latina and El Caribe, Spain and Portugal). Using the search term “milpa,” in SciELO we found 120 studies and, in Redalyc, 1,855 studies. Most of the studies from Redalyc were of social sciences or anthropology; only 164 addressed earth sciences or agrosciences and 124 covered biology. The Scopus results were thus similar to those from Scielo or Redalyc. To ensure that papers published in national journals were not excluded, we reviewed the websites of Agrociencia and Terra Latinoamericana (which are indexed in Scopus) and the Mexican Journal of Agricultural Sciences (which is not indexed in Scopus). The Agrociencia and Terra Latinoamericana reviews turned up no articles not indexed in Scopus. Our search on “milpa” in the Revista Mexicana de Ciencias Agrícolas (Mexican Journal of Agricultural Sciences) uncovered 17 studies, 10 of which were relevant to our review and appear in our qualitative summary. To find additional relevant studies in languages other than Spanish, we searched Scopus with the search terms: “maize” AND “bean” AND “squash”. The results overlapped with those for “milpa” but included nutrition studies. Search on “three sisters”, “maize” AND “polyculture” AND “Mexico”, and “Maize” AND “intercrop” AND “Mexico” resulted in 5 additional studies, leading to a total of 76 relevant studies on milpa agronomy (Supplementary Table 1). Finally, to expand the discussion, in addition to the database search outputs described above, we included relevant studies carried out for other, similar production systems, or those focused on one or two relevant crops that provided information elucidating milpa system agronomy. Based on the literature found, a qualitative review was made to summarize the published research on agronomic aspects of the milpa.
Most (61%) of the identified studies reported on studies in the Maya region, i.e. the Yucatan Peninsula, Chiapas, Guatemala and Belize, 25% reported on the milpa in other regions of Mexico and 14% were not related to a specific location (Figure 4). Especially in the Yucatan Peninsula the milpa has been studied most, with 34% of all studies, while we could only find one study about the milpa of the Purepecha and none about the milpa of the Tarahumara, even though these are also considerably large groups in Mexico. Similarly, a literature review of traditional agroforestry systems in Mexico indicated that most studies addressed the Maya systems and in the southern and southeastern states of Mexico, which may owe to the greater number of traditional systems in that region but also to the lack of research in other areas (Moreno-Calles et al., 2014) or possibly to fewer rural development projects linked to agricultural research in those regions.
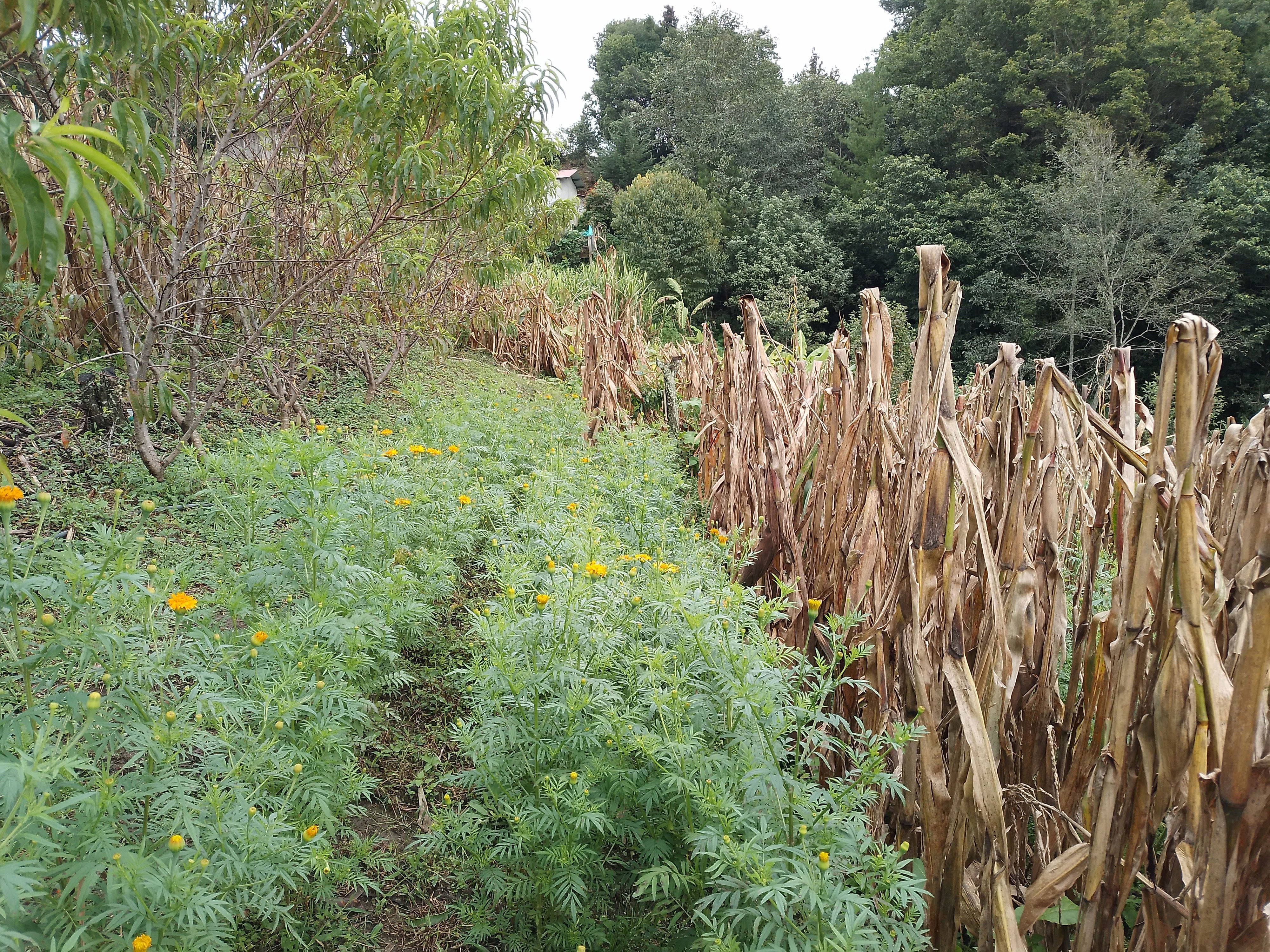
Figure 4 Strip cropping experiment in a Tzotzil milpa in Larrainzar, Chiapas. Beans (Phaseolus vulgaris) are followed by Marigolds (Tagetes erecta) and followed the next year by maize.
3 Current agronomic research on the milpa system
3.1 Soil preparation and planting
The milpa is most often grown on hillsides or superficial or stony soils, since flat lands are prioritized for commercial and mechanized cropping. Hillside soils are frequently degraded, due to water and wind erosion caused by tillage and overgrazing during fallow periods, as well as shortened fallow periods and generally more intensive cropping, due to dwindling land endowments for successive generations of offspring and land use change (Bolaños Gonzalez et al., 2016; SEMARNAT, 2016).
Burning is often used to clear milpa land for planting and, with good management and a sufficiently long fallow cycle (up to 40 years), does not necessarily degrade soils (Hernández Xolocotzi, 1988; Nigh and Diemont, 2013). But burning with reduced fallows can cause nutrient loss, especially of nitrogen, and more so when intense rainfall events wash away fertile ashes. Research on alternatives to burning has compared the yield of maize monoculture and residue burning with the yield of a maize-mucuna intercrop and residue retention in Yucatan, Mexico (Cuanalo and Uicab-Couoh, 2005). Non-burning increased maize yields but farmers’ lack of familiarity with mucuna grain as a food constrained adoption, so they switched to maize associated with lima beans (Phaseolus lunatus) without burning and also obtained higher maize yields.
Milpa farmers commonly sow maize with about a meter between seeded hills and with several seeds per hill, to compensate for expected low germination rates. In addition to causing competition among germinating plantlets in each hill, the large expanse of bare soil propitiates weed growth, especially in the absence of squash or other intercrops. Various crop placement modifications have been promoted to increase productivity and reduce weed incidence through shading and competition for nutrients, but the effects productivity and weed incidence have rarely been quantified. Studies on the effect of modified crop placements on associated crops are even rarer, although they could reduce the light, nutrients and water available for intercrops. In Mexico, Aguilar-Jiménez et al. (2011) and Caudillo Caudillo et al. (2006), reported higher maize yields and profitability with increased density under manual planting, but did not mention the effects on associated crops. Mt.Pleasant and Burt (2010) evaluated three maize densities with three densities of beans and squash in a trial in New York, USA. The highest maize yield came with the highest maize sowing density, but the highest bean yield was obtained under the lowest maize density, while squash yield was not clearly affected by the density of the other two crops. Delgado Martínez et al. (2015) reported that beans at the density of 2 bean seeds per hill yielded over 1 t/ha more green beans than maize and beans with 1 or 3 bean seeds per seeded hill. In three trials in Guatemala, reduced plant spacing increased maize yields at all sites but bean yields at only one (Eash et al., 2019). Farmers’ typical crop spacings and arrangements reflect the crops and varieties used, as well as local climates, soil conditions, and labor availability; proposals for alternative crop placement must be evaluated under local field conditions.
One substitute for mixed placement is sowing crops in alternating strips (Figure 5), which offers the benefits of multicropping and crop rotation as well as facilitating overall crop management (for example, with planting, fertilizing, weed control, or harvesting with low-power tractors) and allowing crops to be planted in their optimal plant spacing for maximum yields (Albino-Garduño et al., 2015). Molina-Anzures et al. (2016) found that placement in alternating strips gave higher system yields than associations.
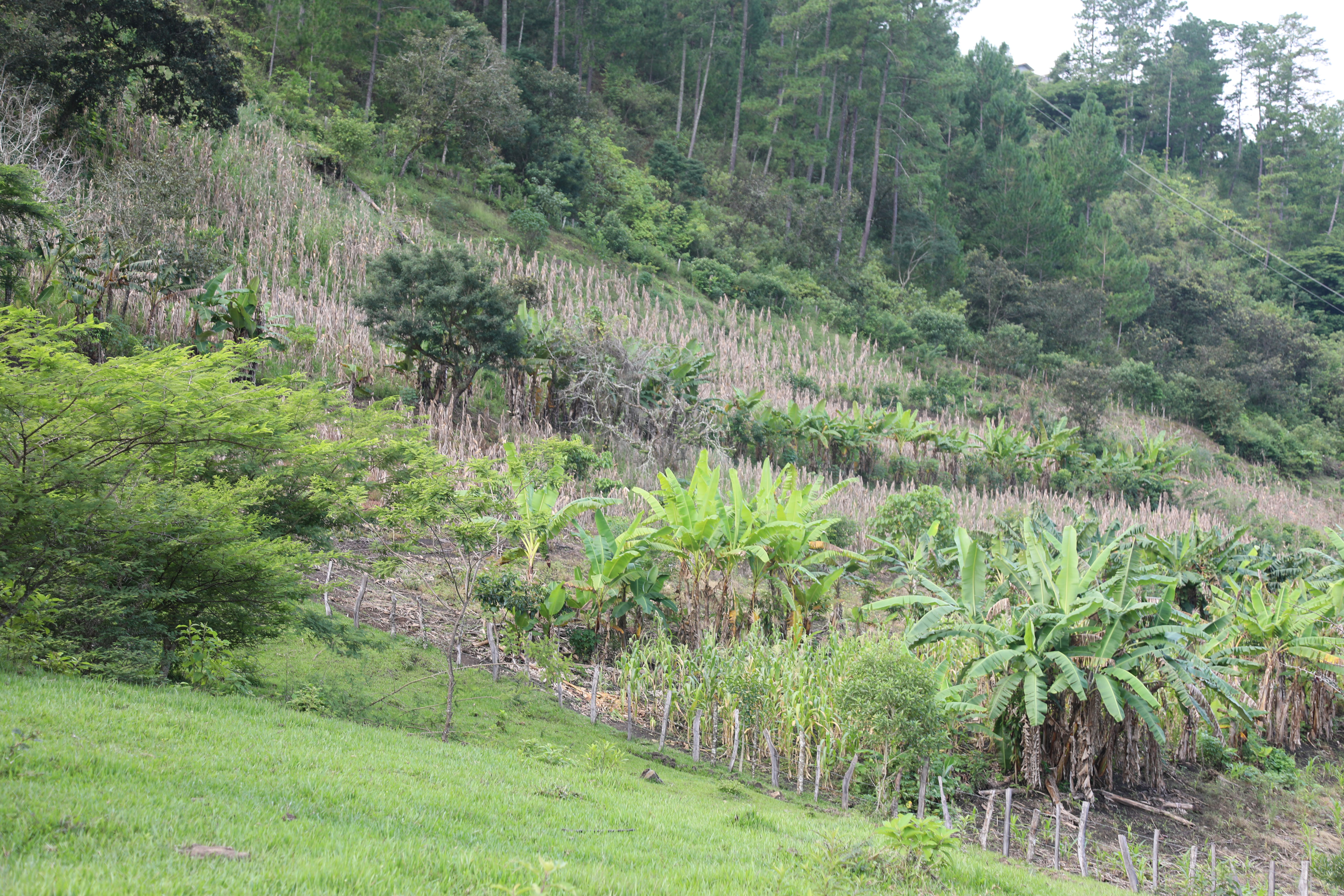
Figure 5 A MIAF (Milpa Intercalada con Arbolos Frutales, Milpa intercropped with fruit trees) agroforestry system with banana trees in Ocosingo, Chiapas, Mexico.
3.2 Varieties and species of crops
The diversity of milpa phenology, growth habits, and water and nutrient requirements makes the system resilient to climate change and a source of diverse, nutritious food throughout the year and even of occasional income from surpluses. Milpa farmers normally grow local varieties of the system’s crops that are adapted to native soils and climate and, above all, culinary preferences (Guzzon et al., 2021). Climate change is expected to reduce and shift growing areas for certain maize landraces and farmers will need to share or exchange seed of the varieties across regions to keep up productivity (Ureta et al., 2012).
Landrace varieties in situ are increasingly threatened by changing environmental conditions, the adoption of scientifically-bred varieties, and rural out-migration and the inexorable disappearance of local cultures and practices. To avoid the loss of these varieties with their large genetic diversity and adoption to local conditions, conservation can take place in farmers’ fields (in situ) and in genebanks (ex situ) (Guzzon et al., 2021). These approaches are complementary and necessary to protect biodiversity. Genebank seed samples capture and preserve a “snapshot” of diversity at a given moment; in situ conservation ensures that varieties adapt to new conditions. In situ, the presence of the wild and semiwild relatives of many crops in the same region, allows for the continued gene flow between domesticated crops in the milpa and these wild relatives. For example, in the coastal regions of Oaxaca, there is evidence of continued gene-flow between domesticated chile peppers (Capsicum annuum) and the wild C. frutescens and/or C. annuum var. glabriusculum and between wild and domesticated common bean (P. vulgaris) that are tolerated and managed in the milpa fields by the farmers (Zizumbo-Villarreal et al., 2005; Pérez-Martínez et al., 2022). In squash, gene flow between C. argyrosperma ssp. Argyrosperma gene and its wild relative C. argyrosperma ssp. Sororia has also been detected, though seed exchange between farmers is a much greater source of genetic variation in the domesticated crop (Montes-Hernández et al., 2005; Sánchez-de la Vega et al., 2018). In these studies, the genetic variation among landraces was as high as the variation in wild relatives, highlighting the importance of conserving these landraces in situ or ex situ to conserve the genetic resources (Zizumbo-Villarreal et al., 2005; Sánchez-de la Vega et al., 2018; Pérez-Martínez et al., 2022).
In a study of 66 families in Morelos who contributed maize seed to CIMMYT’s genebank in 1967, 50 years later (in 2017) only 13 families still grew the same varieties; the other families had stopped growing their materials due to changes in market demand, agricultural policies, urbanization and climate change (McLean-Rodríguez et al., 2019). The genomic diversity of in situ and ex situ collections was similar but the in situ samples had changed as a result of farmer selection during 1967-2017 and particularly, the market demand for maize with larger grains for specialty dishes (McLean-Rodríguez et al., 2021).
Martínez-Castillo et al. (2012), found that between 1979 and 2007 the original lima bean varities sown in northeastern Campeche on the Yucatan Peninsula, had been completely replaced by new varieties. In the same way, Fenzi et al. (2017) observed in Yaxcabá, Yucatán, that over 12 years the planting of the X-nuuk Nal variety, a long cycle type, was reduced by shorter cycle varieties, mainly Nal Xoy, because of the shortening of the rainy season. However, in Yaxcabá the range of maize diversity was maintained (Dyer et al., 2018). In contrast, the loss of maize genetic resources at the national level in Mexico over in the last century has been considerable; during 2002-07 alone the average number of varieties sown per farm fell from 1.43 to 1.22. The lack of diversity at the local level has reduced access to varieties adapted to local conditions (Dyer et al., 2014).
Maize diversity has been studied extensively, but the biodiversity of associated crops and wild plants harvested in the milpa system has been less studied. Lima bean for example – and especially the varieties that are planted infrequently – run the risk of biodiversity loss due to local extinctions, for example after a hurricane or drought (Martínez-Castillo et al., 2008). A study of climbing bean varieties intercropped with maize found that the most competitive, best-performing bean varieties in intercrops did not necessarily perform well in monoculture (Davis and Garcia, 1983). This suggests the need to study and conserve the biodiversity of milpa system crops in association, and particularly species compatibility and varietal availability considering farmers’ preferences and needs.
In addition, there is little breeding dedicated to milpa crops; there are no improved varieties of guide beans in Mexico, for example. The lack of improved varieties for milpas increasingly widens the yield gap between milpa and commercial farmers, inducing milpa farmers to change their varieties for apparently more productive ones but which are not necessarily suitable for sowing in association. The diversity of the milpa is the result of a long process and the system continues to evolve in response to changing environmental and socioeconomic conditions. Such changes may even require the introduction of new crops in milpa systems. Farmers in Yucatan reported being open to such introductions, if it suits their needs, but the performance of new varieties and species must be tested under local conditions before being widely promoted (Fils Pierre et al., 2021).
3.3 Fertility management
As parts of a complex polyculture, milpa crops each have different nutrient requirements at different times in the growing season. The improper use of fertilizers can reduce crop yields and even cause problems such as lodging. At the system level, poorly managed fertilizers can increase production costs and problems with unwanted plants, favor one crop over others, and cause pollution. Although fertilizer use has become common in milpas (Haas, 2021), there is little information regarding the correct use of fertilizers. For example, in the northern highlands of Puebla, Mexico, more than 80% of milpa farmers use synthetic fertilizers (Espidio-Balbuena et al., 2020), and this without knowing milpa soil or crop requirements but based on recommendations for monocultures or merely the local availability of fertilizers. Wies et al. (2022) report a null fertilization effect on native maize yield in Chiapas over a range of fertilization treatments applied by farmers. They used total fertilizer dose as the unit of measurement, however, which is not the best indicator. A database on 1,551 fields in Chiapas, Mexico sown with local maize varieties did show a positive relationship between kg N ha-1 applied and yield (Trevisan et al., 2022).
The various crops in the milpa have different fertilizer requirements and responses from those of maize monoculture. In six trials in Central America, fertilization based on recommendations from agronomists increased maize yields by 11% but had no effect on bean yields, compared to the local common practice of fertilization (Eash et al., 2019), while Delgado Martínez et al. (2015) reported that dosages of 150 kg N ha-1 resulted in higher yields of green beans interspersed in maize in the State of Mexico, Mexico, compared to 0 or 75 kg N ha-1 and Ruiz-González and Victorino-Ramírez (2014) observed an increase in maize yield with increasing fertilization, but not of bean yield in a maize-bean polyculture. As a polyculture, the milpa can potentially take better advantage of nutrients than a monoculture because the roots of the three main crops develop at different depths, so crops planted in the milpa system draw from a greater volume of soil than crops planted in monocultures (Zhang et al., 2014). However, although Zhang et al. (2014) report that maize had mainly surface roots and beans had more evenly distributed roots in the vertical profile, Albino-Garduño et al. (2015) found that beans rooted more superficially than maize. Specific interactions probably depend on soil conditions and nutrient availability. Zhang et al. (2014) reported that the depth of squash rooting depended primarily on phosphorus availability. Little is known about biological soil fertility in milpa systems, but nutrients can be transferred between plants species through the hyphae of arbuscular mycorrhizal fungi (Bethlenfalvay et al., 1991; Schütz et al., 2022), which can potentially contribute to milpa productivity. Fertilization with synthetic fertilizers can reduce mycorrhizal fungi abundance and diversity and unbalanced may thus negatively impact system productivity (Bhadalung et al., 2005). Efficient and economic milpa fertilization thus requires a study of fertilizer effects on all associated crops instead of focusing only on maize.
Fertilizer sources used in the milpa are often chosen based on local availability rather than what is appropriate based on soil conditions. In the mountainous regions of the state of Guerrero, Mexico, farmers commonly apply high amounts of synthetic fertilizers (186 kg N ha-1 and 46 kg ha-1 P2O5), however, at less than 1 t ha-1, the yields of maize, beans and squash are very low (Reyna-Ramírez et al., 2018). Under those conditions of high rainfall and steep slopes, the use of organic fertilizers was more economical, in addition to producing fewer losses from leaching of N in rainy years. The same study showed that organic fertilization increased the yields of associated crops more than synthetic fertilizer, suggesting a greater response of the milpa to organic sources or a better balance of nutrients (such as P). Similarly, Parsons et al. (2011) evaluated the use of manure to recover soil fertility in a milpa in Yucatan and reported that a low dose of manure was able to provide the nutrients necessary for grain production, while a high dose of manure sustained nutrient levels even with the removal of all stubble. Osorio Alcalá and Fabián (2019) evaluated a milpa under synthetic fertilizer, organic fertilization, and a mixture of both, finding that maize yielded more with synthetic fertilizer, while the squash yielded more with organic fertilizer, and bean yields were not affected by fertilization. Given the higher value of squash than maize, organic fertilization earned a higher return on investment. In trials in Oaxaca, several fertilization proposals were evaluated to increase productivity, but fertilization based on soil analysis did not increase yields sufficiently to justify the costs (Fonteyne et al., 2022b). Given the variability in the milpa’s response to organic fertilization and its high costs, including transport, more research is required to support farmers’ decisions on this subject.
Green manure crops offer an alternative to synthetic fertilizers. Traditionally, maize is intercropped with legumes such as common or lima beans, which provide additional food but are less effective nitrogen fixers than other legumes (Unkovich and Pate, 2000; Hardarson et al, 1993; Palmero et al., 2022). There is wide within-species variability in the amount of nitrogen that can be fixed by different varieties, with a range of 12 to 60% of the nitrogen in the grain and 18 to 57% of the nitrogen in the stem coming from nitrogen fixed by plant-associated microorganisms (Tsai et al., 1993). In addition, bean productivity in the milpa is relatively low, in the range of hundreds of kg ha-1, so the total nitrogen contributed is probably low compared to the needs of maize and other crops.
To fix more nitrogen, intercropping or rotating with other legume species has been investigated. Commonly, land planted with milpa is left fallow for several cycles after a crop cycle, to recover soil fertility. The use of green manures during this period, instead of letting wild vegetation take over the land, could increase nitrogen and carbon fixation and also reduce weed incidence (Ruiz-Vega and Loaeza-Ramírez, 2003; Uribe-Valle and Petit-Aldana, 2007). Fonteyne et al. (2022a) evaluated improved fallows in state of Oaxaca, Mexico, where, instead of letting the land fallow, snap bean (Phaseolus dumosus or Phaseolus coccineus) was grown, which increased the yield of the next maize crop and also produced a bean harvest. Alternatively, green manures can be sown as relay crops (before the harvest of the main crop) or in rotation during the winter, if moisture is available. In trials in the Central Valleys of Oaxaca, Mexico, the legumes Crotalaria juncea and Dolichus lablab produced more biomass than common bean or snap bean, thus offering a greater potential to improve the soil and provide nitrogen. Their economic value is lower than that of beans and they do not produce food, but they can be used for fodder (Ruiz-Vega and Loaeza-Ramírez, 2003; Beaupré et al., 2021). Similarly, higher maize yields have been observed after a rotation with mucuna in Las Tuxtlas, Veracruz (Ortiz-Ceballos et al., 2015) and in the Selva de Chiapas Region (Aguilar-Jiménez et al., 2011).
In a three-year evaluation in Yucatan of maize intercropped with mucuna or ib, there was no yield increase over that of monoculture maize (Castillo-Caamal et al., 2010). Castillo-Caamal and Caamal-Maldonado (2011) found that interspersing maize with mucuna at planting or 20 days after planting lowered maize yields, compared to monoculture or interspersing 40 or 60 days after planting. Farmers also have preferences regarding the species grown, due to characteristics such as grain or forage production and a morphology that facilitates management or ruminant feeding, such as the absence of thorns (Ayala Sánchez et al., 2007). These studies only addressed the effects of green manures on maize yield; their effects on associated crops or overall milpa yield have not been evaluated. The effects of green manures depend on diverse factors such as precipitation, rate of biomass degradation, duration of the fallow cycle, and the species grown, so their possible use requires further, in-depth evaluation and adapting them to local conditions.
Milpa agroforestry can also be done with legume trees, which require less work (only pruning) than fruit trees and which can help increase soil fertility, although they do not generate a direct income. Ayala Sánchez et al. (2007) evaluated 18 legume species for use as an improved fallow and concluded that Leucaena leucocephala, Caesalpinea yucataneneis, Piscidia piscipula, Acacia gaumeri, Pithecellobium albicans and Gliricidia sepium offered the greatest potential. There is a great diversity of agroforestry systems in Mexico, many of which have a milpa component (Moreno-Calles et al., 2013). Like the milpa system, the study of agroforestry systems has been limited in Mesoamerica, especially when considering the diversity of possible combinations of crops, fruit trees, and agroecologies, so much more research is needed to understand, adapt, and adopt those systems (Moreno-Calles et al., 2013).
3.4 Weed, pest and disease management
Crop protection is complicated in polyculture systems such as milpa, since synthetic pesticides used to protect one crop species can have negative effects on other crops, while mechanical management is more difficult because of the lower uniformity. The milpa also has aspects that help protect crops; for example, squash suppresses weeds through shading, while the presence of more predators reduces pest damage (Trujillo-Arriaga and Altieri, 1990).
Traditionally, the decision to initiate fallow in slash-and-burn milpa systems is made to address both falling fertility and increased weed growth (Hernández Xolocotzi, 1988; Cuanalo and Uicab-Couoh, 2005). Efficient weed management could reduce the need for fallowing or lengthen the time of continuous cultivation on the same land, if accompanied by improved soil fertility management. Weed control in the milpa is often done manually, which can entail high labor costs, or using animal-drawn implements. The use of herbicides is complicated in polycultures because it is difficult to use selective herbicides, so farmers depend on pre-emergence herbicides without residual effects and subsequently control weeds manually. Wies et al. (2022) reported lower maize yields with herbicide use in traditional systems in Chiapas, Mexico, due to improper use of herbicides. Given the complications of weed management in milpas, many growers opt for monocultures, which require less work, or conclude that the associated crops no longer perform as before, due to improper use of herbicides (Novotny et al., 2021). In sum, efficient and low-cost ways to manage weeds are a need for milpa systems.
Non-burning and the use of live or dead soil covers are alternative weed control options. Farmers in the Lacandon, a region in the state of Chiapas, plant balsa (Ochroma pyramidale), a fast-growing tree, after the milpa to suppress weeds during the fallow and speed the recovery of soil fertility (Nigh and Diemont, 2013). Ayala Sánchez et al. (2007) evaluated 18 tree species as improved fallow, and identified L. leacocephala as the most promising species for its rapid growth, weed reduction effect, wood production and beneficial effect on the subsequent maize crop. Similarly, Tzuc-Martínez et al. (2017) observed a reduction of about 50% in weed incidence in an agroforestry system with L. leucocephala, Guazuma ulmifolia, or Moringa oleifera sown in lines and pruned before maize planting. In the Costa Chica region of the state of Guerrero, intercropping maize with Canavalia spp. or Mucuna spp. reduced the incidence of weeds by 24 to 55%, respectively, compared to monoculture maize (Flores Sanchez, 2015). Similarly, in in the state of Yucatan, mucuna reduced weeds by 46%, when associated with maize in the third crop cycle (Castillo et al., 2010). Cuanalo and Uicab-Couoh (2005) compared milpas with burning, without burning, without burning with cover crops and the use of paraquat, and without burning with manual weed control. The highest yield and profits were found without burning and with cover crops, while manual control gave good yields but was not profitable due to the high labor demand. The use of live covers can reduce weeds in Yucatan by up to 68%, partly due to an allelopathic effect (Caamal-Maldonado et al., 2011). Parsons et al. (2009) compared manual control and herbicide use with an uncontrolled weed control in a milpa in Merida, Yucatan. In the first year, herbicide treatment and to a lesser extent manual control were effective in weed control. In the following year manual control was less effective than the use of herbicides.
Growth of wild plants in the milpa is not necessarily bad but depends on the species; many plant species found in milpas are edible and can represent an important part of the food production for the family (González-Amaro et al., 2009). In the Mixteca region of Oaxaca, weeds are controlled by two weedings to reduce the effect of competition during the critical stage of maize (45 days after planting); plants that emerge after that are used as fodder to feed backyard cattle (Personal observation). On the other hand, the incidence of weeds can also impact the incidence of pests. In a study in Chiapas, the incidence of pests in maize was double in maize with weeds, although yield was not affected due to the greater presence of predatory insects (Magallanes et al., 2003). In general, with low maize yields, weed control does not necessarily affect maize yield or is simply not economically profitable (Fonteyne et al., 2022a). The negative or beneficial effects of weeds depend on the production system, so weed control must be studied under different conditions.
Polycultures generally have fewer pest problems (Iverson et al., 2014), perhaps because they more easily host beneficial insects such as predators or parasitoids than monocultures. In maize and bean polycultures, Altieri et al. (1978) found 45% less Diabrotica balteata and 23% less incidence of fall armyworm (Spodoptera frugiperda) than in maize monocultures, as well as 26% less Empoasca kraemeri than in bean monocultures. In this study, planting times had an important effect on pest incidence, which was lower when crops were sown at different times than at the same time. Trujillo-Arriaga and Altieri (1990) reported more pest predation in the milpa than in a monoculture maize crop, and consequently less damage to crops. Many small farmers do not use insecticides in their milpa, since the return on investment would be low or due to lack of access to these products. When using insecticides, they tend to use obsolete, toxic, broad-spectrum insecticides (Bernardino-Hernández et al., 2019). The improper use of insecticides can reduce milpa productivity, since most of the associated crops depend on pollinators for their production, in addition to the fact that beekeeping is an important source of income for many milpa farmers (Ávila-Bello et al., 2018). A good understanding of the pest dynamics is necessary to avoid the inappropriate use of pesticides in milpa. Finally, apart from insects such as fall armyworm or Diabrotica spp., milpas also suffer from attacks by coatis, raccoons and birds which can cause great damage, but in turn can represent a source of bushmeat for milpa farmers (Lara Ponce et al., 2012; Naranjo et al., 2015; Falkowski et al., 2019).
3.5 Harvesting and storage
Manual harvesting is labor intensive, especially with climbing beans, which have to be harvested pod by pod. Smallholder farmers often harvest over a long period of time or wait for help, leaving crops in the field long after the optimal harvesting time and exposed to fungal infestation, pest damage, theft and adverse weather. Conversely, timely harvesting can likely reduce losses and thus increase yields, but we found no studies on the relationship of harvest date with yield or losses due to pests or fungi. Many milpa fields are on slopes or in terrain of difficult access, making mechanized harvesting difficult, but there are technological solutions that could reduce the workload in certain conditions, such as whole cob harvesters and/or small, two-wheeled-tractor-powered combine harvesters. The interspersed crops, especially the climbing bean, also complicate milpa harvesting, as does the need to harvest different products at different times: maize as cobs or grain, bean as pods or grain, and squash as flower, shoots, fruit or grain, to name a few.
Grain must generally be dried after harvesting to reach adequate moisture content for storage. Storage often uses traditional methods or grain may be stored in bags with pesticides such as aluminum phosphide and losses can be considerable. In tropical areas, up to 40% of maize or beans can be lost in 6 months due to inadequate storage, whereas hermetic storage containers can reduce losses to less than 5% (Odjo et al., 2020). Storage in hermetic containers also maintains maize seed viability, especially in lowlands where germination rate can drop by 56% during a six month storage period under non-hermetic conditions (Odjo et al., 2022b). Mobile shellers for field use directly after harvest could help store grains faster and thus protect them from the elements. Quality shelling to avoid grain damage and proper drying are important prior to storage, especially to avoid the growth of fungi such as Aspergillus spp. that can produce mycotoxins harmful to humans and farm animals (Odjo et al., 2022a). Cobs damaged by insects and birds are more prone to Aspergillus and other fungal infections. Improved harvest and postharvest technologies can thus reduce workloads, increase food availability, and improve food safety.
3.6 Agroforestry
Another proposal to increase soil fertility, diversify production, and avoid soil degradation is agroforestry or including productive trees in the milpa (Camas Gómez et al., 2012). The milpa is considered an agroforestry system by some authors, since during long fallows there may be recovery of secondary forest (Hernández Xolocotzi, 1988; Moreno-Calles et al., 2013; Falkowski et al., 2019). This applies mainly to milpa in Southern Mexico but is not necessarily a feature of all milpa systems. In Mexico, the concept of agroforestry related to the milpa has been promoted as the “Milpa Intercropped with Fruit Trees” (MIAF, for the abbreviation of the Spanish “Milpa Intercalada con Arboles Frutales”) system (Cadena-Iñiguez et al., 2018) (Figure 6). In the MIAF system, strips of milpa are interspersed with strips of fruit trees. A runoff filter, made of pruned branches and crop residues, is placed between the trees, if they are grown on sloping terrain, to gradually form terraces and thus help reduce erosion (Camas Gómez et al., 2012). Milpa in a MIAF arrangement can increase food security, since trees generally are harvested in another time of the year than maize, in addition to generating income, increasing system resilience, and reducing farmers’ risks (Santiago-Mejía et al., 2008). Molina-Anzures et al. (2016) evaluated maize, beans, and squash with and without interspersed fruit tree lines and found higher maize yields with trees, which they attributed to the microclimate generated by the trees. The MIAF system can be highly profitable, given the sale of the fruit, while retaining the milpa as a source of food for the farm household (Fonteyne et al., 2022b). However, the system can be complex, especially the pruning and fertilization of fruit trees, so farmers require technical assistance and access to information on system management (Torres Zambrano et al., 2008; Ruiz Mendoza et al., 2012).
3.7 Workload
The intense workload is one of the reasons farmers abandon the milpa. Novotny et al. (2021), for example, reported that in the Mixteca Alta region of Oaxaca, farmers prefer monocultures for their lower labor requirements. The challenges to mechanization of polycultures, especially for planting and harvesting, have already been mentioned. The literature cites workloads of 27 to 401 days per hectare per crop cycle for milpa systems, depending on the management intensity and the length of time a field has been under cultivation (Table 1). The higher values mentioned in the literature are the result of attempts to control weeds with high intensity manual control; as previously mentioned, the workload and cost are not justified by the small yield increase (Cuanalo and Uicab-Couoh, 2005; Fonteyne et al., 2022a; Fonteyne et al., 2022b). In the Mixteca Alta, Oaxaca, manual sowing may require more than 50 hours of work (Reyna-Ramirez et al., 2020). To reduce the workload of manual sowing for maize, López Gómez and Van Loon (2018) evaluated 6 manual tools, concluding that the traditional planting stick used by an experienced day laborer was more efficient than improved hand sowing tools, but study participants lacked experience with the new tools and perhaps could learn to use them as effectively or more so than the traditional tool. The use of improved hand planting tools adapted for the milpa could save time, since fertilization could be done simultaneously with sowing, which would also increase nutrient use efficiency. Some milpa farmers already mechanize part of their operations, such as in Becal, Yucatan, where farmers sow maize mechanically using tractors and sow beans and squash manually, following maize germination (Fils Pierre et al., 2021). Martínez Pérez (2021), describes participatory development of a seeder-fertilizer for milpa systems powered by a 2-wheel tractor that can sow at a rate of 13 h ha-1 and requires only a single operator. In preliminary investigations (pers. comm. Jesus Lopez-Gomez), it was noted that weed control with small motorized implements might be possible, with a weed removal efficiency greater than manual control and significantly reducing the man hours of work. This indicates that mechanization of small-scale agriculture can reduce the workload and increase productivity and adopting custom scale-appropriate tools and machinery could help sustain the milpa (Van Loon et al., 2020). However, understanding the agrotechnical or socioeconomic limitations for mechanization in milpas requires substantive research and validation.
3.8 Milpa system productivity
To understand the milpa it is important to consider total system productivity, rather than that of its component crops individually. If a milpa is evaluated on maize yields alone (typically from 1 to 3 t ha-1) it appears unproductive, compared to Mexico’s average reported maize yield of 3.8 t ha-1 (SIAP, 2019). But adding up the total production of all milpa products harvested speaks of a highly productive system that can provide food self-sufficiency and complete and healthy diets for farm families (Mt.Pleasant, 2016; Falkowski et al., 2019), altough this must be nuanced by the fact that households not always have enough land to sustain themselves completely from milpa (Lopez-Ridaura et al., 2021).
Measuring the total productivity of a milpa is complicated, given the number of products that can be obtained in polyculture systems and the various harvest windows. The productivity of monocultures and polycultures such as the milpa system can be compared using the Land Equivalent Ratio (LER), which represents the area that would be necessary to produce what is obtained from a polyculture on monoculture land area. If the LER is higher than 1, the polyculture productivity is greater than that of a monoculture; for example, an LER of 1.5 indicates that the productivity of 1 ha of polyculture equals that of 1.5 ha of monocultures (Vandermeer, 1989). We only found 8 studies reported in 6 articles in Mexico and the United States whose experimental design allowed the milpa LER to be determined (Table 2). The LERs in those studies ranged from 1.08 to 2.89, so the milpa in all studies was more productive than monocultures.
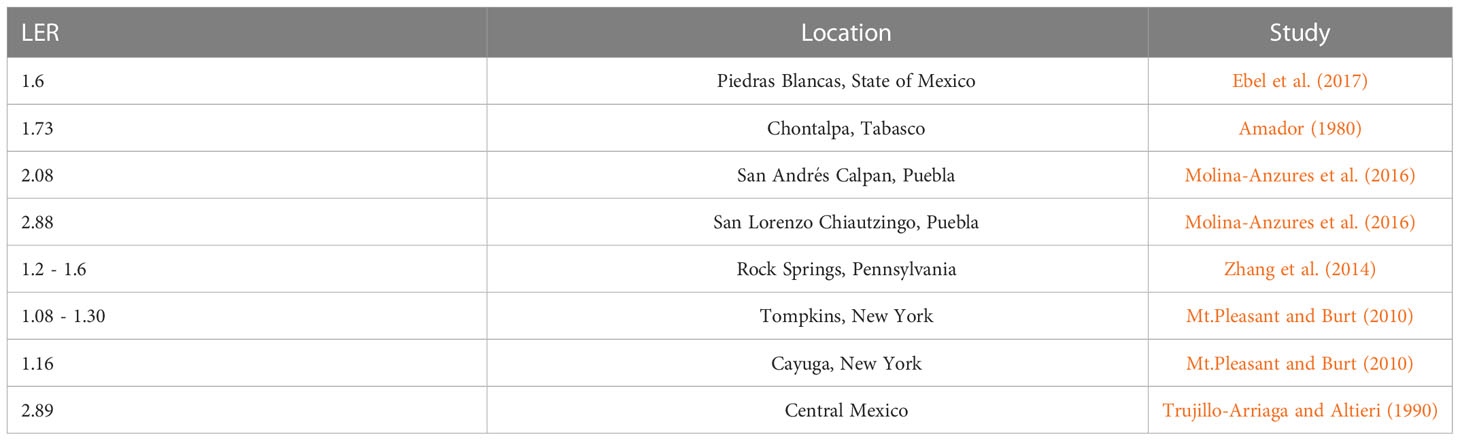
Table 2 Land Equivalent Ratio (LER) reported in studies on milpa as a maize, bean and squash association system, compared to monocultures of the same crops.
Milpa productivity can vary greatly and depends on many factors including the location, average precipitation, soil type, planting density, and varieties grown, among others. In general, in the reported experiments, monoculture and polyculture maize yields were similar, under milpa-type planting arrangements, whereas bean and squash yields tended to be lower. Davis and Garcia (1983) and Ruiz-González and Victorino-Ramírez (2014) found that the yield of maize interspersed with climbing beans was 15 to 30% lower than that of the crop grown as a monoculture, but similar to monoculture output if the beans were relay cropped, as is often the case in a milpa. The milpa’s broad agroecological diversity means that the range of system productivity for its various conditions and locations still needs to be determined.
A limitation of these studies is that yields in t ha-1 were always used to calculate LER, but the nutritional and economic values of squash or beans are much higher than that of maize, so the yield-based LER is not necessarily a good representation of overall milpa productivity or value. Sánchez Morales and Romero Arenas (2018) report that in Tlaxcala maize yields were slightly higher in monoculture, but profitability and total productivity were higher in the milpa. Fonteyne et al. (2022b) showed for an experiment in Oaxaca, Mexico that when economical values of bean and squash yields were taken into account growing milpa was profitable, while this was not necessarily the case when only considering maize. A meta-analysis of 939 observations of polycultures in 126 studies worldwide revealed that polycultures produced 38% more energy and 33% more income than monocultures (Vanloqueren and Baret, 2009). Similarly, to understand the value of the milpa vs monocultures requires an analysis of the economic differences and/or the concentrations of nutrients. Another consideration is that experimental yields are not necessarily equivalent to farmers’ results. To begin with, experimental management can be more intensive under experimental conditions than local conditions, as in the case of Ebel et al. (2017), who obtained high yields but under management practices that were costly and unrealistic. Milpa farmers also do not always grow only maize-bean-squash combinations but may include a wide variety of crops in different part of the field. Ávila-Bello et al. (2018) reported up to 10 crops grown in a milpa, in addition to coffee, firewood, and medicinal and ornamental plants, in the Sierra de Santa Martha, Veracruz. In addition, the crops are generally not sown uniformly throughout the plot, so LERs provide only indicative ranges of production, not absolute values for entire plots or homesteads.
3.9 Milpa to support balanced diets
Milpas are grown not to produce large surpluses for markets but rather to meet the nutritional requirements of the family. Therefore, another method to measure milpa productivity is to calculate how many people can be nourished from a milpa. In terms of nutrition, maize is the base crop and provides the calories in the milpa diet, while beans, squash and the other associated crops supplement protein, fatty acids and micronutrients. In the study by Falkowski et al. (2019), Lacandón farmer Adolfo Chankin recorded for 3 years all harvested produce and animals hunted on his milpa plot in Ocosingo, Chiapas. The results showed that an average Lacandon milpa (2.33 ha) is sufficient to ensure food security for 5.3 individuals per year with a healthy diet, containing all the necessary nutrients and almost all the micronutrients. In the same way, taking into account only the production of maize, beans and squash, Mt.Pleasant (2016) reported that the traditional milpa of the Haudenasaunee in New York produced more energy and more protein per hectare (12.3 x 106 kcal ha-1 and (349 kg ha-1, respectively), than the same crops in monoculture and may be enough to provide 13.4 people ha-1 year-1 with the necessary energy and 15.9 people ha-1 year-1 with the necessary protein. Additionally, the wild plants in the milpa can have a high nutritional value, which is also reflected in their economic value. For example, in a study in Tlaxcala, Mexico, the value of the edible wild plants that can be harvested in a maize field was greater than the production value of 1.5 t ha-1 of maize grain from the same land (González-Amaro et al., 2009).
In addition to plant production, milpa systems help support supplies of meat, both from wild animals around milpa plots and farm animals that feed on milpa surplus output. Wildlife, in the form of bushmeat, adds essential nutrients in the milpa-based diet (Falkowski et al., 2019) and milpas are often active hunting sites; in parts of the Yucatan Peninsula, milpas are sometimes established solely to attract small game (Naranjo et al., 2015).
In Guatemala mountain communities, a study found greater food security in households that grew milpas than in those that grew only monoculture maize (Lopez-Ridaura et al., 2019). Alternative systems such as maize-bean-potato, maize-potato and maize-common bean- broad bean also offered greater food security, while monoculture maize yielded the lowest results (Lopez-Ridaura et al., 2021). In a study in the Mixteca Alta region of Mexico, the milpa was found to provide all nutritional needs, with the exception of vitamin B12 (which is of animal origin), for at least 2 people ha-1 year-1, so cultivating a milpa was associated with greater household food security, over that of families who depended solely on monocultures (Novotny et al., 2021).
4 Discussion and recommendations
4.1 The limited availability of agronomic research on the milpa system
Based on the literature encountered, agronomic research on milpas has been scarce and sporadic, centered on maize and beans while leaving out other crops and, despite the great diversity of milpa systems across Mexico, largely concentrated in the Mayan zone. Even in the Mayan zone, research has focused on environmental or socio-economic, rather than agronomic topics (Rodríguez-Robayo et al., 2020). In this sense, it is important to study the great diversity that exists among milpa systems, since limiting research to Yucatan can generalize unrepresentative conclusions for all milpas (Dyer et al., 2018).
Not all field research by farmers, technicians, students and scientists has been published in scientific articles or books. There have been studies with direct local impact, but, since they have not been documented or represent publications that are difficult to access, have been left out of the search framework defined for this report. Moreno-Calles et al. (2014) reported a similar situation with only 91 of 737 studies found on agroforestry in Mexico published in scientific articles; the rest being other forms such as master’s, bachelor’s or doctoral theses. Greater effort is needed to publish and make accessible the knowledge contained in this literature.
The limited formal agronomic research on milpa systems has left smallholder farmers unattended, so they have adopted technologies developed for other production systems that do not necessarily function in traditional systems (Aguilar et al., 2003; Wies et al., 2022). The same applies to technical assistance programs that promote solutions that are not validated for local conditions and therefore have unknown effects on the productivity of the local cropping systems. Effective technical assistance must be based on field research so that the innovations proposed actually benefit farmers. Similarly, there are no official national data on milpa productivity, since official data are reported per crop. Omitting polyculture data from official statistics probably skews the perception of small-scale farmers’ productivity (Orozco Ramírez and Astier, 2022).
The lack of research on the milpa is characteristic of a general focus in agronomic research on commercial monoculture production systems, while more traditional and diversified agroecosystems in small- and medium-scale agroecologies are relatively ignored (Miles et al., 2017). For the reasons explained in this literary review, smallholder systems do not necessarily respond in the same way to agronomic or biophysical factors as the maize production systems of large farmers in high-latitude countries (Wies et al., 2022). The lack of research on diversified agroecosystems creates a vicious circle in which the lack of academic career and experience opportunities and paucity of literature and reference resources inhibit new researchers from entering the area. To have a sustainable agri-food system with a place for milpa, systemic research on this cropping system requires attention and support (Vanloqueren and Baret, 2009; Miles et al., 2017). Polycultures received renewed mention as a way to make agriculture more sustainable and productive (Bybee-Finley and Ryan, 2018; Martin-Guay et al., 2018), so research to improve agronomic management of milpas would benefit not only milpa farmers but could generate interesting applications for other production systems.
4.2 Conducting research on the milpa system is complicated
Suggesting improvements to the milpa can cause controversy, since the system has been practiced for generations and has great cultural significance. It should be considered that the milpa has evolved over time, incorporating new crops, tools, technologies and practices and adjusting to local conditions; Rodríguez Canto et al. (2016) described three current types of milpa: traditional, continuous, and mechanized, indicating a broad diversity of production systems based on the polyculture components of milpa. Moreover, the conditions in which milpas are grown are changing due to climate change, societal changes, population growth, and cultural changes, so the milpa has to co-evolve. Finally, milpas already incorporate use of herbicides, fertilizers and other inputs initially adapted for commercial agriculture (Mascorro De Loera et al., 2019; Fils Pierre et al., 2021; Wies et al., 2022); these practices then should be adapted to milpa conditions.
Apart from the pioneering research of Hernández-X. in the municipality of Yaxcabá in the 1980s (Hernández et al., 1995), only 12 (20%) of the studies related to agronomy in the Scopus collection (61 studies) address the effects of some intervention in the system or report on one trial with an experimental design; the remaining 80% (49 studies) only describe agronomic management aspects such as fertilization or cover crops as they are practices by farmers. Only Molina-Anzures et al. (2016) and Mt.Pleasant and Burt (2010) report field experiments with different treatments evaluated for maize, beans, and squash. There is thus a great need for research and it will require many scientists, farm advisors and farmers, as well as funding, to carry out. The studies need to be systemic, considering the associated crops and even wild plants.
Agronomic research using field trials is complicated, given the high number of interrelated system variables, including sowing densities, varieties grown, planting arrangements and dates; the list goes on. These factors and the need to adjust experimental designs to the milpa’s variable conditions makes this research more complex, laborious, and expensive than agronomic trials under monocultures. As an alternative to field trials, the milpa can be studied through farmer surveys, as was done by Lopez-Ridaura et al. (2019) and González-Esquivel et al. (2020). Such studies can identify current management practices related to best outcomes, with the advantage that the practices are already locally adapted and can thus directly inform useful recommendations. However, this approach cannot evaluate new practices. One field-based approach that has proven effective is mother-baby trials, where an improved practice is grown in or near farmers’ fields and compared with conventional practices (Snapp, 2002). Nonetheless, the great variability among milpas in a region and even within a field can make it difficult to define a conventional milpa or control treatment. To address some of these issues, farmers need to be involved in study designs, identifying critical factors for investigation and, crucially, those they would prefer not to change, for cultural or other reasons. Farmer input can also improve data collection to generate results and analyses that reflect actual field level conditions for milpas. Considering that milpa is practiced in a wide range of agroecological settings, studies will be needed for many regions of interest, since the results one region will not necessarily be relevant for conditions in another. Finally, as most milpas are grown under rainfed conditions with variable weather conditions, studies should be conducted over several successive years.
4.3 Suggested research priorities
Apart from understanding the sociocultural and biodiversity aspects of the milpa, to conserve this production system it is necessary to solve the agronomic challenges that it faces, and that requires more research and the development of optimized milpas (Ebel et al., 2017; Ebel et al., 2018). Through participatory research in situ and taking into account cultural preferences, the milpa could be improved, not only in terms of productivity but also profitability. For this, it is necessary to identify practices that support system diversity, reduce the workload, increase ecological sustainability, and foster the competitiveness of milpas with large-scale commercial production (Ebel et al., 2018).
Climate change will strongly impact Mesoamerica, requiring changes in varieties and crops in many regions (Ureta et al., 2016). The continuous evaluation of practices under the diverse conditions of milpas, along with crop improvement research, are needed, to generate mitigation and adaptation solutions for milpa farmers in affected areas.
Regarding the imperative of reduced workloads in milpas, one option is research and development on small-scale mechanization for more efficient soil preparation, fertilization, sowing, weed management, harvesting, and post-harvest management. This work should be accompanied by agronomic research on improved planting arrangements and sowing densities, timing of field operations, and precise use of inputs.
Few studies have addressed optimal fertilization for landrace maize, which reacts differently from modern hybrids to fertilizer (Alonso Ferro et al., 2007). In one interesting case, Van Deynze et al. (2018) reported nitrogen fixation by microorganisms associated with landrace maize in the Mixe region and other varieties demonstrating tolerance to acidic or alkaline soils; this suggests the need for more in-depth knowledge regarding the fertilizer needs of local maize varieties, to generate useful recommendations. There is also little knowledge about the fertilization needs of the other milpa crops or regarding soil fertility management of the milpa in its entirety (Reyna-Ramírez et al., 2018; Osorio Alcalá and Fabián, 2019). Reyna-Ramírez et al. (2018) underscored farmers’ lack of knowledge regarding appropriate levels of fertilization and illustrates the tendency of smallholders to apply high amounts of fertilizers with low efficiency, when they have access to these. More systemic research on milpa fertilization (sources, timing, methods, effects on all crops and on soil biology) is necessary.
We found no weed management studies for crops other than maize, and the practices evaluated could have different impacts on different crops. Also, there were no studies on alternatives other than cover crops, herbicide use, or manual weed control. An integrated approach to weed management should evaluate options such as mechanical control, use of milpa-compatible herbicides, rotations and residue covers, and improved planting arrangements, among others. Regarding pests, the milpa’s great agrobiodiversity and consequent lower incidence of pests should make pest management possible without the use of synthetic insecticides. To confirm this, there is a need to study milpa pests, define economic thresholds for damage, evaluate low-impact or biological insecticides, and eliminate use of high-impact and toxic insecticides. In each region, pests, predators, and parasitoids must be catalogued and farmers trained on the value of biodiversity and promoting management that favors system resilience. Solutions are also needed to manage macrofauna such as badgers or raccoons that can cause great losses.
Reported LERs suggest that milpas can be highly productive, coinciding with data from other regions showing that small farms were much more productive than large farms, if total production rather than the yield of a single crop was considered (Heltberg, 1998; FAO, 2016; Baudron et al., 2019). Diversified agricultural systems and polycultures are both more productive on a per-hectare basis, even if their individual crops yields are below those of crops on larger farms, and more resilient due their cropping diversity (Altieri, 1999; Altieri and Manuel, 2011). However, increasing milpa productivity does not necessarily have to aim for greater surpluses, but rather to improve the availability of nutritious food and system resilience in the face of environmental and socioeconomic constraints. Still, the milpa can offer food security to families only if it produces enough and through technological interventions that farmers can implement. Those in turn must be developed and evaluated through participatory research and adapted to local conditions to increase yields and reduce crop losses (González-Esquivel et al., 2020; Fonteyne et al., 2021; Saldivia Tejeda et al., 2020).
5 Conclusions
Agronomic research on the milpa has been surprisingly limited, considering the system’s historical, cultural and agronomic importance. There is little scientific knowledge regarding its agronomic processes or the environmental interactions of its components, and this has constrained the output of useful, science-based agronomic recommendations and support programs for milpa farmers. Changes in climate, public policies, and society require an “update” of milpa systems, with increased attention of the scientific community and research investors. This includes holistic, participative research on the agronomic management of milpas, accounting for the effects of varied interventions on all cultivated species.
Author contributions
SF, NV and JC conceptualized the study. SF reviewed the literature and wrote the first draft, JC, SL, JV, JE, LA, FM, SO, NV reviewed and edited the manuscript. All authors contributed to the article and approved the submitted version.
Funding
This study was supported by AgriLAC Resiliente: Resilient Agrifood Innovation Systems in Latin America and the Caribbean, a CGIAR Initiative. We would like to thank all funders who supported this research through their contributions to the CGIAR Trust Fund. Any opinions, findings, conclusions, or recommendations expressed are those of the authors and do not necessarily reflect the view of the donors.
Acknowledgments
We thank the many researchers, farm advisors, farmers and other stakeholders with whom we discussed the topic of the agronomy of the milpa system, which led to the writing of this document, and Mike Listman for editing the manuscript.
Conflict of interest
The authors declare that the research was conducted in the absence of any commercial or financial relationships that could be construed as a potential conflict of interest.
Publisher’s note
All claims expressed in this article are solely those of the authors and do not necessarily represent those of their affiliated organizations, or those of the publisher, the editors and the reviewers. Any product that may be evaluated in this article, or claim that may be made by its manufacturer, is not guaranteed or endorsed by the publisher.
Supplementary material
The Supplementary Material for this article can be found online at: https://www.frontiersin.org/articles/10.3389/fagro.2023.1115490/full#supplementary-material
References
Aguilar J., Illsley C., Marielle C. (2003). “Los Sistemas agrícolas de maíz y sus procesos técnicos,” in Sin maíz no hay país. Eds. Esteva G., Marielle C. (Mexico City: Consejo Nacional para la Cultura y las Artes), 83–122.
Aguilar-Jiménez C. E., Tolón-Becerra A., Lastra-bravo X. (2011). Integrated assessment of environmental, economic and social sustainability of maize in chiapas, méxico. Rev. FCA Uncuyo 43, 155–174.
Albino-Garduño R., Turrent-Fernández A., Cortés-Flores J. I., Livera-Muñoz M., Mendoza-Castillo M. C. (2015). Distribución de raices y de radiación solar en el dosel de maíz y frijol intercalados. Agrociencia 49, 513–531.
Alonso Ferro R., Brichette I., Evgenidis G., Karamaligkas C., XXXJ M.-G. (2007). Variability in European maize (Zea mays l.) landraces under high and low nitrogen inputs. Genet. Resour. Crop Evol. 54, 295–308. doi: 10.1007/s10722-005-4500-x
Altieri M. A. (1999). Applying agroecology to enhance the productivity of peasant farming systems in Latin America. Environ. Dev. Sustain. 1, 197–217. doi: 10.1023/A:1010078923050
Altieri M. A., Francis C. A., Van Schoonhoven A., Doll J. D. (1978). A review of insect prevalence in maize (Zea mays l.) and bean (Phaseolus vulgaris l.) polycultural systems. F. Crop Res. 1, 33–49. doi: 10.1016/0378-4290(78)90005-9
Altieri M. A., Manuel V. (2011). The agroecological revolution in Latin America: rescuing nature, ensuring food sovereignty and empowering peasants. J. Peasant Stud. 38, 587–612. doi: 10.1080/03066150.2011.582947
Amador M. (1980). Comportamiento de tres especies (Maiz, frijol, calabaza) en policultivos en la chontalpa, Tabasco, Mexico (H Cardenas, Tabasco, Mexico: Colegio Superior de Agricultural Tropical). Thesis.
Appendini K. (2014). Reconstructing the maize market in rural Mexico. J. Agrar. Change 14, 1–25. doi: 10.1111/joac.12013
Ávila-Bello C. H., Hernández-Romero Á.H., Mendoza-Briseño M. A., Vázquez-Luna D. (2018). Complex systems, agroecological matrices, and management of forest resources: An example of an application in Los tuxtlas, veracruz, Mexico. Sustainability 10. doi: 10.3390/su10103496
Ayala Sánchez A., Krishnamurthy L., Basulto Graniel J. A., Leos Rodríguez J. A. (2007). Native tree legumes for improvement of slash-and-Burn maize system in Yucatan, Mexico. Terra Latinoam. 25, 195–202.
Baudron F., Misiko M., Getnet B., Nazare R., Sariah J., Kaumbutho P. (2019). A farm-level assessment of labor and mechanization in Eastern and southern Africa. Agron. Sustain. Dev. 39. doi: 10.1007/s13593-019-0563-5
Beaupré A., Ruiz Vega J., Castañeda H. E., Benítez M., Mora Van Cauwelaert E., González González C. (2021). Pertinence of exotic and local green manures for sustainable maize polyculture in oaxaca, Mexico. Renew. Agric. Food Syst. 36, 138–149. doi: 10.1017/S1742170520000137
Bernardino-Hernández H. U., Mariaca-Méndez R., Nazar-Beutelspacher A., Álvarez-Solís J. D., Torres-Dosal A., Herrera-Portugal C. (2019). Conocimientos, conductas y síntomas de intoxicación aguda por plaguicidas entre productores de tres sistemas de producción agrícolas en los altos de chiapas, méxico. Rev. Int. Contam. Ambient. 35, 7–23. doi: 10.20937/RICA.2019.35.01.01
Bethlenfalvay G. J., Reyes-Solis M. G., Camel S. B., Ferrera-Cerrato R. (1991). Nutrient transfer between the root zones of soybean and maize plants connected by a common mycorrhizal mycelium. Physiologia Plantarum 82, 423–432. doi: 10.1111/j.1399-3054.1991.tb02928.x
Bhadalung N. N., Suwanarit A., Dell B., Nopamornbodi O, Thamchaipenet A, Rungchuang J. (2005). Effects of long-term NP-fertilization on abundance and diversity of arbuscular mycorrhizal fungi under a maize cropping system. Plant Soil 270, 371–382. doi: 10.1007/s11104-004-1829-4
Bolaños Gonzalez M. A., Paz Pellat F., Cruz Gaistardo C. O., Argumedo Espinoza J. A., Romero Benitez V. M., de la Cruz Cabrera J. C. (2016). Erosion map of Mexico soils and its possible implications for soil organic carbon pool. Terra Latinoam. 34, 271–288.
Bybee-Finley K., Ryan M. (2018). Advancing intercropping research and practices in industrialized agricultural landscapes. Agriculture 8, 80. doi: 10.3390/agriculture8060080
Caamal-Maldonado J. A., Jiménez-Osornio J. J., Torres-Barragán A., Anaya A. L. (2011). The use allelopathic legume cover and mulch species for weed control in cropping systems. Agron. J. 93, 27–36. doi: 10.2134/agronj2001.93127x
Cadena-Iñiguez P., Camas-Gómez R., López-Báez W., López-Gómez H. D. C., González-Cifuentes J. H. (2018). El MIAF, una alternativa viable para laderas en áreas marginadas del sureste de méxico: caso de estudio en chiapas. Rev. Mex. Cienc. Agrícolas 9, 1351–1361. doi: 10.29312/remexca.v9i7.1670
Camas Gómez R., Turrent Fernández A., Cortes Flores J. I., Livera Muñóz M., González Estrada A., Villar Sánchez B., et al. (2012). Erosión del suelo, escurrimiento y pérdida de nitrógeno y fósforo en laderas bajo diferentes sistemas de manejo en chiapas, méxico. Rev. Mex. Cienc. Agrícolas 3, 231–243. doi: 10.29312/remexca.v3i2.1459
Castillo-Caamal J. B., Caamal-Maldonado J. A. (2011). Efecto de la fecha de siembra del frijol terciopelo (Mucuna sp.) como cultivo de cobertera en el rendimiento de maíz. Trop. Subtrop. Agroecosystems 14, 101–108.
Castillo-Caamal J. B., Caamal-Maldonado J. A., Jiménez-Osornio J. J. M., Bautista-Zúñiga ,.F., Amaya-Castro M. J., Rodríguez-Carrillo ,. R. (2010). Evaluación de tres leguminosas como coberturas asociadas con maíz en el trópico subhúmedo. Agron. Mesoam. 21, 39–50.
Caudillo Caudillo S., Golicher J. D., van der Wal H., Vargas Domínguez S. (2006). Densidades de siembra, rendimientos y área requerida para maíz en la agricultura de roza, tumba y quema en la chinantla, méxico. Agrociencia 40, 449–460.
Cuanalo E., Uicab-Couoh R. (2005). Participatory research in no burn milpa. Terra Latinoam. 23, 587–597.
Davis J., Garcia S. (1983). Competitive ability and growth habit of indeterminate beans and maize for intercropping. F. Crop Res. 6, 58–75. doi: 10.1016/0378-4290(83)90048-5
Delgado Martínez R., Escalante Estrada J. A. S., Morales Rosales E. J., López Santillan J. A., Rocandio Rodríguez M. (2015). Snap bean (Phaseolus vulgaris l.) production and profitability intercropping with maize in function of density and nitrogen in template climate. Rev. la Fac. Cienc. Agrar. 47, 15–25.
Demchik M., Demchik E. (2014). Observations on the productivity and time required for a corn and squash polyculture, portage county Wisconsin. Plains Anthropol. 56, 71–81. doi: 10.1179/pan.2011.007
Dyer G. A., López-Feldman A., Yúnez-Naude A. (2018). Maize (Zea mays l.) management in yaxcaba, Yucatan, during the twentyfirst century’s first decade is consistent with an overall loss of landrace diversity in southeast Mexico. Genet. Resour. Crop Evol. 65, 29–54. doi: 10.1007/s10722-017-0507-3
Dyer G. A., López-Feldman A., Yúnez-Naude A., Taylor J. E. (2014). Genetic erosion in maize’s center of origin. Proc. Natl. Acad. Sci. 111, 14094–14099. doi: 10.1073/pnas.1407033111
Eash L., Fonte S. J., Sonder K., Honsdorf N., Schmidt A., Govaerts B., et al. (2019). Factors contributing to maize and bean yield gaps in central America vary with site and agroecological conditions. J. Agric. Sci. 157 (4), 1–18. doi: 10.1017/s0021859619000571
Ebel R., Méndez Aguilar M., de J., Putnam H. (2018). Milpa: One sister got climate-sick. the impact of climate change on traditional Maya farming systems. Int. J. Sociol. Agric. Food 24, 175–199. doi: 10.48416/ijsaf.v24i2.103
Ebel R., Pozas Cárdenas J. G., Soria Miranda F., Cruz González J. (2017). Manejo orgánico de la milpa: rendimiento de maíz, frijol y calabaza en monocultivo y policultivo. Terra Latinoam. 35, 149. doi: 10.28940/terra.v35i2.166
Espidio-Balbuena J., Navarro-Garza H., Flores-Sánchez D., Báez-Pérez A. (2020). Diversidad de sistemas de cultivo y transición agroecológica: estudio de caso en la sierra norte del estado de puebla, méxico. Agro Product. 13, 23–29.
Falkowski T. B., Chankin A., Diemont S. A. W., Pedian R. W. (2019). More than just corn and calories: a comprehensive assessment of the yield and nutritional content of a traditional lacandon Maya milpa. Food Secur. 389–404. doi: 10.1007/s12571-019-00901-6
FAO (2016). Save and grow in practice: maize, rice, wheat (Rome: Italy: Food and Agricultural Organization of the United Nations). doi: 10.1017/CBO9781107415324.004
Fenzi M., Jarvis D. I., Manuel L., Reyes A., Moreno L. L., Tuxill J. (2017). Longitudinal analysis of maize diversity in Yucatan, Mexico : influence of agro-ecological factors on landraces conservation and modern variety introduction. Plant Genet. Resour. Charact. Util. 15, 51–63. doi: 10.1017/S1479262115000374
Fils Pierre J., Latournerie-Moreno L., Garruña-Hernández R., Jacobsen K. L., Laboski C. A. M., Salazar-Barrientos L. D. L., et al. (2021). Farmer perceptions of adopting novel legumes in traditional maize-based farming systems in the Yucatan peninsula. Sustainability 13, 11503. doi: 10.3390/su132011503
Flores Sanchez D. (2015). Exploration of agro-ecological options for improving maize-based farming systems in Costa chica, Guerrero, Mexico (The Netherlands: Wagenigen University). Thesis.
Fonteyne S., Burgueño J., Albarrán Contreras B. A., Andrio Enríquez E., Castillo Villaseñor L., Enyanche Velázquez F., et al. (2021). Effects of conservation agriculture on physicochemical soil health in 20 maize-based trials in different agro-ecological regions across Mexico. L. Degrad. Dev. 32, 1–15. doi: 10.1002/ldr.3894
Fonteyne S., Leal Gonzalez A. J., Osorio Alcala L., Villa Alcántara J., Santos Rodriguez C., Núñez Penaloza O., et al. (2022a). Weed management and tillage effect on rainfed maize production in three agro-ecologies in Mexico. Weed Res. 62 (3), 224–239. doi: 10.1111/wre.12530
Fonteyne S., Silva Avendaño C., Ramos Sanchez A., Torres Zambrano J. P., García Dávila F., Pérez Martínez Z., et al. (2022b). Innovating traditional production systems through participatory conservation agriculture and agroforestry research. Front. Agron. 3. doi: 10.3389/fagro.2021.787507
García-Marín P., May P. F. (1992). “El Sistema milpero y sus recursos fitogenéticos,” in La modernización de la milpa en yucatán: Utopía o realidad. Eds. Zizumbo V. D., Rasmussen H., Arias R.L.M.y, Terán C. S., 97–134.
González-Amaro R. M., Martínez-Bernal A., Basurto-Peña F., Vibrans H. (2009). Crop and non-crop productivity in a traditional maize agroecosystem of the highland of Mexico. J. Ethnobiol. Ethnomed. 5, 3–11. doi: 10.1186/1746-4269-5-38
González-Esquivel C. E., Camacho-Moreno E., Larrondo-Posadas L., Sum-Rojas C., de León-Cifuentes W. E., Vital-Peralta E., et al. (2020). Sustainability of agroecological interventions in small scale farming systems in the Western highlands of Guatemala. Int. J. Agric. Sustain. 18, 285–299. doi: 10.1080/14735903.2020.1770152
Granados Sánchez D., Ríos López G. F., Trujillo Murcia E. (1999). La milpa en la zona maya de quintana roo. Rev. Geogr. Agric. 28, 57–72.
Guzzon F., Arandia Rios L. W., Caviedes Cepeda G. M., Céspedes Polo M., Chavez Cabrera A., Muriel Figueroa J., et al. (2021). Conservation and use of Latin American maize diversity: Pillar of nutrition security and cultural heritage of humanity. Agronomy 11, 172. doi: 10.3390/agronomy11010172
Haas E. G. U. (2021). Mitos y realidades sobre los sistemas de producción de maíz en chiapas y yucatán (Mexico: FAO).
Hardarson G., Bliss F. A., Cigales-Rivero M. R., Henson R. A., Kipe-Nolt J. A., Longeri L., et al. (1993). Genotypic variation in biological nitrogen fixation by common bean. Plant Soil 152 (1), 59–70. Available at: http://www.jstor.org/stable/42938917.
Heltberg R. (1998). Rural market imperfections and the farm size— productivity relationship: Evidence from Pakistan. World Dev. 26, 1807–1826. doi: 10.1016/S0305-750X(98)00084-9
Hernández X. E. (1959). “La agricultura,” in Los Recursos naturales del susreste y su aprovechamiento. instituto mexicano de recursos naturales renovables. Eds. Beltrán E., Méx A. C., Tomo D. F. II, 3–57.
Hernández X. E., Bello B. E., y Levy T. S. (1995). “Agricultura tradicional en méxico,” in La milpa en yucatán (un sistema de producción agrícola tradicional). Eds. Hernández X. E., Bello B. E. y Levy T. (Tomo: Colegio de Posgraduados), 15–34.
Isakson S. R. (2009). No hay ganancia en la milpa: The agrarian question, food sovereignty, and the on-farm conservation of agrobiodiversity in the Guatemalan highlands. J. Peasant Stud. 36, 725–759. doi: 10.1080/03066150903353876
Iverson A. L., Marín L. E., Ennis K. K., Gonthier D. J., Connor-Barrie B. T., Remfert J. L., et al. (2014). Do polycultures promote win-wins or trade-offs in agricultural ecosystem services? a meta-analysis. J. Appl. Ecol. 51, 1593–1602. doi: 10.1111/1365-2664.12334
Lara Ponce E., Caso Barrera L., Aliphat Fernández M. (2012). El Sistema milpa roza, tumba y quema de los mayas itzá de San andrés y San josé, peten Guatemala. Ra Ximhai 8, 71–92. doi: 10.35197/rx.08.02.e.2012.06.el
Linares E., Bye R. (2011). “¡La milpa no es solo maiz!,” in Haciendo milpa. Eds. Alvarez-Buylla Roces E., Carreon Garcia A., San Vicente Tello X. X. X. A. (Mexico: UNAM, Coyoacan), 9–12.
López Gómez J. A., Van Loon J. (2018). Agrotechnical evaluation of manual implements for corn planting. Rev. Mex. Cienc. Agric. 21, 4258–4269.
Lopez-Ridaura S., Barba-Escoto L., Reyna C., Hellin J., Gerard B., van Wijk M. (2019). Food security and agriculture in the Western highlands of Guatemala. Food Secur. 11, 817–833. doi: 10.1007/s12571-019-00940-z
Lopez-Ridaura S., Barba-Escoto L., Reyna-Ramirez C. A., Sum C., Palacios-Rojas N., Gerard B. (2021). Maize intercropping in the milpa system. diversity, extent and importance for nutritional security in the Western highlands of Guatemala. Sci. Rep. 11, 3696. doi: 10.1038/s41598-021-82784-2
Magallanes R., Francisco J., Mora V., Cisneros J. (2003). Effect of weeds on insect pests of maize and their natural enemies in southern Mexico. Int. J. Pest Manage. 49, 155–161. doi: 10.1080/0967087021000043111
Martínez-Castillo J., Camacho-Pérez L., Coello-Coello J., Andueza-Noh R. (2012). Wholesale replacement of lima bean (Phaseolus lunatus l.) landraces over the last 30 years in northeastern campeche, Mexico. Genet. Resour Crop Evol. 59, 191–204. doi: 10.1007/s10722-011-9675-8
Martínez-Castillo J., Colunga-GarcíaMarín P., Zizumbo-Villarreal D. (2008). Genetic erosion and in situ conservation of Lima bean (Phaseolus lunatus l.) landraces in its mesoamerican diversity center. Genet. Resour. Crop Evol. 55, 1065–1077. doi: 10.1007/s10722-008-9314-1
Martínez Pérez G. (2021). Diseño, construcción y evaluación de una sembradora fertilizadora para semilla grande con acople para un tractor de dos ruedas (Mexico: Universidad Autonoma Chapingo). Tesis de Ingeneria.
Martin-Guay M., Paquette A., Dupras J., Rivest D. (2018). The new green revolution: Sustainable intensification of agriculture by intercropping. Sci. Total Environ. 615, 767–772. doi: 10.1016/j.scitotenv.2017.10.024
Mascorro De Loera D. R., Ferguson B. G., Perales H., Charbonnier F. (2019). Herbicidas en la milpa: Estrategias de aplicación y su impacto sobre el consumo de arvenses. Ecosistemas y Recur. Agropecu. 6, 477–486. doi: 10.19136/era.a6n18.2076
McLean-Rodríguez F. D., Camacho-Villa T. C., Almekinders C. J. M., Pè M. E., Dell’Acqua M., Costich D. E. (2019). The abandonment of maize landraces over the last 50 years in morelos, Mexico: a tracing study using a multi-level perspective. Agric. Hum. Values 36, 651–668. doi: 10.1007/s10460-019-09932-3
McLean-Rodríguez F. D., Dell Acqua M., Elston D., Tania C., Pè M. E. (2021). Genetic diversity and selection signatures in maize landraces compared across 50 years of in situ and ex situ conservation. Heredity (Edinb). 126, 913–928. doi: 10.1038/s41437-021-00423-y
Miles A., Delonge M. S., Carlisle L., Miles A., Delonge M. S., Carlisle L. (2017). Agroecology and sustainable food systems triggering a positive research and policy feedback cycle to support a transition to agroecology and sustainable food systems. Agroecol. Sustain. Food Syst. 41, 855–879. doi: 10.1080/21683565.2017.1331179
Molina-Anzures M., Chaez-Servia J., Gil-Muñoz A., Loez P., Hernadez-Romero E., Ortiz-Torres E. (2016). Productive efficiencies in corn, bean and squash (Cucurbita pepo l.) associations, intercropped with rows of fruit trees. Phyton (B. Aires). 85, 36–50. doi: 10.32604/phyton.2016.85.036
Montes-Hernández S., Merrick L., Eguiarte L. (2005). Maintenance of squash (Cucurbita spp.) landrace diversity by farmers' activities in Mexico. Genet. Resour Crop Evol. 52, 697–707. doi: 10.1007/s10722-003-6018-4
Moreno-Calles A. I., Galicia-Luna V. J., Casas A., Toledo V. M., Vallejo-Ramos M., Santos-Fita D., et al. (2014). La etnoagroforestería: el estudio de los sistemas agroforestales tradicionales de méxico. Etnobiología 12, 1–16.
Moreno-Calles A. I., Toledo V. M., Casas A. (2013). Los Sistemas agroforestales tradicionales de méxico: Una aproximación biocultural. Bot. Sci. 91, 375. doi: 10.17129/botsci.419
Mt.Pleasant J. (2016). Food yields and nutrient analyses of the three sisters: A haudenosaunee cropping system. Ethnobiol. Lett. 7, 87–98. doi: 10.14237/ebl.7.1.2016.721
Mt.Pleasant J., Burt R. F. (2010). Estimating productivity of traditional Iroquoian cropping systems from field experiments and historical literature. J. Ethnobiol. 30, 52–79. doi: 10.2993/0278-0771-30.1.52
Naranjo E. J., Bello E., Estrada-lugo E. I., Nacional P. (2015). La milpa comedero-trampa como una estrategia de cacería tradicional maya. Estud. Cult. Maya 2574. doi: 10.1016/S0185-2574(13)71387-X
Nigh R., Diemont S. A. W. (2013). The Maya milpa: Fire and the legacy of living soil. Front. Ecol. Environ. 11. doi: 10.1890/120344
Novotny I. P., Tittonell P., Fuentes-Ponce M. H., López-Ridaura S., Rossing W. A. H. (2021). The importance of the traditional milpa in food security and nutritional self-sufficiency in the highlands of oaxaca, Mexico. PloS One 16, 1–21. doi: 10.1371/journal.pone.0246281
Odjo S., Alakonya A. E., Rosales-nolasco A., Molina A. L., Mu C. (2022a). Occurrence and postharvest strategies to help mitigate aflatoxins and fumonisins in maize and their co-exposure to consumers in Mexico and central America. Food Control 138. doi: 10.1016/j.foodcont.2022.108968
Odjo S., Burgueño J., Rivers A., Verhulst N. (2020). Hermetic storage technologies reduce maize pest damage in smallholder farming systems in Mexico. J. Stored Prod. Res. 88. doi: 10.1016/j.jspr.2020.101664
Odjo S., Palacios-rojas N., Burgueno J., Corrado M., Ortner T., Verhulst N. (2022b). Hermetic storage technologies preserve maize seed quality and minimize grain quality loss in smallholder farming systems in Mexico. J. Stored Prod. Res. 96. doi: 10.1016/j.jspr.2022.101954
Orozco Ramírez Q., Astier M. (2022). “La agricultura familiar y la milpa ante la política neoliberal en méxico,” in Integración global: el nuevo entorno de los territorios locales. Eds. Borrego A., Ramírez M., Astier M. (Morelia: Centro de Investigaciones en Geografía Ambiental), 1–17.
Ortiz-Ceballos A. I., Aguirre-Rivera J. R., Salgado-Garcia S., Ortiz-Ceballos G. (2015). Maize-velvet bean rotation in summer and winter milpas: A greener technology. Agron. J. 107, 330–336. doi: 10.2134/agronj14.0276
Osorio Alcalá L., Fabián R. (2019). Practices to improve the milpa system, in the mixteca of oaxaca. Reun. Nac. Investig. Agrícola Mem. 1, 312–315.
Palmero F., Fernandez J. A., Garcia F. O., Haro R. J., Vara Prasad P. V., Fernando Salvagiotti Ciampitti I. A. (2022). A quantitative review into the contributions of biological nitrogen fixation to agricultural systems by grain legumes. Eur. J. Agron. 136, 126514. doi: 10.1016/j.eja.2022.126514
Parsons D., Ketterings Q. M., Cherney J. H., Blake R. W., Ramírez-Aviles L., Nicholson C.F. (2011). Effects of weed control and manure application on nutrient fluxes in the shifting cultivation milpa system of yucatán. Arch. Agron. Soil Sci. 57, 273–292. doi: 10.1080/03650340903307236
Parsons D., Ramírez-Aviles L., Cherney J. H., Ketterings Q., Blake R., Nicholson C. (2009). Managing maize production in shifting cultivation milpa systems in yucatán, through weed control and manure application. Agric. Ecosyst. Environ. 133, 123–134. doi: 10.1016/j.agee.2009.05.011
Pérez-Hernández R. G., Cach-Pérez M. J., Aparacio-Fabre R., van der Wal H., Rodríguez-Robles U. (2020). Physiological and microclimatic consequences of variation in agricultural management of maize. Bot. Sci. 99, 132–148. doi: 10.17129/botsci.2640
Pérez-Martínez A. L., Eguiarte L. E., Mercer K. L., Martínez-Ainsworth N. E., McHale L., van der Knaap E., et al. (2022). Genetic diversity, gene flow, and differentiation among wild, semiwild, and landrace chile pepper (Capsicum annuum) populations in oaxaca, Mexico. Am. J. Bot. 109 (7), 1157–1176. doi: 10.1002/ajb2.16019
Pérez-Toro A. (1981). “La agricultura milpera de los mayas,” in La milpa entre los mayas de yucatán. Ed. Varguez Pasos L. A. (Mérida, Yucatán, México: Universidad de Yucatán), 1–28.
Porter J. R. (2020). Agronomy: The science of food production. Front. Agron. 614861. doi: 10.1142/9781848169845
Reyna-Ramirez C. A., Fuentes M., Rossing W. A. H., Lopez-Ridaura S. (2020). Characterization of mesoamerican smallholder farming systems. Agrociencia 54, 259–277.
Reyna-Ramírez C. A., Rodríguez-Sánchez L. M., Vela-Correa G., Etchevers-Barra J., Fuentes-Ponce M. (2018). Redesign of the traditional mesoamerican agroecosystem based on participative ecological intensification: Evaluation of the soil and efficiency of the system. Agric. Syst. 165, 177–186. doi: 10.1016/j.agsy.2018.06.013
Rodríguez Canto A., González Moctezuma P., Flores Torres J., Nava Montero R., Dzib Aguilar L. A., Pérez Pérez J. R., et al. (2016). Milpas de las comunidades mayas y dinámica de uso del suelo en la península de yucatán (Mérida Yucatán: Centro Regional Universitario Península de Yucatán de la Universidad Autónoma Chapingo), 436.
Rodríguez-Robayo K. J., Méndez-López M. E., Molina-Villegas A., Juárez L. (2020). What do we talk about when we talk about milpa? a conceptual approach to the significance, topics of research and impact of the mayan milpa system. J. Rural Stud. 77, 47–54. doi: 10.1016/j.jrurstud.2020.04.029
Ruiz-González R., Victorino-Ramírez L. (2014). Response of jamaica-bean-corn polyculture system to fertilization treatments in villaflores, chiapas, méxico. Agrociencia 49, 545–557.
Ruiz Mendoza A. D., Jiménez Sánchez L., Figueroa Rodríguez O. L., Morales Guerra M. (2012). Adopción del sistema milpa intercalada en árboles frutales por cinco municipios mixes del estado de oaxaca. Rev. Mex. Cienc. Agrícolas 3, 1605–1621. doi: 10.29312/remexca.v3i8.1324
Ruiz-Vega J., Loaeza-Ramírez G. (2003). Evaluation of green manure intercropped with rainfed maize in the central valleys of oaxaca, Mexico. Terra Latinoam 21, 409–415.
Saldivia Tejeda A., Albarrán Contreras B. A., Verhulst N., Fonteyne S. (2020). Avances en agricultura sustentable-resultados plataformas de investigación, hubs bajío e intermedio 2010-2019 (Texcoco, Mexico: CIMMYT).
Sánchez-de la Vega G., Castellanos-Morales G., Gámez N., Hernández-Rosales H. S., Vázquez-Lobo A., Aguirre-Planter E., et al. (2018). Genetic resources in the “Calabaza pipiana” squash (Cucurbita argyrosperma) in Mexico: Genetic diversity, genetic differentiation and distribution models. Front. Plant Sci. 9. doi: 10.3389/fpls.2018.00400
Sánchez Morales P., Romero Arenas O. (2018). Evaluación de la sustentabilidad del sistema milpa en el estado de tlaxcala, méxico. Rev. El Col. San Luis 15, 107–134. doi: 10.21696/rcsl8152018745
Santiago-Mejía E., Cortés-Flores J. I., Turrent-Fernández A., Hernández-Romero E., Jaen-Contreras D. (2008). Calidad del fruto del duraznero en el sistema milpa intercalada con arboles frutales en laderas. Agric. Técnica en México 34, 159–166.
Schütz L., Saharan K., Mäder P., Boller T., Mathimaran N. (2022). Rate of hyphal spread of arbuscular mycorrhizal fungi from pigeon pea to finger millet and their contribution to plant growth and nutrient uptake in experimental microcosms. App Soil Ecol. 169, 104–156. doi: 10.1016/j.apsoil.2021.104156
SEMARNAT (2016). “Informe de la situación del medio ambiente en méxico,” in Compendio de estadísticas ambientales. indicadores clave, de desempeño ambiental y de crecimiento Verde, Edición 2015(Mexico City, Mexico: SEMARNAT).
SIAP (2019). Panorama agroalimentario 2019 (Mexico City, Mexico: Servicio de Información Agroalimentaria y Pesquera). Ciudad de Mexico, Mexico.
Snapp S. (2002). “Quantifying farmer evaluation of technologies: The mother and baby trial design,” in Quantitative analysis of data from participatory methods in plant breeding. Eds. Bellon M. R., Reeves J. (Mexico, DF: CIMMYT).
Terán S., Rasmussen C. (2009). La milpa de Los mayas (Ciudad Universitaria, Mexico: Universidad Nacional Autónoma de México).
Torres Zambrano J. P., Cortés Flores J. I., Turrent Fernández A., Hernández Romero E., Muratalla Lua A. (2008). Fruit yield and number of scaffold branches in peach trees intercropped with milpa. Terra Latinoam. 26, 265–273.
Trevisan R. G., Martin N. F., Fonteyne S., Verhulst N., Dorado H. A., Jimenez D., et al. (2022). Multiyear maize management dataset collected in chiapas, Mexico. Data Br. 40, 107837. doi: 10.1016/j.dib.2022.107837
Trujillo-Arriaga J., Altieri M. A. (1990). A comparison of aphidophagous arthropods on maize polycultures and monocultures, in central Mexico. Agric. Ecosyst. Environ. 31, 337–349. doi: 10.1016/0167-8809(90)90232-3
Tsai S. M., Da Silva P. M., Cabezas W. L., Bonetti R. (1993). Variability in nitrogen fixation of common bean (Phaseolus vulgaris l.) intercropped with maize. Plant Soil 152, 93–101. doi: 10.1007/BF00016337
Tzuc-Martínez R., Casanova-Lugo F., Caamal-Maldonado A., Tun-Garrido J., González-Valdivia N., Cetzal-Ix W. (2017). Influence of woody species in the dynamics of weeds in agroforestry systems in Yucatan. Agrociencia 51, 315–328.
Unkovich M. J., Pate J. S. (2000). An appraisal of recent field measurements of symbiotic N2 fixation by annual legumes. Field Crops Res. 65, 211–228. doi: 10.1016/S0378-4290(99)00088-X
Ureta C., Martínez-Meyer E., González E. J., Álvarez-Buylla E. R. (2016). Finding potential high-yield areas for Mexican maize under current and climate change conditions. J. Agric. Sci. 154, 782–794. doi: 10.1017/S0021859615000842
Ureta C., Martínez-Meyer E., Perales H. R., Álvarez-Buylla E. R. (2012). Projecting the effects of climate change on the distribution of maize races and their wild relatives in Mexico. Glob. Change Biol. 18, 1073–1082. doi: 10.1111/j.1365-2486.2011.02607.x
Uribe-Valle G., Petit-Aldana J. (2007). Contribución de los barbechos cortos en la recuperación de la fertilidad del suelo en milpas del estado de yucatán, méxico revista. Rev. Chapingo. Ser. Cienc. For. y del Ambient. 13, 137–142.
Vandermeer J. (1989). The ecology of intercropping (Cambridge: Cambridge University Press), 237. doi: 10.1017/CBO978051162352
Van Deynze A., Zamora P., Delaux P.-M., Heitmann C., Jayaraman D., Rajasekar S., et al. (2018). Nitrogen fixation in a landrace of maize is supported by a mucilage-associated diazotrophic microbiota. PloS Biol. 16, e2006352. doi: 10.1371/journal.pbio.2006352
Van Loon J., Woltering L., Krupnik T. J., Baudron F., Boa M., Govaerts B. (2020). Scaling agricultural mechanization services in smallholder farming systems: Case studies from sub-Saharan Africa, south Asia, and Latin America. Agric. Syst. 180, 102792. doi: 10.1016/j.agsy.2020.102792
Vanloqueren G., Baret P. V. (2009). How agricultural research systems shape a technological regime that develops genetic engineering but locks out agroecological innovations. Res. Policy 38, 971–983. doi: 10.1016/j.respol.2009.02.008
Wies G., Navarrete-Segueda A., Ceccon E., Larsen J., Martinez-Ramos M. (2022). What drives management decisions and grain yield variability in mesoamerican maize cropping systems ? evidence from small-scale farmers in southern Mexico. Agric. Syst. 198. doi: 10.1016/j.agsy.2022.103370
Zhang C., Postma J. A., York L. M., Lynch J. P. (2014). Root foraging elicits niche complementarity-dependent yield advantage in the ancient ‘three sisters’ (maize/bean/squash) polyculture. Ann. Bot. 114, 1719–1733. doi: 10.1093/aob/mcu191
Keywords: three sisters, maize-bean-squash, Mexico, intercropping, Guatemala, Central America, corn, bean
Citation: Fonteyne S, Castillo Caamal JB, Lopez-Ridaura S, Van Loon J, Espidio Balbuena J, Osorio Alcalá L, Martínez Hernández F, Odjo S and Verhulst N (2023) Review of agronomic research on the milpa, the traditional polyculture system of Mesoamerica. Front. Agron. 5:1115490. doi: 10.3389/fagro.2023.1115490
Received: 04 December 2022; Accepted: 31 January 2023;
Published: 16 February 2023.
Edited by:
Augustine Obour, Kansas State University, United StatesReviewed by:
Marta Astier, National Autonomous University of Mexico, MexicoJibrin Mohammed Jibrin, Bayero University, Nigeria
Copyright © 2023 Fonteyne, Castillo Caamal, Lopez-Ridaura, Van Loon, Espidio Balbuena, Osorio Alcalá, Martínez Hernández, Odjo and Verhulst. This is an open-access article distributed under the terms of the Creative Commons Attribution License (CC BY). The use, distribution or reproduction in other forums is permitted, provided the original author(s) and the copyright owner(s) are credited and that the original publication in this journal is cited, in accordance with accepted academic practice. No use, distribution or reproduction is permitted which does not comply with these terms.
*Correspondence: Nele Verhulst, bi52ZXJodWxzdEBjZ2lhci5vcmc=