- 1Department of Microbiology, Barkatullah University, Bhopal, India
- 2School of Life Science, Devi Ahilya University, Indore, India
- 3School of Biotechnology, Devi Ahilya University, Indore, India
Fusarium oxysporum is one of the most damaging plant pathogens causing Fusarium wilt in many plants leading to serious economic loss. The fungus colonizes the xylem, which leads to resistance in water flow in the plant thereby affecting the rate of photosynthesis. The present study focuses on the selection of bacterial endophytes isolated from tomato plants and evaluating their potential to antagonize Fusarium oxysporum in tomato in vivo. The results obtained indicated that two endophytic isolates, namely Pseudomonas fluorescens BUMD5 and Bacillus velezensis BUMD9, could act as efficient biocontrol agents (BCAs) as they inhibited the growth of pathogen by 67.2 and 69.1%, respectively, in vitro. Both the isolates were found to produce hydrolytic enzymes chitinase and protease. They also produced siderophore and hydrogen cyanide (HCN). The consortia of both the isolates significantly reduced the infection percentage by about 67% and a 3-fold decrease in disease severity was observed as compared to pathogen control. The treatment of infected plants with these potent isolates was also beneficial in improving the overall photosynthetic performance index (PI). Thus, plants treated with consortia of these isolates exhibited better overall plant growth despite being infected by the pathogen.
Introduction
Fusarium oxysporum, an ascomycete, is a major disease-causing pathogen affecting plants in agricultural settings (Fisher et al., 2012). It is listed among the topmost devastating pathogens worldwide. Fusarium wilt is one of the major plant diseases caused by pathogenic Fusarium oxysporum strains (Dean et al., 2012). The spores produced by the fungus are known to remain in the soil for decades in a viable form thereby leading to the failure of crop rotation schemes (Nelson, 1981). The spores germinate after encountering plant root exudates and initiate the colonization of the host plant, followed by an invasion of vascular bundles. The water uptake system of the plant gets affected thereby causing severe wilting and sometimes death of the host plant (Altinok, 2005).
Tomato is also vulnerable to Fusarium wilt. The symptoms observed in infected plants include stunted growth, wilting, yellowing of leaves and stems, defoliation, marginal leaf necrosis, and vascular necrosis (Singh et al., 2017). The effect of a pathogen can be traced through the entire plant including shoot tips and fruits. The xylem discoloration might be observed only on one side initially but eventually leads to browning of the entire xylem (Cerkauskas, 2017). There are three protein complexes that are responsible for mediating the primary reactions of photosynthesis in the thylakoid membranes of chloroplasts. These include PSII, the cytochrome b6f complex (Cytb6f), and PSI, which are connected in series through the photosynthetic electron transport chain. The light-harvesting systems of PSII and PSI capture light energy and transfer it to the reaction center chlorophylls to create a charge separation across the membrane. This leads to the formation of a strong oxidant on the donor side of PSII capable of splitting water into molecular oxygen, protons, and electrons (Eberhard et al., 2008). The obstruction in the water transport system affects the rate of photosynthesis due to stomatal closure induced by water deficit. It is also found to affect the metabolic pathways of photosynthesis, Rubisco is one of the examples (Duniway and Slatyer, 1971; Lorenzini et al., 1997; Saeed et al., 1999; Pedrosa et al., 2011). Fusarium wilt is responsible for affecting three crucial processes in photosynthesis- the thylakoid electron transport, the carbon reduction cycle, and the stomatal control of the CO2 supply (Allen et al., 1997).
The present-day strategies used to control wilt include the use of chemical fungicides, heat sterilization of soil, and the use of resistant plant varieties; however, none of them proved to be completely successful. Chemical control methods include the application of some broad-spectrum biocides like carbendazim before planting. These chemicals are preventive in nature but cannot treat an infection. These are also found to be harmful to other beneficial soil microbes and can also enter the food chain thereby causing detrimental health (López-Aranda et al., 2016). The application of heat is non-selective and can affect the quality of soil (Mahmood et al., 2014). Developing resistant plant varieties is the most effective method but genetically encoded resistance is not durable for a longer period of time and leads to the emergence of new resistant strains (Takken and Rep, 2010; De Sain and Rep, 2015).
In recent years, endophytic micro-organisms which colonize host tissues internally without causing damage or eliciting disease symptoms, have received increased attention (Sturz et al., 2000; Chaturvedi et al., 2016). Endophytes have been considered successful candidates to be used as BCAs because of their ability to colonize a plant and have a more protective and less competitive environment as compared to their rhizospheric colleagues. The use of BCAs in an efficient manner is a self-sustaining and long-term method to control plant diseases and pests. Bacterial BCAs showing antagonistic actions against plant pathogenic fungi that cause a wide spectrum of plant diseases have been reported. Different mechanisms remain responsible for the antagonism of biocontrol agents that include the production of antifungal metabolites, competition for space and nutrients, mycoparasitism, and induction of the defense responses in plants (Glick, 1995; Howell, 2003; Chaturvedi and Prakash, 2020).
In the present study, we aimed to explore beneficial endophytic bacteria as potent biocontrol agents against Fusarium wilt of tomato. The isolates were selected on the basis of their antagonistic potential against Fusarium oxysporum in vitro. The selected isolates were tested for their capability of producing hydrolytic enzymes like chitinase and protease and plant growth promoting attributes like siderophore and HCN production. Furthermore, this study aims to understand the biocontrol effect of bacterial endophytes on Fusarium wilt in tomato plants and therefore, the photosynthetic apparatus which is affected during wilting. We also analyzed the amount of chlorophyll a (Chl a), chlorophyll b (Chl b), and total chlorophyll content (a+b) in plants treated with pathogen and biocontrol agents. By measuring Chl fluorescence kinetics, the efficiency of Photosystem II (PSII) photochemistry was measured. Energy pipeline models of photosynthetic apparatus visualized the alterations of PSII energy fluxes in response to the pathogen and its recovery by biocontrol agents. In summary, the isolates identified in the present study can be useful in controlling Fusarium wilt of tomato, the consortia of them being even more efficient.
Materials and Methods
Plants, Bacteria, Fungi, and Growth Conditions
The tomato wilt pathogen Fusarium oxysporum f. sp. lycopersici (ITCC 6859) was collected from the Indian type culture collection (ITCC), IARI, Delhi. The pathogen was maintained in Potato Dextrose Agar (PDA) (López-Martínez et al., 1999) at 4°C and periodically sub-cultured in PDA.
The tomato plants were collected from the Bhopal region (23.165964°N 77.328515°E) of Madhya Pradesh. Endophytic bacteria were isolated from roots, stems, and leaves (five samples each) of plants according to the method described by Hallmann et al. (1997) and Sturz et al. (1998). All the isolates were maintained in the nutrient agar slants at 4°C. The efficiency of the surface sterilization process was checked according to Hallmann et al. (1997) (detailed procedure and images in Supplementary File 1).
Antifungal Activity of Isolated Strains
The isolated microbes were screened for their antagonistic potential against Fusarium oxysporum by dual culture assay (Shabanamol et al., 2017). About 2 mm of Fusarium mycelia plug grown on PDA was placed at 2 cm from the edge of the agar plate and challenged on the other end of the plate at 2 cm from the edge with a single streak of the bacterial isolates. Petri plates were incubated at 28 ± 2°C for 7 days and percent (%) inhibition was calculated using the following formula:
where, I = % inhibition in mycelia growth; C = growth of pathogen in control plates; T = growth of pathogen in dual culture plates.
Identification of Selected Endophytes
Total two isolates showing maximum inhibition for the fungal pathogen were selected and identified by molecular means using 16S rRNA sequencing. Twenty-four-hours old cultures of the selected bacterial isolates were subjected to DNA extraction using a bacterial genomic DNA isolation kit (HiMedia) following the manufacturer's instructions. The extracted DNA was used to amplify 16S genes. The polymerase chain reaction (PCR) product was purified by using a PCR product purification kit (HiMedia) and was sent for sequencing (Bio-innovations, Mumbai, Maharashtra). The sequence obtained was analyzed using BLAST and was deposited in NCBI GenBank. The phylogenetic tree had been constructed using the MEGA7 software (Midhun et al., 2017).
Screening for Attributes Aiding to Antagonism
Production of Hydrolytic Enzymes-Chitinase and Protease
The ability of endophytic isolates to produce chitinase was determined by inoculating on a minimal medium amended with colloidal chitin as the sole carbon source (Renwick et al., 1991). Plates were incubated at 28 ± 2°C and analyzed for the zone of clearance for up to 10 days. Protease production was observed according to Smibert et al. (1994). The development of a clear zone around the bacterial colony inoculated in the skimmed milk agar indicated a positive result.
Siderophore Production
The Chrome Azurol S (CAS) agar medium was prepared as described by Schwyn and Neilands (1987) for the qualitative detection of siderophore production. The strain was inoculated in the Chromo Azurol S (CAS) (blue agar) plate and kept for incubation at 37°C for 48 h. The presence of a yellow to light orange halo zone in the medium surrounding the colony indicates the production of siderophore.
HCN Production
The production of HCN was observed according to Bakker and Schippers (1987). The log phase culture (50 μl) of the bacterial strain was spread on a nutrient medium containing glycine (4.5 gL−1). The change of color of the Filter paper (Whatman filter paper 1) soaked in 0.5% picric acid in 1% sodium bicarbonate placed in the upper lid of the Petri plate incubated at 28°C for 96 h from yellow to reddish brown was recorded as an index of positive for cyanogenic activity.
Effect of Selected Isolates on Vigor Index and Seed Germination
Surface sterilized tomato seeds were inoculated in bacteria broth with a final concentration of ~108 CFU per seed and were incubated for 24 h at 28 ± 2°C. Among them, 20 seeds were randomly selected by discarding the broth. They were transferred to the Petri plate containing sterile moistened blotting paper. Seeds treated with sterile distilled water (SDW) served as control. The Petri plates were incubated at 28 ± 2°C for 7 days under the dark condition for germination (Xia et al., 2015). The germination percentage was calculated using the formula:
The seedling vigor index was calculated using the following formula:
Evaluation of Biocontrol Efficacy of Selected Isolates in-vivo
Seed treatment was done as mentioned in section Effect of Selected Isolates on Vigor Index and Seed Germination. For treatment with the consortia of isolates, 10 ml of one culture was combined with 10 ml of another. The confirmation of endophytic colonization was done as mentioned in Supplementary File 2. About three treatments and two controls were considered for the experiment as mentioned in the table. The bacteria-treated tomato seeds were sown in the paper cups. After attaining the two true leaf stage the seedlings were transferred to the individual pots (12.5 × 14.5 cm) containing autoclaved soil. The treatments included in the study are as follows: T1- Plant uninoculated (Healthy Control), T2- Plant inoculated with pathogen, T3- Plant treated with BUMD5 and Fusarium, T4- Plant treated with BUMD9 and Fusarium, and T5- Plant treated with consortia and Fusarium.
Pathogen Inoculation
The Fusarium oxysporum spore suspension of inoculum was prepared by pouring 20 ml of SDW in each culture plate of 5–7 days old fungal mycelium and then gently scraped using the spore harvester. The concentration of conidia was adjusted to 107 conidia ml−1. Then, about 5 ml of prepared spore suspension was used to inoculate each seedling in all five treatments using the soil drenching method (Patil et al., 2011). In the soil drenching method, 5 ml of fungal suspension (i.e., water containing conidia of the pathogen) was inoculated to each of the seedlings by drenching the soil around the root zone with the help of a pipette. Before inoculation, the roots were slightly severed (wounded) by inserting a needle, 1 cm away from the stem. Root severing was done to ensure pathogen penetration through roots.
Symptom severity of the shoot system of the plants was assessed (6 weeks after pathogen inoculation) using the following scales: 0- no symptoms,1-yellowing 1–25% of the leaves near the stem base, 2-Yellowing, and wilt 26–50% of leaves with a simple brown discoloration in the xylem vessels, 3-Yellowing, and wilt 51–75% of the leaves with dark brown coloring in the xylem vessels and 4-Wilt and die 76–100% of leaves (Souza et al., 2010).
The disease severity index and infection percentage were calculated using the following formulae:
where, ni: is the number of plants affected by each degree of severity, si: the degree of severity of the attack (0–4), n: the total number of plants used for each energy level applied.
Estimation of Chlorophyll Content
Sample preparation was done according to Pérez-Patricio et al. (2018). Absorbance readings were performed at wavelengths of 663 and 645 nm. The control was acetone/ethanol (2:1 v/v). The obtained values were substituted in the following formulas, for the estimation of photosynthetic pigments:
Where, A663 and A645 are the absorbance measured from 663 and 645 nm, respectively.
Measurement of Fluorescence Induction Kinetics
Polyphasic chlorophyll a fluorescence transient called O-J-I-P (JIP-test) was measured using a plant efficiency analyzer (PEA, Hansatech, England) in tomato plants. In order to provide a homogeneous illumination, excitation light of 650 nm was focused on the surface of the leaf from an array of three light-emitting diodes. The intensity of light reaching the leaf was 3,000 μmol (photon) m−2 s−1, which was sufficient to produce maximum fluorescence (Fm). Plants were adapted to dark for 20 min prior to measurements. The Biolyzer HP 3 software (the chlorophyll fluorescence analysis program of the Bioenergetics Laboratory, gifted by the University of Geneva, Switzerland) was used to prepare the energy pipeline model.
Statistical Analysis
The data were subjected to one-way ANOVA and Tukey's HSD test at the level of p ≤ 0.05. All the statistical analyses were performed using the SPSS version 16 statistical package (IBM SPSS, USA).
Results
Selection of Potent Strains to Be Used as Biocontrol Agents Against Fusarium Wilt
A total of 36 isolates were collected from the different parts of the plant, viz. roots (17), stem (11), and leaves (8). All the isolates were tested for antifungal activity. A total of eight isolates showed anti-fungal activity in the dual culture method (Supplementary File 3) out of which two isolates, BUMD5 and BUMD9, showing maximum inhibition percentage, i.e., 67.2 and 69.1%, respectively, were chosen for further studies (Figure 1). Both the isolates were found to produce hydrolytic enzymes chitinase and protease (Figures 2A,B). Also, both the strains were positive for siderophore and HCN production (Figures 2C,D). The sequences were submitted in NCBI GenBank and accession numbers MZ223450 and MZ223454 were obtained. The isolate MZ223450 was found to have 99.06% similarity to Pseudomonas fluorescens P21(FJ605510.1) and MZ223454 was found to have 99.9% similarity to Bacillus velezensis HFBPR51 (MT539153.1), respectively, using sequencing and BLAST results. Figure 3 shows the phylogenetic trees obtained using MEGA software.
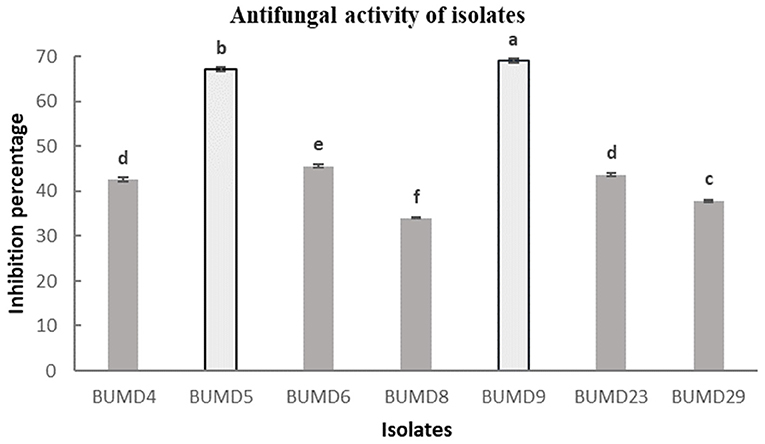
Figure 1. In vitro antifungal activity against Fusarium oxysporum. The strains showing maximum percentage inhibition BUMD5 and BUMD9 were selected for further studies.
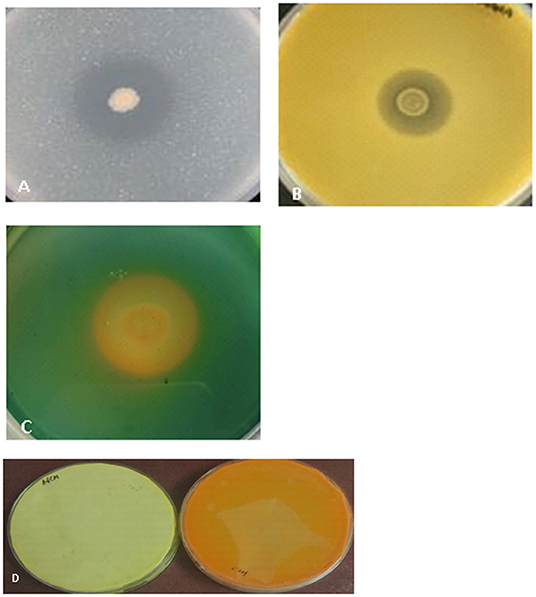
Figure 2. The selected isolates were tested for production of the following. (A) Chitinase- The zone of clearance shows hydrolysis of chitin by the isolate, (B) Protease- The clear zone surrounding the colony shows the production of protease enzyme by the isolate. (C) Siderophore- The orangish-yellow zone around the colony shows the isolate is positive for siderophore production. (D) HCN- The change of color of filter paper from yellow (control) to orangish brown is indicative of the production of HCN by the isolate.
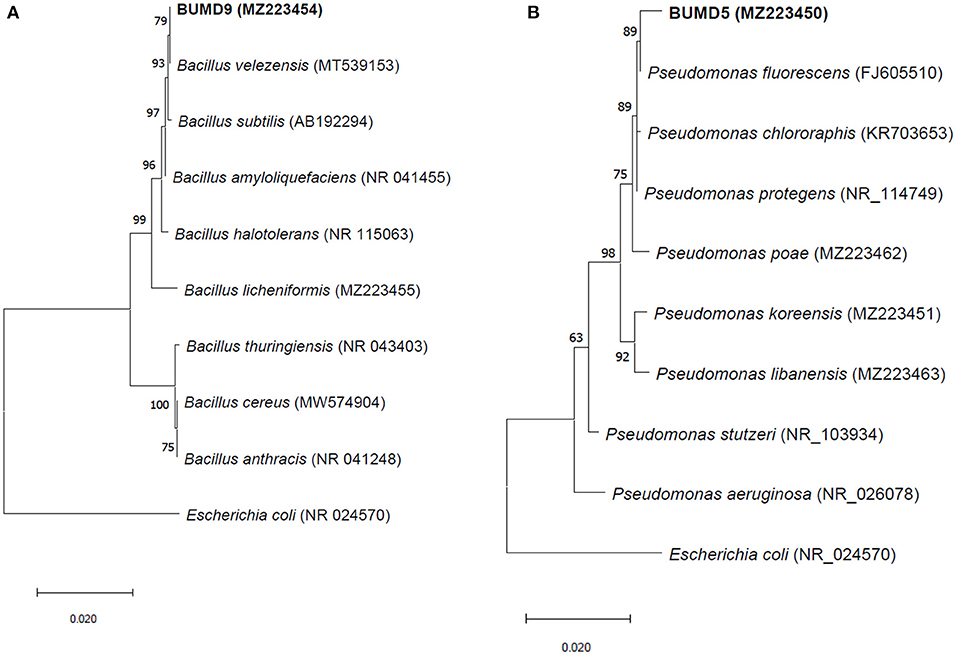
Figure 3. The phylogenetic trees for (A) BUMD9 (MZ223454) and (B) BUMD5 (MZ223450). The tree was constructed using the Neighbor Joining method and Bootstrap value 1,000.
Effect of Selected Isolates on Vigor Index and Seed Germination
The isolates were assessed individually as well in combination for seed germination potential. They showed an enhanced seed germination percentage and vigor index when compared to the uninoculated control after 7 days of incubation. It could be inferred that the seed germination and vigor index was influenced by the seed treatment with the isolates (Table 1).
Evaluation of Biocontrol Efficacy of Selected Isolates in vivo
Effect of Different Treatments on Plant Growth Parameters
It was observed that during the study, treatment T5 significantly reduced the infection by 67% when compared to control followed by treatments T3 and T4, which reduced by 26.7 and 33.3%, respectively (Table 2). Although there was no significant difference observed in the reduction in disease severity between T3, T4, and T5, there is an almost 3-fold reduction in disease severity when compared to the plant only inoculated with the pathogen (T2).
The growth parameters were observed for the plants after 45 days of pathogen inoculation (Figure 4). Maximum root length and shoot length, i.e., 18.1 ± 0.11 cm and 39.7 ± 0.5 cm (Figures 5A,B, respectively), were observed in T5, which is almost equal to that observed in an uninoculated or healthy plant (T1). However, a significant increase in fresh weight and dry weight (Figures 5C,D) was clearly observed in plants under the treatment T5 when compared to a healthy plant (T1).
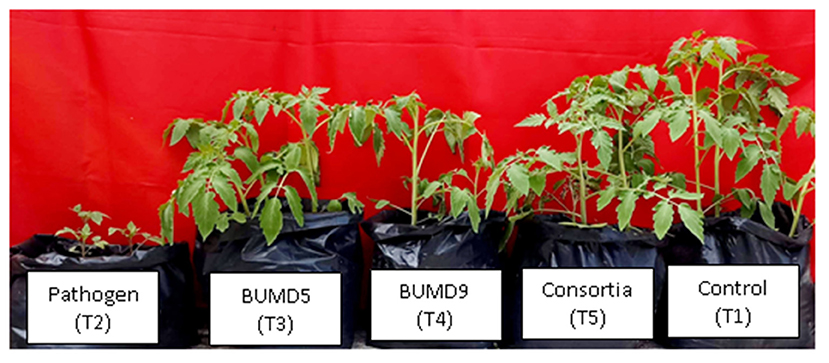
Figure 4. Effect of different treatments on plant growth in a pot experiment. T1- Plant uninoculated (Healthy Control), T2- Plant inoculated with Fusarium, T3- Plant treated with BUMD5 and Fusarium, T4- Plant treated with BUMD9 and Fusarium, and T5- Plant treated with consortia and Fusarium.
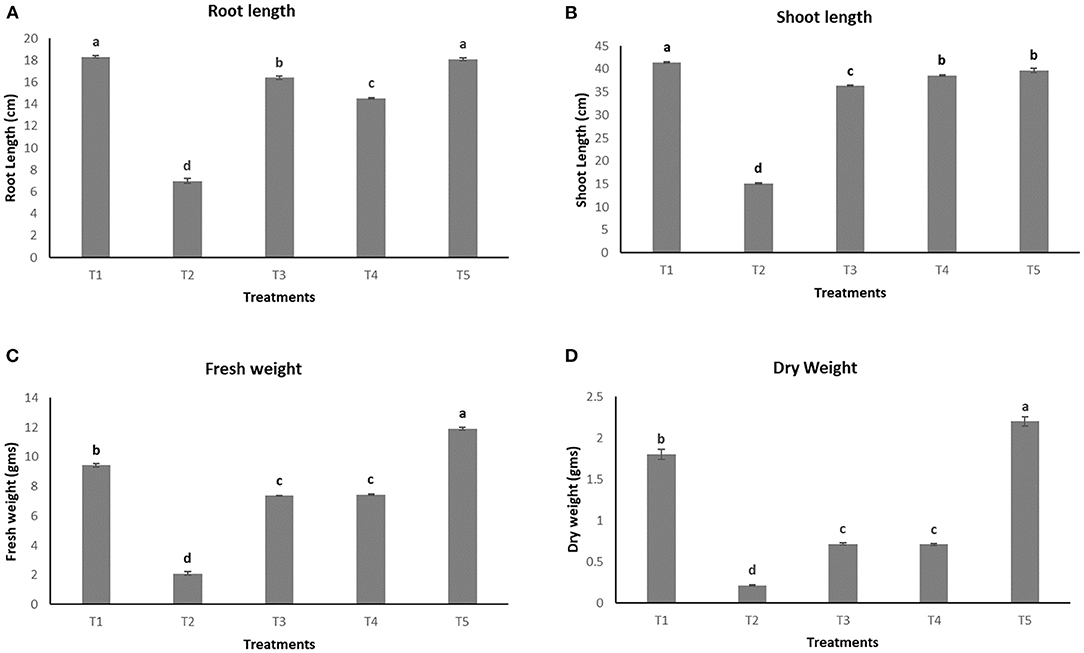
Figure 5. Effect of various treatments on the root length (A), shoot length (B), fresh weight (C), and dry weight (D) of plants.
Estimation of Chlorophyll Content
The effect of different treatments on leaves can be seen in Figure 6. However, no significant difference in concentration of chl a was observed among T1, T4, and T5 (20.05 ± 0.65 μμg/ml, 20.17 ± 1.99 μg/ml, and 20.90 ± 0.19 μg/ml, respectively) whereas the lowest concentration of the same was observed in the pathogen induced T2 (13.92 ± 1.57 μg/ml) as seen in Figure 7A. In the case of chlorophyll b, maximum concentration was seen in T4 and T5 (13.76 ± 1.27 μg/ml and 13.78 ± 1.07 μg/ml, respectively) followed by T1 (12.55 ± 0.70 μg/ml) and lowest concentration was observed in T2 (10.24 ± 1.16 μg/ml) as shown in Figure 7B. Total chlorophyll content (a+b) was found to be highest in T5 (34.69 ± 0.25 μg/ml) followed by T4 (33.95 ± 2.85 μg/ml) and the lowest concentrations were again observed in T2 (21.91 ± 1.33 μg/ml) as seen in Figure 7C.
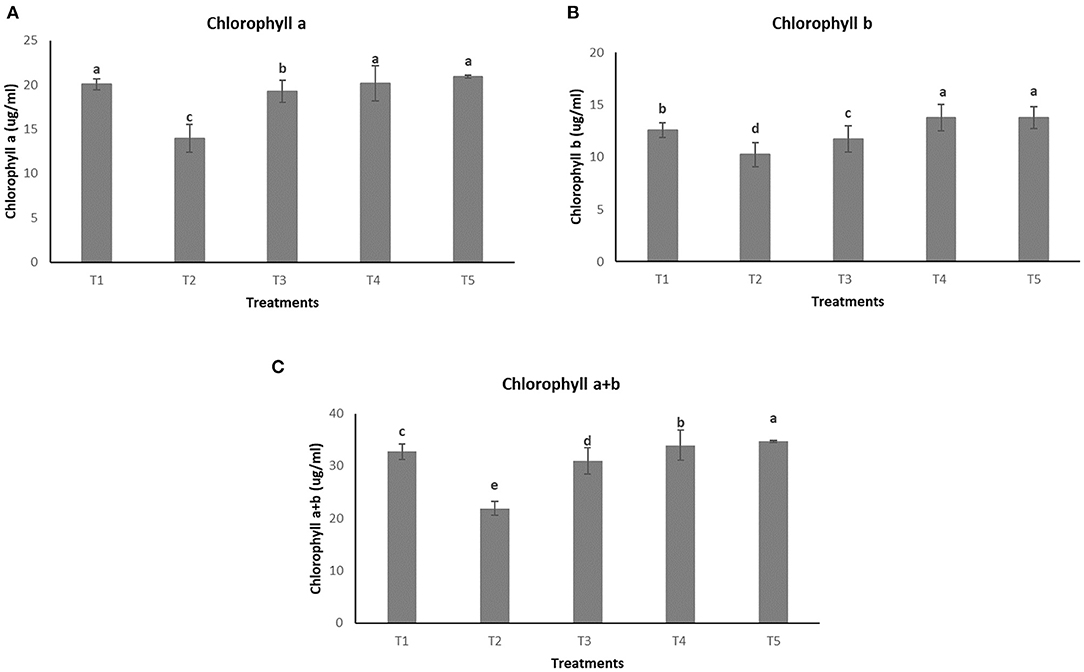
Figure 7. Effect of different treatments on chlorophyll content of plants (A) Chlorophyll a. (B) Chlorophyll b. (C) Total chlorophyll content (a+b).
Measurement of Fluorescence Induction Kinetics
The chlorophyll fluorescence transient curves are shown in Figure 8 and the parameters derived from them are shown in Table 3. A change in the shape of the curve and the fluorescence intensity of the OJIP transient were observed in control and treated plants. This was correlated with a change in the transport of photosynthetic electrons in the tomato plants treated with a pathogen and potent bacteria. As seen in Figure 8, in comparison to T1 (Control), the quantum efficiency of PSII photochemistry (as inferred from the Fv/Fm ratio) significantly decreased by about 13% in T2. Infected plants receiving biocontrol treatment decreased the damaging effect of the pathogen on plants and were least damaged (T5). In T2 plants, the efficiency of the water splitting complex (Fv/Fo) on the donor side of PSII was decreased significantly by 38% in comparison to control plants (T1). It is steadily recovered after the biocontrol treatments. In this study, RC/ABS, which reflects the number of active reaction centers decreased significantly in T2 by 21.5% as opposed to T1 (Control). The efficiency by which an electron trapped can pass further ahead of QA- is equivalent to (1-VJ) or Ψo (Tomar and Jajoo, 2013). No significant variation in the value of a 1-Vj was observed for different treatments except a decrease of around 13% in T2. The performance index (PI) sensitively reflects the function and vitality of the photosynthetic apparatus (Mathur et al., 2018). PI tremendously decreased by 57.1% in infected plants (T2) as compared to T1 (control) (Table 3). However, the application of biocontrol agents improved the PI and the effect was more pronounced in T5 where bacterial consortia could protect photosynthetic apparatus in plants from pathogenic effects.
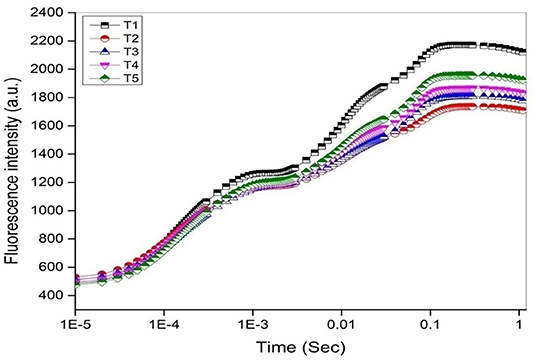
Figure 8. The OJIP chlorophyll a fluorescence transient curves (log time scale) after 45 days under different treatments.
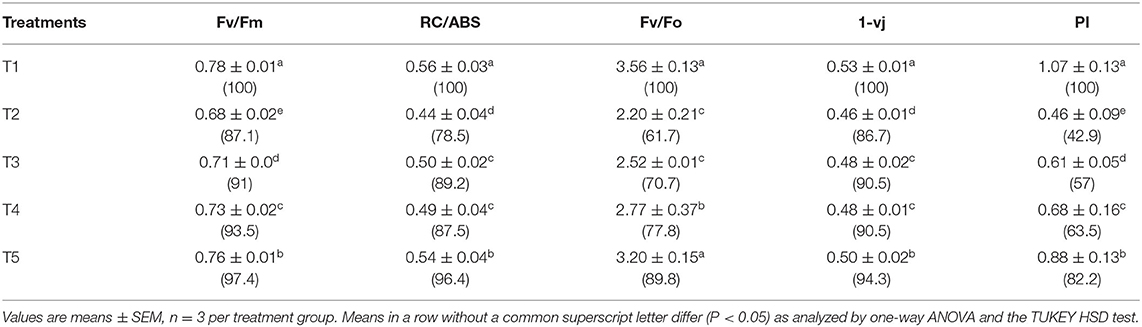
Table 3. Parameters obtained from fluorescence transient curves measured on day 45 under different treatments on tomato plant.
Relevant activities, such as ABS/CS, TR/CS, ET/CS, and DI/CS, indicating the efficiency of light absorption, trapping, electron transport, and dissipation per cross section of PSII, respectively, have been demonstrated in the form of energy pipeline models for various treatments (Figure 9). This model gives information about the efficiency of the flow of energy from antennae to the electron transport chain components through the RC of PSII. As compared to control (T1), T2 showed a decrease of 20% in ABS/CS, 27% in TRo/CS, and 29% in ETo/CS. However, the application of biocontrol treatments (T3, T4, and T5) led to improvement in the number of active reaction centers (indicated by open circles) as well as better rates of electron transport (indicated by the width of the blue line). T5 was found to be best with regard to the performance of the photosynthetic parameters.
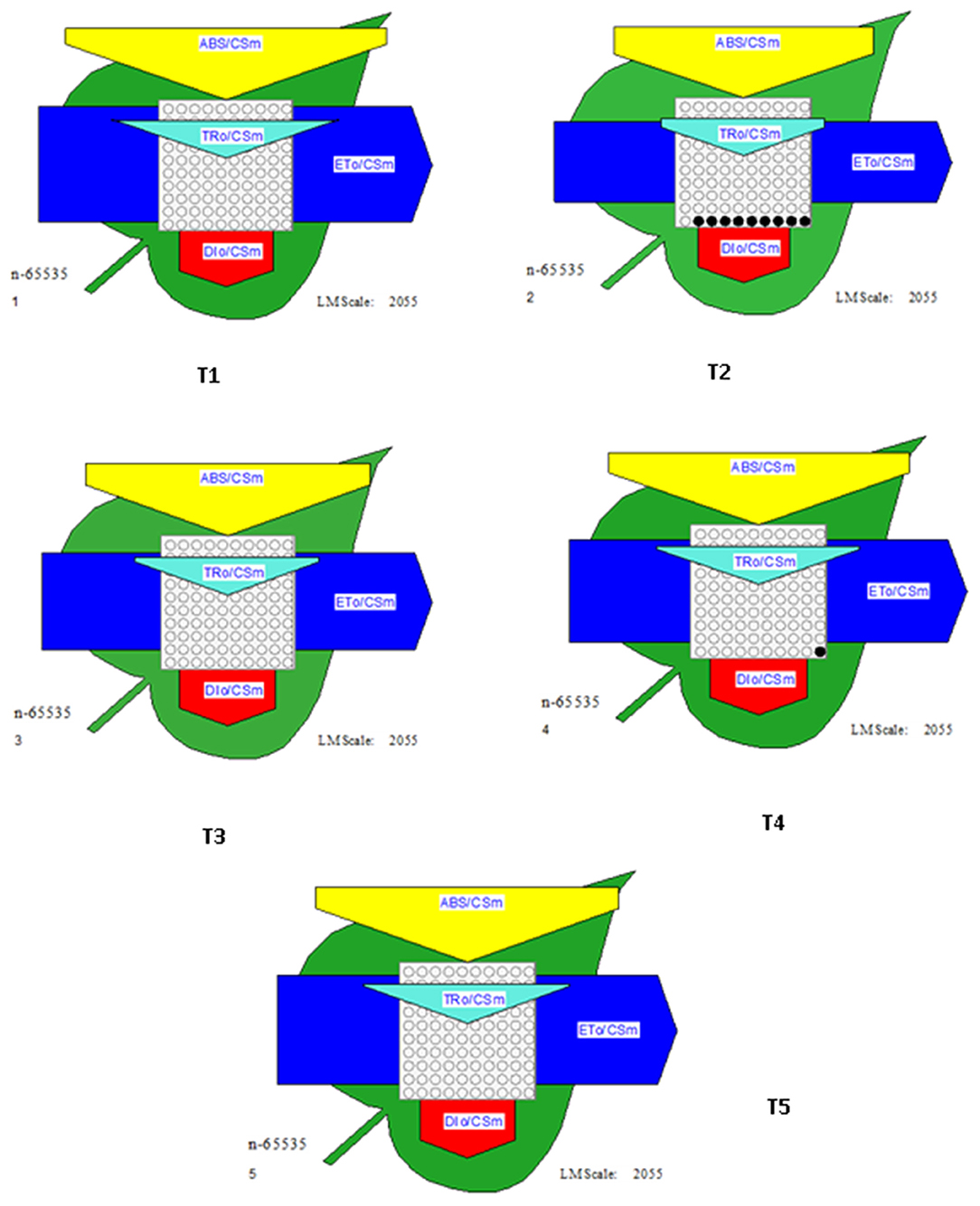
Figure 9. Energy pipeline leaf model showing proportions of phenomenological energy flux parameters within a leaf, calculated per cross-section (CSm) in different treatments. The width of the corresponding arrow denotes the activity of that parameter. Empty and filled black circles indicate the percentage of active and inactive reaction centers of PSII, respectively. ABS/CSm, Absorption per cross section; TRo/CSm, Trapping per cross section; ETo/CSm, electron transport per cross section; DIo/CSm, Dissipation per cross section.
Discussion
Fusarium wilt is among the most devastating diseases of tomato. The xylem colonization by the pathogen is known to increase water flow resistance in the plant, resulting in the leaf water deficit that is responsible for reducing the leaf photosynthesis and transpiration (Nogués et al., 2002). Therefore, it is necessary to seek an effective prevention and control strategy to control the Fusarium wilt disease during crop production. Using endophytes as biological control agents for plant diseases is an efficient and environmental-friendly approach.
In the present study, we have identified two potential strains, Pseudomonas fluorescens BUMD5 and Bacillus velezensis BUMD9, which when used individually as well as in combination can act as potent biocontrol agents for controlling the fusarium wilt in tomato plants. Both the isolates have shown significant antagonistic activity against Fusarium in vitro. They have also been demonstrated to produce hydrolytic enzymes, which aid the endophytes in the initial colonization process. The selected strains also produced HCN and siderophores, which help the endophytes in outcompeting the phytopathogens in the ecological niche. Several studies have shown Bacillus velezensis as a potential plant growth promoter and biocontrol agent. Bacillus velezensis NKG-2 was found to secrete fungal cell wall degrading enzymes, volatile organic compounds (VOCs), and indole-3-acetic acid and siderophore and also helped in reducing the disease severity of Fusarium oxysporum wilt disease on tomato plants both under in vitro and in vivo conditions (Myo et al., 2019). The strains also improved the chlorophyll content of plants as compared to diseased plants. Bacillus velezensis GF267 reduced the intensity of tomato bacterial spots and increased the chlorophyll content in plants as reported by Mates et al. (2019). Various strains of Pseudomonas fluorescens have also been shown to be effective biocontrol agents against multiple plant diseases (Yendyo et al., 2017; Kulimushi et al., 2021; Mosahaneh et al., 2021). As observed in the current study, the combination of these two isolates was more effective in controlling wilt as compared to the treatments containing these isolates alone. It has been evident that mixed inoculants interact synergistically, with a different or complementary mode of action and provide increased disease resistance. Various combinations of microbes have been shown to increase plant productivity and provide better disease resistance to plants. As reported by Pacheco et al. (2021), the microbial consortium increased maize productivity, and at the same time improved Phosphate use efficiency as compared to individual strains. To the best of our knowledge, the use of a combination of two selected isolates, Pseudomonas fluorescens and Bacillus velezensis, has not been reported earlier.
Endophytes have also been studied extensively for plant growth promotion. They have been considered better candidates as compared to rhizospheric microbes because of their close association with plants (Gupta et al., 2015). Many bacterial species including Bacillus, Pseudomonas, etc., have been used to improve plant productivity (Gupta et al., 2019). We have also obtained similar results in which better growth parameters like root length, shoot length, fresh weight, and dry weight have been observed in plants treated with BUMD5, BUMD9, and their consortia despite being treated with the pathogen Fusarium oxysporum.
Improved growth parameters are generally related to the better photosynthetic performance of the plant. To monitor the efficiency of photosynthesis, we used a non-invasive method of Chl a fluorescence kinetics. Chl a fluorescence kinetics parameters have been recognized as ideal predictors of photosynthetic performance and energy conversion efficiency of Photosystem II (PSII) (Mathur et al., 2018). Figure 9 shows the effect of treatments on the shape of chlorophyll a fluorescence transient to analyze changes in electron transfer reactions occurring at PSII (Papageorgiou, 2012). In the present study, decreased Fv/Fm values indicate stress due to a pathogen that damages the photosynthetic apparatus (Goltsev et al., 2016). To localize the effects of treatments on the acceptor side of PSII, the kinetics of relative variable fluorescence (Vj) were calculated. The efficiency by which a trapped electron can move further ahead of is equal to (1 - Vj) or Ψo (Tomar and Jajoo, 2013). Not much variation in the values of (1 - Vj) were observed in plants treated with or without bacteria except for a decline of 13% in T2. It suggests that QA- re-oxidation and electron transport at the acceptor side of PSII was not much affected (Tomar and Jajoo, 2013). The photosynthetic performance index (PI) is an indicator of plant vitality. PItotal is an overall parameter that incorporates biophysical parameters, determined from the JIP-test (Maliba et al., 2019). PI is the product of a dependent parameter for an antenna, reaction center, and electron transport. In the tomato plants under the pathogenic influence, biological treatment has resulted in an improved overall performance index (PI) (Figure 9). It can also be observed in other plant growth parameters and chlorophyll content.
Energy pipeline models of photosynthetic apparatus further visualized the alterations of PSII energy fluxes in response to the pathogen and its recovery by biocontrol agents (Figure 8). This model gives information about the efficiency of the flow of energy from antennae to the electron transport chain components through the cross-section of PSII (Li et al., 2014). It has been shown that ABS/CSm, ETo/CSm, TRo/CSm declined with T2 treatment. ETo/CSm decreased due to lower energy absorption by antenna pigments (ABS/CSm), lower energy trapping by RCs (TRo/CSm), and higher energy loss as heat (DIo/CSm). The decrease in TRo/CSm is mainly due to the decrease in the density of the active RCs and this is an indication that downregulation of PSII is accomplished by the inactivation of the RCs. As a negative effect of the pathogen, a decrease in the density of active reaction centers (indicated as open circles) and a rise in the density of closed reaction centers (indicated as full circles) have been observed (T2). Biological treatments may retrieve the negative effects of pathogens on the overall photochemistry. However, bacterial consortia could protect tomato plants better from the damaging effects of pathogens.
Conclusion
Fusarium wilt is a major disease affecting crops leading to severe economic loss every year. The clogging of the xylem is responsible for wilting leading to the death of the plant. Bacillus velezensis and Pseudomonas fluorescens can be considered potent biocontrol agents to control the disease. They have significantly reduced the deleterious effect on the photosynthetic apparatus, thereby improving plant growth. However, a better understanding of the impact of these isolates on the genes regulating photosynthesis is required for a more focused approach toward their development as bio-control agents on large scale.
Data Availability Statement
The datasets presented in this study can be found in online repositories. The names of the repository/repositories and accession number(s) can be found below: NCBI (accession: MZ223450 and MZ223454).
Author Contributions
HC, AJ, and AP contributed to conception and design of the study. HC organized the database, wrote the manuscript, and performed the statistical analysis. BS wrote sections of the manuscript. All authors contributed to manuscript revision, read, and approved the submitted version.
Funding
This study was supported by Government of India under DST Women Scientist Scheme WOS-A (Sanction order No. SR/WOS-A/LS-66/2017).
Conflict of Interest
The authors declare that the research was conducted in the absence of any commercial or financial relationships that could be construed as a potential conflict of interest.
Publisher's Note
All claims expressed in this article are solely those of the authors and do not necessarily represent those of their affiliated organizations, or those of the publisher, the editors and the reviewers. Any product that may be evaluated in this article, or claim that may be made by its manufacturer, is not guaranteed or endorsed by the publisher.
Supplementary Material
The Supplementary Material for this article can be found online at: https://www.frontiersin.org/articles/10.3389/fagro.2022.831731/full#supplementary-material
References
Allen, D. J., McKee, I. F., Farage, P. K., and Baker, N. R. (1997). Analysis of limitations to CO2 assimilation on exposure of leaves of two Brassica napus cultivars to UV-B. Plant Cell Environ. 20, 633–640. doi: 10.1111/j.1365-3040.1997.00093.x
Altinok, H. H. (2005). First report of Fusarium wilt of eggplant caused by Fusarium oxysporum f. sp. melongenae in Turkey. Plant Pathol. 54, 577–577. doi: 10.1111/j.1365-3059.2005.01235.x
Bakker, A. W., and Schippers, B. O. B. (1987). Microbial cyanide production in the rhizosphere in relation to potato yield reduction and Pseudomonas spp-mediated plant growth-stimulation. Soil Biol. Biochem. 19, 451–457. doi: 10.1016/0038-0717(87)90037-X
Cerkauskas, R. F. (2017). Etiology and management of Fusarium crown and root rot (Fusarium oxysporum) on greenhouse pepper in Ontario, Canada. Can. J. Plant Pathol. 39, 121–132. doi: 10.1080/07060661.2017.1321044
Chaturvedi, H., and Prakash, A. (2020). “Exploitation of plant tissue Invading Rhizospheric microbes as bio-fertilizers,” in Rhizosphere Microbes, eds S. K. Sharma, U. B. Singh, P. K. Sahu, H. V. Singh, and P. K. Sharma (Singapore: Springer Nature), 315–329.
Chaturvedi, H., Singh, V., and Gupta, G. (2016). Potential of bacterial endophytes as plant growth promoting factors. J. Plant Pathol. Microbiol. 7, 1–6. doi: 10.4172/2157-7471.1000376
De Sain, M., and Rep, M. (2015). The role of pathogen-secreted proteins in fungalvascular wilt diseases. Int. J. Mol. Sci. 16, 23970–23993. doi: 10.3390/ijms161023970
Dean, R., Van Kan, J. A. L., Pretorius, Z. A., Hammond-Kosack, K. E., DiPietro, A., Spanu, P. D., et al. (2012). The top 10 fungal pathogens in molecular plant pathology. Mol. Plant Pathol. 13, 414–430. doi: 10.1111/j.1364-3703.2011.00783.x
Duniway, J. M., and Slatyer, R. O. (1971). Gas exchange studies on the transpiration and photosynthesis of tomato leaves affected by Fusarium oxysporum f. sp. lycopersici. Phytopathology 61, 1377–1381. doi: 10.1094/Phyto-61-1377
Eberhard, S., Finazzi, G., and Wollman, F. A. (2008). The dynamics of photosynthesis. Annu. Rev. Genet. 42, 463–515. doi: 10.1146/annurev.genet.42.110807.091452
Fisher, M. C., Henk, D. A., Briggs, C. J., Brownstein, J. S., Madoff, L. C., McCraw, S. L., et al. (2012). Emerging fungal threats to animal, plant and ecosystem health. Nature 484, 186–194. doi: 10.1038/nature10947
Glick, B. R. (1995). The enhancement of plant growth by free-living bacteria. Can. J. Microbiol. 41, 109–117. doi: 10.1139/m95-015
Goltsev, V. N., Kalaji, H. M., Paunov, M., Baba, W., Horaczek, T., Mojski, J., et al. (2016). Variable chlorophyll fluorescence and its use for assessing physiological condition of plant photosynthetic apparatus. Russ. J. Plant Physiol. 63, 869–893. doi: 10.1134/S1021443716050058
Gupta, G., Chaturvedi, H., Snehi, S. K., and Prakash, A. (2019). Role of Plant Growth Promoting Rhizobacteria (PGPR) for Improvement of Sustainable Agriculture in Plant Growth Promoting Microorganisms: Microbial Resources for Enhanced Agricultural Productivity, eds S. R. Niranjan and A. C. Udayashankar. Hauppauge, NY: Nova Publisher.
Gupta, G., Parihar, S. S., Ahirwar, N. K., Snehi, S. K., and Singh, V. (2015). Plant growth promoting rhizobacteria (pgpr): current and future prospects for development of sustainable agriculture. J. Microb. Biochem. Technol. 7,096–102. doi: 10.4172/1948-5948.1000188
Hallmann, J., Quadt-Hallmann, A., Mahaffee, W. F., and Kloepper, J. W. (1997). Bacterial endophytes in agricultural crops. Can. J. Microbiol. 43, 895–914. doi: 10.1139/m97-131
Howell, C. R. (2003). Mechanisms employed by Trichoderma species in the biological control of plant diseases: the history and evolution of current concepts. Plant Dis. 87, 4–10. doi: 10.1094/PDIS.2003.87.1.4
Kulimushi, S. M., Muiru, W. M., and Mutitu, E. W. (2021). Potential of Trichoderma spp., Bacillus subtilis and Pseudomonas fluorescens in the management of early blight in tomato. Biocontrol Sci. Technol. 31, 1–12. doi: 10.1080/09583157.2021.1900784
Li, T., Hu, Y., Du, X., Tang, H., Shen, C., and Wu, J. (2014). Salicylic acid alleviates the adverse effects of salt stress in Torreya grandis cv. Merrillii seedlings by activating photosynthesis and enhancing antioxidant systems. PLoS ONE 9, e109492. doi: 10.1371/journal.pone.0109492
López-Aranda, J. M., Domínguez, P., Miranda, L., de los Santos, B., Talavera, M., Daugovish, O., et al. (2016). Fumigant use for strawberry production in Europe: the current landscape and solutions. Int. J. Fruit Sci. 16, 1–15. doi: 10.1080/15538362.2016.1199995
López-Martínez, R., Hernández-Hernández, F., Bazán-Moraand, E., and Castañón-Olivares, L. R. (1999). Comparative study of two culture conservation methods in medical mycology. World J. Microbiol. Biotechnol. 15, 471–474. doi: 10.1023/A:1008983217783
Lorenzini, G., Guidi, L., Nali, C., Ciompi, S., and Soldatini, G. F. (1997). Photosynthetic response of tomato plants to vascular wilt diseases. Plant Sci. 124, 143–152. doi: 10.1016/S0168-9452(97)04600-1
Mahmood, T., Mehnaz, S., Fleischmann, F., Ali, R., Hashmi, Z. H., and Iqbal, Z. (2014). Soil sterilization effects on root growth and formation of rhizosheaths in wheat seedlings. Pedobiologia 57, 123–130. doi: 10.1016/j.pedobi.2013.12.005
Maliba, B. G., Inbaraj, P. M., and Berner, J. M. (2019). Photosynthetic responses of canola and wheat to elevated levels of CO2, O3 and water deficit in open-top chambers. Plants 8, 171. doi: 10.3390/plants8060171
Mates, A. D. P. K., de Carvalho Pontes, N., and de Almeida Halfeld-Vieira, B. (2019). Bacillus velezensis GF267 as a multi-site antagonist for the control of tomato bacterial spot. Biol. Control 137, 104013. doi: 10.1016/j.biocontrol.2019.104013
Mathur, S., Jain, L., and Jajoo, A. (2018). Photosynthetic efficiency in sun and shade plants. Photosynthetica 56, 354–365. doi: 10.1007/s11099-018-0767-y
Midhun, S. J., Neethu, S., Vysakh, A., Sunil, M. A., Radhakrishnan, E. K., and Jyothis, M. (2017). Antibacterial activity of autochthonous bacteria isolated from Anabas testudineus (Bloch, 1792) and it's in vitro probiotic characterization. Microb. Pathog. 113, 312–320. doi: 10.1016/j.micpath.2017.10.058
Mosahaneh, L., Charehgani, H., Abdollahi, M., and Rezaei, R. (2021). Biological control agents in the management of different initial population densities of Meloidogyne javanica in tomato. Acta Phytopathol. Entomol. Hung. 55, 151–159. doi: 10.1556/038.55.2020.016
Myo, E. M., Liu, B., Ma, J., Shi, L., Jiang, M., Zhang, K., et al. (2019). Evaluation of Bacillus velezensis NKG-2 for bio-control activities against fungal diseases and potential plant growth promotion. Biol. Control 134, 23–31. doi: 10.1016/j.biocontrol.2019.03.017
Nelson, P. E. (1981). Chapter 3 - life cycle and epidemiology of Fusarium oxysporum. Fungal Wilt. Dis. Plants 51–80. doi: 10.1016/B978-0-12-464450-2.50008-5
Nogués, S., Cotxarrera, L., Alegre, L., and Trillas, M. I. (2002). Limitations to photosynthesis in tomato leaves induced by Fusarium wilt. New Phytol. 154, 461–470. doi: 10.1046/j.1469-8137.2002.00379.x
Pacheco, I., Ferreira, R., Correia, P., Carvalho, L., Dias, T., and Cruz, C. (2021). Microbial consortium increases maize productivity and reduces grain phosphorus concentration under field conditions. Saudi J. Biol. Sci. 28, 232–237. doi: 10.1016/j.sjbs.2020.09.053
Papageorgiou, G. C. (2012). “Fluorescence emission from the photosynthetic apparatus,” in Photosynthesis (Dordrecht: Springer), 415–443.
Patil, S., Sriram, S., and Savitha, M. J. (2011). Evaluation of non-pathogenic Fusarium for antagonistic activity against Fusarium wilt of tomato. Biol. Control 25, 118–123.doi: 10.18311/jbc/2011/3739
Pedrosa, F. O., Monteiro, R. A., Wassem, R., Cruz, L. M., Ayub, R. A., Colauto, N. B., et al. (2011). Genome of Herbaspirillum seropedicae strain SmR1, a specialized diazotrophic endophyte of tropical grasses. PLoS genetics 7, e1002064.
Pérez-Patricio, M., Camas-Anzueto, J. L., Sanchez-Alegría, A., Aguilar-González, A., Gutiérrez-Miceli, F., Escobar-Gómez, E., et al. (2018). Optical method for estimating the chlorophyll contents in plant leaves. Sensors 18, 650. doi: 10.3390/s18020650
Renwick, A., Campbell, R., and Coe, S. (1991). Assessment of in vivo screening systems for potential biocontrol agents of Gaeumannomycesgraminis. Plant Pathol. 40, 524–532. doi: 10.1111/j.1365-3059.1991.tb02415.x
Saeed, I. A. M., MacGuidwin, A. E., Rouse, D. I., and Sharkey, T. D. (1999). Limitation to photosynthesis in Pratylenchus penetrans-and Verticillium dahliae-infected potato. Crop Sci. 39, 1340–1346. doi: 10.2135/cropsci1999.3951340x
Schwyn, B., and Neilands, J. B. (1987). Universal chemical assay for the detection and determination of siderophores. Anal. Biochem. 160, 47–56. doi: 10.1016/0003-2697(87)90612-9
Shabanamol, S., Sreekumar, J., and Jisha, M. S. (2017). Bioprospecting endophytic diazotrophic Lysinibacillussphaericus as biocontrol agents of rice sheath blight disease. 3 Biotech 7, 1–11. doi: 10.1007/s13205-017-0956-6
Singh, V. K., Singh, H. B., and Upadhyay, R. S. (2017). Role of fusaric acid in the development of 'Fusarium wilt' symptoms in tomato: physiological, biochemical and proteomic perspectives. Plant Physiol. Biochem. 118, 320–332. doi: 10.1016/j.plaphy.2017.06.028
Smibert, R. M., Krieg, N. R., Gerhardt, P., Murray, R., and Wood, W. (1994). Methods for General and Molecular Bacteriology. Washington, DC: American Society for Microbiology, 607–654.
Souza, L. T., Michereff, S. J., Laranjeira, D., Andrade, D. E., Ferraz, E., Lima, G. S., et al. (2010). Reaction of tomato genotypes to races 2 and 3 of Fusarium oxysporum f. sp. lycopersici. Hortic. Bras. 28, 102–106. doi: 10.1590/S0102-05362010000100019
Sturz, A. V., Christie, B. R., and Matheson, B. G. (1998). Associations of bacterial endophyte populations from red clover and potato crops with potential for beneficial allelopathy. Can. J. Microbiol. 44, 162–167. doi: 10.1139/w97-146
Sturz, A. V., Christie, B. R., and Nowak, J. (2000). Bacterial endophytes: potential role in developing sustainable systems of crop production. CRC. Crit. Rev. Plant Sci. 19, 1–30. doi: 10.1080/07352680091139169
Takken, F. L. W., and Rep, M. (2010). The arms race between tomato and Fusarium oxysporum. Mol. Plant Pathol. 11, 309–314. doi: 10.1111/j.1364-3703.2009.00605.x
Tomar, R. S., and Jajoo, A. (2013). A quick investigation of the detrimental effects of environmental pollutant polycyclic aromatic hydrocarbon fluoranthene on the photosynthetic efficiency of wheat (Triticum aestivum). Ecotoxicology 22, 1313. doi: 10.1007/s10646-013-1118-1
Xia, Y., DeBolt, S., Dreyer, J., Scott, D., and Williams, M. A. (2015). Characterization of culturable bacterial endophytes and their capacity to promote plant growth from plants grown using organic or conventional practices. Front. Plant Sci. 6, 490. doi: 10.3389/fpls.2015.00490
Keywords: biological control, endophytes, photosynthetic apparatus, tomato plant, Fusarium wilt
Citation: Chaturvedi H, Singh B, Jajoo A and Prakash A (2022) Shielding of Photosynthetic Apparatus by Consortia of Bacterial Endophytes in Tomato Plants Suffering From Fusarium Wilt. Front. Agron. 4:831731. doi: 10.3389/fagro.2022.831731
Received: 08 December 2021; Accepted: 31 March 2022;
Published: 18 May 2022.
Edited by:
Rajni Singh, Amity University, IndiaReviewed by:
Bansh Narayan Singh, Banaras Hindu University, IndiaSavita Singh, Babu Shivnath Agrawal College, India
Dibyajyoti Pramanik, Gyeongsang National University, South Korea
Mahendra Vikram Singh Rajawat, National Bureau of Agriculturally Important Microorganisms (ICAR), India
Copyright © 2022 Chaturvedi, Singh, Jajoo and Prakash. This is an open-access article distributed under the terms of the Creative Commons Attribution License (CC BY). The use, distribution or reproduction in other forums is permitted, provided the original author(s) and the copyright owner(s) are credited and that the original publication in this journal is cited, in accordance with accepted academic practice. No use, distribution or reproduction is permitted which does not comply with these terms.
*Correspondence: Anil Prakash, ZHJhbmlscHJha2FzaDk4QGdtYWlsLmNvbQ==