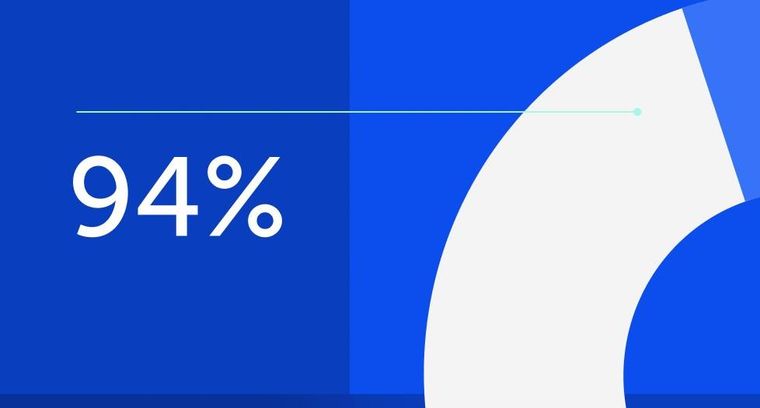
94% of researchers rate our articles as excellent or good
Learn more about the work of our research integrity team to safeguard the quality of each article we publish.
Find out more
PERSPECTIVE article
Front. Agron., 24 May 2022
Sec. Pest Management
Volume 4 - 2022 | https://doi.org/10.3389/fagro.2022.795989
This article is part of the Research TopicCannabis IPM - Insect Pests and DiseasesView all 7 articles
Current biological control strategies in cannabis (Cannabis sativa) cultivation have resulted in poor efficacy for managing certain insect pests. The cannabis industry has grown at a rapid pace, surpassing our ability to develop knowledge on the production systems for this crop. Currently, the research focus is on optimizing agronomic and environmental factors to maximize the yield and quality of cannabis. However, cannabis growers are increasingly challenged by severe insect pest pressure, with few effective options. Decades of research have optimized biological control strategies in other crops. The implementation of effective biological control strategies in cannabis is hindered by a variety of morphological, biochemical, and agronomic factors unique to this crop. Here, we review the rather limited literature relevant to insect pest management in indoor cannabis production. Further, we have identified three factors that we believe are primarily responsible for the ineffectiveness of biological control in cannabis: Plant morphology including trichome density and floral resources, effects of plant biochemistry on prey suitability, and finally the effects of supplemental lighting including photoperiod, intensity, and spectrum. We highlight the importance of prioritizing the evaluation of these factors to improve our understanding of the tritrophic interactions governing the success of biological control in cannabis cultivation. As intensive research efforts are underway to optimize agronomic practices for cannabis, it is also important to consider their relevance to biological control.
In 2018, Canada became the second country to legalize the recreational use of cannabis. Thus, creating a new and rapidly expanding agricultural industry. As of September 2021, licensed cannabis cultivation in Canada exceeded 1.7 million m2 indoors and 8.9 million m2 outdoors (Government of Canada, 2022). The cannabis industry is experiencing a global expansion as additional countries continue to legalize medicinal and recreational uses. Until recently, the cultivation of Cannabis sativa received very little attention from the scientific community resulting in a paucity of reliable information on how to efficiently cultivate cannabis. In fact, within the available cannabis literature, plant science is the least represented scientific discipline (Aliferis and Bernard-Perron, 2020). Hemp1 was prohibited in Canada until 1998 and the USA until 2014 and suffers from a similar lack of reliable scientific knowledge. Hemp is cultivated for industrial fiber, seed for oil and food, and more recently for specialty phytochemicals such as cannabidiol (CBD). It is important to make the distinction between drug-type cannabis (hereafter referred to as cannabis) and industrial hemp due to differences in cultivation methods, secondary metabolites, and end use. However, hemp grown for CBD or other specialty phytochemicals can be similar to cannabis.
A growing body of research is focused on optimizing agronomic practices to improve yield, quality, and the efficiency of cannabis cultivation (Backer et al., 2019). Due to the high value of cannabis and the competitiveness of the industry, cannabis growers have been early adopters of agricultural innovations to improve production efficiency (Owens, 2019). From indoor cultivation in warehouse-style facilities to hybrid greenhouses, growers are implementing new technologies to optimize the environmental parameters desired for cannabis cultivation (e.g., temperature, relative humidity, CO2 concentration, light spectrum and intensity). For example, new and affordable LED (light emitting diodes) technologies have significantly changed the way crops are cultivated indoors (Cohen, 2021). However, the integration of agronomic practices and pest management has been largely ignored. With the absence of insecticides available as rescue treatments, integrated pest management (IPM) strategies must be optimized to prevent pest outbreaks and significant economic losses. The implementation of effective biological control strategies in cannabis is hindered by a variety of morphological, biochemical, and agronomic factors unique to this crop. In this paper, we review key knowledge gaps that may relate to the reduced performance of biological control agents (BCAs) used in IPM programs for insect pests (including mites) in indoor cannabis cultivation. We will first describe the state of insect pest management in cannabis and then focus on how cannabis morphology and its secondary metabolites may present unique challenges for BCAs in cannabis. Finally, as the science and technology of lighting and our understanding of its effects in cannabis cultivation is rapidly changing, we will discuss the impact lighting systems may have on BCAs. This will help shape our understanding of the tritrophic and environmental interactions involved in biological control of insect pests in cannabis that must be understood to ensure growers have reliable pest management solutions.
While more than 270 species of insects and mites have been recorded on C. sativa, few are recorded as economic pests (McPartland, 1996). Nevertheless, indoor cannabis cultivation is challenged by many of the same generalist insect pests as other North American greenhouse crops, including multiple aphid species, western flower thrips (Frankliniella occidentalis Pergande), and two-spotted spider mite (Tetranychus urticae Koch). Additionally, in North America, three insects have emerged as significant pests of cannabis: cannabis aphid (Phorodon cannabis Passerini), hemp russet mite (Aculops cannabicola Farkas), and rice root aphid [Rhopalosiphum rufiabdominale (Sasaki)] (Cranshaw et al., 2018; Lagos-Kutz et al., 2018; Cranshaw and Wainwright-Evans, 2020). The first two species are cannabis specialists, while rice root aphid is a generalist species that is rarely recorded as a pest in other crops. Pest complexes and IPM strategies in hemp are slightly better understood and can be used as a starting point to develop IPM strategies for greenhouse and indoor cannabis cultivation. McPartland et al. (2000) provide a detailed overview of IPM strategies, including pest identification tools, and management recommendations for many insects and pathogens in hemp. With a renewed focus on hemp cultivation in the USA, particularly for the production of phytochemicals, Cranshaw et al. (2019) provide a more recent review of the economically important insects associated with hemp, particularly in Colorado, Virginia, and Tennessee.
Few insecticides have been registered for use on cannabis. In Canada, growers rely on microbial biopesticide (e.g., formulated products containing Beauveria bassiana) as well as insecticidal soaps and horticultural oils. In addition to microbial biopesticides, soaps and oils, many growers rely on biological control to manage insect pests. To date, little research has been conducted on IPM in cannabis, impeding the knowledge transfer required for the development and adoption of effective biological control programs (Murphy, 2014). This lack of knowledge extends to most stakeholders, including researchers, growers, extension agents, and consultants. Notably, there are currently only six peer-reviewed scientific articles that discuss insect pests of indoor cannabis cultivation (Cranshaw et al., 2018; Britt and Kuhar, 2020; Cranshaw and Wainwright-Evans, 2020; Grammenos et al., 2021; McCune et al., 2021; Kostanda and Khatib, 2022). Despite these knowledge gaps, biological control has been rapidly adopted throughout the industry and is often used concurrently with biopesticide programs. Generalist predators, such as Orius insidiosus Say, Chrysoperla carnea (Fitch), aphid parasitoids (e.g., Aphidius ervi Haliday and Aphidius matricariae Haliday), and Phytoseiid mites (e.g., Phytoseiulus persimilis Athias-Henriot and Amblyseius swirskii Athias-Henriot) are commonly used in cannabis cultivation. However, when used in cannabis cultivation, the effectiveness of many BCAs is reduced compared to other crops. Here, we will provide some context as to why BCAs have been less effective in cannabis than in other crops.
The abundance of secondary metabolites produced by cannabis has led to it being called “the plant of one thousand and one molecules” (Andre et al., 2016). Secondary metabolites of interest in cannabis are primarily phytocannabinoids and terpenes. The insecticidal, miticidal, and repellent potential of C. sativa is well-documented in the literature (see McPartland and Sheikh, 2018, for a review). However, secondary metabolites in plants can also affect the third trophic level. Jalali and Michaud (2012) suggest that nicotine, produced by tobacco plants used to rear green peach aphid [M. persicae (Sulzer)] and tobacco aphid (Myzus persicae nicotianae Blackman), decreases the survival and fecundity and increases the development time of the Coccinellid aphid predator Adalia bipunctata L. Multiple instances of Coccinellids rejecting aphids as a result of phytochemicals produced by the host plant have been recorded. For example, A. bipunctata rejected Macrosiphum aconitum vander Goot feeding on Aconitum sp. due to aconitin produced by the host plants (Hodek and Evans, 2012). Additionally, host plants can influence prey suitability regardless of the presence of toxic secondary metabolites. Acyrthosiphum pisum Harris reared on alfalfa (Medicago sativa L.) rather than on faba beans (Vicia faba L.) resulted in better prey for Coccinella septempunctata L., due to greater lipid storage (Giles et al., 2002).
To locate prey, predators and parasitoids use volatile terpenes produced by plants in response to herbivory known as herbivore-induced plant volatiles (HIPV) (Dicke et al., 1990). For example, (E)-β-farnesene (Zhu et al., 1999) and (E)-β-caryophyllene (Sasso et al., 2007) elicit oriented flight behavior and increased landing in parasitoids. These volatile terpenes are also produced by C. sativa in the absence of herbivory. It is possible that the presence of these terpenes interfere with the host finding behavior of parasitoids. It is therefore crucial to determine how volatile secondary metabolites produced by C. sativa influence foraging BCAs.
Microstructures such as trichomes on C. sativa potentially complicate biological control programs. Plant pubescence plays an important role in plant defense against insects (Levin, 1973). Increased densities of glandular trichomes have been found to reduce aphid feeding and infer greater resistance against aphids in various crops including potato (Cho et al., 2017), tomato (Simmons et al., 2003), and melon (Sarria et al., 2010).
Cannabis possesses both glandular and non-glandular trichomes (Ledbetter and Krikorian, 1975). Yet there have been few if any efforts to determine trichome densities across cannabis or hemp genotypes. Trichomes, especially glandular trichomes, are often detrimental to both beneficial and pestivorous insects. For many predatory insects, including some BCAs used in cannabis biological control programs, trichomes were found to do more harm than good (Riddick and Simmons, 2014). However, trichomes are generally beneficial for Phytoseiid mites as they provide refuge from predators, trap additional pollen to be used as a food source, and can facilitate the capture of prey (Schmidt, 2014). Yet, trichomes can also be detrimental to these mites. Notably, Amblyseius andersoni (Chant), prefers glaborous varieties of grapes over pubescent varieties (Camporese and Duso, 1996). Cédola et al. (2001) found that Neoseiulus californicus McGregor, consumed fewer prey on two tomato hybrids with glandular trichomes than reported by Laing and Osborn (1974) on strawberry without glandular trichomes. Finally, Krips et al. (1999) found that at prey densities of 1.3–2.5 eggs/cm2 the predation rate of P. persimilis was negatively affected by trichomes. At prey densities of 8.0 eggs/cm2 no differences between trichome density treatments were observed suggesting that P. persimilis could be less effective at low pest densities in trichome dense crops such as cannabis. Even non-glandular trichomes can reduce the effectiveness of many BCAs, as they struggle to move and find prey on foliage (Cortesero et al., 2000; Stavrinides and Skirvin, 2003; Madadi et al., 2007; Buitenhuis et al., 2014). The aphidophagous midge Aphidoletes aphidimyza was found to preferentially oviposit in areas with denser trichomes, possibly for protection against intra-guild predation (Lucas and Brodeur, 1999).
Few studies have evaluated the consequences of trichome densities on parasitoids. Aphidius colemani was found to have a greater rate of parasitism on a less pubescent variety of chrysanthemum (Soglia et al., 2006). The residency time and searching rate of the whitefly parasitoids Encarsia formosa Gahan and Eretmocerus eremicus (Rose and Zolnerowich), as well as the potato leafhopper parasitoid Anagrus nigriventris Girault have been found to decrease with increasing trichome densities, and this effect appears to be more pronounced in the presence of glandular trichomes (Sütterlin and van Lenteren, 1997; Lovinger et al., 2000; Gruenhagen and Perring, 2001).
In some instance, insects closely associated with a single plant can overcome specific plant defenses, as Eisner et al. (1998) reported for the aphid Macrosiphum mentzeliae Wilson on its highly pubescent host plant Mentzelia pumila Nutt. ex. Torr. & A. Gray. The authors found many insects stuck to the glandular trichomes on M. pumila but no M. mentzeliae. Due to its close association with C. sativa, cannabis aphid has an apparent ability to avoid being trapped by glandular trichomes similar to M. mentzelia on M. pumila. Given the importance of glandular trichomes in the production of secondary metabolites, there is interest for breeding programs to increase trichome density in cannabis (Andre et al., 2016). Identifying BCAs capable of avoiding trichomes could provide significant improvements for the management of cannabis aphid and other pests.
Finally, as cannabis cultivation uses exclusively female plants, there is normally no pollen in cultivation facilities. Furthermore, cannabis is wind pollinated and therefore does not produce nectar. Both pollen and nectar are important resources for maintaining BCA populations in the absence of pests (van Rijn et al., 1999). The absence of supplemental food creates a situation where it may be difficult to maintain inoculative populations of certain BCAs, forcing growers into expensive inundative biological control programs. In other crops, factitious foods such as cattail (Typha latifolia L.) pollen, Ephestia kuehniella Zeller eggs, or Artemia spp. cysts are used to supplement the diets of BCAs when pest populations are low, improving their establishment (Labbé et al., 2018). However, these products can be challenging to use in cannabis as they cannot be applied directly on flowering plants. Alternatively, banker plants can be used to provide resources for certain BCAs such as O. insidiosus and aphids parasitoids in the absence of pests (Brownbridge et al., 2013; Payton Miller and Rebek, 2018). However, banker plant programs require a long-term commitment and technical knowledge to be successfully implemented.
Electric lighting is used for supplemental light in greenhouse cannabis cultivation, and as sole source lighting in warehouse-style cultivation (Zheng, 2021). Additionally, it can be used to control photoperiod (Potter, 2014), increase light intensity (Rodriguez-Morrison et al., 2021) and manipulate light spectrum (Hawley et al., 2018; Magagnini et al., 2018). The manipulation of light intensity and spectrum have become more common with the rapid advancement in LED technologies, especially with the increasing efficacy and affordability of LED fixtures (Cohen, 2021). However, photoperiod, intensity, and spectrum can impact insects (Vänninen et al., 2012). Cannabis cultivation uses a distinct photoperiod for each growth stage: 18–24 h during the propagation and vegetative stages; and 12 h to initiate and maintain the 6–12 weeks flowering stage (Carpentier et al., 2012; Potter, 2014; Yep et al., 2020). Short daylengths (≤ 12 h) induce diapause in many predators, including O. insidiosus (Ruberson et al., 1991) and A. aphidimyza (Boulanger et al., 2019). Additionally, extended photoperiods have been found to increase the parasitism rate of A. ervi (Cochard et al., 2019). Thus, the short photoperiod used during the flowering period can be detrimental to certain BCAs.
Cannabis has been found to benefit from extremely high light intensities (Chandra et al., 2008; Potter and Duncombe, 2012; Eaves et al., 2020; Rodriguez-Morrison et al., 2021). Inflorescence yield was found to increase linearly with light intensity up to photosynthetic photon flux densities of 1,800 μmol·m−2·s−1 (Rodriguez-Morrison et al., 2021). With a 12 h photoperiod, this represents a daily light integral (DLI) of 78 mol·m−2·d−1, which is greater than the outdoor DLI anywhere in North America at any time of the year (Torres and Lopez, 2010). Most research evaluating the effects of light intensity on insects used intensities lower than what is used in cannabis cultivation (<500 μmol·m−2·s−1). Identifying the effects of high light intensities on pests and BCAs will be very important as the general trend is that increasing intensity results in increases to desirable traits for BCAs such as dispersal, host feeding, fecundity, and longevity (Zilahi-Balogh et al., 2006, 2007, 2008; Schirmer et al., 2008; Johansen et al., 2011; Wang et al., 2013; Tan et al., 2014).
LED technologies can provide many different spectra and spectral combinations. Manipulating light spectra is an emerging topic for indoor agriculture as different spectra have different effects on plant morphology, growth, yield, and quality. In cannabis, the potential to manipulate cannabinoids and terpenes through changes in light spectrum has been investigated (Hawley et al., 2018; Magagnini et al., 2018; Wellhoffer, 2020). The effects of different light spectra on BCAs are still not well-understood. Different light spectra are known to affect beneficial insects, including the longevity and fecundity (Labbé and McCreay, 2020), and movement speed (Wang et al., 2013) of Orius, as well as sex ratios (Cochard et al., 2019), locomotor activity (Cochard et al., 2017), and oviposition behavior (Battaglia et al., 2000) of Aphidius wasps. Importantly, Cochard et al. (2019) found that an increase in the proportion of red light resulted in a male biased sex ratio in A. ervi, reaching 80% males at 100% red light. This could have serious impacts on the effectiveness of A. ervi due to a reduction in oviposition. Light spectrum can also have indirect effects on insects through changes to plant secondary metabolite (Lazzarin et al., 2021). For example, light spectra can affect phytohormone mediated plant defenses (El-Esawi et al., 2017; Makowski et al., 2019; Mirzahosseini et al., 2020). Additionally, decreases to the ratio of red to far red light have been found to alter the emission of defensive volatile organic compounds, down regulate induced defenses, and reduce glandular trichome density in tomatoes (Cortés et al., 2016). Manipulating light spectra can also impact other pest management strategies such as sticky card monitoring and mass trapping due to changes in how pests perceive colored traps (Jandricic, 2021). As research is currently focused on optimizing light spectra for cannabis cultivation, it would be advantageous to consider its effects on BCAs, given their necessity as a pest management tool.
Cannabis has morphological and biochemical properties that, in other crops, have been found to reduce the efficacy of many BCAs. Unfortunately, some of these properties, such as dense glandular trichomes, are desirable in cannabis production. Further, as there is considerable effort underway to better understand the agronomic factors that affect the quality and yields of cannabis cultivation, we must consider their effects on the BCAs used to manage pests. Due to the importance and broad adoption of biological control in cannabis, there has never been a more important time to ensure the effectiveness of this management tool. Given the rapid increase and high costs associated with indoor cannabis cultivation, it is imperative that we focus interdisciplinary research efforts into a more systemic approach to crop production and crop protection. Otherwise, focusing solely on crop production could create a situation where decreases in yields are realized due to a lack of effective pest management options. With the seemingly endless number of genotypes cultivated by cannabis growers, breeding is unlikely to provide adequate solutions to these pest problems as market demands are a major driver in selecting cultivars. It is important to note that the factors discussed here are not the only agronomic factors relevant to biological control programs. For example, humidity and air flow are abiotic factors that are known to affect the performance of BCAs (see Prado et al., 2015, for a review of the factors that affect aphid parasitoids). A better understanding of all these tritrophic interactions will ensure that more optimized IPM programs can be deployed across the entire industry. This review has identified three major knowledge gaps associated with the effective use of biological control as part of IPM programs in cannabis. To successfully implement these management options, we must consider the effects of plant morphology, secondary metabolites, lighting, and likely other agronomic factors on the BCAs used to manage insect pests.
The original contributions presented in the study are included in the article/supplementary material, further inquiries can be directed to the corresponding author/s.
JL wrote the manuscript. YZ and CS-D provided critical feedback and contributed to the identification of priority knowledge gaps. All authors contributed to the article and approved the submitted version.
This work was funded through the Ontario Agri-Food Innovation Alliance Research Program (Project Number UG-T1-2021-100999), a joint funding program between the Ontario Ministry of Agriculture, Food, and Rural Affairs and the University of Guelph. Funding for this grant was also provided by Biobest Canada Ltd. and JC Green. Finally, Funding was received from Tilray Canada through Mitacs (Project Number IT17172).
The authors declare that this study received funding from Biobest Canada Ltd, Tilray Canada, and JC Green. The funder was not involved in the study design, collection, analysis, interpretation of data, the writing of this article or the decision to submit it for publication.
The authors declare that the research was conducted in the absence of any commercial or financial relationships that could be construed as a potential conflict of interest.
All claims expressed in this article are solely those of the authors and do not necessarily represent those of their affiliated organizations, or those of the publisher, the editors and the reviewers. Any product that may be evaluated in this article, or claim that may be made by its manufacturer, is not guaranteed or endorsed by the publisher.
We thank Sarah Jandricic and Amy Lemay for their critical review of this manuscript.
1. ^In Canada, like the USA, hemp is legally defined as C. sativa with ≤ 0.3% Δ9-tetrahydrocannabinol (THC) in its foliage and inflorescence [Industrial Hemp Regulations (SOR/2018-145)].
Aliferis, K. A., and Bernard-Perron, D. (2020). Cannabinomics: application of metabolomics in cannabis (Cannabis sativa L.) research and development. Front. Plant Sci. 11, 554. doi: 10.3389/fpls.2020.00554
Andre, C. M., Hausman, J.-F., and Guerriero, G. (2016). Cannabis sativa: the plant of the thousand and one molecules. Front. Plant Sci. 7, 19. doi: 10.3389/fpls.2016.00019
Backer, R., Schwinghamer, T., Rosenbaum, P., McCarty, V., Eichhorn Bilodeau, S., Lyu, D., et al. (2019). Closing the yield gap for cannabis: a meta-analysis of factors determining cannabis yield. Front. Plant Sci. 10, 495. doi: 10.3389/fpls.2019.00495
Battaglia, D., Poppy, G., Powell, W., Romano, A., Tranfaglia, A., and Pennacchio, F. (2000). Physical and chemical cues influencing the oviposition behaviour of Aphidius ervi. Entomol. Exp. Appl. 94, 219–227. doi: 10.1046/j.1570-7458.2000.00623.x
Boulanger, F.-X., Jandricic, S., Bolckmans, K., Wäckers, F. L., and Pekas, A. (2019). Optimizing aphid biocontrol with the predator Aphidoletes aphidimyza, based on biology and ecology. Pest Manag. Sci. 75, 1479–1493. doi: 10.1002/ps.5270
Britt, K. E., and Kuhar, T. P. (2020). Evaluation of miticides to control hemp russet mite on indoor hemp in Virginia, 2019. Arthropod Manag. Tests 45, tsaa082. doi: 10.1093/amt/tsaa082
Brownbridge, M., Buitenhuis, R., Murphy, G., Waite, M., and Scott-Dupree, C. (2013). “Banker plants, trap crops and other bioprotection developments in canadian greenhouse floriculture,” in Proceedings of the 4th International Symposium on Biological Control of Arthropods (Pucón).
Buitenhuis, R., Shipp, L., Scott-Dupree, C., Brommit, A., and Lee, W. (2014). Host plant effects on the behaviour and performance of Amblyseius swirskii (Acari: Phytoseiidae). Exp. Appl. Acarol. 62, 171–180. doi: 10.1007/s10493-013-9735-1
Camporese, P., and Duso, C. (1996). Different colonization patterns of phytophagous and predatory mites (Acari: Tetranychidae, Phytoseiidae) on three grape varieties: a case study. Exp. Appl. Acarol. 20, 1–22. doi: 10.1007/BF00051473
Carpentier, C., Mulligan, K., Laniel, L., Potter, D., Hughes, B., Vandam, L., et al. (2012). “Botany and cultivation of cannabis,” in Cannabis production and markets in Europe, eds C. Carpentier, L. Laniel, and P. Griffiths (Lisban: European Monitoring Centre for Drugs and Drug Addiction), 20–39.
Cédola, C. V., Sánchez, N. E., and Liljesthröm, G. G. (2001). Effect of tomato leaf hairiness on functional and numerical response of Neoseiulus californicus (Acari: Phytoseiidae). Exp. Appl. Acarol. 25, 819–831. doi: 10.1023/A:1020499624661
Chandra, S., Lata, H., Khan, I. A., and Elsohly, M. A. (2008). Photosynthetic response of Cannabis sativa L. to variations in photosynthetic photon flux densities, temperature and CO2 conditions. Physiol. Mol. Biol. Plants 14, 299–306. doi: 10.1007/s12298-008-0027-x
Cho, K.-S., Kwon, M., Cho, J.-H., Im, J.-S., Park, Y.-E., Hong, S.-Y., et al. (2017). Characterization of trichome morphology and aphid resistance in cultivated and wild species of potato. Hortic. Environ. Biotechnol. 58, 450–457. doi: 10.1007/s13580-017-0078-4
Cochard, P., Galstian, T., and Cloutier, C. (2017). Light environments differently affect parasitoid wasps and their hosts' locomotor activity. J. Insect Behav. 30, 595–611. doi: 10.1007/s10905-017-9644-y
Cochard, P., Galstian, T., and Cloutier, C. (2019). The proportion of blue light affects parasitoid wasp behavior in LED-extended photoperiod in greenhouses: increased parasitism and offspring sex ratio bias. Biol. Control 133, 9–17. doi: 10.1016/j.biocontrol.2019.03.004
Cohen, D.. (2021). “The unique challenge of horticultural lighting,” in State of the Cannabis Lighting Market: Six Years of Research (Cannabis Business Times), 16. Available online at: https://fluence.science/state-of-the-cannabis-lighting-market-2021/ (accessed October 21, 2021).
Cortés, L. E., Weldegergis, B. T., Boccalandro, H. E., Dicke, M., and Ballaré, C. L. (2016). Trading direct for indirect defense? Phytochrome B inactivation in tomato attenuates direct anti-herbivore defenses whilst enhancing volatile-mediated attraction of predators. New Phytol. 212, 1057–1071. doi: 10.1111/nph.14210
Cortesero, A.-M., Stapel, J. O., and Lewis, W. J. (2000). Understanding and manipulating plant attributes to enhance biological control. Biol. Control 17, 35–49. doi: 10.1006/bcon.1999.0777
Cranshaw, W., Halbert, S., Favret, C., Britt, K., and Miller, G. (2018). Phorodon cannabis Passerini (Hemiptera: Aphididae), a newly recognized pest in North America found on industrial hemp. Insecta mundi 0662, 1–12.
Cranshaw, W., Schreiner, M., Britt, K., Kuhar, T., McPartland, J., and Grant, J. (2019). Developing insect pest management systems for hemp in the United States: a work in progress. J. Integr. Pest Manag. 10, 26. doi: 10.1093/jipm/pmz023
Cranshaw, W., and Wainwright-Evans, S. (2020). Cannabis sativa as a host of rice root aphid (Hemiptera: Aphididae) in North America. J. Integr. Pest Manag. 11, 15. doi: 10.1093/jipm/pmaa008
Dicke, M., Sabelis, M. W., Takabayashi, J., Bruin, J., and Posthumus, M. A. (1990). Plant strategies of manipulating predatorprey interactions through allelochemicals: prospects for application in pest control. J. Chem. Ecol. 16, 3091–3118.
Eaves, J., Eaves, S., Morphy, C., and Murray, C. (2020). The relationship between light intensity, cannabis yields, and profitability. Agron. J. 112, 1466–1470. doi: 10.1002/agj2.20008
Eisner, T., Eisner, M., and Hoebeke, E. R. (1998). When defense backfires: detrimental effect of a plant's protective trichomes on an insect beneficial to the plant. Proc. Natl. Acad. Sci. U.S.A. 95, 4410–4414. doi: 10.1073/pnas.95.8.4410
El-Esawi, M., Arthaut, L.-D., Jourdan, N., d'Harlingue, A., Link, J., Martino, C. F., et al. (2017). Blue-light induced biosynthesis of ROS contributes to the signaling mechanism of Arabidopsis cryptochrome. Sci. Rep. 7, 13875. doi: 10.1038/s41598-017-13832-z
Giles, K. L., Madden, R. D., Stockland, R., Payton, M. E., and Dillwith, J. W. (2002). Host plants affect predator fitness via the nutritional value of herbivore prey: investigation of a plant-aphid-ladybeetle system. Biocontrol 47, 1–21. doi: 10.1023/A:1014419623501
Government of Canada (2022). Cannabis Licensed Area Market Data. Available online at: https://www.canada.ca/en/health-canada/services/drugs-medication/cannabis/research-data/market/licensed-area.html#tbl-1 (accessed April 21, 2022).
Grammenos, G., Kouneli, V., Mavroeidis, A., Roussis, I., Kakabouki, I., Tataridas, A., et al. (2021). Beneficial insects for biological pest control in greenhouse Cannabis production. Bull. Univ. Agric. Sci. Vet. Med. Cluj-Napoca. Hortic. 72, 85–93. Available online at: https://doi.org/15835/buasvmcn-hort:2021.0037
Gruenhagen, N. M., and Perring, T. M. (2001). Impact of leaf trichomes on parasitoid behavior and parasitism of silverleaf whiteflies (Homoptera: Aleyrodidae). Southwest. Entomol. 26, 279–290.
Hawley, D., Graham, T., Stasiak, M., and Dixon, M. (2018). Improving Cannabis bud quality and yield with subcanopy lighting. HortScience Horts 53, 1593–1599. doi: 10.21273/HORTSCI13173-18
Hodek, I., and Evans, E. W. (2012). “Food relationships,” in Ecology and Behaviour of the Ladybird Beetles (Coccinellidae) Wiley Online Books, eds I. Hodek, H. F. van Emden, and A. Honěk (Chichester: Blackwell Publishing Ltd), 141–274.
Industrial Hemp Regulations (SOR/2018-145). (2018). Available online at: https://laws-lois.justice.gc.ca/eng/regulations/SOR-2018-145/page-1.html#h-851200 (Accessed October 08, 2021).
Jalali, M. A., and Michaud, J. P. (2012). Aphid-plant interactions affect the suitability of Myzus spp. as prey for the two spot ladybird, Adalia bipunctata (Coleoptera: Coccinellidae). Eur. J. Entomol. 109, 345–352. doi: 10.14411/eje.2012.045
Jandricic, S.. (2021). What LED Lighting Means for Pest Monitoring. Greenhouse. Available online at: https://mydigitalpublication.com/publication/?m=1281&i=721730&p=12&ver=html5 (accessed October 08, 2021)
Johansen, N. S., Vänninen, I., Pinto, D. M., Nissinen, A. I., and Shipp, L. (2011). In the light of new greenhouse technologies: 2. Direct effects of artificial lighting on arthropods and integrated pest management in greenhouse crops. Ann. Appl. Biol. 159, 1–27. doi: 10.1111/j.1744-7348.2011.00483.x
Kostanda, E., and Khatib, S. (2022). Biotic stress caused by Tetranychus urticae mites elevates the quantity of secondary metabolites, Cannabinoids and Terpenes, in Cannabis sativa L. Ind. Crops Prod. 176, 114331. doi: 10.1016/j.indcrop.2021.114331
Krips, O. E., Kleijn, P. W., Willems, P. E. L., Gols, G. J. Z., and Dicke, M. (1999). Leaf hairs influence searching efficiency and predation rate of the predatory mite Phytoseiulus persimilis (Acari: Phytoseiidae). Ecol. Evol. Acari 23, 119–131. doi: 10.1023/A:1006098410165
Labbé, R. M., Gagnier, D., Kostic, A., and Shipp, L. (2018). The function of supplemental foods for improved crop establishment of generalist predators Orius insidiosus and Dicyphus hesperus. Sci. Rep. 8, 17790. doi: 10.1038/s41598-018-36100-0
Labbé, R. M., and McCreay, C. (2020). Arthropods and Greenhouse Lighting: Like Moths to a Flame. Greenhouse. Available online at: https://www.greenhousecanada.com/arthropods-and-greenhouse-lighting-like-moths-to-a-flame/ (accessed October 04, 2021).
Lagos-Kutz, D., Potter, B., DiFonzo, C., Russell, H., and Hartman, G. L. (2018). Two Aphid species, Phorodon cannabis and Rhopalosiphum rufiabdominale, identified as potential pests on industrial hemp, Cannabis sativa L., in the US Midwest. Crop. Forage Turfgrass Manag. 4, 180032. doi: 10.2134/cftm2018.04.0032
Laing, J. E., and Osborn, J. A. L. (1974). The effect of prey density on the functional and numerical reponses of three species of predatory mites. Entomophaga 19, 267–277. doi: 10.1007/BF02371052
Lazzarin, M., Meisenburg, M., Meijer, D., van Ieperen, W., Marcelis, L. F. M., Kappers, I. F., et al. (2021). LEDs make it resilient: effects on plant growth and defense. Trends Plant Sci. 26, 496–508. doi: 10.1016/j.tplants.2020.11.013
Ledbetter, M. C., and Krikorian, A. D. (1975). Trichomes of Cannabis sativa as viewed with scanning electron microscope. Phytomorphology 25, 166–176.
Levin, D. A.. (1973). The role of trichomes in plant defense. Q. Rev. Biol. 48(Part 1), 3–15. doi: 10.1086/407484
Lovinger, A., Liewehr, D., and Lamp, W. O. (2000). Glandular trichomes on alfalfa impede searching behavior of the potato leafhopper parasitoid. Biol. Control 18, 187–192. doi: 10.1006/bcon.2000.0835
Lucas, É., and Brodeur, J. (1999). Oviposition site selection by the predatory midge Aphidoletes aphidimyza (Diptera: Cecidomyiidae). Environ. Entomol. 28, 622–627.
Madadi, H., Enkegaard, A., Brodsgaard, H. F., Kharrazi-Pakdel, A., Mohaghegh, J., and Ashouri, A. (2007). Host plant effects on the functional response of Neoseiulus cucumeris to onion thrips larvae. J. Appl. Entomol. 131, 728–733. doi: 10.1111/j.1439-0418.2007.01206.x
Magagnini, G., Grassi, G., and Kotiranta, S. (2018). The effect of light spectrum on the morphology and cannabinoid content of Cannabis sativa L. Med. Cannabis Cannabinoids 1, 19–27. doi: 10.1159/000489030
Makowski, W., Tokarz, B., Banasiuk, R., Królicka, A., Dziurka, M., Wojciechowska, R., et al. (2019). Is a blue–red light a good elicitor of phenolic compounds in the family droseraceae? A Comparative Study. J. Photochem. Photobiol. B Biol. 201, 111679. doi: 10.1016/j.jphotobiol.2019.111679
McCune, F., Murphy, C., Eaves, J., and Fournier, V. (2021). Onion thrips, Thrips tabaci (Thysanoptera: Thripidae), reduces yields in indoor-grown cannabis. Phytoprotection 101, 14–20. doi: 10.7202/1076365ar
McPartland, J., Clarke, R. C., and Watson, D. P. (2000). Hemp Diseases and Pests: Management and Biological Control: An Advanced Treatise. Wallingford: CABI Publishing.
McPartland, J., and Sheikh, Z. (2018). A review of Cannabis sativa-based insecticides, miticides, and repellents. J. Entomol. Zool. Stud. 6, 1288–1299.
Mirzahosseini, Z., Shabani, L., Sabzalian, M. R., and Dayanandan, S. (2020). Comparative physiological and proteomic analysis of Arabidopsis thaliana revealed differential wound stress responses following the exposure to different LED light sources. Environ. Exp. Bot. 169, 103895. doi: 10.1016/j.envexpbot.2019.103895
Murphy, G.. (2014). Grower adoption of biological control in greenhouse ornamentals and the role of technology transfer. Integr. Control Prot. Crop. Temp. Clim. IOBC-WPRS Bull. 102, 163–167.
Owens, B.. (2019). The professionalization of cannabis growing. Nature 572, S10–S10. doi: 10.1038/d41586-019-02527-2
Payton Miller, T. L., and Rebek, E. J. (2018). Banker plants for aphid biological control in greenhouses. J. Integr. Pest Manag. 9, 9. doi: 10.1093/jipm/pmy002
Potter, D. J.. (2014). A review of the cultivation and processing of cannabis (Cannabis sativa L.) for production of prescription medicines in the UK. Drug Test. Anal. 6, 31–38. doi: 10.1002/dta.1531
Potter, D. J., and Duncombe, P. (2012). The effect of electrical lighting power and irradiance on indoor-grown cannabis potency and yield. J. Forensic Sci. 57, 618–622. doi: 10.1111/j.1556-4029.2011.02024.x
Prado, S. G., Jandricic, S., and Frank, S. D. (2015). Ecological interactions affecting the efficacy of Aphidius colemani in greenhouse crops. Insects 6, 538–575. doi: 10.3390/insects6020538
Riddick, E. W., and Simmons, A. M. (2014). Do plant trichomes cause more harm than good to predatory insects? Pest Manag. Sci. 70, 1655–1665. doi: 10.1002/ps.3772
Rodriguez-Morrison, V., Llewellyn, D., and Zheng, Y. (2021). Cannabis yield, potency, and leaf photosynthesis respond differently to increasing light levels in an indoor environment. Front. Plant Sci. 12:646020. doi: 10.3389/fpls.2021.646020
Ruberson, J. R., Bush, L., and Kring, T. J. (1991). Photoperiodic effect on diapause induction and development in the predator Orius insidiosus (Heteroptera: Anthocoridae). Environ. Entomol. 20, 786–789. doi: 10.1093/ee/20.3.786
Sarria, E., Palomares-Rius, F. J., Lopez-Sese, A. I., Heredia, A., and Gomez-Guillamon, M. L. (2010). Role of leaf glandular trichomes of melon plants in deterrence of Aphis gossypii glover. Plant Biol. 12, 503–511. doi: 10.1111/j.1438-8677.2009.00239.x
Sasso, R., Iodice, L., Cristina Digilio, M., Carretta, A., Ariati, L., and Guerrieri, E. (2007). Host-locating response by the aphid parasitoid Aphidius ervi to tomato plant volatiles. J. Plant Interact. 2, 175–183. doi: 10.1080/17429140701591951
Schirmer, S., Sengonca, C., and Blaeser, P. (2008). Influence of abiotic factors on some biological and ecological characteristics of the aphid parasitoid Aphelinus asychis (Hymenoptera: Aphelinidae) parasitizing Aphis gossypii (Sternorrhyncha: Aphididae). Eur. J. Entomol. 105, 121. doi: 10.14411/eje.2008.017
Schmidt, R. A.. (2014). Leaf structures affect predatory mites (Acari: Phytoseiidae) and biological control: a review. Exp. Appl. Acarol. 62, 1–17. doi: 10.1007/s10493-013-9730-6
Simmons, A. T., Gurr, G. M., McGrath, D., Nicol, H. I., and Martin, P. M. (2003). Trichomes of Lycopersicon spp. and their effect on Myzus persicae (Sulzer) (Hemiptera: Aphididae). Aust. J. Entomol. 42, 373–378. doi: 10.1046/j.1440-6055.2003.00376.x
Soglia, N. C. M., Bueno, V. H. P., Sampaio, M. V., Rodrigues, S. M. M., and Ledo, C. A. S. (2006). Development and parasitism of Lysiphlebus testaceipes (Cresson) and Aphidius colemani Viereck (Hymenoptera: Braconidae) on Aphis gossypii glover (Hemiptera: Aphididae) on two chrysanthemum cultivars. Neotrop. Entomol. 35, 364–370. doi: 10.1590/S1519-566X2006000300011
Stavrinides, M. C., and Skirvin, D. J. (2003). The effect of chrysanthemum leaf trichome density and prey spatial distribution on predation of Tetranychus urticae (Acari: Tetranychidae) by Phytoseiulus persimilis (Acari: Phytoseiidae). Bull. Entomol. Res. 93, 343–350. doi: 10.1079/ber2003243
Sütterlin, S., and van Lenteren, J. C. (1997). Influence of hairiness of Gerbera jamesonii leaves on the searching efficiency of the parasitoid Encarsia formosa. Biol. Control 9, 157–165. doi: 10.1006/bcon.1997.0530
Tan, X., Zhao, J., Zhang, F., and Wang, S. (2014). Light dependency of life trails, reproduction, locomotion, and predation in the polyphagous ladybird Hippodamia variegata. Entomol. Exp. Appl. 152, 200–208. doi: 10.1111/eea.12217
Torres, A. P., and Lopez, R. G. (2010). HO-238-W Measuring Daily Light Integral in a Greenhouse Commercial Greenhouse Production Purdue Extension What Is Light and Why Is It Important? Available online at: https://www.extension.purdue.edu/extmedia/ho/ho-238-b-w.pdf (accessed February 22, 2021).
van Rijn, P. C. J., Van Houten, Y. M., and Sabelis, M. W. (1999). Pollen improves thrips control with predatory mites. IOBC/WPRS Bull. 22, 209–212.
Vänninen, I., Pinto, D. M., Nissinen, A. I., Johansen, N. S., and Shipp, L. (2012). “Prospecting the use of artificial lighting for integrated pest management, in Acta Horticulturae [Leuven: International Society for Horticultural Science (ISHS)], 593–608.
Wang, S., Tan, X. L., Michaud, J. P., Zhang, F., and Guo, X. (2013). Light intensity and wavelength influence development, reproduction and locomotor activity in the predatory flower bug Orius sauteri (Poppius) (Hemiptera: Anthocoridae). BioControl 58, 667–674. doi: 10.1007/s10526-013-9522-2
Wellhoffer, J.. (2020). The effects of light spectra on the growth and development of greenhouse cbd hemp (Master's thesis). Cornell University, Ithaca, NY, United States. Available online at: https://ecommons.cornell.edu/bitstream/handle/1813/70206/Wellhofer_John.pdf?sequence=1
Yep, B., Gale, N. V., and Zheng, Y. (2020). Comparing hydroponic and aquaponic rootzones on the growth of two drug-type Cannabis sativa L. cultivars during the flowering stage. Ind. Crops Prod. 157, 112881. doi: 10.1016/j.indcrop.2020.112881
Zheng, Y.. (2021). “Soilless production of drug-type Cannabis sativa,” in Acta Horticulturae [Leuven: International Society for Horticultural Science (ISHS)], 375–382.
Zhu, J., Cossé, A. A., Obrycki, J. J., Boo, K. S., and Baker, T. C. (1999). Olfactory reactions of the twelve-spotted lady beetle, Coleomegilla maculata and the green lacewing, Chrysoperla carnea to semiochemicals released from their prey and host plant: electroantennogram and behavioral responses. J. Chem. Ecol. 25, 1163–1177. doi: 10.1023/A:1020846212465
Zilahi-Balogh, G., Shipp, L., Cloutier, C., and Brodeur, J. (2006). Influence of light intensity, photoperiod, and temperature on the efficacy of two aphelinid parasitoids of the greenhouse whitefly. Environ. Entomol. 35, 581–589. doi: 10.1603/0046-225X-35.3.581
Zilahi-Balogh, G., Shipp, L., Cloutier, C., and Brodeur, J. (2007). Predation by Neoseiulus cucumeris on western flower thrips, and its oviposition on greenhouse cucumber under winter vs. summer conditions in a temperate climate. Biol. Control 40, 160–167. doi: 10.1016/j.biocontrol.2006.10.011
Zilahi-Balogh, G., Shipp, L., Cloutier, C., and Brodeur, J. (2008). Comparison of searching behaviour of two aphelinid parasitoids of the greenhouse whitefly, Trialeurodes vaporariorum under summer vs. winter conditions in a temperate climate. J. Insect Behav. 22, 134–147. doi: 10.1007/s10905-008-9160-1
Keywords: Cannabis sativa, biological control, secondary metabolites, trichomes, lighting, tritrophic interactions
Citation: Lemay J, Zheng Y and Scott-Dupree C (2022) Factors Influencing the Efficacy of Biological Control Agents Used to Manage Insect Pests in Indoor Cannabis (Cannabis sativa) Cultivation. Front. Agron. 4:795989. doi: 10.3389/fagro.2022.795989
Received: 15 October 2021; Accepted: 29 April 2022;
Published: 24 May 2022.
Edited by:
Murray B. Isman, University of British Columbia, CanadaReviewed by:
Puja Ray, Presidency University, IndiaCopyright © 2022 Lemay, Zheng and Scott-Dupree. This is an open-access article distributed under the terms of the Creative Commons Attribution License (CC BY). The use, distribution or reproduction in other forums is permitted, provided the original author(s) and the copyright owner(s) are credited and that the original publication in this journal is cited, in accordance with accepted academic practice. No use, distribution or reproduction is permitted which does not comply with these terms.
*Correspondence: Jason Lemay, amxlbWF5QHVvZ3VlbHBoLmNh
Disclaimer: All claims expressed in this article are solely those of the authors and do not necessarily represent those of their affiliated organizations, or those of the publisher, the editors and the reviewers. Any product that may be evaluated in this article or claim that may be made by its manufacturer is not guaranteed or endorsed by the publisher.
Research integrity at Frontiers
Learn more about the work of our research integrity team to safeguard the quality of each article we publish.