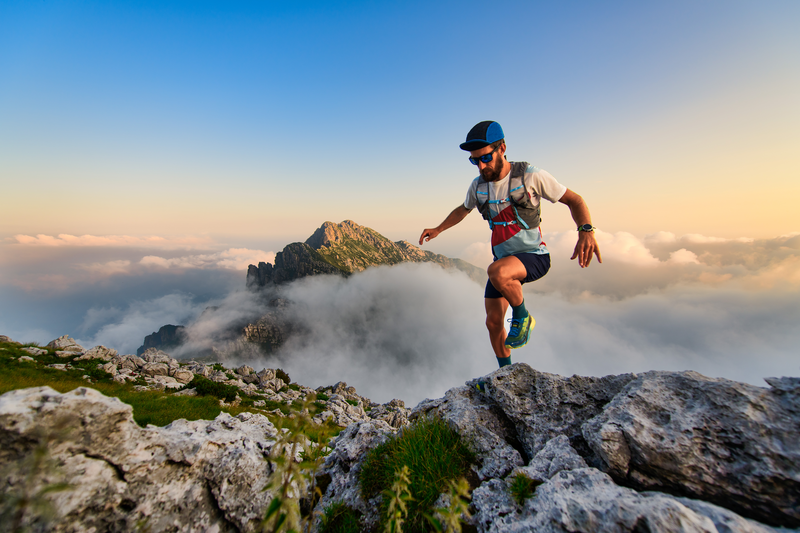
94% of researchers rate our articles as excellent or good
Learn more about the work of our research integrity team to safeguard the quality of each article we publish.
Find out more
ORIGINAL RESEARCH article
Front. Agron. , 31 March 2022
Sec. Agroecological Cropping Systems
Volume 4 - 2022 | https://doi.org/10.3389/fagro.2022.822456
Sowing a second season crop following the harvest of a first crop (hereafter referred to as double cropping) is a practice that allows for temporal diversification of cropping systems to increase the efficiency of land use and yield per unit area while improving the ecosystem services. Sunflower is particularly suitable for double cropping, especially under the current context of Southern Europe. However, planting sunflower in double cropping may result in poor establishment as the crop is very demanding in terms of seedbed preparations. In addition, most sunflower varieties available to date belong to late maturity groups (MGs), which were bred for conventional cropping. Planting these varieties in double cropping may further exacerbate the risk of crop establishment failure. Here, we performed laboratory and growth chamber phenotyping of 11 hybrid oilseed sunflower varieties with contrasting MGs and assessed their field performance for two consecutive years (2020 and 2021). We measured the variables, such as seed germination, seedling emergence dynamics and final rates, and post-emergence damage, as these characteristics are important for a uniform and robust crop establishment. Under laboratory conditions, we found statistically significant effect of varieties on cardinal temperatures and water potential for germination. Under growth chamber conditions, the maximum heterotrophic growth of the hypocotyl was higher (i.e., 85 mm) compared to that of the radicle (i.e., 80 mm). The seedling mortality rates under soil aggregates ranged from 0 to 12%, depending on the size and spatial distribution of soil aggregates in the seedbed. Under field conditions, the final rates of seed germination ranged from 87 to 98% and from 99 to 100%, while those of the seedling emergence ranged from 58 to 87% and from 78 to 94%, in 2020 and 2021, respectively. The average final rates of postemergence damage ranged from 13 to 44% and from 3 to 18% in 2020 and 2021, respectively. Bird damage was the main cause of pre- and postemergence losses. We found that a good sunflower establishment in double cropping is possible in the southwestern conditions of France, provided that there is no water stress in the seedbed. An optimal seedbed moisture ensures a rapid crop emergence and limits pre-and postemergence damage due to birds, by reducing the duration of the crop establishment phase, which is highly vulnerable to bird damage.
Sunflower (Helianthus annuus L.) has potential in facilitating the agro-ecological transition for different reasons, including its reduced reliance on conventional pesticides to manage pests and diseases compared to other field crops, lower need for water input due to its tolerance to water stress, and adaptability as a preceding crop for cereals (Lecomte and Nolot, 2011; Debaeke et al., 2017a; Lamichhane, 2020). More specifically to France, which is the third largest oilseed sunflower producing country in Europe with 1.3 Mt of production in 2019 (with 604,000 ha planted), representing 20% of the hybrid sunflower seeds produced in the European Union (TerresInovia, 2020). In France, sunflower is mainly grown in the southwestern parts, under conventional tillage and in relatively less diversified rotation systems, which increase the risk of disease pressure and soil erosion (Lecomte et al., 2020). This crop is often grown in hilly areas having clay soils that are sensitive to erosion due to their steep slopes, often >10% (Lecomte and Longueval, 2013). Growing sunflower in more sustainable cropping systems with no or minimum tillage could help reduce the negative environmental impacts of conventional tillage while improving the soil fertility level and ensuring productivity in water-limited environments (Ordóñez Fernández et al., 2007; Celik et al., 2013).
Sowing a second season crop following the harvest of the first crop (hereafter referred to as double cropping) may provide a number of agronimic and environmental benefits (Hexen and Boxley, 1986). This system is an example of temporal diversification to increase the efficiency of arable use and yield per unit area while improving the ecosystem services (Heaton et al., 2013). Double cropping could be an important adaptation strategy in the ongoing climate change to benefit from the longer cropping duration of the year (Graß et al., 2015; Seifert and Lobell, 2015). This method represents an effective way to increase productivity while protecting the soil and enhancing the carbon storage of the soil (Chataway et al., 2011). More specifically to sunflower, the introduction of this crop as a second or a catch crop could diversify the cropping system at field, farm, or territory level and, thus, might confer resilience to the existing conventional system (Debaeke et al., 2017b). For instance, in southwestern France, very early-maturing sunflower varieties could be planted after barley (Hordeum vulgare L.) or pea (Pisum sativum L.) completing their cycle in late spring.
Planting sunflower in double cropping in simplified systems (e.g., presence of previous crop residues, a coarse seedbed structure, etc.) might, however, be challenging as the crop is very demanding in terms of seedbed preparations for its successful establishment (Lecomte and Longueval, 2013). This could reduce the seed–soil contact thereby affecting the seed imbibition process, crop emergence, and a uniform and robust crop establishment (Blunk et al., 2021). This is especially true under south European conditions where water stress during the summer is the major limiting factor for field crops both before and after crop establishment (Debaeke et al., 2017b). Sunflower may compensate pre- and postemergence losses by increasing the head size and grain number per head of the remaining plants, provided that the crop is optimally managed to ensure a uniform stand development (Villalobos et al., 1994; Barros et al., 2004). However, this compensation may not occur in double crop sunflower where the risks of uneven stand development are high, due to water stress in the seedbed and bird damage at the crop-establishment phase. In addition, most sunflower varieties available to date have been bred for the conventional system that may not be suitable for double cropping in terms of maturity groups (MGs) or tolerance to water stress that further exacerbates the risk of crop establishment failure. Consequently, there is a need for phenotyping new crop varieties that are adequate for double cropping (Debaeke et al., 2021).
Seed germination and seedling emergence play an important role in the successful sunflower stand development and yield (Connor and Hall, 1997; Celik et al., 2013). Problems during seed germination and seedling emergence result in uneven stands that affect both yield quantity (e.g., number and size of grains per head) and quality (e.g., protein content). While previous studies investigated the role of temperature and moisture on seed germination and seedling emergence (Angus et al., 1980; Villalobos et al., 1994; Toscano et al., 2017), more detailed studies on the intraspecific variability of sunflower is lacking, especially in relation to double cropping. Likewise, there is a knowledge gap in the literature on the heterotrophic growth and resistance of sunflower seedling to mechanical stresses during emergence. This information is of paramount importance to select varieties that better respond to non-optimal seedbed conditions of sunflower, especially under double cropping.
The objectives of this study were three-fold: (i) laboratory and growth chamber phenotyping of sunflower varieties with different MGs to test their response to a range of temperature, water potential, seedling heterotrophic growth, and resistance to mechanical obstacles; (ii) field testing of these varieties in terms of seed germination and seedling emergence dynamics, and final rates, and; (iii) quantification of seedling emergence losses, postemergence damage, and identification of the key factors affecting the establishment of sunflower in double cropping. Besides determining the potential differences among the tested varieties in terms of measured variables, we also focused to create a database that could be used in the future for simulation purposes using the crop emergence model, SIMulation of Plant Emergence (SIMPLE; Dürr et al., 2001). Consequently, all the variables measured in this study were input variables of the SIMPLE. We did not focus on crop yield because we performed destructive measurements in our field study and measuring yield on previously damaged plants would have created a bias.
Laboratory experiments were performed to calculate the reference values on sunflower germination. They included the cardinal temperatures [i.e., the base (Tb), the minimum (Tmin), the optimal (Topt), and the maximum (Tmax) germination temperatures] and the base water potential for germination (Ψb). In addition, the experiments were conducted on early seedling growth with or without mechanical obstacles to assess the capacity of sunflower seedlings to lift soil aggregates and overcome such stresses during the emergence as this information was lacking in the literature.
Seed germination tests were carried out as described previously (Raveneau et al., 2011). Eleven hybrid sunflower varieties, belonging to very early (VE) to early MGs, suitable for double cropping, were used (Table 1). A commercial seed lot of the varieties, which was produced under standard growing conditions in France, was used. The seeds were pretreated with a mixed suspension of fungicides, such as Fludioxonil (Celest Net) and Metalaxyl-M (Apron XL), which were effective against plant pathogenic fungi and oomycetes, respectively (Lamichhane et al., 2020b). For each temperature, four replicates of 25 seeds were placed in 90 mm Petri dishes on the Whatman® filter paper of the same size placed both below and above the seeds and imbibed with 8 ml of deionized water. The dishes were incubated at 5, 10, 15, 20, 25, 30, 35, and 40°C, and the temperatures were recorded hourly with sensors. Depending on the incubation temperature, seed germination was assessed up to three times per day until no further germination was observed. A given seed was considered germinated when the radicle reached 2–3 mm in an isolated embryo, or pierced the seed coat (Gay et al., 1991).
Table 1. Key characteristics of 11 hybrid oilseed varieties of sunflower tested under laboratory conditions in 2020.
At each observation, the germinated seeds were removed from the dishes. Tb, germ was determined by fitting a Gompertz function with the observed germination rates. Adjustments of germination dynamics were made for each temperature, and the values of Tb were defined as the X-intercept of the linear regression between the temperature and germination rate (Gummerson, 1986; Dahal and Bradford, 1994). We determined the range of temperatures for which a strong linear relationship existed between the germination rates (1/time to reach 20, 40, 50, 60, 80, and 90% germination) to calculate the X intercepts. The value, Tb was defined as the intercept of the linear regression with the X-axis: the corresponding temperature is the point at which no germination occurs (Y-axis = 0%). The cardinal temperatures for germination, corresponding to the maximum germination speed, were determined using Yin's model which is a non-linear equation (Yin et al., 1995). In this equation, Tmin, Topt, and Tmax were found as function parameters.
Germination was also tested under seven different water potentials (0, −0.10, −0.25, −0.50, −0.75, −1.0, and −1.25 MPa) by adapting the previously described methods (Raveneau et al., 2011; Gardarin et al., 2016). The same number of seeds per replicate, as for the germination tests, was used. Since the use of polyethylene glycol (PEG) solution delay the seed germination (and increase the length of incubation) with an increased risk of seed contamination by mold, the seeds were disinfected by using 1% of sodium hypochlorite solution for 10 min followed by two rinses in distilled water. Twenty-five disinfected seeds were laid onto flat Whatman® filter paper in 90 mm Petri dishes with 20 ml of osmotic solutions of PEG with high molecular weight (PEG 8000, ref. SIGMA 25322-68-3). The PEG 8000 concentrations 0, 73, 130, 195, 250, 290, and 320 g/l of deionized water were used to obtain the water potentials of 0, −0.10, −0.25, 0.50, −0.75, −1.0 and −1.25 MPa, respectively (Michel, 1983; Michel and Radcliffe, 1995). The quantity of PEG solution used was higher (20 ml) than the quantity of deionized water used (8 ml) since the time to reach the maximum germination is longer in the PEG solution. The same adjustments, as for the germination tests, were used to calculate Ψb.
Destructive measurements of the hypocotyl and radicle growth dynamics under dark conditions (i.e., heterotrophic growth) were performed as described previously (Dürr and Boiffin, 1995; Lamichhane et al., 2020a). Since this experiment is particularly time-consuming, only the reference variety used as the control (i.e., RGT Lluis), was used for this experiment. Seeds were sown at 3 cm depth in polyethylene pots (five seeds per pot; 30 pots in total; ϕ = 10.5 cm; h = 11.5 cm) filled with 500 ml of white sand (150–210 μm). The pots were leaned on underlying plastic pots. The moisture gravimetric content was raised to 0.15 g g−1 before sowing by watering the sand with 75 ml of distilled water. The pots were covered with aluminum foil, to avoid light penetration and to prevent water loss, and incubated in a dark growth chamber at 20°C and 100% relative humidity. Seeds and seedlings were harvested by sampling three pots every day beginning from 2 days after sowing (DAS). At each observation (10 in total), all the emerging seedlings from the three pots were recovered. Plant organs were separated from the sand, washed, and the lengths of the radicle and hypocotyl were measured. Results were fitted to a Weibull and a probability function for hypocotyl and radicle growth, respectively (Dürr et al., 2001).
The test was performed in a growth chamber as described previously (Dürr and Aubertot, 2000). For the same reason as described for heterotrophic growth, only the reference variety RGT Lluis was used for this experiment. Clay loam soil (USDA classification; Table 2) was sampled from 0 to 20 cm soil horizon of a field plot (the same where field experiment was conducted in 2020), sieved through 5 mm holes, and stocked in sealed plastic containers, until further use, to avoid water loss. The gravimetric water content of the soil sample was measured (Gallardo-Carrera et al., 2007). Aggregates of different sizes were collected from the same field plot and air-dried for 4 weeks. The aggregates were grouped into three classes according to their longest axis L: 30–40, 40–50, and 50–60 mm.
Table 2. Seedbed and sowing conditions of hybrid oilseed varieties of sunflower for the two consecutive years, 2020 and 2021 at the Auzeville experimental station.
Two experiments were performed: the first with aggregates completely buried under soil and the second with aggregates placed on the soil surface. In each experiment, three replicates per class of aggregate (24 aggregates/replicate = 72 aggregates/class) were made. Each replicate consisted of a plastic tank (42 × 30 × 10 cm3) within which 4 L of the field soil were placed at the bottom to create a first layer (~4 cm depth). Twenty-four seeds were sown on this soil layer using a grid to define their position in the tank. Another 3 L of the soil were placed over the seeds to make a second layer (~3 cm depth) of the soil. For each class, aggregates were positioned on the top using another grid, so that their centers were just above the seeds. The aggregates were then covered with a third (and last) layer of soil (Ca. 3 cm), until they were completely buried. This final layer of soil was not added in experiments with aggregates laid on the soil surface. All plastic tanks were carefully sealed with aluminum foil, to avoid light penetration and water loss, and were incubated in the dark at 20°C and 100% relative humidity for 2 weeks until the hypocotyl attains the maximum length. Two weeks after incubation, each seed or seedling was observed in a vertical plane, and its state was recorded: germinated or not germinated, abnormal without striking an obstacle, blocked under an aggregate, or not blocked. The percentage of the seedling blocked under aggregates (both under buried conditions and when laid on the soil surface) observed was fitted to a probability function, taking a discrete number of values not necessarily finite (Dürr and Aubertot, 2000).
Field experiments were carried out for two consecutive years 2020 and 2021 at the Auzeville experimental station of INRAE (43.53°N, 1.58°E). In both the years, all the 11 varieties tested under laboratory conditions were planted in the field. The sowing dates and the physico-chemical characteristics of the key soil at the 0–30 cm soil horizon are presented in Table 2.
Daily and mean values of rainfalls, air temperatures, and seedbed temperatures and moistures on the sowing depth from sowing until emergence over the 2-year experiment are reported in Figure 1. The values of cumulative rainfalls from sowing until emergence were 6.5 and 12 mm, in 2020 and 2021, respectively. Daily values of gravimetric water content at the sowing depth ranged from 12 to 20% (average 15 ± 1%) in 2020 (Figure 1A) and from 8 to 11% (average 10 ± 1%) in 2021 (Figure 1B). Seedbed temperatures at the sowing depth ranged from 22 to 29°C in 2020 (mean 25 ± 1°C) (Figure 1A) and from 19 to 30°C (mean 25 ± 2°C) in 2021 (Figure 1B). The temperatures at 5 and 10 cm depth were very similar to that at the sowing depth while the gravimetric moisture was higher with the increasing sowing depth.
Figure 1. Sowing depth temperature, moisture, and rainfall from sowing until emergence of hybrid oilseed varieties of sunflower in 2020 (A), and 2021 (B) at the Auzeville experimental station. Vertical bars reported in the figures represent confidence intervals.
For both the years, immediately after the harvest of the previous crop (i.e., winter pea; Pisum sativum L.), stubble tillage was performed at 10 cm depth followed by a rotary pass (Claas Arion-620 130 hp, Agrisem). Sunflower varieties were sown with a single-disk seed driller (John Deere 5080) commonly used by farmers in France. Sowing was performed in 36 m2 micro plots (each 12 m long and 3 m wide, 3 microplots per variety) at 3 cm sowing depth with 7.2 pure live seeds m−2 and at 50 cm inter-row distance. This sowing density was based on a pure live-seed basis following adjustments for the germination ability of each hybrid at 25°C (i.e., average seedbed temperature at the sowing depth). According to the reports of the French Technical Institute For Oilseed Crops, an emergence density lower than 5 seeds m−2, associated with an irregular stand, strongly reduces the sunflower yield and the oil content (https://www.terresinovia.fr/-/tournesol-les-regles-d-un-semis-reussi). Therefore, we used a higher seeding rate than that in the conventionally-grown sunflower in France (i.e., between 5 and 6 seeds m−2) due to the risks of uniform stand development in double crop sunflower, characterized by a poor seedbed preparation and frequent water stress during the summer. The experimental design used was completely randomized blocks (3 blocks, each containing a microplot per variety). Each microplot consisted of six rows. A pre-emergence herbicide (Mercantor, 1.4 L/ha) was applied at 1 DAS in 2020 while no herbicide was used in 2021. An irrigation intervention was made (20 mm) at 4 DAS in 2020 while no irrigation was performed in 2021. No protection against vertebrate pests was used in 2020 while the plots were covered with a high-density polyethylene monofilament net (F1070-38g/m2 12 MI CRISTAL 100 PEHD) at the soil level in 2021 to avoid being damaged by birds and other animal pests at the crop establishment phase, which is a key problem for sunflower in France (Sausse et al., 2021).
In general, the seedbed structure and the number, size, and, in particular, the spatial distribution of the soil aggregated in the seedbed, affect the speed and final rates of seedling emergence. A simplified method for seedbed structure characterization, based on photographic comparisons of seedbed structure, was used as described previously (Dürr et al., 2016). To this aim, photos of the seedbed surface were taken immediately after sowing and compared with the historical database (Lamichhane et al., 2021). This approximation was used to choose a seedbed structure similar to the one already described in a database associated with the SIMPLE model that describes different types of seedbeds (Lamichhane et al., 2021).
Soil temperature and water content were recorded using climate sensors (ECH O 5TM, METER Group, Inc. USA) as described previously (Lamichhane et al., 2020a). The sensors were installed immediately after sowing at three soil horizons (1 sensor/depth/block at −3 cm, −5 cm, and −10 cm, 4 sensors/depth in total). These sensors delivered hourly temperatures, measured by an onboard thermistor, along with accurate volumetric water content. Data were recorded from sowing until crop emergence. Hourly data of rainfall and air temperatures were obtained from an automatic meteorological station installed at the experimental site. The cumulative degree-days (°Cd) were calculated from sowing (time 0) as the sum of the average daily air temperature minus the base temperature of each sunflower variety (Table 3).
Table 3. Final average rates of germination (± standard deviation) of 11 hybrid oilseed varieties of sunflower tested under laboratory conditions in 2020 under a wide range of temperatures.
The sowing depth was determined at 1 DAS, while the number of germinated seeds was counted every day beginning from 2 DAS as described previously (Lamichhane et al., 2020a). Only three varieties (RGT Capitoll, RGT Lluis and LG50300HOV) were considered as these measurements are time-consuming and they require a semi-destructive approach. A micro-profile was made by moving vertically until reaching the seeds along the row. The measurements were performed for 60 seeds per variety (four replicates of 5 seeds along four rows in diagonal per microplot and for 3 microplots per variety). The seeds were considered to have germinated successfully if the radicle was greater than 0.5 cm in length. The measurements for seed germination were carried out until reaching a plateau (i.e., the same number of germinated seeds for three consecutive days).
Seedling emergence was counted as described previously (Lamichhane et al., 2020a) in 4 m2/microplot, delimited with plastic pegs, with 3 microplots in total (2 linear meters/row, 4 rows in diagonal, for a total of 12 m2/variety). A seedling was considered emerged when it reached the VE stage (Schneiter and Miller, 1981) i.e., when the cotyledons were above the soil surface without any physical contact with the soil. The counting was proceeded until reaching a plateau with no new emerging seedlings for three consecutive days. However, the measurements continued when rainfall was foreseen in the coming days. This is due to the fact that rainfall triggers emergence of those seedlings that did not emerge due to water stress or due to mechanical obstacles, such as a soil surface crust by wetting the seedbed surface (Gallardo-Carrera et al., 2007).
The causes of non-emergence were identified at the VE stage (Schneiter and Miller, 1981) using a visual diagnostic key (Lamichhane, 2021a). Each symptom or characteristic observed in the field was assigned to a specific stress. To this objective, 60 empty points without seedling emergence/variety (5 along the row, 4 rows in diagonal per microplot, and 3 microplots in total) were randomly selected. A micro-profile was made by a spoon at each empty point moving vertically until reaching the non-germinated seeds or non-emerged seedlings. Once the seeds or seedling parts were found, their status was annotated according to their characteristics, and the possible causes of non-emergence were noted.
Postemergence damage due to animal pests is the most important problem leading to poor sunflower establishment in France (Sausse et al., 2021). Therefore, we performed field measurements to quantify postemergence damage. The countings were made on the same surface as for the seedling emergence measurements. At each observation, the total number of emerged seedlings with or without damage was counted as described previously. For the damaged seedlings, the type of damage viz., not severe (when the cotyledons were slightly injured without compromising the seedling growth), and severe (when the cotyledons were detached from the stem or the entire seedling was uprooted) was noted (Sausse et al., 2021). Based on the characteristics of the symptoms, the possible cause of postemergence damage was also noted using a visual diagnostic key (Lamichhane, 2021a). The countings of postemergence damage were performed from the VE to the V4 stages (Schneiter and Miller, 1981). The percentage of postemergence damage was calculated as the ratio between the number of damaged seedlings per damage type (not severe and severe) and the total number of emerged seedlings (Lamichhane, 2021b).
The number of germinated seeds and the emerged seedlings were converted into percentage for each observation. Arcsine and square root transformations were carried out on all percent data prior to the application of statistical analysis to improve the homogeneity of variance (Ahrens et al., 1990). An ANOVA was computed when the data followed a normal distribution according to the Shapiro–Wilk test (p < 0.05) while a non-parametric Kruskal–Wallis test was performed when the data did not meet the assumptions of the ANOVA. A two-way ANOVA was performed to determine the potential interaction of varieties, year, and varieties × year interactions on the measured variables under field conditions. In case of significant effect of the year on the measured variables, a one-way ANOVA followed by a Tukey's HSD post-hoc was computed to assess the significant differences between varieties under field conditions. The same analyses were performed also for the laboratory and growth chamber experiments. All statistical analyses were applied using R Studio (version 1.3).
The average final germination rates of 11 sunflower varieties at different temperatures are presented in Table 3. The ANOVA showed no significant effect of temperature on the final germination rates among the tested varieties at 5, 10, 15, 20, and 40°C while the germination rates among the varieties significantly differed at 25°C (p < 0.05), 30°C (p < 0.001), and 35°C (p < 0.001). Up to 25°C, all the varieties had the final germination rate over 90% while at 30°C, only LG 50268HOV had the final germination rate lower than 75% (i.e., 50%). The final germination rates began to decline for all varieties from 35°C at which all but RGT Bellus and LG 5478 had the germination rate lower than 50%. At 40°C, only LG 50505HOV, RGT Bellus, LG 50418HOV, SY Chronos, and LG 5478 germinated with a final rate of 4, 4, 4, 2, and 1%, respectively.
The average cardinal and base temperature values for the germination of 11 sunflower varieties are presented in Table 3. Statistically significant differences were observed among the tested varieties in terms of Tb (p < 0.01), Topt (p < 0.001), and Tmax (p < 0.001) but for Tmin (p > 0.05). The average values of Tb, Topt, Tmin, and Tmax of the 11 varieties were 4.55, 25.8, 1.18, and 37°C, respectively. The lowest and the highest values of Tb ranged between 3.44°C for SY Illico and 5.97°C for LG 50300HOV, respectively. The efficiency of the adjusted values, determined using Yin's model, ranged from 0.88 to 0.97°C. Likewise, the lowest and the highest values of Topt ranged from 22.5 to 28.2°C for RGT LLuis and LG 505HOV respectively, while those of Tmax ranged from 30 to 38°C for LG 50268HOV and RGT Bellus, respectively. In contrast, only small differences were observed in Tmin values of the tested varieties that ranged from 1 to 2°C.
The quality of adjustments performed by using the Gompertz function, for the germination dynamics of sunflower varieties observed at different temperatures, is reported in Supplementary Material S1. Efficiency of these adjustments ranged from 0.90 to 0.99°C. The thermal time to reach the 50% germination (TT50%germ) of sunflower varieties ranged from 37°Cd to 49°Cd, with an average value of 41°Cd. A graphical representation of the cardinal temperatures, as determined using Yin's model, is presented in Figure 2A. The average germination speed increased linearly from 5 to 25°C while it started to decrease from 30°C until becoming low to very low at 35 and 40°C, respectively.
Figure 2. Graphical representation of the Yin adjustments at 50% of germination (A), and seed germination speed at different water potentials (B) of 11 hybrid oilseed sunflower varieties under laboratory conditions in 2020. The points in the first figure represent the mean observed data, the solid lines indicate the adjusted Yin function while T0, Topt, Tmax indicate cardinal temperatures (refer to Table 3 for detailed information).
The efficiency of the adjustments performed using the Gompertz function with relation to the germination kinetics of each variety observed at different water potentials is reported in Supplementary Material S2. The efficiency ranged from 0.93 to 0.99. The average germination speed was maximal between 0 and −0.25 MPa and began to decrease from−0.5 MPa attaining the low or almost 0% at −1.25 MPa (Figure 2B).
The ANOVA showed significant differences (p < 0.001) among the tested varieties in their response to different water potentials (Table 4). The average final germination rate of the varieties did not significantly differ between −0, −0.1, and −0.25 MPa while it decreased significantly (p < 0.05) at −0.5 MPa, followed by −0.75, −1.0, and −1.25 MPa. At −0.75 MPa, only RGT Lluis had the final germination rate less than 50% at −1.0 MPa; all but Capitoll had the final germination rate under 50%, while at −1.25 MPa, all the varieties had a final germination rate <50%. Among all the tested varieties, RGT Capitoll and RGT Lluis had the highest and the lowest germination rates between 0 and −1.25 MPa, respectively. The value, Ψb was the lowest (−1.26 MPa) and the highest (−0.83 MPa) for RGT Capitoll and RGT Lluis, respectively with the average Ψb of −1.012 MPa (Table 4).
Table 4. Final average rate of seed germination (± standard deviation) of 11 hybrid oilseed varieties of sunflower tested under laboratory conditions in 2020 under a wide range of water potentials.
The hypocotyl attained its maximum length (i.e., 85 mm) at 200°C d after sowing (i.e., at 184°Cd after germination) (Figure 3A). The radicle began to grow before the hypocotyl and it reached its maximum length (i.e., 80 mm) at 95°Cd after sowing (i.e., at 80°Cd after germination) (Figure 3A). Thermal time needed to reach 50% of the final length was at 50°Cd and 100°C after germination for the radicle and hypocotyl, respectively. The seedling emerged and reached 3 cm length at 110°Cd after sowing. The elongation rates between the sowing and emergence were 0.12 and 0.24 mm/°Cd for the radicle and hypocotyl, respectively. The efficiency of the adjustments made with the Weibull function was high with the values of 0.75 and 0.87 for the radicle and hypocotyl, respectively (Supplementary Material S3).
Figure 3. Mean rates of the hypocotyl and radicle elongation (A), and seedling mortality under different soil aggregate sizes and spatial distribution (B) of hybrid oilseed sunflower (cv. RGT Lluis) in relation to cumulative degree-days (°Cd) under growth chamber conditions in 2020. Vertical bars reported in the figures represent confidence intervals.
The rate of seedling mortality was 0 and 12% for the aggregates placed on the surface and for those completely buried in the seedbed, respectively (Figure 3B). The efficiency of the adjustments made with the probability function was quite satisfactory with the corresponding value of 0.69 both for the above-ground and buried clods (Supplementary Material S3).
The photographical comparisons of the seedbed surfaces with those presented in the database showed that the seedbed had a coarse structure with more than 25% of soil aggregates having the diameter greater than 20 mm, in both the experimental years.
The average sowing depth values are reported in Figure 4. The average sowing depth ranged from 2.3 to 2.9 cm and from 3.5 to 3.6 cm in 2020 and 2021, respectively. The sowing depth was significantly different among the varieties in 2020 (p < 0.001) but not in 2021 (Figure 4A). Significant effect of varieties (p < 0.05), year (p < 0.001), and variety × year interactions (p < 0.05) was observed on the average sowing depth (Table 5).
Figure 4. Average sowing depth of three hybrid oilseed varieties of sunflower under field conditions over the two experimental years, 2020 and 2021 at the Auzeville experimental station. Vertical bars reported in the figures represent confidence intervals. The different letters indicate a significant difference between the tested varieties.
Table 5. Results of two-way ANOVA showing the effects of variety, year, and variety × year interaction on the mean sowing depth and the final mean germination and emergence rates, and the results of one-way ANOVA on the mean germination and emergence rates at each counting for individual experimental year.
Seed germination dynamics and the final rates of sunflower varieties under field conditions are reported in Figure 5. Germination in 2020 began at 1 DPS and attained the plateau at 8 DPS (171°Cd) while germination in 2021 initiated at 2 DPS and reached its maximum rate at 9 DPS (147°Cd; Figure 5A). Final germination rates ranged from 87 to 98% in 2020 and from 99 to 100% in 2021. Significant effect of the crop variety was observed on final germination rates in 2020 but not in 2021 (Table 5; Figure 5B). Significant effect of variety (p < 0.05), year (p < 0.01) and variety × year interactions (p < 0.05) was observed on the final germination rates (Table 5).
Figure 5. Mean seed germination dynamics (A) and final rates (B) of three hybrid oilseed varieties of sunflower over the two experimental years, 2020 and 2021 at the Auzeville experimental station. Vertical bars reported in the figure represent confidence intervals.
Seedling emergence dynamics and final rates under field conditions are reported in Figure 6. Emergence began at 6 DPS and attained the maximum values at 15 DPS (310°Cd) in 2020 while it began at 5 DPS and attained the maximum values at 15 DPS (318°Cd) in 2021 (Figure 6A). In both years, the significant effect of varieties was observed on seedling emergence dynamics although this effect was inconsistent over time (Table 5).
Figure 6. Emergence dynamics (A) and final rates (B) of 11 hybrid oilseed varieties of sunflower over the two experimental years, 2020 and 2021 at the Auzeville experimental station. Vertical bars reported in the figure represent confidence intervals. The different letters indicate a significant difference between the tested varieties.
Final emergence rates ranged from 58 to 87% in 2020, and no significant effect of the varieties was observed on this variable (Figure 6B). In contrast, in 2021, final emergence rates ranged from 78 to 94% and with a significant effect (p < 0.05) of the varieties. In 2021, the final emergence rates were the highest for variety LG 50514HOV followed by LG 5478, while the lowest final emergence rates were observed for RGT Lluis followed by RGT Capitoll and LG 50300HOV (Figure 6B). A significant effect of year (p < 0.001) but not that of variety and variety × year interactions was observed on the average final emergence rates (Table 5).
The final rates of non-emergence are reported in Figure 7, while non-emergence rates due to each cause are reported in Supplementary Figure S1. In both the years, seed predation (i.e., absence of seed or presence of damaged seed) due to birds was the key cause of non-emergence while minor impacts of mechanical stresses (i.e., seedling mortality due to a soil surface crust and soil clods) and of soil-dwelling pests were also observed. A significant effect of variety (p < 0.05) was observed in 2021 on seed predation due to birds while no significant effect of variety was observed due to other causes of non-emergence (Supplementary Figure S1). The total rates of non-emergence ranged from 13 to 43% in 2020 and from 7 to 23% in 2021. A significant effect of variety (p < 0.05) was observed on the final rates of non-emergence in 2021 with LG 5478 showing the lowest emergence losses and RGT Bellus and RGT Capitoll showing the highest emergence losses (Figure 7).
Figure 7. Rates of non-emergence of 11 hybrid oilseed varieties of sunflower over the two experimental years, 2020 and 2021 at the Auzeville experimental station. Vertical bars reported in the figure represent confidence intervals. The different letters indicate a significant difference between the tested varieties.
The combined values of severe and non-severe post-emergence damage rates are reported in Figure 8 while individual values of severe and non-severe rates of postemergence damages are presented in Supplementary Figure S2. Overall, postemergence damage ranged from 7 to 24% and from 3 to 18% in 2020 and 2021, respectively (Figure 8B). Significant effect of variety (p < 0.01) was observed on the final rates of postemergence damage in 2020 but not in 2021.
Figure 8. Rates of postemergence damage due to pigeons on the 11 hybrid oilseed varieties of sunflower over the two experimental years, 2020 and 2021 at the Auzeville experimental station. Vertical bars reported in the figure represent confidence intervals. The different letters indicate a significant difference between the tested varieties.
In 2020, the rates of severe postemergence damage due to the common wood pigeon (Columba palumbus L.) ranged from 4 to 22% while those of non-severe damage ranged from 7 to 33% (Supplementary Figure S2). In 2021, the rates of postemergence damage were very low ranging from 0 to 3% for the severe type and from 0 to 10% for the non-severe type. Significant effect of variety (p < 0.05) was observed on the severe type of postemergence damage caused by pigeons in 2020 but not in 2021. In contrast, no significant effect of the varieties was found on the non-severe types of postemergence damage in both years (Supplementary Figure S2). Besides the postemergence damage caused by pigeons, no damage due to other biotic stresses was observed in 2020. In contrast, the non-severe postemergence damage occurred in 2021 that included damping-off like symptoms (0–1%), damage due to wild animals (0–4%), slugs (0–5%), and that due to soil-borne pests (0–3%). Significant effect of varieties was observed on the average final rates of postemergence damage both in 2020 (p < 0.001) and in 2021(p < 0.05; Supplementary Figure S2).
The results of this study showed that the final germination rates of the sunflower varieties are the highest between 5 and 25°C and that they decline above 30°C with no germination at 40°C, which corroborates with the previously reported results (Macchia et al., 1985). We found that low germination rates at higher temperatures were due to an increase in the number of “fresh non-germinated seeds” with reduced germination potential (Maeda and Ungaro, 1985).
Two oleic varieties, LG 50300HOV and LG 50418HOV, had the highest germination speed at different temperatures. This can be explained by their very early maturity class and also by their highest thousand seed mass (i.e., 88.47 and 88.11 g, respectively), as previously suggested (Torres and Martinez-Honduvilla, 1990). Although the type of acid content (i.e., either oleic or linoleic) affects the seed germination at low temperatures (González Belo et al., 2014); we did not find any significant effect of the type of acid composition or the thousand seed mass on the final germination rates, which is in agreement with a previous study (Hocking and Steer, 1989). Other characteristics, such as the internal morphology of the seed and the internal area containing the embryo, may be the reason behind the contrasting genotypic behaviors in the germination speed (Rocha et al., 2014).
We found significant genetic variability in sunflower varieties in terms of Tb, Topt, and Tmax. This information is important while choosing the varieties for double cropping, with relation to the sowing dates. For example, across southern Europe, varieties with higher Topt values (e.g., LG50505HOV, LG 50300HOV, and RGT Bellus) could be more adapted for double cropping due to higher average temperature in these regions during summer. Likewise, varieties with higher and Tmax values (e.g., RGT Bellus and LG 5478) are suitable in these regions, especially for late sowing under double cropping, given a high average temperature in southern Europe. Overall, the average Tb of the 11 varieties found in this study (i.e., 4.55°C) is very similar to the previously reported value of 4.4°C (Tribouillois et al., 2016) and 4.5°C (Trudgill et al., 2000). Likewise, the average thermal time of the tested varieties to reach 50% of germination was 40.68°Cd, which is very similar to the previously described value of 40.7°Cd for sunflower (Mwale et al., 1994).
Significant genetic variability was observed in sunflower varieties in terms of tolerance to water stress, which is very helpful in decision-making related to various choices. In our study, the Ψb values ranged from −0.8 to −1.3 MPa, which are in agreement with the results of previous studies: −1 MPa (Kaufman, 1969), −1.2 MPa (Mwale et al., 2003), and −1.6 MPa (Tribouillois et al., 2016). Varieties with high water stress tolerance (e.g., RGT Capitoll) found in our study could provide a promising result in double cropping under rainfed conditions. In contrast, the varieties characterized by a low tolerance to water stress (e.g., RGT Lluis) should be chosen for double cropping under irrigated conditions. Our results showed that the average Ψb of sunflower is −1.0 MPa, which is very similar to the value reported in the literature (Kaufman, 1969).
When sown at 3 cm depth, it took sunflower 103°Cd to emerge (0.29 mm/°Cd) compared to 67°Cd needed for the soybean (Lamichhane et al., 2020a) showing that the rate of the growth of the sunflower hypocotyl is lower than that of the soybean. Also, the rate of the radicle elongation was lower for sunflower (0.32 mm/°Cd) compared to that of soybean (0.58 mm/°Cd) (Lamichhane et al., 2020a). The radicle growth began before that of the hypocotyl and secondary roots started to appear at 128°Cd. There was an important effect of the moisture content of the substrate on the heterotrophic growth of the sunflower. At higher moisture content (20% in the first experiment), discoloration-like symptoms were observed on the hypocotyls that led to a reduced radicle growth. This abnormality was much less frequent when the moisture content was reduced (15% in the second experiment).
Our observations corroborate with the previously published results that reported excess water as an important abiotic stress for the sunflower, thereby affecting the yield (Chapman and de la Vega, 2002). Excess water disrupts the mechanisms of nutrient and water absorption by the roots (Orchard et al., 1986) as well as leaf senescence, respiration, and photosynthesis in the sunflower (Grassini et al., 2007). Although 20% of moisture content has been reported as an optimal level for several other species including the soybean (Lamichhane et al., 2020a), sunflower seems to better develop at a lower water content. This is consistent with the fact that Ψb of sunflower is much lower than that of soybean (Ψb = −0.67 MPa) (Lamichhane et al., 2020a). This means that sunflower is less sensitive to water stress while it is more sensitive to excess water compared to the soybean. Excess water just after sowing or germination is stressful for sunflower: it affects the seedling emergence and the dry matter content of the roots (Loose et al., 2017). We also observed the development of secondary and adventitious roots near the soil surface at 20% moisture level, which is a reaction of sunflower to excess water (Yasumoto et al., 2011). An increased rate of the hypocotyl and a reduced rate of radicle elongation occurs in sunflower under conditions of hypoxia (Gay et al., 1991), which are consistent with our observations.
When the seedling growth or emergence force is lower than the weight of soil aggregates, seedlings are unable to lift such obstacles, and, as such, remain trapped and die under soil aggregates (Souty and Rode, 1993). However, we found that sunflower seedlings are less sensitive to mechanical obstacles, such as soil aggregates compared to other crop species. Indeed, only 13% of seedlings remained trapped under fully buried clods of 50–60 mm in diameter which is much lower compared to 31 and 75% of the seedling mortality observed under the same conditions for soybean (Lamichhane et al., 2020a) and sugar beet (Beta vulgaris L.; Dürr and Aubertot, 2000), respectively. This information is important while preparing the seedbed for sunflower in double cropping where farmers may not always have sufficient time for a fine seedbed preparation between the harvest of the previous crop and the sowing of sunflower.
The crop establishment phase consists of three sub-phases: sowing to germination, germination to emergence, and emergence to initial competition among young seedlings (Aubertot et al., 2020; Lamichhane, 2021a). This study demonstrated that both abiotic and biotic factors affect these sub-phases of sunflower establishment. More specifically to abiotic stresses, water stress in the seedbed represents a severe constraint for sunflower establishment in years like 2020, which was characterized by almost no rainfall just before or after sowing, which is very common across our study site. Irrigation is needed in such a case to avoid crop emergence failure. In contrast, the year 2021 was characterized by frequent rainfall both pre- and post-sowing, requiring no irrigation, which is uncommon across our study site. Despite a coarse seedbed structure in double cropping, a relatively rapid seed imbibition was ensured by the presence of adequate seedbed moisture due to irrigation and rainfall in 2020 and 2021, respectively. In both the years, the lack of water stress in the seedbed did not allow to observe genetic variability in sunflower varieties in terms of drought tolerance, as observed under laboratory conditions.
Biotic stresses, in general, and bird damage, in particular, were the most important factors affecting the sunflower both in pre-emergence and postemergence. In the absence of protective nets in the experimental plots, as the one observed in 2020, sunflower establishment was particularly affected by birds with an average of 22 and 18% of seed predation and severe seedling damage, respectively. This was the reason for which the experimental plots were protected with nets in 2021. Our results corroborate with those of a recent study (Sausse et al., 2021) across other regions of France. In the absence of protective nets, 98% of the spring-sown sunflower fields under conventional tillage showed partial or total seedling damage symptoms attributable to birds with 10% of the fields presenting over 20% of completely destroyed seedlings (Sausse et al., 2021). Overall, adequate seedbed moisture not only ensures a high rate of seedling emergence but also reduces the duration of the crop establishment phase, which is particularly vulnerable to bird attacks for a number of crops including sunflower (Sausse et al., 2021), soybean (Firake et al., 2016; Lamichhane, 2021b), and maize (Zea mays L.; Furlan et al., 2021). More specifically to sunflower, bird attack on this crop at an earlier growth stage may lead to partial or total seedling damage (Sausse and Lévy, 2021). This can lead to irregular and non-uniform stand development with a consequent impact on the crop yield.
We observed no significant effect of crop variety on germination dynamics and final rates but there were significant effects of varieties on emergence dynamics and final rates. Unlike seed germination, which is mainly affected by soil temperature and moisture, seedling emergence is affected by several other factors including soil aggregates, soil surface crust, soil compaction, water stress, soil temperature, etc. This is especially true for cropping systems characterized by the same tillage system having no heterogeneity in terms of soil–seed contact. The lack of significant genetic variability in seed germination dynamics and final rates could be also related to the fact that these crop varieties have been selected for a number of characteristics including low dormancy rate and high germination power. Therefore, future studies focusing on the genetic variability of sunflower under field conditions should rather focus on the potential differences among the crop varieties in postgermination (i.e., the two sub-phases of the crop establishment phase). Our results corroborate with a previous study on a small-seeded crop (i.e., carrot; Daucus carota L.) under field conditions, where delays in the emergence of the carrot seedling occurred largely in postgermination (Finch-Savage et al., 1998).
In both the experimental years, the average sowing depth temperature was almost the same as the Topt values determined under laboratory conditions (i.e., 25.8°C). In contrast, the mean values of gravimetric water content at the sowing depth were not optimal. This was especially the case in the absence of irrigation in 2021, with the gravimetric moisture content values ranging from 8 to 11% (average 10 ± 1%), very similar to the soil water stress thresholds (i.e., ~10%) for the germination and emergence of the sunflower seed. This was the reason why it took 15 DAS for sunflower to reach the final emergence rates in both the experimental years. Indeed, depending on the varieties, degree days required to attain the final emergence rates ranged from 289 to 327°Cd (mean 310) in 2020 and from 297 to 335°Cd (mean 318) in 2021. These values are much higher than those reported for sunflower (i.e., ~134°C days) to complete the emergence phase under no water stress conditions (Angus et al., 1980). This means that water and mechanical stresses (in particular, soil compaction that results from an interaction between the beating action of the rainfall, soil aggregates, fine soil, and soil surface crust) slowed down the germination and emergence speed, respectively, lengthening the duration of the crop establishment phase.
Similar effects of such stresses have also been observed on soybean establishment across the same study site (Lamichhane et al., 2020a; Lamichhane and Aubertot, 2021). When seedlings encounter soil clods in the seedbed during emergence, they divert their path to reach the seedbed surface. This results in a longer time for emergence as the hypocotyl needs further elongation to reach the seedbed surface (Dürr and Aubertot, 2000). Likewise, when the soil is partially compacted, seedlings remain trapped under the soil and they are not able to overcome such a mechanical barrier unless further humidification of the seedbed surface occurs following a rainfall. This increases the duration of the crop establishment phase under field conditions (Gallardo-Carrera et al., 2007).
Despite a higher seedbed average moisture in 2020, the duration of the sunflower establishment phase was very similar in both years. This was especially because the gravimetric water content of the seedbed was not optimal in 2020 during the first sub-phase of crop establishment as the irrigation was triggered only at 4 DAS. In contrast, there was only a small soil moisture variation in 2021 during the crop establishment phase.
Higher average emergence rates, lower seed predation, and postemergence seedling damage due to birds in 2021 were also due to the use of protective nets. Although such protection is not feasible under on-farm conditions, the difference in the rates of seed predation and postemergence seedling damage between the two experimental years clearly highlights the importance of crop management practices in improving the quality of sunflower seedling establishment in double cropping. To this aim, synchronizing the harvest of winter cereals, such as winter wheat (Triticum sp.) with the sowing of sunflower in double cropping may constitute an effective management lever. This is because birds might be attracted toward wheat plots where they can forage wheat grains lost during the harvest. Alternatively, a direct sunflower sowing in double cropping on the living or dead mulches, followed by irrigation, could be another bird-escape strategy to reduce seed and seedling damage due to birds. Indeed, the presence of crop residues may act as a physical barrier for the foraging activities of birds that needs further investigation by future research.
We found two main factors affecting the quality of sunflower establishment under double cropping across southwestern France: (i) the soil moisture in the seedbed, and (ii) bird damage to seeds and seedlings. We showed that the presence of sufficient seedbed moisture from germination until emergence is important not only to ensure a high emergence rate but also to accelerate the vigor of seed germination and seedling emergence. The latter is strictly related to the duration of the crop establishment phase, which needs to be reduced as much as possible, given the vulnerability of this phase to bird attacks. Therefore, despite being costly and energy-intensive, irrigation may be needed to ensure good sunflower establishment in a double cropping system across temperate and Mediterranean regions.
Sunflower may compensate pre- and postemergence losses by increasing the head size and grain number per head of the remaining plants provided that the crop is managed under optimal conditions to ensure a uniform stand development (Villalobos et al., 1994; Barros et al., 2004). However, this was not the case in double crop sunflower across our study site where there was water stress in the seedbed and bird damage at the crop establishment phase. In addition, the destructive approach used during the field measurements for sowing depth and seed germination resulted in partial or total damage of seeds and seedlings, which may have further affected the uniform seedling establishment. Therefore, we assumed that, under this condition, higher rates of non-emergence and postemergence damage of sunflower would have led to lower yields. However, future studies are needed to test the effect of irrigated vs. non-irrigated and protected vs. non-protected plots on the establishment of quality and yield of sunflower in double cropping.
Among all the tested varieties, the RGT Capitoll resulted to be the most tolerant to water stress under laboratory conditions (Ψb = −1.26 MPa). Therefore, this variety could have a good potential for double cropping where water stress in the seedbed is the most important limiting factor for sunflower establishment under southern European conditions. Overall, our study offers an important dataset on the genetic variability of early growth traits in sunflower, in general, and those adapted for double cropping system, in particular, which were poorly available to date in the literature. Consequently, the results of our study can make a significant contribution to improve or better parameterize both the specific sunflower crop models, such as Sunflo (Casadebaig et al., 2011) and OilCrop-Sun (Villalobos et al., 1996) or generic models, such as STICS (Brisson et al., 2003) or CropSyst applied to sunflower (Stockle et al., 1994), all of which are characterized by a very simplified germination-emergence module.
The datasets presented in this study can be found in online repositories. The names of the repository/repositories and accession number(s) can be found in the article/Supplementary Material.
JL and PD designed the research and acquired the funding for the research project. JL, AW, and CB performed the experiments and analyzed the data. JL wrote the manuscript. All authors contributed to the article and approved the submitted version.
The authors declare that the research was conducted in the absence of any commercial or financial relationships that could be construed as a potential conflict of interest.
All claims expressed in this article are solely those of the authors and do not necessarily represent those of their affiliated organizations, or those of the publisher, the editors and the reviewers. Any product that may be evaluated in this article, or claim that may be made by its manufacturer, is not guaranteed or endorsed by the publisher.
The authors thank the technicians of the Vasco research team, UMR AGIR, and those of the Auzeville experimental unit for field crops (UE APC, Auzeville, INRAE; https://doi.org/10.15454/1.5483266728434124E12), for their kind support during this study. A special thanks to Gilles Tison and Clément Lemouzy, UE APC Auzeville, for implementing field experiments; Damien Marchand, Béatrice Quinquiry, Pierre Perrin, and Benoit Gleizes for their help in the field and laboratory experiments. Thanks also to Euralis Semences, RAGT2n and Syngenta for providing seeds. This study was supported by the Ministry of Agriculture through CASDAR IPHARD (Idéotypage et PHénotypage pour l'Adaptation des variétés de soja et de tournesol à la culture en Relai ou en Dérobé) and by UMT PACTOLE.
The Supplementary Material for this article can be found online at: https://www.frontiersin.org/articles/10.3389/fagro.2022.822456/full#supplementary-material
Supplementary Material S1. Adjustments of the seed germination dynamics of eleven hybrid oilseed varieties of sunflower observed under laboratory conditions in relation to a range of temperatures. The adjustments were performed using a Gompertz function.
Supplementary Material S2. Adjustments of the seed germination kinetics of eleven hybrid oilseed varieties of sunflower observed under laboratory conditions in relation to a range of water potentials. The adjustments were performed using a Gompertz function.
Supplementary Material S3. Adjustments of the dynamic of heterotrophic growth and seedling mortality under clods of hybrid oilseed sunflower (cv. RGT Lluis) under growth chamber conditions. The adjustments related to heterotrophic growth were performed by using a Weibull function while those related to seedling mortality under clods were computed by applying a probability function.
Supplementary Figure S1. Rates and causes of nonemergence of eleven hybrid oilseed varieties of sunflower over the two experimental years, 2020 and 2021 at the Auzeville experimental station. Vertical bars reported in the figure represent confidence intervals. (A) Predation of seeds by birds; (B) damage caused by granivores; (C) pregermination water stress; (D) pre-emergence of damping-off; (E) damage due to wireworm; (F) seedling mortality under a soil crust; (G) seedling mortality under soil aggregates; (H) too high sowing depth; (I1) postgermination water stress.
Supplementary Figure S2. Rates of nonsevere (M1) and severe (M2) types of postemergence damage due to pigeons of eleven hybrid oilseed varieties of sunflower over the two experimental years, 2020 and 2021 at the Auzeville experimental station. Vertical bars reported in the figure represent confidence intervals.
Ahrens, W. H., Cox, D. J., and Budhwar, G. (1990). Use of the arcsine and square root transformations for subjectively determined percentage data. Weed Sci. 38, 452–458. doi: 10.1017/S0043174500056824
Angus, J. F., Cunningham, R. B., Moncur, M. W., and Mackenzie, D. H. (1980). Phasic development in field crops I. Thermal response in the seedling phase. F. Crop. Res. 3, 365–378. doi: 10.1016/0378-4290(80)90042-8
Aubertot, J.-N., Deguine, J.-P., Lamichhane, J. R., Robin, M.-H., Sarthou, J.-P., and Steinberg, C. (2020). “Vers une protection agroécologique des cultures en phase d'implantation,” in Réussir l'implantation des cultures, 107–134.
Barros, J. F. C., de Carvalho, M., and Basch, G. (2004). Response of sunflower (Helianthus annuus L.) to sowing date and plant density under Mediterranean conditions. Eur. J. Agron. 21, 347–356. doi: 10.1016/j.eja.2003.10.005
Blunk, S., Bussell, J., Sparkes, D., de Heer, M. I., Mooney, S. J., and Sturrock, C. J. (2021). The effects of tillage on seed-soil contact and seedling establishment. Soil Tillage Res. 206, 104757. doi: 10.1016/j.still.2020.104757
Brisson, N., Gary, C., Justes, E., Roche, R., Mary, B., Ripoche, D., et al. (2003). An overview of the crop model STICS. Eur. J. Agron. 18, 309–332. doi: 10.1016/S1161-0301(02)00110-7
Casadebaig, P., Guilioni, L., Lecoeur, J., Christophe, A., Champolivier, L., and Debaeke, P. (2011). SUNFLO, a model to simulate genotype-specific performance of the sunflower crop in contrasting environments. Agric. For. Meteorol. 151, 163–178. doi: 10.1016/j.agrformet.2010.09.012
Celik, A., Altikat, S., and Way, T. R. (2013) Strip tillage width effects on sunflower seed emergence yield. Soil Tillage Res. 131, 20–27. doi: 10.1016/j.still.2013.03.004
Chapman, S. C., and de la Vega, A. J. (2002). Spatial and seasonal effects confounding interpretation of sunflower yields in Argentina. F. Crop. Res. 73, 107–120. doi: 10.1016/S0378-4290(01)00185-X
Chataway, R. G., Cooper, J. E., Orr, W. N., and Cowan, R. T. (2011). The role of tillage, fertiliser and forage species in sustaining dairying based on crops in southern Queensland 2. Double-crop and summer sole-crop systems. Anim. Prod. Sci. 51, 904–919. doi: 10.1071/AN11032
Connor, D. J., and Hall, A. (1997). “Sunflower physiology,” in Sunflower Technology and Production, ed. A. A. Schneiter (Agronomy Monograph 35. ASA, CSSA and SSSA, Madison, WI), 113–182.
Dahal, P., and Bradford, K. J. (1994). Hydrothermal time analysis of tomato seed germination at suboptimal temperature and reduced water potential. Seed Sci. Res. 4, 71–80. doi: 10.1017/S096025850000204X
Debaeke, P., Bedoussac, L., Bonnet, C., Bret-Mestries, E., Seassau, C., Gavaland, A., et al. (2017a). Sunflower crop: environmental-friendly and agroecological. OCL 24, 12. doi: 10.1051/ocl/2017020
Debaeke, P., Casadebaig, P., Flenet, F., and Langlade, N. (2017b). Sunflower crop and climate change: vulnerability, adaptation, and mitigation potential from case-studies in Europe. OCL 24, 15. doi: 10.1051/ocl/2016052
Debaeke, P., Casadebaig, P., and Langlade, N. (2021). New challenges for sunflower ideotyping in changing environments and more ecological cropping systems. OCL 28, 29. doi: 10.1051/ocl/2021016
Dürr, C., and Aubertot, J.-N. (2000). Emergence of seedlings of sugar beet (Beta vulgaris L.) as affected by the size, roughness and position of aggregates in the seedbed. Plant Soil 219, 211–220. doi: 10.1023/A:1004723901989
Dürr, C., Aubertot, J. N., Richard, G., Dubrulle, P., Duval, Y., and Boiffin, J. (2001). SIMPLE: a model for SIMulation of PLant Emergence predicting the effects of soil tillage and sowing operations. Soil Sci. Soc. Am. J. 65, 414–442. doi: 10.2136/sssaj2001.652414x
Dürr, C., and Boiffin, J. (1995). Sugarbeet seedling growth from germination to first leaf stage. J. Agric. Sci. 124, 427–435. doi: 10.1017/S002185960007338X
Dürr, C., Constantin, J., Wagner, M.-H., Navier, H., Demilly, D., Goertz, S., et al. (2016). Virtual modeling based on deep phenotyping provides complementary data to field experiments to predict plant emergence in oilseed rape genotypes. Eur. J. Agron. 79, 90–99. doi: 10.1016/j.eja.2016.06.001
Finch-Savage, W. E., Steckel, J. R. A., and Phelps, K. (1998). Germination and post-germination growth to carrot seedling emergence: predictive threshold models and sources of variation between sowing occasions. New Phytol. 139, 505–516. doi: 10.1046/j.1469-8137.1998.00208.x
Firake, D. M., Behere, G. T., and Chandra, S. (2016). An environmentally benign and cost-effective technique for reducing bird damage to sprouting soybean seeds. F. Crop. Res. 188, 74–81. doi: 10.1016/j.fcr.2016.01.008
Furlan, L., Contiero, B., Chiarini, F., Bottazzo, M., and Milosavljević, I. (2021). Risk factors and strategies for integrated management of bird pests affecting maize establishment. Crop Prot. 2021, 105744. doi: 10.1016/j.cropro.2021.105744
Gallardo-Carrera, A., Léonard, J., Duval, Y., and Dürr, C. (2007). Effects of seedbed structure and water content at sowing on the development of soil surface crusting under rainfall. Soil Tillage Res. 95, 207–217. doi: 10.1016/j.still.2007.01.001
Gardarin, A., Coste, F., Wagner, M.-H., and Dürr, C. (2016). How do seed and seedling traits influence germination and emergence parameters in crop species? A comparative analysis. Seed Sci. Res. 26, 317–331. doi: 10.1017/S0960258516000210
Gay, C., Corbineau, F., and Côme, D. (1991). Effects of temperature and oxygen on seed germination and seedling growth in sunflower (Helianthus annuus L.). Environ. Exp. Bot. 31, 193–200. doi: 10.1016/0098-8472(91)90070-5
González Belo, R., Tognetti, J., Benech-Arnold, R., and Izquierdo, N. G. (2014). Germination responses to temperature and water potential as affected by seed oil composition in sunflower. Ind. Crops Prod. 62, 537–544. doi: 10.1016/j.indcrop.2014.09.029
Graß, R., Thies, B., Kersebaum, K.-C., and Wachendorf, M. (2015). Simulating dry matter yield of two cropping systems with the simulation model HERMES to evaluate impact of future climate change. Eur. J. Agron. 70, 1–10. doi: 10.1016/j.eja.2015.06.005
Grassini, P., Indaco, G. V., Pereira, M. L., Hall, A. J., and Trápani, N. (2007). Responses to short-term waterlogging during grain filling in sunflower. F. Crop. Res. 101, 352–363. doi: 10.1016/j.fcr.2006.12.009
Gummerson, R. J. (1986). The effect of constant temperatures and osmotic potentials on the germination of sugar beet. J. Exp. Bot. 37, 729–741. doi: 10.1093/jxb/37.6.729
Heaton, E. A., Schulte, L. A., Berti, M., Langeveld, H., Zegada-Lizarazu, W., Parrish, D., et al. (2013). Managing a second-generation crop portfolio through sustainable intensification: examples from the USA and the EU. Biofuels, Bioprod. Biorefining 7, 702–714. doi: 10.1002/bbb.1429
Hexen, R. W., and Boxley, R. F. (1986). Trends in Double Cropping. Washington, DC: Natural Resource Economics Division, Economic Research Service, U.S. Department of Agriculture. Agric. Econ. Rep.
Hocking, P. J., and Steer, B. T. (1989). Effects of seed size, cotyledon removal and nitrogen stress on growth and on yield components of oilseed sunflower. F. Crop. Res. 22, 59–75. doi: 10.1016/0378-4290(89)90089-0
Kaufman, M. R. (1969). Effects of water potential on germination of lettuce, sunflower, and citrus seeds. Can. J. Bot. 47, 1761–1764. doi: 10.1139/b69-253
Lamichhane, J. R. (2020). Parsimonius use of pesticide-treated seeds: an integrated pest management framework. Trends Plant Sci. 25, 1070–1073. doi: 10.1016/j.tplants.2020.08.002
Lamichhane, J. R. (2021a). Editorial—Impact assessment, ecology and management of animal pests affecting field crop establishment: an introduction to the special issue. Crop Prot. 150, 105779. doi: 10.1016/j.cropro.2021.105779
Lamichhane, J. R. (2021b). Post-emergence seedling damage due to vertebrate pests and its impact on soybean establishment. PeerJ. 9, e11106. doi: 10.7717/peerj.11106
Lamichhane, J. R., and Aubertot, J.-N. (2021). Effect of early and conventional sowings on soybean establishment quality, nodulation and early biomass development under inoculation with Rhizoctonia solani. PhytoFrontiersTM. 1, 205–218. doi: 10.1094/PHYTOFR-12-20-0046-R
Lamichhane, J. R., Boiffin, J., Boizard, H., Dürr, C., and Richard, G. (2021). Seedbed structure of major field crops as affected by cropping systems and climate: results of a 15-year field trial. Soil Tillage Res. 206, 104845. doi: 10.1016/j.still.2020.104845
Lamichhane, J. R., Constantin, J., Schoving, C., Maury, P., Debaeke, P., Aubertot, J.-N., et al. (2020a). Analysis of soybean germination, emergence, and prediction of a possible northward establishment of the crop under climate change. Eur. J. Agron. 113, 125972. doi: 10.1016/j.eja.2019.125972
Lamichhane, J. R., You, M. P., Laudinot, V., Barbetti, M. J., and Aubertot, J. N. (2020b). Revisiting sustainability of fungicide seed treatments for field crops. Plant Dis. 104, 610–623. doi: 10.1094/PDIS-06-19-1157-FE
Lecomte, V., Abella, M., and Micheneau, A. (2020). Le tournesol dans les rotations : un avantage dans de multiples contextes. Perspect. Agric. 482, 35–36.
Lecomte, V., and Longueval, C. (2013). Implantation : difficile de trop simplifier en tournesol. Perspect. Agric. 402, 50–54.
Lecomte, V., and Nolot, J.-M. (2011). Place du tournesol dans le système de culture. Innov. Agron. 14, 59–76.
Loose, L. H., Heldwein, A. B., Lucas, D. D. P., Hinnah, F. D., and Bortoluzzi, M. P. (2017). Sunflower emergence and initial growth in soil with water excess. Eng. Agrícola 37, 644–655. doi: 10.1590/1809-4430-eng.agric.v37n4p644-655/2017
Macchia, M., Benvenuti, A., and Baldanzi, M. (1985). “Temperature requirements during germination in sunflower,” in Proc. 11th International Sunflower Conference (Toowoomba, Australia), 93–97.
Maeda, J. A., and Ungaro, M. R. J. (1985). “Study of sunflower “seed” dormancy,” in Proc. 11th International Sunflower Conference (Toowoomba, Australia), 73–79.
Michel, B. E. (1983). Evaluation of the water potentials of solutions of polyethylene glycol 8000 both in the absence and presence of other solutes. Plant Physiol. 72, 66–70. doi: 10.1104/pp.72.1.66
Michel, B. E., and Radcliffe, D. (1995). A computer program relating solute potential to solution composition for five solutes. Agron. J. 87, 126–130. doi: 10.2134/agronj1995.00021962008700010022x
Mwale, S. S., Azam-Ali, S. N., Clark, J. A., Bradley, R. G., and Chatha, M. R. (1994). Effect of temperature on the germination of sunflower (Helianthus annuus L.). Seed Sci. Technol. 22, 565–571.
Mwale, S. S., Hamusimbi, C., and Mwansa, K. (2003). Germination, emergence and growth of sunflower (Helianthus annuus L.) in response to osmotic seed priming. Seed Sci. Technol. 31, 199–206. doi: 10.15258/sst.2003.31.1.21
Orchard, P. W., Jessop, R. S., and So, H. B. (1986). The response of sorghum and sunflower to short-term waterlogging. IV. Water and nutrient uptake effects. Plant Soil 91, 87–100. doi: 10.1007/BF02181821
Ordóñez Fernández, R., González Fernández, P., Giráldez Cervera, J. V., and Perea Torres, F. (2007). Soil properties and crop yields after 21 years of direct drilling trials in southern Spain. Soil Tillage Res. 94, 47–54. doi: 10.1016/j.still.2006.07.003
Raveneau, M. P., Coste, F., Moreau-Valancogne, P., Lejeune-Hénaut, I., and Durr, C. (2011). Pea and bean germination and seedling responses to temperature and water potential. Seed Sci. Res. 21, 205–213. doi: 10.1017/S0960258511000067
Rocha, C. R. M., da Silva, V. N., and Cicero, S. M. (2014). Internal morphology and germination of sunflower seeds. J. Seed Sci. 36, 48–53. doi: 10.1590/S2317-15372014000100006
Sausse, C., Chevalot, A., and Lévy, M. (2021). Hungry birds are a major threat for sunflower seedlings in France. Crop Prot. 2021, 105712. doi: 10.1016/j.cropro.2021.105712
Sausse, C., and Lévy, M. (2021). Bird damage to sunflower: international situation and prospects. OCL 28, 34. doi: 10.1051/ocl/2021020
Schneiter, A. A., and Miller, J. F. (1981). Description of sunflower growth stages. Crop Sci. 21, 901–903. doi: 10.2135/cropsci1981.0011183X002100060024x
Seifert, C. A., and Lobell, D. B. (2015). Response of double cropping suitability to climate change in the United States. Environ. Res. Lett. 10, 24002. doi: 10.1088/1748-9326/10/2/024002
Souty, N., and Rode, C. (1993). Emergence of sugar beet seedlings from under different obstacles. Eur. J. Agron. 2, 213–221. doi: 10.1016/S1161-0301(14)80131-7
Stockle, C. O., Martin, S. A., and Campbell, G. S. (1994). CropSyst, a cropping systems simulation model: water/nitrogen budgets and crop yield. Agric. Syst. 46, 335–359. doi: 10.1016/0308-521X(94)90006-2
TerresInovia (2020). Guide de culture: Tournesol. Available online at: https://www.terresinovia.fr/p/guide-tournesol (accessed January 13, 2022).
Torres, M., and Martinez-Honduvilla, C. J. (1990). Effect of seed size on the germination and vigour of sunflower. Agric. Mediterr. 120, 220–225.
Toscano, S., Romano, D., Tribulato, A., and Patanè, C. (2017) Effects of drought stress on seed germination of ornamental sunflowers. Acta Physiol. Plant. 39 184. doi: 10.1007/s11738-017-2484-8
Tribouillois, H., Dürr, C., Demilly, D., Wagner, M. H., and Justes, E. (2016). Determination of germination response to temperature and water potential for a wide range of cover crop species and related functional groups. PLoS ONE. 11, e0161185. doi: 10.1371/journal.pone.0161185
Trudgill, D. L., Squire, G. R., and Thompson, K. (2000). A thermal time basis for comparing the germination requirements of some British herbaceous plants. New Phytol. 145, 107–114. doi: 10.1046/j.1469-8137.2000.00554.x
Villalobos, F. J., Hall, A. J., Ritchie, J. T., and Orgaz, F. (1996). OILCROP-SUN: a development, growth, and yield model of the sunflower crop. Agron. J. 88, 403–415. doi: 10.2134/agronj1996.00021962008800030008x
Villalobos, F. J., Sadras, V. O., Soriano, A., and Fereres, E. (1994). Planting density effects on dry matter partitioning and productivity of sunflower hybrids. F. Crop. Res. 36, 1–11. doi: 10.1016/0378-4290(94)90047-7
Yasumoto, S., Terakado, Y., Matsuzaki, M., and Okada, K. (2011). Effects of high water table and short-term flooding on growth, yield, and seed quality of sunflower. Plant Prod. Sci. 14, 233–248. doi: 10.1626/pps.14.233
Keywords: climate change, emergence losses, innovative cropping system, postemergence damage, sustainability
Citation: Lamichhane JR, Wojciechowski A, Bourgeois C and Debaeke P (2022) Genetic Variability for Early Growth Traits in Second Season Sunflower. Front. Agron. 4:822456. doi: 10.3389/fagro.2022.822456
Received: 15 December 2021; Accepted: 28 February 2022;
Published: 31 March 2022.
Edited by:
Eric Justes, Centre de Coopération Internationale en Recherche Agronomique pour le Développement (CIRAD), FranceReviewed by:
Yaosheng Wang, Chinese Academy of Agricultural Sciences (CAAS), ChinaCopyright © 2022 Lamichhane, Wojciechowski, Bourgeois and Debaeke. This is an open-access article distributed under the terms of the Creative Commons Attribution License (CC BY). The use, distribution or reproduction in other forums is permitted, provided the original author(s) and the copyright owner(s) are credited and that the original publication in this journal is cited, in accordance with accepted academic practice. No use, distribution or reproduction is permitted which does not comply with these terms.
*Correspondence: Jay Ram Lamichhane, amF5LXJhbS5sYW1pY2hoYW5lQGlucmFlLmZy
Disclaimer: All claims expressed in this article are solely those of the authors and do not necessarily represent those of their affiliated organizations, or those of the publisher, the editors and the reviewers. Any product that may be evaluated in this article or claim that may be made by its manufacturer is not guaranteed or endorsed by the publisher.
Research integrity at Frontiers
Learn more about the work of our research integrity team to safeguard the quality of each article we publish.