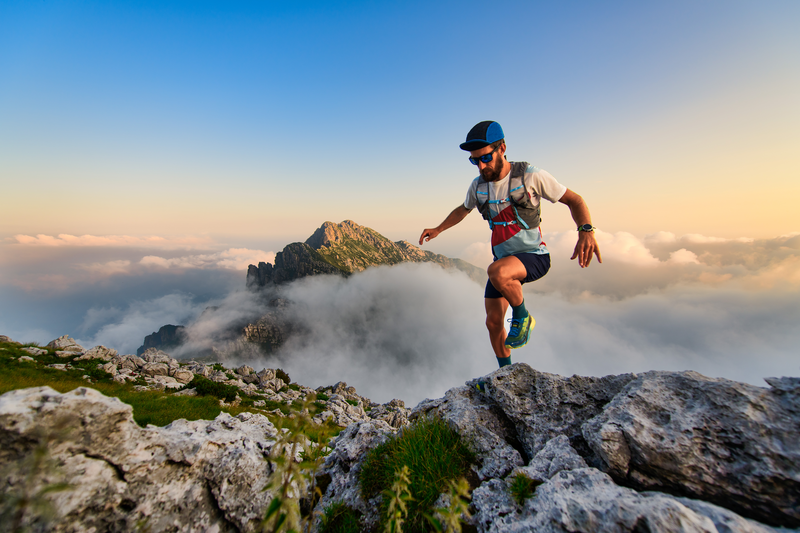
94% of researchers rate our articles as excellent or good
Learn more about the work of our research integrity team to safeguard the quality of each article we publish.
Find out more
PERSPECTIVE article
Front. Agron. , 22 July 2022
Sec. Agroecological Cropping Systems
Volume 4 - 2022 | https://doi.org/10.3389/fagro.2022.911014
Integrating livestock into cropping systems may enhance ecosystem services while still providing efficient food production. Including tanniferous forages in crop-livestock systems could further enhance ecosystem services. Interest in phytochemicals, such as tannins, has increased over the past several decades, and research continues to reveal the potential benefits of tannins in agricultural systems. However, research evaluating the influence of tanniferous forages in integrative crop-livestock systems is limited. We discuss how tannins influence soil microbial dynamics and nutrient cycling, the function of tannins in forages, and the role tannins have in improving the health of foraging animals. We speculate on potential advantages for human health from consumption of animal-based foods from animals that consumed tanniferous forages or supplemental plant materials. Expanding our knowledge and integration of phytochemicals in muti-faceted agroecosystem research and utilizing their influence in agriculture system dynamics may be an effective tool to enhance agroecological sustainability.
● Integrative crop-livestock systems may enhance agricultural sustainability
● Tannins offer agroecological benefits from the soil to the atmosphere
● Tanniferous forages may reduce input requirements in crop-livestock systems
Plants must adapt to evolving environmental stresses through both physical (spines, thorns, etc.) and chemical mechanisms. Through a variety of chemical pathways, plants produce thousands of phytochemicals which they use to interact with their environment in several ways (Pichersky and Gershenzon, 2002; Theis and Lerdau, 2003; Bezemer and van Dam, 2005; Laothawornkitkul et al., 2009; Macel et al., 2010; Macel and van Dam, 2018). Based on biosynthesis, there are three major groups of plant secondary metabolites: 1) terpenoids, 2) alkaloids, and 3) phenylpropanoids and phenolic compounds (Croteau et al., 2000). Phenolic compounds, which include tannins, are widespread throughout the plant kingdom (Croteau et al., 2000), and their role in agroecosystems is diverse. Phenolic compounds influence soil nutrient cycling, enhance the ability of plants to cope with environmental stress, and improve ruminant digestion (Dixon, 2001; Mueller-Harvey, 2006; Smolander et al., 2012).
In general, tannins and other phenolic secondary metabolites are more prevalent in perennial trees and bushes compared to grasses and agricultural plants (Haslam, 1981; Aerts et al., 1999). Tannins may account for a significant proportion of leaf, bark or even root biomass (20-60%), (Baldwin and Schultz, 1984; Kuiters, 1990; Matthews et al., 1997; Hassanpour et al., 2011) but they can be found in some crops and forages such as sorghum and various legumes (Nyachoti et al., 1997; Aerts et al., 1999; Hassanpour et al., 2011; Sharma et al., 2019; Hill and Roberts, 2020).
Enhancing agroecological sustainability involves multiple approaches including increasing plant biodiversity and reducing inputs such as fertilizers and herbicides for forage and/or crop production, and anthelmintics for animal production. Although a subject of research for more than sixty years (e.g., Coulson et al., 1960; Handley, 1961), development of strategies for the use of tannins as management tools in agroecosystems has lagged, in part because studies have only recently attempted to address their synergistic effects on soil, crops, animals and ultimately humans (Maughan et al., 2014; Miele et al., 2019; Clemensen et al., 2020; Slebodnik, 2020). Here, we speculate that integrating crop-livestock systems with plant species containing phenolic compounds such as tannins may reduce the need for external inputs, as we discuss the role of tannins in soil, plants, and livestock. We propose that the incorporation of these species benefits agronomic systems, and that potential research be expanded to include the incorporation of tannin-containing plant species in future agroecosystem research.
The potential for tannins and other phenolic substances to affect important biogeochemical processes in soil such as organic matter formation and nutrient cycling has been widely appreciated (Adamczyk et al., 2018; Crush and Keogh, 1998; Horner et al., 1988; Kuiters, 1990; Hättenschwiler and Vitousek, 2000; Kraus et al., 2003a; von Lützow et al., 2006). While early tannin research was conducted on temperate agricultural soils (Basaraba, 1964; Basaraba and Starkey, 1966; Lewis and Starkey, 1968), much of what we know about their effects in soil has come from studies in forest ecosystems (Rice and Pancholy, 1973; Kuiters, 1990; Kraus et al., 2003a; Kraus et al., 2004; Joanisse et al., 2007; Joanisse et al., 2009; Kraal et al., 2009) and tropical soils (Sivapalan et al., 1983; Sivapalan et al., 1985; Mutabaruka et al., 2007; Coq et al., 2010; Ushio et al., 2013). These studies have tended to emphasize the impacts of tannins on microbially mediated processes.
Tannins enter soil from aboveground living or dead plant material, animal manure or from roots (Castells et al., 2005; Crush and Keogh, 1998; Gallet and Lebreton, 1995; Schofield et al., 1998; Kraus et al., 2003a; Meier et al., 2008) in complex patterns influenced by environmental conditions and interactions between plants, microorganisms, and soil (Suseela et al., 2016; Top et al., 2017; Solaiman and Senoo, 2018; Arnoldi et al., 2020). Accurate estimates of phenolic inputs (including tannins) to soil from cultivated crops are notably lacking but in natural systems are reported to range between 0.33-0.65 oz ft-2 year-1 (Hättenschwiler et al., 2003; Tharayil et al., 2013). Together with non-tannin phenolic substances, they comprise a substantial, dynamic, flux of C substrates in the soil that affect numbers, diversity, and functioning of soil biota.
Interactions between soil microorganisms and tannins that affect organic matter formation and nutrient cycling are complex and influenced by the characteristics of the tannins (Fierer et al., 2001; Kraus et al., 2004; Norris et al., 2011; Schmidt et al., 2013b), plant genetics (Schweitzer et al., 2005; Schweitzer et al., 2008; Selmants et al., 2019), and may vary among different soil microbial groups (e.g. Souto et al., 2000; Selvakumar et al., 2007; Ushio et al., 2013; Zhang and Laanbroek, 2020). Tannins can directly inhibit microbial processes (Scalbert, 1991; Field and Lettinga, 1992; Bhat et al., 1998; Cowan, 1999; Selvakumar et al., 2007; Buzzini et al., 2008) but may also be utilized as a substrate by soil microorganisms resulting in short-term immobilization of soil nitrogen (Kraus et al., 2003a; Kraus et al., 2004; Castells, 2008; Talbot and Finzi, 2008; Schmidt et al., 2013b). Tannins may also influence nutrient cycling by inactivating enzymes (Benoit and Starkey, 1968; Field and Lettinga, 1992; Joanisse et al., 2007; Triebwasser et al., 2012) or by forming occluded tannin–protein complexes (Rillig et al., 2007; Joanisse et al., 2009; Wurzburger and Hendrick, 2009; Adamczyk et al., 2019; Makarov, 2019; Chen et al., 2020).
Traditionally the defining characteristic of tannins is their ability to form crosslinked, complexes with protein (Hagerman, 2012). However, tannins are also known to complex with other organic N compounds including arginine, nitrogen bases, polyamines, chitin, chitosan and glomalin (Halvorson and Gonzalez, 2006; Adamczyk et al., 2011). Complexation with enzymes is widely thought to inhibit enzyme activity but conversely low concentrations of tannins may increase enzyme activity (Adamczyk et al., 2017).
Tannins may also directly affect important abiotic processes in soils, depending in part upon specific structural characteristics, forming complexes with a variety of organic and inorganic fractions and participating important chemical reactions like chelation and oxidation/reduction (Appel, 1993; Kraus et al., 2003b; Kaal et al., 2005; Salminen et al., 2011; Schmidt et al., 2012; Schmidt et al., 2017; Subdiaga et al., 2020). Tannins have been found to rapidly affect soil C/N dynamics (Halvorson and Gonzalez, 2008; Halvorson et al., 2009; Halvorson et al., 2012), ion exchange capacity (Halvorson et al., 2011; Halvorson et al., 2013), and solubility of macro and micronutrients in soil and manure (Halvorson et al., 2013; Schmidt et al., 2013a; Halvorson et al., 2016; Halvorson et al., 2017; Schmidt et al., 2017). These studies provide some understanding of initial abiotic interactions between a few specific tannins and soil but very little is known of the effects of combinations of tannins or other phenolic compounds, for example as a result of crop rotations or intercropping, or how their effects might vary as the result of degradation by chemical and biological factors in the soil. In addition, marked differences between polyphenolic tannins and their monomers observed in the above studies suggest tannin inputs that initially added C and helped conserve N in soil could degrade into compounds that would have no effect or might even increase mobility of plant nutrients. Little information is available about how innate or manageable soil characteristics can alter the effects of tannins, though there is some evidence that inorganic soil solution constituents such as pH can alter the charge, size, and conformation of dissolved organic molecules (like tannins), thus affecting their environmental behavior (Dultz et al., 2020). Importantly, not considered here are the inducible plant-soil interactions that result in formation and exudation of tannins or related phenolic compounds in the soil in response to external stressors such as infertility, competition, pathogens, or toxic conditions (Kinraide and Hagerman, 2010; Zhang et al., 2016).
Probably least understood are possible management implications of tannins on the physical qualities of agricultural soil linked to important attributes such as compaction, aggregate formation, and water infiltration. Tannins have many industrial applications that provide hints as to their potential for management of soil physical characteristics (Shirmohammadli et al., 2018; Binti et al., 2019; Pizzi, 2019; Singh and Kumar, 2019; Das et al., 2020). Currently, commercially available formulations containing tannins are used to improve road surfaces and added to drilling muds and casting sands to improve their physical characteristics (Ismail et al., 2020). In soil, interactions between tannins, polysaccharides and proteins or possibly other proteinaceous compounds have been reported to increase the stability of soil aggregates (Griffiths and Burns, 1972; Erktan et al., 2017) and affect soil plasticity. Sorgho et al. (2014) observed binding between tannin compounds and iron hydroxides on clay surfaces that enhanced compressive strength of clay.
Plants produce various phytochemicals to enhance their survival with continually changing environments (i.e., Pearce et al., 1991; Turlings et al., 1995; Strzalka et al., 2003; González-Lamothe et al., 2009; Agati and Tattini, 2010; Santana et al., 2010; Bruce and Pickett, 2011; Leiss et al., 2011; Pierik et al., 2014; Savatin et al., 2014). Among these are phenolic compounds, including tannins, which are structured with at least one hydroxyl functional group on an aromatic ring and are further divided into either flavonoids or non-flavonoids (Crozier et al., 2001). Flavan-3-ols are a subclass of flavonoids and are the most structurally complex group, which include monomers catechin, epicatechin, and the oligomeric and polymeric proanthocyanidins (Crozier et al., 2001).
Tannins may be found in leaves, seeds, fruits, bark, and glandular trichomes acting primarily as deterrents to pathogens, insects, and larger herbivores, while also having allelopathic effects on other plants (Dixon et al., 2004; Agrawal and Weber, 2015). Further, phenolic compounds may reduce photodamage through antioxidant capacity, as excess light energy beyond photosynthetic necessities creates damaging oxidative byproducts (Close and McArthur, 2002; Kliebenstein, 2004). The abundance of tannins in nature is coupled with the complexity of examining them, as the precise form and structure of tannins is not only species-specific but differs within individual plants. Tannin structural diversity and function is discussed extensively in the literature (i.e., Dixon et al., 2004; Xie and Dixon, 2005; Salminen and Karonen, 2011; Adamczyk et al., 2017). Here, we briefly highlight circumstances in which tanniferous forages exhibit fluctuation of these carbonaceous metabolites in changing environments.
In general, stress (i.e., heat, drought, injury) typically increases production of phytochemicals, enhancing the survivability of plants (Akula and Ravishankar, 2011; Tharayil et al., 2011; Savatin et al., 2014; Isah, 2019). Sainfoin (Onobrychis viciifolia Scop.), a productive leguminous forage, increases production of condensed tannins (CT) with limited water availability during vegetative growth, while also reducing presence of sainfoin rust (Uromyces onobrychidis (Desmazières Léveillé) with increased CT concentration (Malisch et al., 2016). This exemplifies the adaptability of sainfoin to water stress by increasing CT concentration, and how the presence of CT appears to protect sainfoin from fungal pathogens. Although big trefoil (Lotus pendiculatus Cav.) showed increased CT concentration with higher temperatures and limited water (Anuraga et al., 1993), birdsfoot trefoil (Lotus corniculatus L.) had lower CT in leaves, stems and roots when water stressed, and lower CT concentrations with higher temperatures (Carter et al., 1999).
In conditions of high fertility, phenolics such as tannins typically decrease (Lavola et al., 2003), whereas infertile sites seem to result in increased phenolics (Chapin et al., 1986; Northup et al., 1998). This could be due to the influence of these compounds on soil nutrient cycling discussed in the previous section (i.e., Kraus et al., 2003; Kraus et al., 2003b), or to resource allocation. The physiological shift in phenolic production induced by environmental stress is not well understood, and the implications for how to manage systems for phenolics is largely void.
Condensed tannins are known to bind with molecules such as proteins, while hydrolysable tannins (HT) are more oxidatively active, and this distinction depends largely on pH (Salminen and Karonen, 2011), whether in soil or in the gut of an herbivore. Phenolic compounds such as tannins show potential to defend plants against insect defoliation (Schultz, 1989) and other pathogens (Scalbert, 1991) which may reduce insecticidal input needs, while at the same time offering foraging advantages to larger herbivores. Since insect midguts have higher pH where oxidatively active tannins may hinder insect defoliation (Barbehenn et al., 2008; Barbehenn et al., 2009; Barbehenn and Constabel, 2011).
Mammalian herbivore intestines have lower pH, where protein precipitation occurs with CT, benefitting ruminant utilization of protein. In addition, several perennial forages contain phenolics (Cowan, 1999; Allison et al., 2009), including tannins, which have antibacterial, fungicidal, and antiviral effects (Scalbert, 1991; Cowan, 1999; Maddox et al., 2010). Purple prairie clover (Dalea purpurea Vent.), a grazing-tolerant and highly nutritious legume, contains high concentrations of CT which have antimicrobial activity (Hufford et al., 1993; Jin et al., 2015).
At the right dose, phytochemicals offer various benefits to ruminants and other mammals, including humans. Phenolic compounds have anticarcinogenic qualities, antioxidative properties, and in ruminants may reduce parasite load, improve protein digestion, and can reduce enteric methane emission (Waghorn et al., 1994; Barry and McNabb, 1999; Villalba and Provenza, 2007; Waghorn, 2008; Villalba et al., 2013; Stewart et al., 2019). However, tannin concentration above 7% in animal diets have shown detrimental health effects on herbivores and can reduce desired nutrient assimilation and consequential animal performance (Barry and McNabb, 1999: Douglas et al., 1995; Waghorn et al., 1994; Mueller-Harvey, 2006; Min et al., 2012).
Anticarcinogenic properties of phenolic compounds (Ren et al., 2003) have been shown in many human in vivo and in vitro studies (Knekt et al., 2002), though they have not been extensively investigated in ruminants. Flavonoids quench phase I and phase II metabolizing enzymes (e.g., cytochrome P450), which activate procarcinogens to intermediates that ultimately trigger carcinogenesis. Flavonoids inhibit the activities of certain P450 isozymes such as CYP1A1 and CYP1A2, which then inactivate specific procarcinogens by signaling their removal through metabolic pathways (Bu-Abbas et al., 1998; Lahiri-Chatterjee et al., 1999; Le Marchand et al., 2000). Phenolic compounds act in vivo to neutralize excess free radicals in the body (Balasundram et al., 2006; Agati et al., 2012; Katiki et al., 2013), signal internal antioxidant mechanisms and improve immune system function. Antioxidant levels are also an indicator of flavonoids that can potentiate commercial anthelmintics. The antioxidant flavonoid quercetin was reported to increase anthelmintic activity of moxidectin in lambs (Dupuy et al., 2003; Novobilský et al., 2013).
Hydrolyzable tannins and CT form complexes with carbohydrate and nitrogenous compounds and were previously thought to reduce intake and have negative effects on digestibility and animal performance, though only at high levels of tannin concentration (> 10%) (McSweeney et al., 2001; Beauchemin et al., 2008). However, research using moderate level tannins increased forage and nutrient consumption when sheep and/or cattle ingested a tanniferous legume with endophyte-infected tall fescue (Lyman et al., 2008; Lyman et al., 2011; Lyman et al., 2012; Owens et al., 2012a; Owens et al., 2012b), presumably because CT bind with alkaloids such as ergovaline (Clemensen et al., 2018).
Polyphenolic compounds can both deter or enhance the absorption of amino acids in the small intestine, depending on dose and structure (Hagerman and Butler, 1981; Barry and McNabb, 1999). When fed to ruminants at a moderate level, CT blocking of hydrolysis deters enzymatic degradation of proteins in the rumen and consequently alters N excretion (Min et al., 2012). When protein digestion is reduced in the rumen, there is less degradation to urea. Excessive urea requires energy for the animal to excrete, hence when N waste is reduced, energy can be directed for growth. Some protein-tannin complexes are reversed in the abomasum or true stomach, then absorbed and utilized as amino acids in the small intestine. For example, feeding the HT gallic acid to beef cattle altered the pattern of N excretion (Wei et al., 2016).
Tannins can also decrease enteric methane (CH4) production from ruminants (Goel and Makkar, 2011; Yang et al., 2017). Tannins inhibit CH4 production either from direct effects on methanogenic archaea, indirect effects on protozoal methanogen associated CH4 production, and/or reduction of fiber digestion (Patra and Saxena, 2011) due to carbohydrate bonding. An in vitro study showed that HT did not decrease forage degradability and was more effective in reducing enteric CH4 emission than CT (Jayanegara et al., 2010). The effects of HT on microbes and CH4 emissions most likely depend on the core unit structure (McAllister et al., 2005; Tavendale et al., 2005). Hydrolysable tannins have a monosaccharide central core that is partially or totally esterified with gallic acid or ellagic acid (Patra and Saxena, 2011). Though methane is often reduced, concurrent reduction of fiber digestion can reduce feed efficiency. Both positive and negative results on production were shown when methane was reduced, and both alternatives can contribute to ruminant health (Aboagye and Beuchemin, 2019). Future research in agroecosystems that incorporate livestock could involve the titration of appropriate tannin concentrations to enhance livestock health while providing other benefits to the environment, thereby increasing sustainability and enhancing environmental services of agroecosystems.
Research suggests that consuming foods containing phenolic compounds may have health benefits to humans (Cowan, 1999; Figueroa-Espinoza et al., 2015; Tang and Tsao, 2017; Pang et al., 2018), and phenolic compounds are found in spring and winter wheat (Zuchowski et al., 2011), rice (Kesarwani et al., 2014) and several other grains (Dykes and Rooney, 2007). There is increasing interest in how the meat and/or milk from animals foraging phytochemically-rich plants might affect human health. Consumption of red meat may create harmful lipid peroxidation products in the body such as malondialdehyde, which can be found in the blood after a meal of red meat (Gorelik et al., 2008). However, consumption of red wine or coffee (both containing polyphenolics) along with red meat can reduce production of malondialdehyde and therefore reduced modification of low-density lipoprotein, which is associated with formation of atherosclerotic plaque (Gorelik et al., 2013; Sirota et al., 2013). This may suggest that intake of tannins and other phenolic compounds by animals may make their milk and meat healthier for people, though this is still speculative and warrants continued effort to elucidate direct effects on human health. We are unaware of any research trials that focus on human response to intake of milk and/or meat that contains higher concentrations of phenolics. Additionally, it is difficult to determine their healthfulness in milk and meat because concentrations of other compounds such as fatty acids are often changed simultaneously by the specific plant materials consumed by animals. Nonetheless, concentrations of phenolics increase in milk and meat when animals consume plants and plant material containing phenolic compounds and are higher in milk and meat when consumed if milk is not pasteurized and meat is not grilled, roasted or cooked with high heat (Frutos et al., 2020; van Vliet et al., 2021). For example, supplemental quebracho tannin intake led to greater concentration of phenolics in muscle, greater color stability, higher muscle antioxidant capacity, and greater resistance of myoglobin to oxidation in lamb meat (Luciano et al., 2011). Maughan et al. (2014) found better meat quality from cattle grazing tanniferous sainfoin compared to alfalfa, showing greater marbling scores, back-fat thickness, and more desirable coloring, yet there were no differences in consumer preference.
Concentrations of some phenolic compounds can be high in milk and liver (López-Andrés et al., 2013). Compared to people who eat only the muscle of animals, people that eat liver from forage-consuming cattle, sheep and/or goats may obtain more phenolic compounds especially if the forage is immature and fresh. Detailed research on human health benefits from milk or meat of livestock grazing tannin containing forages is warranted, especially as agroecosystems begin to incorporate alternative forages that contain tannins to provide other system benefits.
Integrative crop-livestock systems may address several ecosystem services while continuing to produce food for an expanding human population (Costa et al., 2014; Franzluebbers and Stuedemann, 2014; Kunrath et al., 2014; Lemaire et al., 2014). Diversifying our cropping systems to include tanniferous pasture forages offers potential ecosystem benefits from the soil to the atmosphere. For instance, the presence of a tanniferous N-fixing legume seeded into grain crop production increases plant available N for the grain crop, while the tannin-containing forage may help deter insect herbivory, thereby enhancing the resilience of both crops. In the soil, the presence of tannins inhibits N mineralization, retaining more N in the soil and thereby increasing C sequestration potential as it expands microbial biomass in the soil.
Ruminant livestock grazing tanniferous forages benefit from CT by preventing protein degradation in the rumen, which may increase animal productivity. The reduction of protein degradation can potentially improve environmental sustainability of integrated crop livestock systems by decreasing N excretion from ruminants (McMahon et al., 1999), reduce methane emissions which improve system carbon sequestration of both the livestock and the system itself (Waghorn et al., 2002; Thornton and Herrero, 2010), and decrease manure nitrous oxide emissions (Waghorn and McNabb, 2003). These same benefits have been observed when tanniferous legume hay and silage has been fed (Christensen et al., 2015; Broderick et al., 2017) though with reduced effect compared with fresh forages. Regardless of the state of the forages (fresh or dried), the integrative system that utilizes tanniferous forages improves diet quality as legumes are generally digested more quickly and to a greater extent than grasses, as documented is a multi-year study (MacAdam et al., 2022). MacAdam et al. (2022) showed that legumes had greater crude protein, in vitro true dry matter digestibility, and non-fiber carbohydrates than the grass, while grass had greater neutral detergent fiber, acid detergent fiber, and crude fiber than legumes. Digestibility was found to be greater for the legumes than for the grass as well, and the total digestible nutrients of legumes was always greater than the grass and exceeded that of the high-forage confinement total mixed rations also tested in that study Ruminants consuming a higher quality diet due to the enhanced protein content and digestibility of legumes utilized as cover crops, grazed, or inter-seeded into grains, compared with grazing crop residues and/or grazing grasses, have increased productivity and reduced effect of environmental wastes such as methane or ammonia (MacAdam, et al., 2022). Better quality diets also reduce the CH4 output per unit of product and therefore a target quantity of animal product can be achieved at lower CH4 emissions and with fewer animals, improving both the productivity and sustainability of the agroecosystem.
The biochemistry of forages consumed by animals influences manure quality, and diets that include tannins may release N more slowly into the system (Delve et al., 2001; Clemensen et al., 2020). Stewart et al. (2019) found that CT concentration in cattle feces was proportional to CT in the forages consumed. Management of the quantity and quality of phenolic plant secondary compounds may be part of strategies that intensify productivity of sustainable systems, play a role during transition from one management to another, or as part of efforts to improve or remediate agroecological services.
Tannins and other phenolic compounds influence biological, chemical, and physical elements in the soil ecosystem, and they play an integral role in the ecological function of plants while also benefiting animal health. Enhancing agroecological sustainability by integrating tanniferous forages into crop-livestock systems is speculative and requires further research. It is difficult to provide categoric recommendations as research on tanniferous forages in integrated crop-livestock systems is limited. Consequently, future research might include tracing N mineralization in tanniferous pastures with varying soil types and/or evaluating how regional differences and climatic changes influence tannin concentrations in forages, and the soil-plant dynamics in these systems. This brief perspective paper supports the notion that phenolic compounds such as tannins may assist in efforts to enhance agroecological sustainability by demonstrating the various roles tannins have in agricultural systems.
The original contributions presented in the study are included in the article/supplementary material. Further inquiries can be directed to the corresponding author.
All authors contributed to the writing/reviewing/editing of this perspective manuscript. All authors read and approved the final manuscript.
The authors declare that the research was conducted in the absence of any commercial or financial relationships that could be construed as a potential conflict of interest.
All claims expressed in this article are solely those of the authors and do not necessarily represent those of their affiliated organizations, or those of the publisher, the editors and the reviewers. Any product that may be evaluated in this article, or claim that may be made by its manufacturer, is not guaranteed or endorsed by the publisher.
CT, condensed tannins; HT, hydrolysable tannins.
Aboagye I. A., Beauchemin K. A. (2019). Potential of molecular weight and structure of tannins to reduce methane emissions from ruminants: a review. Animals 9, 856. doi: 10.3390/ani9110856
Adamczyk B., Adamczyk S., Smolander A., Kitunen V. (2011). Tannic acid and Norway spruce condensed tannins can precipitate various organic nitrogen compounds. Soil Biol. Biochem. 43, 628–637. doi: 10.1016/j.soilbio.2010.11.034
Adamczyk B., Adamczyk S., Smolander A., Kitunen V., Simon J. (2018). Plant secondary metabolites–missing pieces in the soil organic matter puzzle of boreal forests. Soil Syst. 2, 2. doi: 10.3390/soils2010002
Adamczyk B., Karonen M., Adamczyk S., Engström M. T., Laakso T., Saranpää P., et al. (2017). Tannins can slow-down but also speed-up soil enzymatic activity in boreal forest. Soil Biol. Biochem. 107, 60–67. doi: 10.1016/j.soilbio.2016.12.027
Adamczyk B., Sietiö O. M., Biasi C., Heinonsalo J. (2019). Interaction between tannins and fungal necromass stabilizes fungal residues in boreal forest soils. New Phytol. 223, 16–21. doi: 10.1111/nph.15729
Adamczyk B., Simon J., Kitunen V., Adamczyk S., Smolander A. (2017). Tannins and their complex interaction with different organic nitrogen compounds and enzymes: old paradigms versus recent advances. ChemistryOpen 6 (5), 610–614. doi: 10.1002/open.201700113
Aerts R. J., Barry T. N., McNabb W. C. (1999). Polyphenols and agriculture: beneficial effects of proanthocyanidins in forages. Agric. Ecosyst. Environ. 75, 1–12. doi: 10.1016/s0167-8809(99)00062-6
Agati G., Azzarello E., Pollastri S., Tattini M. (2012). Flavonoids as antioxidants in plants: location and functional significance. Plant Sci. 196, 67–76. doi: 10.1016/j.plantsci.2012.07.014
Agati G., Tattini M. (2010). Multiple functional roles of flavonoids in photoprotection: letters. New Phytol. 186 (4), 786–793. doi: 10.1111/j.1469-8137.2010.03269.x
Agrawal A. A., Weber M. G. (2015). On the study of plant defence and herbivory using comparative approaches: how important are secondary plant compounds. Ecol. Lett. 18 (10), 985–991. doi: 10.1111/ele.12482
Akula R., Ravishankar G. A. (2011). Influence of abiotic stress signals on secondary metabolites in plants. Plant Signaling Behav. 6 (11), 1720–1731. doi: 10.4161/psb.6.11.17613
Allison G. G., Thain S. C., Morris P., Morris C., Hawkins S., Hauck B., et al. (2009). Quantification of hydroxycinnamic acids and lignin in perennial forage and energy grasses by Fourier-transform infrared spectroscopy and partial least squares regression. Bioresour. Technol. 100 (3), 1252–1261. doi: 10.1016/j.biortech.2008.07.043
Anuraga M., Duarsa P., Hill M. J., Lovett J. V. (1993). Soil moisture and temperature affect condensed tannin concentrations and growth in lotus corniculatus and lotus pedunculatus. Aust. J. Agric. Res. 44 (7), 1667. doi: 10.1071/AR9931667
Appel H. M. (1993). Phenolics in ecological interactions: the importance of oxidation. J. Chem. Ecol. 19, 1521–1552. doi: 10.1007/BF00984895
Arnoldi J. F., Coq S., Kéfi S., Ibanez S. (2020). Positive plant–soil feedback trigger tannin evolution by niche construction: A spatial stoichiometric model. J. Ecol. 108, 378–391. doi: 10.1111/1365-2745.13234
Balasundram N., Sundram K., Samman S. (2006). Phenolic compounds in plants and agri-industrial by-products: Antioxidant activity, occurrence, and potential uses. Food Chem. 99, 191–203. doi: 10.1016/j.foodchem.2005.07.042
Baldwin I. T., Schultz J. C. (1984). Tannins lost from sugar maple (Acer saccharum marsch) and yellow birch (Betula allegheniensis britt.) leaf litter. Soil Biol. Biochem. 16, 421–422. doi: 10.1016/0038-0717(84)90044-0
Barbehenn R. V., Constabel C. P. (2011). Tannins in plant–herbivore interactions. Phytochemistry 72 (13), 1551–1565. doi: 10.1016/j.phytochem.2011.01.040
Barbehenn R. V., Jaros A., Lee G., Mozola C., Weir Q., Salminen J.-P. (2009). Hydrolyzable tannins as ‘quantitative defenses’: limited impact against lymantria dispar caterpillars on hybrid poplar. J. Insect Physiol. 55 (4), 297–304. doi: 10.1016/j.jinsphys.2008.12.001
Barbehenn R., Weir Q., Salminen J.-P. (2008). Oxidation of ingested phenolics in the tree-feeding caterpillar orgyia leucostigma depends on foliar chemical composition. J. Chem. Ecol. 34 (6), 748. doi: 10.1007/s10886-008-9478-3
Barry T. N., McNabb W. C. (1999). The implications of condensed tannins on the nutritive value of temperate forages fed to ruminants. Br. J. Nutr. 81, 263–272. doi: 10.1017/S0007114599000501
Basaraba J. (1964). Influence of vegetable tannins on nitrification in soil. Plant Soil 21, 8–16. doi: 10.1007/BF01373867
Basaraba J., Starkey R. L. (1966). Effect of plant tannins on decomposition of organic substances. Soil Sci. 101, 17–23. doi: 10.1007/BF01373867
Beauchemin K. A., Kreuzer M., O’Mara F., McAllister T. A. (2008). Nutritional management for enteric methane abatement: a review. Aust. J. Exp. Agric. 48, 21–27. doi: 10.1071/EA07199
Benoit R. E., Starkey R. L. (1968). Enzyme inactivation as a factor in the inhibition of decomposition of organic matter by tannins. Soil Sci. 105, 203–208. doi: 10.1097/00010694-196804000-00001
Bezemer T., van Dam N. (2005). Linking aboveground and belowground interactions via induced plant defenses. Trends Ecol. Evol. 20 (11), 617–624. doi: 10.1016/j.tree.2005.08.006
Bhat T. K., Singh B., Sharma O. P. (1998). Microbial degradation of tannins–a current perspective. Biodegradation 9, 343–357. doi: 10.1023/A:1008397506963
Binti R. N. A., Ibrahim W. A., Hassan Z., Bin Sofian A. H. (2019). Tropical tannin for engineering application. Indonesian J Chem 20, 9. doi: 10.22146/ijc.40877
Broderick G. A., Grabber J. H., Muck R. E., Hymes-Fecht U. C. (2017). Replacing alfalfa silage with tannin-containing birdsfoot trefoil silage in total mixed rations for lactating dairy cows. J. Dairy Sci. 100 (5), 3548–3562. doi: 10.3168/jds.2016-12073
Bruce T. J. A., Pickett J. A. (2011). Perception of plant volatile blends by herbivorous insects – finding the right mix”. Phytochemistry 72 (13), 1605–1611. doi: 10.1016/j.phytochem.2011.04.011
Bu-Abbas A., Clifford M. N., Walker R., Ioannides C. (1998). Contribution of caffeine and flavanols in the induction of hepatic phase II activities by green tea. Food Chem. Toxicol. 36, 617–621. doi: 10.1016/S0278-6915(98)00019-2
Buzzini P., Arapitsas P., Goretti M., Branda E., Turchetti B., Pinelli P., et al. (2008). Antimicrobial and antiviral activity of hydrolysable tannins. Mini-Reviews Med. Chem. 8, 1179. doi: 10.2174/138955708786140990
Carter E. B., Morris P., Theodorou M. K. (1999). Responses of lotus corniculatus to environmental change. 2. effect of elevated CO2, temperature and drought on tissue digestion in relation to condensed tannin and carbohydrate accumulation”. J. Sci. Food Agric. 79 (11), 1431–1440. doi: 10.1002/(SICI)1097-0010
Castells E. (2008). “Indirect effects of phenolics on plant performance by altering nitrogen cycling: another mechanism of plant–plant negative interactions,” in Allelopathy in sustainable agriculture and forestry. Ed. Zeng R. S., et al (New York: Springer), 137–156.
Castells E., Peñuelas J., Valentine D. W. (2005). Effects of plant leachates from four boreal understory species on soil n mineralization, and white spruce (Picea glauca) germination and seedling growth. Ann. Bot. 95, 1247–1252. doi: 10.1093/aob/mci139
Chapin F. S., Vitousek P. M., Cleve K. V. (1986). The nature of nutrient limitation in plant communities. The American naturalist 127, 1, 48–58.
Chen C., Qiu H., Ma H., Imran S., Raza T., Gao R., et al. (2020). Response of the subtropical forest soil n transformations to tannin acid-organic nitrogen complexes. SN Appl. Sci. 2, 1–10. doi: 10.1007/s42452-020-3006-7
Christensen R. G., Yang S. Y., Eun J.-S., Young A. J., Hall J. O., MacAdam J. W. (2015). Effects of feeding birdsfoot trefoil hay on neutral detergent fiber digestion, nitrogen utilization efficiency, and lactational performance by dairy cows. J. Dairy Sci. 98 (11), 7982–7992. doi: 10.3168/jds.2015-9348
Clemensen A. K., Rottinghaus G. E., Lee S. T., Provenza F. D., Villalba J. J. (2018). How planting configuration influences plant secondary metabolites and total n in tall fescue (Festuca arundinacea schreb.), alfalfa (Medicago sativa l.) and birdsfoot trefoil (Lotus corniculatus l.): implications for grazing management. Grass Forage Sci. 73, 94–100. doi: 10.1111/gfs.12298
Clemensen A. K., Villalba J. J., Rottinghaus G. E., Lee S. T., Provenza F. D., Reeve J. R. (2020). Do plant secondary metabolite-containing forages influence soil processes in pasture systems? Agron. J. 112 (5), 3744–3757. doi: 10.1002/agj2.20361
Close D. C., McArthur C. (2002). Rethinking the role of many plant phenolics – protection from photodamage not herbivores? Oikos 99 (1), 166–172. doi: 10.1034/j.1600-0706.2002.990117.x
Coq S., Souquet J.-M., Meudec E., Cheynier V., Hättenschwiler S. (2010). Interspecific variation in leaf litter tannins drives decomposition in a tropical rain forest of French Guiana. Ecology 91, 2080–2091. doi: 10.1890/09-1076.1
Costa S. E. V. G. A., Souza E. D., Anghinoni I., Carvalho P. C. F., Martins A. P., Kunrath D., et al. (2014). Impact of an integrated no-till crop–livestock system on phosphorus distribution, availability and stock. Agric. Ecosyst. Environ. Integrated Crop-Livestock System Impacts Environ. Process. 190, 43–51. doi: 10.1016/j.agee.2013.12.001
Coulson C. B., Davies R. I., Lewis D. A. (1960). Polyphenols in plant, humus and soil. 1. polyphenols of leaves, litter and superficial humus from mull and mor sites. J. Soil Sci. 11, 20–29. doi: 10.1111/j.1365-2389.1960.tb02198.x
Cowan M. M. (1999). Plant products as antimicrobial agents. Clin. Microbiol. Rev. 12, 564–582. doi: 10.1128/CMR.12.4.564
Croteau R., Kutchan T. M., Lewis N. G. (2000). Natural products (secondary metabolites). Biochem. Mol. Biol. Plants 24, 1250–1319.
Crozier A., Clifford M. N., Ashihara H. (2001). Plant secondary metabolites: occurrence, structure and role in the human diet (Chichester, United Kingdom: John Wiley). Available at: http://ebookcentral.proquest.com/lib/usu/detail.action?docID=351346.
Crush J. R., Keogh R. G. (1998). A comparison o fthe effects of Lotus and white clover on some nutrient cycling factors. Proceedings of the New Zealand Grassland Association, 60, 83–87.
Das A. K., Islam M. N., Faruk M. O., Ashaduzzaman M., Dungani R. (2020). Review on tannins: Extraction processes, applications and possibilities. South Afr. J. Bot. 135, 58–70. doi: 10.1016/j.sajb.2020.08.008
Delve R. J., Cadisch G., Tanner J. C., Thorpe W., Thorne P. J., Giller K. E. (2001). Implications of livestock feeding management on soil fertility in the smallholder farming systems of Sub-Saharan africa”. Agric. Ecosyst. Environ. 84 (3), 227–243. doi: 10.1016/S0167-8809(00)00244-9
Dixon R. A. (2001). Natural products and plant disease resistance. Nature 411 (6839), 843–847. doi: 10.1038/35081178
Dixon R. A., Xie D.-Y., Sharma S. B. (2004). Proanthocyanidins - a final frontier in flavonoid research? tansley review”. New Phytol. 165 (1), 9–28. doi: 10.1111/j.1469-8137.2004.01217.x
Douglas G. B., Wang Y., Waghorn G. C., Barry T. N., Purchas R. W., Foote A. G, et al (1995). Liveweight gain and wool production of sheep grazing Lotus corniculatus and Lucerne (Medicago sativa). New Zeal. J. Agric. Res. 38 (1), 95–104.
Dultz S., Mikutta R., Kara S. N., Woche S. K., Guggenberger G. (2020). Effects of solution chemistry on conformation of self-aggregated tannic acid revealed by laser light scattering. Sci. Total Environ. 754, 142119. doi: 10.1016/j.scitotenv.2020.142119
Dupuy J., Larrieu G., Sutra J. F., Lespine A., Alvinerie M. (2003). Enhancement of moxidectin bioavailability in lamb by a natural flavonoid: quercetin. Vet. Parasitol. 112 (4), 337–347. doi: 10.1016/s0304-4017(03)00008-6
Dykes L., Rooney W.. (2007). Phenolic Compounds in Cereal Grains and Their Health Benefits. Cereal Foods World 52, 105–111. doi: 10.1094/CFW-52-3-0105
Erktan A., Balmot J., Merino-Martín L., Monnier Y., Pailler F., Coq S., et al. (2017). Immediate and long-term effect of tannins on the stabilization of soil aggregates. Soil Biol. Biochem. 105, 197–205. doi: 10.1016/j.soilbio.2016.11.017
Field J., Lettinga G. (1992). “Toxicity of tannic compounds to microorganisms,” in Plant polyphenols. basic life sciences, (vol 59). Eds. Hemingway R. W., Laks P. E. (Boston, MA: Springer). doi: 10.1007/978-1-4615-3476-1_39
Fierer N., Schimel J. P., Cates R. G., Zou J. (2001). Influence of balsam poplar tannin fractions on carbon and nitrogen dynamics in alaskan taiga floodplain soils. Soil Biol. Biochem. 33, 1827–1839. doi: 10.1016/S0038-0717(01)00111-0
Figueroa-Espinoza M.C., Zafimahova A., Alvarado P. G. M., Dubreucq E., Poncet-Legrand C. (2015). Grape seed and apple tannins: Emulsifying and antioxidant properties. Food Chem. 178, 38–44. doi: 10.1016/j.foodchem.2015.01.056
Franzluebbers A. J., Stuedemann J. A. (2014). Crop and cattle production responses to tillage and cover crop management in an integrated crop–livestock system in the southeastern USA”. Eur. J. Agron. Integrated crop-livestock 57, 62–70. doi: 10.1016/j.eja.2013.05.009
Frutos P., Hervás G., Natalello A., Luciano G., Fondevila M., Priolo A., et al. (2020). Ability of tannins to modulate ruminal lipid metabolism and milk and meat fatty acid profiles. Anim. Feed Sci. Technol. 269, 114623. doi: 10.1016/j.anifeedsci.2020.114623
Gallet C., Lebreton P. (1995). Evolution of phenolic patterns in plants and associated litters, and humus of a mountain forest ecosystem. Soil Biol. Biochem. 27, 157–165. doi: 10.1016/0038-0717(94)00167-Y
Goel G., Makkar H. P. S. (2011). Methane mitigation from ruminants using tannins and saponins. Trop. Anim. Health Prod. 44, 729–739. doi: 10.1007/s11250-011-9966-2
González-Lamothe R., Mitchell G., Gattuso M., Diarra M., Malouin F., Bouarab K. (2009). Plant antimicrobial agents and their effects on plant and human pathogens. Int. J. Mol. Sci. 10 (8), 3400–3419. doi: 10.3390/ijms10083400
Gorelik S., Kanner J., Schurr D., Kohen R. (2013). A rational approach to prevent postprandial modification of LDL by dietary polyphenols. J. Funct. Foods 5, 163–169. doi: 10.1016/j.jff.2012.09.008
Gorelik S., Ligumsky M., Kohen R., Kanner J. (2008). The stomach as a “bioreactor”: when red meat meets red wine. J. Agric. Food Chem. 56, 5002–5007. doi: 10.1016/j.jff.2012.09.008
Griffiths E., Burns R. G. (1972). Interaction between phenolic substances and microbial polysaccharides in soil aggregation. Plant Soil 36, 599–612. doi: 10.1007/BF01373510
Hagerman A. E. (2012). “Fifty years of polyphenol-protein complexes,” in Recent advances in polyphenol research. Ed. Cheyneir V., et al. (West Sussex, UK Boca Raton: Wiley-Blackwell), 71–91.
Hagerman A. E., Butler L. G. (1981). The specificity of proanthocyanidin-protein interactions. J. Biol. Chem. 256, 4494–4497. doi: 10.1016/S0021-9258(19)69462-7
Halvorson J. J., Gollany H. T., Kennedy A. C., Hagerman A. E., Gonzalez J. M., Wuest S. B. (2012). Sorption of tannin and related phenolic compounds and effects on extraction of soluble-n in soil amended with several carbon sources. Agriculture 2, 52–72. doi: 10.3390/agriculture2010052
Halvorson J. J., Gonzalez J. M. (2006). Bradford Reactive soil protein in Appalachian soils: distribution and response to incubation, extraction reagent and tannins. Plant Soil 286, 339–356. doi: 10.1007/s11104-006-9047-x
Halvorson J. J., Gonzalez J. M. (2008). Tannic acid reduces recovery of water-soluble carbon and nitrogen from soil and affects the composition of Bradford-reactive soil protein. Soil Biol. Biochem. 40, 186–197. doi: 10.1016/j.soilbio.2007.07.022
Halvorson J. J., Gonzalez J. M., Hagerman A. E. (2011). Repeated applications of tannins and related phenolic compounds are retained by soil and affect cation exchange capacity. Soil Biol. Biochem. 43, 1139–1147. doi: 10.1016/j.soilbio.2011.01.023
Halvorson J. J., Gonzalez J. M., Hagerman A. E. (2013). Retention of tannin-c is associated with decreased soluble nitrogen and increased cation exchange capacity in a broad range of soils. Soil Sci. Soc. America J. 77, 1199–1213. doi: 10.2136/sssaj2012.0326
Halvorson J. J., Gonzalez J. M., Hagerman A. E., Smith J. L. (2009). Sorption of tannin and related phenolic compounds and effects on soluble-n in soil. Soil Biol. Biochem. 41, 2002–2010. doi: 10.1016/j.soilbio.2009.07.006
Halvorson J., Kronberg S., Hagerman A. (2017). Effects of dietary tannins on total and extractable nutrients from manure. J. Anim. Sci. 95, 3654–3665. doi: 10.2527/jas.2016.1129
Halvorson J. J., Schmidt M. A., Hagerman A. E., Gonzalez J. M., Liebig M. A. (2016). Reduction of soluble nitrogen and mobilization of plant nutrients in soils from U.S northern great plains agroecosystems by phenolic compounds. Soil Biol. Biochem. 94, 211–221. doi: 10.1016/j.soilbio.2015.11.022
Handley W. R. C. (1961). Further evidence for the importance of residual leaf protein complexes in litter decomposition and the supply of nitrogen for plant growth. Plant Soil 15, 37–73. doi: 10.1007/BF01421749
Haslam E. (1981). “Vegetable tannins,” in The biochemistry of plants. secondary plant products vol. 7. Ed. Conn E. E. (New York: Academic Press), 527–556.
Hassanpour S., MaheriSis N., Eshratkhah B. (2011). Plants and secondary metabolites (Tannins): A review. Int. J. Forest Soil Erosion 1, 47–53.
Hättenschwiler S., Hagerman A. E., Vitousek P. M. (2003). Polyphenols in litter from tropical montane forests across a wide range in soil fertility. Biogeochemistry 64, 129–148.
Hättenschwiler S., Vitousek P. M. (2000). The role of polyphenols in terrestrial ecosystem nutrient cycling. Trends Ecol. Evol. 15, 238–243. doi: 10.1016/S0169-5347(00)01861-9
Hill N. S., Roberts C. A. (2020). Plant chemistry and antiquality components in forage. Forages: Sci. Grassl. Agric. 2, 633–658. doi: 10.1002/9781119436669.ch35
Horner J. D., Gosz J. R., Cates R. G. (1988). The role of carbon-based plant secondary metabolites in decomposition in terrestrial ecosystems. Am. Nat. 132, 869–883. doi: 10.1086/284894
Hufford C. D., Jia Y., Croom E. M., Muhammed I., Okunade A. L., Clark A. M., et al. (1993). Antimicrobial compounds from petalostemum purpureum. J. Natural Products 56 (11), 1878–1889. doi: 10.1021/np50101a003
Isah T. (2019). Stress and defense responses in plant secondary metabolites production. Biol. Res. 52 (1), 39. doi: 10.1186/s40659-019-0246-3
Ismail A. R., Norddin M. M., Latefi N. A., Oseh J. O., Ismail I., Gbadamosi A. O., et al. (2020). Evaluation of a naturally derived tannin extracts biopolymer additive in drilling muds for high-temperature well applications. J. Petrol. Explor. Prod. Technol. 10, 623–639. doi: 10.1007/s13202-019-0717-7
Jayanegara A., Leiber F., Kreuzer M. (2010). Meta-analysis of the relationship between dietary tannin level and methane formation in ruminants from in vivo and in vitro experiments. J. Anim. Physiol. Anim. Nutr. 96, 365–375. doi: 10.1111/j.1439-0396.2011.01172.x
Jin L., Wang Y., Iwaasa A. D., Li Y., Xu Z., Schellenberg M. P., et al. (2015). Purple prairie clover (Dalea purpurea vent) reduces fecal shedding of escherichia coli in pastured cattle. J. Food Prot. 78 (8), 1434–1441. doi: 10.4315/0362-028X.JFP-14-426
Joanisse G., Bradley R., Preston C., Bending G. (2009). Sequestration of soil nitrogen as tannin–protein complexes may improve the competitive ability of sheep laurel (Kalmia angustifolia) relative to black spruce (Picea mariana). New Phytol. 181, 187–198. doi: 10.1111/j.1469-8137.2008.02622.x
Joanisse G. D., Bradley R. L., Preston C. M., Munson A. D. (2007). Soil enzyme inhibition by condensed litter tannins may drive ecosystem structure and processes: the case of kalmia angustifolia. New Phytol. 175, 535–546. doi: 10.1111/j.1469-8137.2007.02113.x
Kaal J., Nierop K. G. J., Verstraten J. M. (2005). Retention of tannic acid and condensed tannin by fe-oxide-coated quartz sand. J. Colloid Interface Sci. 287, 72–79. doi: 10.1016/j.jcis.2005.01.104
Katiki L. M., Ferreira J., Gonvalez J. M., Zjac A. M., Lindsay D. S., Chagas A. C., et al. (2013). Antihelmintis effect of plant extracts containing condensed and hydrolyzable tannins on c. elegans and their antioxidant capacity. Vet. Parasitol. 192, 218–227. doi: 10.1016/j.vetpar.2012.09.030
Kesarwani A., Chiang P-Y., Chen S-S. (2014). Distribution of Phenolic Compounds and Antioxidative Activities of Rice Kernel and Their Relationships with Agronomic Practice. The Scientific World Journal. doi: 10.1155/2014/620171
Kinraide T. B., Hagerman A. E. (2010). Interactive intoxicating and ameliorating effects of tannic acid, aluminum (Al3+), copper (Cu2+), and selenate (SeO42-) in wheat roots: a descriptive and mathematical assessment. Physiol. Plant. 139, 68–79. doi: 10.1111/j.1399-3054.2010.01347.x
Kliebenstein D. J. (2004). Secondary metabolites and plant/environment interactions: a view through arabidopsis thaliana-tinged glasses. Plant. Cell Environ. 27 (6), 675–684. doi: 10.1111/j.1365-3040.2004.01180.x
Knekt P., Kumpulainen J., Jarvinen R., Rissanen H., Heliovaara M., Reunanen A., et al. (2002). Flavonoid intake and risk of chronic diseases. Am. J. Clin. Nutr. 76, 560–568. doi: 10.1093/ajcn/76.3.560
Kraal P., Nierop K. G. J., Kaal J., Tietema A. (2009). Carbon respiration and nitrogen dynamics in Corsican pine litter amended with aluminum and tannins. Soil Biol. Biochem. 41, 2318–2327. doi: 10.1016/j.soilbio.2009.08.017
Kraus T. E. C., Dahlgren R. A., Zasoski R. J. (2003a). Tannins in nutrient dynamics of forest ecosystems - a review. Plant Soil 256, 41–66. doi: 10.1023/A:1026206511084
Kraus T. E. C., Yu Z., Preston C. M., Dahlgren R. A., Zasoski R. J. (2003b). Linking chemical reactivity and protein precipitation to structural characteristics of foliar tannins. J. Chem. Ecol. 29, 703–730. doi: 10.1023/A:1022876804925
Kraus T. E. C., Zasoski R. J., Dahlgren R. A., Horwath W. R., Preston C. M. (2004). Carbon and nitrogen dynamics in a forest soil amended with purified tannins from different plant species. Soil Biol. Biochem. 36, 309–321. doi: 10.1016/j.soilbio.2003.10.006
Kuiters A. (1990). Role of phenolic substances from decomposing forest litter in plant-soil interactions. Acta Botanica Neerlandica 39, 329–348. doi: 10.1111/j.1438-8677.1990.tb01412.x
Kunrath T. R., Cadenazzi M., Brambilla D. M., Anghinoni I., de Moraes A., Barro R. S., et al. (2014). Management targets for continuously stocked mixed oat×annual ryegrass pasture in a no-till integrated crop–livestock system. Eur. J. Agron. Integrated crop-livestock 57, 71–76. doi: 10.1016/j.eja.2013.09.013
Lahiri-Chatterjee M., Katiyar S. K., Mohan R. R., Agarwal R. (1999). A flavonoid antioxidant, silymarin, affords exceptionally high protection against tumor promotion in the SENCAR mouse skin tumorigenesis model. Cancer Res. 59, 622–632.
Laothawornkitkul J., Taylor J. E., Paul N. D., Hewitt C. N. (2009). Biogenic volatile organic compounds in the earth system. New Phytol. 183 (1), 27–51. doi: 10.1111/j.1469-8137.2009.02859.x
Lavola A., Aphalo P. J., Lahti M., Julkunen-Tiitto R. (2003). Nutrient availability and the effect of increasing UV-b radiation on secondary plant compounds in scots pine”. Environ. Exp. Bot. 49 (1), 49–60. doi: 10.1016/S0098-8472(02)00057-6
Leiss K. A., Choi Y. H., Verpoorte R., Klinkhamer P. G. L. (2011). An overview of NMR-based metabolomics to identify secondary plant compounds involved in host plant resistance. Phytochem. Rev. 10 (2), 205–216. doi: 10.1007/s11101-010-9175-z
Lemaire G., Franzluebbers A., de Faccio Carvalho P. C., Dedieu B. (2014). Integrated crop–livestock systems: strategies to achieve synergy between agricultural production and environmental quality. Agric. Ecosyst. Environ. Integrated Crop-Livestock System Impacts Environ. Process. 190, 4–8. doi: 10.1016/j.agee.2013.08.009
Le Marchand L., Murphy S. P., Hankin J. H., Wilkens L. R., Kolonel L. N. (2000). Intake of flavonoids and lung cancer. J. Natl. Cancer Inst. 92, 154–160. doi: 10.1093/jnci/92.2.154
Lewis J., Starkey R. (1968). Vegetable tannins, their decomposition and effects of decomposition of some organic compounds. Soil Sci. 106, 241–247. doi: 10.1097/00010694-196810000-00001
López-Andrés P., Luciano G., Vasta V., Gibson T. M., Biondi L., Priolo A., et al. (2013). Dietary quebracho tannins are not absorbed, but increase the antioxidant capacity of liver and plasma in sheep. Br. J. Nutr. 110, 632–639. doi: 10.1017/S0007114512005703
Luciano G., Vasta V., Monahan F. J., López-Andrés P., Biondi L., Lanza M., et al. (2011). Antioxidant status, colour stability and myoglobin resistance to oxidation of longissimus dorsi muscle from lambs fed a tannin-containing diet. Food Chem. 124, 1036–1042. doi: 10.1016/j.foodchem.2010.07.070
Lyman T. D., Provenza F. D., Villalba J. J. (2008). Sheep foraging behavior in response to interactions among alkaloids, tannins and saponins. J. Sci. Food Agric. 88 (5), 824–831. doi: 10.1002/jsfa.3158
Lyman T. D., Provenza F. D., Villalba J. J., Wiedmeier R. D. (2011). Cattle preferences differ when endophyte-infected tall fescue, birdsfoot trefoil, and alfalfa are grazed in different sequences. J. Anim. Sci. 89 (4), 1131–1137. doi: 10.2527/jas.2009-2741
Lyman T. D., Provenza F. D., Villalba J. J., Wiedmeier R. D. (2012). Phytochemical complementarities among endophyte-infected tall fescue, reed canarygrass, birdsfoot trefoil and alfalfa affect cattle foraging. Animal 6 (4), 676–682. doi: 10.1017/S1751731111001996
MacAdam J. W., Pitcher L. R., Bolletta A. I., Guevara Ballesteros R. D., Beauchemin K. A., Dai X., et al. (2022). Increased nitrogen retention and reduced methane emissions of beef cattle grazing legume vs. grass irrigated pastures in the mountain West USA. Agronomy (12), 304. doi: 10.3390/agronomy12020304
Macel M., van Dam N. M. (2018). “Metabolomics of plant resistance to insects,” in The biology of plant-insect interactions, 1st ed (CRC Press), 129–149. doi: 10.1201/9781315119571-7
Macel M., van Dam N. M., Keurentjes J. J. B. (2010). Metabolomics: the chemistry between ecology and genetics: Invited technical review. Mol. Ecol. Resour. 10 (4), 583–593. doi: 10.1111/j.1755-0998.2010.02854.x
Maddox C. E., Laur L. M., Tian L. (2010). Antibacterial activity of phenolic compounds against the phytopathogen xylella fastidiosa. Curr. Microbiol. 60 (1), 53–58. doi: 10.1007/s00284-009-9501-0
Makarov M. (2019). The role of mycorrhiza in transformation of nitrogen compounds in soil and nitrogen nutrition of plants: a review. Eurasian Soil Sci. 52, 193–205. doi: 10.1134/S1064229319020108
Malisch C. S., Salminen J.-P., Kölliker R., Engström M. T., Suter D., Studer B., et al. (2016). Drought effects on proanthocyanidins in sainfoin (Onobrychis viciifolia scop.) are dependent on the plant’s ontogenetic stage. J. Agric. Food Chem. 64 (49), 9307–9316. doi: 10.1021/acs.jafc.6b02342
Matthews S., Mila I., Scalbert A., Donnelly D. M. X. (1997). Extractable and non-extractable proanthocyanidins in barks. Phytochemistry 45, 405–410. doi: 10.1016/S0031-9422(96)00873-4
Maughan B., Provenza F. D., Tansawat R., Maughan C., Martini S., Ward R., et al. (2014). Importance of grass-legume choices on cattle grazing behavior, performance, and meat characteristics. J. Anim. Sci. 92 (5), 2309–2324. doi: 10.2527/jas.2013-7297
McAllister T. A., Martinez T., Bae H. D., Muir A. D., Yanke L. J., Jones G. A. (2005). Characterization of condensed tannins purified from legume forages: Chromophore production, protein precipitation, and inhibitory effects on cellulose digestion. J. Chem. Ecol. 31, 2049–2068. doi: 10.1007/s10886-005-6077-4
McMahon L. R., Majak W., McAllister T. A., Hall J. W., Jones G., Popp J. D., et al. (1999). Effect of sainfoin on in vitro digestion of fresh alfalfa and bloat in steers. Can. J. Anim. Sci. 79, 203–212. doi: 10.4141/A98-074
McSweeney C. S., Palmer B., McNeill D. M., Krause D. O. (2001). Microbial interactions with tannins: Nutritional consequences for ruminants. Anim. Feed Sci. Technol. 91, 83–93. doi: 10.1016/S0377-8401(01)00232-2
Meier C. L., Suding K. N., Bowman W. D. (2008). Carbon flux from plants to soil: roots are a below-ground source of phenolic secondary compounds in an alpine ecosystem. J. Ecol. 96, 421–430. doi: 10.1111/j.1365-2745.2008.01356.x
Miele S., Tegli S., Izquierdo C. G., Cerboneschi M., Bargiacchi E. (2019). Hydrolysable tannins in agriculture. IntechOpen. doi: 10.5772/intechopen.86610
Min B. R., Pinchak W. E., Hernandez K., Hernandez C., Hume M. E., Valencia E., et al. (2012). The effect of plant tannins supplementation on animal responses and in vivo ruminal bacterial populations associated with bloat in heifers grazing wheat forage. Prof. Anim. Sci. 28, 464–472. doi: 10.15232/S1080-7446(15)30386-7
Mueller-Harvey I. (2006). Unravelling the conundrum of tannins in animal nutrition and health. J. Sci. Food Agric. 86 (13), 2010–2037. doi: 10.1002/jsfa.2577
Mutabaruka R., Hairiah K., Cadisch G. (2007). Microbial degradation of hydrolysable and condensed tannin polyphenol-protein complexes in soils from different land-use histories. Soil Biol. Biochem. 39, 1479–1492. doi: 10.1016/j.soilbio.2006.12.036
Norris C., Preston C., Hogg K., Titus B. (2011). The influence of condensed tannin structure on rate of microbial mineralization and reactivity to chemical assays. J. Chem. Ecol. 37, 311–319. doi: 10.1007/s10886-011-9921-8
Northup R. R., Dahlgren R. A., McColl J. G. (1998). Polyphenols as regulators of plant-litter-soil interactions in northern california’s pygmy forest: a positive feedback? Biogeochemistry 42 (1), 189–220. doi: 10.1023/A:1005991908504
Novobilský A., Stringano E., Hayot C. C., Smith L. M., Enemark H. L., Mueller-Harvey I., et al. (2013). In vitro effects of extracts and purified tannins of sainfoin (Onobrychis viciifolia) against two cattle nematodes. Vet. Parasitol. 196, 532–537. doi: 10.1016/j.vetpar.2013.03.024
Nyachoti C., Atkinson J., Leeson S. (1997). Sorghum tannins: a review. World’s Poultry Sci. J. 53, 5–21. doi: 10.1079/WPS19970002
Owens J., Provenza F. D., Wiedmeier R. D., Villalba J. J. (2012a). Supplementing endophyte-infected tall fescue or reed canarygrass with alfalfa or birdsfoot trefoil increases forage intake and digestibility by sheep. J. Sci. Food Agric. 92 (4), 987–992. doi: 10.1002/jsfa.4681
Owens J., Provenza F. D., Wiedmeier R. D., Villalba J. J. (2012b). Influence of saponins and tannins on intake and nutrient digestion of alkaloid-containing foods. J. Sci. Food Agric. 92 (11), 2373–2378. doi: 10.1002/jsfa.5643
Pang Y., Sulaiman A., Yanjie X., Trust B., Zhiwei Z., Yafang S., et al. (2018). Bound phenolic compounds and antioxidant properties of whole grain and bran of white, red and black rice. Food Chem. 240, 212–221. doi: 10.1016/j.foodchem.2017.07.095
Patra A. K., Saxena J. (2011). Exploitation of dietary tannins to improve rumen metabolism and ruminant nutrition. J. Sci. Food Agric. 91, 24–37. doi: 10.1002/jsfa.4152
Pearce G., Strydom D., Johnson S., Ryan C. A. (1991). A polypeptide from tomato leaves induces wound-inducible proteinase inhibitor proteins. Science 253 (5022), 895–897. doi: 10.1126/science.253.5022.895
Pichersky E., Gershenzon J. (2002). The formation and function of plant volatiles: perfumes for pollinator attraction and defense. Curr. Opin. Plant Biol. 5 (3), 237–243. doi: 10.1016/S1369-5266(02)00251-0
Pierik R., Ballaré C. L., Dicke M. (2014). Ecology of plant volatiles: taking a plant community perspective: ecology of plant volatiles. Plant. Cell Environ. 37 (8), 1845–1853. doi: 10.1111/pce.12330
Pizzi A. (2019). Tannins: prospectives and actual industrial applications. Biomolecules 9, 344. doi: 10.3390/biom9080344
Ren W., Wang Z., Zhu H., Zhang L. (2003). Flavonoids: promising anticancer agents. Med. Res. Rev. 23, 519–534. doi: 10.1002/med.10033
Rice E. L., Pancholy S. K. (1973). Inhibition of nitrification by climax ecosystems. II. additional evidence and possible role of tannins. Am. J. Bot. 60, 691–702. doi: 10.2307/2441448
Rillig M. C., Caldwell B. A., Wösten H. A. B., Sollins P. (2007). Role of proteins in soil carbon and nitrogen storage: controls on persistence. Biogeochemistry 85, 25–44. doi: 10.1007/s10533-007-9102-6
Salminen J.-P., Karonen M. (2011). Chemical ecology of tannins and other phenolics: we need a change in approach: chemical ecology of tannins. Funct. Ecol. 25 (2), 325–338. doi: 10.1111/j.1365-2435.2010.01826.x
Salminen J.-P., Karonen M., Sinkkonen J. (2011). Chemical ecology of tannins: recent developments in tannin chemistry reveal new structures and structure–activity patterns. Chem.–A Eur. J. 17, 2806–2816. doi: 10.1002/chem.201002662
Santana A. L. B. D., Maranhão C. A., Santos J. C., Cunha F. M., Conceição G. M., Bieber L. W., et al. (2010). Antitermitic activity of extractives from three Brazilian hardwoods against nasutitermes corniger. Int. Biodeterior. Biodegradation 64 (1), 7–12. doi: 10.1016/j.ibiod.2009.07.009
Savatin D. V., Gramegna G., Modesti V., Cervone F. (2014). Wounding in the plant tissue: the defense of a dangerous passage. Front. Plant Sci. doi: 10.3389/fpls.2014.00470
Scalbert A. (1991). Antimicrobial properties of tannins. Phytochemistry 30 (12), 3875–3883. doi: 10.1016/0031-9422(91)83426-L
Schmidt M. A., Gonzalez J. M., Halvorson J. J., Hagerman A. E. (2013a). Metal mobilization in soil by two structurally defined polyphenols. Chemosphere 90, 1870–1877. doi: 10.1016/j.chemosphere.2012.10.010
Schmidt M., Halvorson J., Gonzalez J., Hagerman A. (2012). Kinetics and binding capacity of six soils for structurally defined hydrolyzable and condensed tannins and related phenols. J. Soils Sediments 12, 366–375. doi: 10.1007/s11368-011-0463-z
Schmidt M. A., Halvorson J. J., Hagerman A. E., Gonzalez J. M. (2017). Macronutrients and metals released from soils by solutions of naturally occurring phenols. J. Plant Nutr. Soil Sci. 180, 544–553. doi: 10.1002/jpln.201700144
Schmidt M. A., Kreinberg A. J., Gonzalez J. M., Halvorson J. J., French E., Bollmann A., et al. (2013b). Soil microbial communities respond differently to three chemically defined polyphenols. Plant Physiol. Biochem. 72, 190–197. doi: 10.1016/j.plaphy.2013.03.003
Schofield J. A., Hagerman A. E., Harold A. (1998). Loss of tannins and other phenolics from willow leaf litter. J. Chem. Ecol. 24, 1409–1421. doi: 10.1023/a:1021287018787
Schultz J. C. (1989). “Tannin-insect interactions,” in Chemistry and significance of condensed tannins (Boston, MA: Springer). doi: 10.1007/978-1-4684-7511-1_26
Schweitzer J. A., Bailey J. K., Hart S. C., Wimp G. M., Chapman S. K., Whitham T. G. (2005). The interaction of plant genotype and herbivory decelerate leaf litter decomposition and alter nutrient dynamics. Oikos 110, 133–145. doi: 10.1111/j.0030-1299.2005.13650.x
Schweitzer J., Madritch M., Bailey J., LeRoy C., Fischer D., Rehill B., et al. (2008). From genes to ecosystems: the genetic basis of condensed tannins and their role in nutrient regulation in a populus model system. Ecosystems 11, 1005–1020. doi: 10.1007/s10021-008-9173-9
Selmants P. C., Schweitzer J. A., Adair K. L., Holeski L. M., Lindroth R. L., Hart S. C., et al. (2019). Genetic variation in tree leaf chemistry predicts the abundance and activity of autotrophic soil microorganisms. Ecosphere 10, e02795. doi: 10.1002/ecs2.2795
Selvakumar G., Saha S., Kundu S. (2007). Inhibitory activity of pine needle tannin extracts on some agriculturally resourceful microbes. Indian J. Microbiol. 47, 267–270. doi: 10.1007/s12088-007-0049-z
Sharma K., Kumar V., Kaur J., Tanwar B., Goyal A., Sharma R., et al. (2019). Health effects, sources, utilization and safety of tannins: a critical review. Toxin Rev 40 (4), 432–44. doi: 10.1080/15569543.2019.1662813
Shirmohammadli Y., Efhamisisi D., Pizzi A. (2018). Tannins as a sustainable raw material for green chemistry: A review. Ind. Crops Products 126, 316–332. doi: 10.1016/j.indcrop.2018.10.034
Singh A. P., Kumar S. (2019). Applications of tannins in industry, tannins-structural properties, biological properties and current knowledge. IntechOpen. doi: 10.5772/intechopen.85984
Sirota R., Gorelik S., Harris R., Kohen R., Kanner J. (2013). Coffee polyphenols protect human plasma from postprandial carbonyl modifications. Mol. Nutr. Food Res. 57, 916–919. doi: 10.1002/mnfr.201200557
Sivapalan K., Fernando V., Thenabadu M. (1983). Humified phenol-rich plant residues and soil urease activity. Plant Soil 70, 143–146. doi: 10.1007/bf02374757
Sivapalan K., Fernando V., Thenabadu M. W. (1985). N-mineralization in polyphenol-rich plant residues and their effect on nitrification of applied ammonium sulphate. Soil Biol. Biochem. 17, 547–551. doi: 10.1016/0038-0717(85)90023-9
Slebodnik K. A. (2020). Effect of plant derived tannins on nitrogen and carbon cycling in pasture soils. All Graduate Theses Diss. 7803. doi: 10.26076/591d-a93f
Smolander A., Kanerva S., Adamczyk B., Kitunen V. (2012). Nitrogen transformations in boreal forest soils–does composition of plant secondary compounds give any explanations? Plant Soil 350 (1–2), 1–26. doi: 10.1007/s11104-011-0895-7
Solaiman Z. M., Senoo K. (2018). Arbuscular mycorrhizal fungus causes increased condensed tannins concentrations in shoots but decreased in roots of lotus japonicus l. Rhizosphere 5, 32–37. doi: 10.1016/j.rhisph.2017.11.006
Sorgho B., Zerbo L., Keita I., Dembele C., Plea M., Sol V., et al. (2014). Strength and creep behavior of geomaterials for building with tannin addition. Mater. struct. 47, 937–946. doi: 10.1617/s11527-013-0104-7
Souto X. C., Chiapusio G., Pellissier F. (2000). Relationships between phenolics and soil microorganisms in spruce forests: significance for natural regeneration. J. Chem. Ecol. 26, 2025–2034. doi: 10.1023/A:1005504029243
Stewart E. K., Beauchemin K. A., Dai X., MacAdam J. W., Christensen R. G., Villalba J. J. (2019). “Effect of tannin-containing hays on enteric methane emissions and nitrogen partitioning in beef cattle”. J. Anim. Sci., skz206. doi: 10.1093/jas/skz206
Strzalka K., Kostecka-Gugala A., Latowski D. (2003). Carotenoids and environmental stress in plants: Significance of carotenoid-mediated modulation of membrane physical properties. Russian J. Plant Physiol. 50, 168–173. doi: 10.1023/A:1022960828050
Subdiaga E., Harir M., Orsetti S., Hertkorn N., Schmitt-Kopplin P., Haderlein S. B. (2020). Preferential sorption of tannins at aluminum oxide affects the electron exchange capacities of dissolved and sorbed humic acid fractions. Environ. Sci. Technol. 54, 1837–1847. doi: 10.1021/acs.est.9b04733
Suseela V., Alpert P., Nakatsu C. H., Armstrong A., Tharayil N. (2016). Plant–soil interactions regulate the identity of soil carbon in invaded ecosystems: implication for legacy effects. Funct. Ecol. 30, 1227–1238. doi: 10.1111/1365-2435.12591
Talbot J., Finzi A. (2008). Differential effects of sugar maple, red oak, and hemlock tannins on carbon and nitrogen cycling in temperate forest soils. Oecologia 155, 583–592. doi: 10.1007/s00442-007-0940-7
Tavendale M. H., Meagher L. P., Pacheco D., Walker N., Attwood G. T., Sivakumaran S. (2005). Methane production from in vitro rumen incubations with lotus pedunculatus and medicago sativa, and effects of ex-tractable condensed tannin fractions on methanogenesis. Anim. Feed Sci. Technol. 123, 403–419. doi: 10.1016/j.anifeedsci.2005.04.037
Tharayil N., Alpert P., Bhowmik P., Gerard P. (2013). Phenolic inputs by invasive species could impart seasonal variations in nitrogen pools in the introduced soils: a case study with polygonum cuspidatum. Soil Biol. Biochem. 57, 858–867. doi: 10.1016/j.soilbio.2012.09.016
Tharayil N., Suseela V., Triebwasser D. J., Preston C. M., Gerard P. D., Dukes J. S. (2011). Changes in the structural composition and reactivity of acer rubrum leaf litter tannins exposed to warming and altered precipitation: climatic stress-induced tannins are more reactive. New Phytol. 191 (1), 132–145. doi: 10.1111/j.1469-8137.2011.03667.x
Theis N., Lerdau M. (2003). The evolution of function in plant secondary metabolites. Int. J. Plant Sci. 164, 93–102. doi: 10.1086/374190
Thornton P. K., Herrero M. (2010). Potential for reduced methane and carbon dioxide emissions from livestock and pasture management in the tropics. Proc. Natl. Acad. Sci. U.S.A. 107 (46), 19667–19672. doi: 10.1073/pnas.0912890107
Top S. M., Preston C. M., Dukes J. S., Tharayil N. (2017). Climate influences the content and chemical composition of foliar tannins in green and senesced tissues of quercus rubra. Front. Pant Sci. 8. doi: 10.3389/fpls.2017.00423
Triebwasser D. J., Tharayil N., Preston C. M., Gerard P. D. (2012). The susceptibility of soil enzymes to inhibition by leaf litter tannins is dependent on the tannin chemistry, enzyme class and vegetation history. New Phytol. 196, 1122–1132. doi: 10.1111/j.1469-8137.2012.04346.x
Turlings T. C., Loughrin J. H., McCall P. J., Rose U. S., Lewis W. J., Tumlinson J. H. (1995). How caterpillar-damaged plants protect themselves by attracting parasitic wasps. Proc. Natl. Acad. Sci. 92 (10), 4169–4174. doi: 10.1073/pnas.92.10.4169
Ushio M., Balser T. C., Kitayama K. (2013). Effects of condensed tannins in conifer leaves on the composition and activity of the soil microbial community in a tropical montane forest. Plant Soil 365, 157–170. doi: 10.1007/s11104-012-1365-6
Tang Y, Tsao R (2017). Phytochemicals in quinoa and amaranth grains and their antioxidant, anti-inflammatory, and potential health beneficial effects: a review. Molecular Nutrition and food Res. 61, 7. doi: 10.1002/mnfr.201600767
Van Vliet S., Provenza F. D., Kronberg S. L. (2021). Health-promoting compounds are higher in grass-fed meat and milk. Front. Sustain. Food Syst. 4. doi: 10.3389/fsufs.2020.555426
Villalba J. J., Miller J., Hall J. O., Clemensen A. K., Stott R., Snyder D., et al. (2013). Preference for tanniferous (Onobrychis viciifolia) and non-tanniferous (Astragalus cicer) forage plants by sheep in response to challenge infection with haemonchus contortus. Small Ruminant Res. 112 (1–3), 199–207. doi: 10.1016/j.smallrumres.2012.11.033
Villalba J. J., Provenza F. D. (2007). Self-medication and homeostatic behaviour in herbivores: learning about the benefits of nature’s pharmacy. Animal 1, 1360–1370. doi: 10.1017/S1751731107000134
von Lützow M., Kögel-Knabner I., Ekschmitt K., Matzner E., Guggenberger G., Marschner B., et al. (2006). Stabilization of organic matter in temperate soils: mechanisms and their relevance under different soil conditions - a review. Eur. J. Soil Sci. 57, 426–445. doi: 10.1111/j.1365-2389.2006.00809.x
Waghorn G. (2008). Beneficial and detrimental effects of dietary condensed tannins for sustainable sheep and goat production–progress and challenges. Anim. Feed Sci. Technol. 147 (1–3), 116–139. doi: 10.1016/j.anifeedsci.2007.09.013
Waghorn G., McNabb W. (2003). Consequences of plant phenolic compounds for productivity and health of ruminants. Proc. Nutr. Soc. 62 (2), 383–392. doi: 10.1079/PNS2003245
Waghorn G. C., Shelton I. D., McNabb W. C., McCutcheon S. N. (1994). Effects of condensed tannins in lotus pedunculatus on its nutritive value for sheep. 2. nitrogenous aspects. J. Agric. Sci. 123 (1), 109–119. doi: 10.1017/S0021859600067836
Waghorn G. C., Tavendale M. H., Woodfield D. R. (2002). Methanogenesis from forages fed to sheep. New Z. Grassl. Assoc. 64, 167–171. doi: 10.33584/jnzg.2002.64.2462
Wei C., Yang K., Zhao G., Lin S., Xu Z. (2016). Effect of dietary supplementation of gallic acid on nitrogen balance, nitrogen excretion pattern and urinary nitrogenous constituents in beef cattle. Arch. Anim. Nutr. 70, 416–423. doi: 10.1080/1745039X.2016.1214345
Wurzburger N., Hendrick R. L. (2009). Plant litter chemistry and mycorrhizal roots promote a nitrogen feedback in a temperate forest. J. Ecol. 97, 528–536. doi: 10.1111/j.1365-2745.2009.01487.x
Xie D.-Y., Dixon R. A. (2005). Proanthocyanidin biosynthesis – still more questions than answers?” Phytochemistry 66 (18), 2127–2144. doi: 10.1016/j.phytochem.2005.01.008
Yang K., Wei C., Zhao G. Y., Xu Z. W., Lin S. X. (2017). Effects of dietary supplementing tannic acid in the ration of cattle on rumen fermentation, methane emission, microbial flora and nutrient digestibility. Anim. Physiol. Anim. Nutr. 101, 302–310. doi: 10.1111/jpn.12531
Zhang Q.-F., Laanbroek H. J. (2020). Tannins from senescent rhizophora mangle mangrove leaves have a distinctive effect on prokaryotic and eukaryotic communities in a distichlis spicata salt marsh soil. FEMS Microbiol. Ecol. 96, fiaa148. doi: 10.1093/femsec/fiaa148
Zhang L., Liu R., Gung B. W., Tindall S., Gonzalez J. M., Halvorson J. J., et al. (2016). Polyphenol–aluminum complex formation: implications for aluminum tolerance in plants. J. Agric. Food Chem. 64, 3025–3033. doi: 10.1021/acs.jafc.6b00331
Keywords: condensed tannins (CT), integrative crop/livestock systems, sustainable agriculture, carbon sequestration, nitrogen mineralization
Citation: Clemensen A, Halvorson JJ, Christensen R and Kronberg SL (2022) Potential benefits of tanniferous forages in integrative crop-livestock agroecosystems. Front. Agron. 4:911014. doi: 10.3389/fagro.2022.911014
Received: 01 April 2022; Accepted: 27 June 2022;
Published: 22 July 2022.
Edited by:
Leslie A. Weston, Charles Sturt University, AustraliaReviewed by:
John Piltz, New South Wales Department of Primary Indust, AustraliaCopyright © 2022 Clemensen, Halvorson, Christensen and Kronberg. This is an open-access article distributed under the terms of the Creative Commons Attribution License (CC BY). The use, distribution or reproduction in other forums is permitted, provided the original author(s) and the copyright owner(s) are credited and that the original publication in this journal is cited, in accordance with accepted academic practice. No use, distribution or reproduction is permitted which does not comply with these terms.
*Correspondence: Andrea Clemensen, YW5kcmVhLmNsZW1lbnNlbkB1c2RhLmdvdg==
Disclaimer: All claims expressed in this article are solely those of the authors and do not necessarily represent those of their affiliated organizations, or those of the publisher, the editors and the reviewers. Any product that may be evaluated in this article or claim that may be made by its manufacturer is not guaranteed or endorsed by the publisher.
Research integrity at Frontiers
Learn more about the work of our research integrity team to safeguard the quality of each article we publish.