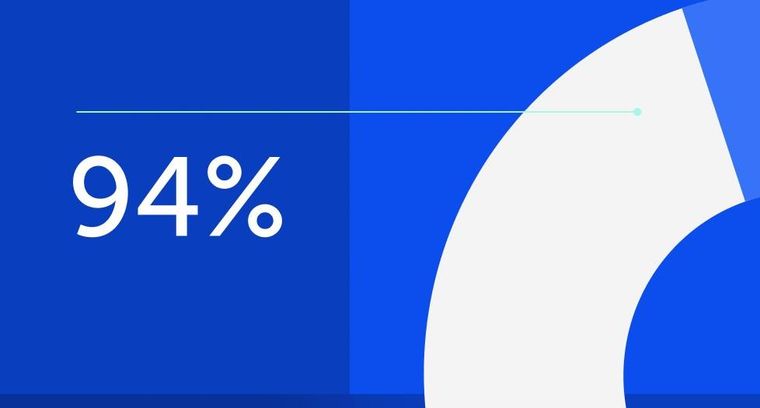
94% of researchers rate our articles as excellent or good
Learn more about the work of our research integrity team to safeguard the quality of each article we publish.
Find out more
ORIGINAL RESEARCH article
Front. Agron., 25 May 2022
Sec. Weed Management
Volume 4 - 2022 | https://doi.org/10.3389/fagro.2022.887629
This article is part of the Research TopicInsights in Weed ManagementView all 5 articles
Palmer amaranth (Amaranthus palmeri S. Watson) is one of the most troublesome agronomic weed species in the United States. Palmer amaranth is prevalent in the Southern Great Plains and the Southeastern United States, and its range is expanding northward through natural dispersal and human intervention. Palmer amaranth dispersal warrants studies assessing species adaptation into new geographies. A study was conducted in 2018 and 2019 to investigate the morphology, flowering, and gender from cohorts of Palmer amaranth growing under corn, soybean, and bareground across five locations in the Midwest United States. Results demonstrated that the first cohort of Palmer amaranth, established in June, produced 42% more biomass than plants from the second cohort (established in July). The first Palmer amaranth cohort produced 75.5 g plant-1 in bareground, 28.3 g plant-1 in soybean, and 16.3 g plant-1 in corn, whereas the second Palmer amaranth cohort produced 62.6, 6.3, and 1.4 g plant-1 in bareground, soybean, and corn, respectively. Palmer amaranth height was most impacted when growing in corn and averaged 85.2 cm tall in the first cohort, and 38.2 cm tall in the second cohort in corn. Moreover, Palmer amaranth flowering window shifted according to crop and cohort timings. Palmer amaranth growing in intense competition, such as under low light in corn, resulted in the longest flowering window. Palmer amaranth gender was slightly influenced by day of year, weight, and height. We documented a high degree of plasticity in Palmer amaranth, which will presumably favor its adaptation and expansion in cropping systems north of its current range. Therefore, preventing Palmer amaranth dispersal into new habitats is the most effective management strategy.
Palmer amaranth (Amaranthus palmeri S. Watson) is currently ranked as one of the most economically detrimental weed species to cropping systems in the United States (Van Wychen, 2020). Unmanaged Palmer amaranth plants compete for water, light, and nutrients, which can drastically impact crop yields (Berger et al., 2015). For example, Palmer amaranth has been documented to reduce up to 91, 68, and 54% corn (Massinga et al., 2001), soybean (Klingaman and Oliver, 1994), and cotton (Morgan et al., 2001) yields, respectively. Moreover, Palmer amaranth has shown a remarkable capacity to evolve resistance to herbicides. To date, Palmer amaranth has evolved resistance to eight herbicide sites of action (Heap, 2022), increasing the weed management complexity (Lindsay et al., 2017) and posing an economical and ecological risk to row-crop agriculture.
Palmer amaranth as a problem weed is a function of both inherent adaptations and selected management practices. Palmer amaranth is a fast growing summer annual forb indigenous to the Sonoran Desert (Sauer, 1957). It became a serious problem weed in US agriculture in the 1990s (Ward et al., 2013). Palmer amaranth weediness is likely a result of human-assisted selection combined with plant biology. Farm mechanization, adoption of conservation agriculture (e.g., no-till), and intensive use of herbicides for weed management are the main human-mediated selections of Palmer amaranth in cropping systems (Ward et al., 2013). Palmer amaranth is a prolific seed producer with a C4 photosynthetic apparatus (Wang et al., 1992). With dioecious nature, Palmer amaranth male and female plants are obligate outcrossers, increasing the chances of exchanging adaptive traits among plants (Oliveira et al., 2018; Jhala et al., 2021). Also, Palmer amaranth's small seeds (e.g., 1 mm) tend to thrive in no-tillage systems (Price et al., 2011), and spread across locations through farm equipment (Sauer, 1972), seed mixes (Hartzler and Anderson, 2016), wildlife (Farmer et al., 2017), etc., making it one of the most successful examples of weed adaptation to current cropping systems.
Palmer amaranth's plasticity allows it to respond successfully to environmental changes. Palmer amaranth demonstrates a high degree of plasticity to light, temperature, water availability, and human management (Jha et al., 2010). Palmer amaranth has an extended germination period throughout the growing season (Ward et al., 2013). Germination of Palmer amaranth was triggered by 18°C soil temperature at 5 cm depth (Keeley et al., 1987), and optimal germination and biomass production occurred at 35/30°C day and night temperatures (Guo and Al-Khatib, 2003). Palmer amaranth emergence can be influenced by tillage and the use of preemergence herbicides (Chahal et al., 2021), and may result in weed germination shifts within a population, as documented in Bassia scoparia (Sbatella and Wilson, 2010). In an experiment where Palmer amaranth was subjected to continuous water stress, it survived and produced at least 14,000 seeds plant-1 (Chahal et al., 2018). Seeds from Palmer amaranth growing with limited water conditions were heavier, less dormant, and prompt for germination (Matzrafi et al., 2021). Growing conditions and management practices also influence Palmer amaranth sex dimorphism and flowering pattern (Korres et al., 2017; Rumpa et al., 2019).
Palmer amaranth's current global range includes agronomic cropland in Italy (Milani et al., 2021), soybean producing regions of Brazil and Argentina (Küpper et al., 2017; Larran et al., 2017), and the Cotton Belt (Bagavathiannan and Norsworthy, 2016; Garetson et al., 2019) and southern Great Plains of the United States (Crespo et al., 2016; Kumar et al., 2020). However, its range appears to be expanding steadily, and in some cases rapidly, in the United States. We hypothesize that in states with large Palmer amaranth infestations its range is gradually pushing north as it displaces other weeds in agronomic row crops, aided by wildlife (Farmer et al., 2017) and agricultural machinery (Ward et al., 2013). But there are other examples where it has suddenly become a problem weed hundreds of miles away from known infestations. New infestations in Michigan, Indiana, and North Dakota appear to have resulted from Palmer amaranth contaminated livestock feed. New infestations in Iowa and Minnesota were associated with planting contaminated pollinator habitat seeds (Yu et al., 2021). Palmer amaranth infestations have not been detected in Canada, but Palmer amaranth seeds were found in sweet potato slips imported into the country (Page et al., 2021). Global warming will create new opportunities for Palmer amaranths invasion. Although agronomic crops in warm environments like Australia and Sub-Saharan Africa are currently at the greatest risk for Palmer amaranth invasion, warming temperatures will reduce barriers that may have limited Palmer amaranths spread into cooler climates like Canada and Northern Europe (Kistner and Hatfield, 2018; Briscoe Runquist et al., 2019).
There are many areas in the US Corn Belt (North Central states) where Palmer amaranth is not yet established, and its potential adaptability is untested. Nonetheless, the rapid expansion of Palmer amaranth across the Northern United States is concerning and warrants investigations on its adaptability. It has been shown that Palmer amaranth caused yield loss in Illinois soybean fields (Davis et al., 2015), but an Iowa study showed that Palmer amaranth was not as well adapted as waterhemp (Amaranthus tuberculatus) to conditions in that geography (Baker, 2021). It is also known that Palmer amaranth plants that establish shortly after row-crop planting have a much greater impact on crop yield than plants that emerge after the crop has produced several leaves (MacRae et al., 2013). Understanding Palmer amaranth morphology and development under different agroecosystems and across wide geography can enhance our knowledge of its adaptability, and may also aid in designing effective tactics to limit its range expansion and minimize its negative effects on row crops. The objective of this study was to investigate the flowering pattern, gender, biomass production, and height of Palmer amaranth cohorts growing in corn, soybean, and bareground environments across five locations in the North Central United States.
A Palmer amaranth accession (Kei3) from Perkins County, Nebraska susceptible to glyphosate was selected for this study (Oliveira et al., 2021). Three weeks prior to the establishment of each cohort, seeds were planted in plastic trays containing pottingmix. Emerged seedlings (1 cm) were transplanted into 200 cm-3 plastic pots (1 plant pot-1). Palmer amaranth seedlings were supplied with adequate water and kept under greenhouse conditions at the University of Wisconsin-Madison, the University of Nebraska-Lincoln, and Western Illinois University; and kept outdoors at the Perkins Extension office in Grant, NE until the 2–3 leaf stage (5–8 cm height) when they were transported to the field.
The experiment was conducted in 2018 and 2019 under field conditions at five locations (Figure 1): Arlington, WI (43.18'N, 89.29'W), Clay Center, NE (40.57'N, 9814'W), Grant, NE (40.85'N, 101.70'W), Lincoln, NE (41.16'N, 96.42'W), and Macomb, IL (40.46'N, 90.67'W).
Fields were conventionally tilled prior to crop planting. Corn and soybean were planted in 76-cm row spacing (Table 1). Monthly mean air temperature and total precipitation were obtained using Daymet weather data from June through September across the five locations in 2018 and 2019 (Correndo et al., 2021) (Figure 2).
Table 1. Field study attributes from Arlington, WI, Clay Center, NE, Grant, NE, Lincoln, NE, and Macomb, IL.
Figure 2. Mean average temperature (C) and total monthly precipitation (mm) at Arlington, WI, Clay Center, NE, Grant, NE, Lincoln, NE, and Macomb, IL.
The field experimental units were three adjacent 9.1 m wide (12 rows) by 10.7 m long. The experimental design were arranged in factorial design with three crops, two transplanting times simulating two cohorts, repeated across five locations. Each field experimental unit was planted with corn, soybean, or maintained as bareground. The two transplant timings were 1 June (first cohort) and 1 July (second cohort). Palmer amaranth seedlings (potting mix + two seedlings) were transplanted (6 cm deep and 8 cm wide). Forty-eight plants were equidistantly placed (0.76 m apart) between rows within each crop. After a week, one plant was eliminated and one was kept, resulting in 24 plants per experimental unit and transplanting time (Figure 3). When needed, Palmer amaranth plants were supplied with water during the first week after transplanting to assure seedling survival.
Figure 3. Palmer amaranth adaptation study layout of a plant cohort timing in bareground, corn, and soybean. Twenty-four Palmer amaranth plants were placed 76.2 cm apart in each field experimental unit.
After transplanting, Palmer amaranth flowering was monitored until the end of the study. When a plant flowered, the day was recorded, plant gender was identified (male or female), and plant height was measured from the soil surface to the top of the plant. Also, the aboveground plant and the aboveground plant organs were harvested, then oven dried at 65°C until a constant weight was reached, and dry biomass (g plant-1) was recorded. Plants were harvested at flowering because Palmer amaranth is not established at the Wisconsin or Illinois research locations. In our study, all locations followed the methodology of plant harvest at flowering initiation, except in Grant, NE. At the Grant, NE, location, all plants from the first cohort were harvested on 6 July 2018 or 6 July 2019, and all plants from the second cohort were harvested on 17 August 2018 or 31 July 2019, regardless of flowering status.
The statistical analyses were performed using R statistical software version 4.0.1 (R Core Team, 2021).
Analyses of Palmer amaranth height and biomass were performed with a linear mixed model using the lmer function from the “lme4” package (Bates et al., 2015). Plant height and biomass were log transformed to meet the model assumption of normality. In the model, crop (bareground, corn, and soybean) and cohort time (first and second) were the fixed effects and year nested with the location were the random effects. Analysis of variance at α 0.05 was performed with anova function from the “car” package (Fox and Weisberg, 2018). Marginal means and compact letter display were estimated with emmeans and cld from packages “emmeans” (Lenth et al., 2021) and “multcomp” (Hothorn et al., 2008), respectively.
Palmer amaranth cumulative flowering estimation was determined across all locations, except Grant, NE. Cumulative flowering estimation was determined using an asymmetrical three-parameter log-logistic Weibull model of the drc package (Ritz et al., 2015):
In this model, Y is the cumulative flowering, d is the upper limit (set to 100), e is the inflection point, and x is the day of year (doy).
The doy for 10, 50, and 90% cumulative flowering were determined using the ED function of the drc package. Also, the 10, 50, and 90% Palmer amaranth cumulative flowering were compared among crops and cohorts using the EDcomp function of the drc package. The EDcomp function compares the ratio of cumulative flowering using t-statistics, where the P-value < 0.05 indicates that we fail to reject the null hypothesis.
A binary logistic regression was fitted to Palmer amaranth's gender. Binary logistic regression is used for predicting binary classes (Bangdiwala, 2018), such as the probability of a plant being female in a dioecious species. Prior to the analysis, missing values were removed from the dataset (including all data from the Grant location). The resulting dataset was split into 80% train and 20% test data. The 80% train is used for model training and the 20% test is used for checking model performance on the unseen dataset. Using the 80% train data, a generalized linear model (base R glm function) was fitted to a binary response variable, the probability of being female (0 to male and 1 to female). The independent variables were harvest doy, height, weight, and crop (without interaction). The model family was binomial with a logit function. The model fit was assessed through pseudo R-squared values (McFadden, Cox and Snell, Cragg and Uhler) and likelihood ratio using the nagelkerke function from the “rcompanion” package (Mangiafico, 2021). The marginal effects computation was performed with Average Marginal Effects (AMEs) at every observed value of x and averaged across the results (Leeper, 2021) using the margins function from the “margins” package (Leeper et al., 2021). The 20% test data was predicted using the predict function with a cutoff estimation for males or females using the performance function from the ROCR package (Sing et al., 2005). The model quality prediction from the classification algorithm was measured with precision (precision function), recall (recall function), and F1-score (f_meas function) using the “yardstick” package (Kuhn et al., 2021). The precision determines the accuracy of positive predictions (female plants), recall determines the fraction of positives that were correctly identified, and the F1-score is a weighted harmonic mean of precision and recall with the best score of 1 and the worst score of 0 (Raoniar, 2021). F1-score conveys the balance between the precision and the recall (Yacouby and Axman, 2020). The area under the receiver operating curve (AUC-ROC) was also estimated with performance function using the true positive and false positive rates. The higher the AUC, the better the model is at distinguishing between female and male Palmer amaranth.
Palmer amaranth plants accumulated more biomass when growing in bareground compared to plants growing in soybean and corn (Figure 4A). Palmer amaranth plants in the first cohort produced 75.5, 28.3, and 16.3 g plant-1 in bareground, soybean, and corn, respectively. Plants from the second cohort produced 62.6 g plant-1 in bareground, followed by 6.3 g plant-1 in soybean, and 1.4 g plant-1 in corn.
Figure 4. Palmer amaranth biomass (A) and height (B) growing in corn, bareground, and soybean nested across Arlington, WI, Clay Center, NE, Grant, NE, Lincoln, NE, and Macomb, IL.
Palmer amaranth height was less affected by cohort timing than weight, with the exception of plants growing in corn (Figure 4B). Plants from the first cohort were on average 69.2 cm tall in bareground, which was not different from the 70.7 cm tall plants from the second cohort timing (P = 0.74). In addition, no difference in Palmer amaranth height (69.3 cm) was detected from first cohort plants in soybean to first and second cohort plants in bareground (P > 0.75). Palmer amaranth plants from the second cohort were nearly 10 cm shorter compared to the first cohort in soybeans (P = 0.04). The tallest (85.2 cm, first cohort) and shortest (38.2 cm, second cohort) plants were observed in corn.
The initiation and duration of Palmer amaranth flowering were strongly influenced by cohort and surrounding vegetation (Figures 4B, 5A). In the first cohort, floral initiation (10% flowering) occurred near the end of June for all three treatments, at doy 180, 180.9, and 181.7 for soybean, bareground, and corn, respectively. In the second cohort, floral initiation occurred earlier on plants growing in the bareground than plants growing in soybean or corn (doy 203.8 vs. doy 210.9 or 216.8, respectively). Palmer amaranth growing in the bareground had the shortest flowering initiation (measured as the difference between 10 and 90% cumulative flowering) in both the first (34 days) and second (28 days) cohorts. Palmer amaranth growing in soybean had a shorter duration of flowering than corn in the first cohort (40 days vs. 71 days), but a longer duration in the second cohort (50 vs. 44 days).
Figure 5. Cumulative flowering of Palmer amaranth at first and second transplant timing (A) and day of year (doy) of 10, 50, and 90 cumulative flowering at first and second cohort transplanting time (B) nested across Arlington, WI, Clay Center, NE, Grant, NE, Lincoln, NE, and Macomb, IL.
Palmer amaranth cumulative flowering in the second cohort occurred from mid-July to mid-September (Figure 5B). Palmer amaranth growing in the bareground resulted in earlier flowering time compared to soybean and corn. Palmer amaranth growing in bareground reached 10, 50, and 90% flowering time at days 203.8, 214.4, and 232.2, respectively. Palmer amaranth growing in soybean reached 10% flowering at doy 210.9, which was 6 days prior to corn (P-value = 0.00). A similar trend was observed at 50% flowering, whereas Palmer amaranth reached 50% flowering in corn (doy 233.0) 4 days after soybeans (doy 228.9; P = 0.00). The 90% Palmer amaranth cumulative flowering occurred on the same day in corn (260.9) and soybean (260.5; P = 0.66).
The model goodness of fit was 0.23, 0.32, 0.40 using the pseudo R-squared test from McFadden, Cox and Snell, and Cragg and Uhler, respectively. The likelihood ratio test showed a p-value of 0.00. The average marginal effects showed that Palmer amaranth growing in corn resulted in 14.8% fewer females plants (Table 2). Moreover, increasing a cumulative flowering unit doy increases the probability of having a female plant by 0.4% (Table 2 and Figure 6A). A similar trend was observed for the weight (Figure 6B) and height (Figure 6C), where the probability of being female increased by 0.1 and 0.2% for each unit increase in weight and height, respectively (Table 2).
Figure 6. The probability (P) of being female Palmer amaranth by doy (A), weight (B), and height (C). Black line represents the model estimation and shaded green the confidence intervals.
The model accuracy evaluation accuracy in the 20% test dataset was 0.62 with a cutoff value for female and male plants of 0.43. The model classification showed a precision of 0.64, recall of 0.66, and an F1-score of 0.65. In addition, the AUC was 0.64.
Our study demonstrates that Palmer amaranth is well-adapted to growing conditions throughout the Midwestern US, and is simply limited in its range by seed dispersal (Davis et al., 2015). We also confirmed Palmer amaranth's extraordinary plasticity to adapt to different agroecosystems. For example, Palmer amaranth mimicked crop architecture in competing for light, where plants growing in corn allocated resources to height, while plants growing in bareground allocated resources to numerous branches. Plants growing in the absence of a crop produced the greatest biomass, responding to a greater abundance of light, nutrients, and water resources (Figures 4A,B). These results support the argument that Palmer amaranth can quickly evolve life-history traits to adapt to different cultural practices, similar to observations in a Palmer amaranth response to nitrogen study (Bravo et al., 2018). Our results highlight Palmer amaranth as a threat to field crops and breeding more competitive crop varieties is likely to select more competitive weed biotypes (Bravo et al., 2017).
Palmer amaranth growth and development in the second cohort were limited due to the crop's competitive ability at advanced developmental stages. Palmer amaranth seedlings were transplanted at greater crop height and width, which reduced Palmer amaranth competitiveness. As a result, Palmer amaranth height and biomass were lower compared to its first cohort. Moreover, Palmer amaranth growing without crop competition produced the highest amounts of biomass. The Palmer amaranth strategy in bareground was to invest biomass in growing plant width and height. Nonetheless, Palmer amaranth produced 17% less biomass in the second cohort compared to the first cohort timing. In a bareground study, early emerged Palmer amaranth without competition was 50% taller than late emerged plants (Webster and Grey, 2015). These results suggest that crop competition is not the only factor limiting late Palmer amaranth establishment. The limited growth of Palmer amaranth in the second cohort is likely a reduced plant response to day length, light availability, and thermal units (e.g., growing degree days). The Amaranthus species are sensitive to photoperiod (Wu and Owen, 2014). We hypothesize that reduced day length or red/far-red effect contributed to smaller plants in the second cohort regardless of the crop. A study in North Carolina and Illinois predicted that less than 10% of Palmer amaranth seedlings' emergence occurred after June (Piskackova et al., 2021). In addition, Palmer amaranth's negative impact on soybean (Korres et al., 2020) and cotton (Webster and Grey, 2015) yields were higher when plants were established close to crop planting. Therefore, early season management is a key strategy to minimize the damaging impact of Palmer amaranth on US Midwest cropping systems.
Seed production was not evaluated due to plant harvest at the initiation of flowering. Nonetheless, a strong positive correlation between Palmer amaranth biomass and seed production has been documented (Schwartz et al., 2016; Spaunhorst et al., 2018). In our study, plants growing from the first cohort accumulated 42% more biomass when compared to the second cohort. Therefore, Palmer amaranth plants growing in the second cohort are likely to produce fewer seeds regardless of crop. Our observation is consistent with the findings that the first Palmer amaranth cohort produced 50% more seeds per plant than Palmer amaranth plants established 6 weeks later in bareground (Webster and Grey, 2015). Still, seed production in the second cohort will replenish the soil seedbank. Seed production and deposition in the seedbank is also a key factor for species perpetuation (Menges, 1987). Palmer amaranth can produce more than a hundred thousand seeds per plant (Schwartz et al., 2016), which can stay viable in the soil seedbank for at least 36 months (Sosnoskie et al., 2013). Therefore, preventing Palmer amaranth seed production or/and seed migration to non-native habitats is an essential strategy to minimize the species' impact in agroecosystems.
An ecological approach to reducing Palmer amaranth seed production encompasses understanding plant biology, including flowering patterns. Floral initiation depends on the complex interaction between a plant's genetic makeup and environmental conditions (Lang, 1965). We observed a significant disruption in flowering caused by surrounding vegetation and cohort timing. Although all three treatments began flowering at the same time in the first cohort (about doy 180), it took much less time for the bareground treatment to reach 90% flowering (34 days vs. 40 for soybean and 71 for corn). The lack of competition (and stress) in the bareground resulted in plants that flowered earlier, and were they not harvested, would have had longer reproductive periods, thereby producing more seeds. Similarly, in the second cohort, the window for all plants to initiate flowering was much shorter for the bareground (28 days) compared to Palmer amaranth in soybean (50 days) or corn (44 days). Significantly, plants in the bareground treatment flowered 1 week earlier than soybean, and almost 2 weeks earlier than corn, again allowing for greater seed production per plant had they not been harvested at initial flowering. In some cases, early flowering may confer an evolutionary advantage, provided the plant has an indeterminate habit and flowering does not restrict plant growth. In other cases, however, early flower initiation may be a response to stressful conditions as a plant attempts to reproduce before running out of resources. In an Iowa study, Palmer amaranth initiated flowering 2 weeks prior to waterhemp (Baker, 2021), and this characteristic may partially explain why waterhemp can displace Palmer amaranth in Iowa, where Palmer amaranth is not well adapted. In a dioecious species like Palmer amaranth, exerting stress on plants to manipulate flowering may be beneficial in limiting seed production (Schliekelman et al., 2005; McFarlane et al., 2018). For example, when growing under water stress, there was a 7-day flowering mismatch between male and female plants (Mesgaran et al., 2021), which can minimize plant outcrossing, and reduce seed production and the exchange of resistant alleles (Jhala et al., 2021).
The mechanisms of gender determination in plant species are intriguing and have aroused the curiosity of many scientists, including Charles Darwin (Darwin, 1888). In our study, the gender model performance was decent (AUC 0.64) considering the biology of plant flowering. A 1:1 male and female sex ratio is a general and evolutionarily stable strategy for plant species perpetuation (Fisher, 1930). However, a slight deviation from the 1:1 sex ratio occurs in some dioecious species. For example, the dioecious Halophila stipulacea is a female-biased plant in its native habitat, but the naturalized H. stipulacea has a 1:1 ratio (Nguyen et al., 2018). The naturalization of H. stipulacea reduced the female-male ratio to expand into its non-native habitat (Morgan et al., 2001). Also, biotic and/or abiotic stress can influence plant gender determination. Palmer amaranth male-to-female ratio was greater under high plant densities (Korres and Norsworthy, 2017) and after herbicide application (Rumpa et al., 2019). We observed sexual dimorphism in Palmer amaranth in response to surrounding vegetation and plant morphological attributes. Our model estimated that late flowering, heavier and taller Palmer amaranth plants slightly deviated from the 1:1 ratio in favor of female plants. It was reported that female Palmer amaranth plants invested more in height, stem, and biomass while male plants invested more in leaf area and leaf dry weight under nutrient deficiency (Korres et al., 2017). We observed more female plants in soybean and bareground compared to corn. Palmer amaranth plants in the corn were more stressed by interspecific competition as evidenced by less biomass and a lower weight:height ratio. Sexual dimorphism is documented in other dioecious species (Barrett and Hough, 2013). For example, stronger female plant competition and greater male tolerance to herbivory were reported in Spinacia oleracea (Pérez-Llorca and Sánchez Vilas, 2019). Research on candidate genes for sex determination in Amaranthus species is currently underway but is far from complete (Montgomery et al., 2019, 2021). Further studies are also needed to understand the ecological basis of Palmer amaranth flowering, including plant behavior under climate change.
Our study demonstrated that Palmer amaranth is adapted to grow on arable land throughout the North Central United States. Palmer amaranth's range will continue to expand if current cropping practices are continued. As waterhemp and Palmer amaranth begin to share the same habitat, it will increase weed management complexity. Preventing Palmer amaranth seed dispersal must be a priority. Regional collaboration is necessary to slow the spread of this aggressive and adaptable weed. Where Palmer amaranth occurs, management tactics should focus on limiting Palmer amaranth establishment until row crops can shade late-emerging plants. Increasing the diversity of crops in rotation, varying row crop planting date, narrowing row width, and increasing residue cover through the use of cover crops are all tactics that can minimize the growth and seed production of Palmer amaranth, and improve the sustainability of cropping systems in the North Central US.
The data and scripts used to analyze the data presented in this work can be found at Zenodo (Oliveira, 2021).
RW and MO designed the experiments. AJ, CP, MB, MO, and SS conducted the experiments. MO analyzed the data and wrote the manuscript. AJ, CP, MB, MO, SS, and RW conceptualized the research. All authors reviewed and revised the manuscript. All authors contributed to the article and approved the submitted version.
The authors declare that the research was conducted in the absence of any commercial or financial relationships that could be construed as a potential conflict of interest.
All claims expressed in this article are solely those of the authors and do not necessarily represent those of their affiliated organizations, or those of the publisher, the editors and the reviewers. Any product that may be evaluated in this article, or claim that may be made by its manufacturer, is not guaranteed or endorsed by the publisher.
Bagavathiannan, M. V., and Norsworthy, J. K. (2016). Multiple-herbicide resistance is widespread in roadside Palmer Amaranth populations. PLoS ONE 11:e0148748. doi: 10.1371/journal.pone.0148748
Baker, R. (2021). Comparative analysis of Palmer amaranth (Amaranthus palmeri) and waterhemp (A. Tuberculatus) in Iowa (dissertion/master's thesis). Iowa State University, Ames, IA, United States. doi: 10.31274/etd-20210609-11
Bangdiwala, S. I. (2018). Regression: binary logistic. Int. J. Inj. Control Saf. Promot. 25, 336–338. doi: 10.1080/17457300.2018.1486503
Barrett, S. C. H., and Hough, J. (2013). Sexual dimorphism in flowering plants. J. Exp. Bot. 64, 67–82. doi: 10.1093/jxb/ers308
Bates, D., Mächler, M., Bolker, B., and Walker, S. (2015). Fitting linear mixed-effects models using Lme4. J. Stat. Softw. 67, 1–48. doi: 10.18637/jss.v067.i01
Berger, S. T., Ferrell, J. A., Rowland, D. L., and Webster, T. M. (2015). Palmer Amaranth (Amaranthus palmeri) competition for water in cotton. Weed Sci. 63, 928–935. doi: 10.1614/WS-D-15-00062.1
Bravo, W., Leon, R. G., Ferrell, J. A., Mulvaney, M. J., and Wood, C. W. (2017). Differentiation of life-history traits among Palmer Amaranth populations (Amaranthus palmeri) and its relation to cropping systems and glyphosate sensitivity. Weed Sci. 65, 339–349. doi: 10.1017/wsc.2017.14
Bravo, W., Leon, R. G., Ferrell, J. A., Mulvaney, M. J., and Wood, C. W. (2018). Evolutionary adaptations of Palmer Amaranth (Amaranthus palmeri) to nitrogen fertilization and crop rotation history affect morphology and nutrient-use efficiency. Weed Sci. 66, 180–189. doi: 10.1017/wsc.2017.73
Briscoe Runquist, R. D., Lake, T., Tiffin, P., and Moeller, D. A. (2019). Species distribution models throughout the invasion history of Palmer amaranth predict regions at risk of future invasion and reveal challenges with modeling rapidly shifting geographic ranges. Sci. Rep. 9:2426. doi: 10.1038/s41598-018-38054-9
Chahal, P. S., Barnes, E. R., and Jhala, A. J. (2021). Emergence pattern of Palmer amaranth (Amaranthus palmeri) influenced by tillage timings and residual herbicides. Weed Technol. 35, 433–439. doi: 10.1017/wet.2020.136
Chahal, P. S., Irmak, S., Jugulam, M., and Jhala, A. J. (2018). Evaluating effect of degree of water stress on growth and fecundity of Palmer amaranth (Amaranthus palmeri) using soil moisture sensors. Weed Sci. 66, 738–745. doi: 10.1017/wsc.2018.47
Correndo, A. A., Moro Rosso, L. H., and Ciampitti, I. A. (2021). Retrieving and processing agro-meteorological data from API-client sources using R software. BMC Res. Notes 14:205. doi: 10.1186/s13104-021-05622-8
Crespo, R. J., Wingeyer, A. B., Borman, C. J., and Bernards, M. L. (2016). Baseline sensitivity of nebraska waterhemp and Palmer Amaranth to Dicamba and 2,4-D. Agron. J. 108, 1649–1655. doi: 10.2134/agronj2015.0465
Darwin, C. (1888). The Different Forms of Flowers on Plants of the Same Species. J. Murray Available online at: http://books.google.com?id=7uMEAAAAYAAJ (accessed August 13, 2021).
Davis, A. S., Schutte, B. J., Hager, A. G., and Young, B. G. (2015). Palmer Amaranth (Amaranthus palmeri) damage niche in Illinois soybean is seed limited. Weed Sci. 63, 658–668. doi: 10.1614/WS-D-14-00177.1
Farmer, J. A., Webb, E. B., Pierce, R. A., and Bradley, K. W. (2017). Evaluating the potential for weed seed dispersal based on waterfowl consumption and seed viability. Pest Manage. Sci. 73, 2592–2603. doi: 10.1002/ps.4710
Fox, J., and Weisberg, S. (2018). An R Companion to Applied Regression. SAGE Publications. Available online at: http://books.google.com?id=uPNrDwAAQBAJ (accessed January 15, 2021).
Garetson, R., Singh, V., Singh, S., Dotray, P., and Bagavathiannan, M. (2019). Distribution of herbicide-resistant Palmer amaranth (Amaranthus palmeri) in row crop production systems in Texas. Weed Technol. 33, 355–365. doi: 10.1017/wet.2019.14
Guo, P., and Al-Khatib, K. (2003). Temperature effects on germination and growth of redroot pigweed (Amaranthus retroflexus), Palmer amaranth (A. Palmeri), and common waterhemp (A. rudis). Weed Sci. 51, 869–875. doi: 10.1614/P2002-127
Hartzler, B., and Anderson, M. (2016). “Palmer Amaranth: it's here, now what?” in 2016 Integrated Crop Management Conference (Ames, IA: Iowa State University). doi: 10.31274/icm-180809-205
Heap, I. (2022). Internation Herbicide-Resistant Weed Database. Available online at: http://www.weedscience.org/Home.aspx (accessed January26, 2022).
Hothorn, T., Bretz, F., and Westfall, P. (2008). Simultaneous inference in general parametric models. Biometr. J. 50, 346–363. doi: 10.1002/bimj.200810425
Jha, P., Norsworthy, J. K., Riley, M. B., and Bridges, W. (2010). Annual changes in temperature and light requirements for germination of Palmer Amaranth (Amaranthus palmeri) seeds retrieved from soil. Weed Sci. 58, 426–432. doi: 10.1614/WS-D-09-00038.1
Jhala, A. J., Norsworthy, J. K., Ganie, Z. A., Sosnoskie, L. M., Beckie, H. J., Mallory-Smith, C. A., et al. (2021). Pollen-mediated gene flow and transfer of resistance alleles from herbicide-resistant broadleaf weeds. Weed Technol. 35, 173–187. doi: 10.1017/wet.2020.101
Keeley, P. E., Carter, C. H., and Thullen, R. J. (1987). Influence of planting date on growth of Palmer Amaranth (Amaranthus palmeri). Weed Sci. 35, 199–204. doi: 10.1017/S0043174500079054
Kistner, E. J., and Hatfield, J. L. (2018). Potential geographic distribution of Palmer Amaranth under current and future climates. Agric. Environ. Lett. 3:170044. doi: 10.2134/ael2017.12.0044
Klingaman, T. E., and Oliver, L. R. (1994). Palmer Amaranth (Amaranthus palmeri) interference in soybeans (Glycine max). Weed Sci. 42, 523–527. doi: 10.1017/S0043174500076888
Korres, N. E., and Norsworthy, J. K. (2017). Palmer Amaranth (Amaranthus palmeri) demographic and biological characteristics in wide-row soybean. Weed Sci. 65, 491–503. doi: 10.1017/wsc.2017.12
Korres, N. E., Norsworthy, J. K., FitzSimons, T., Roberts, T. L., and Oosterhuis, D. M. (2017). Differential response of Palmer Amaranth (Amaranthus palmeri) gender to abiotic stress. Weed Sci. 65, 213–227. doi: 10.1017/wsc.2016.34
Korres, N. E., Norsworthy, J. K., Mauromoustakos, A., and Williams, M. M. (2020). Soybean density and Palmer amaranth (Amaranthus palmeri) establishment time: effects on weed biology, crop yield, and economic returns. Weed Sci. 68, 467–475. doi: 10.1017/wsc.2020.41
Kuhn, M., Vaughan, D., and RStudio (2021). Yardstick: Tidy Characterizations of Model Performance. Available online at: https://CRAN.R-project.org/package=yardstick (accessed August 24, 2021).
Kumar, V., Liu, R., and Stahlman, P. W. (2020). Differential sensitivity of Kansas Palmer amaranth populations to multiple herbicides. Agron. J. 112, 2152–2163. doi: 10.1002/agj2.20178
Küpper, A., Borgato, E. A., Patterson, E. L., Netto, A. G., Nicolai, M., de Carvalho, S. J. P., et al. (2017). Multiple resistance to glyphosate and acetolactate synthase inhibitors in Palmer Amaranth (Amaranthus palmeri) identified in Brazil. Weed Sci. 65, 317–326. doi: 10.1017/wsc.2017.1
Lang, A. (1965). “Physiology of flower initiation,” in Differenzierung und Entwicklung/Differentiation and Development Handbuch der Pflanzenphysiologie/Encyclopedia of Plant Physiology, ed A. Lang (Berlin; Heidelberg: Springer), 1380–1536. doi: 10.1007/978-3-642-50088-6_39
Larran, A. S., Palmieri, V. E., Perotti, V. E., Lieber, L., Tuesca, D., and Permingeat, H. R. (2017). Target-site resistance to acetolactatesynthase (ALS)-inhibiting herbicides in Amaranthus palmeri from Argentina. Pest Manage. Sci. 73, 2578–2584. doi: 10.1002/ps.4662
Leeper, T. J. (2021). Interpreting Regression Results Using Average Marginal Effects With R's Margins. 31. Available online at: https://cran.r-project.org/web/packages/margins/vignettes/TechnicalDetails.pdf (accessed August 23, 2021).
Leeper, T. J., Arnold, J., Arel-Bundock, V., and Long, J. A. (2021). Margins: Marginal Effects for Model Objects. Available online at: https://CRAN.R-project.org/package=margins (accessed August 24, 2021).
Lenth, R. V., Buerkner, P., Herve, M., Love, J., Riebl, H., and Singmann, H. (2021). Emmeans: Estimated Marginal Means, aka Least-Squares Means. Available online at: https://CRAN.R-project.org/package=emmeans (accessed August 24, 2021).
Lindsay K. Popp M. Norsworthy J. Bagavathiannan M. Powles S. and Lacoste M. (2017). PAM: decision support for long-term Palmer Amaranth (Amaranthus palmeri) control. Weed Technol. 31, 915–927. doi: 10.1017/wet.2017.69
MacRae, A. W., Webster, T. M., Sosnoskie, L. M., Culpepper, A. S., and Kichler, J. M. (2013). Cotton yield loss potential in response to length of Palmer Amaranth (Amaranthus palmeri) interference. J. Cotton Sci. 17, 227–232.
Mangiafico, S. (2021). Rcompanion: Functions to Support Extension Education Program Evaluation. Available online at: https://CRAN.R-project.org/package=rcompanion (accessed August 24, 2021).
Massinga, R. A., Currie, R. S., Horak, M. J., and Boyer, J. (2001). Interference of Palmer amaranth in corn. Weed Sci. 49, 202–208. doi: 10.1614/0043-1745(2001)049[0202:IOPAIC]2.0.CO;2
Matzrafi, M., Osipitan, O. A., Ohadi, S., and Mesgaran, M. B. (2021). Under pressure: maternal effects promote drought tolerance in progeny seed of Palmer amaranth (Amaranthus palmeri). Weed Sci. 69, 31–38. doi: 10.1017/wsc.2020.75
McFarlane, G. R., Whitelaw, C. B. A., and Lillico, S. G. (2018). CRISPR-based gene drives for pest control. Trends Biotechnol. 36, 130–133. doi: 10.1016/j.tibtech.2017.10.001
Menges, R. M. (1987). Weed seed population dynamics during six years of weed management systems in crop rotations on irrigated soil. Weed Sci. 35, 328–332.
Mesgaran, M. B., Matzrafi, M., and Ohadi, S. (2021). Sex dimorphism in dioecious Palmer amaranth (Amaranthus palmeri) in response to water stress. Planta 254:17. doi: 10.1007/s00425-021-03664-7
Milani, A., Panozzo, S., Farinati, S., Iamonico, D., Sattin, M., Loddo, D., et al. (2021). Recent discovery of Amaranthus palmeri S. Watson in Italy: characterization of ALS-resistant populations and sensitivity to alternative herbicides. Sustainability 13:7003. doi: 10.3390/su13137003
Montgomery, J. S., Giacomini, D. A., Weigel, D., and Tranel, P. J. (2021). Male-specific Y-chromosomal regions in waterhemp (Amaranthus tuberculatus) and Palmer amaranth (Amaranthus palmeri). New Phytol. 229, 3522–3533. doi: 10.1111/nph.17108
Montgomery, J. S., Sadeque, A., Giacomini, D. A., Brown, P. J., and Tranel, P. J. (2019). Sex-specific markers for waterhemp (Amaranthus tuberculatus) and Palmer amaranth (Amaranthus palmeri). Weed Sci. 67, 412–418. doi: 10.1017/wsc.2019.27
Morgan, G. D., Baumann, P. A., and Chandler, J. M. (2001). Competitive impact of Palmer Amaranth (Amaranthus palmeri) on cotton (Gossypium hirsutum) development and yield. Weed Technol. 15, 408–412. doi: 10.1614/0890-037X(2001)015[0408:CIOPAA]2.0.CO;2
Nguyen, H. M., Kleitou, P., Kletou, D., Sapir, Y., and Winters, G. (2018). Differences in flowering sex ratios between native and invasivepopulations of the seagrass Halophila stipulacea. Botan. Mar. 61, 337–342. doi: 10.1515/bot-2018-0015
Oliveira, M. C. (2021). Data from: Palmer amaranth (Amaranthus palmeri) adaptation to US Midwest agroecosystems. Zenodo Digital Repository. Available online at: https://doi.org/10.5281/zenodo.5236831
Oliveira, M. C., Gaines, T. A., Patterson, E. L., Jhala, A. J., Irmak, S., Amundsen, K., et al. (2018). Interspecific and intraspecific transference of metabolism-based mesotrione resistance in dioecious weedy Amaranthus. Plant J. 96, 1051–1063. doi: 10.1111/tpj.14089
Oliveira, M. C., Giacomini, D. A., Arsenijevic, N., Vieira, G., Tranel, P. J., and Werle, R. (2021). Distribution and validation of genotypic and phenotypic glyphosate and PPO-inhibitor resistance in Palmer amaranth (Amaranthus palmeri) from southwestern Nebraska. Weed Technol. 35, 65–76. doi: 10.1017/wet.2020.74
Page, E. R., Nurse, R. E., Meloche, S., Bosveld, K., Grainger, C., Obeid, K., et al. (2021). Import of Palmer amaranth (Amaranthus palmeri S. Wats.) seed with sweet potato (Ipomea batatas (L.) Lam) slips. Can. J. Plant Sci. doi: 10.1139/CJPS-2020-0321
Pérez-Llorca, M., and Sánchez Vilas, J. (2019). Sexual dimorphism in response to herbivory and competition in the dioecious herb Spinacia oleracea. Plant Ecol. 220, 57–68. doi: 10.1007/s11258-018-0902-7
Piskackova, T. A. R., Reberg-Horton, S. C., Richardson, R. J., Jennings, K. M., Franca, L., Young, B. G., et al. (2021). Windows ofaction for controlling palmer amaranth (Amaranthus palmeri) using emergence and phenology models. Weed Res. 61, 188–198. doi: 10.1111/wre.12470
Price, A. J., Balkcom, K. S., Culpepper, S. A., Kelton, J. A., Nichols, R. L., and Schomberg, H. (2011). Glyphosate-resistant Palmer amaranth: a threat to conservation tillage. J. Soil WaterConserv. 66, 265–275. doi: 10.2489/jswc.66.4.265
R Core Team (2021). R: The R Project for Statistical Computing. Vienna: R Foundation for Statistical Computing Available online at: https://www.r-project.org/ (accessed August 24, 2021).
Raoniar, R. (2021). Modelling Binary Logistic Regression Using R (Research-Oriented Modelling and Interpretation). Available online at:https://bit.ly/3BhucN3 (accessed August 11, 2021).
Ritz, C., Baty, F., Streibig, J. C., and Gerhard, D. (2015). Dose-response analysis using R. PLoS ONE 10:e0146021. doi: 10.1371/journal.pone.0146021
Rumpa, M. M., Krausz, R. F., Gibson, D. J., and Gage, K. L. (2019). Effect of PPO-inhibiting herbicides on the growth and sex ratio of a dioecious weed species Amaranthus palmeri (Palmer Amaranth). Agronomy 9:275. doi: 10.3390/agronomy9060275
Sauer, J. (1957). Recent migration and evolution of the dioecious amaranths. Evolution 11, 11–31.doi: 10.2307/2405808
Sauer, J. D. (1972). The dioecious amaranths: a new species name and major range extensions. Madroño 21, 426–434.
Sbatella, G. M., and Wilson, R. G. (2010). Isoxaflutole shifts Kochia (Kochia scoparia) populations in continuous corn. WeedTechnol. 24, 392–396. doi: 10.1614/WT-D-09-00023.1
Schliekelman, P., Ellner, S., and Gould, F. (2005). Pest control by genetic manipulation of sex ratio. J. Econ. Entomol. 98, 18–34. doi: 10.1093/jee/98.1.18
Schwartz, L. M., Norsworthy, J. K., Young, B. G., Bradley, K. W., Kruger, G. R., Davis, V. M., et al. (2016). Tall Waterhemp (Amaranthus tuberculatus) and Palmer amaranth (Amaranthus palmeri) seed production and retention at soybean maturity. Weed Technol. 30, 284–290. doi: 10.1614/WT-D-15-00130.1
Sing, T., Sander, O., Beerenwinkel, N., and Lengauer, T. (2005). ROCR: Visualizing classifier performance in R. Bioinformatics 21, 3940–3941. doi: 10.1093/bioinformatics/bti623
Sosnoskie, L. M., Webster, T. M., and Culpepper, A. S. (2013). Glyphosate resistance does not affect Palmer Amaranth (Amaranthuspalmeri) seedbank longevity. Weed Sci. 61, 283–288. doi: 10.1614/WS-D-12-00111.1
Spaunhorst, D. J., Devkota, P., Johnson, W. G., Smeda, R. J., Meyer, C. J., and Norsworthy, J. K. (2018). Phenology of five Palmer amaranth (Amaranthus palmeri) populations grown in Northern Indiana andArkansas. Weed Sci. 66, 457–469. doi: 10.1017/wsc.2018.12
Van Wychen, L. (2020). 2020 Survey of the Most Common and TroublesomeWeeds in Grass Crops, Pasture, and Turf in the United States and Canada. Available online at: https://wssa.net/wp-content/uploads/2020-Weed-Survey_grass-crops.xlsx (accessed February 16, 2021).
Wang, J. L., Klessig, D. F., and Berry, J. O. (1992). Regulation of C4 gene expression in developing amaranth leaves. Plant Cell 4, 173–184. doi: 10.1105/tpc.4.2.173
Ward, S. M., Webster, T. M., and Steckel, L. E. (2013). Palmer Amaranth (Amaranthus palmeri): a review. Weed Technol. 27, 12–27. doi: 10.1614/WT-D-12-00113.1
Webster, T. M., and Grey, T. L. (2015). Glyphosate-resistant palmer amaranth (Amaranthus palmeri) morphology, growth, and seedproduction in Georgia. Weed Sci. 63, 264–272. doi: 10.1614/WS-D-14-00051.1
Wu, C., and Owen, M. D. K. (2014). When is the best time to emerge: reproductive phenology and success of natural common aterhemp (Amaranthus rudis) cohorts in the Midwest United States? Weed Sci. 62, 107–117. doi: 10.1614/WS-D-13-00079.1
Yacouby, R., and Axman, D. (2020). “Probabilistic extension of precision, recall, and F1 score for more thorough evaluation ofclassification models,” in Proceedings of the First Workshop on Evaluation and Comparison of NLP Systems, 79–91. [Epub ahead of print].
Keywords: agriculture, evolution, flowering, weed management, pigweed
Citation: Oliveira MC, Jhala AJ, Bernards ML, Proctor CA, Stepanovic S and Werle R (2022) Palmer Amaranth (Amaranthus palmeri) Adaptation to US Midwest Agroecosystems. Front. Agron. 4:887629. doi: 10.3389/fagro.2022.887629
Received: 01 March 2022; Accepted: 04 April 2022;
Published: 25 May 2022.
Edited by:
Bhagirath Singh Chauhan, The University of Queensland, AustraliaReviewed by:
Kassio Ferreira Mendes, Federal University of Vicosa, BrazilCopyright © 2022 Oliveira, Jhala, Bernards, Proctor, Stepanovic and Werle. This is an open-access article distributed under the terms of the Creative Commons Attribution License (CC BY). The use, distribution or reproduction in other forums is permitted, provided the original author(s) and the copyright owner(s) are credited and that the original publication in this journal is cited, in accordance with accepted academic practice. No use, distribution or reproduction is permitted which does not comply with these terms.
*Correspondence: Rodrigo Werle, cndlcmxlQHdpc2MuZWR1
Disclaimer: All claims expressed in this article are solely those of the authors and do not necessarily represent those of their affiliated organizations, or those of the publisher, the editors and the reviewers. Any product that may be evaluated in this article or claim that may be made by its manufacturer is not guaranteed or endorsed by the publisher.
Research integrity at Frontiers
Learn more about the work of our research integrity team to safeguard the quality of each article we publish.