- 1CSIR—Crops Research Institute, Kumasi, Ghana
- 2Michigan State University, East Lansing, MI, United States
- 3Akenten Appiah-Menka University of Skills Training and Entrepreneurial Development, Mampong, Ghana
- 4Forschungszentrum Jülich, Institute of Bio-Geosciences, Plant Sciences, Juelich, Germany
- 5Kwame Nkrumah University of Science and Technology (KNUST), Kumasi, Ghana
- 6Yara International ASA, Nairobi, Kenya
Intensification of staple food crops such as Yam (Dioscorea spp.) while sustaining the environment is imperative in providing food for the expected 9.6 billion global population by 2050. In West Africa, amid the threat posed by climate change on food security, yam production is associated with deforestation and land degradation. Integrated soil fertility management and improved staking options for intensification and sustainable yam production on continuously cropped fields hold the key to addressing this challenge. This review evaluates the last decade's yam production trends in West Africa and in three leading yam-producing countries, Nigeria, Ghana, and Côte d'Ivoire, using the Food and Agriculture Organization Corporate Statistical Database (FAOSTAT) data. A production increase of 1.72, 1.43, and 1.35 times resulted in an area harvest increase of 2.25, 1.23, and 1.59 times in Nigeria, Ghana, and Côte d'Ivoire, respectively. Nigeria and Côte d'Ivoire had the worst yam productivity across the decade by producing at an average yield 17.3 and 12.5%, respectively, of the estimated potential yam yield (50 t ha−1). Even in Ghana, where the productivity increased across the decade, the average productivity was 33% of the estimated potential yield. Thus, it can be observed that the primary reason for the increase in yam production across the globe and in West Africa is mainly because of the increase in area under cultivation in the major yam-producing countries. The projected future erratic rainfall and elevated daily mean temperature as a result of climate change would cause declining tuber yields. Meanwhile, the importation of food such as rice as a strategy to improve food availability cannot be accessed by poor rural households due to the strong correlation between international food price hikes and prices of these foods. However, there is less relationship between international yam price hikes and yam prices in West Africa. Therefore, yam production and sustenance could be vital for food security for the increasing population of West Africa. This study reviews current research on soil fertility and staking options for sustaining yam production on continuously cropped fields. Promotion and adoption of these improved technologies would enhance food security and contribute to achieving Sustainable Development Goals 1 (No poverty), 2 (Zero hunger), 13 (Climate action), and 15 (Life on land).
Introduction and Rationale
To feed the expected 9.6 billion people by mid-century (2050), global food production must increase at least by 70% amid the current threat posed by climate change (Hertel, 2015; Ali and Mujeeb-Kazi, 2021). However, the quest to improve food production should not jeopardize the environmental quality, therefore, the need for a sustainable intensification approach. Sustainable intensification is a food production concept in which food productivity is enhanced with minimal use of additional land while the environment and biodiversity on which the production depends are conserved (Garnett et al., 2013; Petersen and Snapp, 2015). In the face of climate change, climate–smart agriculture and sustainable intensification are complementary concepts being promoted by the World Bank, Food and Agricultural Organization of the United Nations, and International Research Institutions [Consortium of International Agricultural Research Centers (CGIAR)] as a means of improving crop productivity, reducing greenhouse gases emission, and improving resilience to climate change (Campbell et al., 2014; Newell et al., 2019). A vital strategy in achieving food security would be improving and sustaining yields of regional and country staple food crops around the globe (Conceição et al., 2016). Yam (Dioscorea spp.) is a staple and vital food security crop of West Africa and needs such attention.
Yam is a multi-species tuber crop of about 600 species grown in Africa, Asia, and part of Southern America, South Pacific islands, and the Caribbean as food (Asiedu and Sartie, 2010). However, yam production is dominated in West Africa, where it serves as a vital food security and income generation crop. About 94–98% of global yams are produced along the West Africa production zone (FAOSTAT, 2021), extending from Côte d'Ivoire to Nigeria, described as the “West African yam belt”. Yam production has evolved from a food security crop to a cash crop enabling smallholder farmers to generate income. At least 60 million smallholder farmers and their households depend on it as food and a source of income. Yam tuber (Ware yam) marketing, processing, and value addition generate substantial income for rural households along the value chain. Also, sales of excess seed yam brings additional income (Mignouna et al., 2014). Besides its food and nutritional values, yam has high cultural, religious, and social values, which are very important in the traditional ceremonies and cultural rituals of societies that cultivate it (Obidiegwu and Akpabio, 2017; Nweke, 2019).
Despite these essential roles of yam, its production dwindles, with farmers achieving just about 10 t ha−1 (20%) compared to a potential yield of 50 t ha−1 across all yam varieties (Frossard et al., 2017; Neina, 2021). A major reason is that yam has a substantially high demand for soil nutrients, resulting in high nutrient export of nitrogen (N), phosphorus (P), and potassium (K) in tubers upon harvest. Therefore, there is a sharp decline in tuber yield of the second season yam crop upon continued cropping on the same piece of land without appropriate soil fertility replenishment (Frossard et al., 2017; Owusu Danquah, 2020; Neina, 2021).
To cope with this challenge, farmers use a traditional approach of moving from one cultivated field to a new field on a yearly basis, searching for fertile fields and stakes for yams to climb on. This approach is described as “shifting cultivation,” which results in land degradation and deforestation. This practice of shifting cultivation coupled with the increasing human population has led to increased pressure on arable lands and forest reserves in the yam growing communities (Ennin et al., 2014; Owusu Danquah et al., 2017). Farmers have no time for long fallow periods, causing a drastic reduction in fallow periods, if any, on the few available croplands. Even where arable lands are available, they are farther and more difficult to access. Asamoah et al. (2020) observed that the practice of shifting cultivation coupled with slash-and-burn had been a major contributing factor to deforestation in Ghana.
Although yam has received little research attention and is considered an “orphan” crop, several recent research along the West African yam belt have demonstrated improved technology options for sustainable yam production (Acheampong et al., 2017; Frimpong et al., 2020; Kiba et al., 2020). However, the knowledge gap in applying these improved technologies in sustainable yam production still exists. These improved technologies are needed, especially in the face of changing climate and increasing demand for yams as food. This study reviews a decade (2009–2019) of yam production globally, in West Africa, and specifically in the three leading yam-producing countries of Nigeria, Ghana, and Côte d'Ivoire. Implications of yam production on food security, forest lands, and sustainable production in the face of climate change are discussed. Our paper would contribute to the current discussion on intensification, ecosystem sustainability, and food security with sustainable yam production.
Search Methodology and Quality Appraisal
The review followed the approach used by Khan et al. (2003). The search method involved in the FAO database was for yam production, yield, and area under production for the past decade (2009–2019) across the globe, in West Africa, and in three leading yam-producing countries of Nigeria, Ghana, and Côte d'Ivoire. However, the search method for climate–smart yam production technologies were not strictly defined. The research questions were as follows: What is the trend of yam production in the past decade and its implication on West Africa and global food security? What are the major contributors to global yam production increase? Are there innovative climate–smart yam production technologies? How would these technologies contribute to climate change mitigation, adaptation and food security? (Figure 1). Population, Intervention, Comparison, Outcomes, and Study (PICOS) design were employed as a framework to formulate eligibility criteria in the systematic reviews. Current peer-reviewed journal articles (2010–2021) written in English on climate–smart yam technologies were searched and reviewed. The key concepts used in the search were yam productivity, climate–smart yam production, staking options, integrated soil fertility management options, and yam as a food security crop. Center for Internet Security (CIS) were used for the quality assessment of the searched articles following the criteria proposed by Dixon-Woods et al. (2006).
Results
Current Yam Production Trend and Implications on Food Security
The current data (2009–2019) from FAOSTAT shows that about 94% of global yams were produced in West Africa (FAOSTAT, 2021). The three leading yam-producing countries, Nigeria, Ghana, and Côte d'Ivoire, contributed an average of 66.12, 11.06, and 9.85%, respectively, to the total global yam production (Figure 2). Production in West Africa increased from 44 Mt in 2009 to about 70 Mt (about 1.59 times increase) in 2019, mainly due to population increase and demand for yam. During the same period, the area harvested for yam in West Africa increased from 4.22 Mha to 8.35 Mha (about 1.98 times increase) (Figures 2A,B). In the three leading yam-producing countries, Nigeria, Ghana, and Côte d'Ivoire, yam production increased from 29.09, 5.78, and 5.31 Mt in 2009 to 50.05, 8.29, and 7.18 Mt, respectively, in 2019, representing 1.72, 1.43, and 1.35 times increase in Nigeria, Ghana, and Côte d'Ivoire, respectively (Figure 2A). The area harvested for yam in these three leading producing countries also increased from 2.78, 0.38, and 0.81 Mha in 2009 to 6.24, 0.46, and 1.29 Mha in Nigeria, Ghana, and Côte d'Ivoire, respectively, representing an increase of 2.24, 1.21, and 1.59 times in Nigeria, Ghana, and Côte d'Ivoire, respectively. Thus, compared to Nigeria and Côte d'Ivoire, Ghana showed improvement in productivity trends. A production increase of 1.43 times resulted in only 1.21 times increase in area under cultivation within the decade.
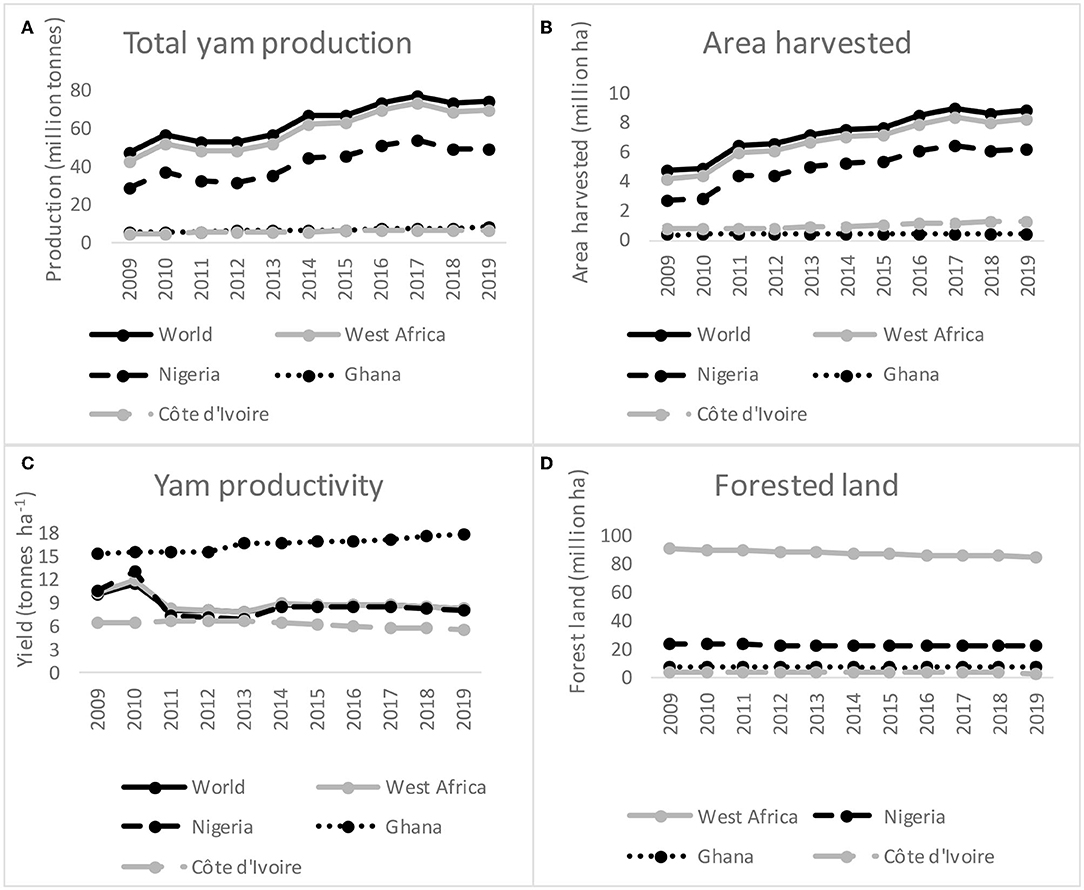
Figure 2. Productivity indicators (A) production, (B) area harvested, (C) yield of yam, and (D) forest loss across the world, in West Africa, and in three leading yam-producing countries. The graphs were drawn from FAOSTAT (2021).
Across the decade, yam production of 42.17, 7.06, and 6.28 Mt resulted in an area harvest of 5.02, 0.43, and 1.01 Mha in Nigeria, Ghana, and Côte d'Ivoire, respectively. Between 2009 and 2019, a production increase of 1.61 times resulted in 1.98 times increase in the area under yam cultivation across West Africa (Figure 2A). These analyses show that the increase in global yam production from West Africa over the past decade is because of an increase in the area under cultivation rather than a rise in productivity per unit area (yield). We show in Figure 2C that yam productivity (yield) in West Africa decreased by 18.74% from 10.30 t ha−1 in 2009 to 8.37 t ha−1 in 2019. Yam productivity (yield) in Nigeria and Côte d'Ivoire in 2009 decreased by 23.47% (10.48 t ha−1) and 14.94% (6.56 t ha−1) to 8.02 t ha−1 and 5.58 t ha−1 in 2019, respectively. Ghana had an increase of 16.97% (17.85 t ha−1) in yield compared to 15.26 t ha−1 in 2009. Thus, two (Nigeria and Côte d'Ivoire) out of the three leading yam-producing countries had a decline in yam productivity even below the already low farmer average yield of 10 t ha−1. Although the productivity in Ghana showed a continuous increase from the beginning (2009) to the end of the decade (2019), an average yield of 17.85 t ha−1 is far below the potential yield of 50 t ha−1 (Frossard et al., 2017) (Figure 2C). These three leading yam-producing countries of Nigeria, Ghana, and Côte d'Ivoire as of 2019 produced yam at 16.04, 35.70, and 11.16% of the potential yield (50 t ha−1), respectively. This observation on declining yield and gap and the corresponding increasing area being cleared for yam production have a significant implication on domestic staple food balance (production and supply), forest, and biodiversity conservation. Although yam production only cannot account for the sharp loss of forestlands in West Africa, the low productivity and increase in area harvested observed in this analysis suggests that yam and other food crop production contribute a lot to this loss. The loss is worse in Côte d'Ivoire than in Ghana, even though Côte d'Ivoire has more land area than Ghana (Figure 2D).
Koch et al. (2019), using the Brazilian Amazon as a case, showed that more forests could be conserved through intensification due to improved yields with available technologies. Several studies have shown the expansion of agricultural land for food production to be the leading cause of deforestation and mainly at the heart of the conflict between food production and biodiversity conservation (Paul and Knoke, 2015; Kubitza et al., 2018). The improved productivity in Ghana may be attributed to more than a decade West Africa Agriculture Productivity Programme's (WAAPP) root and tuber research and development (R&D) effort spearheaded by the CSIR—Crops Research Institute in Ghana as a regional center of excellence in roots and tubers research. Under this program, improved yam technologies were demonstrated through a farmer participatory approach in Ghana's major yam growing areas to encourage adoption (Acheampong et al., 2017).
Thus, improved yam production technologies would enable improved and sustained yam production even under limited land resources to improve food security.
Yam is considered a staple food in West Africa, supplying daily energy and nutrition to most citizens in the order of Ivoirians > Ghanaians > Nigerians (Figure 3A). However, the domestic supply followed the order of Nigeria > Ghana = Côte d'Ivoire (Figure 3B). This suggests that Côte d'Ivoire and Ghana seem to depend more on yam as a staple food than Nigeria. However, the two countries lead in yam exports, while exports from Nigeria are negligible. This may be because of the very high population in Nigeria and, therefore, past government policies to ensure food security, which restricted some food items, including yam, from export until about a decade ago when it was lifted (Akande and Ogundele, 2009). Thus, more food is required in Nigeria than in Ghana, and Côte d'Ivoire accounts for this observation. None of the three leading yam-producing countries imported yam. However, Ghana and Côte d'Ivoire employed yam export to generate income (Figures 4A,B). This has huge implications on food balance, food security, and livelihood of the countries and that of the West African subregion.
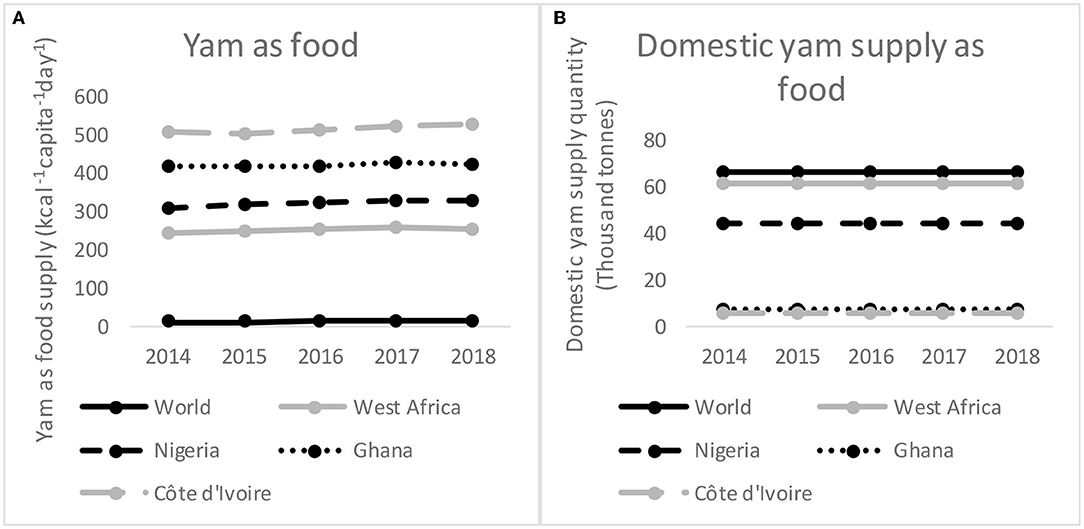
Figure 3. Yam as food (A) and supply (B) in three leading yam-producing countries (Nigeria, Ghana, and Côte d'Ivoire) compared with West Africa and the world. The graphs were drawn with data from FAOSTAT (2021).
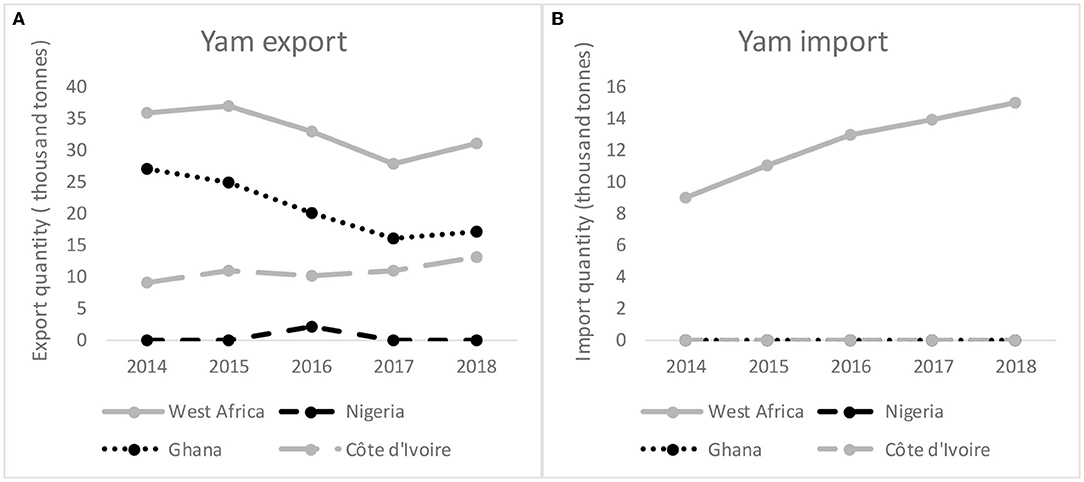
Figure 4. Yam trade (A) export and (B) import in three leading yam-producing countries (Nigeria, Ghana, and Côte d'Ivoire) of West Africa. The graphs were drawn with data from FAOSTAT (2021).
Although a global effort is ongoing toward improving food security, about 218 M (over one-fourth) of the world's malnourished and food insecure population lives in sub-Saharan Africa [UNDP (United Nations Development Programme), 2012] and needs food to be secured. Food security consists of availability, accessibility, utilization, and stability of food. While food availability is essential, food accessibility supersedes it. Vermeulen et al. (2014) observed that climate change would be the greatest threat to food accessibility by urban and rural populations. This is because climate change increases the chance of losing smallholder farmers' yield and income, affecting their capacity to purchase alternative food. Food trade (export and import) does not necessarily jeopardize food security in developing countries; instead, it can improve food security. Food export can enhance smallholder farmers, especially women's income and access to other food sources. While food import may serve as a major shock absorber in a major drought to improve the availability of alternative foods during stress, however, the ability to pay for this alternative food is very crucial (Van den Broeck and Maertens, 2016; Haggblade et al., 2017; Bini, 2018).
Roots and tubers (Cassava and Yam) and cereals (Maize, Millet, and Sorghum), which are the major staple food crops of West Africa, are subjected to production shocks by flooding, drought, pests, and diseases, which reduce their production (Haggblade et al., 2017). Lipton (2012) has shown that 75% of cereals in addition to the roots and tubers produced in Africa provides two-thirds of the energy intake of the populace while the energy deficit of one-third is taken care of through food imports. Thus, making importation of alternative food sources important, especially in an emergency. However, price hikes, especially in rice import to West Africa, would limit poor smallholder farmers' access to these imported foods (Allen, 2017; Bini, 2018). Haggblade et al. (2017), in a climate impact, food security, and vulnerability simulation study, observed that without trade, a reduction of 20% in cereal production would result in a 15% reduction in calorie supply to the rural poor. Also, a 50% increase in the price of rice as an alternative food source would force the poor to reduce calorie consumption by 8%.
Diverse roots and tubers food crops are produced locally in West Africa, whose prices are not highly related to global food hikes (Hatzenbuehler et al., 2017; Taylor et al., 2018; Petsakos et al., 2019). This can be taken as an advantage to improve food availability and accessibility by all, including poor smallholder farmers. Hatzenbuehler et al. (2017) observed that changes in the global price of yam do not virtually influence local prices much in Nigeria and West Africa region as much as in cereals. Thus, cereals are more sensitive to international price hikes than roots and tubers (Haggblade et al., 2017; Bini, 2018). Also, cereals such as maize were more sensitive to climate change and yield decline than roots and tubers such as yam (Aiikulola and Sinnarong, 2018). Therefore, increasing and sustaining the production of roots and tubers such as yam can be taken as an advantage, with improved technologies, to serve as an alternative food source to cereals, especially imported rice, whose prices highly depend on the global market.
Climate Change Impact on Yam Production and Food Security
The observed impact of climate change on global agriculture and food production calls for prompt action to mitigate and adapt to climate change, as indicated by the Sustainable Development Goal (SDG) 13. Agriculture and food production, especially in West Africa, is vulnerable to climate change. Aside from this region being identified to be a hotspot with high susceptibility to climate change impact, crop production is mainly rainfed (IPCC, 2014; Gachene et al., 2015; Kyei-Mensah et al., 2019). Yam production is no exception, and to sustain and increase production, temperature patterns and rainfall distribution should fall in line with the physiological development of yam. Climate parameters have changed while projections on future climate scenarios in West Africa have indicated unstable climatic conditions with detrimental implications on yam yields (Srivastava et al., 2016; Sultan and Gaetani, 2016; Okongor et al., 2021). Several climate forecast studies have projected about 1.5 to 6.5°C increase in future temperature and significant variability (−30 to 30% uncertainty) in rainfall patterns in the near to distant futures of the twenty-first century (Sylla et al., 2016; Adifon et al., 2020; Sagna et al., 2021).
As a result of climate change, the optimum temperature for yam growth and development observed to be between 25 and 30°C can only persist until the middle of the century (Srivastava et al., 2016). Beyond 2050, due to increasing daily mean temperatures above the required temperature, yam growth and productivity would decline. Due to yams' long duration (8–12 months depending on variety) on the field, variable rainfall and increasing daily mean temperature would cause increased evapotranspiration and water stress on the plant. Due to increasing Green House Gases (GHGs) emissions, CO2 concentration elevation in the atmosphere is projected, which is suggested to favor yam photosynthetic rate and growth (Thinh et al., 2017). However, the associated elevated daily temperatures and extreme conditions such as drought may deny the yam this benefit. Yam is a C3 plant; therefore, as the sun intensity and daily temperature increase, the oxygenation reaction along the photorespiratory pathway increases. This results in a loss of about 25–30% in carbon fixation, as observed in most C3 plants (Sage and Kubien, 2007; Raines, 2011). Models have therefore predicted yam productivity decline to be between 18 and 48% across the savannah zones by the middle of the century (Srivastava et al., 2012; Angba et al., 2020). The practice of shifting cultivation would further exacerbate climate change's impact on yam production.
Although farmers have varying knowledge of climate change, they are aware of changes in rainfall and temperature and of how they affect crop production (Oluwatayo and Ojo, 2016). Oruonye and Adebayo (2015) evaluated farmers' awareness of climate change and showed that 88% of the farmers were aware of climate change, although few (48%) knew the causes of climate change. Also, 90% of the farmers claimed the effect of drought, flooding, and extremely high temperatures, resulting from climate change on their production and proposed adaptation measures like that of experts.
Aiikulola and Sinnarong (2018) observed yam to be more resilient to climate change than maize and could be sustained in worse times as a food security crop. Therefore, through a farmer participatory approach, climate–smart technologies can be employed for climate-resilient yam production.
Improved Yam Technologies for Sustainable Production
Increases in yam production globally and in West Africa result from an increase in area under yam cultivation in the leading producing countries. Climate change and the use of traditional shifting cultivation, which has caused limitations in cropland, would further worsen the decline in yam productivity. Therefore, there is a need for sustainable intensification and climate-resilient technologies that would sustain and improve yam production on continuously cropped fields. Discussed in the following sections are several options of improved yam technologies that can be beneficial for ensuring food security.
Climate–Smart Soil Fertility Management Options
The most challenging vision the world has set itself is the SDGs. The SDG 2 envisages no hunger by 2030 and, therefore, the need for increased food availability. As indicated by the UN, food sufficiency or availability is not enough, but the food and nutritional component should be accessible and safe to promote a healthy life. In our quest to produce sufficient food to increase availability and accessibility to all, care must be taken not to jeopardize the quality of the environment and the ecosystem. This is in line with SDG 15 (Life on land), which envisages sustainable management of ecosystems to address forest loss, land degradation, and biodiversity loss. Yet, food production in many regions of Africa uses shifting cultivation, which is observed to be a major contributing factor to forest loss and land degradation. Brandt et al. (2017) observed that the increasing demand for agricultural lands resulting from the increasing human population had caused a loss of about 90% of the original rainforest cover in West Africa, with the remaining being very fragmented and degraded. Yams in West Africa are mainly produced in the forest and derived/forest–savannah transition zones. Coupled with the yearly movement in search of a fertile field, yam production is documented to be associated with forest loss and land degradation (Frossard et al., 2017; Asamoah et al., 2020).
Using a modeling approach to forecast recovery rate after a major disturbance such as slash-and-burn, N'Guessan et al. (2019) indicated a very low biomass recovery rate. Also, depending on the type of crop cultivated before a fallow, it would take an average fallow period of 17, 30, and 51 years to recover 10, 25, and 50% growth forest biomass, respectively. When yam is cultivated before the fallow, a fallow period of over 38 years would be needed for a 50% forest growth biomass recovery. Thus, after yam cultivation on a field, it would take more than 76 years (about a century) to fully recover for the next cultivation. Because of the high demand for agricultural land, fallow periods are less than 3 years when even 5% of the ecosystem is not recovered (Wezel and Haigis, 2002). It should also be noted that the forest ecosystem plays a major role in the global ecosystem as a carbon sink in regulating carbon into the atmosphere, which has implications for global warming and climate change (Brienen et al., 2015; Houdanon et al., 2018). Also, the forest serves as a shelter for Africa's unique species and biodiversity. Therefore, there is a need for sustainable yam production technologies to ensure forest conservation and biodiversity (Melo et al., 2013). These technologies should ensure improved and sustained yam production on continuously cropped fields to reduce pressure on the limited land resources. Discussed in the following sections are proven soil fertility management technologies that can be adapted for this purpose.
Slash-and-Mulch System
This technology is presented as an alternative to the traditional slash-and-burn approach associated with shifting cultivation. In the slash-and-burn, a natural secondary forest area (3–4 years of fallowed land) is cleared, and the biomass is left to dry over time and deliberately burnt off. This approach is used because of the thick vegetation from the newly cleared secondary forest, with shrubs and trees. Farmers have short time intervals from land preparation to planting, making it difficult to wait for the natural decomposition. Farmers, therefore, consider burning the biomass as the fastest and most cost-efficient means of doing away with the biomass for clear fields for planting. However, with the increasing pressure on agricultural lands and reduced fallow, this practice is not sustainable. Therefore, the need for the slash-and-much approach, where the natural secondary forest vegetation biomass upon clearing, is left on the field without burning for decomposition (Norgrove and Hauser, 2015; N'dri et al., 2019). The biomass left on the field improves soil organic matter and nutrition upon decomposition. This is vital for the forest and forest–savannah transition zones soils of West Africa, where yams are mainly produced. These soils are primarily infertile due to a high proportion of low activity clays and sand content (Bationo and Buerkert, 2001). Also, this approach will greatly benefit balancing nutrient export from the field and replenishing soil nutrients for a high nutrient-demanding crop such as yam.
N'dri et al. (2019) compared slash-and-much field and slash-and-burn fields used for yam production in Côte d'Ivoire and observed that about 95, 95, and 74% of carbon (C), nitrogen (N), and potassium (K), respectively, in the aboveground biomass in the field were lost to the atmosphere on comparison with the ashes. Also, soil analysis revealed higher C, total N, K, and magnesium (Mg) in the slash-and-mulch field than in the slash-and-burn field. Yam tuber yield was double on the slash-and-much field (18 Mg ha−1) compared to the slash-and-burn fields (9 Mg ha−1). The study, therefore, indicated a negative relationship between aboveground biomass burning and yam productivity and encouraged the promotion of slash-and-mulch as an alternative to slash-and-burn for sustainable yam production.
Norgrove and Hauser (2015) compared slash-and-burn fields and forests around slash-and-burn fields (Forest margins benchmark areas). This was to assess the impact of burning on soil fertility, labor input, and crop productivity in Southern Cameroon. The study showed that exclusion of burning in field preparation results in higher crop productivity and ecosystem carbon stock than when the field biomass was deliberately burnt. Also, fallow recovery was potentially faster on the unburnt fields than on the slash-and-burnt fields. Thus, although the initial labor cost in field preparation may be high, the aftermath returns on crop productivity and soil fertility sustenance make burning exclusion in field preparation more profitable in the long term.
The slash-and-mulch approach enables more carbon storage in the ecosystem, which improves nutrient cycling to the benefit of the crops. Also, fallow periods can be reduced drastically to fit in the current short duration resulting from pressure on cropland. Thus, sustainable yam production on limited land resources while conserving forest and biodiversity as carbon sink makes it climate–smart.
Integrated Soil Fertility Management (ISFM) With Legumes
Legumes are integrated into cropping systems to take advantage of biological nitrogen fixation (BNF) for soil fertility improvement. Nitrogen is one of the most limiting nutrients in crop production, of which yam is no exception (Bationo and Buerkert, 2001). However, over 60% of global nitrogen fixation is due to BNF, hence the need to take advantage of for a high nutrient-demanding crop such as yam.
Pigeonpea (Cajanus Cajan), a legume shrub, is observed to play a significant role in nitrogen fixation for sustainable yam production upon integration into yam cropping systems (Owusu Danquah et al., 2017; Acheampong et al., 2019; Owusu Danquah, 2020) due to its deep taproot system, it is drought tolerant and can adapt to a wide range of environments. Pigeonpea grains are rich in proteins and vitamins, which can serve as an additional source of nutrition for smallholder farmers (Ouyabe et al., 2019). It fixes more N per unit area in its biomass than other major crop legumes used in cropping systems (Adjei-Nsiah, 2012; Kwena et al., 2019; Mhango et al., 2020). Although it is mainly cultivated in East Africa, recent evaluation on integration into yam cropping systems has shown impressive prospects for West Africa.
Owusu Danquah et al. (2017) evaluated pigeonpea and yam rotation systems with other soil amendments on continuously cropped fields in Ghana. A significant improvement in yam productivity was observed when pigeonpea was introduced as a preceding crop with other soil amendments in yam production. Tuber yield of yam on pigeonpea preceded fields with 3 t ha−1 poultry manure and 15-15-20 kg ha−1 N-P205-K20 were similar to yam tuber yields when the poultry manure and inorganic fertilizer were doubled to 6 t ha−1 and 30-30-40 kg ha−1 N-P205-K20 without pigeonpea as preceding crop. Suggesting the introduction of pigeonpea into the yam cropping system reduces recommended inorganic fertilizer usage on continuously cropped fields from 45-45-60 kg ha−1 N-P205-K20 further down. This allows smallholder farmers who have less income and cannot afford expensive inorganic fertilizers an option for sustainable yam production. Inorganic fertilizer reduction is also important for soil biodiversity, soil health, and fertility sustenance.
Owusu Danquah (2020) again evaluated pigeonpea–yam cropping system options with yam planted in alleys of pigeonpea (Figure 5A) or about two-thirds and a third of the field used for yam and pigeonpea (2:1—yam: pigeonpea as a border) cultivation, respectively, (Figure 5B1) compared to sole yam (Figure 5C) on continuously cropped fields in Ghana. This was to evaluate the dual role of pigeonpea for soil fertility maintenance and as a source of stakes for the yam vines to climb on. Based on the results and field experience, another option of about two-thirds and a third of the field used for yam and pigeonpea at one side (2:1—yam: pigeonpea at one side) was also proposed (Figure 5B2). The results showed that, on continuously cropped fields, even when a farmer has no money for inorganic fertilizer and does not apply inorganic fertilizer in yam production, the tuber yields, and economic returns were significantly better under any of the pigeonpea–yam cropping systems than sole yam cultivation with half the recommended inorganic fertilizer rate (23-23-30 kg ha−1 N-P205-K20). This observation was due to the pigeonpea biomass, which contributed N and other nutrients to boost nutrition for the yam. Also, the biomass shaded and conserved moisture on the ridges and suppressed weeds to encourage tuber bulking. Thus, the presence of the pigeonpea facilitated resource use in the pigeonpea–yam cropping system, resulting in relatively higher resource use efficiency. This affords the resource-poor smallholder farmers the opportunity for sustainable yam production, improved income, and livelihood on limited land resources for long term. This is also important for environmental sustenance, reducing pressure on forests and biodiversity in West Africa, resulting from yam production.
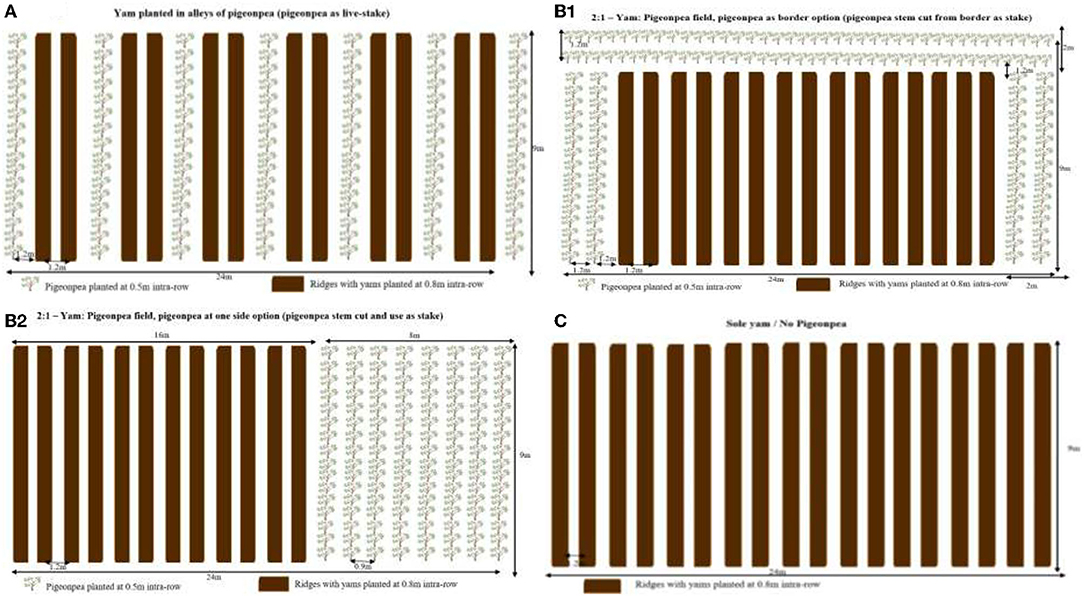
Figure 5. A pictorial illustration of the pigeonpea–yam cropping system options compared with sole yam. (A) Yam planted in alleys of pigeonpea with pigeonpea as live-stake; (B1) 2:1—yam: pigeonpea with pigeonpea as border option, pigeonpea stems cut from the border as stake; (B2) 2:1—yam: pigeonpea with pigeonpea at one side option, pigeonpea stem cut and used as stake; (C) Sole yam, bamboo stakes purchased and sent to the field for staking (Source: Owusu Danquah, 2020).
Ridging as an Alternative to Mounding
This technology is presented as an alternative to manual mounding, which is labor-intensive and drudgery. Thus, yam is described as “a man's crop” discouraging women from venturing into yam farming (Ennin et al., 2014; Owusu Danquah et al., 2018b). Yam requires a low soil bulk density medium for bulking; without it, productivity would be impaired. The traditional approach has been the use of mounds to serve as a low-density medium for yam bulking. However, the use of mounding results in less population density per area, resulting in less resource use efficiency and less tuber yield. Planting on ridges at 1–1.2 m and 0.8–1 m inter- and intra-ridges, respectively, results in a population of about 10,000–10,416 plant ha−1. While planting on mounds results in a sparse population of about 2 m between mounds, resulting in a population of 4,000–6,000 plants ha−1. Field operations such are weeding, mulching, inorganic fertilizer application, and staking are easier with ridges than on mounds (Osei et al., 2015; Owusu Danquah et al., 2018a; Frimpong et al., 2020). Although harvested yam tuber sizes are influenced by seed sett size used for planting, planting density, and seedbed, the use of ridging results in a greater number of small to medium (1–3.5 kg tuber−1) size tubers preferred by the yam export market (Owusu Danquah et al., 2018b).
Ennin et al. (2014) used a farmer participatory approach to evaluate seedbed and inorganic fertilizer use for sustainable yam production on continuously cropped fields in Ghana. The results showed that planting on ridges resulted in a higher planting density of more than 7,000 plants ha−1 than on mounds on the farmers' practice field of about 4,500 plants ha−1. This resulted in a significant nutrient use efficiency, tuber yield, and profitability with ridging and inorganic fertilizer application than mounding with inorganic fertilizer application. Frimpong et al. (2020) also compared improved yam technology package (Seed treatment, inorganic fertilizer application, and trellis staking) with farmers' practice of no seed treatment, no fertilizer application, and optimum staking on continuously cropped fields in the major yam growing areas of Ghana. The study revealed that yam productivity increased by more than 60% across the locations using the improved yam technologies over the farmers' practice.
This indicates that ridging and integrated soil nutrient management approach can be employed on continuously cropped fields to sustain yam production and avoid moving to new fields to address deforestation associated with yam production. Thus, sustainable intensification of yam production on continuously cropped fields is possible with improved technologies. This would reduce expansion to forestlands in our pursuit to increase yam production to address the deforestation and land degradation menace associated with yam production in West Africa.
Climate–Smart Staking Options
Staking in yam production is a cultural practice where yam vines are twined on erected bamboo, cut branches of a tree, ropes, deliberately planted trees, and an already growing tree, for yam vines to climb on. It exposes the yam leaves to the sun for efficient sunlight capture and lifts the leaves from the ground reducing soil-borne diseases. However, because of indiscriminate land clearing, stakes are scarce and costly, increasing the cost of yam production. Depending on the yam variety, staking or not staking influences yam yield. While Dioscorea alata (water yam) gives reasonable yields without staking, the yields of Dioscorea rotundata (white/guinea yam) significantly declined when not staked (Tsado, 2012; Owusu Danquah et al., 2015). Therefore, in the quest to improve and sustain their yam production at a low cost, farmers cut down the nearby trees to use their branches and twigs, resulting in deforestation and environmental degradation.
Tsado (2012) evaluated the possibility of using Polyvinyl Chloride (PVC)-plastic as a substitute for wooden stakes in yam production in Nigeria. The results revealed significant high leafy biomass and tuber yields of yam staked with PVC-plastic than wooden stakes with worse yield under no staking. The study recommended the use of PVC-plastic, but the cost implication of acquiring the PVC-plastics is likely to be a major disincentive for adoption by farmers.
Owusu Danquah et al. (2015) evaluated the influence of No staking, Vertical/ farmers' practice staking, and Trellis staking (30–50% number of stakes used in farmers' practice staking) on the productivity of D. alata and D. rotundata in Ghana. The study indicated that D. alata could be planted without staking with no significant effect on yields. However, the yields of D. rotundata declined by 37–65% when not staked due to a higher incidence of yam mosaic virus and therefore would require staking. Also, trellis staking resulted in a similar yield and better profit to farmers' staking practice for all the varieties and suggested that it can be used for sustainable yam production in the face of climate change.
Owusu Danquah (2020) also evaluated the option of live-staking or growing trees as stakes as part of yam production using the legume shrub—pigeonpea. Growing yams in alleys of pigeonpea with the pigeonpea as live-stakes and growing yams on two-thirds and a third of the field used for growing pigeonpea and mature stems cut and used as stakes were evaluated with farmers' practice as control (Figures 6A–C). The results showed that the presence of the pigeonpea provided readily available stakes from pigeonpea either as live-stake or cut stakes. Also, the biomass of the pigeonpea conserved moisture and added nutrients to the soil to the benefit of the yam. The tuber yields were 17.26, 15.30, and 13.36 t ha−1 for yam in alleys of pigeonpea, cut pigeonpea stem as stake field, and sole yam with bamboo stakes, respectively. Thus, mainly due to the introduction of pigeonpea, which significantly reduced the cost implications of staking, yam production was more resource-use efficient and profitable with the pigeonpea–yam options than the control of sole yam with farmers' staking practice.
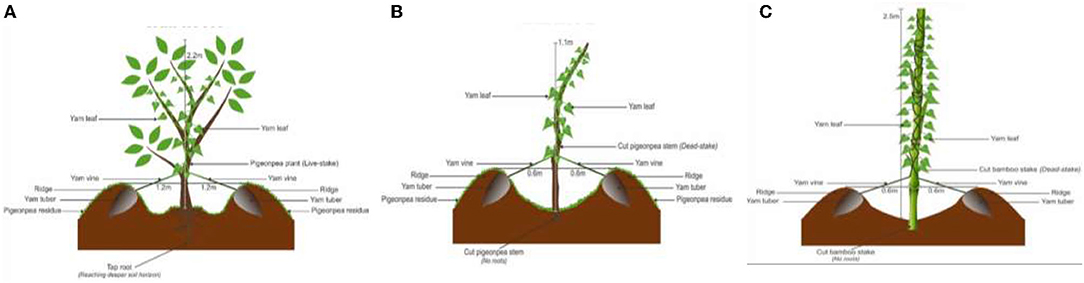
Figure 6. A pictorial illustration of the staking options with pigeonpea in the pigeonpea–yam cropping system options compared with current farmers' practice. (A) pigeonpea live-staking option for yam in an alley of pigeonpea; (B) Cut pigeonpea stem staking option for two-third field and one-third field for yam and pigeonpea cultivation, respectively; (C) Farmer practice of using bamboo staking option; bamboo stakes purchased and transported to yam fields (Source: Owusu Danquah, 2020).
An environmentally sustainable staking option for yam intensification is possible. These options afford farmers with less drudgery option of stakes for yam production and have the potential of improving their yields, income, and livelihoods.
Conclusions and Recommendations
Yam production has increased for the past decade across the globe, West Africa, and in the leading yam-producing countries due to an increase in the area cleared for yam cultivation and not an increase in yield per unit area (productivity). While Ghana has been improving in productivity (yields) for the past decades, probably because of the research and development (R&D) in yam, productivity in Nigeria and Côte d'Ivoire are declining. As a result of shifting cultivation for food production, including yam production, forest and biodiversity in West Africa keep dwindling.
In the face of climate change, declined yam production is forecasted, endangering food and nutrition security in West Africa. Food importation seems a good option; however, international price hikes would make imported foods expensive for the rural and urban poor to access (buy). Improving and sustaining stable roots and tubers food crops such as yam, whose prices are less dependent on international price hikes, become an advantage over importation.
Climate–smart integrated soil fertility management approach of modifying the traditional slash-and-burn to slash-and-mulch and integration (rotating or intercropping) of legume shrubs, such as pigeonpea, have been proposed. Pigeonpea would not only improve nitrogen and other nutrients in the cropping system with its biomass but it would also help conserve moisture and the bulking medium (mounds or ridges) to the advantage of the yam. Also, upon right arrangement or design, the pigeonpea can serve as a live-stake or readily available source of stakes for staking yam.
The review has shown that addressing the challenge of sustaining yam production to feed the increasing population while conserving the forest and biodiversity in West Africa in the face of climate change is possible through deliberate farmer participatory R&D and government policies. Farmer participatory R&D in yam production would be vital along the West Africa yam belt to improve productivity and food security. Demonstrating improved yam technologies as in the Ghana example could be beneficial to other yam-producing countries. Breeding for yam varieties that can produce appreciable yield under no staking would help address staking issues. Also, yam varieties tolerant to increased daily temperatures and drought would be crucial as the climate keeps changing. Government policies and subsidies should support farmers who adopt and practice environmentally sound yam production to serve as motivation. This would make continuous crop fields sustainable for yam production, preventing movement from field to field every year to address the deforestation and land degradation menace associated with yam production.
Data Availability Statement
The original contributions presented in the study are included in the article/supplementary material, further inquiries can be directed to the corresponding author/s.
Author Contributions
EO, FD, FF, KD, CW, SE, MAs, MB, HD, AA-D, DN, MAk, and AO conceptualized and designed the study. EO, FD, FF, KD, MAs, MB, HD, AA-D, DN, MAk, and AO conducted database and literature search. CW and SE delivered editorial responsibility. All authors proofread and approved the final version of manuscript.
Conflict of Interest
DN was employed by Yara International ASA.
The remaining authors declare that the research was conducted in the absence of any commercial or financial relationships that could be construed as a potential conflict of interest.
Publisher's Note
All claims expressed in this article are solely those of the authors and do not necessarily represent those of their affiliated organizations, or those of the publisher, the editors and the reviewers. Any product that may be evaluated in this article, or claim that may be made by its manufacturer, is not guaranteed or endorsed by the publisher.
Acknowledgments
Authors are grateful to the technical staff and students at CSIR—Crops Research Institute and CSIR—College of Science and Technology (CCST) of Ghana, respectively, for assisting in literature desk studies and FAOSTAT database analysis. The sponsorship provided for the doctoral studies of the lead author by the Borlaug Higher Education for Agricultural Research and Development (BHEARD) and the Alliance for African Partnership (AAP) all at the Michigan State University are duly acknowledged.
References
Acheampong, P. P., Nimo-Wiredu, A., Amengor, N. E., Nisah-Frimpong, B., Haleegoah, J., Adu-Appiah, A., et al. (2017). Root and Tuber Crops Technologies Adoption and Impact Study in Ghana: A Case of Improved Yam Technologies. Technical Report on West Africa Agricultural Productivity Programme (WAAPP), CSIR—Crops Research Institute, Ghana.
Acheampong, P. P., Owusu Danquah, E., Dissanayake, H. G., Hayford, P., and Weebadde, C. (2019). A socioeconomic study of transition zone yam farmers: Addressing constraints and exploring opportunities for integrated pigeonpea into yam cropping systems. Sustainability. 11, 717. doi: 10.3390/su11030717
Adifon, F. H., Atindogb,é, G., Bello, D. O., Balogoun, I., Yabi, I., Dossou, J., et al. (2020). Effect of Climate Variability on Yams (Dioscorea spp.) Production in Central and Northern Benin. Am. J. Clim. Change, 9,423–440. doi: 10.4236/ajcc.2020.94027
Adjei-Nsiah, S. (2012). Role of pigeonpea cultivation on soil fertility and farming system sustainability in Ghana. Int. J. Agron. 702506. doi: 10.1155/2012/702506
Aiikulola, O. I., and Sinnarong, N. (2018). Potential Effects of Climate Change on Stapple Crops in West Africa. Maejo University, Thailand.
Akande, S. O., and Ogundele, O. O. (2009). “Yam |production in Nigeria: a policy analysis matrix.” in Securing Livelihoods through Yams. IFAD TAG 704, IITA, Nigeria, eds. Nkamleu, B., Annang, D., N.M. Bacco, 10–25.
Ali, N., and Mujeeb-Kazi, A. (2021). “Food production: global challenges to mitigate climate change”. in Physiological, Molecular, and Genetic Perspectives of Wheat Improvement, eds. Wani, S. H., Mohan, A., Singh, G. P (Springer, Cham), 1–13
Angba, C. W., Baines, R. N., and Butler, A. J. (2020). Examining yam production in response to climate change in Nigeria: a co-integration model approach. Soc. Sci. 9, 42. doi: 10.3390/socsci9040042
Asamoah, O., Kuittinen, S., Abrefa Danquah, J., Quartey, E. T., Bamwesigye, D., Mario Boateng, C., et al. (2020). Assessing wood waste by timber industry as a contributing factor to deforestation in Ghana. Forests. 11, 939. doi: 10.3390/f11090939
Asiedu, R., and Sartie, A. (2010). Crops that feed the world 1. Yams. Food Secur. 2, 305–315. doi: 10.1007/s12571-010-0085-0
Bationo, A., and Buerkert, A. (2001). “Soil organic carbon management for sustainable land use in sudano-sahelian West Africa.” in Managing organic matter in tropical soils: Scope and limitations. Dev. Plant Soil Sci. vol. 93 (Dordrecht: Springer), 249–262. doi: 10.1007/978-94-017-2172-1_13
Bini, V. (2018). Food security and food sovereignty in West Africa. Afr. Geogr. Rev. 37, 1–13. doi: 10.1080/19376812.2016.1140586
Brandt, M., Rasmussen, K., Peñuelas, J., Tian, F., Schurgers, G., Verger, A., et al. (2017). Human population growth offsets climate-driven increase in woody vegetation in sub-Saharan Africa. Nat. Ecol. Evol 1,1–6. doi: 10.1038/s41559-017-0081
Brienen, R. J., Phillips, O. L., Feldpausch, T. R., Gloor, E., Baker, T. R., Lloyd, J., et al. (2015). Long-term decline of the Amazon carbon sink. Nature. 519, 344–348. doi: 10.1038/nature14283
Campbell, B. M., Thornton, P., Zougmor,é, R., Van Asten, P., and Lipper, L. (2014). Sustainable intensification: What is its role in climate smart agriculture? Curr. Opin. Environ. Sust. 8, 39–43. doi: 10.1016/j.cosust.2014.07.002
Conceição, P., Levine, S., Lipton, M., and Warren-Rodríguez, A. (2016). Toward a food secure future: ensuring food security for sustainable human development in Sub-Saharan Africa. Food Policy. 60, 1–9. doi: 10.1016/j.foodpol.2016.02.003
Dixon-Woods, M., Bonas, S., Booth, A., Jones, D. R., Miller, T., Sutton, A. J., et al. (2006). How can systematic reviews incorporate qualitative research? Crit. Perspec. Qual Res. 6, pp.27–44. doi: 10.1177/1468794106058867
Ennin, S. A., Issaka, R. N., Acheampong, P. P., Numafo, M., and Owusu Danquah, E. (2014). Mechanization, Fertilization and staking options for environmentally sound yam production. Afr. J. Agric. Res. 9, 2222–2230. doi: 10.5897/AJAR2014.8487
FAOSTAT. (2021). Food and Agriculture Organization of the United Nations. FAOSTAT Statistical Database. Rome. Available at: https://fao.org/faostat/en/#compare (assessed October 15, 2021).
Frimpong, F., Owusu Danquah, E., Aidoo, A. K., Ennin, S. A., Asumadu, H., and Maroya, N. (2020). Demonstrating the efficacy of existing yam technologies in the forest-savannah transition zone of Ghana. J. Agric. Sci. Pract. 5, 107–118.
Frossard, E., Aighewi, B. A., Ak,é, S., Barjolle, D., Baumann, P., Bernet, T., et al. (2017). The challenge of improving soil fertility in yam cropping systems of West Africa. Front. Plant Sci. 8, 1953. doi: 10.3389/fpls.2017.01953
Gachene, C. K. K., Karuma, A. N., and Baaru, M. W. (2015). “Climate change and crop yield in Sub-Saharan Africa.” in Sustainable Intensification to Advance Food Security and Enhance Climate Resilience in Africa, eds. Lal, R., Singh, B., Mwaseba, D., Kraybill, D., Hansen, D., Eik, L. (Cham: Springer), 165–183. doi: 10.1007/978-3-319-09360-4_8
Garnett, T., Appleby, M. C., Balmford, A., Bateman, I. J., Benton, T. G., Bloomer, P., et al. (2013). Sustainable intensification in agriculture: premises and policies. Science. 341, 33–34. doi: 10.1126/science.1234485
Haggblade, S., Me-Nsope, N. M., and Staatz, J. M. (2017). Food security implications of staple food substitution in Sahelian West Africa. Food Policy. 71, 27–38. doi: 10.1016/j.foodpol.2017.06.003
Hatzenbuehler, P. L., Abbott, P. C., and Abdoulaye, T. (2017). Price transmission in Nigerian food security crop markets. J. Agric. Econ. 68, 143–163. doi: 10.1111/1477-9552.12169
Hertel, T. W. (2015). The challenges of sustainably feeding a growing planet. Food Secur. 7, 185–198. doi: 10.1007/s12571-015-0440-2
Houdanon, R. D., Mensah, S., Gnangl,è, C., Yorou, N. S., and Houinato, M. (2018). Ecosystem services and biomass stock from bamboo stands in central and southern Benin, West Africa. Energ. Ecol. Environ. 3, 185–194. doi: 10.1007/s40974-018-0084-0
IPCC. (2014). Climate Change 2013: The Physical Science, B.asis., Contribution of Working Group I to the Fifth Assessment Report of the Intergovernmental Panel on Climate Change [T. F., Stocker, D., Qin, G.-K., Plattner, M., Tignor, S. K., Allen, J., Boschung, A., Nauels, Y., Xia, V., and Bex, P. M., Midgley (Eds.)]. Cambridge University Press, Cambridge, United Kingdom.
Khan, K. S., Kunz, R., Kleijnen, J., and Antes, G. (2003). Five steps to conducting a systematic review. J R Soc Med. 96,118–121. doi: 10.1258/jrsm.96.3.118
Kiba, D. I., Hgaza, V. K., Aighewi, B., Ak,é, S., Barjolle, D., Bernet, T., et al. (2020). A transdisciplinary approach for the development of sustainable yam (Dioscorea sp.) production in West Africa. Sustainability. 12, 4016. doi: 10.3390/su12104016
Koch, N., zu Ermgassen, E. K., Wehkamp, J., Oliveira Filho, F. J., and Schwerhoff, G. (2019). Agricultural productivity and forest conservation: evidence from the Brazilian Amazon. Am. J. Agric. Econ. 101, 919–940. doi: 10.1093/ajae/aay110
Kubitza, C., Krishna, V. V., Urban, K., Alamsyah, Z., and Qaim, M. (2018). Land property rights, agricultural intensification, and deforestation in Indonesia. Ecol. Econ. 147, 312–321. doi: 10.1016/j.ecolecon.2018.01.021
Kwena, K., Karuku, G. N., Ayuke, F. O., and Esilaba, A. O. (2019). Nitrogen deficiency in semi-arid kenya: can pigeonpea fix it? East Afr. Agric. For. J. 83, 322–340. doi: 10.1080/00128325.2019.1658696
Kyei-Mensah, C., Kyerematen, R., and Adu-Acheampong, S. (2019). Impact of rainfall variability on crop production within the Worobong Ecological Area of Fanteakwa District, Ghana. Adv. Agric. 2019. doi: 10.1155/2019/7930127
Lipton, M. (2012). Learning from others: Increasing agricultural productivity for human development in Sub-Saharan Africa. United Nations Development Programme Regional Bureau for Africa Working Paper, 7. UNDP Africa Policy Notes, 2012–007.
Melo, F. P., Arroyo-Rodríguez, V., Fahrig, L., Martínez-Ramos, M., and Tabarelli, M. (2013). On the hope for biodiversity-friendly tropical landscapes. Trends Ecol. Evol. 28, 462–468. doi: 10.1016/j.tree.2013.01.001
Mhango, W. G., Snapp, S., and Kanyama-Phiri, G. Y. (2020). “Biological nitrogen fixation of pigeonpea and groundnut: quantifying response across 18 farm sites in Northern Malawi.” in Just Enough Nitrogen, eds. Sutton, M. A., Mason, K. E., Bleeker, A. Hicks, W. K., Masso, C., Raghuram, N., Reis, S., Bekunda, M (Springer, Cham), 139–153. doi: 10.1007/978-3-030-58065-0_10
Mignouna, D.B., Akinola, A.A., Suleman, I., Nweke, F., and Abdoulaye, T. (2014). Yam: A cash crop in West Africa. YIIFSWA working paper series No. 3. Yam Improvement for Income and Food Security in West Africa (YIIFSWA) (Ibadan, Nigeria: International Institute of Tropical Agriculture (IITA)).
N'dri, A. B., Kone, A. W., Loukou, S. K., Barot, S., and Gignoux, J. (2019). Carbon and nutrient losses through biomass burning, and links with soil fertility and yam (Dioscorea alata) production. Exp. Agric. 55, 738–751. doi: 10.1017/S0014479718000327
Neina, D. (2021). Ecological and edaphic drivers of yam production in West Africa. Appl. Environ. Soil Sci. 5019481. doi: 10.1155/2021/5019481
Newell, P., Taylor, O., Naess, L. O., Thompson, J., Mahmoud, H., Ndaki, P., et al. (2019). Climate smart agriculture? Governing the sustainable development goals in Sub-Saharan Africa. Front. Sustain. Food Syst. 3, 55. doi: 10.3389/fsufs.2019.00055
N'Guessan, A. E., N'dja, J. K., Yao, O. N., Amani, B. H., Gouli, R. G., Piponiot, C., et al. (2019). Drivers of biomass recovery in a Secondary forested landscape of West Africa. For. Ecol. Manag. 433, 325–331. doi: 10.1016/j.foreco.2018.11.021
Norgrove, L., and Hauser, S. (2015). Estimating the consequences of fire exclusion for food crop production, soil fertility, and fallow recovery in shifting cultivation landscapes in the humid tropics. Environ. manage. 55, 536–549. doi: 10.1007/s00267-014-0431-7
Nweke, F. I. (2019). African mysticism: A worldview that is a blessing and an impediment to technological change in West African yam food crop sector. Int. J. Arts Humanit. 7, 513–525.
Obidiegwu, J. E., and Akpabio, E. M. (2017). The geography of yam cultivation in southern Nigeria: Exploring its social meanings and cultural functions. J. Ethn. Foods. 4, 28–35. doi: 10.1016/j.jef.2017.02.004
Okongor, G., Njoku, C., Essoka, P., and Efiong, J. (2021). Climate variability and yam production: Nexus and projections. Sarhad J. Agric. 37. doi: 10.17582/journal.sja/2021/37.2.406.418
Oluwatayo, I. B., and Ojo, A. O. (2016). Awareness and adaptation to climate change among yam-based farmers in rural Oyo state, Nigeria. J. Dev. Areas. 1:97–108. doi: 10.1353/jda.2016.0078
Oruonye, E. D., and Adebayo, A. A. (2015). An assessment of the level of farmers awareness and adaptation to climate change in Northern Taraba State, Nigeria. J. Soc. Sci. Res. 1, 79–85.
Osei, K., Ennin, S. A., Danso, Y., Owusu Danquah, E., and Adomako, J. (2015). Management of plant parasitic nematodes with fulan in mechanized yam production. Afr. J. Plant Sci. 9, 346–351. doi: 10.5897/AJPS2015.1275
Ouyabe, M. H, Kikuno, N., Tanaka, P., Babil, H., and Shiwachi. (2019). Isolation and identification of nitrogen-fixing bacteria associated with Dioscorea alata L. and Dioscorea esculenta L. Microb. Resour. Syst. 35, 3–11.
Owusu Danquah, E. (2020). Evaluation of pigeonpea—white yam (Cajanus cajan [L] Millsp—Dioscorea rotundata [L] Poir) cropping system for improved yam productivity and livelihood of smallholder farmers. Doctoral Dissertation, Michigan State University, U.S.A. (2020).
Owusu Danquah, E., Ennin, S. A., and Acheampong, P. P. (2017). Integrated soil nutrient management options for sustainable yam production. Agron. Afr. 29, 69–81.
Owusu Danquah, E., Ennin, S. A., Frimpong, F., Acheampong, P. P., and Akom, M. (2018b). Producing white yam for export: The influence of seed sett size and planting density on tuber sizes. Agric. Food Sci J. Gh. 11, 929-936. https://csirspace.csirgh.com/handle/123456789/1416
Owusu Danquah, E., Ennin, S. A., Frimpong, F., Akom, M., and Acheampong, P. P. (2018a). Improved agronomic practices for sustainable yam production: The on-farm experience. Agric. Food Sci. J. Gh., 11, 904–908.
Owusu Danquah, E., Ennin, S. A., Lamptey, J. N. L., and Acheampong, P. P. (2015). Staking options for sustainable yam production in Ghana. Sustain. Agric. Res. 4:106–113. doi: 10.5539/sar.v4n1p106
Paul, C., and Knoke, T. (2015). Between land sharing and land sparing–what role remains for forest management and conservation? Int. For. Rev. 17, 210–230. doi: 10.1505/146554815815500624
Petersen, B., and Snapp, S. (2015). What is sustainable intensification? Views from experts. Land Use Policy. 46, 1–10. doi: 10.1016/j.landusepol.2015.02.002
Petsakos, A., Prager, S. D., Gonzalez, C. E., Gama, A. C., Sulser, T. B., Gbegbelegbe, S., et al. (2019). Understanding the consequences of changes in the production frontiers for roots, tubers, and bananas. Glob. Food Sec. 20, 180–188. doi: 10.1016/j.gfs.2018.12.005
Raines, C. A. (2011). Increasing photosynthetic carbon assimilation in C3 plants to improve crop yield: current and future strategies. Plant Physiol. 155, 36–42. doi: 10.1104/pp.110.168559
Sage, R. F., and Kubien, D. S. (2007). The temperature response of C3 and C4 photosynthesis. Plant Cell Environ. 30, 1086–1106. doi: 10.1111/j.1365-3040.2007.01682.x
Sagna, P., Dipama, J. M., Vissain, E. W., Diomand,é, B. I., Chabi, P. A. B., et al. (2021). “Climate Change and Water Resources in West Africa: A Case Study of Ivory Coast, Benin, Burkina Faso, and Senegal.” in Climate Change and Water Resources in Africa, eds. Diop, S., Scheren, P., Niang, A. (Springer, Cham), 55–86. doi: 10.1007/978-3-030-61225-2_4
Srivastava, A. K., Gaiser, T., and Ewert, F. (2016). Climate change impact and potential adaptation strategies under alternate climate scenarios for yam production in the sub-humid savannah zone of West Africa. Mitig. Adapt. Strateg. Glob. Chang. 21, 955–968. doi: 10.1007/s11027-015-9639-y
Srivastava, A. K., Gaiser, T., Paeth, H., and Ewert, F. (2012). The impact of climate change on Yam (Dioscorea alata) yield in the savanna zone of West Africa. Agric. Ecosyst. Environ. 153, 57–64. doi: 10.1016/j.agee.2012.03.004
Sultan, B., and Gaetani, M. (2016). Agriculture in West Africa in the twenty-first century: climate change and impacts scenarios, and potential for adaptation. Front. Plant Sci. 7, 01262. doi: 10.3389/fpls.2016.01262
Sylla, M. B., Nikiema, P. M., Gibba, P., Kebe, I., and Klutse, N. A. B. (2016). “Climate change over West Africa: recent trends and future projections.” in Adaptation to Climate Change and Variability in Rural West Africa, eds. Yaro, J., Hesselberg, J. (Springer, Cham), 25–40. doi: 10.1007/978-3-319-31499-0_3
Taylor, M., Lebot, V., McGregor, A., and Redden, R. J. (2018). “Sustainable production of roots and tuber crops for food security under climate change.” in Food Security and Climate Change, eds. S.S. Yadav, R.J. Redden, J.L. Hatfield, A.W. Ebert, D. Hunter (John Wiley and Sons Ltd), 359–376. doi: 10.1002/9781119180661.ch15
Thinh, N. C., Shimono, H., Kumagai, E., and Kawasaki, M. (2017). Effect of elevated CO2 concentration on growth and photosynthesis of Chinese yam under different temperature regimes. Plant Production Science 20, 227–236. doi: 10.1080/1343943X.2017.1283963
Tsado, E. K. (2012). Substituting wooden sticks with plastic stakes in yam production in Niger State, Nigeria. J. Nat. Sci. Res. 2, 88–96.
UNDP (United Nations Development Programme) (2012). Africa Human Development Report 2012 Towards a Food Secure Future (No. 267636). United Nations Development Programme. New York, U.S.A. https://doi.org/10.22004/ag.econ.267636
Van den Broeck, G., and Maertens, M. (2016). Horticultural exports and food security in developing countries. Glob. Food Sec. 10, 11–20. doi: 10.1016/j.gfs.2016.07.007
Vermeulen, S. J., Grainger-Jones, E., and Yao, X. (2014). Climate change, food security and small-scale producers: An analysis offindings of the fifth assessment report (AR5) of the intergovernmental panel on climate change (IPCC). CCAFS Info Note, CGAIR Research Programme on Climate Change, Agriculture and Food Security (CCAFS), Copenhagen.
Keywords: sustainable development goals (SDGs), integrated soil fertility management (ISFM), staking options, pigeonpea-yam cropping system, deforestation and land degradation
Citation: Owusu Danquah E, Danquah FO, Frimpong F, Dankwa KO, Weebadde CK, Ennin SA, Asante MOO, Brempong MB, Dwamena HA, Addo-Danso A, Nyamekye DR, Akom M and Opoku AY (2022) Sustainable Intensification and Climate-Smart Yam Production for Improved Food Security in West Africa: A Review. Front. Agron. 4:858114. doi: 10.3389/fagro.2022.858114
Received: 19 January 2022; Accepted: 22 April 2022;
Published: 10 June 2022.
Edited by:
J. S. Mishra, ICAR Directorate of Weed Research, IndiaReviewed by:
Paterne Agre, International Institute of Tropical Agriculture (IITA), NigeriaGordon Yenglier Yiridomoh, University for Development Studies, Ghana
Joseph Ogeh, University of Benin, Nigeria
Copyright © 2022 Owusu Danquah, Danquah, Frimpong, Dankwa, Weebadde, Ennin, Asante, Brempong, Dwamena, Addo-Danso, Nyamekye, Akom and Opoku. This is an open-access article distributed under the terms of the Creative Commons Attribution License (CC BY). The use, distribution or reproduction in other forums is permitted, provided the original author(s) and the copyright owner(s) are credited and that the original publication in this journal is cited, in accordance with accepted academic practice. No use, distribution or reproduction is permitted which does not comply with these terms.
*Correspondence: Eric Owusu Danquah, ericdany7@gmail.com