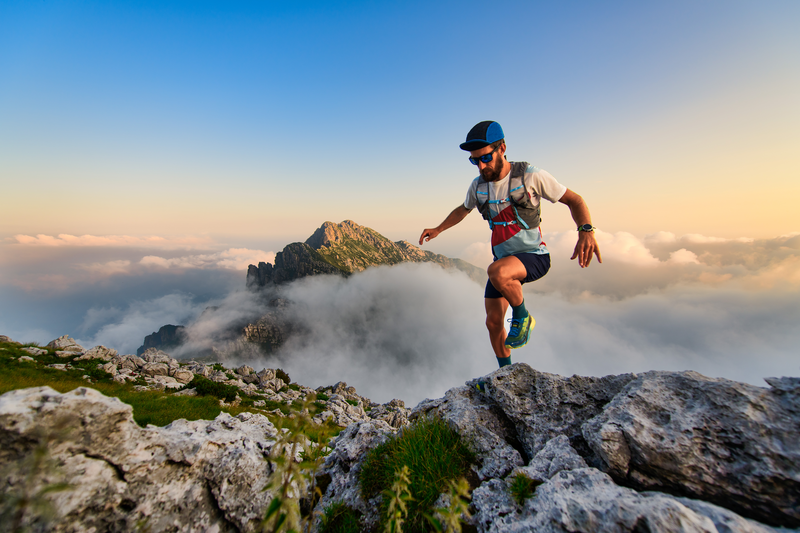
94% of researchers rate our articles as excellent or good
Learn more about the work of our research integrity team to safeguard the quality of each article we publish.
Find out more
ORIGINAL RESEARCH article
Front. Agron. , 10 January 2022
Sec. Agroecological Cropping Systems
Volume 3 - 2021 | https://doi.org/10.3389/fagro.2021.787507
This article is part of the Research Topic Conservation Agriculture: Knowledge Frontiers Around the World View all 11 articles
Maize (Zea mays L.), the staple crop of Mexico, is often produced by smallholder farmers on sloping terrains. Historically, little agronomic research has been performed under the conditions of these farmers to support them in the sustainable intensification of their production systems. We set up trials at two locations in the state of Oaxaca to evaluate conservation agriculture and agroforestry in collaboration with local farmers. Crop diversification through crop rotations, multicropping, relay cropping or agroforestry increased system yields the most, by up to 1.4 t ha−1 in Teopoxco and 1.7 t ha−1 in Tamazulapam. Increased input use through increased fertilization or liming did not increase profitability enough to justify their use. Zero tillage and residue retention increased yields and reduced production costs. Conservation agriculture with agroforestry was a high-yielding, profitable system that also reduced farmers' risk by providing several harvests per year. The most profitable combinations of agroforestry and conservation agriculture could produce up to $4,854 USD ha−1 in Teopoxco and $2,143 USD ha−1 in Tamazulapam, while the control treatments in the same sites and years produced $175 USD ha−1 and $92 USD ha−1 respectively. In several years the main crop failed, while the trees were able to produce due to their different growing season compared to maize. Through adaptive investigation under farmers' conditions, sustainable intensification of traditional production system is possible with low-cost changes that are locally adapted and within farmers' possibilities.
Maize (Zea mays L.), the traditional staple crop in Mexico, is often produced by smallholder farmers on sloping terrains. Widespread soil degradation and erosion caused by tillage, stubble burning and residue removal has reduced maize productivity in many regions of Mexico (SEMARNAT, 2016). In the state of Oaxaca, over 60% of soils have been affected by erosion (Bolaños Gonzalez et al., 2016). Smallholder farmers who grow maize on hillsides would typically shift cultivation across their land and fallow sections to recover soil fertility, but population growth, land use changes and soil degradation have resulted in shorter fallow cycles, in turn worsening land degradation and the pressure on the available land (Contreras-Hinojosa et al., 2005; Vergara-Sánchez et al., 2005). The soils in the region are high in organic matter but acid and poor in nutrients, therefore the use of lime, compost and synthetic fertilizers has been suggested as a means to improve productivity (Vergara-Sánchez et al., 2005). Added to this, farm output is often not enough to meet household dietary or financial needs (Pfeiffer et al., 2009; Novotny et al., 2021). At the same time, despite the need to improve hillside farming systems to reduce land degradation and improve their productivity and the food security of households that depend on them, there has been insufficient agronomic research regarding maize farming systems on sloping land in Mexico and the scientific literature provides little guidance regarding which sustainable intensification practices are most suited to the needs of the farmers in these systems (Hellin and López-Ridaura, 2016).
Sustainable intensification through approaches such as conservation agriculture or agroforestry can improve productivity and reduce soil degradation (Govaerts et al., 2017; Thierfelder et al., 2018). Conservation agriculture, which is based on the combination of minimal tillage, maintaining a permanent soil cover, and diversifying cropping (Lal, 2015), has been shown to increase maize yields and improve soil health and improve profitability in a wide range of Mexican production systems (Fonteyne et al., 2019, 2021; Monjardino et al., 2021). In the context of the hillside farmers minimal tillage can reduce erosion, while crop diversification may improve food security (Reyes Jaramillo, 2016; Novotny et al., 2021). Agroforestry, the combination of trees and agricultural crops, is currently being promoted in Mexico as the “Milpa Intercalada con Arboles Frutales” (MIAF; or “milpa”, the traditional maize-based multicrop system, interspersed with rows of fruit trees) system (Torres Zambrano et al., 2008). The fruit trees are intended to generate income and reduce erosion while maize and other crops provide food security (Cadena-Iñiguez et al., 2018). Maize residues and tree clippings are placed in tree rows to catch run-off and gradually create terraces. Some studies have concluded that conservation agriculture and agroforestry are not compatible (Turrent Fernández et al., 2017; Ndoli et al., 2018), while other authors see them as complementary (Sims et al., 2009; Bayala et al., 2011; Reyes Jaramillo, 2016). Their compatibility probably depends on local agro-ecological and socio-economic conditions and how both practices are implemented and adapted to those conditions. The MIAF system is widely promoted by public programs in Mexico but few scientific studies have evaluated it. At the same time, smallholder farmers in the Mexican state of Oaxaca lack training or resources to undertake farming system innovations alone, so innovations have to be co-developed, evaluated under local conditions and adapted to the production system (Ruiz Mendoza et al., 2012).
Our study evaluated options for the sustainable intensification of smallholder maize production in the mountainous regions of Mexico. Two similar trials were implemented from 2014 to 2019 in the Cañada and the Sierra Norte regions of the state of Oaxaca, as part of a project aiming to improve the local agri-food system through evidence-based recommendations co-created together with local farmers and researchers (Gardeazabal et al., 2021; Govaerts et al., 2021). In the last decades land use in the Cañada and the Sierra Norte regions has changed from forestry to agriculture, with maize being grown on steep slopes (>30%) on soils of low fertility and high acidity and using few or no inputs (Vergara-Sánchez et al., 2005). Local farmers initially reported low yields due to the poor fertility, insect damage and a lack of technical advice. We proposed several practices to address those constraints, including changes in plant spacing and fertilization, as well as zero tillage, a legume rotation, and agroforestry. Treatments were designed to represent possible improved production systems and those were compared to the common practices (control treatment). We reviewed the treatments regularly with farmers and adjusted them if they were found not to be cost-effective. Ultimately, we sought to determine if sustainable intensification of maize production could be achieved by (1) replacing the traditional fallow period with a legume rotation, (2) applying conservation agriculture, and (3) practicing soil amendments or fertilization. These treatments were evaluated in farmers' fields with a MIAF system in place to evaluate if they could effectively be combined with MIAF.
The trial was located in San Nicolás in the Santa María Teopoxco Municipality in the Mazateca Alta subregion of the Cañada in the North of Oaxaca state (N18.1518, W96.9528) (Figure 1). The trial was begun in 2015 in the field of a local farmer at about 2036 m above sea level with an average slope of 28%. The soil has a variable depth of ~1 m, a loamy texture, a pH of 4.7, an organic matter concentration of 9.3%, a cation exchange capacity of 31.2 meq/100 g and is low in nitrogen and phosphorous. The soil is classified as a Cambisol. The mean temperature is 16°C and annual rainfall is ~1,500 mm, falling mostly from June to October but with a variation of over 1,000 mm between years, during the period of the experiment. Climate data from the weather station closest to the site is presented in Supplementary Table 1. The main weeds at the site were Pteridium aquilinum, Bidens odorata, Amaranthus spp, Rumex crispus, Portulaca oleracea and Phaseolus coccineus. In 2008 the field was planted with three lines of Hass variety avocado trees (Persea Americana) in a MIAF system. Between 2008 and 2015, the farmer sporadically planted maize in the fields between the tree rows.
Figure 1. Map indicating the locations of the trials in Oaxaca state, as well as the climatic zones.
We conducted the trial in Puente Ardilla of the Tamazulapam del Espiritu Santo Municipality in the Alta Mixe subregion of the Sierra Norte region in the state of Oaxaca (N17.067869, W96.044833) (Figure 1). The field lies at about 1878 m above sea level and has an average slope of 30%. The soil has a variable depth of ~20–30 cm, a clayey texture, a pH of 4.7, an organic matter concentration of 3.0%, a cation exchange capacity of 15.7 meq/100g and is low in nitrogen and phosphorous. The soil is classified as a Cambisol. The mean temperature is 18 °C and annual rainfall is 1000-1200 mm, falling mostly in summer. Climate data from the weather station closest to the site is presented in Supplementary Table 2. The main weeds at the site are Tagetes spp, Oxalis spp), Tithonia spp. The MIAF system was implemented in 2005 with the planting of peach trees (Prunus persica) of the variety Diamante, which in contrast to the local landrace ripen before the onset of the rainy season, producing high quality fruit before the local market is saturated with peaches. From 2005 onwards, a milpa was sown for two years followed by two years of fallow until 2014, when the trial began.
The experimental design was the same at both sites. Treatments were evaluated in a randomized complete block design with three replications (Figure 2) and each block having a row of trees perpendicular to the slope and in the middle. In Teopoxco the plots were 6.0 × 10.6 m, or 63.6 m2 of which 38.4 m2 was sown to maize and 25.2 m2 occupied by trees, while in Tamazulapam plots were 8.0 × 8.6 m, with 38.4 m2 sown to maize and trees on 28.8 m2.
Figure 2. Map of the trial in Teopoxco. The field layout was similar in Tamazulapam, but with different measurements. ML, maize-legume rotation; MF, maize-fallow rotation; CT, conventional tillage; ZT, zero tillage; F1, fertilization 1; F2, fertilization 2; F3, fertilization 3. P indicates plot number.
The treatments were designed in collaboration with local farmers to evaluate the effect on yield and profitability of minor interventions in the production system. Both trials compared a control treatment representing the local production system with four treatments representing potentially improved production systems, as the small field size did not allow for a full factorial design (Table 1). Both trials had five treatments, with treatment 1 (MF, CT, F1) being the control treatment with conventional tillage, residue removal, and three years of maize cultivation, followed by at least one year of fallow and fertilization of 80 kg N ha−1 as ammonium sulfate. In the first years (2014, 2015, 2016) in treatment 1 (MF, CT, F1), maize plants were sown with a hoe or mattock at a distance of 1 m between plants and 4 seeds per hole to obtain a planting density of 40,000 plants ha−1. Later, in 2018 and 2019, the planting arrangement was standardized at 50,000 plants ha−1 for all treatments in both experiments. Treatment 2 (ML, CT, F2) used conventional tillage but with an improved planting arrangement (50,000 plants ha−1, with 2 seeds per hole and 50 cm between holes in a row and 80 cm between rows), improved fertilization (Table 2) and in the last two years a legume crop after maize in the autumn-winter cycle, instead of a fallow period. Treatment 3 (MF, ZT, F3+Lime/F2) had the same plant density as treatment 2, improved fertilization and zero tillage combined with the retention of half of the maize residues on the field and a fallow period after maize cultivation. Treatments 4 (ML, ZT, F2) and 5 (ML, ZT, F3/F2+lime) were similar to treatment 3 but with a legume rotation instead of fallow and different fertilization regimes. No previous research existed on which to base the trials, therefore the innovation treatments were based on field experience and consultation with the cooperating farmers and were adjusted over the years according to farmers' observations and the experimental results.
Fertilization rates were changed during the trial because the initial high rates were not cost effective (Table 2). Higher fertilization levels were included in the innovation treatments, as farmers and extensionist expressed the need for information on whether fertilization could alleviate the problems of low soil fertility. In Tamazulapam dolomite lime was added to treatment ML, ZT, F2+lime at a rate of 4 t ha−1 in 2014 and 2018. In Tamazulapam the fallow/legume cycle had to be repeated in 2017, because social conflict and heavy rains impeded the timely sowing of maize. That year broad beans (Vicia faba) were planted but the crop was lost due to the heavy rainfall. The maize-legume rotation treatments were changed in 2018. To increase cropping intensity and diversity, from the fall-winter cycle of 2018–2019, pea (Pisum sativum) was interseeded between maize in mid-October in treatments that featured a rotation and harvested in March. This was successful in Teopoxco, but the pea crop failed in Tamazulapam due to a lack of residual soil moisture.
At both sites conventional tillage was done by inverting soil with hand hoes, while in zero tillage weeds were removed by scraping the soil with hand hoes or using glyphosate in 2016 in Teopoxco. In Teopoxco sowing was done in March using residual humidity for germination, while in Tamazulapam sowing was done at the end of May at the onset of summer rains. An overview of the approximate time of main operations is given in Figure 3. The timing of each operation depended on the specific conditions of each site and season; therefore, a full overview of the dates of field operations is included in Supplementary Table 3. All maize was sown manually with a planting stick. In Teopoxco treatments, only maize was grown, while in Tamazulapam we grew a milpa, with the maize planted in orderly rows and bean (Phaseolus vulgaris) and fig leaf gourd (Cucurbita ficifolia) planted at 1 m and 4 m distances between plantings respectively, as is the local custom. In conventional tillage treatments, all maize residues were placed between the trees as a run-off barrier, while in zero tillage treatments, 50% of maize residues was used for the barriers and the rest as a soil cover. All crops were local landraces; the legume sown was Phaseolus dumosus in Teopoxco (2017) and Phaseolus coccineus in 2016 and Vicia faba in 2017 in Tamazulapam.
Figure 3. Crop calendar for both sites. Blue bars indicate approximate timing of field operations at the Teopoxco site, green bars indicate approximate timing of field operations at the Tamazulapam site.
Maize fertilizer was given in two applications: half of the N and all P, K and compost were applied at planting, while the rest of the nitrogen was applied at V6 stage. The 80-00-00 dose of fertilizer was applied with ammonium sulfate, while other fertilization doses were applied using a mixture of urea and triple superphosphate. The compost was made by the farmers using locally available inputs—approximately 40% forest soil, 40% goat manure and 20% chicken manure—and was applied at 2.5 t ha−1. Weeds were controlled manually in all treatments, control was performed as needed (2–3 times per season) using a hand hoe. In treatments with conventional tillage the soil was turned to control weeds, while in treatments with zero tillage the soil was scraped with the hand hoes, in order to move the soil minimally. In treatments with conventional tillage, soil was heaped at the base of maize plants during the first weeding, a traditional practice to reduce lodging. Maize seed was treated with Thiodicarb at both sites until 2018 to help control grubs of Phyllophaga sp.; the main pests were Macrodactylus mexicanus in maize and Trichaltica sp. in beans and imidacloprid or chlorpyrifos were also applied to control them. In Teopoxco in 2016, we used cypermethrin to control Spodoptera frugiperda. After farmers commented in 2018 that insecticides were expensive and hard to obtain, and that they were applied in maize or bean crops only when donated by the local government, because farmers would thus not consistently use insecticides, it was decided to stop synthetic insecticide use and apply only locally made chile (Capsicum annuum) extracts. The maize crop was manually harvested in November in Teopoxco and in Tamazulapam maize and bean were harvested in December and fig-leaf gourd (Cucurbita ficifolia) was harvested in February.
The MIAF system was implemented at both sites by planting rows of trees along the contour lines with a distance between rows of 10.6 m and between trees of 1 m in Tamazulapam (900 trees ha−1) and 2 m in Teopoxco (450 trees ha−1). Maize was sown on 60% of the area and 40% was under fruit trees. Crop residues and pruned branches were placed at the base of the trees to catch run-off water and help form terraces. Trees were managed uniformly at both sites, independent of the treatments, and were fertilized with 500 g triple 17 (17–17–17 NPK) per tree; in Teopoxco they also received 2 kg of compost per tree and one application of a commercial micronutrient mixture containing magnesium, zinc, and manganese. Diseases were controlled with Bordeaux mixture consisting of 10 g CuSO4 + 7.5 g Ca (OH)2 per liter water, sprayed on the tree with a backpack sprayer before flowering. Fruits were harvested for sale or household consumption between November and February in Teopoxco and in April in Tamazulapam. Because trees are harvested manually over a long period and the harvest could thus not be measured in a single occasion, fruit yield data are based on information provided by the farmers. Fruit tree yield was determined in the whole field starting in 2018 in Teopoxco and in 2017 in Tamazulapam, after consulting with farmers on the importance of obtaining the data to understand the system.
Maize, bean and pea yields were determined by harvesting the two central rows in the subplot above and below the tree line over a distance of 5 m in Teopoxco and 7 m in Tamazulapam. In 2015 the farmer in Teopoxco harvested some maize before yield could be determined. Moisture content of the grain was determined on a subsample and adjusted to 14% for maize and 12% for beans. Peas were harvested and marketed fresh, so fresh weight was used to determine yield and profitability. The yields of the intercrops (common runner bean and fig leaf gourd) in Tamazulapam were determined on a per hectare basis by harvesting all plants in the trial.
To calculate production costs and profitability, all farm operations were logged and labor costs set as the price of hired labor ($7.5 USD day−1) and adjusted to a per hectare basis. Input prices were obtained from local markets (Table 3). Production costs were first calculated separately for maize and fruit trees per hectare and per treatment. To calculate fruit production, the production of all trees in the trial was estimated and extrapolated to 1 hectare. After calculating yield and profitability for all treatments on a per hectare basis, the costs and profitability of the MIAF system were then calculated. Profitability was calculated by assuming 60% of the area was sown with crops. trees in a MIAF system are in a special, high density, arrangement, the calculated yield per hectare for the fruit trees would thus be the actual yield of these trees in such an arrangement and was used directly to calculate profitability. MIAF profitability was thus calculated by adding fruit tree profitability with 0.6 times the treatment profitability per hectare. Costs and profitability were expressed in US Dollar (USD) taking an average conversion rate of 20 $MXN $USD−1.
All statistical analyses were performed in R version 3.6.0 (R Core Team, 2020). Data of grain yield and net profit were analyzed with the model:
With Y being the response variable, m the overall mean, T the effects of the treatment and R the replicate effects within a site. Given the large differences in conditions between sites, data for the two sites were analyzed separately. There were significant year × production system and year × management interactions, so years were analyzed separately. Replications were considered as independent random factors with zero means and some variance. We analyzed the model through ANOVA using the glm and aov functions from the “stats” package. The ANOVA table can be found in Supplementary Tables 4, 5.
Maize yields did not differ significantly between treatments for two out of the three years (Figure 4). Only in 2018, when the crops were grown after the fallow or the Phaseolus dumosus rotation, were maize yields significantly higher in treatments MF, ZT, F3+Lime (2.8 t ha−1) and ML, ZT, F3 (3.0 t ha−1) than for the control MF, CT, F1 (1.6 t ha−1), whereas results for treatments ML, CT, F2 and ML, ZT, F2 did not differ significantly from those for the other treatments. Neither pea nor bean yields differed significantly across treatments, which is to be expected because the management practices for each did not differ across treatments. In 2019, poor rainfall reduced yields, so the effect on summer maize yields of growing a winter pea crop could not be determined.
Figure 4. Yield of maize, beans and peas per treatment and growing cycle in the trial in Teopoxco, Oaxaca, Mexico. Error bars indicate standard error. Treatments with the same letter are not significantly different in 2018. Yields could not be determined in 2015 due to miscommunication with the collaborating farmer.
In terms of income per hectare, the potential of winter cropped peas stands out (Table 4). Given their good price in the region and good yields in these trials, the income from peas was considerably higher than that possible from maize or beans. For maize, the control treatment was never profitable, while the conservation agriculture treatments were profitable in years with adequate rainfall.
Table 4. Total cost, income and profit for maize, beans and peas per treatment and avocado trees in the whole trial per growing cycle as well as the MIAF system profitability, calculated as the sum of the income from the trees and the treatment if grown in a MIAF arrangement in which 40% of area is planted with trees and 60% of area with crops.
In 2016 the only profitable treatment was ML, ZT, F3 (Table 4), due to the high costs from the depreciation of the liming ($124 USD ha−1 year−1 over 3 years) and the expenses of foliar fertilization and labor to apply inputs and control weeds, in the other treatments. As a result, we subsequently reduced inputs and labor use, especially for weeding and foliar fertilization, thereby increasing profitability with no loss in yields (Figures 5A,B). From 2018 onwards, lime was applied only in MF, ZT, F3+Lime, to evaluate its cost-effectiveness under trial conditions. In fallow years, profitability was negative due to the cost of weed management. The winter pea crops were highly profitable because of their low cost (requiring only sowing and harvesting) and relatively high yields and market price. Peas also suppressed weeds during the winter season, reducing labor costs for the subsequent maize crop. Due to drought in 2019, the potential effect on maize yields of growing peas in the subsequent crop could not be evaluated but growing a winter crop assured at least one harvest from the plot, even if the summer crop failed.
Figure 5. Total yearly labor costs (A) and input costs (B) per treatment in Teopoxco. Note that in treatments with maize-legume rotation the cost includes the winter pea crop in 2018 and 2019. Labor costs of ML, ZT, F2 and ML, ZT, F3 and input costs of ML, CT, F2 and ML, ZT, F2 mostly overlap.
Avocado yields under MIAF were 1.1 t ha−1 in 2018 and 1.7 t ha−1 in 2019. At the local prices, the income per hectare of fruits in the MIAF arrangement was $1,609 USD ha−1 in 2018 and $1,314 USD ha−1 in 2019. Prices in 2019 were only half those of 2018, causing lower income even though the yield was higher. The total income from one year of MIAF (avocado, maize and peas) ranged between $1,714 USD ha−1 for the control treatment and $4,854 USD ha−1 for ML, ZT, F3 in 2018 and between $1,229 USD ha−1 for the control treatment and $2,309 USD ha−1 for ML, ZT, F3 in 2019 (Table 4). In both years ML, ZT, F3 was most profitable because of the income generated by peas, but MIAF with conventional practices was still highly profitable compared to the conventional practice of growing maize alone. Compared to farmers' conventional practices, the MIAF system is thus more profitable when the maize is sown conventionally, and MIAF combined with additional sustainable innovations provides yet greater profits on average.
Maize yields differed significantly between treatments in 2014, 2018 and 2019, at Tamazulapam (Figure 6). In the first year, yield was significantly higher in the control because of an overly dense crop stand in the innovation treatments. In the second year, there were no significant differences between treatments, although the zero tillage treatment yields were higher on average. After the 2016-2017 fallow or legume rotation, 2018 and 2019 yields were higher in treatments that had been rotated with legumes than in the control with fallow. Yields were also higher on average in the treatment with zero tillage (MF, ZT, F2) and no rotation, although not significantly different from those of other treatments. Bean yields in 2016 did not differ significantly between treatments. In 2017, the faba bean crop failed to produce grain. Intercropped bean and fig-leaf gourd yields over the whole trial were 274 and 339 kg ha−1 in 2018 and 119 and 530 kg ha−1 in 2019.
Figure 6. Average yield adjusted to 14% moisture for maize and 12% moisture for bean (2016, striped bars) for the trial in Tamazulapam del Espiritu Santo, Oaxaca, Mexico. Error bars indicate standard errors. Treatment with the same letters are not significantly different in a given year. No yields were recorded in 2017 due to crop failure.
Considering only maize, the average profitability was lowest ($148 USD ha−1) in the control treatment, MF, CT, F1, and highest in the treatments with zero tillage and a legume rotation, at $284 USD ha−1 for treatment ML, ZT, F2 and $267 USD ha−1 for treatment ML, ZT, F2+lime, due to their superior yields and lower production costs, which were, as in Teopoxco, also reduced in concert with farmers after the first year of trial (Table 4). In 2014 an average of $249 USD ha−1 was spent on inputs and 113 days of work per hectare performed, whereas in 2019 an average of $129 USD ha−1 was spent on inputs and 55.6 days of labor performed on each hectare, without compromising yields.
Rotations with P. dumosus beans were not profitable in 2016 because of the labor required, totaling 103 person days per hectare for sowing, fertilization, weeding, and harvesting. Under zero tillage, 7 fewer work person days per hectare were necessary but the higher yields under conventional tillage covered this cost (Figures 7A,B). The beans suffered high losses due rabbit feeding and could potentially be profitable with reduced labor costs and good pest control or when used as a cover crop. In any case, given the net loss in beans in 2016 and the failed faba bean crop in 2017, crop rotation treatments were less profitable on average over 6 years than treatments without a bean rotation.
Figure 7. Input costs (A) and labor costs (B) per treatment and year in the trial in Tamazulapam del Espiritu Santo, Oaxaca.
The profitability of beans and fig-leaf gourd was calculated only for 2018 and 2019, totaling $123 USD ha−1 and $10 USD ha−1 respectively in 2018 and $95 USD ha−1 and $44 USD ha−1 respectively. Taking these crops into account, the conventional milpa system was profitable in all years.
At 2.0 t ha−1 in 2017, 4.3 t ha−1 in 2018, and 3.6 t ha−1 in 2019, peach yields under the MIAF produced per-hectare incomes of $1,641 USD ha−1, $1,857 USD ha−1, and $1,650 USD ha−1 in each of those years. The total yearly per-hectare income from the MIAF (peach, maize, beans and fig-leaf gourd) ranged from $1,222 USD ha−1 for ML, ZT, F2+Lime in 2017 to $2,158 USD ha−1 for ML, ZT, F2 in 2018 (Table 5). The MIAF was less profitable in Tamazulapam than in Teopoxco due to the lower market price in the former for peaches, but the MIAF was always more profitable than the control treatment. The most profitable MIAF treatments were those with a legume rotation instead of fallowing in the years with a successful maize crop, 2018 and 2019, and even when the crop failed in 2017, these treatments were profitable because the peaches were harvested and sold in the off season and thus did not suffer the drought.
Table 5. Total cost, income and profit for maize, beans, fig leaf gourd and peas per treatment and peach trees in the whole trial per growing cycle as well as the MIAF system profitability, calculated as the sum of the income from the trees and the treatment if grown in a MIAF arrangement in which only 60% of the area is planted with crops.
At both sites it was possible to increase the yields of the local landrace maize under smallholder conditions through simple agronomic interventions. The designs of the trials were not full factorial but rather representations of improved cropping systems, making it possible to identify improved systems but complicating the precise identification of best practices. The treatment component that seemed to have the highest impact on maize yields was rotation with a legume instead of fallowing, as the yields in Teopoxco in 2018 and Tamazulapam in 2018 and 2019 show, but legume crops in the fallow year were not always profitable, due to farmers' lack of experience with them and to rabbit damage. In 2018 the annual rotation was changed to rotation with a pea relay crop. This failed in Tamazulapam due to a lack of residual soil moisture but was successful in Teopoxco, except for 2019 when a drought caused the failure of all treatments. In addition to being very profitable, the pea crop reduced weeds. Weed management is a major issue in conservation agriculture (Nichols et al., 2015) and can reduce yields as much as 90% under CA practices in Oaxaca, if not controlled (Fonteyne et al., 2017b). One weeding is necessary at pea planting in October, which is beneficial because farmers normally do not control weeds during the fallow period, letting them grow and produce seeds during winter. After the pea harvest, farmers were able to sow maize with less weeding, making zero tillage more attractive and feasible for them. Weeding labor costs were reduced over the course of trial, to increase profitability. In systems of high productivity, weeds are a major constraint on yields. In our trials maize yields were low and did weeds may not have been the main constraint, as yields did not seem to be impacted by less weeding. Similarly, in a study in three regions of Oaxaca, weeds could reduce yields by 90% in the systems of high yields in the Mixteca region, while weed control was often not economical in the semi-arid Valles Centrales (Fonteyne et al., 2017b).
Liming and increased fertilization have been proposed as a solution for low maize yields in the region (Vergara-Sánchez et al., 2005; Reyes Jaramillo, 2016), but our data indicate that these are not cost-effective measures. Fertilization treatments had little effect on maize yields and proved very costly; regarding liming, there was little indication of benefits for maize at either site. Likely the local landraces are adapted to the acid soils, other maize landraces in the region have been shown to be adapted to adverse conditions such as low nitrogen, alkaline soils, or high rainfall (Fonteyne et al., 2017a; Van Deynze et al., 2018). Furthermore, soils at both sites are high in organic matter, which may reduce the effects of fertilization or acidity (Aitken et al., 1990). Little is known about the correct fertilization of Mexican maize landraces, especially on hillsides, even though farmers often apply fertilizers (Reyna-Ramírez et al., 2018). Landrace fertilization should be studied in dedicated field trials in the different agro-ecologies of Mexico, in order to provide adequate recommendations to the local farmers.
Zero tillage increased yields in 2018 in Teopoxco and possibly in 2018 and 2019 in Tamazulapam, although due to the trial design it is impossible to rule out fertilization or planting density effects. Zero tillage did save an average 8 labor days per hectare in land preparation for maize and might in the long term reduce labor costs associated with weed control (Fonteyne et al., 2020), especially if combined with relay cropped peas. Reduced over the course of the trials from originally high levels, labor costs can still be lowered, for example by using animal- or two-wheel-tractor-drawn seeders, which would cut the time for sowing from 11.5 to 2.0 days per hectare while improving the quality of sowing and fertilization (Van Loon et al., 2020). Likewise, harvest and postharvest processing account for up to 20% of production costs and would benefit from increased mechanization. Furthermore, postharvest losses of maize under the farmers' conditions due to insect, fungi and rodent damage are on average already 12% after three months but can be as high as 30% in the highlands of Oaxaca (Odjo et al., 2020), so improving storage practices and technologies could increase grain availability as much as the best agronomic interventions.
Overall, high input use was not economical, so research in these conditions should focus on non-monetary inputs. These can be more important for smallholder farmers but are often overlooked, with the focus of outsider interventions on purchased inputs such as fertilizers, pesticides or improved seeds, whereas rotations, optimal plant spacing, sowing time or conservation agriculture can sustainably increase yields without the need for additional inputs. Improved treatments can increase yields but also require investment from resource-constrained farmers, which may reduce their effectiveness in farmers' fields. Furthermore, we counted all labor as a cost but labor in the region is often done by family members or reciprocal among farmers. Therefore, the income of the control treatments was sometimes negative but farmers would not necessarily appreciate this in the same way, because maize farming might often be the most economical option due to the lack of other work opportunities. Nevertheless, the most effective interventions, rotation and zero tillage, do not necessarily require a larger investment and can reduce labor cost.
Maize yields were relatively high at both sites compared to the local average of about 1 t of maize per hectare (SIAP, 2020), indicating no adverse competition effect from trees and probably a result of the differences in production periods, with fruit growing in the spring and maize in summer (Torres Zambrano et al., 2008). Fruit production is knowledge and resource intensive and avocado and peach yields in our trials were relatively low. Adverse weather was a factor, but farmers' lack of management experience and knowledge was the foremost constraint. Ruiz Mendoza et al. (2012) reported similar constraints in a study about MIAF adoption in the Mixe region. More research is needed on all aspects of fruit tree management in the highly diverse mountainous regions of Mexico, to determine optimal practices, varieties and species and training on these aspects should be a part of future MIAF promoting projects (Santiago-Mejía et al., 2008).
The fruit trees provided additional food and income at different times from the maize crop, enhancing the economic resilience and food security of smallholder farm households. In Tamazulapam in 2017 or Teopoxco in 2019, the trees bore fruit in spring whereas the main crop failed in summer. Climate change is likely to increase the frequency of such growing seasons, especially in the south of Mexico maize yields are expected to decline under most climate change scenarios (Murray-Tortarolo et al., 2018; Ureta et al., 2020). Crop diversification would improve resilience of smallholder production under climate change and reduce the risk of overall crop failure (Donatti et al., 2019). Overall, fruit production was more profitable than maize, so a MIAF system to cover food security with the rest of the available land used for fruit production would be most profitable and resilient. Land scarcity is often cause of conflict in the region (Villavicencio Rojas, 2013), so available land must be used intensively and effectively. Under the MIAF a field can be continuously cultivated with no need for fallowing (Ruiz Mendoza et al., 2012), providing more food from the a given area of land.
Turrent Fernández et al. (2017) hypothesized that conservation agriculture and the MIAF system for smallholder maize farmers are mutually exclusive, but our results showed that the two can be combined effectively. MIAF is often promoted with tillage to generate erosion and thus form terraces through use of run-off filters in tree lines that capture soil (Camas Gomez et al., 2012), but tillage depletes organic matter and soil fertility over the long term (Verhulst et al., 2011; Fonteyne et al., 2021) which eventually reduces yield (Contreras-Hinojosa et al., 2005). The combination of zero tillage and MIAF to decrease erosion is therefore more sustainable.
Crop diversification had several benefits in this study. Treatments with bean rotations were under continuous production from 2014 to 2020, showing that a fallow period is not necessary to maintain maize productivity and that an extra food crop can be produced instead. In similar trials in Kenya and Nigeria, a one-year fallow period did not increase maize yields over uninterrupted monoculture, whereas rotating maize with a legume did (Bünemann et al., 2004; Yusuf et al., 2009). The data suggest that the traditional milpa produced more food than a maize monoculture in Tamazulapam, but it was difficult to obtain complete harvest data, as fresh beans, fig-leaf gourd flowers, and young shoots and edible plants were harvested continually throughout the growing season. In Falkowski et al. (2019), the collaborating farmer recorded all production from a 2-hectare milpa in the Lacandon region of the state of Chiapas, Mexico, near Guatemala, for three years. System output was enough to meet nearly all dietary needs of a family of five, lacking only in saturated fat, cholesterol, sodium, calcium, and iodine, although the actual amounts of food harvested varied within and between years. The edible secondary crops in a milpa systems, which provide most of the nutrients, often have a higher value than the main crop, maize, which provides most of the dietary energy (González-Amaro et al., 2009; Falkowski et al., 2019). Likewise, in Teopoxco, the vegetable peas proved a much higher value crop than maize.
Milpa systems are often mistaken for mainly maize production systems by scientists and extensionists (Falkowski et al., 2019; Lopez-Ridaura et al., 2021; Novotny et al., 2021). Taking maize, beans, and fig-leaf gourd production in Tamazulapam into account, the conventional milpa treatment was profitable all years. Association crops should thus not be overlooked, when trying to improve the profitability and food security potential of maize-based production systems. Maize-focused interventions such as increased nitrogen fertilization or maize cropping density may increase maize yields but can also reduce bean or fig-leaf gourd yields (Reyna-Ramírez et al., 2018). Research regarding the impacts of interventions on the yield of other components of multicrop systems is needed, because to date there is little published on the yield, profitably, or environmental impacts of traditional multicrop systems or the effects intercropping in general (Mt. Pleasant, 2016; Bybee-Finley and Ryan, 2018; Espidio et al., 2020).
Though fruit and vegetable production can be highly profitable, there are also limits to diversifying smallholder, hillside, maize-based cropping systems with those crops. In remote towns bringing produce to market can be difficult, especially if the harvest is relatively small. Teopoxco farmers sell their produce in the Mexico City wholesale market, but this is not an option for farmers in more remote towns of the mountainous region of our study such as Tamazulapam. Fruit and vegetable prices can also vary widely, as occurred in Teopoxco in 2018 and 2019. Nevertheless, despite market volatility, fruit production was more profitable than maize production at both sites. Strictly from an economic standpoint, it might be better for farmers to convert completely to fruit production, but this might reduce food security.
Our trial treatments had to be adapted over time to better suit the local conditions. Reyna-Ramírez et al. (2018) conducted a trial in a similar production system in Guerrero and also needed to adjust their treatments after learning more about local conditions in the initial year. These experiences largely reflect the lack of previous research in the study areas and highlight the need for adaptive trials on sustainable agriculture in agro-ecologies across the country (Fonteyne et al., 2021). Production systems are highly diverse across Mexico, and different interventions may be needed to improve these systems. To support the smallholder farmers in improving their production, it is therefore necessary to conduct more similar adaptive and participative field trials in other regions of the country.
The yields of landrace maize varieties can be increased sustainably in smallholder hillside cropping systems without the need for expensive inputs. Crop rotations and zero tillage were the practices that raised maize yields the most, while fertilization or liming were costly and relatively ineffective in that regard. Alley cropping increased the profitability of the system. Intensification made the systems more productive, with the inclusion of a relay crop of peas in Teopoxco or the maize-fig-leaf gourd-bean multicrop in Tamazulapam. Through adaptive investigation under farmers' conditions, locally adapted and sustainable intensification of traditional production system is possible through low-cost changes that lie within farmers' possibilities.
The datasets presented in this study can be found in online repositories. The names of the repository/repositories and accession number(s) can be found here: the datasets generated for this study can be found in Dataverse: https://hdl.handle.net/11529/10548616.
CS, AR, JT, FG, ZP, AG, and LC contributed to conception, design and investigation. CS and LC curated the data. SF, CS, and JT analyzed the data and wrote the first draft. NV reviewed and edited the manuscript. All authors contributed to manuscript revision, read, and approved the submitted version except for the late AR.
The trials were part of Cultivos para México/MasAgro Productor made possible by support of the Mexican Government through SADER. The data analysis and writing of the article was funded by CCAFS. The work of CCAFS is supported by CGIAR Fund Donors and through bilateral funding agreements. For details please visit https://ccafs.cgiar.org/donors. This work is part of the CGIAR Research Program on Maize (MAIZE) with support from W1&W2 donors, which include the Governments of Australia, Belgium, Canada, China, France, India, Japan, Korea, Mexico, Netherlands, New Zealand, Norway, Sweden, Switzerland, U.K., U.S., and the World Bank.
This paper is dedicated to the memory of Dr. Angel Ramos Sanchez (08/08/1934-15/06/2018) who initiated and designed the experiments and supported the first years of data collection and analysis.
Any opinions, findings, conclusion, or recommendations expressed are those of the authors and do not necessarily reflect the view of the donors.
The authors declare that the research was conducted in the absence of any commercial or financial relationships that could be construed as a potential conflict of interest.
All claims expressed in this article are solely those of the authors and do not necessarily represent those of their affiliated organizations, or those of the publisher, the editors and the reviewers. Any product that may be evaluated in this article, or claim that may be made by its manufacturer, is not guaranteed or endorsed by the publisher.
We thank the people from Mujeres Campesinas de Tamazulapam del Espíritu Santo for their collaboration in the trial in Tamazulapam del Espiritu Santo and Don Anselmo Hernández and Doña Aurelia Quevedo for their collaboration in the trial in Santa Maria Teopoxco, as well as everybody from CIMMYT who contributed to the trials over the years. We also thank Mike Listman for editing the draft manuscript and Juan Arista Cortes for the map.
The Supplementary Material for this article can be found online at: https://www.frontiersin.org/articles/10.3389/fagro.2021.787507/full#supplementary-material
Aitken, R., Moody, P., and Mckinley, P. (1990). Lime requirement of acidic Queensland soils. I. Relationships between soil properties and pH buffer capacity. Soil Res. 28:695. doi: 10.1071/SR9900695
Bayala, J., Kalinganire, A., Tchoundjeu, Z., Sinclair, F., and Garrity, D. (2011). Conservation agriculture with trees in the West African Sahel a review. ICRAF Occasional Paper No. 14. Nairobi, Kenya: World Agroforestry Centre.
Bolaños Gonzalez, M. A., Paz Pellat, F., Cruz Gaistardo, C. O., Argumedo Espinoza, J. A., Romero Benitez, V. M., and de la Cruz Cabrera, J. C. (2016). Erosion map of Mexico soils and its possible implications for soil organic carbon pool. Terra Latinoam. 34, 271–288.
Bünemann, E. K., Smithson, P. C., Jama, B., Frossard, E., and Oberson, A. (2004). Maize productivity and nutrient dynamics in maize-fallow rotations in western Kenya. Plant Soil 264, 195–208. doi: 10.1023/B:PLSO.0000047749.43017.fd
Bybee-Finley, K., and Ryan, M. (2018). Advancing intercropping research and practices in industrialized agricultural landscapes. Agriculture 8:80. doi: 10.3390/agriculture8060080
Cadena-Iñiguez, P., Camas-Gómez, R., López-Báez, W., López-Gómez, H. D. C., and González-Cifuentes, J. H. (2018). El MIAF, una alternativa viable para laderas en áreas marginadas del sureste de México: caso de estudio en Chiapas. Rev. Mex. Ciencias Agrícolas 9, 1351–1361. doi: 10.29312/remexca.v9i7.1670
Camas Gomez, R., Turrent Fernández, A., Cortes Flores, J., Livera Muñoz, M., González Estrada, A., Villar Sancehz, B., et al. (2012). Erosión del suelo, escurrimiento y pérdida de nitrógeno y fósforo en laderas bajo diferentes sistemas de manejo en Chiapas, México. Rev. Mex. Ciencias Agrícolas 3, 231–243.
Contreras-Hinojosa, J., Volke-Haller, V., Oropeza-Mota, J., Rodríguez-Franco, C., Martínez-Saldaña, T., and Martínez-Garza, Á. (2005). Reduccion del rendimiento de maiz por la erosion del suelo en Yanhuitlan, Oaxaca, Mexico. Terra Latinoam. 23, 399–408.
Donatti, C. I., Harvey, C. A., Martinez-Rodriguez, M. R., Vignola, R., and Rodriguez, C. M. (2019). Vulnerability of smallholder farmers to climate change in Central America and Mexico: current knowledge and research gaps. Clim. Dev. 11, 264–286. doi: 10.1080/17565529.2018.1442796
Espidio, J., Navarro-Garza, H., Flores-Sánchez, D., and Báez-Pérez, A. (2020). Diversidad de sistemas de cultivo y transición agroecológica: estudio de caso en la Sierra Norte del estado de Puebla, México. Agro Product. 13, 23–29. doi: 10.32854/agrop.vi.1530
Falkowski, T. B., Chankin, A., Diemont, S. A. W., and Pedian, R. W. (2019). More than just corn and calories: a comprehensive assessment of the yield and nutritional content of a traditional Lacandon Maya milpa. Food Secur. 2019, 389–404. doi: 10.1007/s12571-019-00901-6
Fonteyne, S., Burgueño, J., Albarrán Contreras, B. A., Andrio Enríquez, E., Castillo Villaseñor, L., and Enyanche Velázquez, F. (2021). Effects of conservation agriculture on physicochemical soil health in 20 maize-based trials in different agro-ecological regions across Mexico. L. Degrad. Dev. 2021, 1–15. doi: 10.1002/ldr.3894
Fonteyne, S., González Regalado, J., López Ramírez, A., and Verhulst, N. (2017a). “Aumento del rendimiento y la rentabilidad del cultivo de maíz usando agricultura de conservación en Molcaxac, Puebla,” in Red de plataformas de investigacion MasAgro—Resultados PV2016 y OI 2016-17, eds S. Fonteyne and N. Verhulst (Texcoco, Mexico: CIMMYT), 172–178.
Fonteyne, S., Martinez Gamiño, M.-A., Saldivia Tejeda, A., and Verhulst, N. (2019). Conservation agriculture improves long-term yield and soil quality in irrigated maize-oats rotation. Agronomy 9:845. doi: 10.3390/agronomy9120845
Fonteyne, S., Peñaloza, O. N., Alcalá, L. O., Rodríguez, C. S., and Villalcantara, J. (2017b). “Control de malezas en ma?z en tres regiones de Oaxaca: Primeros resultados de diferentes manejos de malezas en labranza convencional, mínima y cero,” in Red de Plataformas de Investigacion MasAgro - Resultados PV2016 y OI 2016-17, eds S. Fonteyne and N. Verhulst (Mexico: CIMMYT), 146–151.
Fonteyne, S., Singh, R. G., Govaerts, B., and Verhulst, N. (2020). Rotation, mulch and zero tillage reduce weeds in a long-term conservation agriculture trial. Agronomy 10:962. doi: 10.3390/agronomy10070962
Gardeazabal, A., Lunt, T., Jahn, M. M., Verhulst, N., Hellin, J., and Govaerts, B. (2021). Knowledge management for innovation in agri-food systems: a conceptual framework, Knowledge Management Research & Practice. doi: 10.1080/14778238.2021.1884010
González-Amaro, R. M., Martínez-Bernal, A., Basurto-Peña, F., and Vibrans, H. (2009). Crop and non-crop productivity in a traditional maize agroecosystem of the highland of Mexico. J. Ethnobiol. Ethnomed. 5, 3–11. doi: 10.1186/1746-4269-5-38
Govaerts, B., François, I., and Verhulst, N. (2017). “Conservation agriculture (CA) for sustainable intensifi cation of maize and other cereal systems: the case of Latin America,” in Conservation agriculture for sustainable intensification of maize and other cereal systems (London, UK: Burleigh Dodds Science Publishing Limited), 81–106.
Govaerts, B., Negra, C., Camacho Villa, T. C., Chavez Suarez, X., Espinosa, A. D., Fonteyne, S., et al. (2021). One CGIAR and the Integrated Agri-food Systems Initiative: from short-termism to transformation of the world's food systems. PLoS ONE 16:e252832. doi: 10.1371/journal.pone.0252832
Hellin, J., and López-Ridaura, S. (2016). Soil and water conservation on Central American hillsides: if more technologies is the answer, what is the question? Agric. Food 1, 194–207. doi: 10.3934/agrfood.2016.2.194
Lal, R. (2015). Sequestering carbon and increasing productivity by conservation agriculture. J. Soil Water Conserv. 70, 55A−62A. doi: 10.2489/jswc.70.3.55a
Lopez-Ridaura, S., Barba-Escoto, L., Reyna-Ramirez, C. A., Sum, C., Palacios-Rojas, N., and Gerard, B. (2021). Maize intercropping in the milpa system. Diversity, extent and importance for nutritional security in the Western Highlands of Guatemala. Sci. Rep. 11:3696. doi: 10.1038/s41598-021-82784-2
Monjardino, M., Santiago, L., Van Loon, J., Mottaleb, K. A., Kruseman, G., Ortiz, E., et al. (2021). Disaggregating the value of conservation agriculture to inform smallholder transition to sustainable farming: a Mexican case study. Agronomy 11:1214. doi: 10.3390/agronomy11061214
Mt. Pleasant, J. (2016). Food yields and nutrient analyses of the three sisters: A Haudenosaunee cropping system. Ethnobiol. Lett. 7, 87–98. doi: 10.14237/ebl.7.1.2016.721
Murray-Tortarolo, G. N., Jaramillo, V. J., and Larsen, J. (2018). Food security and climate change: the case of rainfed maize production in Mexico. Agric. For. Meteorol. 253–254, 124–131. doi: 10.1016/j.agrformet.2018.02.011
Ndoli, A., Baudron, F., Sida, T. S., Schut, A. G. T., van Heerwaarden, J., and Giller, K. E. (2018). Conservation agriculture with trees amplifies negative effects of reduced tillage on maize performance in East Africa. F. Crop. Res. 221, 238–244. doi: 10.1016/j.fcr.2018.03.003
Nichols, V., Verhulst, N., Cox, R., and Govaerts, B. (2015). Weed dynamics and conservation agriculture principles: a review. F. Crop. Res. 183, 56–68. doi: 10.1016/j.fcr.2015.07.012
Novotny, I. P., Tittonell, P., Fuentes-Ponce, M. H., López-Ridaura, S., and Rossing, W. A. H. (2021). The importance of the traditional milpa in food security and nutritional self-sufficiency in the highlands of Oaxaca, Mexico. PLoS ONE 16, 1–21. doi: 10.1371/journal.pone.0246281
Odjo, S., Burgueño, J., Rivers, A., and Verhulst, N. (2020). Hermetic storage technologies reduce maize pest damage in smallholder farming systems in Mexico. J. Stored Prod. Res. 88:101664. doi: 10.1016/j.jspr.2020.101664
Pfeiffer, L., López-Feldman, A., and Taylor, J. E. (2009). Is off-farm income reforming the farm? Evidence from Mexico. Agric. Econ. 40, 125–138. doi: 10.1111/j.1574-0862.2009.00365.x
R Core Team (2020). R: A Language and Environment for Statistical Computing. R Foundation for Statistical Computing, Vienna, Austria. Available Online at: https://www.R-project.org/.
Reyes Jaramillo, I. (2016). Propiedades edáficas de parcelas cultivadas con milpa usando labranza mínima en la sierra de Oaxaca, donde crecía bosque mesófilo de montaña. Polibotánica 41, 133–151. doi: 10.18387/polibotanica.41.9
Reyna-Ramírez, C. A., Rodríguez-Sánchez, L. M., Vela-Correa, G., Etchevers-Barra, J., and Fuentes-Ponce, M. (2018). Redesign of the traditional Mesoamerican agroecosystem based on participative ecological intensification: evaluation of the soil and efficiency of the system. Agric. Syst. 165, 177–186. doi: 10.1016/j.agsy.2018.06.013
Ruiz Mendoza, A. D., Jiménez Sánchez, L., Figueroa Rodríguez, O. L., and Morales Guerra, M. (2012). Adopción del sistema milpa intercalada en árboles frutales por cinco municipios mixes del estado de Oaxaca. Rev. Mex. Ciencias Agrícolas 3, 1605–1621. doi: 10.29312/remexca.v3i8.1324
Santiago-Mejía, E., Cortés-Flores, J. I., Turrent-Fernández, A., Hernández-Romero, E., and Jaen-Contreras, D. (2008). Calidad del fruto del duraznero en el sistema milpa intercalada con Árboles frutales en laderas. Agric. Técnica en México 34, 159–166.
SEMARNAT (2016). Informe de la Situación del Medio Ambiente en México. Compendio de Estadísticas Ambientales. Indicadores Clave, de Desempeño Ambiental y de Crecimiento Verde. Edición 2015. Mexico City, Mexico.
SIAP (2020). Estadística de Producción Agrícola. Available online at: http://infosiap.siap.gob.mx/gobmx/datosAbiertos.php (accessed September 22, 2020).
Sims, B., Friedrich, T., Kassam, A., and Kienzle, J. (2009). “Agroforestry and conservation agriculture: complementary practices for sustainable development,” in Annual General Meeting, Royal Overseas League, 9 December 2009., II World Congress of Agroforestry, Nairobi, Kenya, 23-28 August 2009.; TAA South-West Group meeting on Ethiopia-a review of some conservation. 13–18. Available online at: http://www.cabdirect.org/abstracts/20103040585.html.
Thierfelder, C., Baudron, F., Setimela, P., Nyagumbo, I., Mupangwa, W., Mhlanga, B., et al. (2018). Complementary practices supporting conservation agriculture in southern Africa. A review. Agron. Sustain. Dev. 38:16. doi: 10.1007/s13593-018-0492-8
Torres Zambrano, J. P., Cortés Flores, J. I., Turrent Fernández, A., Hernández Romero, E., and Muratalla Lua, A. (2008). Fruit yield and number of scaffold branches in peach trees intercropped with milpa. Terra Latinoam 26, 265–273.
Turrent Fernández, A., Cortés Flores, J. I., Espinosa Calderón, A., Hernández Romero, E., Camas Gómez, R., Torres Zambrano, J. P., et al. (2017). MasAgro o MIAF ¿Cuál es la opción para modernizar sustentablemente la agricultura tradicional de México. Rev. Mex. Ciencias Agrícolas 8:1169. doi: 10.29312/remexca.v8i5.116
Ureta, C., González, E. J., Espinosa, A., Trueba, A., Piñeyro-Nelson, A., and Álvarez-Buylla, E. R. (2020). Maize yield in Mexico under climate change. Agric. Syst. 177:102697. doi: 10.1016/j.agsy.2019.102697
Van Deynze, A., Zamora, P., Delaux, P.-M., Heitmann, C., Jayaraman, D., Rajasekar, S., et al. (2018). Nitrogen fixation in a landrace of maize is supported by a mucilage-associated diazotrophic microbiota. PLOS Biol. 16:e2006352. doi: 10.1371/journal.pbio.2006352
Van Loon, J., Woltering, L., Krupnik, T. J., Baudron, F., Boa, M., and Govaerts, B. (2020). Scaling agricultural mechanization services in smallholder farming systems: Case studies from sub-Saharan Africa, South Asia, and Latin America. Agric. Syst. 180:102792. doi: 10.1016/j.agsy.2020.102792
Vergara-Sánchez, M. Á., Etchevers-Barra, J. D., and Padilla-Cuevas, J. (2005). Hillside soils fertility at the Oaxaca's Northern Sierra, México. Agrociencia. 39, 259–266.
Verhulst, N., Govaerts, B., Nelissen, V., Sayre, K. D., Crossa, J., Raes, D., et al. (2011). The effect of tillage, crop rotation and residue management on maize and wheat growth and development evaluated with an optical sensor. For. Crop. Res. 120, 58–67. doi: 10.1016/j.fcr.2010.08.012
Villavicencio Rojas, J. M. (2013). Tierra y Violencia en la Sierra Sur de Oaxaca, México. Hist. y Mem. 67:1963. doi: 10.19053/20275137.1963
Yusuf, A. A., Abaidoo, R. C., Iwuafor, E. N. O., Olufajo, O. O., and Sanginga, N. (2009). Rotation effects of grain legumes and fallow on maize yield, microbial biomass and chemical properties of an Alfisol in the Nigerian savanna. Agric. Ecosyst. Environ. 129, 325–331. doi: 10.1016/j.agee.2008.10.007
Keywords: maize (Zea mays L.), beans (Phaseolus vulgaris L.), squashes, multicropping, crop rotation, milpa, MIAF
Citation: Fonteyne S, Silva Avendaño C, Ramos Sanchez A, Torres Zambrano JP, García Dávila F, Pérez Martínez Z, García Dávila A, Castillo Villaseñor L and Verhulst N (2022) Innovating Traditional Production Systems Through On-Farm Conservation Agriculture and Agroforestry Research. Front. Agron. 3:787507. doi: 10.3389/fagro.2021.787507
Received: 30 September 2021; Accepted: 07 December 2021;
Published: 10 January 2022.
Edited by:
Lydiah Gatere, Independent Researcher, Nairobi, KenyaReviewed by:
Mina Devkota, International Center for Agricultural Research in the Dry Areas (ICARDA), MoroccoCopyright © 2022 Fonteyne, Silva Avendaño, Ramos Sanchez, Torres Zambrano, García Dávila, Pérez Martínez, García Dávila, Castillo Villaseñor and Verhulst. This is an open-access article distributed under the terms of the Creative Commons Attribution License (CC BY). The use, distribution or reproduction in other forums is permitted, provided the original author(s) and the copyright owner(s) are credited and that the original publication in this journal is cited, in accordance with accepted academic practice. No use, distribution or reproduction is permitted which does not comply with these terms.
*Correspondence: Nele Verhulst, bi52ZXJodWxzdEBjZ2lhci5vcmc=
†Deceased
Disclaimer: All claims expressed in this article are solely those of the authors and do not necessarily represent those of their affiliated organizations, or those of the publisher, the editors and the reviewers. Any product that may be evaluated in this article or claim that may be made by its manufacturer is not guaranteed or endorsed by the publisher.
Research integrity at Frontiers
Learn more about the work of our research integrity team to safeguard the quality of each article we publish.