- University of Kentucky Research and Education Center, University of Kentucky, Princeton, KY, United States
Dicamba injury to sensitive soybean and other broadleaf crops due to drift is a major issue. Dicamba label restrictions have been created to mitigate the off-target movement of dicamba. One restriction is the mandated use of low-drift nozzles to spray dicamba; these nozzles produce large droplet spectrums and minimize the production of driftable fines. Experiments were conducted to evaluate herbicide coverage, deposition, and efficacy as influenced by spray nozzle design and density of waterhemp, goosegrass, and large crabgrass in dicamba-resistant soybean. Dicamba plus glyphosate was applied to 5- to 10-cm-tall weeds with a Turbo TeeJet (TT11005) nozzle and two drift reduction nozzles approved for dicamba applications: Turbo TeeJet Induction (TTI11005) and Pentair Ultra Lo-Drift (ULD12005). Weed densities were categorized into different levels and established in a 0.25-m2 quadrat prior to postemergence application. Deposition of herbicide spray solution onto targeted weeds was not different despite coverage differences observed on Kromekote spray cards. Coverage of herbicide solution was consistently lower with the low-drift TTI11005 nozzle as compared to the TT11005 nozzle. Herbicide efficacy on waterhemp plants was the lowest at the highest waterhemp densities of 54 plants per m2 with the drift-reducing TTI11005 nozzle, although weed control was not lowered at any density when applications were made with the ULD nozzle as compared to the TT11005 nozzle. Additionally, herbicide efficacy was reduced as large crabgrass density increased. Overall, the use of a drift-reducing nozzle can be successful for waterhemp control and Poaceae control postemergence in soybean when weed densities are suppressed or reduced through methods such as the use of a residual preemergence herbicide or cereal rye cover crop.
Introduction
Dicamba-resistant soybean varieties became available in 2016 followed by the approval of new dicamba formulations for application to dicamba-resistant soybean in 2017 (USEPA United States Environmental Protection Agency 2020). Since their introduction, Kentucky growers have implemented the dicamba-resistant soybean system into their weed management programs to control troublesome weeds such as waterhemp (Amaranthus tuberculatus (Moq.) J.D. Sauer), Palmer amaranth (Amaranthus palmeri S. Watson), giant ragweed (Ambrosia trifida L.), and horseweed (Erigeron canadensis L.). The technology has given growers an additional site of action for postemergence weed control in soybean, but an increase in dicamba off-site movement causing damage to sensitive plants has also occurred. Dicamba injury is unique and consists of stem epinasty, leaf cupping and curling, and bud suppression; at high rates, leaf necrosis can also occur (Marth and Mitchell, 1944; Chang and Born, 1971; Johnson et al., 2012). Crops such as non-dicamba-resistant soybean, tobacco, and tomato are all sensitive to dicamba drift. Soybean and tomato have been demonstrated to show visual injury at rates of 0.06 and 0.5 g ae ha−1, respectively, and yield losses in tomatoes occurred at a rate of 2.3 g ae ha−1 (Johnson et al., 2012; Kruger et al., 2012; Robinson et al., 2013).
There are many factors that influence herbicide off-target movement, and more specifically physical drift, during a postemergence application including environmental conditions of wind speed, air temperature, and humidity (Carlsen et al., 2006). Wind speed has the greatest influence on the physical drift with increasing velocities correlating with increased distances of downwind depositions (Carlsen et al., 2006; Sousa Alves et al., 2017). Even though environmental factors are out of the control of the applicator, they must still be aware of their potential influence. There are many factors that an applicator can manipulate to aid in reducing physical drift. The applicator can control droplet size by selecting a nozzle that produces a very coarse, extremely coarse, or ultra-coarse droplet and reduces the amount of driftable fines present in the spray volume (Creech et al., 2015a). Very coarse, extremely coarse, and ultra-coarse droplets are classified and defined using the ASABE S572.4 procedure and contain minimal driftable fines (Fritz et al., 2012; ASABE American Society of Agricultural and Biological Engineers 2020). Boom height, pressure, and spray speed also affect physical drift when making an herbicide application (Nuyttens et al., 2006; Nuyttens et al., 2007; Al Heidary et al., 2014; Creech et al., 2015a). Increasing any of these three factors is likely to increase potential drift. To reduce the potential of dicamba off-target movement events, a series of regulations have been included in the approved dicamba labels for over-the-top dicamba applications to dicamba-tolerant soybean (USEPA United States Environmental Protection Agency 2020). These regulations are intended to reduce the risk of potential dicamba off-target movement and include the mandatory use of specific nozzles and pressure ranges to produce extremely coarse and ultra-coarse droplets.
Increasing the droplet size not only influences potential drift during an application but also influences the amount of coverage and the overall performance of the herbicide on weed management. Nozzles that produce larger droplet spectrums can decrease weed control, especially when applied at low spray volumes and when applying contact herbicides (Creech et al., 2016; Meyer et al., 2016a). There are conflicting data on the relative influence of carrier volume, spray droplet size, and herbicide active ingredient on herbicide performance (Knoche, 1994), but recent investigations have suggested that carrier volume may have more influence than droplet size on herbicide performance (Creech et al., 2015b; Legleiter and Johnson, 2016; Meyer et al., 2016a), although the overall influence of droplet size and carrier volume on weed control is also dependent on herbicide active ingredient being applied and weed species being evaluated.
Weed density may also have a potential effect on the overall performance of herbicides. It is well known that weed density correlates with crop competition and yield loss. In a study conducted by Bensch et al. (2003), it was reported that when eight waterhemp plants m−2 grew in competition with soybean, the yield was negatively impacted by 56%. Large crabgrass (Digitaria sanguinalis (L.) Scop.) can reduce soybean yields by up to 37% at densities of 16 plants m−2 (Basinger et al., 2019). While it is known that higher levels of weed density can affect the overall crop yield, minimal recent research has been conducted looking at the influence that weed density has on herbicide spray coverage and deposition. Further understanding the impact of weed density on herbicide coverage and deposition is a crucial knowledge gap that needs to be filled to ensure maximum performance of postemergence herbicides as we face increasing herbicide resistance events in weeds. The architecture of the target weed can also affect herbicide spray coverage and deposition. Dicot weed leaves have a greater surface area to capture herbicide solution droplets when compared to monocot leaves (Dorr et al., 2008). The use of an air-induction nozzle type that produces an ultra-coarse droplet- versus a coarse droplet-producing nozzle could result in a reduction in annual grass control (Wolf et al., 2000; Meyer et al., 2016b; Carter et al., 2017).
The objective of this study was to determine the influence of spray nozzle and weed density on herbicide coverage, deposition, and efficacy of a dicamba and glyphosate postemergence application onto waterhemp and Poaceae species.
Materials and Methods
Field Site Establishment and Maintenance
Two field experiments (Trial 1 and Trial 2) were conducted during the summer of 2019 on a commercial production field with a Sadler silt loam soil near Princeton, Kentucky, United States. Both experiments were conducted in the same field but were separated both spatially and temporally to create two separate environments for the two trials. Trial 1 was conducted in May and June of 2019, and Trial 2 was conducted in June and July 2019. This site had a well-established population of suspected glyphosate-resistant waterhemp.
Two additional field experiments were conducted in 2018 and 2019, evaluating Poaceae species at The University of Kentucky Research and Education Center located near Princeton, Kentucky. The soil type for this location is a Crider silt loam. In 2018, the predominate Poaceae species population at the site was goosegrass (Eleusine indica (L.) Gaertn.). In 2019, there was a mixed stand of Poaceae species, which consisted of large crabgrass (D. sanguinalis (L.) Scop.), goosegrass, giant foxtail (Setaria faberi Herrm.), and Johnsongrass (Sorghum halepense (L.) Pers.), with the majority of the population being large crabgrass.
A dicamba- and glyphosate-resistant soybean variety (Asgrow 42X6, Bayer Crop Science, St. Louis, MO, USA) was planted on May 24, 2019, and June 18, 2019, for the waterhemp experiments, and May 15, 2018, and April 30, 2019, for the Poaceae species studies. All trials were planted with a Precision Planting vacuum planter on 38-cm row spacing at an approximate seeding rate of 346,000 to 370,500 seeds ha−1.
Plots were maintained as a no-tillage system with existing vegetation prior to planting being removed, and an application of glufosinate at a rate of 655 g ai ha−1 was performed to the first waterhemp. The second waterhemp trial received an application of paraquat at a rate of 560 g ai ha−1 at planting. In the Poaceae trial, a burndown application of glyphosate at 1,260 g ae ha−1 was applied prior to planting. In addition, dicamba at 560 g ae ha−1 was applied on May 30, 2018, and June 3, 2019, to control the non-grass weeds such as horseweed and giant ragweed.
Experimental Design and Establishment
The experimental design was a two-way factorial treatment structure in a randomized complete block with four replications. Individual plots were considered one experiment unit and measured 3 m by 8 m with 6-m alleyways between blocks to allow for high-speed applications. The two factors included three nozzle designs and three or four weed density levels depending on the target species. The first factor was nozzle design and included a TT11005 nozzle (Turbo TeeJet, TeeJet Technologies, Glendale Heights, IL, USA) and two drift reduction nozzles approved for dicamba applications: TTI11005 (Turbo TeeJet Induction, TeeJet Technologies, Glendale Heights, IL, USA) and ULD12005 (Pentair Ultra Lo-Drift, Pentair, Minneapolis, MN, USA). The second factor was weed density with three and four density target levels in the waterhemp and Poaceae trials, respectively. The Poaceae trials contained four density levels that were approximately 100%, 75%, 50%, and 25% of the natural population. The naturally occurring population of goosegrass in 2018 and crabgrass in 2019 was 25 and 29 plants per 0.25 m2, respectively (Table 1). The weed densities for the Poaceae trial were manipulated with a preemergence herbicide application made on May 16, 2018, and April 30, 2019. Pyroxasulfone (Zidua ® SC Herbicide, BASF Corporation, Research Triangle Park, NC, USA) was used as the preemergence herbicide and was applied at rates of 280, 180, and 90 g ai ha−1 to the 25%, 50%, and 75% density level treatments, respectively. The naturally occurring density or 100% density level received no preemergence herbicide. The waterhemp density in the 100% density treatments or naturally occurring populations was 54 plants per 0.25 m2 in both Trial 1 and Trial 2 (Table 2). The waterhemp trials were limited to three density levels due to space limitations in the commercial production field. The waterhemp density levels were targeted at 100%, 50%, and less than 25% of the naturally occurring population. The less than 25% weed density for Trial 1 was manipulated with a preemergence herbicide application of flumioxazin at 90 g ai ha−1 and pyroxasulfone at 110 g ai ha−1 (Fierce Herbicide®, Valent USA Corporation, Walnut Creek, CA, USA). The preemergence herbicide application was made using a CO2 backpack sprayer pressurized at 221 kPa while traveling at 6 kph fitted with XR11002 nozzles spaced at 50 cm. The plots that contained the 50% and 100% densities were hand weeded to the appropriate ranges in Trial 1. Due to the excessively low weed densities in the treatments receiving the preemergence herbicide in Trial 1 and the initiation of Trial 2 later in the growing season beyond peak waterhemp emergence, a preemergence herbicide was not applied to the lowest density treatments of Trial 2. Thus, all treatments in Trial 2 were hand thinned to appropriate weed densities to match the density levels in Trial 1. A 0.25-m2 quadrat was established in each plot for all trials to contain the different weed density levels and are reported in Tables 1, 2.
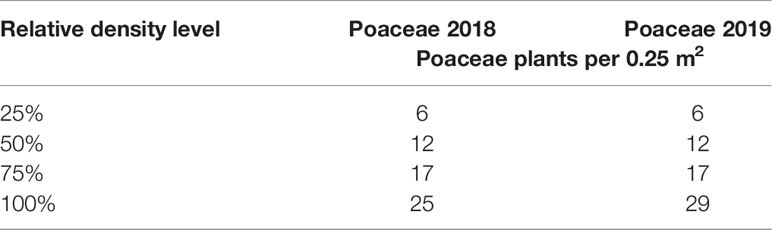
Table 1 Relative density levels as compared to the natural populations of Poaceae plants in 0.25-m2 quadrats.
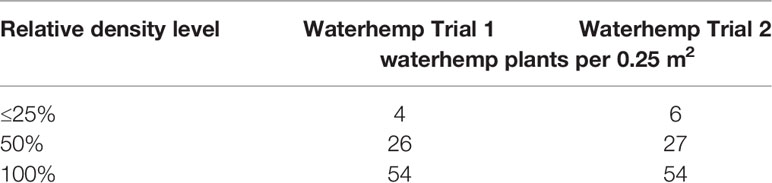
Table 2 Relative density levels as compared to the natural populations of waterhemp in 0.25-m2 quadrats.
Postemergence Herbicide Application
Herbicide application methods were designed to mimic a commercial postemergence herbicide application. Applications were made using an all-terrain vehicle (ATV) with a 3-m side boom traveling at a speed of 16 kph with an output of 140 L ha−1. The 3-m side boom was outfitted with four nozzles on 50-cm spacing and pressurized to 262 to 290 kPa depending on the nozzle. Applications were made when waterhemp and Poaceae plants were approximately 5 to 10 cm tall. In waterhemp Trial 1, the postemergence applications were made on two different dates due to the less than 25% density waterhemp treatments being delayed in reaching the 5- to 10-cm height by the residual herbicide application as compared to the 50% and 100% density treatments. The first application in waterhemp Trial 1 for the 50% and 100% density levels was made on June 14, 2019, and the second application for the less than 25% density was made on June 25, 2019. Waterhemp Trial 2 postemergence applications were made on July 8, 2019; all treatments were applied on the same date due to all density manipulations being conducted by hand thinning, allowing all plants to reach 5- to 10-cm height at the same time. All Poaceae trial postemergence applications were made on the same date in both trial years. In 2018, the postemergence application was made on June 18, and in 2019, the application was made on June 12. The tank mixture for all applications consisted of 1,100 g ae ha−1 glyphosate (Roundup PowerMAX® Bayer Crop Science, St. Louis, MO, USA), 560 g ae ha−1 dicamba (XtendiMax™, Bayer Crop Science, St. Louis, MO, USA). On Target (On Target®, WinField Solutions, LLC, St. Paul, MN, USA) was also included at 0.5% v/v as a drift reduction agent (DRA) as required by the XtendiMax label when tank mixing with Roundup PowerMAX. Vision Pink (Vision Pink™, Garrco Products Inc., Converse, IN, USA) and fluorescent 1,3,6,8-pyrene tetra sulfonic acid (PTSA) (Spectra Trace SH-P, Spectra Colors Corporation, Kearny, NJ, USA). The Vision Pink and PTSA dyes were added to the tank mixture at 0.25% v/v and 600 ppm, respectively, to facilitate analysis of spray solution coverage and deposition.
Droplet Spectrum Analysis
A droplet spectrum analysis was conducted to determine the droplet sizes for the spray nozzles used in these experiments. The same spray nozzles and tank mixture used in the field experiments, excluding the Vision Pink and PTSA dyes, were used during the analysis. Only one nozzle per nozzle type was tested and was selected from the boom at random. The analysis was conducted in North Platte, Nebraska, at the University of Nebraska Pesticide Application Technology Laboratory. The analysis was conducted in a low wind tunnel with a constant wind speed of 24 kph to evacuate droplets from the spray plume to avoid duplicate droplet measurements. The droplet spectrum produced by the nozzle was evaluated using laser diffraction with a Sympatec Helos Vario KR particle size analyzer assembled with an R7 lens. The spray plume was traversed through the laser three times to represent three replications. The report from the analysis included the Dv10, Dv50, and Dv90, which translates into 10%, 50%, and 90%, of the droplets in the spray volume that are at or below the reported diameter in microns. The report also gives the percentage of droplets less than 200 µm, which represents the percentage of driftable fines each nozzle produces. Based on the Dv10, Dv50, and Dv90 values, each nozzle was classified into a droplet size category per ASABE S572.4.
Spray Card Coverage
Kromekote spray cards (22 cm by 28 cm) were used to measure herbicide spray coverage. The Vision Pink dye in the herbicide tank mix allows for visual markings of depositions on the glossy surface of the Kromekote cards. Prior to the herbicide application, spray cards were placed within the soybean canopy, at the same height as the target weed species, so that each card was centered between two of the three middle rows within the plot. After the herbicide application, the cards were allowed to dry, collected from the field, and transferred back to the lab. Each card was scanned into 600 by 600-dpi, 24-bit color digital images using a duplex scanner (Brother ADS-2200 Duplex Scanner, Brother International Corporation, Bridgewater, NJ, USA). An analysis was conducted using APS Assess Software (ASSESS 2.0-Image Analysis Software for Plant Disease Quantification, The American Phytopathological Society, St. Paul, MN, USA), which separates the pink depositions from the white background on the card. The output from the software was an area of coverage measured in cm2 and deposition counts, which were converted into percent of coverage and depositions per cm2, respectively, using the known size of the cards.
Herbicide Solution Deposition
A fluorescent dye (PTSA) was added to the tank mixture as a tracer to analyze spray solution deposition on the target weed species. Two target weed species plants measuring in height between 5 and 10 cm were harvested from the 0.25-m2 quadrat at the soil surface immediately after the post-application to collect herbicide spray solution deposition. Each harvested plant was washed in a 200-ml solution consisting of water and 0.1% v/v Triton X-100 (Triton™ X-100, EMD Chemicals Inc., Gibbstown, NJ, USA). After washing, each plant sample was placed in an envelope and transferred to the lab to conduct further analysis. Prior to the field sample analysis, a standard linear curve of raw fluorescent values was established using standardized wash solutions that contained 0.0001 to 1 ppm of PTSA. Raw fluorescence for each field wash solution was measured three times with a Trilogy Laboratory Fluorometer (Trilogy Laboratory Fluorometer, Turner Designs, San Jose, CA, USA) equipped with the PTSA-specific module. A LI-3100 leaf area meter (LI-3100, LI-COR Inc., Lincoln, NE, USA) was used to analyze the whole plant leaf area in cm2 for each sample. By knowing the concentration of PTSA in the herbicide tank mixture, the amount of wash solution used (200 ml), the leaf area of the plant (cm2), and the concentration of PTSA in the wash solutions, calculations were made to determine how much spray solution was deposited onto the waterhemp samples in µl cm−2. All methods and calculations were based on the methodology outlined by Fritz et al., 2011.
Herbicide Efficacy
Herbicide efficacy was evaluated using visual assessments of whole plots using a 0 to 100 rating scale 21 days after treatment (DAT). Zero percent represented no control, and 100% represented complete weed death or full control.
Data Analysis
Normality and equality of variances were checked prior to data analysis. Square root transformations were conducted when assumptions were not met. After each assumption was met, treatment differences were determined using ANOVA with SAS 9.4 PROC GLIMMIX. Means were separated at alpha = 0.05 adjusted with Tukey’s honestly significant difference (HSD) when effects were significant and the means for all data are presented using the raw data.
Trial interaction analysis was conducted for herbicide spray solution deposition and efficacy for waterhemp trials. Interactions between trial, spray nozzle design, weed density, and the interaction of all three factors were used in the analysis. Interactions of trial*nozzle were found for spray solution deposition; thus, the trials were analyzed separately for all data evaluations.
A trial interaction analysis was also conducted for spray card coverage and spray card deposition density for both the waterhemp trials and Poaceae trials. The interaction analysis excluded the weed density factor, as spray cards were placed at weed height and would not have been influenced by weed density. Interactions of trial and nozzle were not found in the waterhemp trial analysis for either spray card coverage or spray card deposition density; thus, further analysis of spray card data was pooled across waterhemp trials. Inversely, an interaction of trial by nozzle was found for spray card coverage in the Poaceae trial data set; thus, all spray card data were analyzed by year similar to the remainder of the Poaceae trial data as discussed below.
The authors chose to analyze Poaceae trial years separately due to the difference in Poaceae species between the two site years. In 2018 for the Poaceae trial, the leaf area (cm2) of plants collected for herbicide solution deposition was more variable than desired; therefore, a covariance using leaf area (p = 0.0001) was added to the ANOVA. Collection teams were instructed to more carefully collect target size plants of 5 to 10 cm in 2019, leaf areas were therefore more consistent in 2019, and leaf area (cm2) was not added to the ANOVA for that year.
Results and Discussion
Droplet Spectrum Analysis
With the use of the standard nozzles established by ASABE S572.4, a standard curve was established for droplet classification at the PAT Laboratory. The Dv10, Dv50, and Dv90 values from the three nozzles used in the trials were plotted onto the standard curve to establish droplet size classifications for each nozzle. The TT11005 nozzle was classified as very coarse droplet size, while both the ULD12005 and TTI11005 were classified in the ultra-coarse droplet size category (Table 3). As expected, the TT11005 nozzle has the lowest Dv (10, 50, and 90) values when compared to the two drift-reducing nozzles (ULD12005 and TTI11005) (Table 3). The TTI11005 nozzle produced the smallest percentage of driftable fines at 0.6%, while the TT11005 recorded the greatest driftable fine percentage at 7.8% (Table 3). The ULD12005 percentage of driftable fines was between the two TeeJet nozzles at 2.2% (Table 3).
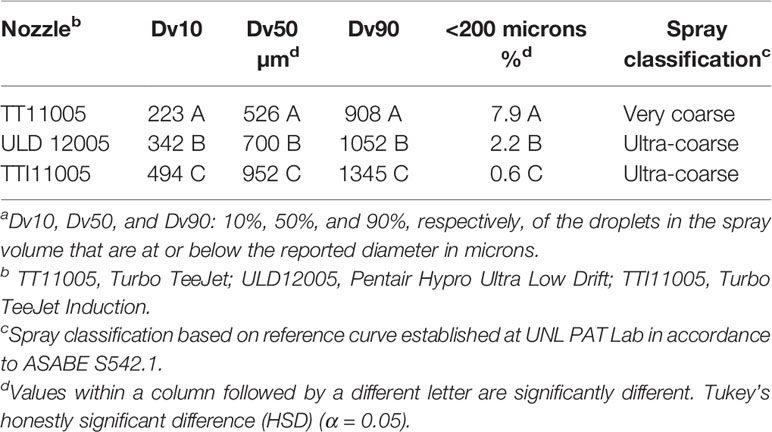
Table 3 Dv10a, Dv50a, Dv90a, percent of driftable fines, and spray classification for each nozzle at field use rate of dicamba plus glyphosate plus a drift reduction agent.
Spray Card Coverage
In the waterhemp trials, the TT11005 had the greatest percent coverage of the three nozzle types at 49% coverage (Table 4). The two drift reduction nozzles both resulted in lower coverage than the TT11005, although the ULD12005 resulted in 15% greater coverage than the TTI11005 (Table 4).
Deposition density results in the waterhemp trials had a similar trend in differences between nozzle types as the percent coverage results. Both drift reduction nozzles had significantly lower deposition density as compared to the TT11005 nozzle, which had 47 depositions cm−2 (Table 4). As with the percent coverage, the ULD12005 nozzle resulted in greater deposition density than the TTI11005 with deposition densities of 42 and 27 deposits cm− (Table 4).
Results from 2018 and 2019 Poaceae trials for the spray cards indicated differences among nozzles when considering both coverage and deposition density (Table 4). Herbicide spray solution coverage was lower with the use of the TTI11005 nozzle in both years (2018 and 2019) when compared to the TT11005 nozzle (Table 4). In 2018, the ULD 12005 nozzle had similar coverage compared to both TeeJet nozzle types (TT11005 and TTI11005) (Table 3). In 2019, the ULD 12005 nozzle, which produces ultra-coarse droplets and is approved to make dicamba applications, did not decrease coverage when compared to the TT11005 nozzle, which produces very coarse droplets, which are not approved for dicamba applications.
Poaceae trial deposition densities were the greatest for the TT11005 nozzle producing very coarse droplets in both years with densities of 39 and 45 depositions cm−2 when compared to the TTI11005 nozzle type that produced ultra-coarse droplets (Table 4). In 2018, the ULD12005 decreased deposition densities to 28 cm−2, and the TTI11005 had the lowest deposition densities at 18 cm−2 (Table 3). In 2019, despite the fact that the ULD 12005 is considered a drift-reducing nozzle, no differences were observed compared to the TT11005 nozzle. As expected, the TTI11005 produced the fewest depositions cm−2.
These results for both the waterhemp and Poaceae trials were expected and support previous literature when increasing droplet size spectrum; coverage and deposition density were reduced (Knoche, 1994; Ferguson et al., 2016).
Herbicide Solution Deposition on Target Weeds
Results from all trials for waterhemp and Poaceae species did not show an interaction between the two factors of spray nozzle design and weed density on dicamba plus glyphosate spray solution deposition (Tables 5, 6). The deposition volume of herbicide spray solution onto the target plants ranged from 0.65 to 1.24 µl cm−2 in waterhemp Trial 1 and from 0.52 to 1.03 µl cm−2 in waterhemp Trial 2 (Table 5). In the 2018 Poaceae trial, the deposition volume of herbicide spray solution ranged from 1.02 to 2.26 µl cm−2, and in 2019, deposition ranged from 0.46 to 0.84 µl cm−2 (Table 6). Weed density and spray nozzle type were analyzed separately for both waterhemp and Poaceae trials due to lack of interaction. Analysis of weed density (p = 0.5606 and p = 0.2877) and spray nozzle type (p = 0.7513 and p = 0.4698) showed a lack of influence on spray solution deposition in both years for the Poaceae trials. Weed density did not influence the amount of spray solution deposition volume for waterhemp Trial 1 (p = 0.2636) or waterhemp Trial 2 (p = 0.3522). Spray nozzle design also did not have an influence on spray deposition in waterhemp Trial 1 (p = 0.0792), but in Trial 2, spray nozzle design did have a significant p-value less than 0.05 (p = 0.0434). Despite the fact that the p-value for spray nozzle design was significant, Tukey’s test was unable to separate the difference between nozzle types (data not shown). In efforts to gain more knowledge, a Student–Newman–Keuls (SNK) test was conducted on both trials looking at spray solution deposition. The SNK test results were similar to those of Tukey’s test and were unable to separate any differences between spray nozzle designs for waterhemp Trial 2 (data not shown).

Table 5 Herbicide solution deposition onto waterhemp plants as influenced by waterhemp density and nozzle type.
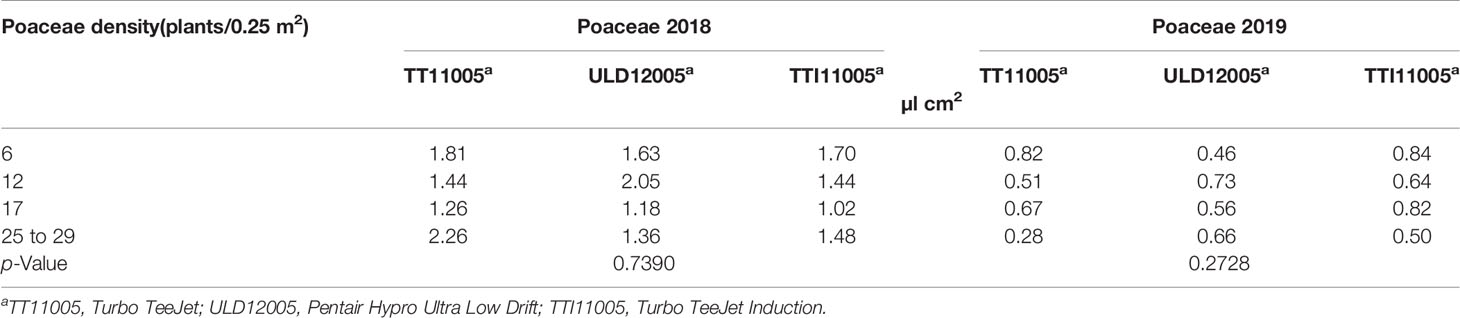
Table 6 Herbicide spray solution deposition onto Poaceae plants as influenced by grass density and nozzle design.
Despite the fact that differences were observed between spray nozzles on the spray cards regarding percent coverage and depositions cm−2, there were no differences observed among spray nozzles on the amount of spray solution volume deposited onto waterhemp, goosegrass, and crabgrass plants. The two drift-reducing nozzles (TTI11005 and ULD 12005) provided an equivalent amount of spray solution deposition compared to the traditional broadcast nozzle (TT11005). Percent coverage and depositions cm−2 on the spray cards are measurements of a two-dimensional area, while the spray solution deposition onto target plants represents volume over an area or a three-dimensional measurement. These are two different measurements being conducted; therefore, the measurements can conflict with their observations.
Herbicide Efficacy
Differences in percent waterhemp control 21 DAT resulted in differing observations between waterhemp Trials 1 and 2 (Table 7). In waterhemp Trial 1, no interaction was observed between nozzle type and weed density (p = 0.4705), while an interaction did occur in waterhemp Trial 2 (p = 0.0251). In waterhemp Trial 1, the control of waterhemp ranged from 78% to 94%, while the control of waterhemp ranged from 60% to 99% in waterhemp Trial 2 (Table 7). Waterhemp control in waterhemp trial 2 was lower with the use of the TTI11005 nozzle at the highest waterhemp density level as compared to all the TT11005 and ULD12005 density combinations (Table 7). In waterhemp Trial 1, weed density had an influence on waterhemp control with the highest density-reducing waterhemp control by 16% as compared to the lowest density (p = 0.0017) (Table 7), while nozzle type did not have an influence on waterhemp control (p = 0.4810).
Interactions of nozzle type and Poaceae species density did not occur for visual control 21 DAT in either year (p = 0.3221 and p = 0.2028). In 2018, Poaceae control ranged from 84% to 99%, and in 2019, it ranged from 58% to 99% among the nozzle type and weed density treatments (data not shown). Each factor was analyzed separately within each year due to a lack of factor interaction. In 2018, there was no influence of nozzle or weed density on the percent control of Poaceae species (p = 0.5144 and p = 0.2478, respectively). Differences in percent Poaceae control did occur in 2019 with the factor of weed density (p = 0.0002). The highest density, with an average of 29 plants per 0.25 m2, reduced percent control of Poaceae species by 24% when compared to the other density levels (Table 8). In 2019, no differences in percent control of Poaceae species were observed between the three nozzle types (p = 0.0983) (data not shown).
Herbicide efficacy analyzed using visual evaluations 21 DAT showed that weed densities and a combination of density and nozzle type influence weed control in waterhemp and Poaceae species. In the waterhemp Trial 1 as well as the 2019 Poaceae trial, results indicate that a reduction in percent control occurs at the highest densities of 54 waterhemp plants per 0.25 m2 and 29 crabgrass plants per 0.25 m2. Results from the waterhemp Trial 2 were similar, as the combination of the highest waterhemp density (54 waterhemp per 0.25 m2) and the use of the TTI11005 nozzle producing an ultra-coarse droplet resulted in lower waterhemp control.
Discussion
The spray coverage and deposition density on spray cards in the waterhemp and Poaceae trials support previous literature where increasing droplet size spectrum corresponds with lower coverage and deposition density (Knoche, 1994; Ferguson et al., 2016). In all cases within this research, the ultra-coarse droplet-producing TTI11005 nozzle had lower coverage and deposition density as compared to the TT11005 nozzle, which produced a smaller droplet spectrum. The ULD12005 nozzle, which produced an ultra-coarse droplet pattern similar to the TTI11005 nozzle, had greater coverage and deposition density than the TTI11005 nozzle. Despite the ULD12005 and TTI11005 nozzles both producing a droplet spectrum in the ultra-coarse category, it should be noted that the ULD12005 did have a lower DV10, DV50, and DV90 as compared to the TTI11005 nozzle, thus explaining the differences in spray coverage and deposition density between the two nozzles. The ULD12005 and TTI11005 are both approved nozzles for applications of dicamba to dicamba-resistant soybean; the results from this research indicate that a nozzle such as the ULD12005 may be better suited to achieve maximum coverage while still maintaining reduced drift potential with less than 2.5% driftable fines within the droplet spectrum.
The results of this research also indicate that weed density does influence herbicide performance on waterhemp and large crabgrass. This influence of density on herbicide performance can be compounded in some cases with the use of low-drift nozzles such as the TTI11005 nozzle. Decreased herbicide efficacy was observed at the higher density plant populations of 54 waterhemp plants and 29 crabgrass plants per 0.25 m2, regardless of the nozzle being used to make the application in two of the studies in this research. Furthermore, in waterhemp Trial 2, it was observed that herbicide efficacy on waterhemp was lower in plots with a high waterhemp density of 54 plants per 0.25 m2 that received the herbicide application using the TTI11005 nozzle as compared to all other nozzle and density combinations. This is complementary to previous research evaluating the influence of droplet size and nozzle design on weed control. Meyer et al. (2016a) found a decrease in Palmer amaranth and barnyard grass (Echinochloa crus-galli (L.) P. Beauv.) control with the use of TTI nozzles at reduced spray volumes of 94 L ha−1. Herbicide efficacy was similar in plots receiving dicamba plus glyphosate applications with the ULD12005 nozzle in comparison to the TT11005 nozzle despite the fact that the ULD12005 has a larger droplet spectrum as compared to the TT11005 and being in the same droplet category as the TTI11005. Again, it is important to note that despite the ULD12005 and TTI11005 being categorized in the same droplet category, the DV10, DV50, and DV90 values were significantly different between the two nozzles. In a similar conclusion to spray coverage results, the ULD12005 may be better suited to maximize dicamba plus glyphosate application efficacy, especially when being applied to higher density populations such as 54 waterhemp plants per 0.25 m2, as compared to the TTI11005 nozzle.
The results of this research indicated differences in spray coverage on spray cards and differences in herbicide efficacy on waterhemp and crabgrass among weed densities and nozzle types, even though no differences were observed in herbicide spray solution deposition onto targeted plants across weed density and nozzle combinations. This is similar to the findings of Legleiter et al. (2016), in which differences in spray coverage on cards were found between XR11004, TT11004, AIXR11004, and TTI11004 nozzles, while deposition onto waterhemp, giant ragweed, marestail, and Palmer amaranth remained the same among the four nozzles. It is important to note that the sampling method used during the experiments may not have captured a complete representation of solution deposition in the high-density quadrats. Herbicide deposition was only collected from two random plants per plot after application, due to time constraints to effectively capture herbicide solution from plant surfaces prior to losses due to solution absorption, drying, or evaporation. This sampling may not have accounted for smaller plants occurring lower in the weed species canopy, especially in the higher density treatments with 29 to 54 plants per 0.25 m2. Thus, the smaller understory plants could have potentially had reduced deposition and a likely reduction in herbicide performance in the high-density plots.
Overall, the results of these experiments emphasize the importance of limiting or suppressing weed density prior to the postemergence application, regardless of the nozzle type being used to make the herbicide application, although this research has shown that this is especially true when using a low-drift nozzle such as the TTI11005 as mandated by dicamba labels for applications to dicamba-resistant soybean. The effective use of integrated weed control practices such as the use of a cover crop or the use of preemergence herbicides can be key to reducing weed density at the postemergence application. A study conducted by Vyn et al. (2007) that evaluated multiple preemergence herbicides concluded that effective herbicides provided 96% to 98% control of waterhemp plants and reduced the density to 1 to 2 waterhemp plants m−2 as compared to the untreated check of 53 to 126 plants m−2. Similarly, a study conducted by Aulakh and Jhala (2015) found that residual herbicide reduced green foxtail and large crabgrass densities to less than 2 plants m−2 as compared to untreated checks with 10 plants m−2. Research conducted by Cornelius and Bradly (2017) found that a cereal rye cover crop can reduce early-season waterhemp emergence by up to 35% and was similar to suppression provided by a preemergence residual herbicide. When considering the data from these studies, it can be concluded that targeted waterhemp and annual grass species can be effectively controlled with dicamba plus glyphosate using a low-drift nozzle as long as weed densities are suppressed prior to the postemergence application using methods such as applications of preemergence herbicides and/or the use of cover crops.
Data Availability Statement
The raw data supporting the conclusions of this article will be made available by the authors, without undue reservation.
Author Contributions
MK conducted the in-field investigation, experiment management, data collection, and data analysis and wrote the original draft of the manuscript. TL conceptualized and developed the methods of the research, administered and supervised the project, conducted data analysis review, and reviewed and edited the final manuscript for submission. All authors listed have made a substantial, direct, and intellectual contribution to the work and approved it for publication.
Funding
The Kentucky Soybean Promotion Board provided partial funding for this research.
Conflict of Interest
The authors declare that the research was conducted in the absence of any commercial or financial relationships that could be construed as a potential conflict of interest.
Publisher’s Note
All claims expressed in this article are solely those of the authors and do not necessarily represent those of their affiliated organizations, or those of the publisher, the editors and the reviewers. Any product that may be evaluated in this article, or claim that may be made by its manufacturer, is not guaranteed or endorsed by the publisher.
Acknowledgments
The authors would like to acknowledge Dr. Greg Kruger and the University of Nebraska Pesticide Application Technology Lab in North Platte, Nebraska, for the use of their low-speed wind tunnel and laser diffraction system and assistance in analyzing the spray droplet spectrum of the broadcast nozzles used in this study.
References
Al Heidary M., Douzals J. P., Sinfort C., Vallet A. (2014). Influence of Spray Characteristics on Potential Spray Drift of Field Crop Sprayers: A Literature Review. Crop Prot 63, 120–130. doi: 10.1016/j.cropro.2014.05.006
ASABE American Society of Agricultural and Biological Engineers (2020). Spray Nozzle Classification by Droplet Spectra (St. Joseph, MI: ASABE Standard).
Aulakh J. S., Jhala A. J. (2015). Comparison of Glufosinate-Based Herbicide Programs for Broad-Spectrum Weed Control in Glufosinate-Resistant Soybean. Weed Tech. 29 (3), 419–430. doi: 10.1614/WT-D-15-00014.1
Basinger N. T., Jennings K. N., Monks D. W., Jordan D. L., Everman W. J., Hestir E. L., et al. (2019). Large Crabgrass (Digitaria Sanguinalis) and Palmer Amaranth (Amaranthus Palmeri) Intraspecific and Interspecific Interference in Soybean. Weed Sci. 67 (6), 649–656. doi: 10.1017/wsc.2019.43
Bensch C. M., Horak M. J., Peterson D. (2003). Interference of Redroot Pigweed (Amaranthus Retroflexus), Palmer Amaranth (A. Palmeri), and Common Waterhemp (A. Rudis) in Soybean. Weed Sci. 51, 37–43. doi: 10.1614/0043-1745(2003)051[0037:IORPAR]2.0.CO;2
Carlsen S. C. K., Spliid N. H., Svensmark B. (2006). Drift of 10 Herbicides After Tractor Spray Application.2. Primary drift (droplet drift) Chemosphere 64, 778–786. doi: 10.1016/j.chemosphere.2005.10.060
Carter O. W., Prostko E. P., Davis J. W. (2017). The Influence of Nozzle Type on Peanut Weed Control Programs. Peanut Sci. 2, 3–99. doi: 10.3146/PS17-4.1
Chang F. Y., Born W. H. V. (1971). Dicamba Uptake, Translocation, Metabolism, and Selectivity. Weed Sci. 19, 113–117. doi: 10.1017/S0043174500048414
Cornelius C., Bradley K. (2017). Influence of Various Cover Crop Species on Winter and Summer Annual Weed Emergence in Soybean. Weed Technol. 31 (4), 503–513. doi: 10.1017/wet.2017.23
Creech C. F., Henry R. S., Fritz B. K., Kruger G. R. (2015a). Influence of Herbicide Active Ingredient, Nozzle Type, Orifice Size, Spray Pressure, and Carrier Volume Rate on Spray Droplet Size Characteristics. Weed Technol. 29 (2), 298–310. doi: 10.1614/WT-D-14-00049.1
Creech C. F., Henry R. S., Werle R., Sandell L. D., Hewitt A. J., Kruger G. R. (2015b). Performance of Postemergence Herbicides Applied at Different Carrier Volume Rates. Weed Technol. 29 (3), 611–624. doi: 10.1614/WT-D-14-00101.1
Creech C. F., Moraes J. G., Henry R. S., Luck J. D., Kruger G. R. (2016). The Impact of Spray Droplet Size on the Efficacy of 2,4-D, Atrazine, Chlorimuron-Methyl, Dicamba, Glufosinate, and Saflufenacil. Weed Technol. 30 (2), 573–586. doi: 10.1614/WT-D-15-00034.1
Dorr G., Hanan J., Adkins S., Hewitt A., O’Donnell C., Noller B. (2008). Spray Deposition on Plant Surfaces: A Modelling Approach. Funct. Plant Biol. 35, 988–996. doi: 10.1071/FP08056
Ferguson J.C., Hewitt A.J., O’Donnell C.C (2016). Pressure, Droplet Size Classification, and Nozzle Arrangement Effects on Coverage and Droplet Number Density Using Air-Inclusion Dual Fan Nozzles for Pesticide Applications. Crop Prot 89, 231–238.
Fritz B. K., Hoffmann W. C., Czaczyk Z., Bagley W., Kruger G., Henry R. (2012). Measurement and Classification Methods Using the ASAE S572.1 Reference Nozzles. J. Plant Prot Res. 52 (4), 447–457. doi: 10.2478/v10045-012-0072-x
Fritz B. K., Hoffmann W. C., Jank P. (2011). A Fluorescent Tracer Method for Evaluating Spray Transport and Fate of Field and Laborartoy Spray Applications. J. ASTM Int. 8 (3), 1–9. doi: 10.1520/JAI103619
Johnson V., Fisher L., Jordan D., Edmisten K., Stewart A., York A. (2012). Cotton, Peanut, and Soybean Response to Sublethal Rates of Dicamba, Glufosinate, and 2,4-D. Weed Technol. 26, 195–206. doi: 10.1614/WT-D-11-00054.1
Knoche M. (1994). Effect of Droplet Size and Carrier Volume on Performance of Foliage-Applied Herbicides. Crop Prot. 13, 163–178. doi: 10.1016/0261-2194(94)90075-2
Kruger G. R., Johnson W. G., Doohan D. J., Weller S. C. (2012). Dose Response of Glyphosate and Dicamba on Tomato (Lycopersicon Esculentum) Injury. Weed Technol. 26, 256–260. doi: 10.1614/WT-D-11-00073.1
Legleiter T. R., Johnson W. G. (2016). Herbicide Coverage in Narrow Row Soybean as Influenced by Spray Nozzle Design and Carrier Volume. Crop Prot. 83, 1–8. doi: 10.1016/j.cropro.2016.01.009
Marth P. C., Mitchell J. W. (1944). 2, 4-Dichlorophenoxyacetic Acid as a Differential Herbicide. Bot. Gaz 106, 224–232. doi: 10.1086/335289
Meyer C. J., Norsworthy J. K., Kruger G. R., Barber T. (2016a). Effect of Nozzle Selection and Spray Volume on Droplet Size and Efficacy of Engenia Tank-Mix Combinations. Weed Tech. 30, 377–390. doi: 10.1614/WT-D-15-00141.1
Meyer C. J., Norsworthy J. K., Kruger G. R., Barber T. (2016b). Effects of Nozzle Selection and Ground Speed on Efficacy of Liberty and Engenia Applications and Their Implication on Commercial Field Applications. Weed Tech. 30 (2), 401–414. doi: 10.1614/WT-D-15-00145.1
Nuyttens D., Baetens K., De Schampheleire M., Sonck B. (2007). Effect of Nozzle Type, Size, and Pressure on Spray Droplet Characteristics. Biosyst. Eng. 97, 333–345. doi: 10.1016/j.biosystemseng.2007.03.001
Nuyttens D., De Schampheleire M., Steurbaut W., Baetens K., Verboven P., Nicolai B., et al. (2006). Experimental Study of Factors Influencing the Risk of Drift From Field Sprayers Part 2: Spray Application Technique. Aspects Appl. Biol. 77 (2), 331–339.
Robinson A. P., Simpson D. M., Johnson W. G. (2013). Response of Glyphosate-Tolerant Soybean Yield Components to Dicamba Exposure. Weed Sci. 61, 526–536. doi: 10.1614/WS-D-12-00203.1
Sousa Alves G., Kruger G., Da Cunha J., De Santana D., Guimaraes F., Zaric M. (2017). Dicamba Spray Drift as Influenced by Wind Speed and Nozzle Type. Weed Technol. 32, 724–731. doi: 10.1017/wet.2017.61
USEPA United States Environmental Protection Agency (2020) Registration of Dicamba for Use on Dicamba-Tolerant Crops. Available at: https://www.epa.gov/ingredients-used-pesticide-products/registration-dicamba-use-genetically-engineered-crops (Accessed December 15, 2020).
Vyn J., Sikkema C., Swanton S., Weaver P. (2007). Control of Herbicide-Resistant Common Waterhemp (Amaranthus Tuberculatus Var. Rudis) With Pre- and Post-Emergence Herbicides in Soybean. Can. J. Plant Sci. 87, 175–182. doi: 10.4141/P06-016
Keywords: drift reduction, herbicide spray deposition, herbicide spray coverage, weed density, spray droplet diameter
Citation: Kramer MD and Legleiter TR (2022) Influence of Broadcast Nozzle Design and Weed Density on Dicamba Plus Glyphosate Deposition, Coverage, and Efficacy in Dicamba-Resistant Soybean. Front. Agron. 4:903669. doi: 10.3389/fagro.2022.903669
Received: 24 March 2022; Accepted: 04 May 2022;
Published: 02 June 2022.
Edited by:
Hanwen Wu, New South Wales Department of Primary Industries, AustraliaReviewed by:
Kassio Ferreira Mendes, Federal University of Viçosa, BrazilMaxwel Coura Oliveira, University of Wisconsin-Madison, United States
Copyright © 2022 Kramer and Legleiter. This is an open-access article distributed under the terms of the Creative Commons Attribution License (CC BY). The use, distribution or reproduction in other forums is permitted, provided the original author(s) and the copyright owner(s) are credited and that the original publication in this journal is cited, in accordance with accepted academic practice. No use, distribution or reproduction is permitted which does not comply with these terms.
*Correspondence: Travis R. Legleiter, VHJhdmlzLkxlZ2xlaXRlckB1a3kuZWR1; orcid.org/0000-0002-5278-3495