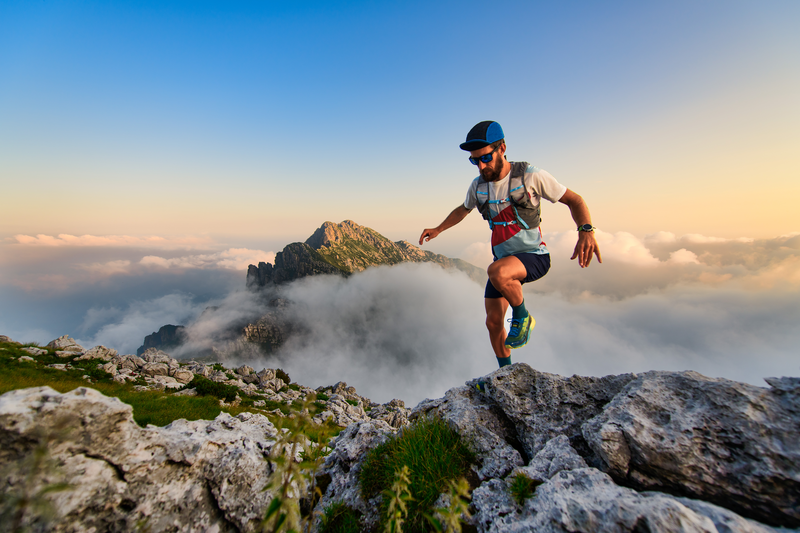
95% of researchers rate our articles as excellent or good
Learn more about the work of our research integrity team to safeguard the quality of each article we publish.
Find out more
ORIGINAL RESEARCH article
Front. Agron. , 03 August 2022
Sec. Climate-Smart Agronomy
Volume 4 - 2022 | https://doi.org/10.3389/fagro.2022.938331
The present investigation was carried out in a holistic mode to study the interactions among the integrated farming system (IFS) components and to develop and design a sustainable IFS model which is technologically sound, economically viable, environmentally benign, and socially acceptable for the middle Indo-Gangetic Plains. For efficient utilization of farm resources and to enhance the income per unit area of land, 10 IFS models have been developed at the farmers’ fields of Patna, Nalanda, and Vaishali districts of Bihar, India, during 2016–2021, involving components like crops, poultry, cattle, goat, mushroom farming, fishery, and duckery in different combinations. Out of 10 different integrations, three primary cropping systems prevailing in the state were undertaken and seven other components were integrated in a synergistic mode. Each system was allocated an area of 0.8 ha (2,000 m²), viz., (i) rice–wheat, (ii) rice–maize, (iii) rice–maize–moong (crop), (iv) crop + vegetable + goat, (v) crop + fish + goat, (vi) crop + fish + cattle, (vii) crop + fish + duck + goat, (viii) crop + fish + duck, (ix) crop + fish + mushroom, and (x) crop + fish + poultry. To sustain the productivity of soil health, inorganic fertilizers combined with organic wastes, obtained from various components of IFS, viz., recycled pond silts, poultry manure, duck manure, goat manure and cow dung as farmyard manures (FYMs), composted residues, and vermicompost were applied to crops grown under different IFS models. The nutrient content of manure increased manifolds after recycling as compost and vermicompost. Residue recycling revealed that integration of crops with fish and duck resulted in higher fish productivity and higher net returns (increased by USD13) in comparison to poultry dropping fed fishes. Due to the recycling of droppings, viz., poultry, duck, goat, cattle, and plant wastes, an additional quantity of 56.5 kg N, 39.6 kg P2O5, and 42.7 kg K2O was added to the soil during the study. Crop integrated with fish + duck + goat had the maximum rice grain equivalent yield (RGEY), net returns, and employment opportunity (467 man-days/year) from 0.8 ha of land, followed by crop + fish + poultry integration. The sustainability index (0.77) and net energy gain (95,770 MJ) were also found highest with crop + fish + duck+ goat integration indicating the optimum efficiency of all the farming system integrations for the region.
The global demand for farm products is expected to increase by 70% for food products and almost double for livestock products by 2050 as the population is expected to reach 9.5 billion (Goldstone, 2010). It poses a challenge to the researchers and policymakers to feed the burgeoning population where more than 80% of available lands have been covered under cultivation and other development activities (Young, 1999). Moreover, agricultural production is strongly affected by changes in climatic factors, i.e., rising temperatures, changing rainfall regimes, and variations in frequency and intensity of extreme climatic events such as floods and droughts (Brida et al., 2013). The estimated impacts of the climate change indicate that yield loss of grain could be up to 35% for rice, 20% for wheat, 50% for sorghum, 13% for barley, and 60% for maize (Porter et al., 2014). As a result of feed scarcity and climatic stresses, livestock production is also expected to be negatively affected (Kakamoukas et al., 2021). Both farmers and researchers need to find an efficient, sustainable, and affordable method in order to strengthen the resilience of agriculture to climate change and the synergistic effects of livestock on the system.
Indian agriculture has been shouldering the responsibility of providing food and nutrition to its teeming millions. The widespread occurrence of ill effects of green revolution technologies in intensively cultivated states like Punjab and Haryana is threatening the sustainability of agricultural systems and national food security goals. Besides, the gradual declining trend in the size of landholding poses a serious challenge to the sustainability and profitability of farming. The average size of landholding has declined from 2.28 ha in 1970–1971 to 1.16 ha during 2010–2011. If this trend continues, the average size of holding in India would be further reduced to 0.32 ha by 2030 (Agriculture Census, 2010-11). This situation in India necessitates an urgent integrated effort to address the emerging livelihood security issues. It is imperative to develop strategies and agricultural technologies that enable adequate income and employment opportunities, especially for small and marginal farmers who constitute more than 85% of the farming community in the country. Under such prevailing conditions, the integrated farming system (IFS) approach can be considered the most powerful tool for enhancing the profitability of smallholders. These IFS should be need-based and location-specific and are required to be well planned, designed, analyzed, and implemented for increasing the productivity, profitability, and sustainability of farms and farmers.
The IFS mode of food production supports a high standard of products with minimum environmental impact even in vulnerable climatic conditions using available resources accessible to farm households (Russelle et al., 2007). The IFS has revolutionized conventional farming of livestock, aquaculture, horticulture, agro-industry, and allied activities in some countries, including India (Ansari et al., 2013). The crop-livestock farming system is an age-old tradition in India which was developed 8 to 10 million years ago (Kumar et al., 2012) and is reported to be highly productive and environmentally sustainable (Allen et al., 2007). This productivity often reflects the improved soil structure and fertility, weed suppression, and disruption of pest cycles created by diverse crop rotations and livestock presence (Tracy and Zhang, 2008; Tracy and Davis, 2009). The IFS provides not only the means of production, such as fuel, fertilizer/manure, and feed, but also a healthy environment for ecological balance (Gill et al., 2010). However, these systems also need to be socially acceptable, economically viable, and eco-friendly. The integration of enterprises leads to greater dividends and support than a single enterprise-based farming production system, especially for small and resource-poor farming communities.
Most farmers are unlikely to change their practices radically within a short spell but seek ways to reduce their unit cost of production. Increasingly, they are prepared to adopt more rational approaches for nutrient and pesticide use and to exploit alternative measures that minimize risks. There are many ways to reduce production costs, either selectively or holistically, as part of strategies to improve farm income. Selectively focused component research provides options for reductions in agro-chemical use which could minimize the environmental contaminations. These include integrated nutrient management (INM) and pest and disease forecasting systems, reliant upon a basic understanding of population dynamics and other risk factors; reduced doses of herbicides, knowledge of the effects and interactions of weed growth, and weather and soil conditions on herbicide performance; and improved spray technology (Jordan et al., 1997).
Agricultural productivity evaluation using energy budgeting is important to make efficient use of available natural resources and to obtain the maximum sustainable economic yield. Energy consumption in agriculture has increased consistently over some time in the form of inputs such as fossil fuel, fertilizers, pesticides, herbicides, electricity, and machinery, causing environmental and human health problems. Erdal et al. (2007) concluded that efficient use of energy in agriculture would minimize environmental problems, reduce the destruction of natural resources, and promote sustainable agriculture as an economical production system. Therefore, such energy-efficient IFS models should be developed which could cope with environmental issues.
Based on the survey conducted in the Eastern Indo-Gangetic Plain (EIGP), different location-specific IFS models were designed and developed in three locations (three districts of Bihar) involving different combinations of crop/animals/fish/birds considering the needs and requirements of farm families, market opportunities, and environmental sustainability. These IFS models were implemented to explore the feasibility and constraints of adopting such systems of production by smallholders in a specific agro-climatic situation. This study aims to demonstrate and evaluate the most feasible enterprises of agriculture that encourage farming practices which are compatible with environmental changes and provide on-site training in principles and practices available for implementation, to appraise the skills of members of the farming community toward adoption and also to learn by seeing that such systems are technically and economically viable.
Field studies on the integration of different components of farming with crop production in IFS mode were carried out focusing on resource recycling within the system at three locations, viz., Patna, Nalanda, and Vaishali districts of Bihar, from 2016 to 2021 (6 years) involving crops, poultry, cattle, goat, mushroom farming, fish, and ducks in different combinations. Patna located at 25°37′N, 85°12′E and 53 m above mean sea level (a.s.l.) receives 1,101 mm average annual rainfall. Soils are of clay loam in texture and neutral in reaction. Nalanda is located at 25°26′N, 86°47′E and 67 m above mean sea level and receives 1,110 mm average annual rainfall with silty loam soil (texture) and pH of 6.94. Vaishali (25°51′N, 85°21′E and 46 m a.s.l) receives 1,135 mm average annual rainfall, and the texture of the soil is clay loam texture with 7.2 pH (Table 1). Daily weather parameters like maximum and minimum temperature and rainfall were measured at the Automatic Weather Station, Patna, and the Meteorological Observatory located in Nalanda and Vaishali districts (Table 1).
At the beginning of the experiment, initial soil samples were taken at each site from a 0–30-cm depth from different places, using a core sampler of 5-cm diameter. Samples (12 soil cores from each site) were mixed and bulked, and a representative sample was drawn for chemical analysis. These soil samples were pulverized using a wooden pestle–mortar and sieved through a 100-mesh nylon sieve. Processed samples were analyzed for OC (Walkley and Black method), available N (alkaline KMnO4 method), 0.5 M NaHCO3 extractable P (Olsen method), and 1 N NH4OAC-extractable K, following Page et al. (1982). The initial physicochemical properties of the soil of all three sites are presented in Table 1. Similarly, raw samples of plant materials like crop and orchard wastes, animal wastes, wastes of birds (poultry/duck), recycled products like vermicompost, FYM, goat and poultry manure, and pond silt were also analyzed for nitrogen (N), phosphorus (P), and potassium (K) content.
Ten farming system treatments were evaluated for which each has been allocated an area of 0.8 ha (2 acre). These systems were (i) rice (Oryza sativa)–wheat (Triticum aestivum), (ii) rice–maize (Zea mays), (iii) rice–maize–moong (Vigna radiata), (iv) crop (rice–maize–moong) + vegetable + goat [Cr+V+G], (v) crop + fish + goat [Cr+F+G], (vi) crop + fish + cattle [Cr+F+C], (vii) crop + fish + duck + goat [Cr+F+D+G], (viii) crop + fish + duck [Cr+F+D], (ix) crop + fish + mushroom [Cr+F+M], and (x) crop + fish + poultry [Cr+F+P]. In 2-acre land, an area of 0.1 ha was assigned for growing fodder crops to feed cattle (2 cows + 2 calves) and goat (20 female goat + 1 buck), 0.02 ha allocated for goat shed, 0.02 ha for cattle shed, 0.02 ha for mushroom shed, 0.01 ha for farmyard manure (FYM) and vermi-pits, and the remaining 0.12 ha was allotted to two fish ponds. The cropping area of each system varies depending upon the area occupied by different components/enterprises of that farming system (Table 2). In another 0.8 ha, the conventional cropping system as practiced by farmers (rice–wheat and rice–maize) and improved practices (rice–maize–moong) was taken up for comparison in an area of 2.4 ha.
Crops were grown with the recommended dose of fertilizer, i.e., 120: 60:40 kg NPK/ha each for rice, wheat, and maize and 20:60 kg/ha N and P for moong. To sustain the productivity of soil, inorganic fertilizers combined with organic wastes obtained from various components of IFS, viz., recycled pond silt, poultry manure, duck manure, goat manure and cow dung as FYM, composted residues (cereal residues), and vermicompost, were applied at 10 t/ha to the crops grown under different IFS modules. FYM, vermicompost, poultry manure, duck manure, goat manure, and poultry and ducks’ recycled silt were used once a year for raising crops. The rest of the nutrients were applied to each crop in the form of inorganic fertilizers as per recommendation. Water was applied as per the requirement of different systems. Optimum agronomic management was provided to all crops. All crops were irrigated on the basis of optimum irrigation water/cumulative pan evaporation (IW/CPE) ratio, and 5 cm water was applied for each irrigation. A system of two fodder sequences, viz., maize (Zea mays L.)–napier grass (Pennisetum purpureum)–berseem (Trifolium alexandrinum), and MP Chari (Sorghum vulgare)–cowpea (Vigna unguiculata)–oat fodder (Avena sativa) was followed in 0.5 ha of land.
One hundred broiler chickens and 35 + 5 (female + male) ducks sheltered over two fish ponds and a cattle unit located in a cattle shed were linked to supplement feed requirements of 500 fingerlings reared in each pond to assess the feasibility of rearing fish by using different manure types as feed. Vermi-pits and FYM pits were also linked with cattle and crops. Under the goat component, 20 female goats and one buck (20 + 1) of local breed (Black Bengal) were reared for meat purposes and available goat droppings were used as manure for crops. In 1 year, 60 kids were reared and sold at USD 2.9/kg live weight (kids were sold at the age of 10 months). Under the poultry component, 100 broiler chicks/batch (total of six batches/year) were maintained. Each batch was maintained for 35 days, and broilers attained an average weight of 1.5 kg, which were sold at USD 1.30/kg live weight. In the duck enterprise (Khaki Campbell), 30 females and five male ducks were integrated into a pond. Only duck droppings were fed to fishes, and no outside feed was provided. Healthy and hygienic conditions were maintained for animals and birds as per recommendation.
Under the fish component, mixed fish farming was practiced. In both ponds, freshwater fish rohu (Labeo rohita) as a column feeder (30%), catla (Catla catla) as surface feeders (30%), and mrigal (Cirrhinus mrigala) as bottom feeders (40%) were raised. At the end of the first year, adult fishes were harvested thrice at 20-day intervals. In all, 50% of poultry droppings/litters were used in the pond as fish feed and 50% were used as manure for crops. Water was drained out from the ponds, and the settled silt (5 t) was removed and applied as organic fertilizer to first crop in cropping sequences.
Year-round mushroom production was also included in the system by using a small hut made up of available local materials in an area of 0.02 ha. From March to September, paddy straw mushroom (Volvariella spp.) and milky mushroom (Calocybe indica), and from October to February Oyster mushroom (Pleurotus spp.), were raised by making bamboo racks in the shed. About 75%–80% humidity was maintained in the hut during the crop season by sprinkling water over the walls of the hut and bags.
To compare the productivity of different systems, the yield of each component was converted into rice grain equivalent yield (RGEY). The prices used for converting yield into RGEY and for computing the economics were the prevailing market price of different commodities (average price of 6 years; 2016–2021), viz., rice grain at USD 0.24/kg, wheat at USD 0.26/kg, moong at USD 0.60/kg, poultry at USD 1.32/kg, duck egg at USD 0.07/egg (USD 0.70/kg), goat meat at USD 3.5/kg, fish at USD 2.03/kg, and milk at USD 0.52/l. Observations were made on productivity in terms of rice–grain equivalent yield, economics, and employment for different farming systems, as well as conventional cropping systems.
The capital cost of establishing different IFS models varies with the enterprises involved in each system. Costs incurred are as follows: construction of pond of 0.06 ha at USD 762.0 per pond (USD 1,524 for two ponds); construction of FYM/vermi-pits (six pits) at USD 320; construction of thatched cattle shed at USD 430; thatched goat shed at USD 342; thatched poultry shed (100 birds capacity) at USD 327; thatched duck shed (35 ducks capacity) at USD 342; thatched mushroom shed (100-bag capacity) at USD 450; price of a cow at USD 400 (USD 800 for two cows); price of a goat at USD 10/goat (USD 210 for 21 goats); and price of duck at USD 2.0/duck (USD 70.0 for 35 ducks); in case of poultry, cost of 1-day-old chick was taken under recurring cost. In addition, USD 14.8 was provided as a subsidy under the Farming System Development Programme by the State Government of Bihar (Kumar et al., 2011). Total production cost was calculated by summing up the recurring cost of different components, land revenue, depreciation value, interest on working capital at 4%, and interest on fixed capital at 3% per annum (Giudice et al., 2016). Depreciation (D) per year was calculated using the straight-line method [D = (asset value – junk value)/life of asset] assuming that the system has a life of at least 10 years. The life of a duck was assumed as 3 years, and the life of other livestock and components was assumed as 10 years. In case the farmer opts for a loan, he has to repay the financial agency either monthly or annually for a maximum period of 5 years. The repayment will reduce his income for 5 years. Therefore, the value has been distributed over the system’s life and an annual repayment calculated.
IFS models were evaluated using a sustainability index described by Vittal et al. (2002). The sustainability index for any IFS model can be computed by using the formula:
where NR stands for net returns obtained under any model, SD stands for the standard deviation of the net returns of all models, and MNR stands for maximum net returns attained under any model.
A suitable and viable IFS model could be identified for their existence based on net returns, sustainability index, employment generation, and improvement in soil fertility attained over a period of time. For comparing the efficiency of different integrations, the link relative index (LRI) was also calculated by using the formula
where NI0 means net income of improved/farmer’s practice on 1.0 ha (base 2021 prices) and NI1 means net income of the system (base 2016 prices).
Further, energy budgeting and different energy indices for individual components and all other IFS models were calculated by using formulae as mentioned in Table 3.
Productivity of individual components, as well as different combinations (crop/fish/duck/goat/cattle/poultry), was calculated as rice grain equivalent yield (RGEY) for making better comparisons. Among the three cropping systems, rice–maize–moong recorded the highest average mean yield of 11.5 t/ha over conventional rice–wheat and rice–maize systems (8.21 and 9.12 t/ha), respectively. Cr+F+C integration had the maximum RGEY (20.2 t/ha) at all experimental sites (Patna, Nalanda, and Vaishali) followed by Cr+F+D+G goat integration (19.41 t/ha) as depicted in Table 4. While considering individual units, higher productivity of 7.5 t/2,000 m2 area was obtained from a cattle unit followed by goat unit (5.8 t/2,000 m2) over crop components, i.e., rice–maize–moong (8.21 t/8,000 m2). In addition to this cattle, goat, duck, and poultry units also produced 9.0 t of FYM, 2.8 t of goat manure, 1.4 t of duck droppings, and 2.6 t of poultry droppings/annum, respectively, which were recycled into the system. The feasibility of rearing fishes by using poultry and duck droppings as feed for fishes was also assessed, and average fish yields of 1.82 t and 1.70 t per 0.06 ha area were recorded with fish fed upon poultry and duck droppings, respectively (Table 5).
Table 4 Average productivity (RGEY) t/ha and economics of different combinations at three places in developed IFS models (mean data of 6 years, 2016–2021).
Table 5 Average productivity (t) and economics of individual components (USD) in developed IFS (8,000 m2) (mean value of 6 years, 2016–2021).
The study on recycling of residues revealed that the crop grown with integrated nutrient application from vermicompost, poultry, and goat manure resulted in higher productivity of 9.68, 9.32, and 9.30 t/8,000 m2, respectively, over crops raised with farm yard manure (8.72 t) or green manuring (8.9 t) and thus resulted in higher net returns too (Table 5). The average quantity of waste materials obtained from plant and animal sources along with their nutrient content is presented in Table 6. The poultry unit had produced 2.66 t of poultry droppings, and out of this quantity, 50% of droppings were fed to fish and the rest 50% of droppings were used in the preparation of poultry manure and applied to the crops. The duck unit produced a total quantity of 1.46 t of raw droppings, and the whole quantity was applied to the pond as fish feed. FYM, prepared from dairy wastes (9.0 t/annum), added 35.6 kg N, 45.9 kg P2O5, and 51.7 kg of K2O in the soil, whereas the goat unit added 23.7 kg N, 15.5 kg P2O5, and 11.7 kg of K2O into the soils on an annual basis in the form of goat manure (Table 6). The highest quantity of NPK was added through vermicomposting which was prepared from plant wastes (73.8, 79.7, and 44.8 kg/annum, respectively).
Table 6 Nutrient recycling within developed IFS at Patna, Nalanda, and Vaishali (mean value of 6 years: 2016–2021).
Recycling of droppings through fish ponds enhanced nutrient content by 2- to 2.5-fold (44.0, 20.5, and 32.5 and 41.9, 26.6, and 23.2 kg of N, P2O5, and K2O, respectively, in terms of nutritive values) for 50% of poultry and whole of duck droppings, respectively. Besides, the poultry unit also provided 39.2, 29.5, and 15.4 kg of N, P2O5, and K2O from poultry manure (Table 6). After analyzing all types of plant and animal wastes, it could be emphasized that dairy manure contributed the highest quantity of P2O5 and K2O whereas poultry unit (700 birds/annum) generated the highest N upon recycling (Table 6). Application of organic forms of manure received upon resource recycling like pond silt, vermicompost, FYM, and manures of goat, duck, and poultry had a synergistic effect on crop productivity and sparing effect on the input cost by saving an appreciable amount of chemical fertilizers (Table 6).
Analysis of the economic efficiency of different integrations (Table 4) revealed that integration of any enterprise with crop component provided better net returns over sole cropping. Integration of different enterprises not only enhanced RGEY but also resulted in more income. Considering gross returns of different combinations (Table 4), Cr+F+C integration gave the highest gross returns (USD 4,645) and secured the first rank followed by Cr+F+D+G (USD 4477/annum), but after economic analysis of different integrations, the crop integrated with cattle ranked seventh in terms of net annual returns (USD 1,269) as well as per-day net returns (USD 3.5/day) because of the higher production cost of cattle (USD 3,376/annum) over other animals. Hence, crops integrated with fish, duck, and goat provided the highest net returns of USD 1,954/annum and higher per-day net returns (USD 5.4/day) for which an annual expenditure of USD 2,523 was incurred as input cost. Cr+F+G integration was evaluated as the next best integration in terms of net returns and net returns/day (Table 7). Economic analysis of individual components also showed higher incurred expenditure on the rearing of cattle (USD 1,187) over goat rearing (USD 702). Further, a higher average return of USD 952/year was received with the goat unit from an area of 200 m2 whereas production of crops alone provided only USD 780 from an area of 8,000 m2. Application of vermicompost and duck recycled droppings (pond manure) in crops was more profitable (USD 952 and USD 918, respectively) than other organic manures (Table 6).
Table 7 Net returns and sustainability index of different integrations (mean value of 6 years, 2016–2021).
Six years of studies on integration at different sites revealed that the average sustainability index was higher in the case of Cr+F+D+G integration (0.77) and was again followed by Cr+F+G (0.65) and Cr+F+P integration (0.65) while cropping sequences (rice–maize–moong) resulted in a lower SI value of 0.36. Conventional cropping systems (rice–wheat and rice–maize) resulted in the least SI values of 0.07 and 0.15, respectively, indicating the least profitable system (Table 7). Systems with a higher sustainability index advocate that these integrations are sustainable for the abovementioned locations in the longer term in respect of productivity and economics.
The link relative index (LRI) was computed by taking either improved practices or farmers’ practice as a standard base among different combinations or say farming systems models (Table 8). The highest LRI value (170 and 337 for farmers’ and improved practices, respectively) through improved practices was obtained with Cr+F+D+G integration in 8,000 m2 of CV indicates coefficient of variation.the farm, which was followed by Cr+F+G (150 and 297) and Cr+F+P (149 and 296) integration, advocating efficient and useful resource recycling within the integrated components. Conversion of prices at a fixed level and then computing LRI eliminated the factor of variation due to differences in prices in different years. The coefficient of variation (CV) was worked out for finding the goodness of fit of these LRIs in the calculation which shows large variations in Cr+F+D+G integration and conventional farming (rice–wheat).
Table 8 Net income and link relative index (LRI) of different integrations (mean value of 6 years, 2016–2021).
Employment generated through individual components and by IFS is depicted in Figure 1. The mean average additional man-days requirement increased by 230, 221, 198, 165, 125, 113, and 106 per annum through Cr+F+G+P, Cr+F+C, V+G, Cr+F+G, Cr+F+M, Cr+F+P, and Cr+F+D integrations, respectively, over the rice–wheat system (237 man-days/annum) only depending upon labor requirement by individual component as well as its number and area. Farming with a conventional system (rice–wheat) required the least man-days (237 man-days/annum), whereas farming with an improved cropping system (rice–maize–moong) followed under different IFS integrations required 40 more man-days due to the inclusion of one more crop into the sequence. The average man-days requirement was increased up to 467 per 8,000 m2 area for Cr+F+D+G integration over cropping alone in the same area of land (237 for rice–wheat, 252 for rice–maize, and 277 man-days/year for rice–maize–moong). In other words, Cr+F+D+G, Cr+F+C, and V+G integration generated additional employment of 230, 221, and 198 man-days/year, respectively, over the rice–wheat system.
In developed IFS, considering individual components, labor energy input was markedly high in field crops followed by cattle and goat rearing (Table 9). Among the different integrations, the dairy unit (2 cows + 2 calves) consumed the maximum energy input (70,300 MJ) while the mushroom unit (100 bags/season) consumed the least energy input (190 MJ). The dairy unit also provided the maximum energy output (71,500 MJ), while the least energy output was obtained from the mushroom unit (1,540 MJ) but in terms of energy efficiency ratio (EER), the highest EER was obtained from the horticultural unit, i.e., fruit (8.19) followed by mushroom unit (8.11), vegetables (6.01), fodder unit (5.75), and crop unit (4.30) while least EER was obtained through the dairy unit (1.02). In terms of human energy profitability (HEP), the fruit unit provided maximum HEP (28.9) and was followed by the goat unit (26.18), while the mushroom unit resulted in the least HEP (15.3). Net energy gain (NEG) was calculated to be highest in the case of crop unit (4,000 m2) by 47,960 MJ and was followed by goat unit (20 female + 1 male), i.e., 37,380 MJ, indicating more efficient units in terms of NEG (Table 9).
Table 9 Energy budgeting of individual components in developed IFS systems (pooled data of 6 years: 2016–2021).
Among the different integrations, Cr+F+D+G integration provided the maximum NEG (95,770 MJ) and was followed by Cr+F+G integration (91,910 MJ) indicating lesser input energy requirement and producing higher output energy which is a deciding factor for making any integration (Table 10). Cr+F+C (55,730 MJ), Cr+Fcrop + fish (54,530 MJ), and cropping alone (47,960 MJ) recorded the least NEG. The energy efficiency ratio was highest with CrF+P (4.33) followed by cropping alone (4.30), Cr+F+M (4.16), and Cr+F (4.12). Integration of larger animals in the system resulted in lower EER as a huge amount of energy is required for their maintenance. Further, the highest HEP was obtained through Cr+F+D+G integration followed by Cr+F+G, indicating that these combinations require less manpower than other integrations (Table 10).
Through intensification of crop and associated components, yield and productivity per unit area get enhanced under the IFS. Singh et al. (2004) emphasized that integration of crops which are needed either for farm family or for the system must be included into the system like paddy, wheat, maize, oilseeds, and pulses. Further, maize should be included in the cropping system as it not only provides grains as feed for livestock and poultry but also provides green fodder to livestock and can be grown almost throughout the year. Setimala et al. (2017) reported the yield advantage of 23.2% from maize crop even under stress conditions and advocated including it in the cropping system as maize is one of the main crops which support livestock in the system. Further, integration of larger livestock requires more man-hours and energy for their maintenance and thus attributed to more cost of production in the rearing of cattle (cows), especially in man-power requirement and feeding cost (Dey et al., 2019), but the integration of cattle or goat in the cropping system is proved to be beneficial in terms of overall production and productivity from a single piece of land. Here, the finding of Singh et al. (2017) on the IFS in Southern Rajasthan conforms with results obtained in the Nalanda, Patna, and Vaishali districts of Bihar. Rearing of the small livestock, viz., goat, poultry, duck, and pig, was profitable, and hygiene was maintained along with proper management of animals and vaccination at the right time and right age (Kumar et al., 2012); otherwise, it is a risky component due to outburst of severe diseases like Ranikhet in which a loss up to 100% was recorded.
Upon integration of various components on the same piece of land, a huge quantity of agriculture and animal wastes is obtained which can be easily recycled and reused within the system which adds organic matter to the farm soil and curtails the cost of production by providing a sizeable amount of plant nutrients to crops and offers feed to the animals and birds. Chen et al. (2011) also reported similar benefits in respect of yield and CH4 emission while recycling nutrients within the system. Devendra and Thomas (2002) also reported that the application of recycled products as organic not only increased system productivity but also curtailed an ample quantity of chemical fertilizers equivalent to their nutrient content. A higher link relative index (LRI) is characteristic of the greater number of integration due to large variations. Here, Cr+G+F+D reported a larger variation over any cropping system. A similar LRI was also reported by Laxmi et al. (2015) from their study on horticulture–cum–fish farming in the Malwa region of Madhya Pradesh under semiarid tropical conditions.
Integration of different components, viz., field crops, vegetables, fishery, animals, and birds, enhances gross production and income of the system by supplementing recycled nutrients to crops as well as feed materials to animals and birds through means of resource recycling and better resource use efficiency (Kumar et al., 2017). Jayanthi et al. (2003) in Tamil Nadu and Korikanthimath and Manjunath (2005) in Goa also reported an increased income of up to 200% through different integrations with crops over traditional cropping alone. A higher benefit/cost (B:C ratio) was obtained when crops were fertilized with inorganic fertilizers + recycled manures in form of FYM/duck droppings/poultry droppings/vermicompost/green manuring over the crop fertilized with chemical fertilizers only and also minimized the cost of production through saving of an equivalent amount of chemical fertilizers and cost of purchase (Kumar et al., 2015; Kumar and Narayanagowda, 2017).
Farming in IFS mode is labor intensive as manpower requirement is enhanced due to an increase in the level of management. To carry out daily management activities either in crops, livestock, or fishery, more man-hours are required over the sole cropping system. These enhanced man-hours could meet through the hiring of laborers on a payment basis or by engaging family laborers. In other words, farm family labor is fully utilized, and if farm family labor is not available then employment opportunity gets increased. Manjunath et al. (2017) also reported that labor involvement in IFS was spread throughout the year with the potential for additional wages. Further, this employment potential reduces the pressure of peak labor requirements and engages farm families for most of the year. Purnomo et al. (2021) concluded that inclusion of animals/fish/birds with crops or even inclusion of one or more types of crops in the system enhanced man-days requirement in the range of 43%–55%.
Nowadays, the energy use efficiency of any agricultural production system is regarded as an indicator of crop performance. Agricultural productivity evaluation using energy budgeting is important to make efficient use of the available natural resources and to obtain maximum sustainable economic yield. Energy consumption in agriculture has increased consistently over some time in the form of inputs such as fossil fuel, fertilizers, pesticides, herbicides, electricity, and machinery, causing environmental and human health problems (Paramesh et al., 2019). The amount of energy used in agricultural production, processing, and distribution should be significantly higher to feed the expanding population and to meet other social and economic goals, and therefore, sufficient availability of the right energy and its effective and efficient use are prerequisites for improved agricultural production (Stout, 1990). While selecting the components for the IFS, one should keep in mind the energy requirement by individual component. Less energy-demanding components should be preferred for any system. Taki et al. (2012) reported that the dairy unit is not energy efficient as a large quantity of energy was used in the maintenance of animal muscle, tissues, or bones. Taki et al. (2012) also advocated that greater energy profitability (EF) should be taken into consideration while integrating different components in IFS. Lesser energy requirements and higher output energy should be the deciding factor for making any integration. Esengun et al. (2007) emphasized that the best way to lower the environmental hazard caused due to extensive energy use in agriculture systems was to increase the EER and NEG of any system.
Providing food and nutritional security to the farm family is one of the aims of an integrated farming system. Well-integrated complementary IFS systems provide dietary needs of farm families partially or fully depending upon the intensity of integration and size of the farm. Such systems form the base of the Indian agriculture and help to provide most of the staples consumed by millions of farm families, as the IFS offers scope to utilize land and time for growing short-duration vegetable crops, pulses, and fodder for livestock, and for multiple uses of water like rearing of fish/duck/poultry along with irrigation to the crops. These systems are very critical for achieving future food and nutrition for the burgeoning Indian population. Here, Cr+F+D+G and Cr+F+C integration provided almost all the staple food to the farm families (Table 11). Homestead farming integrated with livestock and fish in Bihar (0.4 ha) supports a farm family of seven members with cereals, pulses, oilseeds, fruits, vegetables, milk, meat, eggs, and mushroom throughout the year (Kumar et al., 2018). Production obtained from different components of IFS as depicted in Table 11 shows the potential of the IFS in diversifying the food basket of small and marginal farmers from a small piece of land and also enlightened the importance of the IFS in producing feed and fodder required for livestock and fuel wood for household consumption. Sujatha and Bhat (2015) also emphasized the importance of the IFS for poor small and marginal farmers to meet the protein and other dietary requirements through grains and fibers from the crops, eggs, milk, meat, and fish.
Although the IFS has several advantages, farmers are unable to adopt IFS systems due to several constraints, viz., financial, biophysical and sociocultural, institutional, or policy. Among these constraints, the financial constraints emerged as major limitations in adopting the IFS approach of food production (Pandey et al., 2019) due to high initial investment for the establishment of the pond, animal shed, and purchase of livestock. Further, biophysical constraints like non-availability of quality seed and planting materials, lack of knowledge about new crops, and availability of animal health services formed major constraints in adopting the crop–livestock system (Mahapatra and Behera, 2011). However, socioeconomic constraints like idiosyncratic character, the attitude of the farmers, caste and creed-related issues, investment capacity, and risk-bearing capacity are found as major constraints in adopting an IFS system (Patra and Samal, 2018) as reported from Indonesia. Apart from this, farmers hesitate to change and are found laggards in the adoption of improved and advanced technologies, improved crops, and livestock breeds. Nearly 35%–40% of farmers at the surveyed location (Patna, Nalanda, and Vaishali) did not show their interest in IFS adoption (Kumar et al., 2017). Hence, anchoring suitable motivation and encouragement to the farmers through live demonstration, training, and field visits along with the provisioning of easy credit facilities may trigger the adoption of the IFS. A concrete national policy and institutional support for the adoption of the IFS in different agro-climatic regions of the country are the demand of the modern era under the climatic changing scenario. Further, farming is closely linked with the environment and has a greater impact on soil, water, CO2–O2 level, CH4 emission, landscape, and biodiversity (Chen et al., 2011). Hence, there is a need for location-specific policy to provide crop-specific prices, insurance, and income support to protect the farmers from market fluctuations and conserve agro-ecological assets, as well as maintain ecological balance through sustainable use of natural resources (Wezel et al., 2014). Here, it will be imperative to say that one should not go for the development of the new IFS system, but a change in the existing farming system is strongly advocated. This will create faith among farmers on IFS approaches with the learning-by-doing approach.
Science-based resource optimization approaches with environmental stewardship (for example, use of the farm design model), standardization of acreage to support a family of five to eight as a sole means of livelihood security, the role of IFS in mitigation of climate change impact on agriculture, scalable arrangements and incentives for horizontal expansion of technology, small farm mechanization, and strong policy support for promotion are some of the researchable issues that need to be addressed in future.
Farming in an integrated mode is more beneficial for small and marginal farmers than the cropping system alone as per our research findings across the different locations of the state. SD means standard deviation and CV means coefficient of variance.Crop + fish + duck + goat integration had shown higher rice grain equivalent yield, net returns, and energy efficiency without any negative effect on soil or system and proved to be environment-friendly through resource recycling of farm wastes which also curtailed the input cost in the form of chemical fertilizers, pesticides, and weedicide. Moreover, small and marginal farmers face different types of problems in comparison with large farmers as they depend on farming for their household needs and a majority of these farmers are resource-poor and have a low level of education and technical knowledge. Suitable agricultural technologies are, therefore, required for the development of this group of farmers and for which farming in integrated mode will prove to be worthy than specialized cropping alone. The results obtained from this research may be useful for diversification in agriculture by the farmers as well as researchers and designing of effective agricultural planning by government and policymakers. Future research should further investigate the wellbeing of laborers, farmers, and consumers and their interaction with farm size and with other social and environmental outcomes.
The original contributions presented in the study are included in the article/supplementary material. Further inquiries can be directed to the corresponding author.
SK: Conceptualization, methodology, formal analysis, investigation, visualization, designing of experiment, writing-original draft, writing- review SH: Data analysis, performed the experiments, draft editing and review writing AD: Data collection and analysis related to livestock and fishery, review writing UK: Data pertaining to social parameters, editing and review collection RK, SM and MB: soil data analysis, graphics, writing draft, review and editing. AK: Data collection, analysis and editing All authors contributed to the article and approved the submitted version.
The authors gratefully acknowledge the Indian Council of Agricultural Research (ICAR), New Delhi, and the Indian Institute of Farming System Research, Modipuram, Meerut (India), for providing financial support in conducting the above research activities.
The authors declare that the research was conducted in the absence of any commercial or financial relationships that could be construed as a potential conflict of interest.
All claims expressed in this article are solely those of the authors and do not necessarily represent those of their affiliated organizations, or those of the publisher, the editors and the reviewers. Any product that may be evaluated in this article, or claim that may be made by its manufacturer, is not guaranteed or endorsed by the publisher.
Allen V. G., Baker M. T., Segarra E., Brown C. P. (2007). Integrated irrigated crop-livestock systems in dry climates. Agron. J. 99, 346–360. doi: 10.2134/agronj2006.0148
Ansari M. A., Prakash N., Baishya L. K., Punitha P., Yadav J. S., Sharma P. K., et al. (2013). Comparative study on conventional and improved integrated farming systems for sustainable production, income generation and employment opportunity among the tribal farmers in hilly regions of manipur. Indian J. Agric. Sci. 83 (7), 765–772.
Brida A. B., Owiyo T., Sokona Y. (2013). Loss and damage from the double blow of flood and drought in Mozambique. Int. J. Glob.Warm. 5, 514–531. doi: 10.1504/IJGW.2013.057291
Chen W., Wolf B., Zheng X., Yao Z., Butterbach- Bahl K., Brüggemann N., et al. (2011). Annual methane uptake by temperate semiarid steppes as regulated by stocking rates, aboveground plant biomass and topsoil air permeability. Global Change Biol. 17 (9), 2803–2816. doi: 10.1111/j.1365-2486.2011.02444.x
Devendra C., Thomas D. (2002). Smallholder farming systems in Asia. Agric. Syst. 71 (1–2), 17–25. doi: 10.1016/S0308-521X(01)00033-6
Dey A., Sarma K., Kumar U., Mohanty S., Kumar T., Bhatt B. P. (2019). Prospects of rice-fish farming system for low lying areas in bihar, India. Org. Agric. 9 (1), 99–106. doi: 10.1007/s13165-017-0204-8
Erdal G., Esengün K., Erdal H., Gündüz O. (2007). Energy use and economical analysis of sugar beet production in Tokat province of Turkey. Energy 32(1), 35–41.
Esengun K., Gündüz O., Erdal H. (2007). An economic analysis and energy use in stake-tomato production in tokat province of Turkey. Renewable Energ. 32, 1873–1881. doi: 10.1016/j.renene.2006.07.005
Gill M. S., Singh J. P., Gangwar K. S. (2010). Integrated farming system and agriculture sustainability. Indian J. Agro. 54 (2), 128–139.
Giudice V. D., Manganelli B., Paola P. D. (2016). “Depreciation methods for firm’s assets”, in International conference on computational science and its applications (Springer, Cham, Switzerland), 214–227).
Goldstone J. A. (2010). The new population bomb: The four megatrends that will change the world. Foreig. Affair. 89, 31.
Jayanthi C., Balusamy M., Chinnusamy C., Mythili S. (2003). Integrated nutrient supply system of linked components in lowland integrated farming system. Indian J. Agro. 48 (4), 241–246.
Jordan V. W. L., Hutcheon J. A., Dolandson G. V., Farmer D. P. (1997). Research into and development of integrated farming systems for less- intensive arable crop production: experimental progres-1994) and commercial implementation. Agricult. Ecosyst. Environ. 64, 141–148. doi: 10.1016/S0167-8809(97)00032-7
Kakamoukas G., Sarigiannidis P., Maropoulos A., Lagkas T., Zaralis K., Karaiskou C. (2021). Towards climate smart farming- a reference architecture for integrated farming system. Telecom 2, 52–74. doi: 10.3390/telecom2010005
Korikanthimath V. S., Manjunath B. L. (2005). Resource use efficiency in integrated faming systems. in: Proceeding of a symposium on alternative farming systems: Enhanced income and employment generation options for small and marginal farmers. ICAR-PDCSR. Modipur., 109–118.
Kumar S., Bhatt B. P., Dey A., Shivani, Kumar U., Idris M., et al. (2018). Integrated farming system in India: Current status, scope and future prospects in changing agricultural scenario. Indian J. Agric. Sci. 88 (11), 13–27.
Kumar S., Bhatt B. P., Dwivedi S. K. (2015). Family farming- a mechanism to contribute towards global food security. Indian Farm. 64 (12), 13–18.
Kumar S. S., Narayanagowda K. (2017). Development of scale to measure attitude of schedule caste farmers towards integrated farming system in southern karnataka and its application. Mysore. J. Agric. Sci. 51 (2), 297–302.
Kumar S., Shivani, Samal S. K., Dwivedi S. K., Manibhushan (2017). Enhancement in productivity and income sustainability through integrated farming system approaches for small and marginal farmers of Eastern India. J. AgriSear. 4 (2), 85–91. doi: 10.21921/jas.v4i2.7777
Kumar S., Singh S. S., Shivani, Dey A. (2011). Integrated farming system for eastern India. Indian J. Agro. 56 (4), 297–304.
Kumar S., Subhash N., Shivani, Singh S. S., Dey A. (2012). Evaluation of different components under integrated farming system (IFS) for small and marginal farmers under semi-humid climatic environment. Exp. Agricult. 48 (3), 399–413. doi: 10.1017/S0014479712000087
Laxmi L., Gupta N., Singh M., Sharma R. P. (2015). Evaluation of integrated horticulture-cum- fish farming in malwa region of Madhya pradesh. India. Curr. World Environ. 10 (2), 667–671. doi: 10.12944/CWE.10.2.33
Mahapatra I. C., Behera U. K. (2011). Rice (Oryza sativa)-based farming systems for livelihood improvement of Indian farmers. Indian J. Agron. 56 (1), 1–19.
Manjunath B. L., Paramesh V., Mahajan G. R., Das B., Reddy K. V., Chakurkar E. B., et al. (2017). Sustainability through resource recycling, soil fertility and carbon sequestration from integrated farming systems in west coast India. Bioscan. 12, 1–6.
Page A. L., Millar R. H., Keeney D. R. (1982). “Methods of soil analysis: Part 2,” in American Society of Agronomy/Soil science society of America Soil Science Society of America (Madison,W.I).
Pandey P. R., Gupta J. K., Narvariya R. K., Meena S. C., Narwariya D. (2019). Constraints faced by farmers in adoption of integrated farming system in vindhyan plateau of Madhya pradesh. Plant Arch. 19 (2), 512–514.
Paramesh V., Parajuli R., Chakurkar E. B., Sreekanth G. B., Kumar H. B. C., Gokuldas P. P., et al. (2019). Sustainability, energy budgeting, and life cycle assessment of crop- dairy-fish-poultry mixed farming system for coastal lowlands under humid tropic condition of India. Energy 188, 116101. doi: 10.1016/j.energy.2019.116101
Patra S., Samal P. (2018). Integrated farming system in India: A holistic approach to magnify the economic status of innovative farmers. J. Pharmacogn. Phytochem. 7 (3), 3632–3636.
Porter J. R., Xie L., Challinor A. J., Cochrane K., Howden S. M., Iqbal M. M., et al. (2014). Food security and food production systems (Cambridge, UK: Cambridge University Press).
Purnomo S. H., Sari A. I., Emawati S., Rahayu E. T. (2021). Factors influencing the adoption of integrated crop-livestock to support land conservation of organic agriculture in mojosongo area, karanganyar, indonesia. IOP conference series. Earth Environ. Sci. 724 (1), 12049. doi: 10.1088/1755-1315/724/1/012049
Russelle M. P., Entz M. H., Franzluebbers A. J. (2007). Reconsidering integrated crop-livestock systems in north America. Agron. J. 99, 325–334. doi: 10.2134/agronj2006.0139
Setimala P. S., Mangorokosho C., Lunduka R., Gasura E., Makumbi D., Tarekenge A. (2017). On -farm yield gains with stress- tolerant maize in Eastern and southern Africa. Agron. J. 109, 406–417. doi: 10.2134/agronj2015.0540
Singh H., Burark S. S., Sharma S. K., Jajoria D. K., Sharma R. P. (2017). Economic evaluation of farming systems for agricultural production in southern rajasthan. Eco. Affair. 62 (1), 47. doi: 10.5958/0976-4666.2017.00025.0
Singh K., Singh A. K., Singh K. K., Singh C. S. (2004). Analysis of farming systems in north-eastern plain zone of uttar pradesh. J. Farm. Syst. Res. Dev. 10 (1–2), 1–6.
Stout B. A. (1990). Handbook of energy for world agriculture (Elsevier, Texas), ISBN: ISBN: 9780444598547.
Sujatha S., Bhat R. (2015). Resource use and benefits of mixed farming approach in arecanut ecosystem in India. Agric. Syst. 141, 126–137. doi: 10.1016/j.agsy.2015.10.005
Taki M., Ajabshirchi Y., Mobtaker H. G., Abdi R. (2012). Energy consumption, input–output relationship and cost analysis for greenhouse productions in esfahan province of Iran. Am. J. Exp. Agricult. 2 (3), 485–501. doi: 10.9734/AJEA/2012/1461
Tracy B. F., Davis A. S. (2009). Weed biomass and species composition as affected by an integrated crop-livestock system. Crop Sci. 49, 1523–1530. doi: 10.2135/cropsci2008.08.0488
Tracy B. F., Zhang Y. (2008). Soil compaction, corn yield response, and soil nutrient pool dynamics within an integrated crop-livestock system in Illinois. Crop Sci. 48, 1211–1218. doi: 10.2135/cropsci2007.07.0390
Vittal K. P. R., Sankar G. R., Singh H. P., Sharma J. S. (2002). “Sustainability index,” in Sustainability of practices of dryland agriculture, methodology and assessment, ICAR Central Research Institute for Dryland Agriculture(Hyderabad, India).
Wezel A., Casagrande M., Celette F., Vian J.-F., Ferrer A., Peigné J. (2014). Agroecological practices for sustainable agriculture: a review. Agron. Sustain. Dev. 34 (1), 1–20. doi: 10.1007/s13593-013-0180-7
Keywords: Employment generation, Energetics, Integrated Farming System, Income, Livelihood security, Resource recycling, System Productivity, Sustainability
Citation: Kumar S, Shivani, Dey A, Kumar U, Kumar R, Mondal S, Kumar A and Manibhushan (2022) Location-specific integrated farming system models for resource recycling and livelihood security for smallholders. Front. Agron. 4:938331. doi: 10.3389/fagro.2022.938331
Received: 07 May 2022; Accepted: 08 July 2022;
Published: 03 August 2022.
Edited by:
JS Mishra, ICAR Directorate of Weed Research, IndiaReviewed by:
Raghavendra Singh, Indian Institute of Pulses Research (ICAR), IndiaCopyright © 2022 Kumar, Shivani, Dey, Kumar, Kumar, Mondal, Kumar and Manibhushan. This is an open-access article distributed under the terms of the Creative Commons Attribution License (CC BY). The use, distribution or reproduction in other forums is permitted, provided the original author(s) and the copyright owner(s) are credited and that the original publication in this journal is cited, in accordance with accepted academic practice. No use, distribution or reproduction is permitted which does not comply with these terms.
*Correspondence: Rakesh Kumar, cmFrZXNoYmh1MDhAZ21haWwuY29t
Disclaimer: All claims expressed in this article are solely those of the authors and do not necessarily represent those of their affiliated organizations, or those of the publisher, the editors and the reviewers. Any product that may be evaluated in this article or claim that may be made by its manufacturer is not guaranteed or endorsed by the publisher.
Research integrity at Frontiers
Learn more about the work of our research integrity team to safeguard the quality of each article we publish.