- Eastern Regional Research Center, United States Department of Agriculture (USDA) Agricultural Research Service, Wyndmoor, PA, United States
An increasing demand for organic produce, coupled with the need to reduce reliance on the diminishing resource of rock phosphate, has bolstered interest in the use of arbuscular mycorrhizae (AMF) as a biofertilizer. AMF are symbiotic fungi that colonize the roots of most crops and transfer nutrients from the soil to their hosts in exchange for carbon. While past studies have shown that mixed AMF communities grown from field soil can increase the yield of many crops, the targeted use of individual AMF species on host plants is a promising avenue to enhance nutrient uptake. We inoculated sweet corn (Zea mays) and butternut squash (Cucurbita moschata) seedlings with nine individual species of AMF and one mixed indigenous population to determine the most beneficial symbionts for enhancing mineral nutrient concentration and yield. Overall, level of root colonization was correlated with phosphorus (P) concentration of aboveground biomass. Corn and squash grown in association with AMF species in the Rhizophagus genus had the highest level of root colonization and tissue P concentration. Claroideoglumus etunicatum and Gigaspora margarita increased calcium (Ca) and magnesium (Mg) concentration in corn and Gigaspora rosea increased calcium in squash. S. constrictum and G. rosea positively impacted sweet corn seedling biomass. Based on this evidence, AMF species vary in their benefit to plant nutrient uptake and the most beneficial species depend on host. Further research on the effectiveness of inoculating individual AMF species across a range of hosts and ecosystems will prove useful in the development of host-targeted AMF biofertilizers.
Introduction
A growing demand for organically produced food, coupled with increased evidence that the historical overuse of chemical fertilizers has negatively impacted the environment have recently bolstered interest in the use of arbuscular mycorrhizal fungi (herein AMF) as a biofertilizer to supplement or replace agrichemicals (Sadhana, 2014; Berruti et al., 2016; Igiehon and Babalola, 2017; Madawala, 2021; Jerbi et al., 2022). AMF are obligate symbionts that grow in association with about 80% of terrestrial plants including most crops (Brundrett and Tedersoo, 2018). AMF vastly enhance the surface area of root systems and increase accessibility to water and nutrients in the soil which are exchanged with the plant for photosynthetically derived carbon, including sugars and lipids (Read and Perez‐Moreno, 2003; Keymer et al., 2017). As such, AMF are an integral component of crop nutrition, growth and present a promising research avenue for enhancements in yield and nutrient concentration of vegetables.
The use of on-farm produced mixed species inoculants of AMF have been shown to increase the yield of a variety of crops including eggplant, peppers, potatoes, sweet potatoes, and strawberries by up to 15% (Douds Jr. and Reider, 2003; Douds Jr. et al., 2007; Douds Jr. et al., 2008; Douds Jr. et al., 2015; Douds Jr. et al., 2017). Typically, these mixed species inoculants are produced by growing a host plant such as Bahia grass (Paspalum notatum Flugge) or Sorghum-Sudan grass in pot cultures containing soil from the farm on which the inoculated crops are then grown. The pot cultures are maintained allowing AMF to sporulate and the potting media is used as an inoculant (Douds Jr. et al., 2005; Douds et al., 2010). While mixed species inocula have proven promising in enhancing crop yield, disentangling the impact of individual species of AMF on crop growth and nutrient concentration is less straightforward. It is likely that individual AMF species and host plants lie on a spectrum of mutualism wherein the carbon (C) cost of resource return (and vice versa) of the relationship varies by host and AMF species (Werner and Kiers, 2015). We hypothesized that while one AMF species may benefit one host, the same species may not benefit a different host.
Most research on the inoculation of crops and most commercially produced inoculant products focus on three ‘generalist’ and geographically ubiquitous AMF species: Rhizophagus intraradices, Rhizophagus irregularis, and Funneliformis mosseae (Berruti et al., 2016; Basiru et al., 2020). In addition, commercially sourced products used in studies to examine the effectiveness of single species inoculation may already be treated with agrichemicals to avoid pest contamination, may lose viability through prolonged storage (Douds Jr. et al., 2005), and labelling may not accurately reflect AMF species present in the product (Berruti et al., 2013). Possibly owing to these issues, the effectiveness of commercial inoculants in enhancing mycorrhizal colonization of crops is mixed (Tarbell and Koske, 2007; Salomon et al., 2022) and impacts of these products on yield is not consistent (Corkidi et al., 2004; Faye et al., 2013). As such, the potential for a range of single species AMF to be successful biofertilizers remains unclear.
In this study, we examined the impact of nine individual AMF species representing six genera, and one mixed species inoculant, on nutrient concentration and seedling biomass of sweet corn (Zea mays, cv. Coastal, herein corn) and butternut squash (Cucurbita moschata, cv. Waltham, herein squash). Corn and squash were chosen for this study due to the ease by which the common growers’ practice of greenhouse germination of seedlings for both crops can be adapted to incorporate AMF inoculum, thus the inoculation method and the findings have practical relevance towards real world crop production scenarios. To determine the extent to which individual mycorrhizal species benefited soil nutrient foraging capacity of corn and squash, we measured mycorrhizal colonization 15- and 30-days post-germination via microscopy and molecular quantification and subsequently determined whether enhanced mycorrhizal colonization led to differences in above and belowground biomass as well as macro and micronutrient concentration of aboveground tissues.
Methods
Trap culturing of AMF by Bahia grass and preparation of inoculum
Single species AMF accessions were acquired from the International Culture Collection of Vesicular-Arbuscular Mycorrhizal Fungi at West Virginia University, Morgantown, WV; USA (INVAM), or in the case of Septoglomus constrictum, from the collection of the United States Department of Agriculture, Agricultural Research Service, Eastern Regional Research Center, Wyndmoor, PA; USA (Table 1). These fungi were maintained in Bahia grass (Paspalum notatum Flugge) pot cultures grown in a mixture of sterile sand, soil, vermiculite, and turface (herein SSVT; 1:0.75:1:0.75 v/v; Douds et al., 2010). To produce a mixed mycorrhizal inoculum, Bahia grass plants were grown in a 1:10 dilution of field soil and SSVT. The field soil was a composite sample collected in November 2019 from organic plots within the Vegetable Systems Trial at the Rodale Institute, Kutztown, PA; USA in SSVT. Bahia grass trap cultures were watered daily and fertilized weekly with Hoagland’s solution modified to contain.10X the original P (Hoagland and Arnon, 1950). To prepare the mycorrhizal inoculum, the aboveground portion of the Bahia grass plants was removed, their roots were cut into ~1cm lengths, and the roots were reincorporated with SSVT medium. The mycorrhizal inoculum was diluted 1:50 with sterile SSVT before planting. The treatments included 9 individual mycorrhizal species, the mixed species inoculum, and a non-mycorrhizal control comprised of media and roots from uninoculated Bahia grass pot cultures for a total of 11 treatments (Table 1).
Planting
For each treatment, 24 Sweet corn cv. Coastal (Seedway, Hall NY) and 24 butternut squash cv. Waltham (Johnny’s Selected Seeds, Winslow ME) seeds were sown, one seed per pot, in SC10 Ray Leach Cone-tainers™ (164 mL; Steuwe & Sons, Inc., Tangent OR) 25-Mar-2021 containing the 1:50 inoculum:SSVT media. In each pot there were approximately 300 spores, plus hyphae and colonized root fragments. Treatments were randomly organized on raised benches and were grown in a heated (18-25°CC) greenhouse at the Rodale Institute, Kutztown PA under natural light. Plants were fertilized once per week with Hoagland’s solution, modified to contain 0.1X P and watered daily (Hoagland and Arnon, 1950).
Biomass sampling
Roots were sampled at 15- and 30-days post planting. On both dates, 5 representative plants were destructively harvested from each treatment and aboveground tissue were separated from roots. Roots were gently washed with water to remove media and refrigerated before microscopy and molecular analysis. On the second sampling date, aboveground and belowground biomass was measured and aboveground tissue was further dried at 85°CC for ~24 h and ground to a powder for nutrient and mineral analysis.
Mycorrhizal colonization
To examine the efficiency at which different mycorrhizal species colonized roots, percent colonization of roots was determined on day 15 and 30 on five corn and squash seedlings. In brief, roots were soaked at room temperature in 10% potassium hydroxide for ~96 h and then subsequently brought to a boil for five minutes to clear cells and remove pigment. Roots were then acidified in 1% hydrochloric acid for five min at 90°CC and stained with 0.5% trypan blue (Phillips and Hayman, 1970). Percent of root length colonized by AMF was then determined by the gridline intersect method (Giovannetti and Mosse, 1980). For molecular quantification, DNA from root 50 mg subsamples was extracted and used for multiplex qPCR using the methods previously described in (Heller and Carrara, 2022). ZM-HMG, the Zea mays High Mobility Group (HMG) A gene (Scholdberg et al., 2009) was used as a reference to quantify corn DNA from root samples.
Shoot and leaf nutrient and mineral concentration
Dried and ground aboveground biomass of corn and squash seedlings collected on the second sampling date (30 days) were submitted to the Penn State University Agricultural Analytical Laboratory (State College, PA). To determine the extent to which AMF inoculation altered nutrient uptake, we analyzed tissue concentration of 5 macronutrients including phosphorus (P), potassium (K), calcium (Ca), magnesium (Mg), and sulfur (S). We analyzed an additional 6 micronutrients including iron (Fe), copper (Cu), boron (B), aluminum (Al), zinc (Z), and sodium (Na). Nutrient concentrations were determined by HNO3 and H2O2 digestion followed by inductively coupled plasma–optical emission spectrometry analysis (Huang and Schulte, 1985).
Statistical analysis
To examine the extent to which AMF inoculation altered tissue nutrient concentration and biomass we performed one-way analysis of variance (ANOVA) on each independent variable (i.e. biomass, nutrient concentration) with mycorrhizal treatment as the main factor after checking if the assumptions of normality (Shapiro-Wilkes test) and heteroskedasticity (Breush-Pagan test) were met. We performed ANOVA on corn and squash separately because we were chiefly interested in the impact of inoculation on nutrient concentration and biomass for each host rather than making comparisons between squash and corn. If the results of the ANOVA were determined to be significant (significance threshold p<0.05), we then performed post hoc Tukey-Kramer HSD tests to examine for significant differences between treatments. In cases that assumptions of AVOVA were not met, we performed non-parametric Kruskall-Wallace H tests followed by Conover’s multiple comparisons test. We determined the level of correlation between % mycorrhizal colonization and tissue nutrient concentration using Pearson’s product-moment correlation across all treatments. All statistics were completed in R Studio v.1.4.1106 (R Studio Team, 2020).
Results
Mycorrhizal colonization
The effectivity of establishing mycorrhizal colonization varied across AMF species and between squash and corn. R. intraradices was the best colonizer of both corn and squash on both sampling dates. After 15 days, mycorrhizal colonization of corn seedlings ranged from 64% in the R. intraradices treatment to 0.7% in the S. constrictum treatment (Figure 1A). After 30 days, colonization ranged from 87% in in the R. intraradices treatment to 3% in the G. margarita treatment. In squash, colonization was generally lower and ranged from 45% in the R. intraradices treatment to 3% in the S. constrictum treatment after 15 days and from 95% in the R. intraradices treatment to 3% in the S. constrictum treatment after 30 days (Figure 1B).
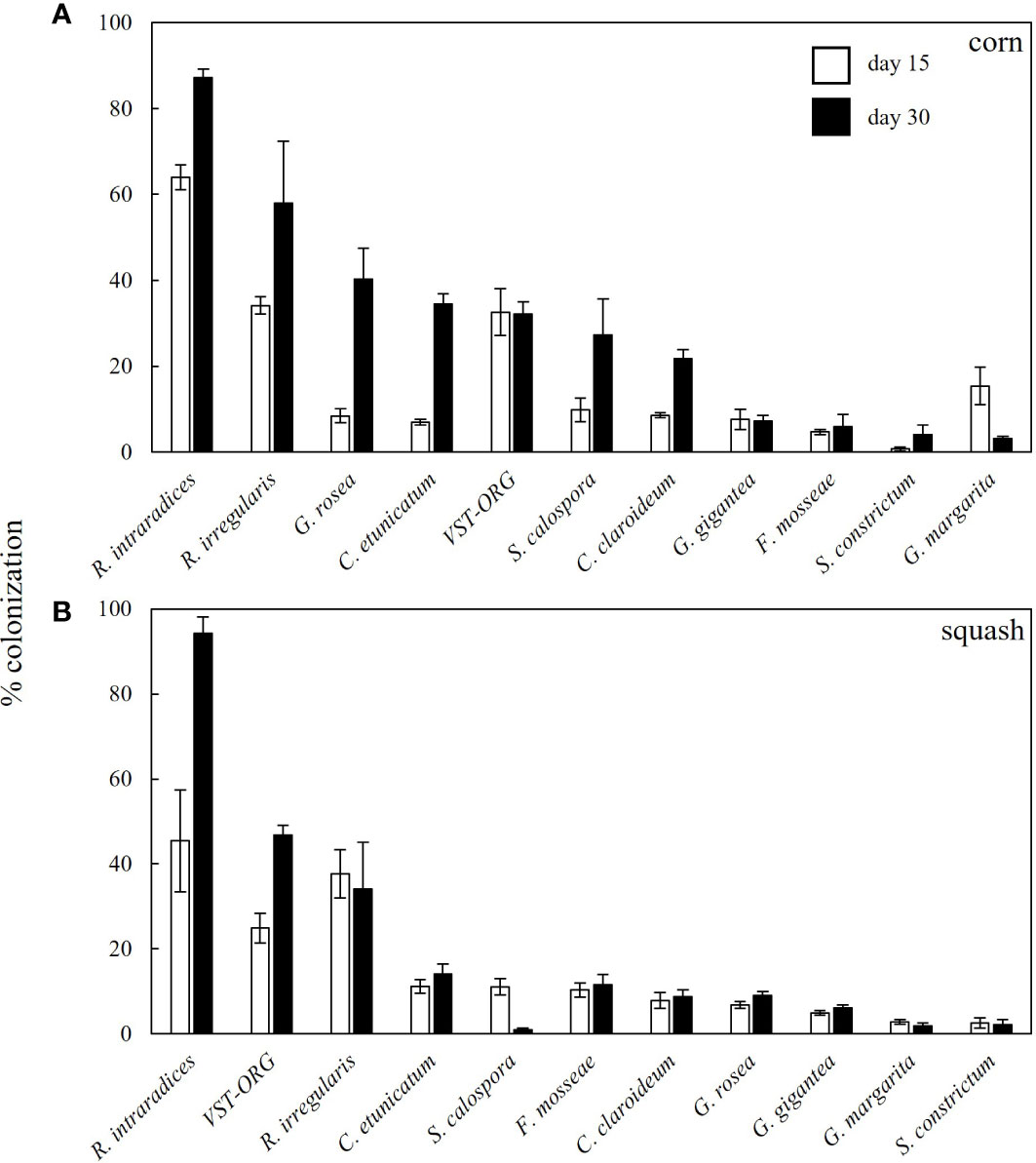
Figure 1 Percent colonization of roots varied across AMF inocula. Percent colonization values of (A) corn and (B) squash roots. Valued are means (n=5 plants) presented with standard error as measured fifteen days after planting (open bars) and 30 days after planting (black bars).
Relative quantification of fungal DNA to plant DNA (Figure 2) revealed that roots of corn seedlings inoculated with F. mosseae were associated with 10-fold higher fungal DNA on day 30 compared to day 15. For plants inoculated with C. etunicatum, the relative quantity of fungal DNA increased by 5.8-fold on day 30 compared to day 15, whereas for R. irregularis inoculated plants, the fungal DNA was the same on both sampling dates, and for R. intraradices inoculated plants, fungal DNA decreased by nearly half on day 30.
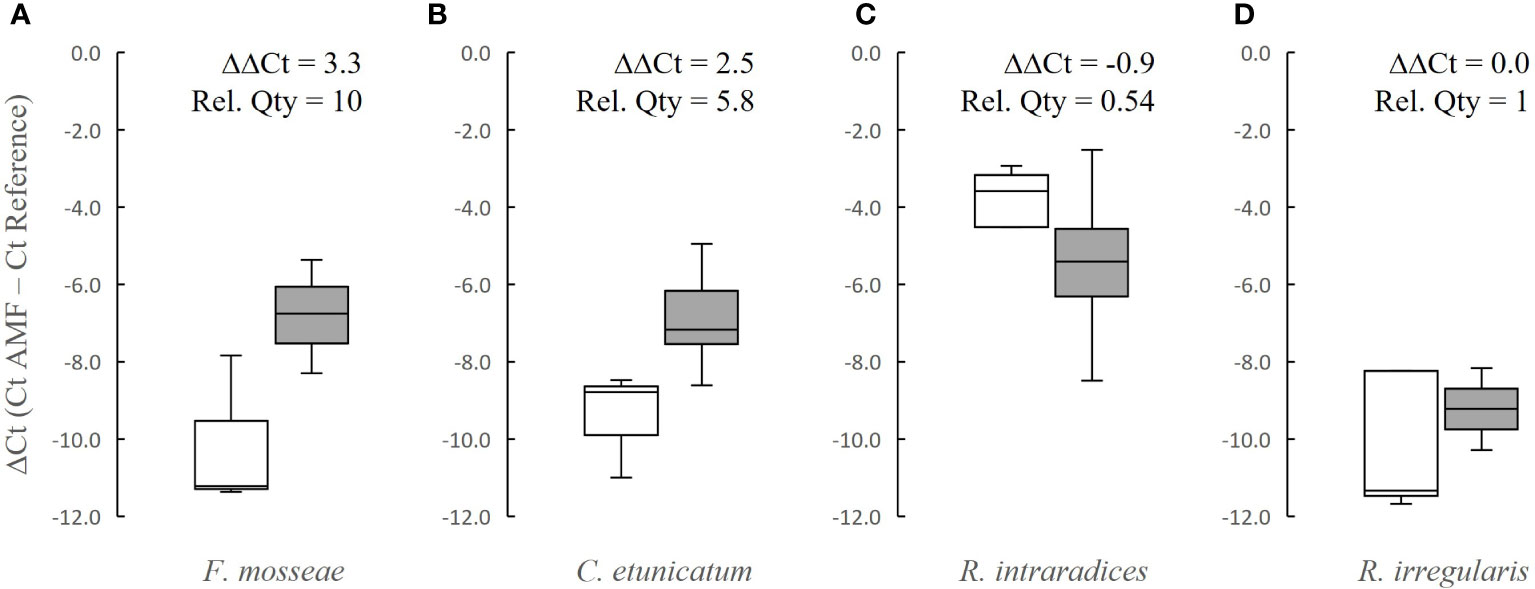
Figure 2 Box and whisker plots showing relative quantification of AMF DNA associated with inoculated corn seedling roots after 15 days (white bars) and 30 days (gray bars) for (A) F. mosseae, (B) C. etunicatum, (C) R. intraradices, and (D) R. irregularis. The difference between the average ΔCt value on days 30 and 15 (ΔΔCt) was used to calculate the relative quantity (Rel. Qty) for each fungal DNA target.
Aboveground tissue nutrient and mineral concentration
Overall, percent mycorrhizal colonization of squash and corn roots was positively correlated with leaf tissue P concentration across all mycorrhizal treatments (Figures 3A, B). P concentration was 139% higher in corn inoculated with R. intraradices and 125% higher in corn inoculated with R. irregularis (Figure 4A). In addition to higher P, R. irregularis inoculated corn had 129% more Mg than uninoculated corn (Table 2). C. etunicatum increased Mg by 156% and Ca by 146% (Table 2). Finally, G. margarita inoculated corn had 155% more Mg and 140% more Ca than uninoculated corn but had 13% less K (Table 2). Finally, seven mycorrhizal treatments had lower Na concentration than uninoculated corn (Table 2). There were no other differences in macro- and micronutrients between uninoculated and inoculated corn seedlings (Table S1).
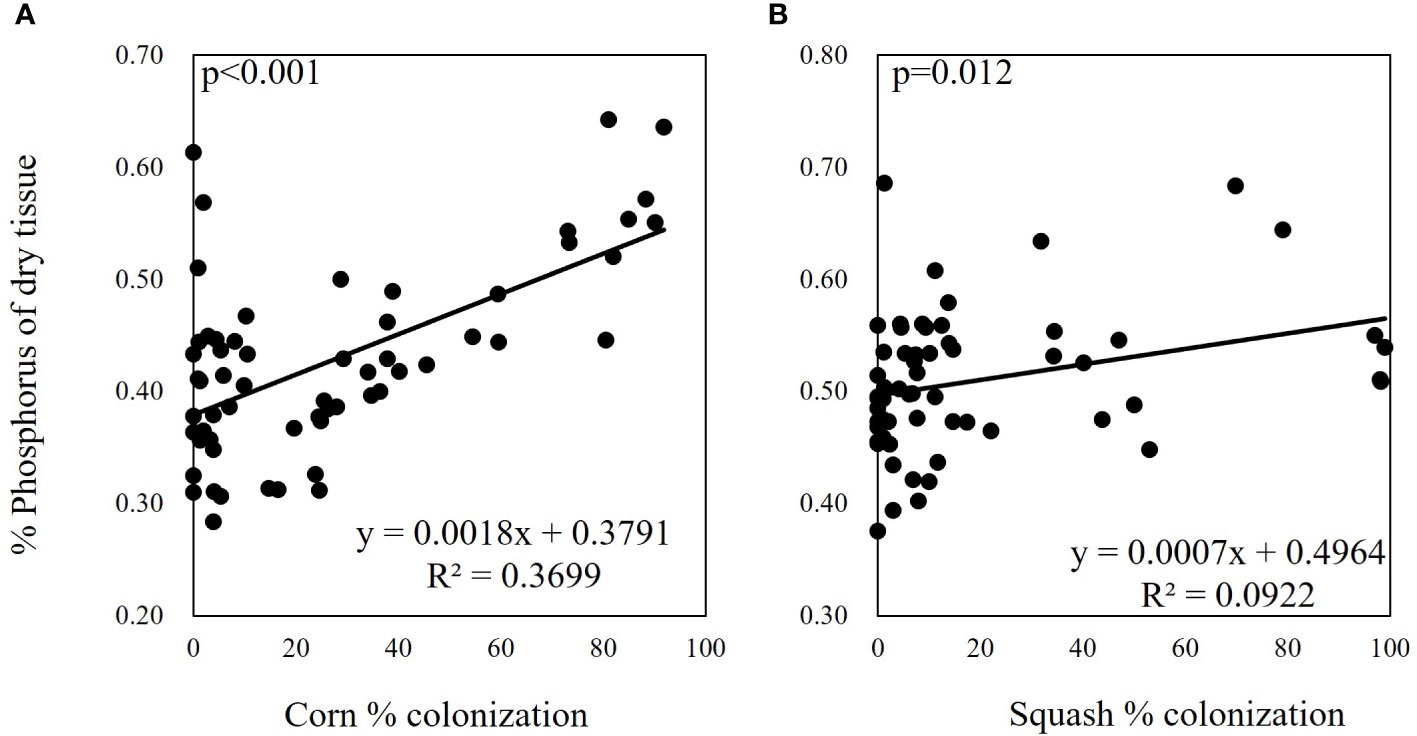
Figure 3 Percent root colonization across all seedlings was significantly correlated with % aboveground tissue phosphorus in corn (A) and squash (B). P values are from Pearson’s product moment correlation. Percent colonization data presented are from the day 30 sampling.
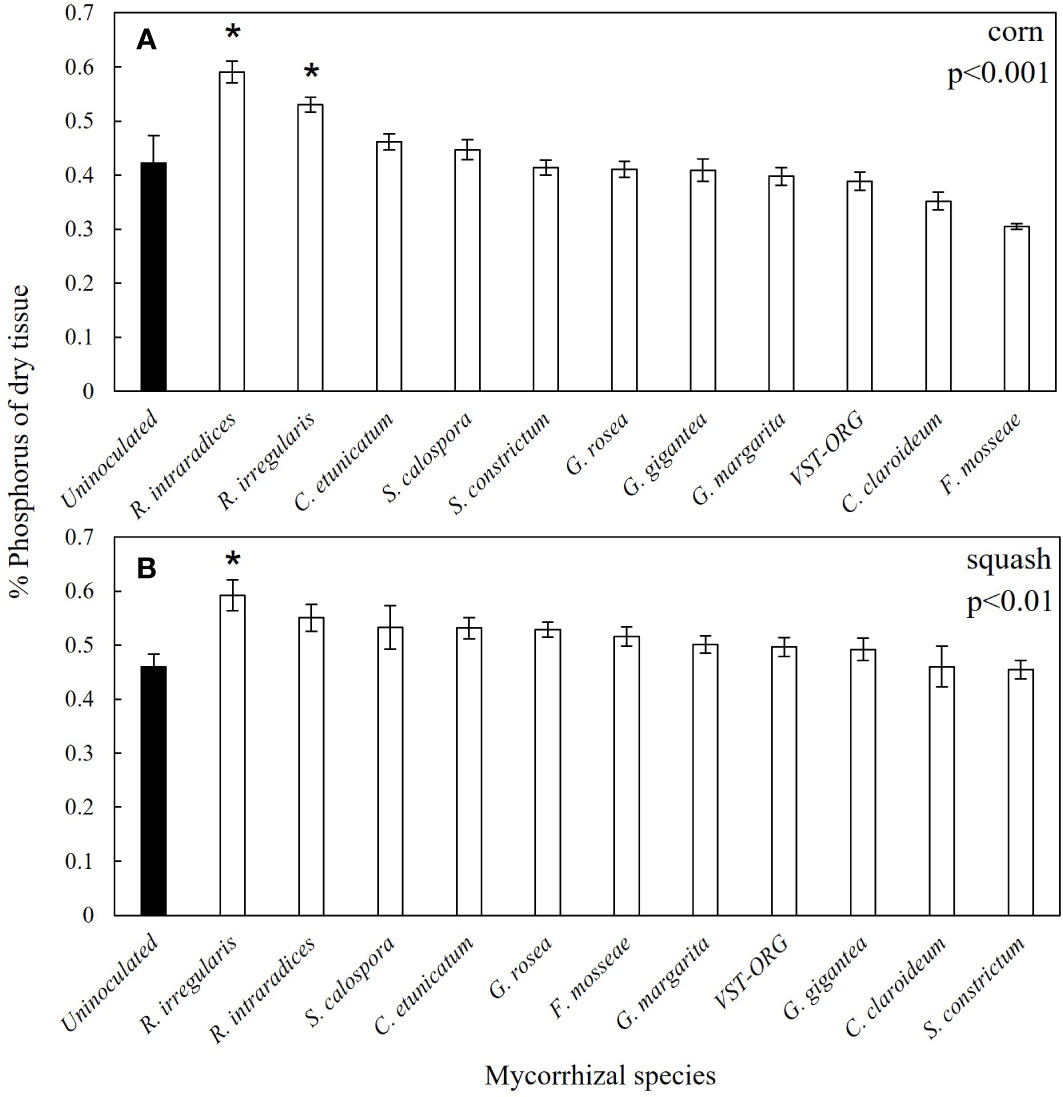
Figure 4 Both Rhizophagus species enhanced P uptake in corn and R. irregularis enhanced P uptake in squash. Values are mean % aboveground tissue phosphorus (n=5) presented with standard error in corn (A) and squash (B). The closed are uninoculated seedlings and the open bars are inoculated seedlings. P values are from Kruskall-Wallace H test. Asterisks represent significant differences between uninoculated seedlings and inoculated seedlings p<0.05. Dagger represents significant differences between uninoculated seedlings and inoculated seedlings p<0.10.
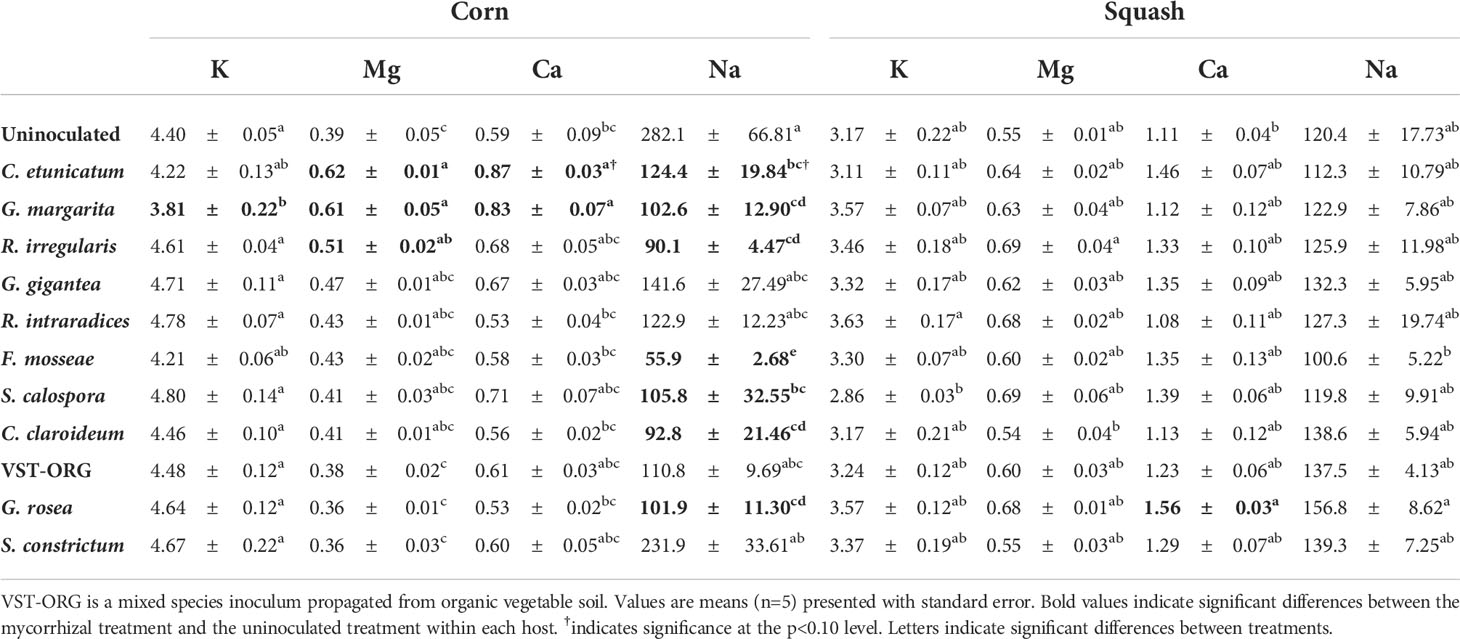
Table 2 Potassium (K), magnesium (Mg), calcium (Ca), and sodium (Na) concentration (ppm) in corn and squash seedlings inoculated with mycorrhizae.
There were fewer differences in aboveground nutrient concentration between inoculated and uninoculated squash. R. irregularis inoculated squash had 129% more P than uninoculated squash and G. rosea inoculated squash had 141% more Ca (Figure 4B and Table 2). VST-ORG inoculated squash had 23% less S (Table S2).
Aboveground tissue and root biomass
In corn, plants associated with S. constrictum had 200% more aboveground biomass relative to control plants (Figure 5). G. rosea associated corn had 162% more aboveground biomass and 200% more belowground biomass than uninoculated plants (Figures 5, 6A). In squash, plants associated with G. margarita had 62% less root biomass than uninoculated plants and plants associated with G. rosea had 63% less root biomass (Figure 6B). The other mycorrhizal treatments had no effect on squash root biomass.
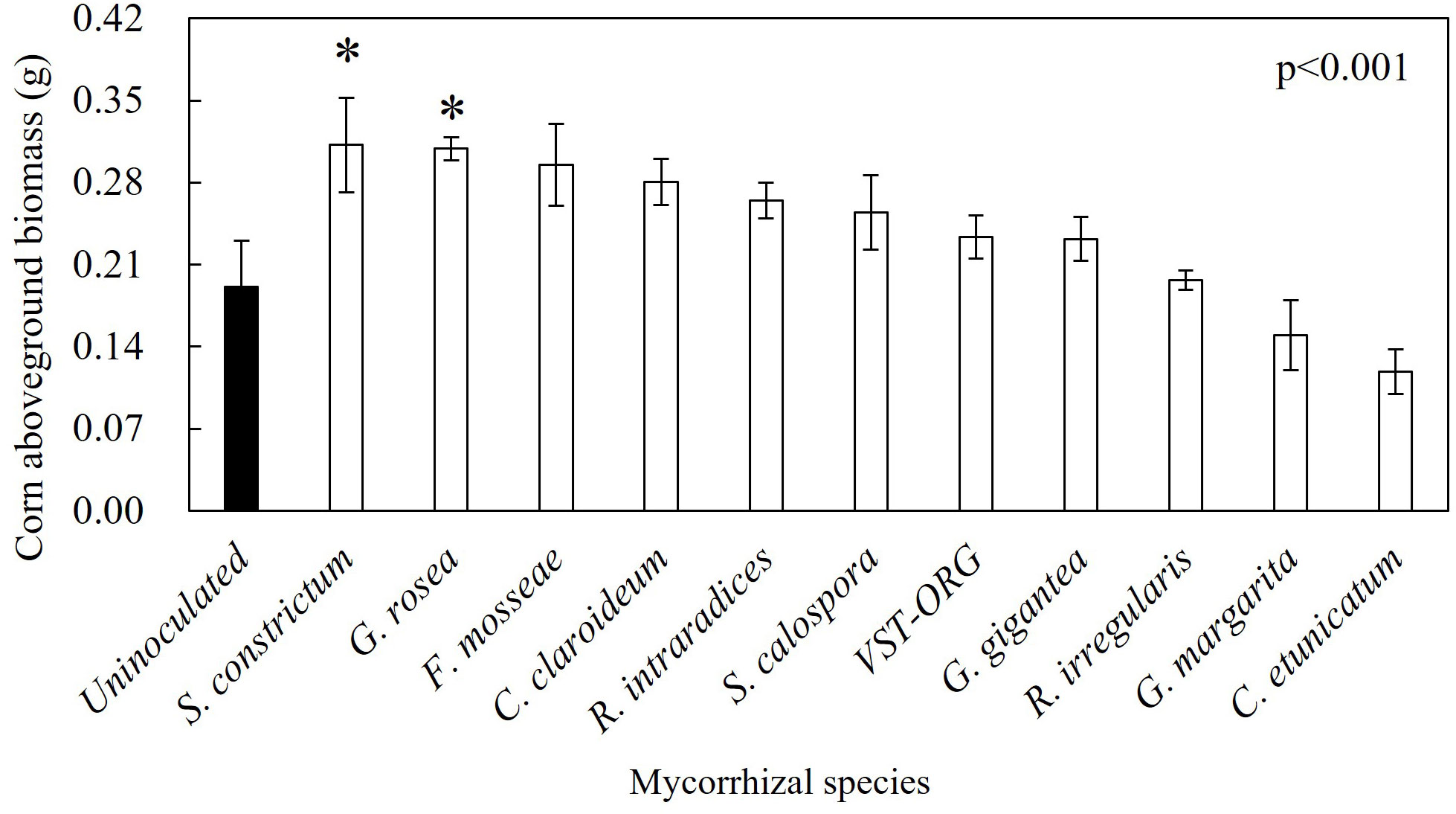
Figure 5 S. constrictum and G. rosea enhanced corn seedling aboveground biomass 30 days post germination. The closed bars are uninoculated seedlings and the open bars are inoculated seedlings. P values are from one-way ANOVA. Asterisks represent significant differences between uninoculated seedlings and inoculated seedlings.
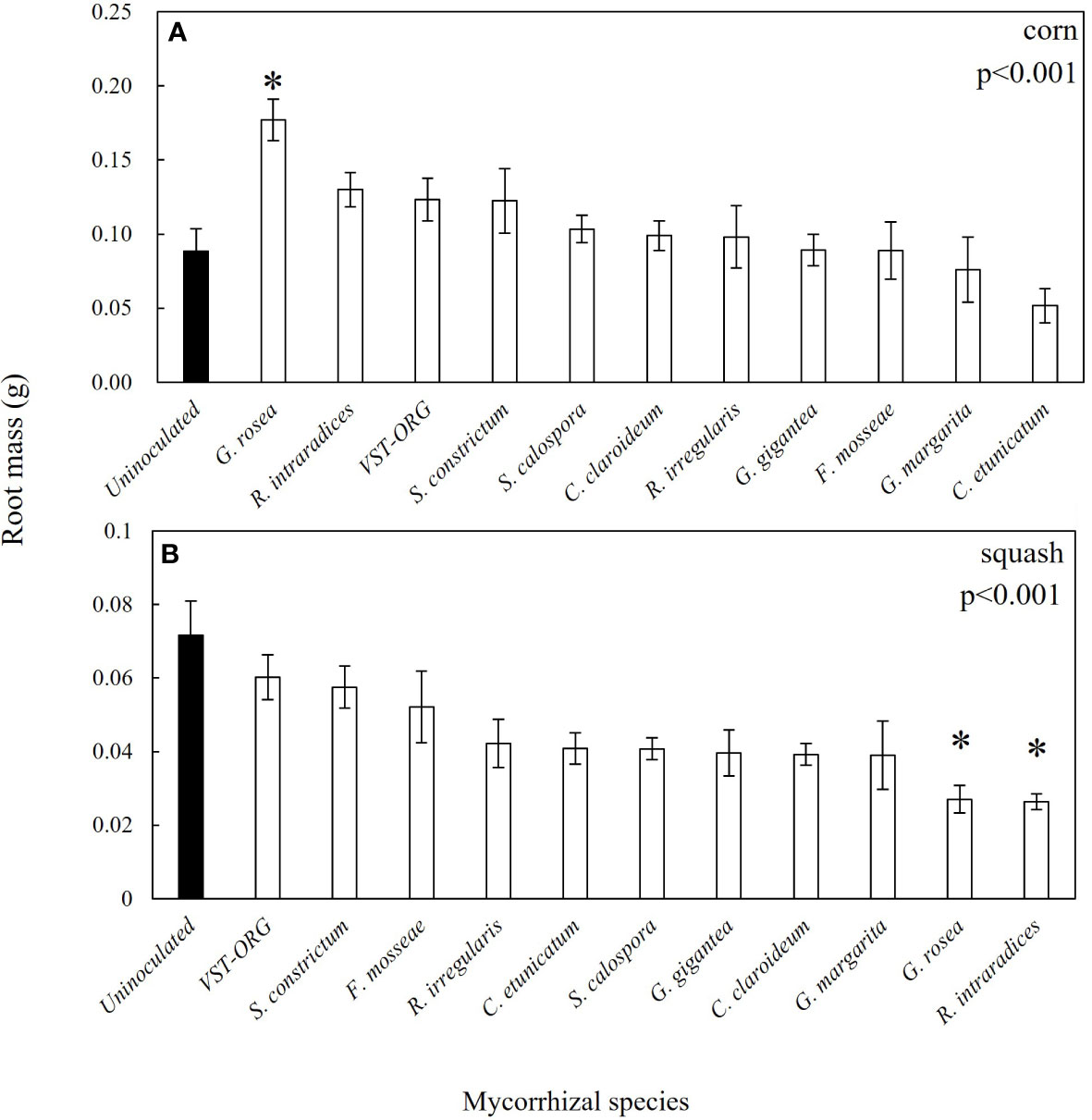
Figure 6 Root biomass of uninoculated and AMF inoculated corn (A) and squash (B) seedlings 30 days post germination. Most AMF inoculated squash seedlings had less root biomass than the uninoculated seedlings. The closed bars are uninoculated seedlings and the open bars are inoculated seedlings. P values are from the Kruskall Wallace H test. Asterisks represent significant differences between uninoculated seedlings and inoculated seedlings.
Discussion
Here we show that AMF inoculation is a promising candidate as an alternative to traditional fertilizers. We found that different mycorrhizal species have a range of efficiencies in colonizing corn and squash seedlings and appear to vary in their ability to enhance nutrient uptake efficiency between hosts. Specifically, we found that while inoculation with certain AMF species (namely those in the Rhizophagus genus) enhanced P uptake, other AMF species appear to benefit Ca and Mg uptake and biomass accumulation.
The wide range of colonization efficiencies across AMF species was possibly due differences in the plant carbon cost of nutrient acquisition between AMF species. In other words, because plants control the amount of C allocated to mycorrhizae to maximize nutrient return on C investment, mycorrhizal species with higher efficiencies of nutrient acquisition and lower C costs will have a greater benefit to plant nutrient status (Nouri et al., 2014). Supporting this mechanism, tissue culture studies have reported an inverse relationship between P availability and root-exudate production and mycorrhizal hyphal branching (Nagahashi et al., 1996). At a broader scale, this mechanism is evidenced by strong reductions in colonization under P and N fertilization across field studies (Treseder, 2004; Jeske et al., 2018; Han et al., 2020). As each inoculum had a similar initial number of AMF propagules (i.e. spores and root fragments), it is clear that species in the Rhizophagus genus have a distinct advantage over other AMF species in maintaining mycorrhizal relationships with both hosts in this study. This is consistent with recent research that showed that evolutionarily younger genera of AMF including Rhizophagus, outperformed more ancient evolutionary lineages (including all other genera used here) in colonization and P concentration in leeks (Säle et al., 2021). This advantage appears to be driven by the foraging and transfer efficiency of P of this genus (Figure 4). These results are further in line with past work that showed Rhizophagus have a unique ability to exploit P nutrient patches over other AMF species including F. mosseae and G. margarita (Cavagnaro et al., 2005). In fact, across all treatments we found a positive relationship between percent root colonization and aboveground tissue P concentration in both squash and corn suggesting that plants actively allocated C to mycorrhizal colonization to alleviate P limitation, regardless of species (Figure 3A, B). In an alternative experimental design, for instance, if N was limited, we may expect to see a similar relationship between colonization and aboveground tissue N concentration.
While Rhizophagus species appear to enhance the uptake of the immobile nutrient P in both species, C. etunicatum and G. margarita increased the uptake of soluble nutrients Mg and Ca in corn (R. irregularis also enhanced Mg to a lesser extent; Table 2). In squash, Ca was enhanced by G. rosea (Table 2). While most studies focus on mycorrhizal foraging capacity of insoluble nutrients, these results are consistent with previous studies that showed enhancement of Mg and Ca concentration of corn (as well as beans and tomatoes) grown with indigenous AMF communities compared to sterile soil (Buttery et al., 1988; El-Shanshoury et al., 1989; Kothari et al., 1990; Liu et al., 2000; Zare-Maivan et al., 2017). Unlike P, soluble nutrients are mostly taken up by plants through mass-flow as water is absorbed into root systems (Liu et al., 2000). AMF have been shown to contribute to plant water status and enhance water flow through increased belowground surface area and access to water in pore spaces not accessible to roots (Delavaux et al., 2017). While the morphological attributes of hyphal networks of different AMF species have not been well characterized, and hyphal density was not measured in this study, past research has shown that plants inoculated with different species of AMF exhibit a range of water uptake efficiencies with F. mosseae inoculated plants (formerly G. mosseae) being more efficient than some others (Ruiz‐Lozano and Azcón, 1995; Marulanda et al., 2003). There are two possible explanations as to why C. etunicatum, G. margarita, and G. rosea enhanced Ca and Mg uptake to a greater extent than other species. First, it is possible that these species have a physical advantage in water access over other species (i.e., more surface area per unit mass, smaller diameter) Second, these species may have higher rates of direct transfer of water from hyphae to roots. Regardless of the mechanism, these species appear to positively contribute to plant uptake of soluble nutrients.
Using a similar approach as (Bodenhausen et al., 2021), we used qPCR to measure the relative quantity of AMF DNA to plant DNA at two sampling points after germination of sweet corn seeds in inoculated soil. We were able to use this approach for quantification of four of our AMF inocula, which were readily detectable by qPCR, and only on corn (not squash) as a plant gene reference assay was not available for C. moschata. Consistent with the increased colonization observed by root staining (Figure 1A), F. mosseae DNA was 10-fold higher at 30 days compared to 15 days for seedlings inoculated with that species (Figure 2A), indicating that the increase in DNA quantity may be due to overall increase in fungal biomass. However, for C. etunicatum, the 5.8-fold increase detected in fungal DNA was not matched with an increase in colonization, which could possibly have been caused by growth of extraradical mycelium that did not coincide with growth within the roots. The relative quantification of the two Rhizophagus spp. changed the least between days 15 and 30. For R. intraradices, the relative quantity was reduced by about half on the later date. More experimentation is necessary to further investigate the significance of these findings, but under these conditions with this cultivar of corn and these fungal isolates, colonization by both of the Rhizophagus spp. occurred most rapidly and reached saturation after 15 days growth, whereas dramatic increases in colonization and biomass of F. mosseae were detected at 30 days relative to 15 days by both staining and molecular methods, and increases in C. etunicatum growth were observed by the molecular method only.
Interestingly, there was no relationship between percent mycorrhizal colonization and aboveground or root biomass accumulation in either corn or squash. In fact, although Rhizophagus enhanced P uptake in corn and squash, these seedlings were not larger than uninoculated seedlings (Figures 4, 5). This finding is consistent with other studies that show AMF can enhance P uptake with no impact on growth in cucumbers, flax, tomato, and medic (Pearson and Jakobsen, 1993; Smith et al., 2003; Smith et al., 2004). While many field trials show positive growth responses to mixed AMF inoculum (Nagahashi et al., 1996; Douds Jr. and Reider, 2003; Douds Jr. et al., 2007; Douds Jr. et al., 2008; Douds Jr. et al., 2015; Douds Jr. et al., 2017), the mixed inoculum used in this study did not appear to impact above or belowground growth during the first 30 days of plant growth. Only S. constrictum and G. rosea inoculated seedlings yielded more biomass than uninoculated seedlings in corn. It is possible that the lack of a growth response in corn and squash across most AMF species in this study is due to the seedlings becoming root-bound by day 30. On the other hand, we found lower root biomass in R. intraradices and G. rosea inoculated squash compared to uninoculated squash which may reflect a shift in C investment from root production toward mycorrhizal colonization in order to obtain P. One reason why corn and root biomass had divergent responses to AMF may be differences in root growth rates and root architecture between these hosts (Postma and Lynch, 2012; Zhang et al., 2014). Field studies are necessary to determine the extent to which differences in early nutrient uptake and biomass accumulation between AMF treatments translate to fruit production and yield at harvest.
Based on these findings, not all AMF species are equal in their ability to increase colonization, nutrient uptake, and biomass accumulation in squash and corn. In addition, we show that AMF species that may improve yield or nutrient status of one host may have no benefit to a different host. This work builds on past research that showed mixed-species on-farm produced AMF biofertilizers enhance the yield of many crops and suggests that future development of AMF fertilizer products will benefit from trials that determine host-specific AMF species. As AMF functional traits vary between soil types and ecosystems, farmers will benefit by testing different cultures to maximize the effectiveness of AMF species in nutrient uptake at local scales. This work has been used to inform an AMF inoculation field trial, wherein the most beneficial AMF species to corn and squash as determined here was selected for inoculation of seedlings for out-planting in conventional and organic farming systems.
Data availability statement
The original contributions presented in the study are included in the article/Supplementary Material. Further inquiries can be directed to the corresponding author.
Author contributions
WH designed the study. WH and JC collected, analyzed, and interpreted the data. JC wrote the first draft and both authors edited subsequent versions. All authors contributed to the article and approved the submitted version.
Funding
This study was funded by USDA-ARS in-house project 8072–12000-013–000-D.
Acknowledgments
We would like to thank The Rodale Institute for providing greenhouse space for this study. We additionally thank Lindsay McKeever and Jacob Lucabeche for technical assistance.
Conflict of interest
The authors declare that the research was conducted in the absence of any commercial or financial relationships that could be construed as a potential conflict of interest.
Publisher’s note
All claims expressed in this article are solely those of the authors and do not necessarily represent those of their affiliated organizations, or those of the publisher, the editors and the reviewers. Any product that may be evaluated in this article, or claim that may be made by its manufacturer, is not guaranteed or endorsed by the publisher.
Supplementary material
The Supplementary Material for this article can be found online at: https://www.frontiersin.org/articles/10.3389/fagro.2022.1040054/full#supplementary-material
References
Basiru S., Mwanza H. P., Hijri M. (2020). Analysis of arbuscular mycorrhizal fungal inoculant benchmarks. Microorganisms 9 (1), 81. doi: 10.3390/microorganisms9010081
Berruti A., Borriello R., Della Beffa M. T., Scariot V., Bianciotto V. (2013). Application of nonspecific commercial AMF inocula results in poor mycorrhization in camellia japonica l. Symbiosis 61 (2), 63–76. doi: 10.1007/s13199-013-0258-7
Berruti A., Lumini E., Balestrini R., Bianciotto V. (2016). Arbuscular mycorrhizal fungi as natural biofertilizers: let's benefit from past successes. Front. Microbiol. 6, 1559. doi: 10.3389/fmicb.2015.01559
Bodenhausen N., Deslandes-Hérold G., Waelchli J., Held A., van der Heijden M. G., Schlaeppi K. (2021). Relative qPCR to quantify colonization of plant roots by arbuscular mycorrhizal fungi. Mycorrhiza 31 (2), 137–148. doi: 10.1007/s00572-020-01014-1
Brundrett M. C., Tedersoo L. (2018). Evolutionary history of mycorrhizal symbioses and global host plant diversity. New Phytol. 220 (4), 1108–1115. doi: 10.1111/nph.14976
Buttery B., Park S., Findlay W., Dhanvantari B. (1988). Effects of fumigation and fertilizer on growth, yield, chemical composition, and mycorrhizae in white bean and soybean. Can. J. Plant Sci. 68 (3), 677–686. doi: 10.4141/cjps88-081
Cavagnaro T., Smith F. A., Smith S., Jakobsen I. (2005). Functional diversity in arbuscular mycorrhizas: Exploitation of soil patches with different phosphate enrichment differs among fungal species. Plant Cell Environ. 28 (5), 642–650. doi: 10.1111/j.1365-3040.2005.01310.x
Corkidi L., Allen E. B., Merhaut D., Allen M. F., Downer J., Bohn J., et al. (2004). Assessing the infectivity of commercial mycorrhizal inoculants in plant nursery conditions. J. Environ. Hortic. 22 (3), 149–154. doi: 10.24266/0738-2898-22.3.149
Delavaux C. S., Smith-Ramesh L. M., Kuebbing S. E. (2017). Beyond nutrients: a meta-analysis of the diverse effects of arbuscular mycorrhizal fungi on plants and soils. Ecology 98 (8), 2111–2119. doi: 10.1002/ecy.1892
Douds D. Jr., Carr E., Shenk J., Ganser S. (2017). Positive yield response of eggplant (Solanum melongena l.) to inoculation with AM fungi produced on-farm. Sci. Hortic. 224, 48–52. doi: 10.1016/j.scienta.2017.05.017
Douds D. Jr., Lee J., Shenk J., Ganser S. (2015). Inoculation of sweet potatoes with AM fungi produced on-farm increases yield in high p soil. J. Appl. Hortic. 17 (3), 171–175. doi: 10.37855/jah.2015.v17i03.32
Douds D. D. Jr., Nagahashi G., Hepperly P. R. (2010). On-farm production of inoculum of indigenous arbuscular mycorrhizal fungi and assessment of diluents of compost for inoculum production. Bioresour Technol. 101 (7), 2326–2330. doi: 10.1016/j.biortech.2009.11.071
Douds D. Jr., Nagahashi G., Pfeffer P., Kayser W., Reider C. (2005). On-farm production and utilization of arbuscular mycorrhizal fungus inoculum. Can. J. Plant Sci. 85 (1), 15–21. doi: 10.4141/P03-168
Douds D. D. Jr., Nagahashi G., Reider C., Hepperly P. R. (2007). Inoculation with arbuscular mycorrhizal fungi increases the yield of potatoes in a high p soil. Biol. Agric. Hortic. 25 (1), 67–78. doi: 10.1080/01448765.2007.10823209
Douds D. D. Jr., Nagahashi G., Shenk J. E., Demchak K. (2008). Inoculation of strawberries with AM fungi produced on-farm increased yield. Biol. Agric. Hortic. 26 (3), 209–219. doi: 10.1080/01448765.2008.9755084
Douds D. Jr., Reider C. (2003). Inoculation with mycorrhizal fungi increases the yield of green peppers in a high p soil. Biol. Agric. Hortic. 21 (1), 91–102. doi: 10.1080/01448765.2003.9755251
El-Shanshoury A., Hassan M., Abdel-Ghaffar B. (1989). Synergistic effect of vesicular-arbuscular mycorrhizas and azotobacter chroococcum on the growth and the nutrient contents of tomato plants. Phyton. 29, 203–212.
Faye A., Dalpé Y., Ndung'u-Magiroi K., Jefwa J., Ndoye I., Diouf M., et al. (2013). Evaluation of commercial arbuscular mycorrhizal inoculants. Can. J. Plant Sci. 93 (6), 1201–1208. doi: 10.4141/cjps2013-326
Giovannetti M., Mosse B. (1980). An evaluation of techniques for measuring vesicular arbuscular mycorrhizal infection in roots. New Phytol. 84(3):489–500. doi: 10.1111/j.1469-8137.1980.tb04556.x
Han Y., Feng J., Han M., Zhu B. (2020). Responses of arbuscular mycorrhizal fungi to nitrogen addition: A meta-analysis. Global Change Biol. 26 (12), 7229–7241. doi: 10.1111/gcb.15369
Heller W. P., Carrara J. E. (2022). Multiplex qPCR assays to distinguish individual species of arbuscular mycorrhizal fungi from roots and soil. Mycorrhiza 32 (2), 155–164. doi: 10.1007/s00572-022-01069-2
Hoagland D. R., Arnon D. I. (1950). The water-culture method for growing plants without soil. Circular. California Agric. experiment Station 347.
Huang C. Y. L., Schulte E. (1985). Digestion of plant tissue for analysis by ICP emission spectroscopy. Commun. Soil Sci. Plant Anal. 16 (9), 943–958. doi: 10.1080/00103628509367657
Igiehon N. O., Babalola O. O. (2017). Biofertilizers and sustainable agriculture: Exploring arbuscular mycorrhizal fungi. Appl. Microbiol. Biotechnol. 101 (12), 4871–4881. doi: 10.1007/s00253-017-8344-z
Jerbi M., Labidi S., Laruelle F., Tisserant B., Jeddi F. B., Sahraoui A. L.-H. (2022). Mycorrhizal biofertilization improves grain yield and quality of hulless barley (Hordeum vulgare ssp. nudum l.) under water stress conditions. J. Cereal Sci. 104, 103436. doi: 10.1016/j.jcs.2022.103436
Jeske E. S., Tian H., Hanford K., Walters D. T., Drijber R. A. (2018) Long-term nitrogen fertilization reduces extraradical biomass of arbuscular mycorrhizae in a maize (Zea mays l.) cropping system. Agriculture Ecosyst. Environ. 255, 111–118. doi: 10.1016/j.agee.2017.11.014
Keymer A., Pimprikar P., Wewer V., Huber C., Brands M., Bucerius S. L., et al. (2017). Lipid transfer from plants to arbuscular mycorrhiza fungi. elife 6, e29107. doi: 10.7554/eLife.29107.051
Kothari S., Marschner H., Römheld V. (1990). Direct and indirect effects of VA mycorrhizal fungi and rhizosphere microorganisms on acquisition of mineral nutrients by maize (Zea mays l.) in a calcareous soil. New Phytol. 116 (4), 637–645. doi: 10.1111/j.1469-8137.1990.tb00549.x
Liu A., Hamel C., Hamilton R., Ma B., Smith D. (2000). Acquisition of Cu, zn, Mn and fe by mycorrhizal maize (Zea mays l.) grown in soil at different p and micronutrient levels. Mycorrhiza 9 (6), 331–336. doi: 10.1007/s005720050277
Madawala H. (2021). Arbuscular mycorrhizal fungi as biofertilizers: Current trends, challenges, and future prospects. Biofertilizers 1, 83–93. doi: 10.1016/B978-0-12-821667-5.00029-4
Marulanda A., Azcon R., Ruiz-Lozano J. M. (2003). Contribution of six arbuscular mycorrhizal fungal isolates to water uptake by lactuca sativa plants under drought stress. Physiol. Plantarum 119 (4), 526–533. doi: 10.1046/j.1399-3054.2003.00196.x
Nagahashi G., Douds D. Jr., Abney G. (1996). Phosphorus amendment inhibits hyphal branching of the VAM fungus gigaspora margarita directly and indirectly through its effect on root exudation. Mycorrhiza 6 (5), 403–408. doi: 10.1007/s005720050139
Nouri E., Breuillin-Sessoms F., Feller U., Reinhardt D. (2014). Phosphorus and nitrogen regulate arbuscular mycorrhizal symbiosis in petunia hybrida. PloS One 9 (3), e90841. doi: 10.1371/journal.pone.0090841
Pearson J., Jakobsen I. (1993). The relative contribution of hyphae and roots to phosphorus uptake by arbuscular mycorrhizal plants, measured by dual labelling with 32P and 33P. New Phytol. 124 (3), 489–494. doi: 10.1111/j.1469-8137.1993.tb03840.x
Phillips J. M., Hayman D. (1970). Improved procedures for clearing roots and staining parasitic and vesicular-arbuscular mycorrhizal fungi for rapid assessment of infection. Trans. Br. mycol. Soc. 55 (1), 158–IN118. doi: 10.1016/S0007-1536(70)80110-3
Postma J. A., Lynch J. P. (2012). Complementarity in root architecture for nutrient uptake in ancient maize/bean and maize/bean/squash polycultures. Ann. Bot. 110 (2), 521–534. doi: 10.1093/aob/mcs082
Read D., Perez-Moreno J. (2003). Mycorrhizas and nutrient cycling in ecosystems–a journey towards relevance? New Phytol. 157 (3), 475–492. doi: 10.1046/j.1469-8137.2003.00704.x
R Studio Team (2020). RStudio: Integrated Development for R. RStudio, PBC, Boston, MA. Available at: http://www.rstudio.com/.
Ruiz-Lozano J., Azcón R. (1995). Hyphal contribution to water uptake in mycorrhizal plants as affected by the fungal species and water status. Physiol. Plantarum 95 (3), 472–478. doi: 10.1111/j.1399-3054.1995.tb00865.x
Sadhana B. (2014). Arbuscular mycorrhizal fungi (AMF) as a biofertilizer-a review. Int. J. Curr. Microbiol. App. Sci. 3 (4), 384–400.
Säle V., Palenzuela J., Azcón-Aguilar C., Sánchez-Castro I., da Silva G. A., Seitz B., et al. (2021). Ancient lineages of arbuscular mycorrhizal fungi provide little plant benefit. Mycorrhiza 31 (5), 559–576. doi: 10.1007/s00572-021-01042-5
Salomon M., Demarmels R., Watts-Williams S., McLaughlin M., Kafle A., Ketelsen C., et al. (2022). Global evaluation of commercial arbuscular mycorrhizal inoculants under greenhouse and field conditions. Appl. Soil Ecol. 169, 104225. doi: 10.1016/j.apsoil.2021.104225
Scholdberg T. A., Norden T. D., Nelson D. D., Jenkins G. R. (2009). Evaluating precision and accuracy when quantifying different endogenous control reference genes in maize using real-time PCR. J. Agric. Food Chem. 57 (7), 2903–2911. doi: 10.1021/jf803599t
Smith S. E., Smith F. A., Jakobsen I. (2003). Mycorrhizal fungi can dominate phosphate supply to plants irrespective of growth responses. Plant Physiol. 133 (1), 16–20. doi: 10.1104/pp.103.024380
Smith S. E., Smith F. A., Jakobsen I. (2004). Functional diversity in arbuscular mycorrhizal (AM) symbioses: The contribution of the mycorrhizal p uptake pathway is not correlated with mycorrhizal responses in growth or total p uptake. New Phytol. 162 (2), 511–524. doi: 10.1111/j.1469-8137.2004.01039.x
Tarbell T. J., Koske R. E. (2007). Evaluation of commercial arbuscular mycorrhizal inocula in a sand/peat medium. Mycorrhiza 18 (1), 51–56. doi: 10.1007/s00572-007-0152-3
Treseder K. K. (2004). A meta-analysis of mycorrhizal responses to nitrogen, phosphorus, and atmospheric CO2 in field studies. New Phytol. 164 (2), 347–355. doi: 10.1111/j.1469-8137.2004.01159.x
Werner G. D., Kiers E. T. (2015). Partner selection in the mycorrhizal mutualism. New Phytol. 205 (4), 1437–1442. doi: 10.1111/nph.13113
Zare-Maivan H., Khanpour-Ardestani N., Ghanati F. (2017). Influence of mycorrhizal fungi on growth, chlorophyll content, and potassium and magnesium uptake in maize. J. Plant Nutr. 40 (14), 2026–2032. doi: 10.1080/01904167.2017.1346119
Keywords: mycorrihizae, biofertilizer, soil health, zea mays, cucurbita moschata, nutrient uptake
Citation: Carrara JE and Heller WP (2022) Arbuscular mycorrhizal species vary in their impact on nutrient uptake in sweet corn (Zea mays) and butternut squash (Cucurbita moschata). Front. Agron. 4:1040054. doi: 10.3389/fagro.2022.1040054
Received: 08 September 2022; Accepted: 04 October 2022;
Published: 26 October 2022.
Edited by:
Naser A. Anjum, Aligarh Muslim University, IndiaReviewed by:
Debasis Mitra, National Rice Research Institute (ICAR), IndiaRaghavendra Singh, Indian Institute of Pulses Research (ICAR), India
Copyright © 2022 Carrara and Heller. This is an open-access article distributed under the terms of the Creative Commons Attribution License (CC BY). The use, distribution or reproduction in other forums is permitted, provided the original author(s) and the copyright owner(s) are credited and that the original publication in this journal is cited, in accordance with accepted academic practice. No use, distribution or reproduction is permitted which does not comply with these terms.
*Correspondence: Wade P. Heller, wade.heller@usda.gov
†ORCID: Joseph E. Carrara, 0000-0003-0597-1175
Wade P. Heller, 0000-0002-5964-9715