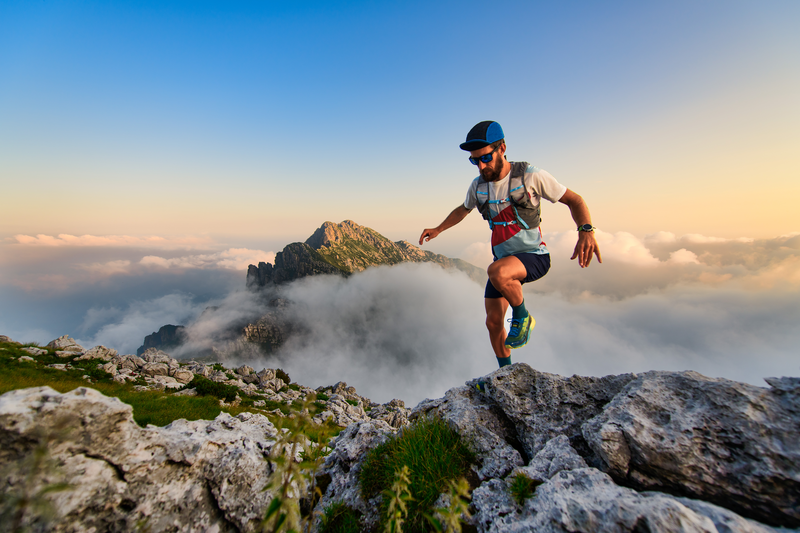
95% of researchers rate our articles as excellent or good
Learn more about the work of our research integrity team to safeguard the quality of each article we publish.
Find out more
ORIGINAL RESEARCH article
Front. Agron. , 19 July 2022
Sec. Weed Management
Volume 4 - 2022 | https://doi.org/10.3389/fagro.2022.930378
This article is part of the Research Topic Insights in Weed Management View all 5 articles
Palmer amaranth (PA) is one of the major weeds in sweetpotato reducing its quantity and quality. The widespread and repeated use of chemical herbicides has led PA to develop resistance for such chemicals. In addition, chemical herbicides are incompatible with the organic production system. It is imperative to find sustainable weed management strategies to provide weed control suitable for organic cultivation and detain the development of herbicide-resistant weeds under conventional crop production. In the present study, seventeen sweetpotato varieties were screened for their allelopathic (weed-suppressing) effect on the growth of PA. The experiment was conducted in a greenhouse using a stair-step system. Each plant in the stair-step system had its height (cm), chlorophyll concentration (cci) and shoot biomass (g) measured. The variation in the height, chlorophyll and shoot biomass reduction of PA was significant after the third week of transplanting. Three weeks after transplanting (WAT), only three varieties, i.e., Morado (75%), Bayou belle (62%), and Vardaman (61%), reduced PA’s height by >60% compared to the control. While 5 WAT, four varieties, i.e., 529 (93%), Morado (93%), Heartogold (85%), and Centennial (81%), reduced PA height by >80%. Hatteras, Centennial, and 529 reduced the chlorophyll content of PA by >50%. In the presence of Beauregard, the commercial cultivar, there was no reduction in shoot biomass of PA. Cluster analysis also demonstrated that the four allelopathic sweetpotato varieties, i.e., Heart-O-Gold, Centennial, 529, and Morado, were clustered together, indicating that these varieties have similar potential to suppress the growth and development of PA. Combining allelopathic sweetpotato cultivars (Heart-O-Gold, Centennial, 529, and Morado) with other sustainable weed control measures, such as cover crops and hand-weeding, can improve the weed management, espicially in organic farming. However, field experiments should be conducted to confirm the allelopathic as well as yield potential of these varieties in an agronomic setting. The availability of the allelopathic sweetpotato cultivars will benefit organic producers by enhancing crop productivity and decrease reliance on chemical herbicides in conventional farming systems.
Sweetpotato [Ipomoea batatas (L.) Lam.] is a nutrition rich food. It is the fifth most important crop worldwide, also termed ‘famine relief’ food as it meets the nutritional needs of impoverished regions (Kakwani and Son, 2016; Vithu et al., 2019). In the United States, it is mostly grown in North Carolina, California, Mississippi and Louisiana (USDA, 2020; Duque et al., 2022). Weed management is a major challenge for sweetpotato growers (Nwosisi et al., 2019). One of the most problematic weeds of sweetpotatoes is Palmer amaranth (PA) which is a broad-leaved, annual dicot weed with the ability of fast early-season growth and profuse seed production (Culpepper et al., 2010; Barkley et al., 2016; Smith et al., 2020). PA if provided with optimal moisture and temperature conditions, has the ability of 100% viable seed germination on the first day of sowing (Smith et al., 2020). Palmer amaranth’s competition and interference with the crop plants result in significant crop yield loss, higher production costs, environmental issues, loss of biodiversity, and threaten ecosystem safety (Shen et al., 2018a). In addition, it serves as alternate host for many disease-causing pathogens and pests (Morgan et al., 2001; Finney and Creamer, 2008). Amaranthus palmeri (Palmer amaranth) can reduce sweetpotato yield by up to 81% (Meyers et al., 2010). Being a plant of vine nature, sweetpotato grows close to the soil surface, thus hand-weeding is the only mechanical option for weed management on a sweetpotato field (Treadwell et al., 2007). In order to maintain and promote crop productivity and minimize labor requirements, chemical herbicides have been widely applied for weed control in crop fields. However, long-term and extensive herbicide applications have increased number of herbicide-resistant weed species (Webster and Nichols, 2012; Norsworthy et al., 2012; Comont et al., 2019). Resistance to the most commonly used chemical herbicide to control Palmer amaranth, i.e., glyphosate, was reported after continuous application for only 4 to 6 years (Legleiter and Bradley, 2008). Similarly, several weeds exhibit multiple herbicide resistance, such as glufosinate and paraquat resistance in goosegrass (Seng et al., 2010). Inappropriate herbicide uses such as application during wrong stage of crop development or during inapt weather conditions could cause serious issues, such as groundwater contamination or residue accumulation in the soil (Walker et al., 2013). Furthermore, these chemical herbicides are not suitable for use under organic farming system (Nwosisi et al., 2019). Awareness among people about the associated threats and increasing demand for high-quality agricultural produce poses a critical need for safer and environmentally sustainable weed management approaches (Głąb et al., 2017).
Consequently, economically sustainable and environment-friendly weed management strategies, such as cover crops and/or allelopathic crops are gaining popularity among researchers. Allelopathy, an old and natural phenomenon, is an effective weed control strategy and is gaining the interest of researchers all over the world (Li et al., 2010). Allelopathy is the release of secondary metabolites, also known as allelochemicals, by one plant species that directly or indirectly affect its neighboring plants (Rice, 1984; Alsaadawi et al., 2012; Dadkhah, 2015). According to studies, these allelochemicals are environment-friendly and biodegradable (Rizvi, 2012). Weed suppressing ability has been reported in several crops including rice, sorghum, canola, and wheat (Kong et al., 2011; Rice, 2012; Khan et al., 2015). It is vital to identify the varieties with ability to produce high levels of weed-suppressive chemicals that can then be used by breeders to develop sweetpotato varieties with considerable weed suppressive potential. In addition to inhibiting the evolution of herbicide-resistant weeds, these allelopathic sweetpotato varieties can encourage organic or sustainable sweetpotato production.
Allelopathic property of sweetpotato has been demonstrated to reduce the growth and development of weeds such as alfalfa, and yellow nutsedge (Harrison and Peterson, 1986; Shen et al., 2018a). It is, therefore, critical to screen potential sweetpotato varieties for weed suppressive ability against troublesome Palmer amaranth. Therefore, the present study evaluates the allelopathic potential of seventeen sweetpotato varieties against Palmer amaranth.
Seventeen sweetpotato varieties representing landraces, breeding lines, and cultivated varieties were selected and obtained from the International Potato Center in Lima, Peru, the National Germplasm Repository in Griffin, GA, and North Carolina State University’s Micropropagation and Repository Unit in Raleigh, NC.
All the 17 varieties and Palmer amaranth were screened in the greenhouse at the R.R. Foil Plant Science Research Center, Mississippi State University, with a day/night temperature and cycle set to 28/24°C and 16/8 hr, respectively. The experiment was carried out in a completely randomized design using a stair-step structure (Figure 1A) with three replications. Quickrete Play Sand (silicon dioxide) was used as a growing medium which eliminated algae formation that could have changed the nutrient composition of the flowing water and provided a medium for easy flow of water from one pot to the next. The slips collected from more than three months old plants with four nodes were transplanted in the pots with sand. After 10 days of transplanting when the plant established their roots, these pots were placed into the stair-step structure.
Figure 1 (A) Stair-step structure with plants on four steps. Collecting tanks are at the bottom and bottles are at the top to cycle the solution, (B) A diagrammatic representation of the arrangement of plants in the stair-step structure.
Rockwool was used to germinate Palmer amaranth seeds in the growth chamber (53% humidity, 16/8 hr day/night cycle). The rockwool was soaked in water for 30 minutes and 10% acetic acid was added to achieve a pH of 5.8 before transferring it to a dixie tray to insert Palmer amaranth seeds in it. A greenhouse top was used to cover the tray to maintain the moisture. Upon germination, PA seedlings were transplanted into the pots with sand. Three Palmer amaranth seedlings were transplanted in one pot and were then allowed to establish in the sand for 2-3 weeks. After establishment, these pots were also placed into the stair-step set-up.
The stair-step system consisted of six rows in each column; a bottle was fitted on the top-most step and a collecting tank with a pump on the bottom-most step. The middle four steps were occupied by the pots of size 15 × 10 cm (diameter × depth) with the experiment plants. There were two columns, i.e. the control column and the treatment column (Figure 1B), potato variety tested. In the control column all the four rows had one pot containing two plants of one sweetpotato variety, while in the treatment two pots containing the same sweetpotato variety and two containing Palmer amaranth were placed in alternate rows. Also, one column (per replication) with pots containing all the Palmer amaranth plants served as weed control. The pumps in the collecting tanks were fitted with plastic tubing that connected the bottles on top step to the respective collecting tank of each column. The pumps were also connected to a timer and every six hours the water from the collection tank was pumped to the bottles on top-most step through the plastic tubing. From there the water dripped to the descending pots and ultimately drained to the collection tank. The timers were on and distilled water was used throughout the duration of experiment. In order to prevent nutrient deficiency, quarter strength Hoagland’s No. 2 basal salts (Caisson Laboratories, Smithfield, UT, USA) was added in the collecting tank containing distilled water after every two weeks of transplanting. Each column was a closed loop structure with its own tubing, bottle, pump and collecting tank and was independent of the others.
The height of all the plants in the stair-step system was recorded at 2, 3, 4, 5, and 6 weeks after transplanting (WAT). The chlorophyll content of the plants at 3 WAT was recorded using a CCM300 chlorophyll meter (Opti-Science, Hudson, NH, USA). After six weeks, the plants were harvested (above ground), dried in a hot air oven for three days at 65°C and shoot biomass (SB) was recorded.
The allelopathic effect of the sweetpotato varieties based on the percent inhibition of the Palmer amaranth was calculated using the following equations:
Data were analyzed using JMP 14.1.0 (SAS Institute Inc., Cary, NC) software at p ≤ 0.05. Principle Component Analysis (PCA) was performed using PAST software v 4.02 (Hammer et al., 2001).
In the present study, plant height (weekly from 2 WAT to 6 WAT), chlorophyll content and shoot biomass of all the plants in the stair-step were recorded. The Analysis of Variance (ANOVA) showed singificant (Table 1) variation among the sweetpotato varieties for plant height (at 3, 4, and 5 WAT); chlorophyll content and SB of Palmer amaranth at p < 0.005 and α = 0.05.
Table 1 Analysis of variance for plant height, chlorophyll content, and SB of Palmer amaranth among the sweetpotato varieties.
Based on the height reduction values, most of the sweetpotato varieties reduced Palmer amaranth height to a considerable extent compared to the control column (without sweetpotato) (Table 2).
Table 2 Mean height reduction (3, 4, and 5 WAT); chlorophyll reduction (3 WAT), and shoot biomass reduction values of Palmer amaranth in the presence of sweetpotato varieties.
The least significant difference (LSD) among means was calculated using the students-t-test (α = 0.05). Significantly different means were assigned the different letters. The mean variation for the traits recorded was also compared using the students- t-test and the variation for percent reduction in height (at 3, 4, and 5 WAT), chlorophyll content and SB of PA was significantly different in the presence of different varieties. Morado (74.66%), Bayou belle (61.85%), Vardaman (61.36%), 529 (59.03%), and Centennial (52.44%) significantly reduced PA’s height compared to the control after 3 weeks of transplanting (Figure 2A). On the other hand, the height of PA was only reduced by 27.23% in the presence of the commercial variety, Beauregard (B14). Morado (83.21%), 529 (76.21%), Centennial (75.39%), Heart-O-Gold (68.15%), and Hatteras (68.15%) exhibited a greater reduction in PA height at 4 WAT and this reduction increased even at 5 WAT (Figure 2B). Variety 529 showed a maximum (93.22%) reduction in PA height at 5 WAT, followed by Morado (93.08%), Heart-O-Gold (84.96%), and Centennial (81.49%).
Figure 2 Graphs representing PA’s (receiver plant) height reduction (%) at, (A) 3, 4 and 5 WAT; (B) 4 WAT; in presence of donor plant varieties of sweetpotato along the X-axis.
Variety 529 reduced Palmer amaranth chlorophyll content the most, followed by Centennial, Hatteras, and Heart-O-Gold (Figure 3A). Maximum shoot biomass reduction was observed in the presence of Hatteras (97.75%), followed by Evangeline (96.75%), Covington (95.82%), and 529 (91.65%) (Figure 3B).
Figure 3 Graphs representing PA’s reduction (%) in, (A) chlorophyll; (B) shoot biomass; in presence of donor plant varieties of sweetpotato along the X-axis.
The Ward’s method was used to cluster the varieties based on the traits recorded (height, chlorophyll, and shoot biomass reduction of Palmer amaranth). The dendrogram grouped all the varieties into two major clusters (I & II) consisting of 4 and 13 varieties, respectively (Figure 4). The four varieties (Heart-O-Gold, Centennial, 529, and Morado), which suppressed Palmer amaranth height by at least 68%, were clustered (cluster I) separately from the rest. Cluster II consisted of the remaining 13 varieties. Among the varieties in cluster II, B14 was the one with least reduction values for PA’s height, chlorophyll and SB, which made it group separately from the other varieties.
Figure 4 Clustering of the sweetpotato varieties using the Ward’s method. Cluster I (green color) consisted of varieties with the most weed-suppressive potential against Palmer amaranth, while cluster II (red color) consisted of the remaining 13 varieties that were less suppressive.
A PCA was also performed based on the variation among the traits recorded (Figure 5). The PCA indicated that the total variance in weed-suppressing traits was explained by the five principal components (PC). PC1 explained 68.03% of the total variation while PC2, PC3, PC4, and PC5 explained 19.66%, 8.86%, 2.40%, and 1.05% of the total variation, respectively. Similar to cluster analysis, PCA also clustered all varieties majorly in two groups. Group I consisted of four varieties, i.e., Heartogold, Centennial, 529, and Morado, with high growth-suppressive ability against Palmer amaranth. Group II consisted of the remaining varieties except for B14 with no allelopathic effect on Palmer amaranth and grouped separately.
Figure 5 Principle Component Analysis (PCA) with five components, Palmer amaranth’s (1) mean height reduction at 3 WAT (%), (2) mean height reduction at 4 WAT (%), (3) mean height reduction at 5 WAT (%), (4) mean chlorophyll reduction at 3 WAT (%), (5) mean chlorophyll reduction (%).
The demand for integrated weed management practice is increasing with time to reduce the reliance on chemicals. Excessive use of chemical herbicides has residual hazardous effects and causes resistance development in weeds. Allelopathy is an effective, eco-friendly approach of weed suppression that can reduce the dependency on herbicides. The effective use of allelopathic weed suppression could reduce the cultivation cost which ultimately leads to higher profit for the producer/farmer.
The use of the stair-step structure is an effective way to determine the allelopathic effect one plant species may have on the other as it eliminates competition and the influence of other plant species. Previously, this structure was used efficiently to study the allelopathic potential of Oryza sativa against Echinochloa crus-galli (Schumaker et al., 2020) and Gossypium hirsutum against A. palmeri (Fuller et al., 2021). Also, the use of sand instead of a soil/potting medium was helpful in eliminating any type of change in nutrient composition that could have changed the phenotype of the plants in the experiment (Camberato, 2007). Allelochemical production is controlled by both genetic and environmental factors (Xiong et al., 2007). Thus, the expression of the genes responsible for allelopathic impact greatly depends on the environmental conditions provided during the experiment (Kruse et al., 2000). The stair-step set-up and the greenhouse in our experiment have provided ideal conditions to identify the weed-suppressive effect of different sweetpotato varieties on PA.
Sweetpotato is one of the important crops cultivated in the southern states of the USA and is one of the most widely grown root crop in African countries like Nigeria, Madagascar, and Angola (Low et al., 2009). Varieties such as Centennial, 529, and Morado have already been reported to produce allelochemicals such as chlorogenic acid, caffeic acid, coumarin (Soni et al., 2019). These allelochemicals inhibited the growth of weeds in crops like Oryza sativa, Artemisia argyi (Khanh et al., 2007; Chen et al., 2021). A reduction in cowpea growth was reported when it was grown on the field following the sweetpotato due to the allelopathic effects of leaf litters and decaying residues from the sweetpotato (Dahiya et al., 2017). The weed-suppressive effect of sweetpotato on the bitter vine (Mikania micrantha) was studied by Shen and coworkers and they observed that the allelopathic impact varied with sweetpotato varieties (Shen et al., 2018b).
Allelopathic varieties with the potential to suppress weed growth can be useful for breeding cultivars designed for organic production systems. The present study reported for the first time that Hatteras exhibited allelopathic effects, which further paves the way for identifying allelochemicals associated with these varieties. Allelochemical production increases when the allelopathic crop varieties grow in close proximity of the competing weed plants (Chen et al., 2010; Kato-Noguchi, 2011).
Allelopathic weed suppression has already been used successfully in other crops like rice and sorghum. Several studies have shown a reduction in barnyardgrass (Echinochloa crus-galli) by allelochemicals produced by allelopathic rice (Oryza sativa) varieties (Zhao et al., 2005; Kong et al., 2006; Kato-Noguchi, 2011). Sorghum species release an allelochemical named Sorgoleone, which is released by specialized root hair cells also known as natural herbicide factories (Dayan et al., 2007; Głąb et al., 2017). A 90% weed control was observed in the wheat field by using root and leaf extracts containing sorgoleone in combination with a 50% field rate of mesosulfuron plus iodosulfuron (Khaliq et al., 2013). The allelopathic suppression of the growth and development of weeds such as alfalfa, yellow nutsedge, Palmer amaranth, bitter vine, have been demonstrated by using sweetpotato varieties (Harrison and Peterson, 1986; Varsha et al., 2021).
The results of the cluster analysis and PCA have complemented each other by grouping the varieties with significant Palmer amaranth-suppressing ability, i.e., Heart-O-Gold, Centennial, 529, and Morado, into one cluster and the remaining in another. Clustering the varieties with similar weed suppressive potential assists the researcher to have a better understanding of the breeding material for crop improvement. Similar strategies have been previously used to cluster cotton (Fuller et al., 2021) and rice (Schumaker et al. 2020) varieties with allelopathic weed-suppressing abilities.
The reduction in the height of weed plants is one of the essential morphological criteria in determining the allelopathic effect of sweetpotato against weeds. Sweetpotato varieties 529, Morado, Centennial, and Hatteras were demonstrated to have an allelopathic effect on Palmer amaranth weed. Our work represents the first study to show that Hatteras has allelopathic properties against PA. Field trials need to be conducted to study the allelopathic effect of these varieties under natural environmental conditions. The quantification of allelochemicals and identification of gene(s) responsible for producing those allelochemicals will be of prime importance. The genes may aid in the commercial synthesis and production of allelochemicals, which could be used as potential bioherbicides promoting sustainable weed management.
The original contributions presented in the study are included in the article/supplementary material. Further inquiries can be directed to the corresponding author.
VS conducted the research, collected data, analyzed the data, and wrote the original manuscript. T-MT designed the experiment. T-MT, MS, CM, and SM supervised the research and reviewed the manuscript. WS and MGF assisted in the greenhouse work and gave their suggestions in the manuscript.
Funding for this project was provided by the Mississippi Department of Agriculture and Commerce/United States Department of Agriculture Specialty Crop Block Grant Program. This material is based upon work that is supported by the National Institute of Food and Agriculture, US Department of Agriculture, Hatch project under accession number 230100.
The authors declare that the research was conducted in the absence of any commercial or financial relationships that could be construed as a potential conflict of interest.
All claims expressed in this article are solely those of the authors and do not necessarily represent those of their affiliated organizations, or those of the publisher, the editors and the reviewers. Any product that may be evaluated in this article, or claim that may be made by its manufacturer, is not guaranteed or endorsed by the publisher.
We acknowledge the MSU Flatwoods Branch Experiment Station for kindly providing the sweetpotato cultivars and lines to be evaluated in this study. We also acknowledge the broad assistance provided by the students and staff of the Weed Physiology Lab at MSU.
Alsaadawi I. S., Sarbout A. K., Al-Shamma L. M. (2012). Differential allelopathic potential of sunflower (Helianthus annuus l.) genotypes on weeds and wheat (Triticum aestivum l.) crop. Arch. Agron. Soil Sci. 58 (10), 1139–1148. doi: 10.1080/03650340.2011.570335
Barkley S. L., Chaudhari S., Jennings K. M., Schultheis J. R., Meyers S. L., Monks D. W. (2016). Fomesafen programs for palmer amaranth (Amaranthus palmeri) control in sweetpotato. Weed. Technol. 30 (2), 506–515. doi: 10.1614/WT-D-15-00150.1
Camberato J. J. (2007). Cation exchange capacity-everything you want to know and much more. Magnesium 2 (24), 240.
Chen L., Li J., Zhu Y., Guo L., Ji R., Miao Y., et al. (2021). Caffeic acid, an allelochemical in artemisia argyi, inhibits weed growth via suppression of mitogen-activated protein kinase signaling pathway and the biosynthesis of gibberellin and phytoalexin. Front. Plant Sci. 12, 802198–802198. doi: 10.3389/fpls.2021.802198
Chen K. J., Zheng Y. Q., Kong C. H., Zhang S. Z., Li J., Liu X. G. (2010). 2, 4-Dihydroxy-7-Methoxy-1, 4-Benzoxazin-3-One (DIMBOA) and 6-Methoxy-Benzoxazolin-2-One (MBOA) levels in the wheat rhizosphere and their effect on the soil microbial community structure. J. Agric. Food Chem. 58 (24), 12710–12716. doi: 10.1021/jf1032608
Comont D., Hicks H., Crook L., Hull R., Cocciantelli E., Hadfield J., et al. (2019). Evolutionary epidemiology predicts the emergence of glyphosate resistance in a major agricultural weed. New Phytol. 223 (3), 1584–1594. doi: 10.1111/nph.15800
Culpepper A. S., Webster T. M., Sosnoskie L. M., York A. C., Nandula V. K. (2010). Glyphosate-resistant palmer amaranth in the united states. glyphosate resistance in crops and weeds: History, development, and management (Hoboken, NJ: John Wiley and Sons), 195–212.
Dadkhah A. (2015). Allelopathic potential of canola and wheat to control weeds in soybean (Glycine max). Russian agricultural sciences 41 (2), 111–114.
Dahiya S., Kumar S., Khedwal R. S., Jakhar S. R. (2010). Allelopathy for sustainable weed management. J. Pharmacognosy Phytochemistry 6, 832–837.
Dayan F. E., Watson S. B., Nanayakkara N. D. (2007). Biosynthesis of lipid resorcinols and benzoquinones in isolated secretory plant root hairs. J. Exp. Bot. 58 (12), 3263–3272. doi: 10.1093/jxb/erm173
Duque L. O., Sánchez E., Pecota K., Yencho C. (2022). A win–win situation: Performance and adaptability of petite sweetpotato production in a temperate region. Horticulturae 8 (2), 172. doi: 10.3390/horticulturae8020172
Finney D. M., Creamer N. (2008). Weed management on organic farms (Raleigh, NC, USA: North Carolina Cooperative Extension Service), 34.
Fuller M. G., Saha S., Stelly D. M., Jenkins J. N., Tseng T. M. (2021). Assessing the weed-suppressing potential of cotton chromosome substitution lines using the stair-step assay. Plants 10 (11), 2450. doi: 10.3390/plants10112450
Głąb L., Sowiński J., Bough R., Dayan F. E. (2017). Allelopathic potential of sorghum (Sorghum bicolor (L.) moench) in weed control: A comprehensive review. Adv. Agron. 145, 43–95. doi: 10.1016/bs.agron.2017.05.001
Hammer O., Harper D. A., Ryan P. D. (2001). PAST: Paleontological statistics software package for education and data analysis. Palaeontol. Electron. 4 (1), 9.
Harrison H. F., Peterson J. K. (1986). Allelopathic effects of sweetpotatoes (Ipomoea batatas) on yellow nutsedge (Cyperus esculentus) and alfalfa (Medicago sativa). Weed. Sci. 34 (4), 623–627. doi: 10.1017/S0043174500067552
Kakwani N., Son H. H. (2016). Measuring food insecurity: Global estimates. in social welfare functions and development (London: Palgrave Macmillan), 253–294.
Kato-Noguchi H. (2011). Barnyard grass-induced rice allelopathy and momilactone b. J. Plant Physiol. 168 (10), 1016–1020. doi: 10.1016/j.jplph.2010.12.021
Khaliq A., Shakeel M., Matloob A., Hussain S., Tanveer A., Murtaza G. (2013). Influence of tillage and weed control practices on growth and yield of wheat. Philippine. J. Crop Sci. (PJCS). 38 (3), 00–00.
Khanh T. D., Xuan T. D., Chung I. M. (2007). Rice allelopathy and the possibility for weed management. Ann. Appl. Biol. 151 (3), 325–339. doi: 10.1111/j.1744-7348.2007.00183.x
Khan E. A., Khakwani A. A., Munir M. (2015). Effects of allelopathic chemicals extracted from various plant leaves on weed control and wheat crop productivity. Pakistan J. Bot. 47 (2), 735–740. doi: 10.1111/j.1744-7348.2007.00183.x
Kong C. H., Chen X. H., Hu F., Zhang S. Z. (2011). Breeding of commercially acceptable allelopathic rice cultivars in China. Pest Manage. Sci. 67 (9), 1100–1106. doi: 10.1002/ps.2154
Kong C. H., Li H. B., Hu F., Xu X. H., Wang P. (2006). Allelochemicals released by rice roots and residues in soil. Plant Soil 288 (1), 47–56. doi: 10.1007/s11104-006-9033-3
Kruse M., Strandberg M., Strandberg B. (2000). Ecological effects of allelopathic plants-a review. NERI. Tech. Rep. 315:35–49.
Legleiter T. R., Bradley K. W. (2008). Glyphosate and multiple herbicide resistance in common waterhemp (Amaranthus rudis) populations from Missouri. Weed. Sci. 56 (4), 582–587. doi: 10.1614/WS-07-204.1
Li Z. H., Wang Q., Ruan X., Pan C. D., Jiang D. A. (2010). Phenolics and plant allelopathy. Molecules 15 (12), 8933–8952. doi: 10.3390/molecules15128933
Low J., Lynam J., Lemaga B., Crissman C., Barker I., Thiele G., et al. (2009). “Sweetpotato in Sub-Saharan Africa,” in The sweetpotato (Springer publication (USA): Springer, Dordrecht), 359–390.
Meyers S. L., Jennings K. M., Schultheis J. R., Monks D. W. (2010). Interference of palmer amaranth (Amaranthus palmeri) in sweetpotato. Weed. Sci. 58 (3), 199–203. doi: 10.1614/WS-D-09-00048.1
Morgan G. D., Baumann P. A., Chandler J. M. (2001). Competitive impact of palmer amaranth (Amaranthus palmeri) on cotton (Gossypium hirsutum) development and yield. Weed. Technol. 15 (3), 408–412. doi: 10.1614/0890-037X(2001)015[0408:CIOPAA]2.0.CO;2
Norsworthy J. K., Ward S. M., Shaw D. R., Llewellyn R. S., Nichols R. L., Webster T. M., et al. (2012). Reducing the risks of herbicide resistance: Best management practices and recommendations. Weed. Sci. 60 (SP1), 31–62. doi: 10.1614/WS-D-11-00155.1
Nwosisi S., Nandwani D., Hui D. (2019). Mulch treatment effect on weed biomass and yields of organic sweetpotato cultivars. Agronomy 9 (4), 190. doi: 10.3390/agronomy9040190
Rice E. L. (2012). Allelopathy. Second edition, Physiological ecology, Academic press, INC. Harcourt Brace Jovanovich, USA
Rizvi S. J. (2012). Allelopathy: Basic and applied aspects (Berlin/Heidelberg, Germany: Springer Science and Business Media).
Schumaker B. C., Stallworth S., De Castro E., Fuller M. G., Shrestha S., Tseng T. M. (2020). Repeatable stair-step assay to access the allelopathic potential of weedy rice (Oryza sativa ssp.). MyJoVE. Corp (155), e60764. doi: 10.3791/60764.
Shen S., Xu G., Li D., Clements D. R., Jin G., Liu S., et al. (2018b). Allelopathic effects of three sweetpotato cultivars (Ipomoea batatas) on the invasive plant mikania micrantha. Pakistan J. Biol. Sci. PJBS. 21 (1), 8–15. doi: 10.3923/pjbs.2018.8.15
Seng C. T., Van Lun L. O.W., San C. T., SAHID I.B. (2010). Initial report of glufosinate and paraquat multiple resistance that evolved in a biotype of goosegrass (Eleusine indica) in Malaysia. Weed Biology and Management. 10 (4), 229–223. doi: 10.1111/j.1445-6664.2010.00388.x
Shen S., Xu G., Li D., Clements D. R., Jin G., Liu S., et al. (2018a). Allelopathic potential of sweetpotato (Ipomoea batatas) germplasm resources of yunnan province in southwest China. Acta Ecol. Sin. 38 (6), 444–449. doi: 10.1016/j.chnaes.2018.03.002
Smith S. C., Jennings K. M., Monks D. W., Chaudhari S., Schultheis J. R., Reberg-Horton C. (2020). Critical timing of palmer amaranth (Amaranthus palmeri) removal in sweetpotato. Weed. Technol. 34 (4), 547–551. doi: 10.1017/wet.2020.1
Soni B., Tseng T. M. P., Yue Z. (2019). Identification and quantification of allelochemicals from selected sweetpotato (Ipomoea batatas (L.) lam.) cultivars. Am. J. Plant Sci. 10 (12), 2354.
Treadwell D. D., Creamer N. G., Schultheis J. R., Hoyt G. D. (2007). Cover crop management affects weeds and yield in organically managed sweetpotato systems. Weed. Technol. 21 (4), 1039–1048. doi: 10.1614/WT-07-005.1
USDA National Agricultural Statistics Service. Available at: https://data.nal.usda.gov/dataset/nass-quick-stats.
Varsha V., Werle I. S., Shankle M. W., Tseng T. (2021). Allelopathic effects of sweetpotato varieties on palmer amaranth growth. In. Proc. South. Weed. Sci. Soc. 74, 18.
Vithu P., Dash S. K., Rayaguru K. (2019). Post-harvest processing and utilization of sweetpotato: A review. Food Rev. Int. 35 (8), 726–762.
Walker S., Widderick M., McLean A., Cook T., Davidson B. (2013). Improved chemical control of c onyza bonariensis in wheat limits problems in the following fallow. Weed. Biol. Manage. 13 (4), 144–150. doi: 10.1111/wbm.12021
Webster T. M., Nichols R. L. (2012). Changes in the prevalence of weed species in the major agronomic crops of the southern united states: 1994/1995 to 2008/2009. Weed. Sci. 60 (2), 145–157. doi: 10.1614/WS-D-11-00092.1
Xiong J., Jia X., Deng J., Jiang B., He F., Lin W. (2007). Analysis of epistatic effect and QTL interactions with environment for allelopathy in rice (Oryza sativa l.). Allelopathy. J. 20 (2), 259–267.
Keywords: Amaranthus palmeri, cluster analysis, Ipomoea batatas, shoot biomass reduction, stair-step, sustainable weed management
Citation: Singh V, Segbefia W, Fuller MG, Shankle MW, Morris CJ, Meyers SL and Tseng T-M (2022) Allelopathy: an eco-friendly approach to control palmer amaranth using allelopathic sweetpotato. Front. Agron. 4:930378. doi: 10.3389/fagro.2022.930378
Received: 27 April 2022; Accepted: 28 June 2022;
Published: 19 July 2022.
Edited by:
Bhagirath Singh Chauhan, The University of Queensland, AustraliaReviewed by:
Qaiser Javed, Jiangsu University, ChinaCopyright © 2022 Singh, Segbefia, Fuller, Shankle, Morris, Meyers and Tseng. This is an open-access article distributed under the terms of the Creative Commons Attribution License (CC BY). The use, distribution or reproduction in other forums is permitted, provided the original author(s) and the copyright owner(s) are credited and that the original publication in this journal is cited, in accordance with accepted academic practice. No use, distribution or reproduction is permitted which does not comply with these terms.
*Correspondence: Te-Ming Tseng, dHQxMDI0QG1zc3RhdGUuZWR1
Disclaimer: All claims expressed in this article are solely those of the authors and do not necessarily represent those of their affiliated organizations, or those of the publisher, the editors and the reviewers. Any product that may be evaluated in this article or claim that may be made by its manufacturer is not guaranteed or endorsed by the publisher.
Research integrity at Frontiers
Learn more about the work of our research integrity team to safeguard the quality of each article we publish.