- 1Department of Crop, Soil and Environmental Sciences, Auburn University, Auburn, AL, United States
- 2National Soil Dynamics Laboratory, Agricultural Research Service, United States Department of Agriculture, Auburn, AL, United States
- 3University of Ioannina, Árta, Greece
An increasing number of herbicide-resistant weeds, in addition to troublesome weeds, pose a significant challenge for chemical weed control in corn. Simultaneously, high-biomass cover crop adoption has gained popularity among farmers as an efficient weed control strategy. While the critical period of weed control (CPWC) following conventional tillage has been well documented, there is little knowledge of CPWC following high residue cover crops in corn. A two-year field experiment was conducted to estimate the influence of a high biomass crimson clover cover crop and conservation tillage on the critical period of weed control (CPWC) in corn. The experiment was implemented in a split-plot design in which the main plots were conventional tillage (CVT), conservation tillage following winter fallow (CT + WF), and conservation tillage following crimson clover (CT + CC), and the subplot included multiple durations of weedy plots (estimation of critical timing of weed removal (CTWR), i.e., beginning of weed control) and weed-free plots (estimation of critical weed-free period (CWFP), i.e., end of weed control). The results described that the estimated duration of CPWC in three systems, included CT + CC, CT + WF and CVT equals 2.8 weeks, 3.5 weeks, and 4.9 weeks respectively in 2019. In 2020, the predicted value of CTWR under CT + CC equals 3.8 weeks after planting and the predicted values of CWFP were 5.1 and 5.7 weeks after planting under CT + WF and CVT systems, however, the model did not predict some values within the fitted 8 weeks of time. In conclusion, the presence of a crimson clover cover crop delayed the CTWR and caused the early beginning of CWFP and hence shortened CPWC in 2019. During most of the growing season, weed biomass production was less under CT + CC plots than CVT and CT + WF systems of weedy treatment in both years. While weed biomass production fluctuated in CT + CC, CVT and CT + WF systems in weed-free treatment.
1 Introduction
Corn (Zea mays L.) is one of the major grain crops cultivated worldwide, with the U.S. leading production globally. Corn has extensive uses, including food products and cooking oil, animal feed, industrial purposes, and ethanol production. Since the late 90s, potential corn yield losses have been increasing due to weed competition from herbicide-resistant and troublesome weed species (Chandler et al., 1984; Vissoh et al., 2004). Integrated weed management approach included the utilization of diverse herbicide modes of action and cover crops to decrease the selection pressure of herbicide resistance and control of glyphosate-resistant Palmer amaranth (Amaranthus palmeri) in corn (Wiggins et al., 2015). Therefore, the understanding of innovative strategies that reduce growers’ reliance on herbicide should be adopted for increased weed control continues to be important. Best management practices to sustain or increase weed control included cultural, mechanical, and biological practices illustrated in the “Herbicide Resistant Weeds” section (Norsworthy et al., 2012). In the southeastern U.S., the adoption of conservation tillage utilizing high residue cover crops is increasing in corn and cotton (Gossypium hirsutum L.) production systems due to numerous advantages (Price et al., 2006; Price and Kelton, 2013; Reeves et al., 2005). Among other benefits, cover crops improve soil organic matter, nutrient cycling, and soil water conservation (Holderbaum et al., 1990; Sainju and Singh, 1997; Kaspar et al., 2001). Cover crops, including legumes, inhibit weed seed germination and seedling growth due to physical suppression and through allelopathic properties (Barnes and Putnam, 1983; Chase et al., 1991; Akemo et al., 2000; Teasdale and Mohler, 2000; Price et al., 2006; Price et al., 2008). Moreover, cover crops can also improve the soil’s physical, chemical, and biological properties by increasing the soil organic matter content in case of grass cover crops with a higher C:N ratio and, nitrogen availability in case of leguminous cover crop species (Hubbard et al., 2013; Romdhane et al., 2019). The crimson clover (Trifolium incarnatum) contained N is an essential source of nitrogen for the succeeding crops. However, the rate of N disappearance was more rapid in conventional tillage than no-tillage system (Wilson and Hargrove, 1986). A study in Alabama suggested that conservation tillage with the utilization of crimson clover decreased the weed biomass and suppress the germination of early season weed species in corn. Further, lowest weed biomass recorded was 36 kg ha-1 corresponding to crimson clover biomass of 2453 kg ha-1 and the highest was 158 kg ha-1 corresponding to crimson clover biomass of 373 kg ha-1 (Saini et al., 2006). Hence, with the utilization of crimson clover in conservation tillage, it is necessary to establish the critical period of weed control (CPWC) parameters in an integrated weed management system to further understand cover crop weed suppressive attributes and efficient utilization of chemical herbicides (Swanton and Weise, 1991). Moreover, CPWC information is necessary and can be valuable in making decisions based on the need and timing of weed management (Hall et al., 1992; Van Acker et al., 1993). Also, cover crop seeding, and cultivation timing could be improved based upon CPWC knowledge.
The critical period of weed control (CPWC) is described as a ‘window’ of weed competition period during the crop growing season in which it is essential to control weeds to maintain crop potential yield (Swanton and Weise, 1991). CPWC has two independent components, including critical timing of weed removal (CTWR), which defines the beginning of the critical period from which weeds must be controlled and the maximum tolerance of the crop to the early emerging weeds without causing any unacceptable yield loss (>5%). While the critical weed-free period (CWFP) describes the end of weed control, to prevent considerable potential yield losses by late-emerging weeds (Knezevic et al., 2002; Williams et al., 2007; Korres and Norsworthy, 2015; Price et al., 2018). Thus, the weed interference duration in weedy plots represented CTWR and the weed-free duration in weed-free plots represented CWFP, with both parameters’ length defined by 5% yield loss. Ultimately, weedy plots represented CTWR (beginning of weed control) and weed-free plots represented CWFP (end of weed control) and difference of CWFP and CTWR described the duration of CPWC.
The objective of this research was to evaluate the effect of a high residue crimson clover (Trifolium incarnatum) on the critical period of weed control in corn. Therefore, a field study was performed comparing a conservation tillage system with a clover cover crop (CT + CC) managed for maximum biomass, a conservation tillage system with winter fallow (CT + WF), and a conventional tillage (CVT) system on the CPWC.
2 Materials and methods
2.1 Location site
Field experiments were conducted in 2019 and 2020 at the E.V. Smith Research Center Field Crops Unit (32.4417° N, 85.8974° W) Shorter, Alabama. The soil characteristics at the research site were sandy loam, (coarse-loamy, siliceous, sub-active, thermic Paleudults) with pH 6.2 and 0.8% organic matter. The average temperature ranged from 18.1°C to 27.6°C and precipitation was 8.26 mm to 1.25 mm from April to August 2019. In 2020, the average temperature ranged from 17.27°C to 26.98°C and precipitation was 2.03 mm to 3.37 mm from April to August.
2.2 Experimental design
The study was conducted in a split-plot design with four replications. As previously stated, the three systems i.e., conservation tillage with a crimson clover cover crop (CT + CC), conservation tillage with winter fallow (CT + WF), and conventional tillage (CVT), were considered in the main plots. The durations of weedy plots described the beginning of weed removal (CTWR), and the durations of weed-free plots illustrated the end of weed control (CWFP). Hence, these durations in weedy and weed-free plots from 0 to 8 weeks after planting were considered in subplots.
2.3 Cover crop management and corn establishment
Crimson clover cultivar “Dixie” was seeded at a rate of 22.4 kg ha-1 using a grain drill. Termination of crimson clover was accomplished using a roller-crimper (Ashford and Reeves, 2003) followed by an application of glyphosate (Roundup Powermax®, Monsanto Company, St. Louis, MO) plus glufosinate (Liberty®, Bayer Crop Science, Research Triangle Park, NC) herbicides sprayed at the rate of 841 g ae ha-1 and 492 g ae ha-1 respectively. Within all plots, a KMC 4-row parabolic subsoiler (Kelly Manufacturing Company, Tifton, GA) was used to disrupt naturally occurring hard pans found at this location before planting corn in all treatments to prevent deep-tillage interaction. Subsequently, CVT plots were cultivated using three disks, and two field cultivator passes. Corn (Pioneer® 1197 YHR) was planted using a precision planter with the population set at 12950 seeds ha-1 on April 16, 2019, and April 27, 2020, respectively. A starter application of nitrogen, phosphorus, and potassium (NPK) fertilizer was applied at a rate of 45 kg ha-1 after planting corn. A tank mixture of glyphosate plus acetochlor (Warrant, Monsanto Company, St. Louis, MO) herbicide sprayed at the rate of 841 g ae ha-1 and 1682 g ae ha-1, respectively, followed by hand hoeing, was utilized for weed control in a weed-free period and after weedy intervals using TDI 11004 nozzles. The corn was harvested on August 19, 2019, and August 27, 2019.
2.4 Data collection
Crimson clover biomass samples were collected randomly from a 0.25 m2 area per plot before termination. The collected samples were placed in a forced air drier for 72 h at 65°C, and then the weight was recorded. Weed biomass was collected from a randomly selected 0.25 m2 quadrat from weedy plots (CTWR) immediately before applying herbicides. For example, W2, i.e., two weeks weedy; herbicides sprayed at two weeks after planting and weed biomass collected just before application. Additionally, weed biomass collected once at the end of the growing season in the weed-free duration plots. Weed species inside the randomly selected area were cut at the soil surface, placed in a forced air drier for 72 h at 65°C, and then weighed.
2.5 Critical period for weed control estimation
A sigmoidal logistic model was fitted for the weedy periods (i.e., CTWR), while the Gompertz model was fitted for the weed-free periods (i.e., CWFP) in each winter fallow (CT + WF), conventional tillage (CVT), and cover crop treatments (CT + CC). The inverse prediction method applied at 95% relative yield to estimate the CTWR and CWFP (i.e., weeks on the x-axis). The estimation of CPWC components were the next steps under which there were not a relative yield reduction greater than 5%, as the acceptable yield losses (AYL) were considered at 5% for both curves Gompertz and logistic as described by Knezevic et al. (2002); Blankenship et al. (2003), and Price et al. (2018). Regression of relative yield was performed as a function of time for both CTWR and CWFP, and then nonlinear regression models were fitted to assess the CPWC, as illustrated by Knezevic et al. (2002); Williams et al. (2007) and Korres and Norsworthy (2015).
For the weedy periods to estimate CTWR, a logistic model with three parameters was fitted to relative corn yield under all three treatments.
Moreover, for the weed-free periods to evaluate CWFP, a Gompertz model with three parameters was fitted to relative corn yield under all three treatments.
Where y is the relative corn yield, α is the asymptote, b is the slope of the curve, x0 is the point of inflection, and x is time (i.e., weeks after planting).
Hence, the difference between CWFP and CTWR components described the CPWC estimation with a 5% acceptable corn yield loss in CT + CC, CT + WF, and CVT systems. As described previously, weed control experiments estimate the relation between weed interference timings and relative crop yield and then determine the CPWC.
The collected weed biomass was quantified as a function of critical timing of weed removal (CTWR) and the critical weed-free period (CWFP) for each CT + CC, CT + WF, and CVT system using equations 1 and 2 mentioned above, in which y represents weed biomass. A sigmoidal logistic model was fitted for various weed-free periods, while the Gompertz model was fitted for the weedy periods in CT + CC, CT + WF, and CVT systems to assess weed biomass.
2.6 Statistical data analysis
The ANOVA was applied to estimate treatment effects on actual and relative (percentage of long season weed-free period) corn yield data, and means were separated through Fisher’s LSD at α=0.05. The CPWC was estimated separately for each year due to significant treatments × year interaction. Sigma Plot 14.0 (Systat Software, San Jose, CA) and JMP Pro v. 13 (SAS Institute, Cary, NC) was used for the estimation of ANOVAs, inverse predictions, curve fitting regressions, and significance model parameters. The model parameters were utilized to support the predicted values of an explanatory variable (i.e., type of independent variable) CTWR and CWFP based on the response variable of relative corn yield. Coefficient of determination (R2) was used to check the fitness of the regression model to the observed data. The comparisons between model parameters were used to evaluate the effect of experimental treatments, including CT + CC, CT + WF, and CVT, on weed biomass production.
3 Results and discussion
3.1 Crimson clover biomass and corn yield
At clover termination, the cover crop biomass was 4,204 kg ha-1 and 3,890 kg ha-1 in 2019 and 2020, respectively. The average yield following crimson clover was 7,575 kg ha-1, winter fallow 6,478 kg ha-1, and conventional tillage 7,400 kg ha-1 in 2019. The average yield following crimson clover was 8,253 kg ha-1, winter fallow 7,224 kg ha-1, and conventional tillage 7,280 kg ha-1 in 2020.
3.2 Critical period of weed control
Again, 5% acceptable yield loss (AYL) was considered to estimate the values of CTWR and CWFP as described by Blankenship et al. (2003) and Knezevic et al. (2002). In 2019, the predicted value of CTWR equals 2.5, 2.8, and 1.5 weeks after planting (WAP) for CT + CC, CT + WF and CVT systems, respectively (Figure 1 and Tables 1, 2). In addition, the predicted value of CWFP equals 5.3, 6.3, and 6.4 weeks after planting for CT + CC, CT + WF, and CVT, respectively (Figure 1 and Tables 1, 3). In 2019, based on the predicted values of CTWR for each system individually, the CTWR following the CT + CC system was delayed approximately 1.0 weeks compared with CVT system, while the beginning of CTWR under both CT + WF and CT + WF systems was in between second to third weeks (Figure 1 and Tables 1, 2). Additionally, comparing CT + CC system with CT + WF and CVT systems, the presence of crimson clover caused the early ending of CWFP at about 1.0 and 1.1 weeks respectively. However, the ending of CWFP under CT + WF and CVT systems were almost same during the weeks of 6 WAP.
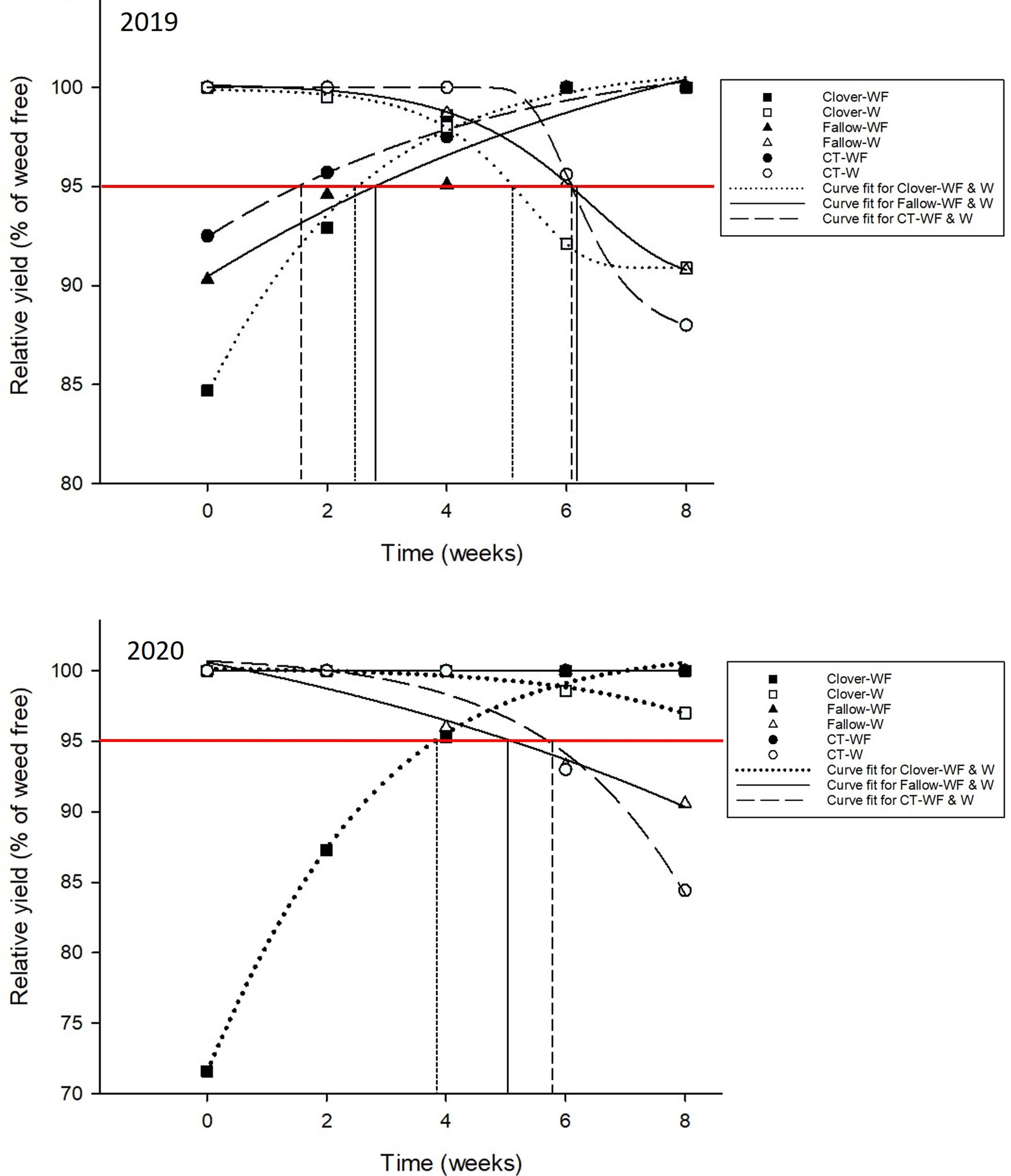
Figure 1 The critical period for weed control and its components (critical timing for weed control [CTWR, i.e., weedy] and critical weed-free period [CWFP, i.e., weed free]) for each of the conservation tillage following a crimson clover cover crop (CT + CC), conservation tillage following winter fallow (CT + WF), and conventional tillage without a cover crop (CVT) treatment in 2019 and 2020. Point estimates for CTWR and CWFP for CT + CC, CT + WF, and CVT treatments are presented in Tables 1–3. In Figure 1, WF depicted weed-free, and W represented weedy; Fallow referred to winter fallow (CT + WF), clover referred to cover crop (CT + CC), and CT depicted conventional tillage system (CVT).
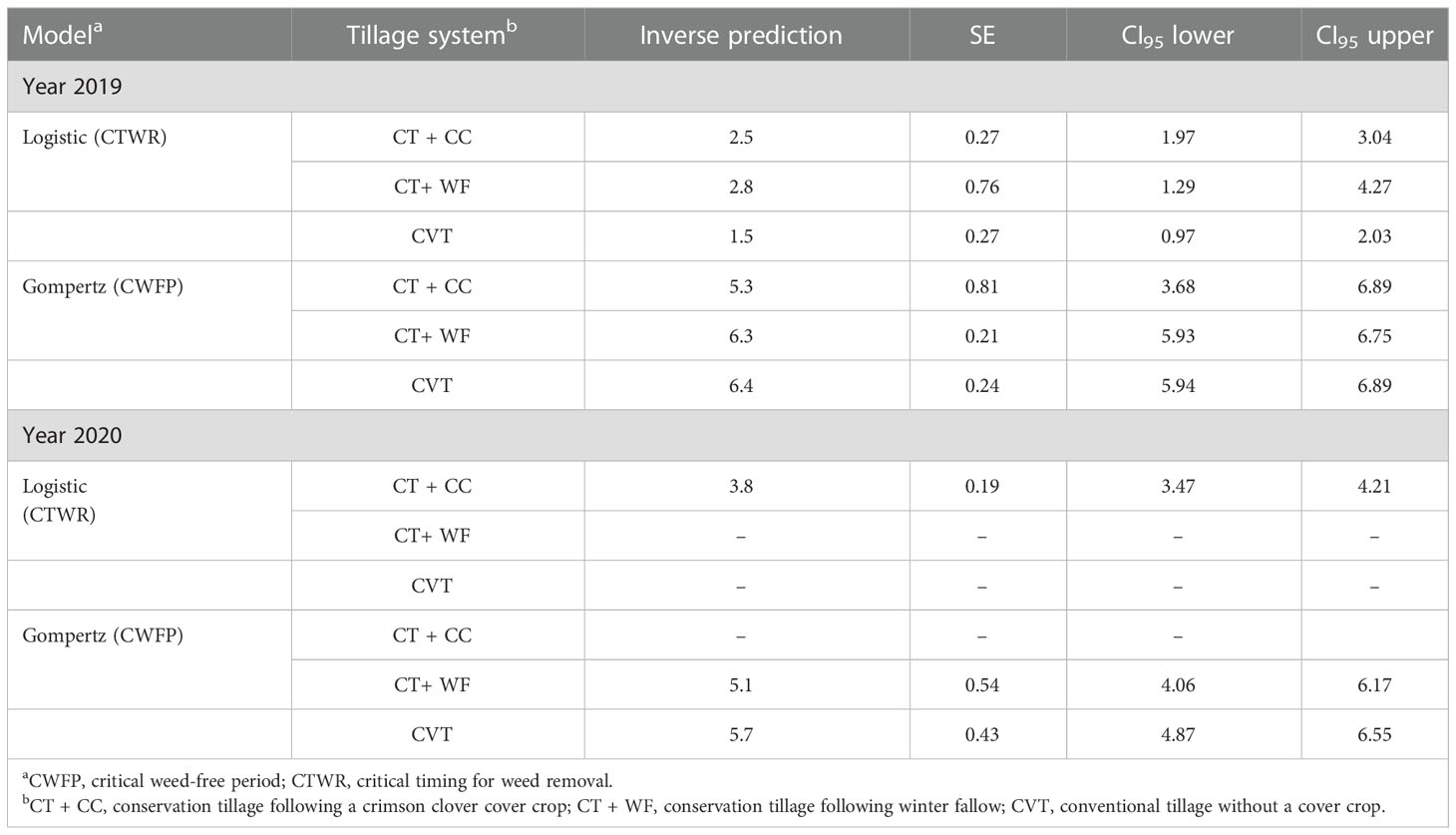
Table 1 The estimation of points (i.e., inverse predictions), standard errors (SE) of inverse predictions, and confidence intervals (CI95) corresponding to a 5% acceptable yield loss for the Logistic and Gompertz models used to estimate the beginning and end of the critical period in 2019 and 2020 for weed control in corn under three different tillage systems.
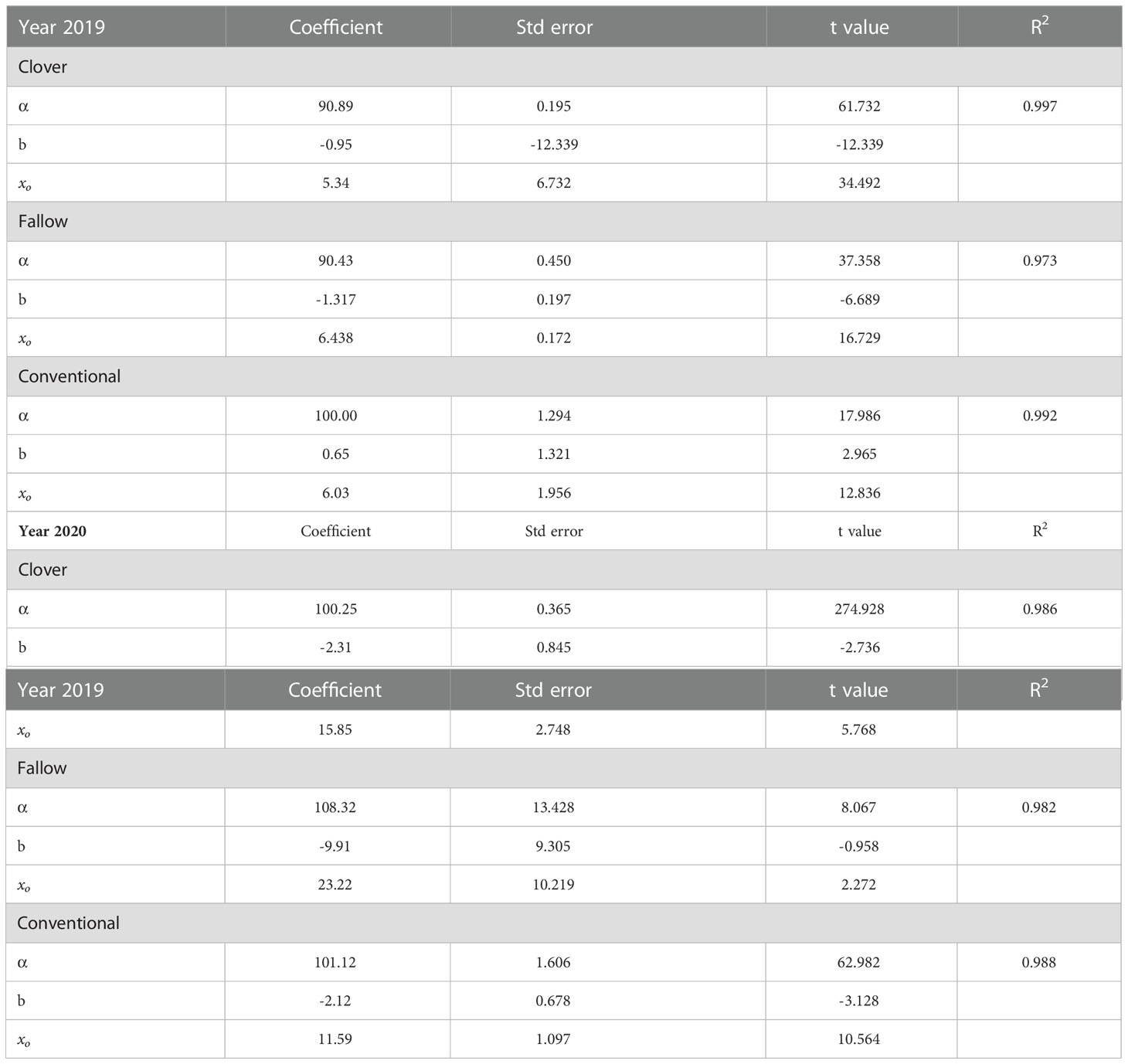
Table 2 Statistics of the three-parameter logistic regression model fitted to relative corn yield to estimate the critical weedy period (CTWR) for each of the conservation tillage following a crimson clover cover crop (CT + CC), conservation tillage following winter fallow (CT + WF), and conventional tillage without a cover crop (CVT) treatment in 2019 and 2020.
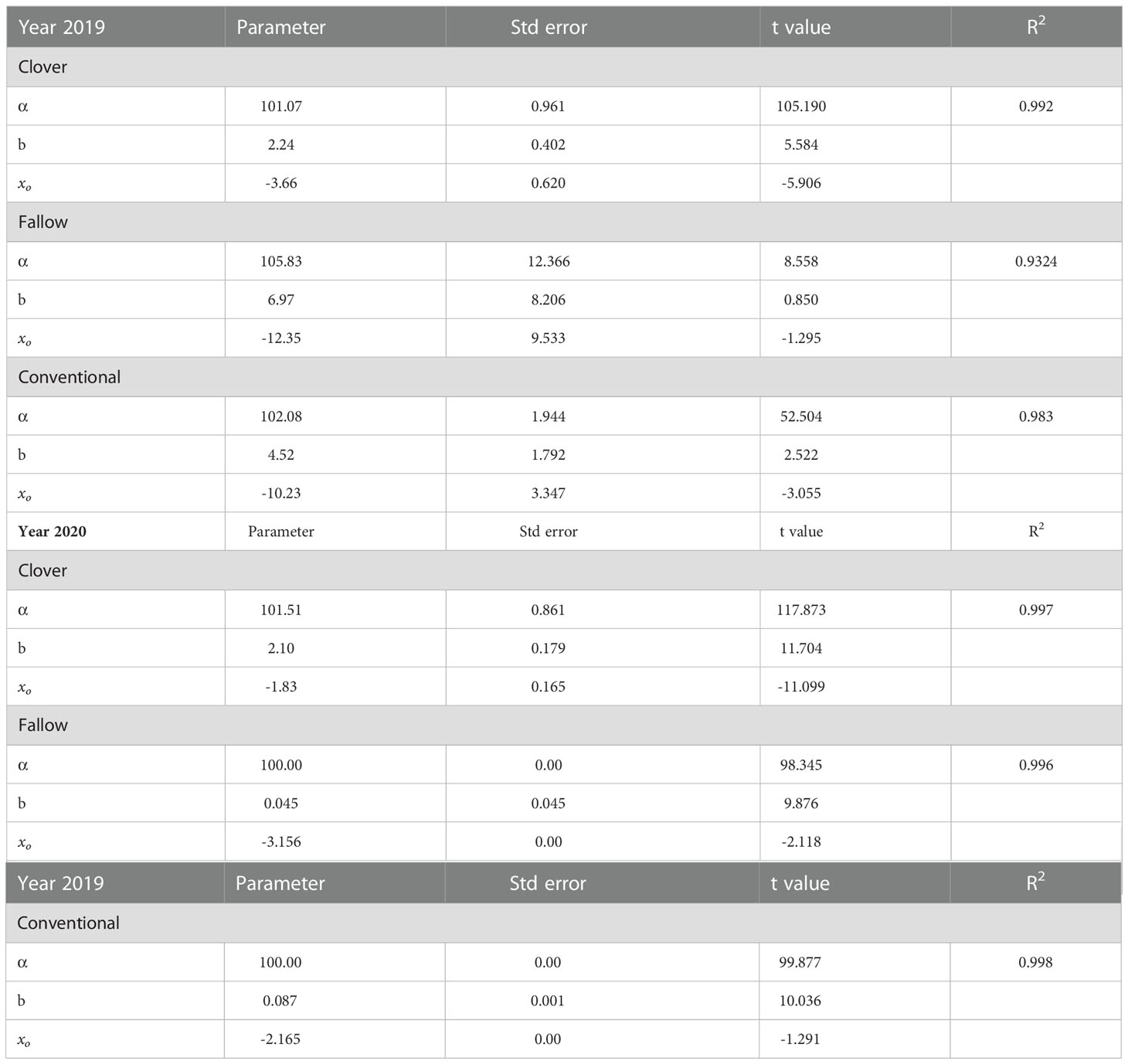
Table 3 Statistics of the three-parameter Gompertz regression model fitted to relative corn yield to estimate the critical weed-free periods (CWFP) for each conservation tillage following a crimson clover cover crop (CT + CC), conservation tillage following winter fallow (CT + WF), and conventional tillage without a cover crop (CVT) treatment in 2019 and 2020.
In 2020, the predicted value of CTWR equals 3.8 WAP for CT + CC system. While the relative yield was above the threshold level of 95% for 8 weeks, so the model did not predict the CTWR value for CT + WF and CVT systems because curves were fitted only for 8 weeks (Figure 1 and Tables 1, 2). Moreover, the predicted values of CWFP equals 5.1, and 5.7 WAP for CT + WF and CVT, respectively, whereas for CT + CC system, the model did not predict the value due to greater than 95% relative yield during most of growing season (Figure 1 and Tables 1, 3). Hence, comparing the CVT system with CT + WF system, conventional tillage and winter fallow had almost same ending period during 5th weeks of timing (Figure 1 and Tables 1, 3).
We observed yield loss increased with the extent in time of weed infestation, and Gantoli et al. (2013) reported the same in the estimation of corn CPWC. Although our points of estimated critical period were not exact same among two years because of different weed pressure in two years (Figure 2). Some previous publications indicated that the CPWC differed remarkably when estimated in respect of days after planting or days after germination (Gantoli et al., 2013). Moreover, several corn studies have estimated the critical period of weed control, and there was great variability in the CPWC. The starting of the corn CPWC was more variable (3-14 leaf stage) than the end (14-leaf stage) in Canada (Hall et al., 1992). In contrast, Halford et al. (2001) illustrated that starting of the CPWC was more stable (around 6-leaf stage) than the end period (9-13 leaf stage or 24 to 46 DAE) in corn. Results reported by Evans et al. (2003) described that the starting of CPWC was estimated from germination up to the seven-leaf stage, while the end of the CPWC was estimated from seven-leaf stage up to anthesis in corn crop. A field experiment was conducted in Canada to compare the CPWC between conventional and no-till corn and summarized that the CPWC starting and ending period was earlier under a no-till system than in conventional tillage systems (Halford et al., 2001). In addition, the previous study concluded that the estimated value of CPWC in narrow-row spacing was different than wide rows spacing in corn due to higher competition for late-germinating weeds (Murphy et al., 1996). Thus, high-density corn planted in narrow row spacing would most likely decrease the end of the CPWC (Teasdale, 1998). However, Norsworthy and Oliveira (2004) concluded that there was no significant difference between light interception in narrow and wide row spacing of corn; hence CPWC and competition of late germination weeds were almost the same in these two systems.
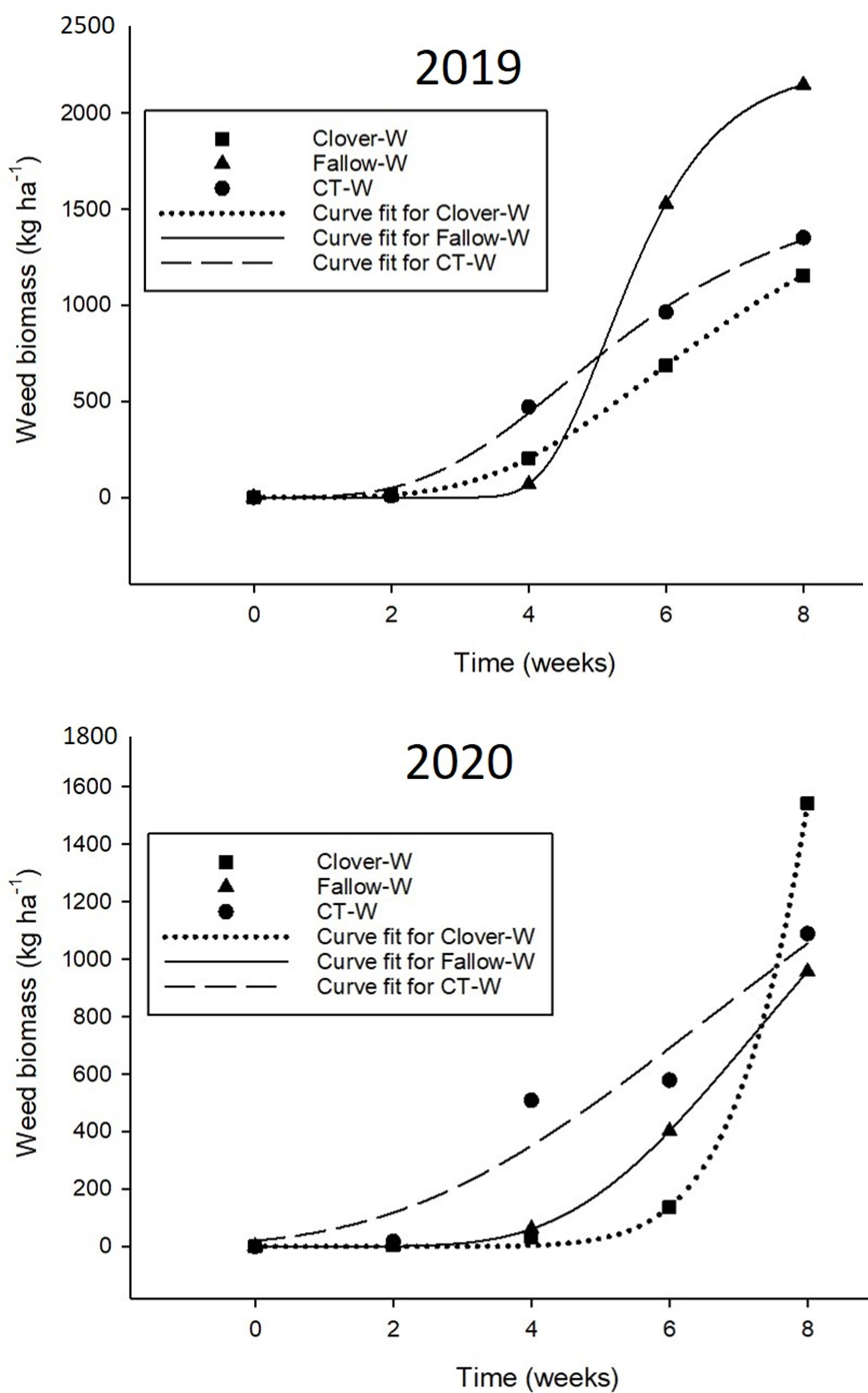
Figure 2 Weed biomass as a function of critical timing for weed removal CTWR (duration of weed interference with corn) for each of the conservation tillage following a crimson clover cover crop (CT + CC), conservation tillage following winter fallow (CT + WF), and conventional tillage without a cover crop (CVT) treatment in 2019 and 2020. Parameters of the models are presented in Table 4. W represented weedy; Fallow referred to winter fallow (CT + WF), clover referred to cover crop (CT + CC), and CT depicted conventional tillage (CVT).
3.3 Treatment effects on weed biomass production
The most common and troublesome weed species found in the southeastern United States cropping systems are Palmer amaranth (Amaranthus spp.), sicklepod [Senna obtusifolia (L.)], large crabgrass [Digitaria sanguinalis (L.) Scop.], morning glory (Ipomoea spp.), and nutsedges (Cyperus spp.) (Van Wychen, 2016). In 2019, weed removal needed to start before 150-200 kg ha-1 of weed biomass for all systems (Figure 2), based on the predicted values CTWR that started at approx. 3 WAP under CT + CC and CT + WF systems while approx. 1.5 WAP following the CVT system to prevent a yield loss greater than 5% in each system (Figure 2 and Table 4). In 2020 the recorded dry weight of weed biomass based on prediction value of CTWR (3.8 WAP) for CT + CC treatment was 30 kg ha-1 approximately. Although weed biomass of CT + WF and CVT systems were approx. 60 and 400 kg ha-1 respectively (Figure 2 and Table 4) in between 3 to 4 WAP in 2019. In both years, the weed biomass increased as the critical timing of weed removal (CTWR) increased. However, results showed differences in point estimates between slope and inflection points under each system for both years due to difference in weed pressure among both years. It has been observed that weed density was lower in 2020 than in 2019 (Figures 2, 3 and Tables 4, 5).
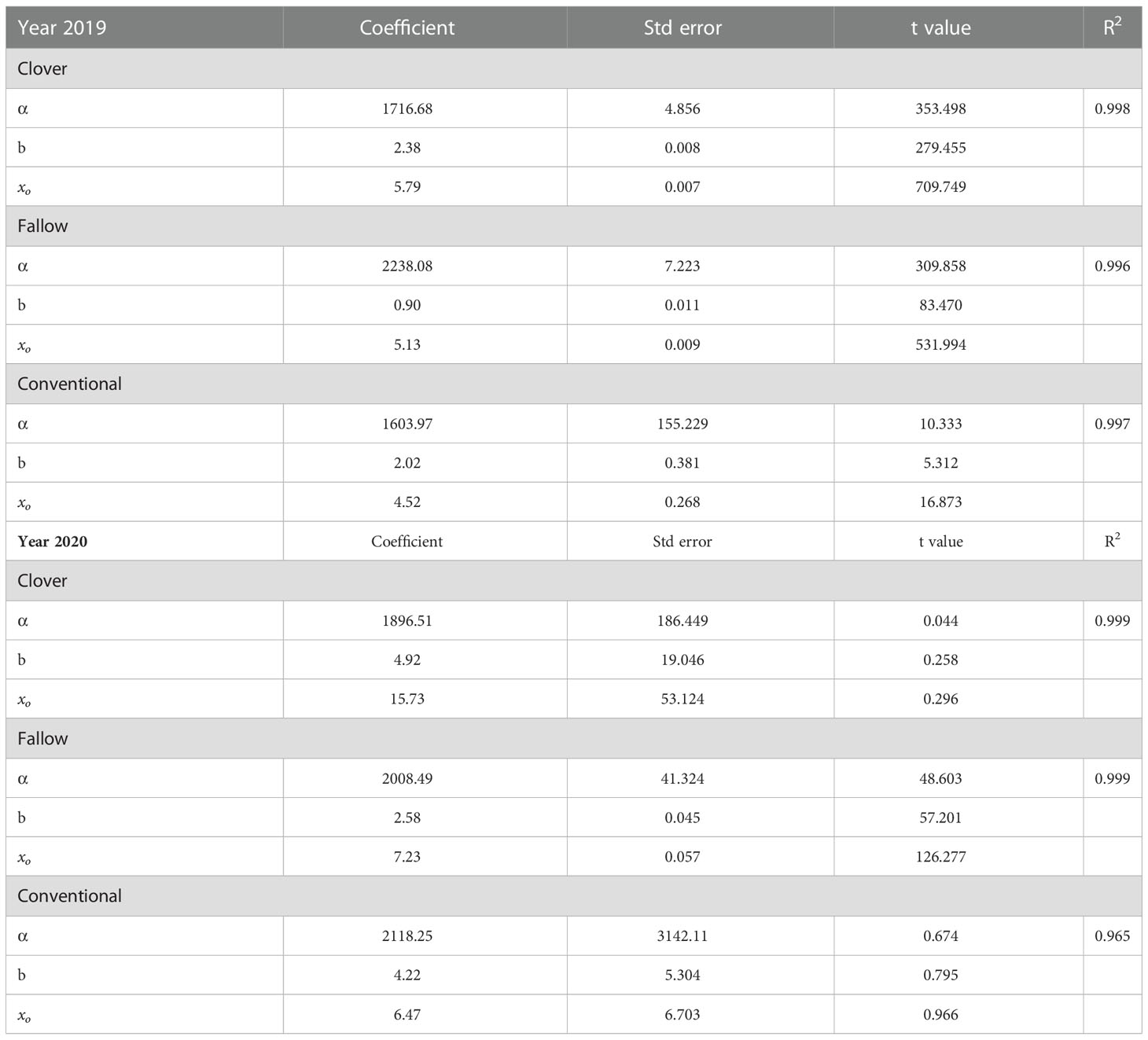
Table 4 Statistics for the three parameters Gompertz model used for fitting weed biomass production under various weedy periods for each of the conservation tillage following a crimson clover cover crop (CT + CC), conservation tillage following winter fallow (CT + WF), and conventional tillage without a cover crop (CVT) treatment in 2019 and 2020.
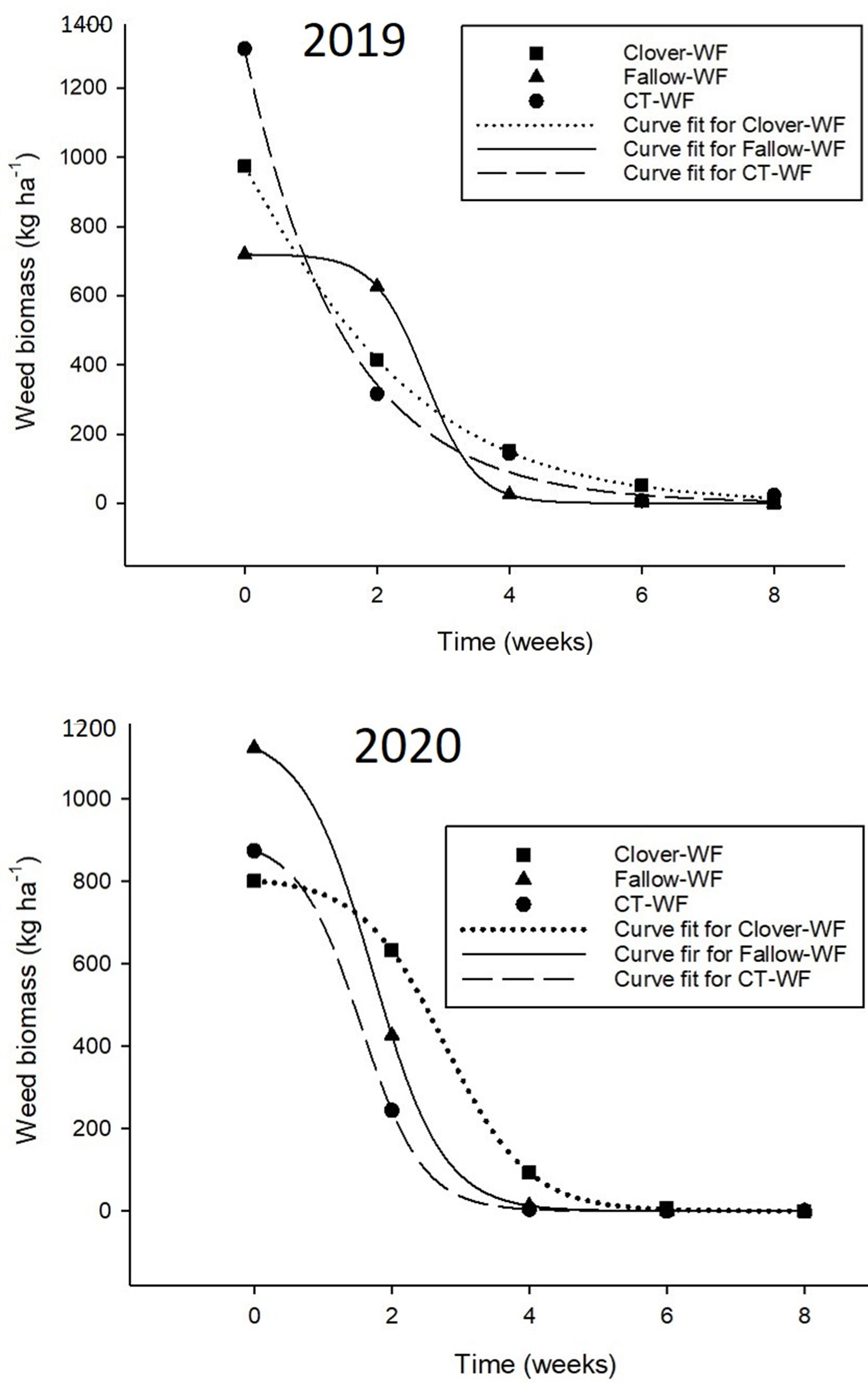
Figure 3 Weed biomass as a function of critical weed-free period CWFP for each of the conservation tillage following a crimson clover cover crop (CT + CC), conservation tillage following winter fallow (CT + WF), and conventional tillage without a cover crop (CVT) treatment in 2019 and 2020 experimental treatments. Parameters of the model are presented in Table 5. WF depicted weed-free; Fallow referred to winter fallow (CT + WF), clover referred to cover crop (CT + CC), and CT depicted conventional tillage (CVT).
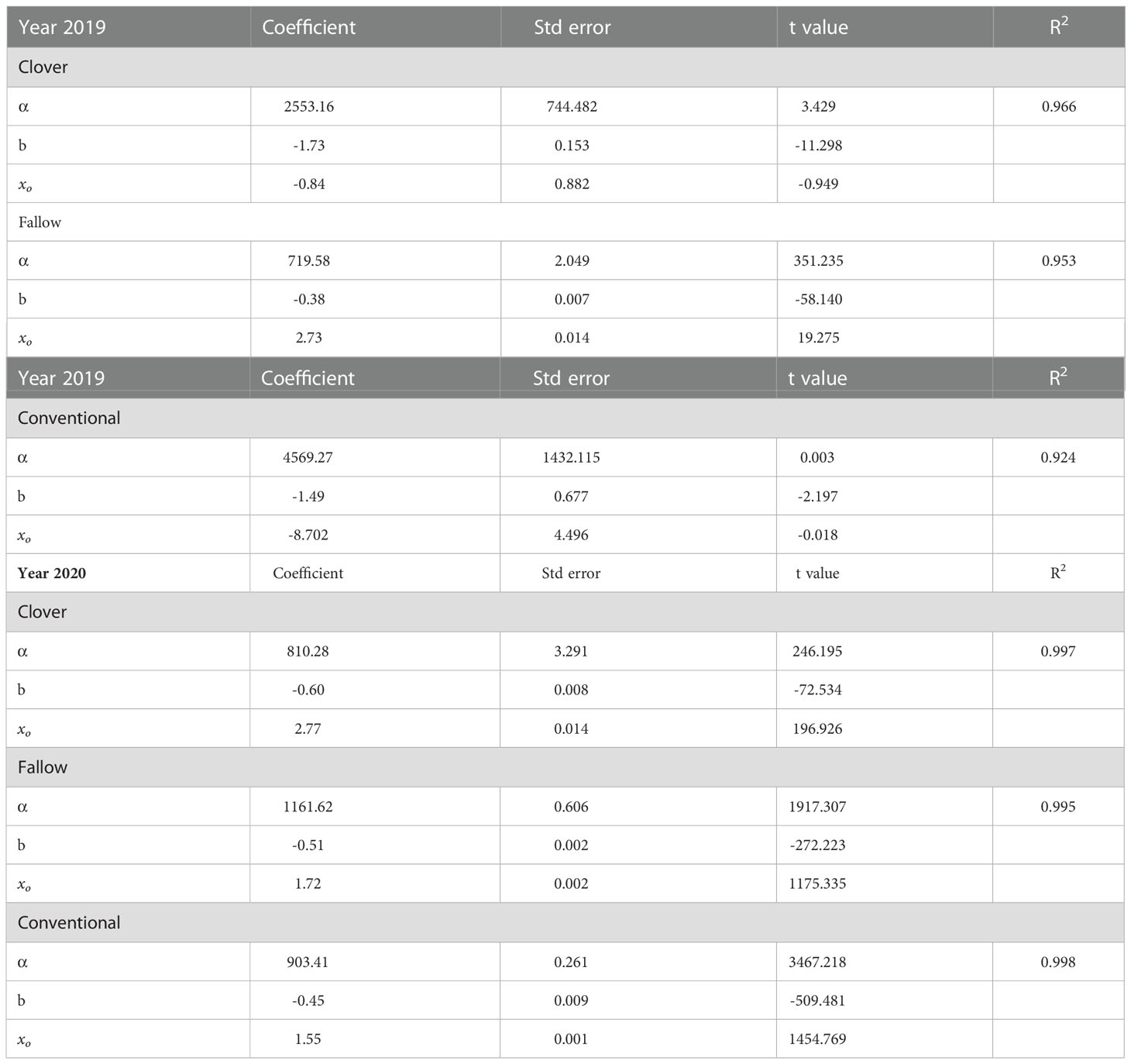
Table 5 Statistics for the three parameters logistic model used for fitting weed biomass production under various weed-free periods for each of the conservation tillage following a crimson clover cover crop (CT + CC), conservation tillage following winter fallow (CT + WF), and conventional tillage without a cover crop (CVT) treatment in 2019 and 2020.
The same strategy was followed in the case of the critical weed-free period (i.e., CWFP) following CT + CC, CT + WF, and CVT systems in both years (Figure 3 and Table 5). In 2019 the weed biomass was recorded during the predicted value of CWFP (5.3 WAP) following CT + CC treatment was approx. 100 kg ha-1. However, in case of CT + WF and CVT systems, the recorded dry weight was approx. 50-60 kg ha-1 at 6 WAP (Figure 3 and Table 5). In 2020, the recorded weed biomass level at predicted value of CWFP following CT + WF treatment (i.e., 5.1 WAP) and CVT treatment (i.e., 5.7 WAP) was 50 kg ha-1 approximately.
Moreover, the recorded maximum production of weed biomass level in both weedy and weed-free plots following CT + CC (cover crop) treatment was lower as compared to CT + WF and CVT systems under both years (Figures 2 and 3, Tables 4 and 5). This is likely due to the cover crop inhibiting weed seed (mainly small, seeded weeds) germination and decreased growth through physical suppression and allelopathy in the conservation tillage system (Akemo et al., 2000; Haramoto and Gallandt, 2004; Korres and Norsworthy, 2015). The practical application for this research is to understand the critical period of weed control (CPWC) in row crops to maintain crop yield potential is a key point in the cropping system. In addition, it is very important to have knowledge about how different cultural practices, including cover crops among others, can influence the critical period for weed removal (CPWC) and weed biomass production. Estimation of critical period of weed control (CPWC) indicated that use of residual herbicides for weed control is required (Korres and Norsworthy, 2015). The use of effective POST herbicides could effectively control the problematic weed species, especially when the critical weed-free period is short Van Acker et al. (1993). A better understanding of the CPWC in different systems, including a high residue cover crop in corn, should help farmers to maintain yield and schedule appropriate weed control timing.
4 Conclusions
In general, a difference of CWFP (i.e., end of weed control) and CTWR (i.e., beginning of weed removal) estimated the CPWC (critical period of weed control, i.e., duration) as we discussed previously. In 2019, the cover crop system had a predicted value of critical timing of weed removal (i.e., starting time) equal 2.5 weeks after planting, and critical weed-free period (i.e., ending time) equal 5.3 weeks after planting, hence the estimated duration of critical period of weed control based on two components was 2.8 weeks. While for the winter fallow system the predicted values of critical timing of weed removal equal 2.8 weeks after planting and critical weed-free period equal 6.3 weeks after planting, hence the estimated duration of critical period of weed control based on two components was 3.5 weeks in 2019. For the conventional tillage system, we found that the estimated values of critical timing of weed removal equal 1.5 weeks after planting and critical weed-free period equal 6.4 weeks after planting, hence the determined duration of critical period of weed control based on two components was 4.9 weeks in the same experimental year. Therefore, the evaluated duration of critical period of weed control in three systems, including cover crop, winter fallow and conventional tillage had 2.8, 3.5, and 4.9 weeks respectively in 2019. The presence of crimson clover cover crop delayed the critical timing of weed removal and caused the early beginning of critical weed-free period and hence shortened critical period of weed control in the 2019 experimental year likely because of later weed emergence and suppression of growth thus a crimson clover cover crop will likely provide a significant competitive advantage to corn against troublesome weed species. In 2020, as we discussed above the model did not predict the critical timing of weed removal values for winter fallow and conventional tillage system since the relative corn yield is above the 95% threshold during most of the growing season. For the critical weed-free period the estimated values were 5.1 and 5.7 weeks after planting following winter fallow and conventional tillage systems, but no prediction following the cover crop system due to the same reason of a greater 95% relative yield in 2020. In conclusion, conservation tillage following crimson clover cover crop shortened the length of critical period of weed control in corn. Moreover, the end of weed control was almost similar (in between 5 to 6 weeks after planting) under winter fallow and conventional tillage systems depending on the weed pressure during the growing season. Also, the beginning of weed removal under cover crop treatment was quite stable from the 2.5 to 3.5 weeks after planting depending on weed density during growing season. Weed control during critical periods offered a significant benefit to corn against troublesome weeds and maintained relative corn yield.
Data availability statement
The original contributions presented in the study are included in the article/supplementary material. Further inquiries can be directed to the corresponding author.
Author contributions
AP and AK: Outline the study and wrote the manuscript. NK, AG, SL, and AP: reviewed and editing. NK and AK: data analysis. All authors listed made a significant and intellectual contribution to the manuscript and approved the final manuscript for publication.
Funding
The authors declare that this research received no specific grant from any funding agency or the commercial or not-for-profit sectors.
Acknowledgments
The authors would like to thank the staff at E.V. Smith Research and Extension Center for their help maintaining and harvesting our field experiments.
Conflict of interest
The authors declare that the research was conducted in the absence of any commercial or financial relationships that could be construed as a potential conflict of interest.
Publisher’s note
Mention of a trade name, proprietary product, or specific equipment does not constitute a guarantee or warranty by USDA and does not imply endorsement of a product to the exclusion of others that may be suitable.
References
Akemo M. C., Regnier E. E., Bennett M. A. (2000). Weed suppression in spring-sown rye (Secale cereal) pea (Pisum sativum) cover crop mixes. Weed Technol. 14, 545–549. doi: 10.1614/0890-037X(2000)014[0545:WSISSR]2.0.CO;2
Ashford D. L., Reeves D. W. (2003). Use of a mechanical roller-crimper as an alternative kill method for cover crops. Am. J. Altern. Agric. 18, 37–45. doi: 10.1079/AJAA200232
Barnes J. P., Putnam A. R. (1983). Rye residues contribute to weed suppression in no-tillage cropping systems. J. Chem. Ecol. 9, 1045–1057.
Blankenship E. E., Stroup W. W., Evans S. P., Knezevic S. Z. (2003). Statistical inference for calibration points in nonlinear mixed-effects models. J. Agr. Biol. Env. Stat. 8, 455–468.
Chandler J. M., Hamill A. S., Thomas A. G. (1984). Crop losses due to weeds in Canada and the united states. WSSA special publication Champaign IL.
Chase W. R., Nair M. G., Putman A. R. (1991). 2,29-oxo-1,19- azobenzene–selective toxicity of rye (Secale cereal l.) allele chemicals to weed and crop species: II. J. Chem. Ecol. 19, 9–19.
Evans S. P., Knezevic S. Z., Lindquist J. L., Shapiro C. A., Blankership E. E. (2003). Nitrogen application influences the critical period for weed control in corn. Weed Sci. 51, 408–417. doi: 10.1614/0043-1745(2003)051[0408:NAITCP]2.0.CO;2
Gantoli G., Ayala V. R., Gerhards R. (2013). Determination of the critical period for weed control in corn. Weed Technol. 27, 63–71. doi: 10.1614/WT-D-12-00059.1
Halford C., Hamill A. S., Zhang J., Doucet C. (2001). Critical period of weed control in no-till soybean and corn (Zea mays). Weed Technol. 15, 737–744. doi: 10.1614/0890-037X(2001)015[0737:CPOWCI]2.0.CO;2
Hall M., Swanton C. J., Anderson G. W. (1992). The critical period of weed control in corn (Zea mays). Weed Sci. 40, 441–447. doi: 10.1017/S0043174500051882
Haramoto E. R., Gallandt E. R. (2004). Brassica cover cropping for weed management: A review. renewable agric. Food Syst. 19, 187–198. doi: 10.1079/RAFS200490
Holderbaum J. F., Decker A. M., Meisinger J. J., Mulford F. R., Vough L. R. (1990). Fall-seeded legume cover crops for no-tillage corn in the humid east. Agron. J. 82, 117–124. doi: 10.2134/agronj1990.00021962008200010026x
Hubbard R. K., Strickland T. C., Phatak S. (2013). Effects of cover crop systems on soil physical properties and carbon/nitrogen relationships in the coastal plain of southeastern USA. Soil Tillage Res. 126, 276–283. doi: 10.1016/j.still.2012.07.009
Kaspar T. C., Radke J. K., Laflen J. M. (2001). Small grain cover crops and wheel traffic effects infiltration, runoff, and erosion. J. Soil Water Conserv. 56, 160–164.
Knezevic S. Z., Evans S. P., Blankenship E. E., Van Acker R. C., Lindquist J. L. (2002). Critical period for weed control: The concept and data analysis. Weed Sci. 50, 773–786. doi: 10.1614/0043-1745(2002)050[0773:CPFWCT]2.0.CO;2
Korres N. E., Norsworthy J. K. (2015). Influence of a rye cover crop on the critical period for weed control in cotton. Weed Sci. 63, 346–352. doi: 10.1614/WS-D-14-00075.1
Murphy S. D., Yakubu Y., Weise S. F., Swanton C. J. (1996). Effect of planting patterns on intra row cultivation and competition between corn and late emerging weeds. Weed Sci. 44, 865–870. doi: 10.1017/S0043174500094844
Norsworthy J. K., Oliveira M. J. (2004). Comparison of the critical period for weed control in wide-and narrow-row corn. Weed Sci. 52, 02–807. doi: 10.1614/WS-03-165R
Norsworthy J. K., Ward S. M., Shaw D. R., Llewellyn R. S., Nichols R. L., Webster T. M., et al. (2012). Reducing the risks of herbicide resistance: best management practices and recommendations. Weed Sci. 60, 31–62. doi: 10.1614/WS-D-11-00155.1
Price A. J., Kelton J. A. (2013). Integrating herbicides in a high-residue cover crop conservation-agriculture setting. Herbicides - Curr. Res. Case Stud. Use 652, 563–588. doi: 10.5772/56142
Price A. J., Korres N. E., Norsworthy J. S., Li S. (2018). Influence of a cereal rye cover crop and conservation tillage on the critical period for weed control in cotton. Weed Technol. 32, 683–690. doi: 10.1017/wet.2018.73
Price A. J., Reeves D. W., Patterson M. G. (2006). Evaluation of weed control provided by three winter cereals in conservation-tillage soybean. Renewable Agric. Food Syst. 21, 159–164. doi: 10.1079/RAF2005135
Price A. J., Stoll M. E., Bergtold J. S., Arriaga F. J., Balkcom K. S., Kornecki T. S., et al. (2008). Effect of cover crop extracts on cotton and radish radicle elongation. Commun. Biometry Crop Sci. 3, 60–66.
Reeves D. W., Price A. J., Patterson M. G. (2005). Evaluation of three winter cereals for weed control in conservation-tillage non-transgenic cotton. Weed Technol. 19, 731–736. doi: 10.1614/WT-04-245R1.1
Romdhane S., Spor A., Busset H., Falchetto L., Martin J., Bizouard F., et al. (2019). Cover crop management practices rather than composition of cover crop mixtures affect bacterial communities in no-till agroecosystems. Front. Microbiol. 10. doi: 10.3389/fmicb.2019.01618
Saini M., Price A. J., Van Santen E. (2006). Cover crop residue effects on early-season weed establishment in a conservation-tillage corn-cotton rotation. In 28th South. Conserv. Tillage Conf. 28, 175–178.
Sainju U. M., Singh B. P. (1997). Winter cover crops for sustainable agricultural systems: Influence on soil properties, water quality, and crop yields. Hortic. Sci. 32, 21–28.
Swanton C. J., Weise S. F. (1991). Integrated weed management: the rationale and approach. Weed Technol. 5, 657–663. doi: 10.1017/S0890037X00027512
Teasdale J. R. (1998). Influence of corn (Zea mays) population and row spacing on corn and velvetleaf (Abutilon theophrasti) yield. Weed Sci. 46, 447–453. doi: 10.1017/S0043174500090883
Teasdale J. R., Mohler C. L. (2000). The quantitative relationship between weed emergence and the physical properties of mulches. Weed Sci. 48, 385–392. doi: 10.1614/0043-1745(2000)048[0385:TQRBWE]2.0.CO;2
Van Acker R. C., Swanton C. J., Weise S. F. (1993). The critical period of weed control in soybean. (Glycine max). Weed Sci. 41, 194–200. doi: 10.1017/S0043174500076050
Van Wychen L. (2016). Survey of the most common and troublesome weeds in broadleaf crops, fruits & vegetables in the united states and Canada. Weed Sci. Soc. America Natl. Weed Survey Dataset.
Vissoh P. V., Gbehoungou G., Ahantch A., Kuyper T. W., Rolling N. G. (2004). Weeds as agricultural constraint to farmers in Benin: Results of a diagnostic study. NJAS – Wageningen J. Life Sci. 52, 308–329. doi: 10.1016/S1573-5214(04)80019-8
Wiggins M. S., McClure M. A., Hayes R. M., Steckel L. E. (2015). Integrating cover crops and POST herbicides for glyphosate-resistant palmer amaranth (Amaranthus palmeri) control in corn. Weed Technol. 29, 412–418. doi: 10.1614/WT-D-14-00145.1
Williams M. M., Ransom C. V., Thompson W. M. (2007). Volunteer potato density influences critical time of weed removal in bulb onion. Weed Technol. 21, 136–140. doi: 10.1614/WT-06-009.1
Keywords: critical weed-free period, critical timing of weed removal, conservation tillage, integrated weed management, relative yield, cover crop
Citation: Kumari A, Price AJ, Korres NE, Gamble A and Li S (2023) Effect of crimson clover on the critical period of weed control in conservation tillage corn. Front. Agron. 4:1068365. doi: 10.3389/fagro.2022.1068365
Received: 12 October 2022; Accepted: 28 December 2022;
Published: 16 January 2023.
Edited by:
Simerjeet Kaur, Punjab Agricultural University, IndiaReviewed by:
Manpreet Singh, Punjab Agricultural University, IndiaKasio Ferreira Mendes, Federal University of Viçosa, Brazil
Copyright © 2023 Kumari, Price, Korres, Gamble and Li. This is an open-access article distributed under the terms of the Creative Commons Attribution License (CC BY). The use, distribution or reproduction in other forums is permitted, provided the original author(s) and the copyright owner(s) are credited and that the original publication in this journal is cited, in accordance with accepted academic practice. No use, distribution or reproduction is permitted which does not comply with these terms.
*Correspondence: Andrew J. Price, andrew.price@ars.usda.gov