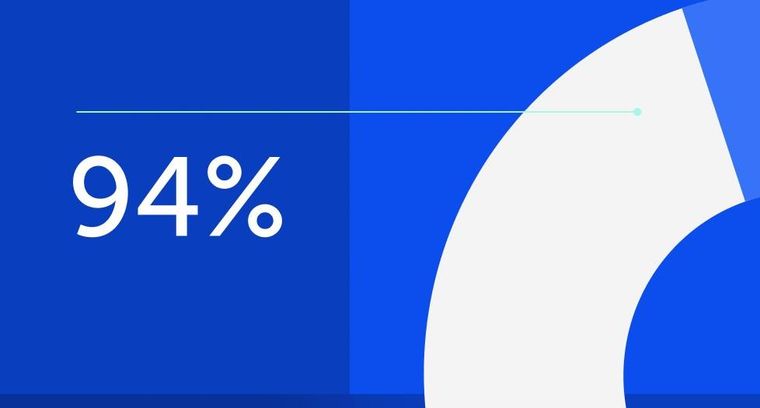
94% of researchers rate our articles as excellent or good
Learn more about the work of our research integrity team to safeguard the quality of each article we publish.
Find out more
ORIGINAL RESEARCH article
Front. Agron., 09 September 2021
Sec. Plant-Soil Interactions
Volume 3 - 2021 | https://doi.org/10.3389/fagro.2021.725813
Soybean [Glycine max (L.) Merr.] is the world's leading legume crop and the largest oilseed crop. It forms a symbiotic relationship with rhizobia bacteria residing in root nodules that provide fixed nitrogen to host plants through symbiotic nitrogen fixation (SNF). In soybean, it has been widely reported that the highest SNF occurs at the pod-filling stage, associated with the peak demand for nitrogen. However, the majority of seed nitrogen is derived from remobilizing root/shoot nitrogen, representing cumulative SNF from the seedling stage to the pre-pod-fill stage. Therefore, the question arises as to whether there has also been selection for improved SNF at these earlier stages, or whether pre-pod-fill SNF traits have drifted. To test this hypothesis, in this study, pre-pod SNF-related traits were evaluated in soybean cultivars that span 100 years of breeding selection in the Canadian Province of Ontario. Specifically, we evaluated SNF traits in 19 pedigree-related historical cultivars and 25 modern cultivars derived from the University of Guelph soybean breeding program. Field trials were conducted at Woodstock, Ontario, Canada in 2016 and 2017, and various SNF-related traits were measured at pre-pod-fill stages (R1-R3), including nitrogen fixation capacity. Considerable variation was observed among Canadian soybean cultivars released over the past 100 years for pre-pod-fill nitrogen fixation. The modern soybean cultivars had similar or moderately higher pre-pod-fill SNF compared to the historical lines in terms of the percentage of nitrogen derived from the atmosphere (%Ndfa) and total shoot fixed nitrogen. These findings suggest that, despite no direct selection by breeders, pre-pod-fill nitrogen fixation, and associated SNF traits have been maintained and possibly improved in modern soybean breeding. However, the low level of pre-pod-fill SNF in some modern cultivars, and generally wide variation observed in SNF between them, suggest some level of genetic drift for this trait in some pedigrees. Specific historical and modern soybean cultivars were identified as potential parents to enable targeted breeding for improved pre-pod-fill SNF. This retrospective study sheds light on our understanding of the impact of decades of recent selective breeding on pre-pod-fill nitrogen fixation traits in soybean in a temperate environment.
Soybean or soya bean [Glycine max (L.) Merr.] is the most important legume crop in the world in terms of production, and a major source of human protein, animal feed, and vegetable oil. Soybean oil is now also becoming an important feedstock for biodiesel production (Hartman et al., 2011). Soybean production has increased substantially during the last five decades, mainly due to the expansion of soybean growing areas (Liu et al., 2020). It is widely believed that cultivated soybean originated and was domesticated from wild soybean Glycine soja (Sieb. & Zucc.) in East Asia 6000–9000 years ago (Guo et al., 2010; Wang et al., 2016) and then introduced to North America in 1765 (Hymowitz and Harlan, 1983). Soybean was introduced to the Canadian province of Ontario in 1881 (Shurtleff and Aoyagi, 2010), and has now grown into one of the region's most valuable field crops. In Canada, 2.05 million ha of agricultural lands were cultivated with soybean in 2020, producing 6.36 MMT of grain (Soy Canada, 2021).
One of the fascinating features associated with soybean is that its roots can form a mutualistic interaction with specialized soil bacteria termed rhizobia that inhabit underground root nodules; the rhizobia convert atmospheric nitrogen to fixed ammonia (Thilakarathna and Raizada, 2017). Generally, soybean is considered to be a high nitrogen fixing legume, wherein 68% of the plant's nitrogen, on average, can be derived from symbiotic nitrogen fixation (SNF) (Herridge et al., 2008). Because of this high nitrogen fixation ability, soybean is often used in crop rotations, where it facilitates a nitrogen credit to the proceeding crop (Karlen et al., 2006; Ziadi et al., 2014; Attia et al., 2015). However, the percentage of nitrogen derived from the atmosphere (%Ndfa) in soybean can vary from 0 to 95% (Herridge et al., 2008). This high variation in nitrogen fixation can be due to both biotic factors (e.g., genotypes, rhizobia spp.) as well as abiotic factors (e.g., drought, temperature, soil nitrogen availability) (Hungria and Vargas, 2000; Giller, 2001; Thilakarathna and Raizada, 2017, 2018; Santachiara et al., 2019).
Nitrogen fixation in soybean can vary depending on the growth stages of the plant. In general, SNF is lowest during the vegetative stage, then rapidly increases between R1 and R3 growth stages, before peaking then dropping upon pod formation (post R5 stage) (Zapata et al., 1987; Pitumpe Arachchige et al., 2020). In soybean, a significant portion (59%) of seed N is derived from remobilization of vegetative N, which represents N accumulated from SNF and soil uptake at the R5.5 stage (Ortez et al., 2019). In-season SNF provides nitrogen inputs to develop vegetative biomass, and SNF is positively correlated to the aboveground plant biomass in soybean (Córdova et al., 2019). Therefore, SNF inputs during the vegetative stage, flowering stage, and pre-pod-fill stage help to build the plant's N reserves and subsequently contribute to seed yield and seed protein production (Ortez et al., 2019).
Modern soybean breeding programs such as the program in Ontario have mainly focused on improving yield, oil content and quality, disease resistance, and days to maturity (Morrison et al., 2000; Jin et al., 2010; Bruce et al., 2019a; Liu et al., 2020). By contrast, direct selection for improved symbiotic nitrogen fixation traits has not been a priority. Similar to the practice of farmers, the Guelph soybean breeding program has been conducted in the absence of synthetic nitrogen inputs, instead relying on residual soil nitrogen. We hypothesized that there may have been inadvertent selection for enhanced SNF traits (e.g., nodule number) resulting in improved overall nitrogen fixation. The corollary hypothesis is that modern cultivars are not suffering from genetic drift with respect to SNF traits, as a result of continuous indirect selection.
The Ontario Agriculture College (OAC) at the University of Guelph began breeding soybeans in 1970, aiming to produce high-yielding cultivars for Ontario growers (Bruce et al., 2019b). A recent study categorized the cultivars released from the University of Guelph into two groups, pedigree-related historical germplasm (1913–1985) and modern cultivars (cultivars developed at the Guelph campus and released between 1983 and 2015) (Bruce et al., 2019a,b). These cultivars have not been systematically analyzed for pre-pod-fill SNF related traits. The objectives of this study were: (1) to evaluate and compare the Guelph cultivars, based on their year of release from the historical group and modern group, for trends in pre-pod-fill SNF-related traits and accumulated vegetative nitrogen fixation as measured during the R1-R3 stages; (2) to understand the relationships between different agronomic traits for pre-pod-fill SNF; and (3) to identify historic and modern soybean cultivars as potential sources for improved nitrogen fixation at this stage.
Soybean cultivars for this study were selected from a large study that evaluated the changes in soybean traits over 100 years of selection in Ontario, Canada (Bruce et al., 2019a). Among the 44 soybean cultivars selected for this study, 19 cultivars were pedigree related historical cultivars that were released between 1913 and 1985, and 25 cultivars were selected from the Guelph soybean (modern) breeding program, which were released between 1983 and 2015 (Figure 1; Table 1). The list of published cultivar registration papers with the year of public release is previously described by Bruce et al. (2019a). Pedigrees were visualized using GraphViz 2.38 (www.graphviz.org) allowing historical connections to be organized according to year-of-release for studied accessions (Supplementary Figure 1). All the soybean cultivars were grown at the Woodstock Research Station (Woodstock, ON; 43.145223° N, 80.782389° W; Guelph loam soil type [fine-loamy, mixed, active, mesic Haplic Glossudalfs]) in 2016 and 2017, as part of a larger breeding program study. Each field was previously cultivated with corn in 2015 and 2016. In 2016, the field was supplied with N (10 kg N ha−1), P (40 kg P ha−1), and K (40 kg K ha−1) fertilizer, wherein only K (10 kg ha−1) fertilizer was applied in 2017 before soybean seeding. Broadstrike (RC 86.5 g ha−1) and Dual II Magnum (1.73 L ha−1) were applied as pre-plant herbicides, and Reflex (1 L ha−1), Pinnacle Toss-n-Go (1 pouch ha−1), Venture (2 L ha−1), and Turbocharge (1 L ha−1) were applied as post-emergence herbicides in both production years. Soybean cultivars were planted as a replicated randomized completely block design, with two blocks, wherein each plot consisted of 4 rows, spaced 35 cm apart, and 5 m in length. A 40 cm space between plots and 2 m alley was maintained. Seeds were planted on 8 June in both 2016 and 2017 using a tractor-mounted planter at a seeding rate of 500 seeds per plot. Seeds were not inoculated with commercial rhizobia inoculants as the fields have been used for soybean in the past. Plants were grown under rainfed conditions and plots were not irrigated. Monthly daily maximum, minimum, daily mean temperatures, and precipitation during the 2016 and 2017 growing seasons are shown in Supplementary Table 1. Total rainfall received during the two growing seasons (May-June) was 448.8 mm (2016) and 442.9 mm (2017). The field site at Woodstock, Ontario received 3,421 crop heat units (CHU) in 2016 and 3,408 CHU in 2017.
Figure 1. Images of historical (19) and modern-Guelph (25) soybean cultivars used in this study with name and release year.
Table 1. Ranking of soybean cultivars based on accumulated nitrogen derived from the atmosphere (%Ndfa) in shoots prior to the pod-filling stage in the 2016 and 2017 seasons.
In 2016 and 2017, three representative plants were collected at the R1-R3 stage from the middle rows at 8 weeks after seeding from each plot representing each soybean genotype. Plants were uprooted using a shovel, where roots and shoots were separated to measure the number of nodules, nodule dry weight, root dry weight, and shoot dry weight. The dry weight of tissue samples was measured after drying samples for 3 days in a forced air oven at 60°C. Shoot samples were ground using a Wiley mill standard model 3 (Arthur H Thomas Co., Philadelphia, PA) to pass through a 1-mm sieve followed by a Bead Ruptor 12 Homogenizer (OMNI International GA) (Thilakarathna et al., 2017). The shoot materials were analyzed for 15N (shoot-δ15N), %N (shoot-N%), and %C using an Isotope Ratio Mass Spectrometer (IRMS) fitted with a Flash 2000 Elemental Analyzer (Thermo Fisher Scientific, Voltaweg, Netherlands) and Conflo IV (Thermo Fisher Scientific, Bremen, Germany) interface between the IRMS and the analyzer at the Stable Isotope Lab, Agriculture and Agri-Food Canada, Lethbridge Research and Development Centre. The proportion of N derived from the atmosphere (%Ndfa) in different soybean cultivars was calculated according to the natural abundance method (Wilker et al., 2019):
Where δ 15N is the 15N enrichment of shoot materials relative to atmospheric nitrogen. The δ 15N reference plant was the mean of two reference weeds {Thistle [Cirsium arvense (L.) Scop.] and Lamb's quarters [Chenopodium album L.]} collected from the same fields, which was used as the non-N-fixing reference plant. The B value is the 15N enrichment of the soybean shoots grown solely on atmospheric N, where a B value of −1.674 δ15N was used. The amount of shoot N derived from nitrogen fixation was calculated based on the shoot N content (mg N plant−1) and %Ndfa (shoot-N content × %Ndfa/100).
Phenotypic data of SNF-related traits [nodule number, nodule dry weight, shoot and root biomass, shoot total N content, shoot 15N abundance (δ15N), %Ndfa, and total shoot N fixed] were analyzed using analysis of variance (ANOVA). Also, a nested t-test was performed to compare historical vs. modern-Guelph cultivars within each production year for agronomic traits. Results were expressed at a significance level of p < 0.05. Linear regression of different plant traits by year of cultivar release was conducted (p < 0.05). Correlation analysis between different plant traits was performed using the Pearson correlation test. All these statistical analyses were performed using GraphPad Prism Software (v9, GraphPad Software, San Diego, CA, USA).
The panel of 44 soybean cultivars was selected for this study as representative of Central Canadian (Ontario) soybean germplasm diversity over the last 100 years. Of these cultivars, 19 were historical founding accessions as traced through pedigree records (released between 1913 and 1985) while 25 were elite cultivars derived from modern breeding at the University of Guelph (released between 1983 and 2015) (Figure 1). As such, this panel represents the current diversity of Ontario soybean germplasm. All these cultivars were grown at the Woodstock Research Station (University of Guelph, ON, Canada) in 2016 and 2017, and phenotypic data for 10 SNF-related traits were measured (Figure 2). Significant year × cultivar interaction was observed for all the pre-pod-fill parameters tested, except for shoot dry weight (SDW) (Supplementary Table 2). Therefore, data are presented separately for each year.
Figure 2. Distribution of SNF-related traits in historical and modern-Guelph lines in 2016 and 2017 prior to pod-fill. (A,B) Number of nodules per plant. (C,D) Nodule dry weight per plant. (E,F) Root dry weight per plant. (G,H) Shoot dry weight per plant. (I,J) Shoot nitrogen percentage. (K,L) Shoot total nitrogen content per plant. (M,N) Shoot C/N ratio. (O,P) Shoot δ15N. (Q,R) Percentage nitrogen derived from the atmosphere (%Ndfa). (S,T) Shoot nitrogen fixed per plant. Each data point represents the mean value of a single cultivar. The asterisks (*, **) indicate significant difference between the 19 historical and 25 modern-Guelph cultivars at p < 0.05 and p < 0.01, respectively.
In general, the pre-pod-fill nodule number, nodule dry weight, root dry weight, and shoot dry weight were higher in 2017 compared to the 2016 production year (Figures 2A–H). Historical cultivars had higher number of nodules in 2016 (Figure 2A). No significant differences were found for pre-pod-fill nodule dry weight, root and shoot dry weight, shoot-N%, shoot-N content, shoot-C/N ratio, and shoot-δ15N among the historical vs. modern-Guelph categories in 2016 (Figures 2C–P). However, the root and shoot dry weight were significantly higher in modern-Guelph cultivars compared to the historical cultivars in 2017. The shoot-δ15N content was higher in historical cultivars in 2017 compared to the modern-Guelph cultivars (Figure 2P).
We observed a similar level of pre-pod-fill %Ndfa (53%) between 2016 and 2017 (Figures 2Q,R). When %Ndfa was analyzed for each year, no significant differences were found between historical and modern-Guelph cultivars in 2016, but modern-Guelph cultivars had higher %Ndfa (55.5%) compared to the historical cultivars (49.5%) in 2017 (Figure 2R). However, the amount of shoot-N fixed prior to the pod-fill stage was higher in 2016 (123.7 mg N plant−1) compared to 2017 (114.1 mg N plant−1) (Figures 2S,T). Similar to the %Ndfa trend, no significant differences in the amount of total shoot-N fixed were found between historical and modern-Guelph cultivars in 2016 prior to pod fill (Figure 2S). However, modern-Guelph cultivars fixed more total shoot N (122 g N plant−1) in 2017 compared to the historical cultivars (105 g N plant−1) (Figure 2T).
All the soybean cultivars were ranked based on their shoot vegetative nitrogen fixation capacity prior to pod fill as measured by the %Ndfa (Table 1). Across the two growing seasons, %Ndfa varied from 29.9% to 67.6%, with an average value of 53.2%. In 2016, %Ndfa varied from 29.2% (OAC Nation) to 69.1% (OAC 211), with an average value of 53.1%, whereas in 2017 %Ndfa varied from 26.8% (AK Harrow) to 69.5% (OAC Champion), with an average of 52.9%. Although no significant difference was found for %Ndfa between historical and modern-Guelph groups in the 2016 season, when ranked overall, four of the five bottom ranked cultivars (Capital, AK Harrow, Ozzie, Evans) belonged to the historical category (Table 1). As mentioned above, a significant difference was found for shoot %Ndfa between historical and modern-Guelph cultivars in 2017; it was observed that four of the five top-ranked cultivars in 2017 (OAC Champion, OAC Frontier, OAC Scorpio, OAC Bayfield) belonged to the modern-Guelph category (Table 1).
Regression analysis was performed across different agronomic traits by year of cultivar release to identify trends since 1915. For nodule number and nodule dry weight prior to pod-fill, no significant trends were identified based on the year of cultivar release either in 2016 or 2017 (Figures 3A–D). Similarly, no significant trends were identified for root and shoot dry weights based on the year of cultivar release in both 2016 and 2017 (Supplementary Figures 2A–D). Furthermore, no significant trends were observed for shoot N% (Supplementary Figures 2E,F), shoot C/N ratio (Supplementary Figures 2G,H), and total shoot N content prior to pod fill (Supplementary Figures 2I,J) based on the year of cultivar release either in 2016 or 2017.
Figure 3. Trait by year of cultivar release regression plots for pre-pod-fill SNF-related traits. (A,B) Number of nodules per plant. (C,D) Nodule dry weight per plant. (E,F) Shoot δ15N. (G,H) Percentage nitrogen derived from the atmosphere (%Ndfa). (I,J) Shoot nitrogen fixed per plant. Each blue and purple dot represents a historical and modern-Guelph cultivar, respectively. %Ndfa and N-fixed represent the vegetative shoot values. NS, not significantly different at p < 0.05.
We found a significant negative trend for pre-pod fill shoot δ15N (p = 0.0151, r = −0.3640) based on the year of cultivar release in 2017 (Figure 3F), but this trend was not observed in 2016 (Figure 3E). In 2017, significant positive trends were found for %Ndfa (p = 0.0169, r = 0.3586) and total shoot-N fixed (p = 0.0297, r = 0.3282) based on the year of cultivar release (Figures 3H,J, respectively), but no significant trends were observed for %Ndfa and total shoot-N fixed in 2016 (Figures 3G,I).
Across all 44 cultivars, we found that pre-pod-fill nodule number was positively correlated to nodule dry weight (r = 0.68, 0.66), root dry weight (r = 0.31, 0.35), shoot dry weight (r = 0.34, 0.30), and total shoot-N content (r = 0.30, 0.32) in both 2016 and 2017, respectively (Figure 4). Additionally, there was a negative correlation between nodule number and shoot-C/N ratio (r = −0.32) in 2016. Nodule dry weight was positively correlated to root dry weight (r = 0.56, 0.48), shoot dry weight (r = 0.70, 0.59), and total shoot-N content (r = 0.47, 0.39) in 2016 and 2017, respectively (Figure 4). We also found that root dry weight was positively correlated with shoot dry weight (r = 0.53, 0.71), and total shoot-N content (r = 0.39, 0.61) in both 2016 and 2017, respectively (Figure 4). Across the cultivars, there was no significant correlation between the pre-pod-fill nodule number and %Ndfa or amount of shoot-N fixed either in 2016 or 2017. However, nodule dry weight was positively correlated to %Ndfa in 2016 (r = 0.30) and amount of shoot-N fixed in 2016 (r = 0.50) and 2017 (r = 0.37) (Figure 4). Additionally, the amount of shoot-N fixed prior to pod fill was positively correlated to root dry weight (r = 0.31, 0.44), shoot dry weight (r = 0.64, 0.54), total shoot-N content (r = 0.75, 0.73), and %Ndfa (r = 0.71, 0.39) in 2016 and 2017, respectively (Figure 4).
Figure 4. Pearson correlation matrix for pre-pod-fill nodulation parameters (nodule number and dry weight), plant growth (root and shoot dry weight), shoot nitrogen (nitrogen concentration and total nitrogen), shoot δ15N, shoot C/N ratio, and shoot nitrogen fixation (%Ndfa and total nitrogen fixed) in soybean in 2016 and 2017 (using mean data). Correlation coefficients (r) in individual cells represent each correlation between variables. The boldness of the color represents the strength of the relationship between variables, with stronger correlations having bolder colors.
Accumulated symbiotic nitrogen fixation starting from the seedling stage to the pre-pod fill stage is important to fulfill part of the N demand for soybean grain yield and seed protein. Despite peak nitrogen demand occurring at the pod fill stage in soybean, overall the results of this study highlight that modern soybean breeding in the Canadian province of Ontario has maintained, and possibly modestly improved, the absolute rates of pre-pod-fill symbiotic nitrogen fixation, based on 2 years of field trials. Three lines of evidence support this statement. First, in the 2017 trial, across the 44 cultivars, the pre-pod-fill shoot %Ndfa was significantly higher amongst the modern cultivars, though no significant difference was observed in 2016. Based on the cultivar year of release, the total nitrogen fixed and shoot %Ndfa prior to pod fill showed a significant but modest positive trend in the 2017 trial with no trend in 2016. Among the bottom ranked 10 cultivars for %Ndfa, seven cultivars were historical cultivars, suggesting modern cultivars may have improved SNF; perhaps breeding has removed some but not all of the worst SNF traits prior to pod fill. Improvement in pre-pod-fill SNF has been modest: the average nitrogen fixation capacity (averaging both trial years) was 51.3% for the historical cultivars compared to 54.6% for the modern cultivars. These SNF results align with the recently published average soybean %Ndfa values (56%) (Ciampitti and Salvagiotti, 2018).
Despite the above trends, a critical observation is that there was wide variation amongst the modern Guelph lines for %Ndfa (41–68%) and other SNF traits prior to pod fill, suggesting that in some pedigrees, SNF may have suffered from some level of genetic drift because of a lack of direct selection for SNF traits prior to the peak demand for nitrogen at the pod fill stage. To the best of our knowledge, soybean breeding in Ontario has not directly selected for improved SNF rates either before or during pod fill. This was consistent with the results of this study that showed no significant trends for nodulation parameters and shoot nitrogen based on the year of cultivar release. In soybean, nodulation and SNF rates vary depending on the growth stage (Zapata et al., 1987; Yinbo et al., 1997; Gan et al., 2003). In general, nitrogen fixation sharply increases starting at the flowering stage (R1) and peaks at R4-R5; the pre-pod SNF provides substantial N inputs to develop the aboveground biomass (Zapata et al., 1987; Yinbo et al., 1997; Ortez et al., 2019). These nitrogen reserves in the vegetative biomass help to meet subsequent seed nitrogen demand in soybean, especially since SNF drops post- R5.5, during the pod filling stages and/or under limited soil nitrogen supply (Córdova et al., 2019). It would be interesting to explore whether changes in alleles responsible for vegetative nitrogen remobilization to pods (Masclaux-Daubresse et al., 2010; Zhao et al., 2014) have an inverse correlation with alleles that promote SNF traits late in development. In soybean, variation in N remobilization from leaves during pod fill has been observed (Locke and Ramirez, 2020). Limited soil nitrogen during pod-filling is relevant to Canadian growing conditions, since farmers do not apply nitrogen fertilizer for soybean production. Therefore, it may be helpful to identify soybean varieties that have high SNF prior to the pre-pod-fill stage to meet the subsequent nitrogen demand of the seeds.
Modern soybean breeding programs, including in Ontario, mostly focus on yield and seed oil quality improvement, not nitrogen fixation as already noted (Bruce et al., 2019a; Liu et al., 2020). However, as soybean breeding trials in Ontario have been conducted in the absence or minimum of nitrogen inputs, breeding for improved grain yields may have maintained or improved biological nitrogen fixation even at the pre-pod-fill stage—despite breeders not directly selecting for improved SNF rates. Previous studies have shown positive correlations between grain yield and SNF in soybean (Salvagiotti et al., 2008; Collino et al., 2015; Ciampitti and Salvagiotti, 2018).
Nodules are the major nitrogen source tissues that support vegetative and reproductive sink development. It is highly likely that selecting for improved soybean yield (i.e., reproductive sink development) has inadvertently impacted the source (nodule) tissue strength, including source-to-sink allocation processes. This could account for the observed stability or slight improvement in the symbiotic nitrogen fixation trait. In addition to impacting nodule mass, a positive effect on nodule metabolism may have been at play here, a notion supported by the observation that the increased nodule to shoot nitrogen allocation leads to improved seed yield (Carter and Tegeder, 2016).
This study did not extend into the pod-fill stage; it would be interesting to know the extent to which the SNF-related trends identified in this study correlate with later stages of soybean development. At the molecular level, it would be interesting to know if loci that regulate SNF traits during vegetative stages always control these traits during pod-fill, or whether different loci act at different growth phases. In pea, a genotype was identified wherein the majority of its nodulation occurred during the reproductive stage (Depret and Laguerre, 2008).
Furthermore, seeds were not inoculated with rhizobia strains, as the field sites have been used for growing soybean many times in the past and hence there would be a reservoir of compatible rhizobia in the soil. However, new soybean varieties might be more adapted to newer rhizobia strains that are available through commercial inoculants—in this case, this study may be underestimating improvements in SNF made by modern breeding. Soybean breeding under low N inputs and inoculation with effective rhizobia strains was the paradigm in Brazil for their success in improved biological nitrogen fixation in soybean (Alves et al., 2003), helping Brazil to become the world's highest soybean producer in 2019.
We did not find any positive correlation between the number of nodules per plant and %Ndfa. Although nodule number has been used as a major indicator of nitrogen fixation capacity (%Ndfa) in the literature, it has also shown limited proxy value in some studies (Hungria and Bohrer, 2000; Hungria et al., 2001; Thilakarathna and Raizada, 2017). However, in this study, nodule dry weight was positively correlated with the total shoot-N fixed. Among these two nodulation traits, nodule dry weight had a positive correlation with the amount of shoot-N fixed; thus, it can be used as a better proxy for effective nitrogen fixation, at least for Ontario soybean breeding. Hungria and Bohrer (2000) also found that nodule mass was a better indicator of nitrogen fixation than nodule number in terms of shoot-N accumulation. In soybean, various studies have identified loci that contribute to nodule size including GmINS1 (Hwang et al., 2014; Li et al., 2018), so it would be interesting to know if they have been under selection in breeding programs. We also found positive correlations between nodule number/dry weight, and shoot and root dry weights, similar to the recent findings (Torkamaneh et al., 2020), where 297 African soybean cultivars were screened for nitrogen fixation related traits. In the current study, shoot N fixed also had a consistent positive correlation with the total shoot N content, suggesting that nitrogen fixation is critical for building up nitrogen reserves even during the pre-pod formation stage. Recent findings by Córdova et al. (2019) suggest that biomass production is the best predictor of SNF in Midwest US soybean. Our correlation data also showed that shoot biomass production was a good predictor of total N fixed but not a good predictor of %Ndfa. At the molecular level, it would be interesting to explore the relationship between alleles that regulate hormone pathways or other pathways involved in shoot growth promotion including branching (Ongaro and Leyser, 2008; Haq et al., 2017) with those that regulate SNF traits. For example, in soybean, GmKIX8-1 has been shown to increase the size of aerial plant organs including leaves as well as seeds (Nguyen et al., 2021).
The results of this study show that considerable variation exists in Canadian soybean cultivars released over the past 100 years for nitrogen fixation prior to pod fill. The above noted drifting among modern cultivars, especially the low level of SNF in some modern cultivars (e.g., OAC Morden), and wide variation available in modern lines, suggests opportunities for deliberate selection for SNF prior to pod fill, and possibly during pod fill. The best cultivar from this study derived ~70% of its nitrogen from the atmosphere prior to pod fill, which would be considered average based on previous studies in soybean around the world (Herridge et al., 2008). Either this represents an upper limit for SNF in the generally fertile soils of Southern Ontario, where the soil itself can contribute N, or this limit can be raised, given that previous studies have shown that up to 95% of soybean nitrogen can come from the atmosphere (Herridge et al., 2008). On average, among the top-10 ranked cultivars for %Ndfa, five belonged to the modern-Guelph category (OAC Champion, OAC Frontier, OAC Huron, OAC Bayfield, OAC Dorado), and five belonged to the Guelph-Historical group (OAC 211, Hodgson, McCall, KG 20, Hark). This observation suggests that some of the historical and modern cultivars may be good sources of SNF alleles for future breeding programs, especially if similar results are obtained during pod fill. None of these best 5 historical cultivars are close parents of modern cultivars in Ontario, suggesting the best historical lines have been under-utilized. This study has also identified historic and modern cultivars with low %Ndfa (e.g., AK Harrow, OAC Eramosa), suggesting that these cultivars may possess alleles with poor absolute performance for pre-pod-fill SNF. An important caveat to these statements is that the trials in this study were conducted at one site (used for soybean breeding), whereas the breeding historically has been undertaken at multiple sites within Southern Ontario. Perhaps some of the poor performing lines for %NDFA might do better at other locations, having a sandy soil, cooler/warmer temperature, different organic matter, higher/lower soil N or specific micronutrients, and/or local/introduced rhizobia strains.
Overall, this study has revealed that pre-pod-fill nitrogen fixation capacity and associated symbiotic nitrogen fixation traits, in modern soybean cultivars (post-1983 year of release) from Ontario, Canada, are similar or modestly higher as a group compared to earlier historical lines (starting in 1913). This data supports the hypothesis that, despite a lack of direct selection, there has been indirect selection pressure to maintain and possibly improve pre-pod-fill nitrogen fixation traits in modern soybean breeding. Cultivars with higher pre-pod-fill SNF may significantly contribute to the nitrogen reserves in vegetative parts and meet the subsequent seed nitrogen demand during the pod filling stage. However, the wide variation observed for SNF among modern cultivars, in the context of the study limitations, suggests some level of genetic drift for pre-pod-fill SNF in the absence of direct selection for this trait. As some historical and modern soybean cultivars possess high nitrogen fixation capacity, they may have the potential to improve this trait in future breeding programs especially if these genotypes behave similarly at later stages.
The original contributions presented in the study are included in the article/Supplementary Material, further inquiries can be directed to the corresponding author/s.
MT, DT, and MR conceived of the manuscript. MT, MR, RB, and IR conceptualized the study. MT, RB, GC, and CG conducted all experiments. KS and BH involved in sample analysis. MT wrote the manuscript. DT and MR edited the manuscript. All authors discussed the results, commented on the manuscript, and read and approved the final manuscript.
This research was supported by the Canadian International Food Security Research Fund (CIFSRF), jointly sponsored by the International Development Research Centre (IDRC, Ottawa) and Global Affairs Canada (GAC). The study by Bruce et al. (2019a,b), which formed the basis for the germplasm used in this study was supported by National Science and Engineering Research Council (NSERC) Collaborative Research and Development (CRD) grant awarded to IR.
The authors declare that the research was conducted in the absence of any commercial or financial relationships that could be construed as a potential conflict of interest.
All claims expressed in this article are solely those of the authors and do not necessarily represent those of their affiliated organizations, or those of the publisher, the editors and the reviewers. Any product that may be evaluated in this article, or claim that may be made by its manufacturer, is not guaranteed or endorsed by the publisher.
Many thanks to Jennifer Humphries, Sidra Mohammed, Korinna Chu, Jia Chu, Sujal Chapagain, Kirsten Radcliffe, Myla Manser, Adam Hoffman, Jacob Kaszas, and Ramani Ketheeswaranathan for their help with sample and data collection.
The Supplementary Material for this article can be found online at: https://www.frontiersin.org/articles/10.3389/fagro.2021.725813/full#supplementary-material
Supplementary Figure 1. Pedigree relationships of the 44 historical and modern-Guelph accessions tracing back to historical founder accessions, plotted by year of release (cultivars). Blue shows historical cultivars, while red shows modern-Guelph cultivars. Data from: (Bruce et al., 2019b).
Supplementary Figure 2. Trait by year of cultivar release regression plots for pre-pod-fill agronomic traits. (A,B) Root dry weight per plant. (C,D) Shoot dry weight per plant. (E,F) Shoot N%. (G,H) Shoot C/N ratio. (I,J) Total shoot nitrogen content. Each blue and purple dot represents a historical and modern-Guelph variety, respectively. NS, not significantly different at p < 0.05.
Supplementary Table 1. Monthly daily maximum, minimum, daily mean temperatures, and total precipitation at Woodstock, Ontario during the 2016 and 2017 growing seasons.
Supplementary Table 2. Analysis of variance (ANOVA) table for the plant parameters tested across 44 soybean cultivars in two production years (2016 and 2017).
Alves, B. J. R., Boddey, R. M., and Urquiaga, S. (2003). The success of BNF in soybean in Brazil. Plant Soil 252, 1–9. doi: 10.1023/A:1024191913296
Attia, A., Shapiro, C., Kranz, W., Mamo, M., and Mainz, M. (2015). Improved yield and nitrogen use efficiency of corn following soybean in irrigated sandy loams. Soil Sci. Soc. Am. J. 79, 1693–1703. doi: 10.2136/sssaj2015.05.0200
Bruce, R. W., Grainger, C. M., Ficht, A., Eskandari, M., and Rajcan, I. (2019a). Trends in soybean trait improvement over generations of selective breeding. Crop Sci. 59, 1870–1879. doi: 10.2135/cropsci2018.11.0664
Bruce, R. W., Torkamaneh, D., Grainger, C., Belzile, F., Eskandari, M., and Rajcan, I. (2019b). Genome-wide genetic diversity is maintained through decades of soybean breeding in Canada. Theor. Appl. Genet. 132, 3089–3100. doi: 10.1007/s00122-019-03408-y
Carter, A. M., and Tegeder, M. (2016). Increasing nitrogen fixation and seed development in soybean requires complex adjustments of nodule nitrogen metabolism and partitioning processes. Curr. Biol. 26, 2044–2051. doi: 10.1016/j.cub.2016.06.003
Ciampitti, I. A., and Salvagiotti, F. (2018). New insights into soybean biological nitrogen fixation. Agron. J. 110, 1185–1196. doi: 10.2134/agronj2017.06.0348
Collino, D. J., Salvagiotti, F., Perticari, A., Piccinetti, C., Ovando, G., Urquiaga, S., et al. (2015). Biological nitrogen fixation in soybean in Argentina: relationships with crop, soil, and meteorological factors. Plant Soil 392, 239–252. doi: 10.1007/s11104-015-2459-8
Córdova, S. C., Castellano, M. J., Dietzel, R., Licht, M. A., Togliatti, K., Martinez-Feria, R., et al. (2019). Soybean nitrogen fixation dynamics in Iowa, USA. Field Crops Res. 236, 165–176. doi: 10.1016/j.fcr.2019.03.018
Depret, G., and Laguerre, G. (2008). Plant phenology and genetic variability in root and nodule development strongly influence genetic structuring of Rhizobium leguminosarum biovar viciae populations nodulating pea. New Phytol. 179, 224–235. doi: 10.1111/j.1469-8137.2008.02430.x
Gan, Y., Stulen, I., Van Keulen, H., and Kuiper, P. J. C. (2003). Effect of N fertilizer top-dressing at various reproductive stages on growth, N2 fixation and yield of three soybean (Glycine max (L.) Merr.) genotypes. Field Crops Res. 80, 147–155. doi: 10.1016/S0378-4290(02)00171-5
Giller, K. E. (2001). Nitrogen Fixation in Tropical Cropping Systems. Wallingford: CAB International. doi: 10.1079/9780851994178.0000
Guo, J., Wang, Y., Song, C., Zhou, J., Qiu, L., Huang, H., et al. (2010). A single origin and moderate bottleneck during domestication of soybean (Glycine max): Implications from microsatellites and nucleotide sequences. Ann. Bot. 106, 505–514. doi: 10.1093/aob/mcq125
Haq, B. U., Ahmad, M. Z., Rehman, N., Wang, J., Li, P., Li, D., et al. (2017). Functional characterization of soybean strigolactone biosynthesis and signaling genes in Arabidopsis MAX mutants and GmMAX3 in soybean nodulation. BMC Plant Biol. 17, 1–20. doi: 10.1186/s12870-017-1182-4
Hartman, G. L., West, E. D., and Herman, T. K. (2011). Crops that feed the World 2. Soybean-worldwide production, use, and constraints caused by pathogens and pests. Food Secur. 3, 5–17. doi: 10.1007/s12571-010-0108-x
Herridge, D. F., Peoples, M. B., and Boddey, R. M. (2008). Global inputs of biological nitrogen fixation in agricultural systems. Plant Soil 311, 1–18. doi: 10.1007/s11104-008-9668-3
Hungria, M., and Bohrer, T. R. J. (2000). Variability of nodulation and dinitrogen fixation capacity among soybean cultivars. Biol. Fertil. Soils 31, 45–52. doi: 10.1007/s003740050622
Hungria, M., Campo, R. J., Chueire, L. M. O., Grange, L., and Megías, M. (2001). Symbiotic effectiveness of fast-growing rhizobial strains isolated from soybean nodules in Brazil. Biol. Fertil. Soils 33, 387–394. doi: 10.1007/s003740100338
Hungria, M., and Vargas, M. A. T. (2000). Environmental factors affecting N2 fixation in grain legumes in the tropics, with an emphasis on Brazil. Field Crops Res. 65, 151–164. doi: 10.1016/S0378-4290(99)00084-2
Hwang, S., Ray, J. D., Cregan, P. B., King, C. A., Davies, M. K., and Purcell, L. C. (2014). Genetics and mapping of quantitative traits for nodule number, weight, and size in soybean (Glycine max L. [Merr.]). Euphytica 195, 419–434. doi: 10.1007/s10681-013-1005-0
Hymowitz, T., and Harlan, J. R. (1983). Introduction of soybean to North America by Samuel Bowen in 1765. Econ. Bot. 37, 371–379. doi: 10.1007/BF02904196
Jin, J., Liu, X., Wang, G., Mi, L., Shen, Z., Chen, X., et al. (2010). Agronomic and physiological contributions to the yield improvement of soybean cultivars released from 1950 to 2006 in Northeast China. Field Crops Res. 115, 116–123. doi: 10.1016/j.fcr.2009.10.016
Karlen, D. L., Hurley, E. G., Andrews, S. S., Cambardella, C. A., Meek, D. W., Duffy, M. D., et al. (2006). Crop rotation effects on soil quality at three northern corn/soybean belt locations. Agron. J. 98, 484–495. doi: 10.2134/agronj2005.0098
Li, X., Zheng, J., Yang, Y., and Liao, H. (2018). INCREASING NODULE SIZE1 expression is required for normal rhizobial symbiosis and nodule development. Plant Physiol. 178, 1233–1248. doi: 10.1104/pp.18.01018
Liu, S., Zhang, M., Feng, F., and Tian, Z. (2020). Toward a “green revolution” for soybean. Mol. Plant 13, 688–697. doi: 10.1016/j.molp.2020.03.002
Locke, A. M., and Ramirez, M. E. (2020). Increased nitrogen fixation and remobilization may increase seed protein without a yield penalty in a soybean introgression line. J. Crop Improv. 35, 486–507. doi: 10.1101/602748
Masclaux-Daubresse, C., Daniel-Vedele, F., Dechorgnat, J., Chardon, F., Gaufichon, L., and Suzuki, A. (2010). Nitrogen uptake, assimilation and remobilization in plants: challenges for sustainable and productive agriculture. Ann. Bot. 105, 1141–1157. doi: 10.1093/aob/mcq028
Morrison, M. J., Voldeng, H. D., and Cober, E. R. (2000). Agronomic changes from 58 years of genetic improvement of short-season soybean cultivars in Canada. Agron. J. 92, 780–784. doi: 10.2134/agronj2000.924780x
Nguyen, C. X., Paddock, K. J., Zhang, Z., and Stacey, M. G. (2021). GmKIX8-1 regulates organ size in soybean and is the causative gene for the major seed weight QTL qSw17-1. New Phytol. 229, 920–934. doi: 10.1111/nph.16928
Ongaro, V., and Leyser, O. (2008). Hormonal control of shoot branching. J. Exp. Bot. 59, 67–74. doi: 10.1093/jxb/erm134
Ortez, O. A., Tamagno, S., Salvagiotti, F., Prasad, P. V. V., and Ciampitti, I. A. (2019). Soybean nitrogen sources and demand during the seed-filling period. Agron. J. 111, 1779–1787. doi: 10.2134/agronj2018.10.0656
Pitumpe Arachchige, P. S., Rosso, L. H. M., Hansel, F. D., Ramundo, B., Torres, A. R., Asebedo, R., et al. (2020). Temporal biological nitrogen fixation pattern in soybean inoculated with Bradyrhizobium. Agrosystems Geosci. Environ. 3, 2–11. doi: 10.1002/agg2.20079
Salvagiotti, F., Cassman, K. G., Specht, J. E., Walters, D. T., Weiss, A., and Dobermann, A. (2008). Nitrogen uptake, fixation and response to fertilizer N in soybeans: a review. Field Crops Res. 108, 1–13. doi: 10.1016/j.fcr.2008.03.001
Santachiara, G., Salvagiotti, F., and Rotundo, J. L. (2019). Nutritional and environmental effects on biological nitrogen fixation in soybean: a meta-analysis. Field Crops Res. 240, 106–115. doi: 10.1016/j.fcr.2019.05.006
Shurtleff, W., and Aoyagi, A. (2010). History of Soybeans and Soyfoods in Canada (1831-2010): Extensively Annotated Bibliography and Sourcebook. Lafayette, CA: Soyinfo Center.
Soy Canada (2021). Canadian Soybean Industry. Available online at: https://soycanada.ca/statistics/at-a-glance/ (accessed July 25, 2021).
Thilakarathna, M. S., Moroz, N., and Raizada, M. N. (2017). A biosensor-based leaf punch assay for glutamine correlates to symbiotic nitrogen fixation measurements in legumes to permit rapid screening of rhizobia inoculants under controlled conditions. Front. Plant Sci. 8:1714. doi: 10.3389/fpls.2017.01714
Thilakarathna, M. S., and Raizada, M. N. (2017). A meta-analysis of the effectiveness of diverse rhizobia inoculants on soybean traits under field conditions. Soil Biol. Biochem. 105, 177–196. doi: 10.1016/j.soilbio.2016.11.022
Thilakarathna, M. S., and Raizada, M. N. (2018). Challenges in using precision agriculture to optimize symbiotic nitrogen fixation in legumes: progress, limitations, and future improvements needed in diagnostic testing. Agronomy 8:78. doi: 10.3390/agronomy8050078
Torkamaneh, D., Chalifour, F. P., Beauchamp, C. J., Agrama, H., Boahen, S., Maaroufi, H., et al. (2020). Genome-wide association analyses reveal the genetic basis of biomass accumulation under symbiotic nitrogen fixation in African soybean. Theor. Appl. Genet. 133, 665–676. doi: 10.1007/s00122-019-03499-7
Wang, J., Chu, S., Zhang, H., Zhu, Y., Cheng, H., and Yu, D. (2016). Development and application of a novel genome-wide SNP array reveals domestication history in soybean. Sci. Rep. 6:20728. doi: 10.1038/srep20728
Wilker, J., Navabi, A., Rajcan, I., Marsolais, F., Hill, B., Torkamaneh, D., et al. (2019). Agronomic performance and nitrogen fixation of heirloom and conventional dry bean varieties under low-nitrogen field conditions. Front. Plant Sci. 10:952. doi: 10.3389/fpls.2019.00952
Yinbo, G., Peoples, M. B., and Rerkasem, B. (1997). The effect of N fertilizer strategy on N2 fixation, growth and yield of vegetable soybean. Field Crops Res. 51, 221–229. doi: 10.1016/S0378-4290(96)03464-8
Zapata, F., Danso, S. K. A., Hardarson, G., and Fried, M. (1987). Time course of nitrogen fixation in field-grown soybean using nitrogen-15 Methodology. Agron. J. 79, 172–176. doi: 10.2134/agronj1987.00021962007900010035x
Zhao, X., Zheng, S. H., Suzuki, A., and Arima, S. (2014). Varietal difference in nitrogen redistribution from leaves and its contribution to seed yield in soybean. Plant Prod. Sci. 17, 103–108. doi: 10.1626/pps.17.103
Keywords: legumes, soybean, symbiotic nitrogen fixation, cultivars, breeding, nitrogen
Citation: Thilakarathna MS, Torkamaneh D, Bruce RW, Rajcan I, Chu G, Grainger CM, Szczyglowski K, Hill B and Raizada MN (2021) Testing Whether Pre-Pod-Fill Symbiotic Nitrogen Fixation in Soybean Is Subject to Drift or Selection Over 100 Years of Soybean Breeding. Front. Agron. 3:725813. doi: 10.3389/fagro.2021.725813
Received: 15 June 2021; Accepted: 16 August 2021;
Published: 09 September 2021.
Edited by:
Mohsin Tanveer, University of Tasmania, AustraliaReviewed by:
Anket Sharma, University of Maryland, College Park, United StatesCopyright © 2021 Thilakarathna, Torkamaneh, Bruce, Rajcan, Chu, Grainger, Szczyglowski, Hill and Raizada. This is an open-access article distributed under the terms of the Creative Commons Attribution License (CC BY). The use, distribution or reproduction in other forums is permitted, provided the original author(s) and the copyright owner(s) are credited and that the original publication in this journal is cited, in accordance with accepted academic practice. No use, distribution or reproduction is permitted which does not comply with these terms.
*Correspondence: Malinda S. Thilakarathna, dGhpbGFrYXJAdWFsYmVydGEuY2E=
Disclaimer: All claims expressed in this article are solely those of the authors and do not necessarily represent those of their affiliated organizations, or those of the publisher, the editors and the reviewers. Any product that may be evaluated in this article or claim that may be made by its manufacturer is not guaranteed or endorsed by the publisher.
Research integrity at Frontiers
Learn more about the work of our research integrity team to safeguard the quality of each article we publish.