- Department of Crop Science, North Carolina State University, Raleigh, NC, United States
Field experiments were conducted at two locations in North Carolina (Clayton and Rocky Mount) to determine the influence of row spacing and planting date on the critical period of weed control (CPWC) of grass weeds in ALS-tolerant grain. Grain sorghum was planted in May and June 2019, with either a 38 or 91 cm row spacing using an ALS-tolerant sorghum variety. Treatments consisted of “weedy” or “weed-free” plots up to 2, 3, 5, and 7 weeks after crop planting (WAP) and two control treatments of weedy and weed-free all season. Selection of grass weed species was achieved by controlling broadleaf weeds with a premix of bromoxynil plus pyrasulfutole at a rate of 264 g a.i. ha-1.Grass weeds were controlled using nicosulfuron at a rate of 69 g of a.i. ha-1. The CPWC was significantly different across locations. Row spacing and planting date factors did not influence the CPWC at Clayton. Planting date was a significant factor for the CPWC at Rocky Mount, however row spacing did not have any effect on the CPWC. Results for the CPWC are presented in terms growing degree days (GDD) from the date of crop sowing. The CPWC for grass weeds in grain sorghum at Clayton was from 368 to 849 GDD. The CPWC at Rocky Mount for May-planted grain sorghum was from 405 to 876 GDD, while the CPWC for June-planted grain sorghum ranged from 228 to 1042 GDD. These results demonstrate that cultural and environmental factors may influence the beginning, duration and end of the CPWC.
Introduction
Grain sorghum (Sorghum bicolor (L) Moench.) is a cereal crop grown in the United States primarily in semi-arid regions, where grain sorghum may be superior to other crops due to its ability to tolerate harsh environmental conditions of abiotic stress such as drought and heat (Stahlman and Wicks, 2000; Ottman and Olsen, 2009; Ghani et al., 2015). Sorghum can be used as an alternative to other grains used in livestock and poultry feeding programs due to its great nutritional value. However, despite grain sorghum’s potential for feed value and crop hardiness, the total worldwide area under grain sorghum production has declined, mainly due to difficulties faced with weed management (Kumar et al., 2012; USDA-NASS, 2016). In addition to other biotic and abiotic factors, weeds are a major constraint in grain sorghum, causing up to 97% yield losses (Peerzada et al., 2017). It is calculated that US farmers would lose 5.7 billion kg of grain sorghum valued at $953 million annually if weeds were not controlled (Dille et al., 2020). Most of the production costs of grain sorghum come from weed management, where US sorghum producers spend approximately $100 million dollars a year (Bridges, 1992; Walker et al., 2005).
Grain sorghum is a poor competitor against weeds due to slow growth and poor early vigor during the first month of growth (Rizzardi et al., 2004). In addition, the limited availability of soil moisture in dry environments where grain sorghum is typically produced can restrict the activation and reduce the efficacy of pre-emergence herbicides (Henning et al., 2010), forcing growers to depend on postemergence weed control options. Other complications encountered with weed management are the high cost of labeled herbicides for use on grain sorghum, likelihood of herbicidal resistance development, and lack of an adequate knowledge of appropriate timing of weed control (Gholami et al., 2013; Mishra et al., 2015). Most of the postemergence herbicides registered for sorghum are effective against broadleaf species, but have limited activity on grass weed species (Werle et al., 2016). Poaceae species are considered to be the most common and troublesome weeds for grain sorghum in the United States, particularly those species from the genus Urochloa, Echinochloa, Panicum, Digitaria and Sorghum (Elmore, 1998; Van Wychen, 2020). However, herbicides that control grasses in grain sorghum are limited as selective chemical control alternatives have not been readily available for producers. In the past, research on herbicide use and integration of mechanical and chemical approaches with cultural weed control strategies was focused on to help in solving these challenges (Hozayn et al., 2012).
The use of multiple crop management strategies has proven to have weed suppressive characteristics and help in the development of effective weed management strategies in sorghum (Mishra et al., 2015). Selection of a competitive crop cultivar should be the first step of an integrated weed management plan. Doing so is one way to potentially suppress weed growth without sacrificing crop yield (Frick, 2000). Another component of an integrated weed management plan includes planting at appropriate crop densities. Studies have shown that increased sorghum populations reduce light interception by weeds (Gholami et al., 2013; Besançon et al., 2017a). Increasing grain sorghum planting densities (207,000 plants ha-1) compared to lower densities (120,000 plants ha-1) reduce weed growth (Burnside, 1977; Besançon et al., 2017b). An additional step of integrated weed management is the selection of appropriate row width. In grain sorghum row spacing may range from 15 cm to 100 cm, with 76 cm being the most common, and it is planted with a variety of configuration, generally planted on beds or flats (Vanderlip et al., 1998; Ottman and Olsen, 2009; Hewitt, 2015; Besançon et al., 2017a). Studies have shown that reduced row spacing can increase sorghum’s ability to compete for incoming light more efficiently, increase crop productivity, and reduce weed emergence (Holt, 1995; Grichar et al., 2004; Besançon et al., 2017a). Row spacing arrangements of 45 cm can result in improved grain sorghum yields and reduced weed population (25-54%) as compared to 60-90 cm row spacing (Bishnoi et al., 1990). In heavily weed infested fields, narrow row spacing suppressed weed biomass and increased yield by 72% and 45% (Marin and Weiner, 2014). In semi-arid tropical regions, hand weeding and mechanical cultivation are the most common method for weed control in grain sorghum (Mishra et al., 2015). Unfortunately, labor scarcity and elevated mechanical equipment restricts these strategies on a larger scale (Peerzada et al., 2017).
An integrated weed management plan includes several cultural practices as well as herbicides. Growers typically depend on different chemical and non-chemical approaches for controlling weeds, but they predominantly focus on herbicides (Hozayn et al., 2012). Grass control in grain sorghum can be difficult due to limited options for selective control. Quinclorac was introduced for weed control in sorghum in 2013 (Anonymous, 2022). For some grass weed species it can be an effective herbicide when timely applications are done (at or before weeds reach 5 cm), as delayed applications may present inconsistent control (Vincent, 2015). Susceptible grass species include large crabgrass (Digitaria sanguinalis (L.) Scop.), broadleaf signalgrass (Urochloa platyphylla (Nash) R.D. Webster) and fall panicum (Panicum dichotomiflorum Michx.). Texas millet (Urochloa texana Buckl.), goosegrass (Eleusine indica (L.) Gaertn.), and crowfootgrass (Dactyloctenium aegyptium (L.) Willd.) are not controlled by quinclorac (Vincent, 2015). Acetolactate synthase (ALS) -inhibiting herbicides used for grass control are not used in conventional sorghum due to its susceptibility to them, however a wild sorghum accession with tolerance to ALS herbicides was identified in Kansas in 2005 (Tuinstra and Al-Khatib, 2007). The genetic trait was identified and introgressed from wild sorghum to develop ALS-tolerant grain sorghum hybrids adapted for use in sorghum-producing areas (Tuinstra and Al-Khatib, 2007; Tuinstra et al., 2009). DuPont® acquired the rights for this trait and labeled the technology as “Inzen” (Werle et al., 2017). Inzen sorghum varieties contain a double mutation in the ALS gene, Val560Ile and Trp574Leu (Tuinstra and Al-Khatib, 2007). The aforementioned confer tolerance to the sulfonylurea and imidazolinone families (Werle et al., 2016). Bowman et al. (2021) evaluated Inzen sorghum for cross resistance to multiple ALS-inhibitor herbicides and found it to survive herbicides from five chemical families: sulfonylureas, imidazolinones, pyrimidinylthiobenzoics, triazolinones, and triazolopyrimidines. The ALS-inhibiting herbicide active ingredients in this case were nicosulfuron and rimsulfuron. Studies have shown that both herbicides provide excellent control of grass weeds, and grain sorghum hybrids with tolerance to them have provided growers with an option of selective postemergence grass control (Henning et al., 2010).
Integrated weed management plans should include an appropriate timing for weed removal that results in effective weed control. Previous studies have indicated that during the first two weeks after crop emergence, weed competition usually does not reduce grain sorghum yields, regardless of weed species (Burnside et al., 1964; Feltner et al., 1969; Smith et al., 1990). Weed competition beyond two weeks after crop emergence may reduce yields depending on species and environmental conditions (Burnside et al., 1964; Feltner et al., 1969; Smith et al., 1990). In order to better protect potential yield, is important for grain sorghum producers to understand the periods where grass weed control is vital. The critical period of weed control (CPWC) explores the period in a crop’s growth in which weeds must be controlled to prevent considerable yield losses (Knezevic et al., 2002; Everman et al., 2008). The CPWC represents the time interval between two separately measured crop-weed competition components: the critical time for weed removal (CTWR) and the critical weed free period (CWFP). The CTWR conditions the maximum amount of time that early season weed competition can be tolerated by the crop before it suffers from an irreversible yield reduction (Knezevic and Datta, 2015). This is used to determine the beginning of the CPWC. Concurrently, the CWFP describes the minimum weed-free period required from the time of crop emergence to avoid unacceptable yield losses (Knezevic and Datta, 2015). Thus, the CWFP indicates the end of the CPWC. Both components are subjected to a regression approach to generate weedy and weed-free curves that depend on the level of acceptable yield loss (AYL) used to predict the beginning and the end of the CPWC (Knezevic and Datta, 2015). The majority of CPWC studies set the threshold at 5% AYL. Theoretically, weed competition outside of the CPWC does not contribute to crop relative yield loss greater than the set threshold.
The CPWC within a crop is subject to biological factors such as characteristics of the crop itself, weed species, and weed density as well as abiotic factors such as temperature, precipitation, and cultural practices implemented (Hall et al., 1992). There are multiple chemical options available for broadleaf weed control in grain sorghum, however options for grass weed control are limited. This study will focus on investigating the CPWC for grass weeds in ALS-tolerant grain sorghum in the Mid-Atlantic region due to a need for increased feed grains and a renewed interest in producing sorghum (Balota et al., 2018). Additionally, understanding the effect of cultural practices such as planting date, row spacing, and herbicide tolerant varieties may lead to a better understanding of the effect cultural practices have on the CPWC and could provide guidelines for integrated grass weed management programs in grain sorghum. Therefore, this study was designed with the objective to determine the CPWC of grass weeds in ALS-inhibitor tolerant grain sorghum as influenced by planting date and row spacing.
Materials and methods
Field studies were established at two locations to evaluate the effect of planting date and row spacing on the critical period of weed control for grasses in ALS-inhibitor tolerant grain sorghum during the summer of 2019. The experiments took place at the Central Crops Research Station at Clayton, NC (35.675094, -78.510803) and at the Upper Coastal Plains Research Station in Rocky Mount, NC (35.896962, -77.672884). The USDA web soil survey (USDA-NCRS 2020) designates the Clayton soil as a Dothan loamy sand (fine-loamy, kaolinitic, thermic Plinthic Kandiudult) and the Rocky Mount soil as a Rains fine sandy loam (fine-loamy, siliceous, semiactive, thermic Typic Paleaquult). Both sites were prepared for sowing under conventional tillage. The sorghum variety used was ADV G2250ALS (Advanta Seeds, Irving TX), which contained the Inzen sorghum trait which confers tolerance to ALS-inhibiting herbicides to the sulfonylurea family. Sorghum was planted at a rate of 270,000 seed ha-1 using two separate row spacing arrangements; 38 and 91 cm between rows. The experiment had two planting dates per site, the first planting date being May 15, 2019 at Rocky Mount and May 16, 2019 at Clayton. The second planting date was June 14, 2019 at Rocky Mount and June 15, 2019 at Clayton. These planting dates are henceforth referred to as May-planted and June-planted grain sorghum, respectively. Plots were 4 meters wide by 9 meters long. Standard management practices were followed regarding fertilization and insect control following guidelines by the Grain Sorghum Best Management Practices for Mid-Atlantic Production (2016). The experiment plots were not supplied with additional irrigation other than rainfall.
The experiment was established in a factorial treatment arrangement with factors consisting of two planting dates, two row widths, and eight weed interference periods established as a randomized complete block replicated four times. Weed interference periods hereby referred to as “treatments” were comprised of periods consisting of 2, 3, 5 and 7 weeks after crop planting (WAP), resulting in eight “weedy” and “weed-free” treatments. In the weedy treatments, grasses were allowed to grow in the plot up to the corresponding treatment WAP, when it was treated with nicosulfuron at a rate of 69 g of a.i. ha-1 and maintained weed-free for the remainder of the growing season. In weed-free treatments, plots were kept weed free by manual removal of emerging weeds after which they were permitted to grow for the rest of the season. Additionally, there were two control treatments for each factor consisting of weedy and weed-free all-season plots, where grasses were either allowed to grow in the plots or controlled during the entire season. Grasses were controlled with nicosulfuron at a rate of 69 g of a.i. ha-1 using a CO2 pressurized backpack sprayed calibrated for an output of 140 L ha-1 at 207 kPa with TeeJet flat fan XR11002 nozzles when treatment applications were required. Grass weed selection was done controlling broadleaf weed species with a premix of bromoxynil plus pyrasulfutole at a rate of 264 g a.i. ha-1 when weeds were less than 5 cm tall. Weed density data were collected from the critical time of weed removal plots by subsampling the plot with a 0.25m2 square at each removal timing. Weeds were counted by isolating the crown of the plant to ensure individual plants were accounted for. Grain sorghum was harvested using a small plot combine at the end of the season and adjusted to a 12.5% moisture content to obtain total yields. Yields were then standardized based on percentage to obtain relative yield, with the weed-free all season treatment indicating 100% yield
Data analysis
Data for grass weed densities and relative yield were subjected to an ANOVA using PROC GLM in SAS 9.4 (SAS Institute, Cary, NC 27513) to test the statistical significance (p < 0.05) of location, planting date, row spacing arrangement, treatments and their interactions (Knezevic et al., 2007; Knezevic and Datta, 2015). A significant location effect for relative yield was detected therefore subsequent statistical analysis were performed separately for both locations (Clayton and Rocky Mount). There were no significant differences for planting date or row spacing at Clayton thus data for the CPWC of this location are combined. At Rocky Mount a significant planting date by treatment interaction was detected therefore data are presented separately by planting date. Regression analysis of the CPWC was performed with R software (R Core Team, 2019) using statistical package drc (dose-response curve) (Ritz and Streibig, 2005). Treatment comparison was based on a non-linear regression analysis in which timing of weed removal and weed-free periods were associated to relative yield. Curves were fit to a four-parameter log-logistic model after which parameter estimates were combined and tested for significance, where differences in parameter estimates indicate different curves. The log logistic function used is given in Equation 1:
Y indicates relative yield as a percentage, C is the lower limit, D is the upper limit, X is the time expressed as WAP and E is the WAP giving a 50% response between upper and lower limits, also known as the inflection point or ED50 (Knezevic and Datta, 2015). The CTWR and CWFP curves were graphically represented using a four-parameter logistic regression model and a four-parameter Gompertz model in Sigmaplot version 14.0 (Systat Software, San Jose, CA), both appropriate for graphical representation of weedy and weed-free curves (Knezevic et al., 2002).
The duration of the CPWC is presented in growing degree days following recommendations of previous studies (Elezovic et al., 2012; Knezevic et al., 2013; Knezevic and Datta, 2015). The formula to calculate GDD is presented in Equation 2:
Where Tmin and Tmax are the daily minimum and maximum temperatures, respectively, and Tb is the base temperature of 10° C (USDA, 2022).
Results
Grass weed density
Evaluations of grass weed densities represent the species present at each site at different timings of weed removals corresponding to the experiment sampling date (at 2, 3, 5 and 7 WAP). Both locations’ grass weeds composition consisted of multiple species at each location (Table 1). Species composition varied across locations with the exception of large crabgrass and goosegrass which were present at both Clayton and Rocky Mount.
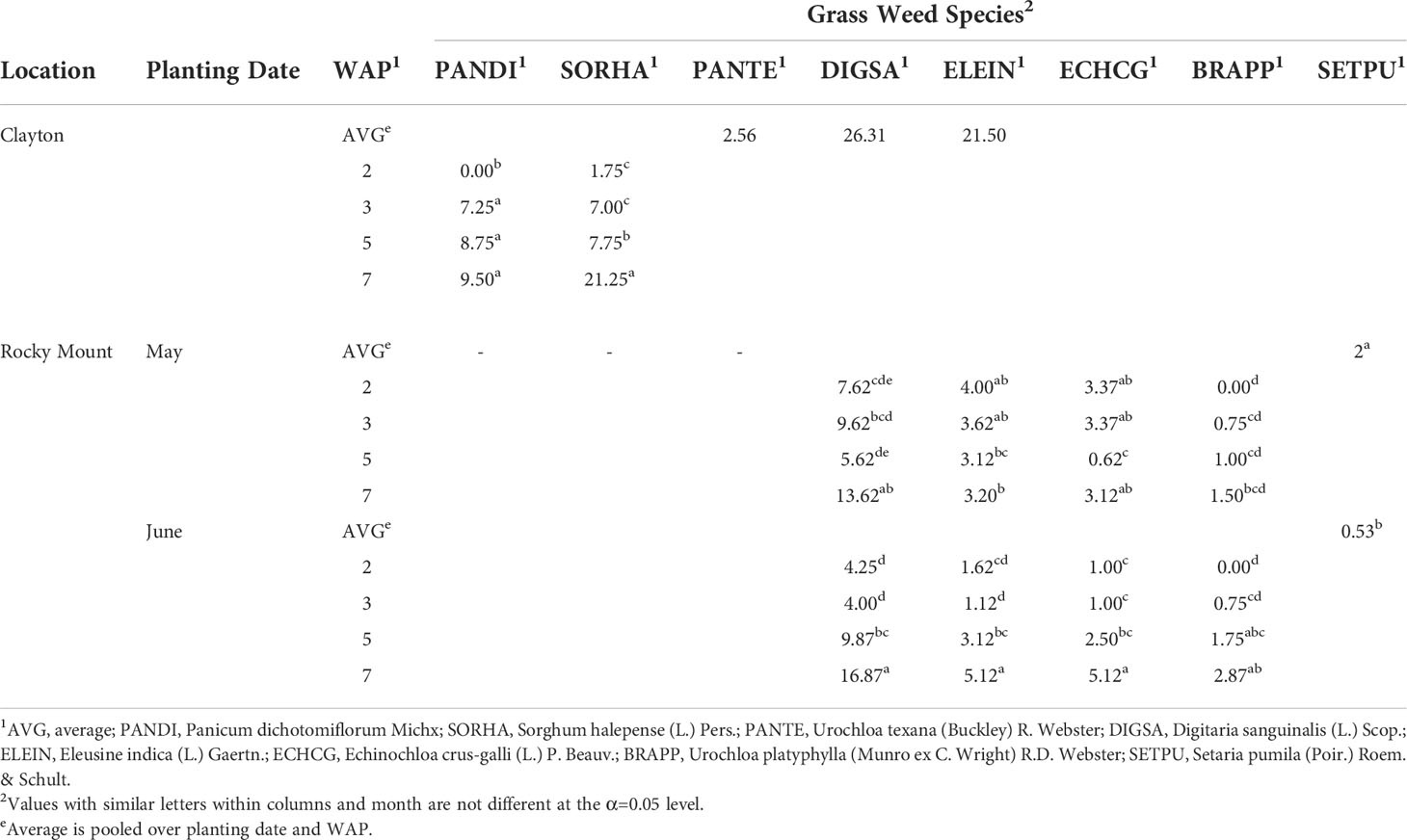
Table 1 Grass weed species densities (plants per square meter) at Clayton and Rocky Mount over weeks after planting (WAP). Grass weed species listed in BAYER codes as follow:
An ANOVA was conducted by species and location to understand if planting date or row spacing arrangement had effects on grass weed density (Table 1). None of the locations in this experiment presented a row spacing effect on any grass weed species density, therefore data were pooled across row spacing arrangements. Overall grass densities were greatest at the Clayton location, with greater than 80 plants per m2 at 7 WAP while densities reached 30 per m2 at Rocky Mount at the same collection date.
At Clayton, Texas millet, large crabgrass and goosegrass had no differences in weed density due to planting date throughout 7 weeks after planting (WAP), therefore data presented is pooled over these factors (Table 1). Fall panicum (Panicum dichotomiflorum Michx.) and johnsongrass density were significantly affected by sampling date, but no planting date effect was observed. There was no fall panicum emergence at 2 WAP, however it was found to have similar densities at 3, 5 and 7 WAP. Similarly, johnsongrass density was lowest at 2 WAP, then increased and stayed consistent at 3 and 5 WAP before it increased around 3 times as much density at 7 WAP.
At Rocky Mount, all but one species had a sampling date by planting date interaction effect, therefore data is presented by planting date and sampling date (Table 1). Yellow foxtail density (Setaria pumila [Poir.] Roem. & Schult.) did not have a planting date by sampling date interaction. However, yellow foxtail did have a planting date effect, therefore it is presented pooled over sampling date but separated by planting date. Yellow foxtail density was greater in the May planting date. For both planting dates, large crabgrass density was similar at the early sample dates then increased as the season progressed with greatest density at 7 WAP. Goosegrass densities were consistent and not significantly different for all treatments with a May planting date. However densities in the June planting date were significantly lower at 2 and 3 WAP and increased until reaching the greatest density at 7 WAP. Barnyardgrass (Echinochloa crus-galli [L.] P. Beauv.) densities were consistent at all sampling dates in the May planting date. Barnyardgrass densities in the June planting date were lower compared to the May planting date at 2 and 3 WAP, but they follow an increasing trend through 5 and 7 WAP. Broadleaf signalgrass was absent in both planting dates at 2 WAP, however emergence occurred by 3 WAP and densities increased through 7 WAP.
Grain sorghum yield loss due to weed interference and CPWC
Grain sorghum yield is expressed as relative yield in terms of percentage, where yield from plots kept weed-free throughout the growing season represent 100% relative yield. Both locations had grain sorghum yield reductions with continued duration of grass weed interference with the crop. In the experiment conducted at Clayton, total yield losses for uncontrolled grass weed growth reached up to 100% of relative yield (Figure 1). At Rocky Mount, grain sorghum yield loss due to uncontrolled grass weed growth all season ranged between 55 to 85% (Figure 2).
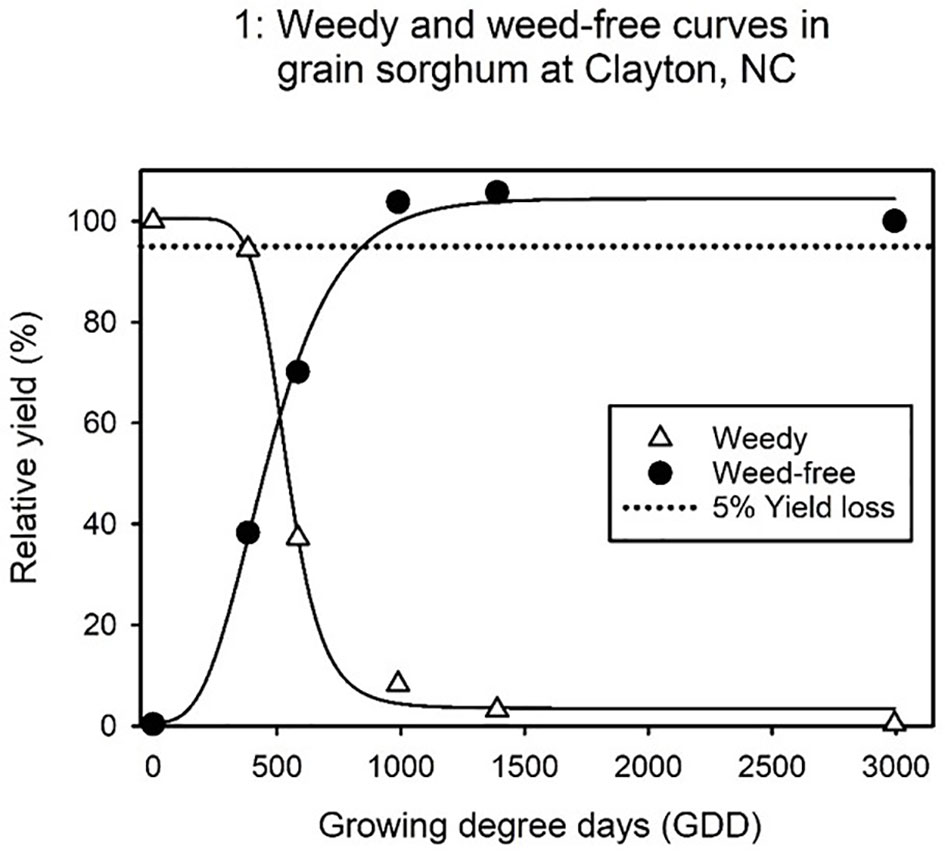
Figure 1 ALS-tolerant grain sorghum relative yield (%) as influenced by the timing of grass weed removal and the extent of the weed-free period, expressed in growing degree days (GDD). Data fit to a four-parameter log-logistic model (Equation 1) results in the weedy and weed free curves at Clayton. An acceptable 5% yield loss reference line is added to provide a visual representation of the critical timing of weed removal (CTWR) and the critical weed free period (CWFP) which encompass the critical period for weed control (CPWC).
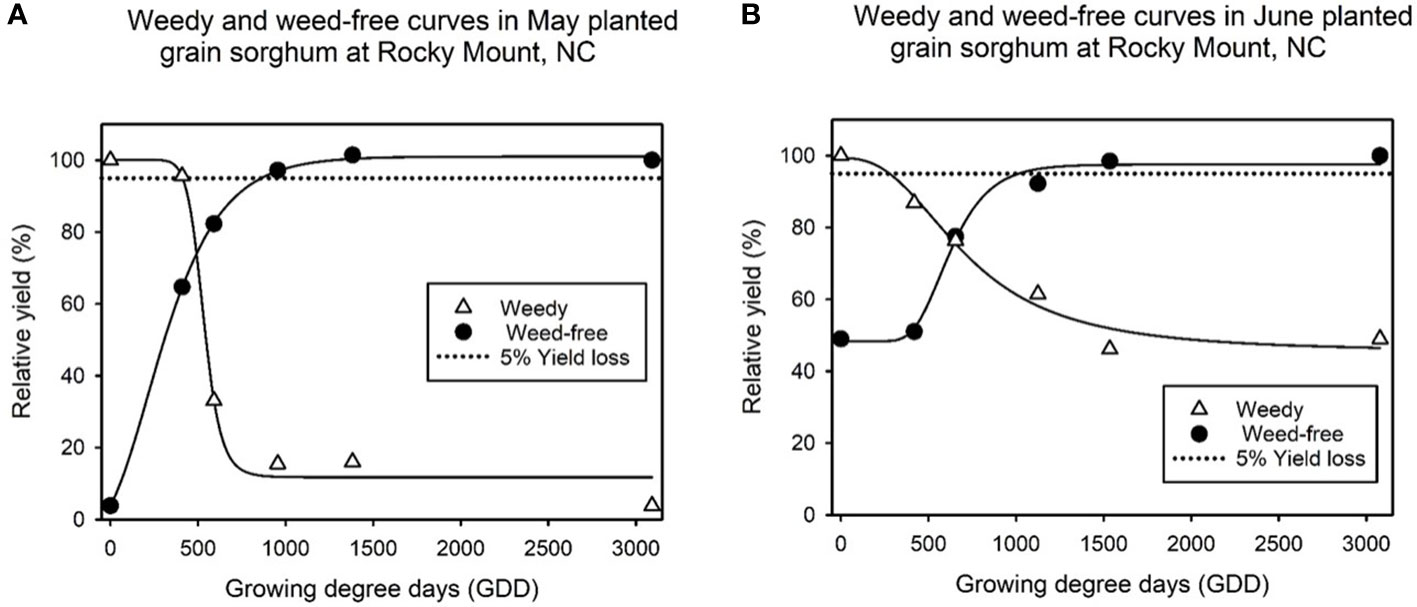
Figure 2 ALS-tolerant grain sorghum relative yield (%) as influenced by the timing of grass weed removal and the extent of the weed-free period, expressed in growing degree days (GDD). Data fit to a four-parameter log-logistic model (Equation 1) results in the weedy and weed free curves at (A) Rocky Mount planted in May and (B) Rocky Mount planted in June. An acceptable 5% yield loss reference line is added to provide a visual representation of the critical timing of weed removal (CTWR) and the critical weed free period (CWFP) which encompass the critical period for weed control (CPWC).
Critical period for weed control
The CPWC for grass weed species in ALS-tolerant grain sorghum was significantly different between locations (Clayton and Rocky Mount) and by planting month at Rocky Mount (Figures 1, 2). At Clayton, the CTWR or beginning of the CPWC for grain sorghum is 368 GDD (Table 2). At Rocky Mount, the CTWR for May and June planted grain sorghum is 405 and 228 GDD, respectively. At Clayton, the CWFP or the end of the CPWC is 849 GDD while the CWFP for May and June planted grain sorghum in Rocky Mount is 876 and 1042 GDD, respectively. The CPWC for ALS-tolerant grain sorghum at Clayton ranged from 368 to 849 GDD (Table 3). The CPWC for May planted ALS-tolerant grain sorghum at Rocky Mount was from 405 to 876 GDD, and the CPWC for June planted ALS-tolerant grain sorghum at Rocky Mount was from 228 to 1042 GDD.
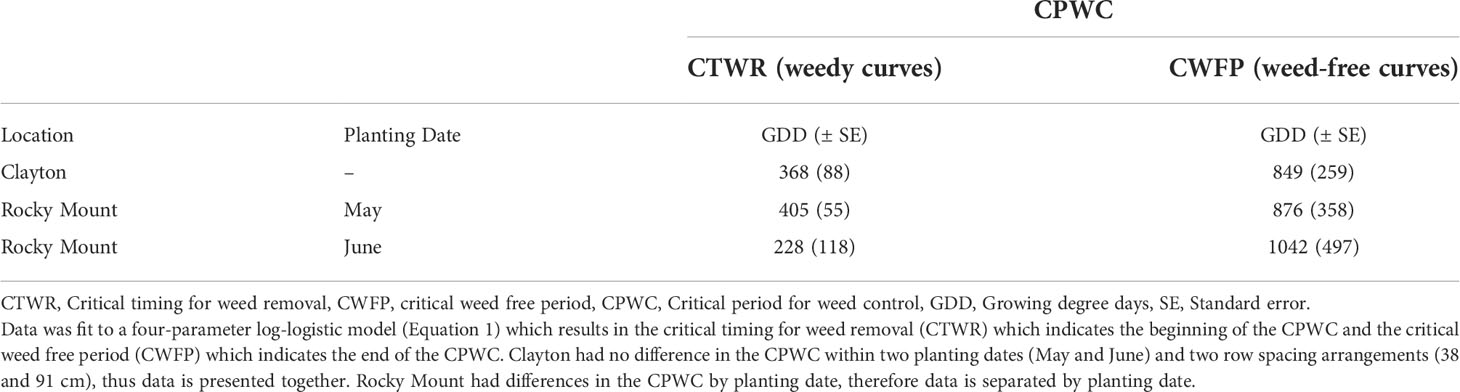
Table 2 The critical period for grass weed control (CPWC) for ALS-tolerant grain sorghum at an acceptable yield loss of 5% compared to weed-free all season management, at two locations in North Carolina (Clayton and Rocky Mount) expressed in growing degree days (GDD).
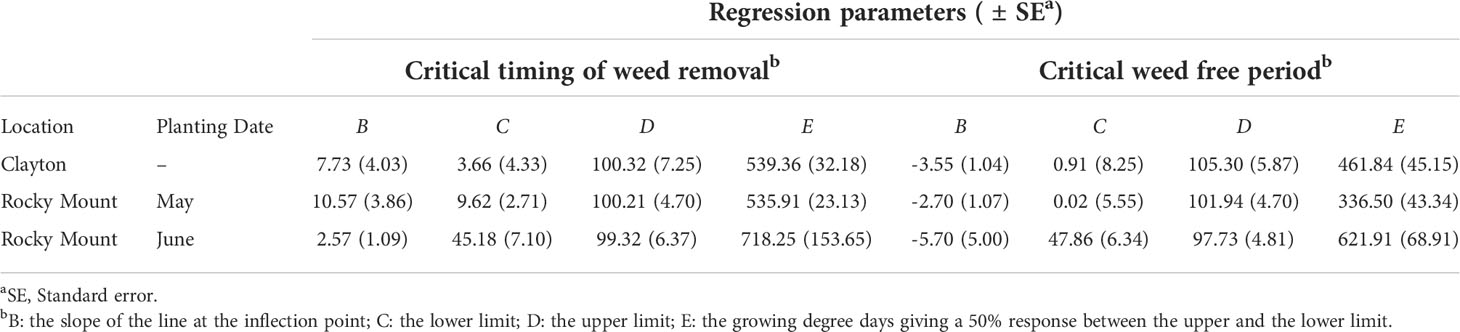
Table 3 Regression parameter estimates by location (Clayton and Rocky Mount) and planting date at Rocky Mount (May and June) for the four-parameter log-logistic model (Equation 1) for the critical timing of weed removal and the critical weed free period curves.
Discussion
Grass weed density
Several of the grass weed species investigated are among the most common and troublesome weed species for grain sorghum growers (Van Wychen, 2020). These include johnsongrass (Sorghum halepense [L.] Pers.), Texas millet (Urochloa texana [Buckley] R. Webster) and large crabgrass at Clayton, while broadleaf signalgrass (Urochloa platyphylla [Munro ex C. Wright] R.D. Webster) and large crabgrass were found at Rocky Mount. Even though multiple grass weed species were present at both locations, the experiment had similar results to those described in a previous study where narrow row spacing did not reduce weed density (Besançon et al., 2017b). This could be due to North Carolina’s climate compared to other parts of the country where grain sorghum is grown in rain limited environments.
Grain sorghum yield loss due to weed interference
The ranges of yield losses in this experiment due to no weed control are similar and even greater than those described by Dille et al. (2020) where grain sorghum producers in 7 of the top sorghum-producing states in the country reported loses between 37% to 60% of their grain sorghum yields when there was no weed control. A previous study presented similar results where uncontrolled weed growth resulted in yield losses of up to 60% (Magani, 2008). However the increased yield losses observed in North Carolina compared to these reported by Dille et al. (2020) could be due to climate, as more moisture availability could lead to more competitive weeds.
Critical period for weed control
The CPWC was different in each location, which is often observed due to variations in environment. It has been previously stated that a correct approach to the use of the CPWC is more site specific as different environments found in multiple locations, may result in different CPWC (Zimdahl, 2004). At Clayton, cultural practices (planting date and row spacing) were not significantly different. The CTWR at Clayton begins at 368 GDD, approximately 2 weeks after planting, is similar to previous research which reported that the first two weeks of weed interference with grain sorghum does not typically reduce yields (Burnside et al., 1964; Feltner et al., 1969; Smith et al., 1990). The end of the CWFP at Clayton is at 849 GDD, 4.5 weeks after planting, which indicates that grass weed control throughout the whole season is not required to maintain yield losses at a minimum.
Row spacing was not a significant factor at Rocky Mount, however planting dates (May and June) were significantly different for the CPWC of grain sorghum. The CPWC for grain sorghum planted in May was similar to that observed in Clayton, starting at 405 GDD and finishing at 876 GDD which corresponds to 2 and 5 weeks, respectively. These results corroborate findings of previous studies identifying that early weed interference does not reduce grain sorghum yields (Burnside et al., 1964; Feltner et al., 1969; Smith et al., 1990). The CPWC of June planted grain sorghum at Rocky Mount, however, had an earlier starting point and a longer duration, starting at 228 GDD and ending at 1042 GDD. However, in terms of weeks, the CPWC was 1 to 4 weeks, indicating that GDD were accumulated faster in June compared to May. These results suggest that to maintain yields in later planted sorghum, earlier weed control must be achieved and it must extend for more GDD when compared to the earlier planting date.
While there are no previous studies reported for the critical period for weed control in grain sorghum, there have been studies conducted looking at the critical period for weed control in other grass species crops. A study looking at field corn, popcorn and sweet corn (Tursun et al., 2016) determined the CPWC for these to range from 165 to 788 GDD, 92 to 678, and 182 to 632 GDD, respectively. However, this study looked at the combination of grass and broadleaf weeds’ effect on the CPWC as opposed to only grasses. Another study looked specifically at the effect of johnsongrass in the CPWC of field corn (Ghosheh et al., 1996), which resulted in a CPWC of 3 to 6.5 weeks after crop emergence.
Grass weeds were purposely targeted in this study because of the greater availability of chemistry for broadleaf weed control in grain sorghum compared to grasses. However, future studies should focus on looking at the whole spectrum of weeds and their influence over the CPWC. Studies could be designed to understand the differences in the CPWC between grass and broadleaf weed species, as well as a combination of both in grain sorghum as it has been in other crops (Everman et al., 2008). Another consideration would be repeating the experiment across different years to capture additional environmental effects. Conducting the study in a more arid region of the country could also provide a better understanding of weed interference under different climatological conditions and its impact on the CPWC, resulting on a better picture of the CPWC for grain sorghum.
Conclusion
In North Carolina and the Mid-Atlantic region, grain sorghum can tolerate grass competition early or late in the season, however the length of this period can vary among location and with crop planting date. The CPWC of a crop may depend on other factors such as weed species, crop cultivar, population, and row spacing (Knezevic et al., 2002; Zimdahl, 2004). This experiment demonstrates season long weed control is not necessary to maintain acceptable yields. The various planting arrangements investigated in this experiment were not only implemented as a basis of comparison, but also as an aid for potential equipment or timing limitations for grain sorghum growers. The data provided delivers a guideline for weed control timings that help the grower protect their yields regardless of the planting conditions. However, a grower should be cautious when utilizing the CPWC, implementing it as part of an integrated weed management program rather than the only strategy.
Data availability statement
The raw data supporting the conclusions of this article will be made available by the authors, without undue reservation.
Author contributions
All authors listed have made a substantial, direct, and intellectual contribution to the work and approved it for publication.
Acknowledgments
We would like to thank the North Carolina Small Grains Growers Association and The Sorghum Checkoff for funding of this project. We also want to thank the members of the Weed Science Laboratory at North Carolina State University that have assisted with this project, as well as the staff at the Central Crops Research Station in Clayton, NC and the Upper Coastal Plains Research Station for their support with the experiment.
Conflict of interest
The authors declare that the research was conducted in the absence of any commercial or financial relationships that could be construed as a potential conflict of interest.
Publisher’s note
All claims expressed in this article are solely those of the authors and do not necessarily represent those of their affiliated organizations, or those of the publisher, the editors and the reviewers. Any product that may be evaluated in this article, or claim that may be made by its manufacturer, is not guaranteed or endorsed by the publisher.
References
Balota M., Thomason W., Mehl H., Cahoon C., Reay-Jones F., Taylor S., et al. (2018). Revival of grain sorghum in the mid-Atlantic. Crops Soils 51 (1), 32–47. doi: 10.2134/cs2018.51.0110
Besançon T. H., Heiniger R. W., Weisz R., Everman W. J. (2017a). Grain sorghum and palmer amaranth (Amaranthus palmeri) response to herbicide programs and agronomic practices. Weed Tech. 31, 781–792. doi: 10.1017/wet.2017.53
Besançon T., Heiniger R., Weisz R., Everman W. J. (2017b). Weed response to agronomic practices and herbicide strategies in grain sorghum. Agron. J. 109, 1642–1650. doi: 10.2134/agronj2016.06.0363
Bishnoi U. R., Mays D. A., Fabasso M. T. (1990). Response of no-till and conventionally planted grain sorghum to weed control method and row spacing. Plant Soil 1290, 117–120. doi: 10.1007/BF00032403
Bowman H., Barber T., Norsworthy J., Roberts T., Kelley J., Gbur E. (2021). Resistance of inzen™ grain sorghum to multiple PRE- and POST-applied acetolactate synthase–inhibiting herbicides. Weed Technol. 35 (1), 57–64. doi: 10.1017/wet.2020.69
Bridges D. C. (1992). “Crop losses due to weeds in the United States,” in Proc. Weed Sci. Soc. Am. USA, 2002. 403.
Burnside O. C. (1977). Control of weeds in non-cultivated, narrow-row sorghum. Agron. J. 69, 851–854. doi: 10.2134/agronj1977.00021962006900050031x
Burnside O. C., Wicks G. A., Fenster C. R. (1964). Influence of tillage, row spacing, and atrazine on sorghum and weed yields from nonirrigated sorghum across Nebraska. Weeds 12 (3), 211–215. doi: 10.2307/4040732
Dille J., Stahlman P., Thompson C., Bean B., Soltani N., Sikkema P. (2020). Potential yield loss in grain sorghum (Sorghum bicolor) with weed interference in the united states. Weed Technol. 34 (4), 624–629. doi: 10.1017/wet.2020.12
Elezovic I., Datta A., Vrbnicanin S., Glamoclija D., Simic M., Malidza G., et al. (2012). Yield and yield components of imidazolinone-resistant sunflower (Helianthus annuus l.) are influenced by pre-emergence herbicide and time of postemergence weed removal. Field Crops Res. 128, 137–146. doi: 10.1016/j.fcr.2011.12.020
Elmore C. D. (1998). Weed survey: southern states: grass rops subsection. Proc. Weed Sci. Soc Am. USA, 41, 395–410.
Everman W., Clewis S., Thomas W., Burke I., Wilcut J.. (2008). Critical Period of Weed Interference in Peanut. Weed Technology 22 (1), 63–67. doi: 10.1614/wt-07-052.1
Feltner K. C., Hurst H. R., Anderson L. E. (1969). Tall waterhemp competition in grain sorghum. Weed Sci. 17, 214–216. doi: 10.1017/S0043174500031349
Frick B. (2000). “Weed management,” in Back to the basics: A manual for weed management on organic farms (Virden, MB: Organic Producers Association of Manitoba), 3–30.
Ghani A., Saeed M., Hussain D., Arshad M., Shafique M. M., Shah S. A. S. (2015). Evaluation of different sorghum (Sorghum bicolor l. moench) varieties for grain yield and related characteristics. Sci. Lett. 3, 72–74.
Gholami S., Minbashi M., Zand E., Noormohammadi G. (2013). Non-chemical management of weeds effects on forage sorghum production. Int. J. Adv. Biol. Biomed. Res. 1, 614–623.
Ghosheh H., Holshouser D., Chandler J. (1996). The critical period of johnsongrass (Sorghum halepense) control in field corn (Zea mays). Weed Sci. 44 (4), 944–947. doi: 10.1017/S0043174500094960
Grain sorghum best management practices for Mid-Atlantic Production (2016). United sorghum checkoff program (Lubbock, TX: United Sorghum Checkoff Program).
Grichar W. J., Besler B. A., Brewer K. D. (2004). Effect of row spacing and herbicide dose on weed control and grain sorghum yield. Crop Prot. 23, 263–267. doi: 10.1016/j.cropro.2003.08.004
Hall M. R., Swanton C. J., Anderson G. W. (1992). The critical period of weed control in grain corn (Zea mays). Weed Sci. 40, 441–447. doi: 10.1017/S0043174500051882
Henning D. S., AL-Khatib K., Tuinstra M. R. (2010). Postemergence weed control in acetolactate synthase-resistant grain sorghum yield. Crop Prot. 23, 263–267. doi: 10.1614/WT-D-09-00014.1
Hewitt C. A. (2015). Effect of row spacing and seeding rate on grain sorghum tolerance of weeds (Manhattan, Kansas: Kansas State University), 103.
Holt J. S. (1995). Plant responses to light: A potential tool for weed management. Weed Sci. 43, 474–482. doi: 10.1017/S0043174500081509
Hozayn M., El-Shahawy T. A. E., Sahara F. A. (2012). Implication of crop row orientation and row spacing for controlling weeds and increasing yield in wheat. Aust. J. Basic Appl. Sci. 6, 422–427.
Knezevic S. Z., Datta A. (2015). The critical period for weed control: Revisiting data analysis. Weed Sci. 63, 188–202. doi: 10.1614/WS-D-14-00035.1
Knezevic S. Z., Elezovic I., Datta A., Vrbnicanin S., Glamoclija D., Simic M., et al. (2013). Delay in the critical time for weed removal in imidazolinone-resistant sunflower (Helianthus annuus) caused by application of pre-emergence herbicide. Int. J. Pest Manag 3, 229–235. doi: 10.1080/09670874.2013.830797
Knezevic S., Streibig J., Ritz C. (2007). Utilizing R software package for dose-response studies: The concept and data analysis. Weed Technology. 21(3), 840–848. doi: 10.1614/WT-06-161.1
Knezevic S. Z., Evans S. P., Blankenship E. E., Van Acker R. C., Lindquist J. L. (2002). Critical period for weed control: the concept and data analysis. Weed Sci. 50, 773–786. doi: 10.1614/0043-1745(2002)050[0773:CPFWCT]2.0.CO;2
Kumar U., Craufurd P. Q., Gowda C. L. L., Ashok K. A., Claessens L. (2012). “Sorghum,” in Impacts of climate change on the agricultural and aquatic systems and natural resources within the CGIAR’s mandate (Copenhagen, Denmark: Consultative Group on International Agricultural Research), 136–144.
Magani I. E. (2008). Weed control in sorghum-groundnut mixture in the simultaneous farming system of southern Guinea savanna zone of Nigeria. J. Anim. Plant Sci. 1, 3–8.
Marin C., Weiner J. (2014). Effects of density sowing pattern on weed suppression and grain yield in three varieties of maize under high weed pressure. Weed Res. 54, 467–474. doi: 10.1111/wre.12101
Mishra J., Rao S., Patil J. (2015). Response of grain sorghum (Sorghum bicolor) cultivars to weed competition in semi-arid tropical India. Ind. J. Agric. Sci. 85, 688–594.
Ottman M., Olsen M. (2009). Growing grain sorghum in Arizona (Tucson, AZ: University of Arizona, College of Agriculture and Life Sciences).
Peerzada A. M., Ali H. H., Chauhan B. S. (2017). Weed management in sorghum [Sorghum bicolor (L.) moench] using crop competition: A review. Crop Prot. 95, 74–80. doi: 10.1016/j.cropro.2016.04.019
R Core Team (2019). R: A language and environment for statistical computing. R foundation for statistical computing, Vienna, Austria. http://www.R-project.org/.
Ritz C. J., Streibig C. (2005). Bioassay analysis using r. J. Stat. Softw 12, 1–22. doi: 10.18637/jss.v012.i05
Rizzardi M. A., Karam D., Cruz M. B. (2004). “Manejo e controle de plantas daninhas em milho e sorgo,” in Manual de manejo e controle de plantas daninhas. Eds. Vargas L., Oman E. S. (Bento Concalves: Embrapa Uva e Vinho), 571–594.
Smith B. S., Murray D. S., Green J. D., Wanyahaya W. M., Weeks D. L. (1990). Interference of three annual grasses with grain sorghum (Sorghum bicolor). Weed Technol. 18, 658–664. doi: 10.1017/S0890037X00025343
Smith K., Scott B. (2010). “Weed control in grain sorghum,” in Grain sorghum production handbook. Eds. Espinoza L., Kelley J. (Little Rock, AR, USA: Cooperative Extension Service, University of Arkansas), 47–49.
Soil Survey Staff, Natural Resources Conservation Service, United States Department of Agriculture. Web Soil Survey. Available online at the following link: http://websoilsurvey.sc.egov.usda.gov/. Accessed [02/20/2020].
Sorghum Checkoff. Growth and Development (2022). Available at: https://www.sorghumcheckoff.com/our-farmers/grain-production/growth-and-development/ (Accessed 1 August 2022).
Stahlman P. W., Wicks G. A. (2000). “Weeds and their control in grain sorghum,” in Sorghum: Origin, history, technology, and production. Eds. Smith C. W., Frederikson R. A. (New York, USA: Wiley Publishers), 535–582.
Tuinstra M. R., Al-Khatib K. (2007). “New herbicide tolerance traits in sorghum,” in Proc. Of 2007 Corn, Sorghum, and Soybean Seed Research Conference and Seed Exposition, Chicago, Il (Alexandria, VA, USA: American Seed Trade Association).
Tuinstra M. R., Soumana S., Al-Khatib K. (2009). Efficacy of herbicide seed treatments for controlling Striga infestation of sorghum. Crop Sci. 4, 215–231. doi: 10.2135/cropsci2008.06.0357
Tursun N., Datta A., Sakinmaz M., Kantarci Z., Knezevic S., Chauhan B. (2016). The critical period for weed control in three corn (Zea mays l.) types. Crop Prot. 90, 59–65. doi: 10.1016/j.cropro.2016.08.019
USDA (2022). Available at: https://ipad.fas.usda.gov/cropexplorer/description.aspx?legendid=315®ionid=na (Accessed August 30, 2022).
USDA-NRCS (2022) Web soil survey. Available at: http://websoilsurvey.sc.egov.usda.gov/App/HomePage.htm (Accessed February 10, 2022).
Vanderlip R., Roozeboom K., Fjell D., Kichman J., Kok H., Shroyer J., et al. (1998). Grain sorghum production handbook Vol. 3 (Manhattan, KS: Kansas Coop. Extn. Serv), C–687.
Van Wychen L. (2020) Survey of the most common and troublesome weeds in grass crops, pasture, and turf in the united states and canada. weed science society of America national weed survey dataset. Available at: https://wssa.net/wp-content/uploads/2020-Weed-Survey_grass-crops.xlsx.
Vincent W. J. (2015). Evaluating quinclorac for grass weed management in grain sorghum in north Carolina (North Carolina State University: United States).
Walker S. R., Taylor I. N., Milne G., Osten V. A., Hoque Z., Farquharson R. J. (2005). A survey of management and economic impact of weeds in dryland cotton cropping systems of subtropical Australia. Ani. Prod. Scie. 45, 79–91. doi: 10.1071/EA03189
Werle R., Jhala A. J., Yerka M. K., Anita D. J., Lindquist J. L. (2016). Distribution of herbicide-resistant shattercane and johnsongrass populations in sorghum production areas of Nebraska and northern Kansas. Agron. J. 108, 321–328. doi: 10.2134/agronj2015.0217
Werle. R., Tenhumberg B., Lindquist J. (2017). Modeling shattercane dynamics in herbicide-tolerant grain sorghum cropping systems. Ecol. Modeling 343, 131–114. doi: 10.1016/j.ecolmodel.2016.10.023
Keywords: weed removal, weed free period, grass competition, grain sorghum, ALS-inhibitor
Citation: Contreras D, Leon RG, Post AR and Everman WJ (2022) Critical period of grass weed control in ALS-tolerant grain sorghum (Sorghum bicolor) is affected by planting date and environment. Front. Agron. 4:1014801. doi: 10.3389/fagro.2022.1014801
Received: 09 August 2022; Accepted: 12 September 2022;
Published: 29 September 2022.
Edited by:
Bhagirath Singh Chauhan, The University of Queensland, AustraliaReviewed by:
Alessandra Carrubba, Università di Palermo, ItalyAmanullah, University of Agriculture, Peshawar, Pakistan
Arnold Mashingaidze, Chinhoyi University of Technology, Zimbabwe
Copyright © 2022 Contreras, Leon, Post and Everman. This is an open-access article distributed under the terms of the Creative Commons Attribution License (CC BY). The use, distribution or reproduction in other forums is permitted, provided the original author(s) and the copyright owner(s) are credited and that the original publication in this journal is cited, in accordance with accepted academic practice. No use, distribution or reproduction is permitted which does not comply with these terms.
*Correspondence: Wesley J. Everman, d2VzX2V2ZXJtYW5AbmNzdS5lZHU=