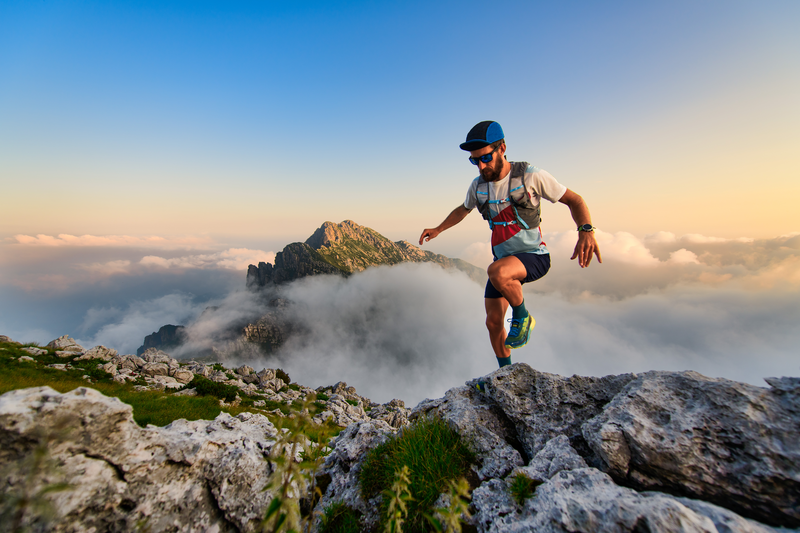
94% of researchers rate our articles as excellent or good
Learn more about the work of our research integrity team to safeguard the quality of each article we publish.
Find out more
ORIGINAL RESEARCH article
Front. Agron. , 23 September 2021
Sec. Agroecological Cropping Systems
Volume 3 - 2021 | https://doi.org/10.3389/fagro.2021.698968
This article is part of the Research Topic Crop Diversification: A Key Pillar for the Agroecological Transition View all 7 articles
Diversification of cropping and farming systems is a central agroecological principle, which may improve resource use efficiency, reduce pests and diseases, diversify income sources, and enhance the resilience of the production. The main objective of this study was to identify challenges related to the sustainability of organic cropping systems that were diversified according to one or several of the following practices: diverse crop rotation, integration of cover crops, and intercropping. The sustainability assessments were made using a multi-criteria decision aid method (MCDA) and a framework based on the FAO Sustainability Assessment of Food and Agricultural Systems (SAFA) guidelines. Social, economic and environmental aspects were integrated in the sustainability assessments and combined with semi-structured interviews to identify and discuss farmer's perceptions of barriers to crop diversification and sustainability transition. The results showed that diversified organic cropping systems could achieve high overall sustainability, especially in the environmental dimension thanks to non-inputs of pesticides or mineral fertilizers and efficient use of resources. On the other hand, social and economic dimensions were more variable, with challenges of lower sustainability in profitability and management complexity for several of the diversified cropping systems. Limited access to knowledge, technology and markets for minor crops, and concerns about the consistency of policies were highlighted by farmers as barriers for crop diversification. We discuss how the identified challenges can be overcome and argue that fostering collaboration among stakeholders may increase investment capacity and improve access to new or alternative markets, thereby stimulating transitions toward more diversified and sustainable cropping systems.
Agricultural sustainability is still in need of significant developments to balance food production with environmental goals aligned with an economically viable and socially fair food system (Foley et al., 2011; Rockstrom et al., 2017; Willett et al., 2019). Scientists have called for more ambitious and holistic goals for future agricultural systems, focusing on challenges such as climate change, losses of agricultural land, negative impact on biodiversity and nutrient losses from agriculture that cause eutrophication (Garnett et al., 2013; Hunter et al., 2017). Research and policy play a key role in supporting sustainable food production while ensuring environmental improvements (Lang and Barling, 2012). The launch of the recent strategy “Farm to fork” within the frame of the EU green deal aims for enhancing the European food systems provision of fair and healthy food, with a neutral or positive environmental impact. Furthermore, this strategy highlights the need for supporting more sustainable practices in agriculture (European Commission, 2020).
Diversification of cropping systems is central for reaching the goals of ensuring the availability of resources (e.g., nutrients, water, land) for future generations, increasing the reliance on ecosystem services that replace external inputs, and promoting diverse diets, healthy agroecosystems, and securing livelihoods (IPES-Food, 2016). The addition of functional biodiversity to cropping systems across multiple spatial and temporal scales, through diversified crop rotations, integration of cover crops, green manures, and species mixtures (inter- and multi-cropping), can enhance resource use efficiency, promote the provision of ecosystem services and reduce negative environmental impacts (Kremen and Miles, 2012) without compromising crop yields in the production of food, feed, and raw materials (Tamburini et al., 2020).
These three groups of crop diversification practices are defined as follows: (i) diversified crop rotations means combining different type of crops in the temporal sequence grown on the same field (Koschke et al., 2013); (ii) cover crops are commonly growing in periods between main crops, not harvested for either food or feed but used e.g., to improve soil quality or reduce nutrient losses (Schipanski et al., 2014; Tribouillois et al., 2016). Green manures are non-harvested cover crops or main crops, that are incorporated in the soil after their growth period to improve nutrition of the subsequent crops (Thorup-Kristensen et al., 2003); (iii) species mixtures are used to increase the within-field crop diversity, i.e., intercropping being defined as the simultaneous growth of two or more species in the same field during a specific period of time (Willey, 1979). In the context of this study, intercropping refers to the situation where two or more crops are grown and harvested together, as a mixed crop.
During the last 20 years, several different tools have been developed for assessing the sustainability of agricultural systems (Hayashi, 2000; Riley, 2001; Rao and Rogers, 2006; Lampridi et al., 2019; Chopin et al., 2021), including a large variety of indicators adapted to relevant scales to fit site-specific environmental and socio-economic conditions (Payraudeau and van der Werf, 2005; Bockstaller et al., 2008, 2009). Sustainability assessment tools have been used, e.g., for identifying challenges and obstacles in the development of more sustainable agricultural practices, management, and policy (Lampridi et al., 2019) and for comparing different production systems (such as conventional and organic) (Pacini et al., 2003; de Olde et al., 2016). Research using such tools has focused on management practices, e.g., integrated pest management (Pelzer et al., 2012) and conservation agriculture (Trivino-Tarradas et al., 2019), increasing crop diversity in arable cropping systems by introducing grain legumes (Pelzer et al., 2017) or service crops in vegetable cropping systems (Diacono et al., 2019), and most recently a framework for assessing crop diversification practices (Iocola et al., 2020). While there is a recent study on the sustainability of cropping systems that combine several crop diversification practices in conventional production systems (Viguier et al., 2021), there seems to be a lack of studies that assess the sustainability of organic cropping systems when including several crop diversification practices.
The recent development of a large and diverse body of literature about sustainability assessment tools and methods has highlighted the context-specificity of such assessments, especially economic and social sustainability (Kremen et al., 2012). In this respect, little has been done in assessing the sustainability in farming systems in Swedish specific context (Röös et al., 2019). Therefore, there is a need to evaluate the performance of diversified cropping systems in a local context, recognizing synergies and trade-offs between different elements that compose the systems. Thus, it enables to expand the understanding of how the integration of several crop diversification practices can contribute to more sustainable agricultural systems.
The overall objective of this study was to assess challenges related to the implementation of diversified cropping systems in certified organic production in the region of Scania (southern Sweden). We used a multi-criteria decision aid method (MCDA) (Pelzer et al., 2012) and a sustainability assessment framework (SAF) based on the FAO (2013) guidelines for Sustainability Assessment of Food and Agriculture Systems (SAFA) (Iocola et al., 2020) to perform cropping system sustainability assessments. These assessments were combined with semi-structured interviews to identify strengths and challenges in examples of organic cropping systems that represent different crop diversification practices. Social, economic, and environmental aspects are integrated into the assessments, and complemented with farmers' perceptions of barriers (social, technical, and administrative) to sustainability transition. The present study is guided by the following research questions:
i) How can crop diversification affect the different dimensions of sustainability of the cropping systems?
ii) What are the strengths and challenges of organic cropping systems where different crop diversification practices are implemented?
iii) How do farmers' perceptions of barriers to crop diversification relate to the outcomes of the sustainability assessment tools?
The cropping systems compared in this study are located in Scania County in southern Sweden, which is characterized by agricultural activities, covering around 440,000 ha of arable land (39% of the total Swedish arable land area). The area currently used for organic production in Scania is 37 004 ha (8.5% of the total arable land area in Scania and 6.7% of total organic production area in Sweden) (Statistics Sweden, 2020a). In 2016, there were a total of 8,196 agricultural enterprises in the Scania region (where only 410 enterprises were under organic production), of which 39% within crop production, 25% in animal production, and 7% in mixed production (both crop and animal). The remaining percentage corresponds to small-scale farming and other types of agricultural production. Cereal production covered almost half of the arable area with 211,000 ha, of which only 8,100 are under organic production (Statistics Sweden, 2020b). Vegetable production grown on open land corresponds to an area of 6,000 ha, and only 15% is under organic production (Statistics Sweden, 2020c). In Scania, cereal-based farming is concentrated on the open plains in the southwest, where soils generally have relatively high clay content (Piikki and Söderström, 2019) and high soil fertility (Soinne et al., 2020). Mixed farming and livestock production are distributed along with the northern and eastern parts, where less fertile moraine soils dominate and where fields are less accessible due to high levels of hinders (landscape features) and other impediment occurrences resulting in smaller sized fields. Thus, the context of this study includes farming systems characterized by stockless crop production, livestock production, or vegetable production. The present study assessed the sustainability of 11 organic cropping systems, including the reference and diversified systems from a field experiment and five commercial farms.
Two of the studied cropping systems were based on a field experiment, which comprised one reference cropping system and one system with additional crop diversification practices. The field experiment was established in spring 2018 at the SITES (Swedish Infrastructure for Ecosystem Science) Lönnstorp field research station, on land managed according to the certification for organic production, located at Alnarp, Swedish University of Agricultural Sciences. The experiment was initiated within the European project DiverIMPACTS (Diversification through Rotation, Intercropping, Multiple Cropping, Promoted with Actors and value-Chains toward Sustainability). Both the reference and diversified cropping systems from the field experiments were designed by researchers, using inputs from farmers and agricultural advisors to integrate practical relevance and stakeholders' interests along with research questions into the experimental design. The reference system is a 6-year crop rotation with crops that are common in stockless arable organic cropping systems in the Scania region (Figure 1 and Table 1). This reference system is characterized by crops with high nutrient demand and, due to the absence of on-farm animal production, dependency on external inputs of manure or other organic fertilizers. The diversified cropping system from the field experiment was based on the same crops as in the reference and also included intercrops (mixtures of two main crops) and species mixtures as cover crops between main crops. All crops in both systems are represented each year in the experiment, which contains four replicates in a randomized block design with plots measuring 6 m × 15 m.
Figure 1. Illustration of the 11 cropping systems included in the sustainability analysis. (W) refers to winter crops and (S) refers to spring crops. OSR, oilseed rape; RC, red clover; FB, faba bean; BL, blue lupin; M-barley, malting barley; S-Beet, sugarbeet; BO, black oats; POT, potatoes; GM, green manure; B-root, beetroots; LETT, lettuce. CC corresponds to cover crops.
Table 1. Description of the 11 cropping systems included in the analysis, based on field experiments and rotations on five commercial farms.
Cropping systems (nine in total) currently implemented on five commercial organic farms were also assessed compared to the reference in the field experiment. These included a variety of different crop production such as specialized arable cropping systems (no livestock), animal farming systems (all crops used as on-farm livestock feed), mixed farming systems (crops for selling and for on-farm livestock feed), and vegetable production. These farming systems included variable levels of crop diversity in terms of the number of different crops in rotation, such as grain and forage legumes and green manures, crop mixtures (intercrops), and cover crops (including species mixtures). Further, theses systems were grouped in the following crop diversification practices: diversified crop rotation and cover crops (DR+CC), diversified crop rotation including cover crops and intercrops (DR+CC+IC), and diversified crop rotations with cover crops and green manures (DR+CC+GM). The tillage management varied between systems; some farms use plowing to control weeds, while others have implemented reduced tillage practices. In the case of the stockless farming systems, they rely on off-farm manure coming from neighboring farms. Further details about the crop sequences, soil tillage practices and fertilization in the experimental and on-farm cropping systems included in the study are given in Figure 1 and Table 1.
The impact of crop diversification practices on cropping systems was assessed using the multi-criteria decision aid (MCDA) tool DEXi-PM and a sustainability assessment framework (SAF). DEXI-PM was developed from the DEXi software (Bohanec, 2008) for ex-ante assessments of innovative cropping systems with limited use of pesticides (Pelzer et al., 2012). The DEXi-PM decision tree evaluates cropping systems' sustainability using social, economic and environmental dimensions expressed in qualitative terms. The SAF was developed by Iocola et al. (2020) in a multi-actor network in the DiverIMPACTS project, where the indicators were co-designed by researchers and stakeholders involved. The guidelines of the Sustainability Assessment of Food and Agriculture (SAFA) (FAO, 2013) was used as a baseline for the SAF to define indicators that could highlight synergies and trade-offs of crop diversification practices in cropping systems. The DEXi-PM tool was initially developed to assess cropping systems for integrated pest management. However, some indicators are included to address off-site aspects of the cropping systems such as farm, landscape and regional context (Pelzer et al., 2012). The SAF complements this tool by using more quantitative indicators, especially on environmental aspects, and by focusing on crop diversification practices. However, the SAF includes only two social indicators, and thereby DEXi-PM may complement the framework due to a higher number of indicators included for this dimension. The Dexi-PM tree is based on six hierarchical levels, where aggregated indicators are further divided into more specific indicators until reaching the basic indicators: the entry points of input data for the sustainability assessment. In total, DEXi-PM includes 75 basic indicators and 86 aggregated indicators that describe the cropping systems and regional context. In the SAF, we used 29 indicators out of the 32 developed, excluding the land equivalent ratio (LER) and yield coefficient of variation (YCV) indicators due to lack of data to calculate these. In addition, the supplier/customer contribution to profitability indicator (SCCP) was calculated only for suppliers since farmers in the studied context sell the products mainly to suppliers (crop products from the studied farms are not or very rarely sold directly to consumers) (Supplementary Table 1).
The MCDA tool used, DEXi-PM, is primarily intended for ex ante assessments, but it is a flexible tool that can also be used to assess the sustainability of existing cropping systems (Pelzer et al., 2012). In our study, the ex ante approach was useful both for the experimental and on-farm cropping systems. Even though the field experiment has generated quantitative data on e.g., inputs and crop yields, the short time period since initiating the experiment (2018) and the set-up in experimental plots mean that the experimental systems are suitable as prototypes representing a reference and an innovative diversified cropping system. For the on-farm cropping systems, farmers provided information that represents their typical crop rotations, management and results for an average year, since the specific crop choices, crop sequences, input levels (e.g., fertilizers and weed control measures), yields, costs and incomes vary between years. Therefore, the input data for the on-farm assessments were also suitable for an ex ante approach to assess the sustainability of examples of commercial cropping systems.
The MCDA (DEXi-PM) indicators used for assessing the criteria (referring to the aggregated indicators at hierarchical level 3, i.e., profitability and economic viability), were complemented with indicators from the SAF regarding productivity, profitability, dependency on external inputs, product quality and local valorization (Table 2).
Table 2. List of Indicators from the MCDA tool and SAF used to assess the sustainability of field experiments and commercial farms.
We assessed social sustainability by three criteria in the MCDA tool: value chain, social sustainability for the farmer and the interaction of the system with society. The SAF includes only two indicators in the social dimension, treatment frequency index (TFI, regarding pesticides) and work overload (WOL) (Table 2).
Environmental sustainability was assessed based on three criteria in the MCDA tool: resource use, environmental quality, and biodiversity. The SAF included 21 environmental indicators, which covered criteria on soil and water, greenhouse gas emission, non-renewable resources, and biodiversity (Table 2).
Farms with highly diversified cropping systems were selected for the sustainability assessments and interviews (see below) based on the overall criteria that they should be located in Scania, have organic production of crops for selling (on at least part of the farm), and implement one or several crop diversification practices. We used purposive (i.e., sample participants in a strategic way so it is relevant to the research question that is being pose) and convenience (i.e., selection of participants that are more readily accessible) sampling to identify farming systems within organic production with a high crop diversity level (Bryman, 2012). Thus, irrespective of size and production focus, farming systems were selected based on farmers' active engagement in implementing new main and cover crops in their rotations and management practices to enhance sustainability. Based on a list of known farmers fulfilling the selection criteria, five were available and interviewed to collect data on nine crop rotations (one or two rotations per farm; see Figure 1 and Table 1). Three of the farmers had also participated in a workshop where they gave inputs to the design of the field experiment used in this study (see section Reference and Diversified Cropping Systems in a Field Experiment). For the MCDA analysis, one assessment per farm was made, using input data representing either one crop rotation or the average on farms with more than one rotation, while the SAF analyses were made for each crop rotation. One individual interview was performed with each farmer, both to collect specific data for the MCDA and SAF assessments and to gather qualitative data about farmers' opinions and perceptions of crop diversification (see below).
The stepwise integration of indicators into higher hierarchical levels of the MCDA tool is based on “if-then” decision rules and weighting, which will define the value of the aggregated indicator at the next level up in the hierarchy (Pelzer et al., 2012; Hawes et al., 2019). The first step in the sustainability assessment process was to evaluate the reference system, followed by the assessments of the diversified field experiment treatments and cropping systems on the commercial farms. The MCDA tool is developed for farm-level assessments. For the cropping systems in the field experiment (DRREF and DRFE), knowledge and experience of researchers and technicians involved in the field experimental design and management were therefore used to identify appropriate entry data for some of the indicators in the social and economic dimensions. We presented the results using the 23 aggregated indicators at the hierarchical level four (L4) in the MCDA tree and weights in the aggregation steps were left as the default setting proposed by the developers of DEXi-PM (Supplementary Table 2). The results from each cropping system are displayed in a heat map, i.e., including the reference and the alternative system applied in the field experiment and each commercial farm. Five levels express the overall level for the economic, social and environmental dimensions and each of the 23 indicators in L4 range from very low to very high sustainability.
Similar to the assessment with the MCDA tool, the SAF assessment used information regarding inputs and crop rotation management provided by the team working in the field experiment (DRREF and DRFE) and by the farmers. The specific calculations used for each indicator can be found in Iocola et al. (2020). The impacts related to crop diversification for both DRFE and farmers crop rotations were compared to the DRREF system, for which each indicator was set to zero. Thus, the results show the relative value as difference compared to DRREF in each indicator for each of the diversified cropping system/crop rotation. It is important to highlight that the indicators relevant to pesticides and mineral fertilizer use (TFI, LeachAI, QAI, VolAI, MNUGHG, MNUNRJ, and MPU) were set to zero since all the cropping systems assessed are organic production systems. Furthermore, the number of crops in the rotation with cultivar mixture (CCM) was set to zero for all the crop rotations since not all farmers provided detailed information to calculate this indicator. Furthermore, bare soil during erosion risk (BSOeros) was also set to zero for all the cropping systems since the farmers and manager of the field experiments expressed a lack of risk for run-off or potential erosion problems (Supplementary Table 1). Input data for calculating the indicators were derived from the farmer interviews and researchers and technicians regarding the field experiment and complemented with information from literature and national statistics (e.g., crop prices, markets, and value chains). Information related to organic fertilizers and amendments were obtained from the Swedish Board of Agriculture (2014). In case information on crop prices was not obtained in the interviews, this was supplemented with data from Statistics Sweden database (Statistics Sweden, 2020c). The total values for each indicator and cropping systems in the SAF are presented in Supplementary Table 3.
Semi-structured interviews were chosen as a data collection method, given their utility to gather in-depth information necessary for performing the MCDA and SAF assessments. Semi-structured interviews also provide flexibility to capture quantitative and qualitative data, including farmers' views, thus assisting in applying mixed methods (Bryman, 2012). The reason to conduct qualitative data collection during the interviews was to gain a deeper understanding of how the participating farmers perceive their situation in relation to crop diversification and the sustainability of cropping systems (Bryant and Charmaz, 2007). We intended to collect farmers' statements regarding barriers to and drivers for crop diversification and sustainability transition.
Semi-structured interviews allow for flexibility and the emergence of new aspects and questions and provide an in-depth examination of farmers' experiences. Assessing the meaning of the interviews helped us to interpret the data into categories before analysis. We interviewed four farmers and a farm manager; all were men between 35 and 65 years old and lived in Scania. All were fully dependent on farming as owners or employed managers. The five farming enterprises were independent of each other. However, the farmers and manager knew each other (or knew about each other), e.g., from having participated in farmer group activities (field visits, workshops) organized by themselves or other farmers, advisors, or researchers. The interviews took place at the farmers' home, and office (in the case of the farm manager), and each interview took about 3 h. The interviews were transcribed and subject to thematic analysis using a deductive-inductive coding scheme (Mayring, 2000). This coding processes were done first to identify farmers' opinions and perceptions on crop diversification practices and then identify challenges, barriers, and drivers for crop diversification. The results were grouped into five themes: knowledge, technology, market and value chains, policy and regulations, and climate.
The cropping systems at the commercial farms and the diversified field experiment performed from high to very high in terms of the overall sustainability related to the high performance of the DRREF system according to the MCDA method (Figure 2). Similar results were obtained using the SAF, where the majority of the indicators showed positive values compared to DRREF for most of the cropping systems (Figure 3). The high performance in terms of environmental sustainability in all systems (including the DRREF) was attributed to the non-use of synthetic fertilizers and pesticides, a requirement in organically certified crop production (Figure 2). However, the tools were not able to account for organic and natural products. For both sustainability assessment methods, the social dimension was characterized by a high score for all the systems, including the DRREF system (in the case of the MCDA). In terms of economic sustainability, the diversified cropping systems performed from medium to high according to the MCDA method, with the diversified field experiment (DRFE) being the only exception with low economic sustainability (Figure 2). In the SAF results, economic sustainability showed more positive than negative values for the diversified cropping systems compared to the reference (Figure 3).
Figure 2. Heat map illustrating the sustainability assessment results from the MCDA method, including the overall and three dimensions of sustainability and 23 aggregated indicators (L4) of the seven cropping systems.
Figure 3. Schematic diagrams of the sustainability framework assessment for 10 diversified crop rotations in the (A) group including cover crops (DR+CC), (B) group including cover crops and intercrops (DR+CC+IC), (C) diversified field experiment (DRFE), and (D) group including cover crops and green manures (DR+CC+GM). The different colors reflect the three dimensions of sustainability (ECON, economic; SOC, social; ENV, Environmental). An asterisk in the PLWR indicator designates a lack of data to calculate the indicator, so it was set to zero. In the overall sustainability table, the total number of positive (+), neutral (N) and negative (–) indicators are presented for the overall sustainability of the SAF assessment. †indicates that all the indicators were counted (including the ones that were set to zero, see Supplementary Table 1). EY, Energetic yield; RGM, Average gross margin at rotation level; DEI, Total input/turnover; PSQ, Product standard quality required by the sector/market; PSC, Proportion of short food supply chain and local distribution; SCCP, Supplier contribution to profitability; WOL, Work overload; CDI, Crop diversity Index; %SNH, % Semi natural habitat; LEG, % Legumes in rotation; CCD, Crop-cultivar diversity; NWHC, Proportion of crops harvested in wet conditions; ACI, C input during the rotation; PLWR, Pressure on local water resources; BSOleach, Bare soil during drainage periods; NBAL, Surface N balance; PBAL, Surface P balance; NU, Nitrogen use; FCFGHG, Total fuel consumption at farm level for global warming potential calculation; FCFNRJ, Total fuel consumption at farm level for fossil energy use calculation.
The two cropping systems that combined diverse rotation and cover crops (DR+CC) showed lower sustainability in terms of production risk (MCDA) than the other commercial cropping systems, including intercropping or green manure (Figure 2). Regardless of diversification practice, all cropping systems had low sustainability in the indicator potential profitability (MCDA; Figure 2). There was no clear distinction between crop diversification practices among the SAF economic indicators (Figure 3). However, the energy yield indicator was often higher in the diversified crop rotations that included both cover crops and intercrops (DR+CC+IC) or green manure (DR+CC+GM), thus suggesting an increase in productivity by including these crop diversification practices. Lastly, it is essential to highlight that the total input/turnover indicator was the only indicator, which was positive for all commercial cropping systems, indicating a lower dependency on external inputs than in the DRREF.
Operational difficulties was the MCDA indicator that most clearly distinguished between crop diversification practices: the two cropping systems with diversified rotations and cover crops (DR+CC) showed high sustainability while all other cropping systems showed low or very low sustainability (Figure 2). The cropping systems with relatively low complexity, DRREF and DR1+CC, showed very high sustainability for the MCDA indicators access to inputs and access to output market, while these indicators were often at medium sustainability for the cropping systems that combined more than two crop diversification practices or included less common crops such as legumes or vegetables (DR+CC+IC; DR+CC+GM) (Figure 2). There was no clear pattern among crop diversification practices for the remaining social sustainability indicators of the MCDA (Figure 2), nor for work overload, which was the only SAF social sustainability indicator that varied between cropping systems (Figure 3).
Land use was the only MCDA indicator for which none of the cropping systems reached very high sustainability, with the field experiments (DRREF and DRFE) standing out with very low sustainability due to the lack of uncropped land (Figure 2). For water use, the cropping systems where irrigation was used showed medium to low sustainability, and the other cropping systems had very high sustainability. The field experiments and DR1+CC showed low sustainability in soil quality, for which all other cropping systems had very high sustainability (Figure 2).
In the SAF results, DR1+CC stood out as the only cropping systems which had more negative than positive differences (relative to DRREF) in the environmental sustainability indicators (Figure 3). There was no clear distinction between crop diversification practices among the few cases of negative differences in environmental indicators for the other commercial cropping systems (Figure 3). The proportion of semi-natural habitat consistently showed large positive differences for all commercial cropping systems, similar to the results of the MCDA for the land use indicator (Figures 2, 3).
Farmers highlighted several barriers to increasing diversity in cropping systems (Table 3). Regarding knowledge, some of the farmers agreed on the lack of knowledge when it comes to increasing diversity in cropping systems. It is difficult to find sources or tools that can help them design crop rotations, such as synchronizing cover crops and main crops in the rotation to get better results without increasing problems such as weeds and diseases. Farmers also pointed out how difficult it may be to find suitable advice regarding cover crops and alternative crops. According to farmers (DR2-3+CC and DR3-4+CC+GM):
“Lack of knowledge of which crops can best adapt to the main crop or preceding crop.”
“It is difficult to find useful advice, for example, for cover crops.”
Table 3. Thematic description of challenges, barriers, and farmers' views on crop diversification in organic farming systems.
Farmers need guidance, new skills, and support to acquire existing knowledge within the field. Managing the complex farming system is perceived as a major barrier by farmers to crop diversification. According to farmers, there is a need for special advisors and help with increased motivation to implement new farming systems. Furthermore, the lack of suitable technology is another challenge mentioned by the farmers; it is difficult for them to find machinery well-adapted to organic and diversified cropping systems.
Regarding market and value chains, the lack of infrastructure to support diversified products is a structural challenge that requires collaboration between different networks to accomplish a solution. Furthermore, according to farmers, restrictions in market stability and low payment are other major questions. In this context, according to farmers, their relationship with distributors and retailers is affected negatively due to retailers' and distributors' expectations on buying very cheap products. All farmers sell nearly all their crop products to regional (vegetables, certain grain legumes and niche crops) or national (cereals) distributors, with rare exceptions of selling directly to consumers.
Farmers further highlighted less inclusive and lack of local markets and a poor relationship between producer/consumer or food industry that could help strengthen the value chains and overcome technological barriers during the post-harvest of alternative or minor crops. As farmer (DR1-2+CC+GM) stated:
“The market is quite limited when you want to do more [include minor crops or intercrops]; otherwise, you have to be able to refine the product yourself.”
Another issue that farmers might face with some of the alternative crops is the risk of not achieving the quality required by the market. Sometimes the quality is not high enough for food consumption, and then the product is only possible to sell for animal feed at a lower price.
Concerning policy and regulations, there is a huge need for policy changes, clear criteria, and simple structures. As it is today, according to farmers, there is unclear and non-supportive regulation regarding sustainability transition. Farmers also mentioned weather variations and changing climate as a constraint since uncertainties regarding, e.g., unforeseen weather events such as drought or warm winters make it difficult to plan their choice of crops, cover crops, and management. According to farmer (DR3-4+CC+GM):
“There is a lot of climate variation. For example, in the case of some cover crops left in the field until they have frozen. It might be that due to the mild winters, they do not freeze at all.”
These results complement the sustainability assessments and align with some of the MCDA outcomes in the economic and social dimension: in particular regarding the medium to low sustainability in the MCDA indicators potential profitability, access to inputs, access to output markets, and operational difficulties.
The studied diversified cropping systems showed high scores in overall sustainability, according to our assessments. These results are in line with other studies showing that diversified cropping systems improve the use of resources (Lin, 2011; St. Luce et al., 2020), improve soil quality (Bardgett and van der Putten, 2014; Barbieri et al., 2019), and enhance the associated biodiversity (Beillouin et al., 2021) which in turn can strengthen the resilience via the increased provision of ecosystem services (Davis et al., 2012; Liebman et al., 2015; Bullock et al., 2017; Lichtenberg et al., 2017).
While most of the assessed cropping systems showed high sustainability in the environmental indicators, results were more variable in the economic and social dimensions. In particular, potential profitability stands out as the MCDA indicator with low sustainability for almost all cropping systems. The average gross margin indicator of the SAF showed similar levels or negative differences compared to the reference system. The relative disadvantage in economic sustainability is likely a consequence of increased costs for cover crop seeds and labor costs to manage more diverse cropping systems. Furthermore, a lack of value addition for products derived from diversified cropping systems, compared to equivalent products from less diverse systems, could play an important role. Indeed, during the interviews farmers pointed out the lack of economic incentives for crop diversification, and in particular that it is challenging to find output markets for minor or alternative crops that could diversify their crop rotations (Table 3). These results are consistent with other studies showing that the economic performance of diversified cropping systems is highly influenced by profitability, economic stability, dependency on external inputs and access to markets and investment capacity (Colomb et al., 2012; Pelzer et al., 2012; Deytieux et al., 2016; Iocola et al., 2020). However, various limitations influence farmers' decisions on crop choice, including market constraints (e.g., crop prices), availability of technologies for production, and policies and regulations that may facilitate or hinder trades (Bowman and Zilberman, 2013). Lack of market flexibility and poor establishment of local markets, along with high costs for seeds of alternative and cover crops are likely to reduce profitability and/or increase the dependency on subsidies of the diversified cropping systems. Uncertainty regarding market prices and low capacity to develop more sustainable markets do affect the competitiveness of alternative crops related to the well-established cereal production systems in Europe (Magrini et al., 2016; Zander et al., 2016; Meynard et al., 2018). Morel et al. (2020) identified obstacles downstream in the value chain (food and processing enterprises, retailers and consumers), where market actors focus on large volumes of few crops, making it difficult for the farmers to compete with small volumes of alternative crops.
Another challenge highlighted in the MCDA assessment and farmer interviews is that more diversified cropping systems tend to cause increased operational difficulties. Farmers lifted the limited knowledge and access to information about choice and management of cover crops as a critical barrier for this diversification practice (Table 3). Furthermore, farmers highlighted difficulties in acquiring knowledge about new crops to be introduced in the rotations, raising unanswered questions such as which crops, how many crops, and which combinations in mixtures to select, and the right time for crop establishment. These issues also relate to advisory services, technologies and markets, which farmers perceive as being based mainly on a few commodity crops. Therefore, farmers need to invest their own time to find appropriate machinery and knowledge for the management of their diversified cropping systems, as well as for building relationships with potential buyers of niche crops such as minor cereals, grain legumes and vegetables (Voisin et al., 2013; Magrini et al., 2018).
The increase of activities to synchronize several crops in the rotation, difficulties in employing additional labor in periods of intensive labor, and regulations that define the timing for crops to be established might also have contributed to the low sustainability shown by the indicator of operational difficulties. Indeed, higher cost for inputs, including labor, combined with low-profit margins, may disincentivize farmers' ability to acquire more labor. Mamine and Farès (2020) highlighted the higher cost of crop production on cereal-legume intercropping by increasing management operations in the establishment and the sorting operations after harvest. Further, there is evidence that the adjustment of the management practices in each growing season may discourage farmers from using continuous cover crops for long-term periods (Dunn et al., 2016; Roesch-McNally et al., 2017).
Despite farmers acknowledging the intensity of the work when having many crops in the systems, e.g., higher level of system complexity, this study recognized the efficiency by the farmers in carrying out the management practices according to the positive score in the work overload indicator (SAF) in most commercial cropping systems compared to the reference (Figure 3). The reason is likely due to farmer awareness on enhancing the sustainability of their farming systems by diminishing the environmental impact of their crop production while using the resources efficiently (e.g., by reducing fuel consumption and increasing crop diversification practices such as cover crops, alternative crops, leys, and flower strips). However, there are still many economic disadvantages and risks for farmers who replace main crops with green manures or leys, and thus, a need for policy interventions to encourage such practices. During the interviews, some farmers suggested a cost-share mechanism to alleviate the economic pressure. Furthermore, if the intention of national and European policy makers is to increase the sustainability of agricultural systems, not only financial incentives should be awarded to the farmers but also the creation of markets for alternative crops to cereals that could be integrated into crop rotations. In this context, farmers perceived that crop diversification could be hindered by the rural and agricultural policies that define the crops that can be included in the crop rotation and the timing for the crops to be established. Current agricultural policies may not provide sufficient incentives to farmers to increase crop diversification to a larger scale. Agricultural production systems are assessed mainly through their economic performance with insufficient focus on the potential that crop diversification practices may have in improving the social and environmental contexts (Kremen and Miles, 2012).
There seems to be a need for improved education and training of skills, for farmers and advisors and other actors in the agricultural knowledge systems (e.g., agricultural schools), about managing diversified cropping systems. Another area where actions are needed to facilitate crop diversification is the market development for alternative crops, which could be stimulated, e.g., via increased consumer awareness and policies that promote more localized/regionalized food systems and short value chains (Morel et al., 2020). Here, research can contribute by identifying factors that enable the development of consumer-producer relationships that support crop diversity or investigate how barriers to crop diversification can be overcome.
The use of manure by the commercial farms was valued as positive for soil quality and soil carbon inputs, compared to the reference and diversified field experiment, which did not receive any manure (they only received organic fertilizers based on by-products from the slaughter and food industry). A recent meta-analysis highlighted the positive effect of agricultural diversification practices on soil quality, driven mainly by organic amendments and reduced tillage (Tamburini et al., 2020). Hence, the application of organic amendments results in an immediate increase in soil organic carbon (Larney and Angers, 2012) and might be an option for carbon sequestration in the local context (field and farm), in addition to enhanced crop productivity. On the other hand, carbon losses could occur due to increased rates of decomposition of soil organic matter and microbial respiration in response to inputs of labile carbon via manure (Yazdanpanah et al., 2016; Isbell et al., 2017). Soil disturbance stimulates carbon losses, even shallow tillage and hoeing to control weeds can have negative impacts that might partly counteract the positive effects of significant carbon inputs, e.g., green manure and crop residues. For instance, introducing grain legumes without cover crops showed higher carbon losses than when only having cover crops without the grain legumes (Plaza-Bonilla et al., 2018). The combination of crop patterns and diversity may be an effective way to restore soil functioning.
N fertilizers are the main contributors of greenhouse gas emissions in cereal-based cropping systems. The use of organic fertilizers instead of mineral fertilizers could reduce the N2O emission by 40 % per hectare due to enhanced soil organic carbon and microbial biomass (Skinner et al., 2019). Besides, including N2-fixing crops in the crop rotation reduces greenhouse gas emissions compared with N-fertilized crops (Jensen et al., 2011). Furthermore, all assessed cropping systems in our study showed high sustainability in energy use (MCDA) and greenhouse gas emissions from fuel consumption (SAF). Despite the increased activities establishing alternative and cover crops, farmers may have found a way to synchronize management activities according to the crop choice. Indeed, a combination of crop rotation and reduced tillage may reduce greenhouse gas emissions and N and C losses (Renwick et al., 2019).
Several studies acknowledge the environmental benefits of diversified cropping systems using legumes (Nemecek et al., 2008). Whether included as main crops or cover crops, legumes provide the cropping system with inputs of biologically fixed nitrogen (Peoples et al., 1995) and may promote microbial activity resulting in enhanced phosphorus mobilization (Drinkwater and Snapp, 2007), reducing the reliance on external nutrient inputs. Legumes used in cover crop mixtures with non-legumes species can also improve the N availability for the subsequent crops and at the same time decrease N leaching (Tribouillois et al., 2016; Watson et al., 2017). The interviews highlighted the farmers' view that lentils and lupines for human consumption might be attractive crops due to growing market demand and higher prices than more common grain legumes such as peas. However, the variability of legume grain yield (Cernay et al., 2015) and unstable markets limit farmers' adoption to a larger extent (Watson et al., 2017).
Increased crop diversity (e.g., by integrating cover crops) has been shown to improve water regulation and quality (Tamburini et al., 2020). The interviewed farmers also mentioned these advantages, mainly when soil cover was ensured during the periods with a high risk of runoff or leaching. Indeed, Tonitto et al. (2006) reported a reduction of nitrate leaching by almost 70% by winter cover crops than bare soil and, hence, without sacrificing the main crop yields. Overall, the diversified cropping systems that were assessed here performed far better in terms of nutrient use efficiency than the reference (Figure 3, NU: Nitrogen use indicator). The cropping systems were also characterized for improving the nutrient balance through better synchrony of main crops and cover crops. Several studies have shown that increasing the spatial and temporal plant diversity results in a more efficient use of nutrients or improved nutrient cycling, e.g., by the retention and accumulation of nitrogen and by reducing fertilizer inputs (e.g., Isbell et al., 2017).
All commercial cropping systems had high sustainability in land use and occurrence of semi-natural habitats, which is partly artificial since the field experiment did not include any non-crop area and thus no semi-natural habitat. Even though the comparison with the reference is biased for this indicator, it is worth highlighting the value of semi-natural habitats or other non-crop areas for the sustainability of commercial cropping systems. Non-crop areas in connection to cultivated fields might improve the landscape value (Hails et al., 2019), e.g., by providing opportunities for recreation and conserving cultural, spiritual, or aesthetic values but also to enhance insect biodiversity such as pollinators and natural predators for pest control (Haaland and Gyllin, 2009). On the other hand, farmers stressed the low acceptability or awareness that society can have about the benefits offered by increasing the diversity of crops in the fields and associated impacts on the rural landscape. These limitations might indicate a gap between the societal values of diversified agricultural landscapes (e.g., for recreation and biodiversity conservation) and the appreciation that farmers receive for managing and maintaining this landscape—including the conservation of semi-natural and other non-crop areas.
The small number of farms where cropping systems were assessed, and the lack of low-diversity cropping systems for comparison, limit our possibilities to analyze the effects of increased crop diversity on sustainability (the experimental reference is also a diverse cropping system, even if it lacks cover crops and intercrops since it has six different crops that represent different plant families and life cycles). Thanks to the combination of sustainability assessment tools and farmer interviews, we could obtain a deep insight into the strengths and challenges of crop diversification despite the limited sample size, although a structured analysis across crop diversity levels was not possible. Another critical point is that the two field experiments stand out as a contrast to the commercial farms, and using an experimental system as a reference is somewhat artificial to the context of real farms. The experimental cropping systems were included since they represent a relevant case for an ex ante comparison of sustainability between a reference and an innovative, diversified system in the regional context and within organic production. The fact that three of the participating farmers had provided inputs when designing these experimental systems added further relevance to assess these in a similar way as the commercial systems. Lastly, the direct access to detailed descriptions of crops and management in the field experiment (where all crops in the rotation was present each year) made it helpful to use the two experimental systems as representative prototypes of diversified organic cropping systems in the region. However, even though efforts were made to set realistic input data for indicators that could not be determined based on the experimental protocol and results (notably in the economic and social dimensions), it is clear that an experimental setting cannot provide a complete representation of a commercial farm context. Therefore, differences in the results between the experimental and commercial cropping systems should be interpreted with caution, since these might be caused not only by the differences in system design and crop management but also by the contrasting contexts. While our study is context specific, we find that it has generated a rich understanding about challenges related to diversification and sustainability of organic cropping systems. We believe that it will be valuable if other studies apply a similar approach, i.e., combining sustainability assessments and qualitative interviews in other contexts, e.g., in conventional cropping systems.
The lower sustainability in some of the economic and social indicators of the diversified system in the field experiment compared to several of the commercial cropping systems is interpreted as a consequence of larger complexity, resulting in a higher level of operational difficulties in coordinating management activities, increasing the risks of crop failure or other problems in the production. On the other hand, some of the positive impacts expected from the combination of crop diversification practices were achieved, such as the improvement of nutrient use efficiency, reduced N inputs, and increased associated biodiversity.
The results of sustainability assessments studies depend to a large extent on the methodological framework used (Sala et al., 2015) in terms of sustainability indicators included and the assumptions underlying the calculations. We acknowledge that the focus on organic production was not sufficiently captured by the indicators we used. In particular, the lack of indicators for organic fertilizers or amendments may have over- or underestimated the environmental impact of the diversified cropping systems. Furthermore, some indicators on genetic diversity may be less accurate because of limited information on the different crops, varieties, and cultivars included in the assessed commercial cropping systems. The imbalance between sustainability dimensions regarding the number of indicators (fewer indicators in the economic dimension of both tools, and fewer indicators in the social dimension of SAF) is another issue. This could affect the sustainability assessment results by influencing the perception of what is important for sustainable development and thus increasing the risk that the respondent, in this case, the farmer, overestimates the score. Our approach to combine two tools is a way to reduce the risk of bias or missing important aspects due to the lack of indicators. However, neither of the tools used in this study included indicators which cover aspects of producing crops for healthy food. In particular, indicators that focus on the amount and diversity of food (per hectare) that the cropping systems could provide would have been relevant and interesting to include. Iocola et al. (2020) highlighted the lack of more appropriate indicators that could cover farmers' opinions and insights on life quality. Since life quality aspects such as work satisfaction and appreciation from society can be very difficult to frame objectively in indicators for sustainability assessments, qualitative interviews can be very useful to complement the assessment with deeper insights by farmers' own description of their situations (Bryant and Charmaz, 2007). This was clearly the case in our study, and as elaborated above (sections Challenges: Profitability and Complexity and Strengths in Terms of Soil Quality, Climate Impact, and Resource Use Efficiency), the interview results enriched the understanding of how farmers might perceive challenges of access to knowledge and markets, complexity in managing diversified cropping systems, and low appreciation from society for their efforts to preserve or enhance landscape biodiversity. However, the combined collection of data for the sustainability assessments and qualitative data on farmers' perceptions required long interviews, and data processing for MCDA, SAF as well as the thematic analysis of the interview results are time-demanding processes. Therefore, while the mixed method approach that we applied has benefits in providing a rich and deep understanding of the studied context, time constraints (both for researchers and farmers) might pose limits to the feasible sample size (as in our case).
Diversification of cropping systems achieves the main goal of increased sustainability. The assessed organic cropping systems already included high plant diversity at the field and farm scale, which promoted positive effects on the environment by the efficient use of nutrients and reducing the risk of pollution by non-use of synthetic fertilizers and pesticides, increasing the associated biodiversity. However, diversified organic cropping systems still have many challenges to overcome, especially in the economic and social dimensions. Farmers are aware that crop diversification practices do not stand alone but depend on the development of more inclusive markets, boosting more local value chains and making the benefits of diversified cropping systems recognized by the society—these are key factors for the transformation of the agri-food systems toward enhanced sustainability. Lastly, our study showed that sustainability assessment tools combined with qualitative interviews might help identify synergies and trade-offs of crop diversification. However, the complexity and time-consuming operation of these methods may limit their use. There is a need for more simple ways to monitor sustainability, not only for farmers but also for researchers, advisors, consumers and other actors.
The original contributions presented in the study are included in the article/Supplementary Material, further inquiries can be directed to the corresponding author/s.
Ethical review and approval was not required for the study on human participants in accordance with the local legislation and institutional requirements. The patients/participants provided their written informed consent to participate in this study.
CR and GC formulated the ideas and research questions. CR performed all interviews and data analyses and wrote the first draft of the manuscript. L-MDM and MZ provided inputs to the formulation of research questions. L-MDM participated in writing of the first draft. GC participated in data analyses. All authors contributed with the final version of this manuscript.
The authors declare that the research was conducted in the absence of any commercial or financial relationships that could be construed as a potential conflict of interest.
All claims expressed in this article are solely those of the authors and do not necessarily represent those of their affiliated organizations, or those of the publisher, the editors and the reviewers. Any product that may be evaluated in this article, or claim that may be made by its manufacturer, is not guaranteed or endorsed by the publisher.
We are very grateful to the farmers who participated in the study by sharing information about their farms. We thank Erik Rasmusson for providing information about the technical management of the field experiments included in the assessments. We acknowledge the DiverIMPACTS project (funded by the European Union's Horizon 2020 research and innovation programme under grant agreement No. 727482) to develop the sustainability assessment framework and make the indicators of the framework available to us. Thanks also to Erik Steen Jensen for contributions in the initial part of the study, and Frédérique Angevin, Loïc Viguier, and Nicolas Carton for valuable assistance in the use of sustainability assessment indicators and tools.
The Supplementary Material for this article can be found online at: https://www.frontiersin.org/articles/10.3389/fagro.2021.698968/full#supplementary-material
Supplementary Table 1. Indicators from the sustainability assessment framework that were excluded or set to zero.
Supplementary Table 2. MCDA tree including the indicators at the different hierarchical levels.
Supplementary Table 3. Values obtained from the sustainability assessment framework for all the crop rotations included in the study.
Barbieri, P., Pellerin, S., Seufert, V., and Nesme, T. (2019). Changes in crop rotations would impact food production in an organically farmed world. Nat. Sustain. 2, 378–385. doi: 10.1038/s41893-019-0259-5
Bardgett, R. D., and van der Putten, W. H. (2014). Belowground biodiversity and ecosystem functioning. Nature 515, 505–511. doi: 10.1038/nature13855
Beillouin, D., Ben-Ari, T., Malézieux, E., Seufert, V., and Makowski, D. (2021). Positive but variable effects of crop diversification on biodiversity and ecosystem services. Glob. Change Biol. 1–14. doi: 10.1111/gcb.15747
Bockstaller, C., Guichard, L., Keichinger, O., Girardin, P., Galan, M.-B., and Gaillard, G. (2009). Comparison of methods to assess the sustainability of agricultural systems. A review. Agron. Sustain. Dev. 29, 223–235. doi: 10.1051/agro:2008058
Bockstaller, C., Guichard, L., Makowski, D., Aveline, A., Girardin, P., and Plantureux, S. (2008). Agri-environmental indicators to assess cropping and farming systems. A review. Agron. Sustain. Dev. 28, 139–149. doi: 10.1051/agro:2007052
Bohanec, M. (2008). DEXi: Program for Multi-attribute Decision Making. User's Manual, Version 3.00. IJS Report DP-9989. Ljubljana: Jozef Stefan Institute.
Bowman, M. S., and Zilberman, D. (2013). Economic factors affecting diversified farming systems. Ecol. Soc. 18:33. doi: 10.5751/ES-05574-180133
Bryant, A., and Charmaz, K. (2007). The SAGE Handbook of Grounded Theory. London: SAGE Publications.
Bullock, J. M., Dhanjal-Adams, K. L., Milne, A., Oliver, T. H., Todman, L. C., Whitmore, A. P., et al. (2017). Resilience and food security: rethinking an ecological concept. J. Ecol. 105, 880–884. doi: 10.1111/1365-2745.12791
Cernay, C., Ben-Ari, T., Pelzer, E., Meynard, J. M., and Makowski, D. (2015). Estimating variability in grain legume yields across Europe and the Americas. Sci. Rep. 5:11171. doi: 10.1038/srep11171
Chopin, P., Mubaya, C. P., Descheemaeker, K., Öborn, I., and Bergkvist, G. (2021). Avenues for improving farming sustainability assessment with upgraded tools, sustainability framing and indicators. A review. Agron. Sustain. Dev. 41:19. doi: 10.1007/s13593-021-00674-3
Colomb, B., Carof, M., Aveline, A., and Bergez, J.-E. (2012). Stockless organic farming: strengths and weaknesses evidenced by a multicriteria sustainability assessment model. Agron. Sustain. Dev. 33, 593–608. doi: 10.1007/s13593-012-0126-5
Davis, A. S., Hill, J. D., Chase, C. A., Johanns, A. M., and Liebman, M. (2012). Increasing cropping system diversity balances productivity, profitability and environmental health. PLoS One 7:e47149. doi: 10.1371/journal.pone.0047149
de Olde, E., Oudshoorn, F., Bokkers, E., Stubsgaard, A., Sørensen, C., and de Boer, I. (2016). Assessing the sustainability performance of organic farms in Denmark. Sustainability 8:957. doi: 10.3390/su8090957
Deytieux, V., Munier-Jolain, N., and Caneill, J. (2016). Assessing the sustainability of cropping systems in single- and multi-site studies. A review of methods. Eur. J. Agron. 72, 107–126. doi: 10.1016/j.eja.2015.10.005
Diacono, M., Persiani, A., Testani, E., and Montemurro, F. (2019). Sustainability of agro-ecological practices in organic horticulture: yield, energy-use and carbon footprint. Agroecol. Sustain. Food Syst. 44, 726–746. doi: 10.1080/21683565.2019.1704961
Drinkwater, L. E., and Snapp, S. S. (2007). “Understanding and managing the rhizosphere in agroecosystems,” in The Rhizosphere, eds. Z. G. Cardon, and J. L. Whitbeck (Burlington: Academic Press), 127–153.
Dunn, M., Ulrich-Schad, J. D., Prokopy, L. S., Myers, R. L., Watts, C. R., and Scanlon, K. (2016). Perceptions and use of cover crops among early adopters: findings from a national survey. J. Soil Water Conserv. 71, 29–40. doi: 10.2489/jswc.71.1.29
European Commission (2020). Farm to Fork Strategy: For a Fair, Healthy and Environmentally-Friendly Food System. Available online at: https://ec.europa.eu/food/sites/food/files/safety/docs/f2f_action-plan_2020_strategy-info_en.pdf (accessed November 25, 2020).
Foley, J. A., Ramankutty, N., Brauman, K. A., Cassidy, E. S., Gerber, J. S., Johnston, M., et al. (2011). Solutions for a cultivated planet. Nature 478, 337–342. doi: 10.1038/nature10452
Garnett, T., Appleby, M. C., Balmford, A., Bateman, I. J., Benton, T. G., Bloomer, P., et al. (2013). Agriculture. Sustainable intensification in agriculture: premises and policies. Science 341, 33–34. doi: 10.1126/science.1234485
Haaland, C., and Gyllin, M. (2009). Butterflies and bumblebees in greenways and sown wildflower strips in southern Sweden. J. Insect Conserv. 14, 125–132. doi: 10.1007/s10841-009-9232-3
Hails, R. S., Chaplin-Kramer, R., Bennett, E., Robinson, B., Daily, G., Brauman, K., et al. (2019). “Determining the value of ecosystem services in agriculture,” in Agricultural Resilience, eds. R. S. Hails, S. M. Gardner, and S. J. Ramsden (Cambridge: Cambridge University Press), 60–89.
Hawes, C., Young, M. W., Banks, G., Begg, G. S., Christie, A., Iannetta, P. P. M., et al. (2019). Whole-systems analysis of environmental and economic sustainability in arable cropping systems: a case study. Agronomy 9:438. doi: 10.3390/agronomy9080438
Hayashi, K. (2000). Multicriteria analysis for agricultural resource management: a critical survey and future perspectives. Eur. J. Oper. Res. 122, 486–500. doi: 10.1016/S0377-2217(99)00249-0
Hunter, M. C., Smith, R. G., Schipanski, M. E., Atwood, L. W., and Mortensen, D. A. (2017). Agriculture in 2050: recalibrating targets for sustainable intensification. Bioscience 67, 386–391. doi: 10.1093/biosci/bix010
Iocola, I., Angevin, F., Bockstaller, C., Catarino, R., Curran, M., Messéan, A., et al. (2020). An actor-oriented multi-criteria assessment framework to support a transition towards sustainable agricultural systems based on crop diversification. Sustainability 12:5434. doi: 10.3390/su12135434
IPES-Food (2016). From Uniformity to Diversity: A Paradigm Shift from Industrial Agriculture to Diversified Agroecological Systems. International Panel of Experts on Sustainable Food Systems.
Isbell, F., Adler, P. R., Eisenhauer, N., Fornara, D., Kimmel, K., Kremen, C., et al. (2017). Benefits of increasing plant diversity in sustainable agroecosystems. J. Ecol. 105, 871–879. doi: 10.1111/1365-2745.12789
Jensen, E. S., Peoples, M. B., Boddey, R. M., Gresshoff, P. M., Hauggaard-Nielsen, H. J. R., et al. (2011). Legumes for mitigation of climate change and the provision of feedstock for biofuels and biorefineries. A review. Agron. Sustain. Dev. 32, 329–364. doi: 10.1007/s13593-011-0056-7
Koschke, L., Fürst, C., Lorenz, M., Witt, A., Frank, S., and Makeschin, F. (2013). The integration of crop rotation and tillage practices in the assessment of ecosystem services provision at the regional scale. Ecol. Indic. 32, 157–171. doi: 10.1016/j.ecolind.2013.03.008
Kremen, C., Iles, A., and Bacon, C. (2012). Diversified farming systems: an agroecological, systems-based alternative to modern industrial agriculture. Ecol. Soc. 17:44. doi: 10.5751/ES-05103-170444
Kremen, C., and Miles, A. (2012). Ecosystem services in biologically diversified versus conventional farming systems: benefits, externalities, and trade-offs. Ecol. Soc. 17:40. doi: 10.5751/ES-05035-170440
Lampridi, M., Sørensen, C., and Bochtis, D. (2019). Agricultural sustainability: a review of concepts and methods. Sustainability 11:5120. doi: 10.3390/su11185120
Lang, T. I. M., and Barling, D. (2012). Food security and food sustainability: reformulating the debate. Geogr. J. 178, 313–326. doi: 10.1111/j.1475-4959.2012.00480.x
Larney, F. J., and Angers, D. A. (2012). The role of organic amendments in soil reclamation: a review. Can. J. Soil Sci. 92, 19–38. doi: 10.4141/cjss2010-064
Lichtenberg, E. M., Kennedy, C. M., Kremen, C., Batary, P., Berendse, F., Bommarco, R., et al. (2017). A global synthesis of the effects of diversified farming systems on arthropod diversity within fields and across agricultural landscapes. Global Change Biol. 23, 4946–4957. doi: 10.1111/gcb.13714
Liebman, M., Schulte, L. A., Kapuscinski, A. R., Salvador, R. J., and Locke, K. (2015). Enhancing agroecosystem performance and resilience through increased diversification of landscapes and cropping systems. Elementa 3:000041. doi: 10.12952/journal.elementa.000041
Lin, B. B. (2011). Resilience in agriculture through crop diversification: adaptive management for environmental change. Bioscience 61, 183–193. doi: 10.1525/bio.2011.61.3.4
Magrini, M.-B., Anton, M., Chardigny, J.-M., Duc, G., Duru, M., Jeuffroy, M.-H., et al. (2018). Pulses for sustainability: breaking agriculture and food sectors out of lock-in. Front. Sustain. Food Syst. 2:64. doi: 10.3389/fsufs.2018.00064
Magrini, M.-B., Anton, M., Cholez, C., Corre-Hellou, G., Duc, G., Jeuffroy, M.-H., et al. (2016). Why are grain-legumes rarely present in cropping systems despite their environmental and nutritional benefits? Analyzing lock-in in the French agrifood system. Ecol. Econ. 126, 152–162. doi: 10.1016/j.ecolecon.2016.03.024
Mamine, F., and Farès, M. H. (2020). Barriers and levers to developing wheat-pea intercropping in Europe: a review. Sustainability 12:6962. doi: 10.3390/su12176962
Mayring, P. (2000). Qualitative content analysis. Forum Qual. Soc. Res. 1:20. doi: 10.17169/fqs-1.2.1089
Meynard, J.-M., Charrier, F., Fares, M. H, Le Bail, M., Magrini, M.-B., et al. (2018). Socio-technical lock-in hinders crop diversification in France. Agron. Sustain. Dev. 38:54. doi: 10.1007/s13593-018-0535-1
Morel, K., Revoyron, E., San Cristobal, M., and Baret, P. V. (2020). Innovating within or outside dominant food systems? Different challenges for contrasting crop diversification strategies in Europe. PLoS One 15:e0229910. doi: 10.1371/journal.pone.0229910
Nemecek, T., von Richthofen, J.-S., Dubois, G., Casta, P., Charles, R., and Pahl, H. (2008). Environmental impacts of introducing grain legumes into European crop rotations. Eur. J. Agron. 28, 380–393. doi: 10.1016/j.eja.2007.11.004
Pacini, C., Wossink, A., Giesen, G., Vazzana, C., and Huirne, R. (2003). Evaluation of sustainability of organic, integrated and conventional farming systems: a farm and field-scale analysis. Agric. Ecosyst. Environ. 95, 273–288. doi: 10.1016/S0167-8809(02)00091-9
Payraudeau, S., and van der Werf, H. M. G. (2005). Environmental impact assessment for a farming region: a review of methods. Agric. Ecosyst. Environ. 107, 1–19. doi: 10.1016/j.agee.2004.12.012
Pelzer, E., Bourlet, C., Carlsson, G., Lopez-Bellido, R. J., Jensen, E. S., and Jeuffroy, M. H. (2017). Design, assessment and feasibility of legume-based cropping systems in three European regions. Crop Pasture Sci. 68, 902–914. doi: 10.1071/CP17064
Pelzer, E., Fortino, G., Bockstaller, C., Angevin, F., Lamine, C., Moonen, C., et al. (2012). Assessing innovative cropping systems with DEXiPM, a qualitative multi-criteria assessment tool derived from DEXi. Ecol. Indic. 18, 171–182. doi: 10.1016/j.ecolind.2011.11.019
Peoples, M. B., Herridge, D. F., and Ladha, J. K. (1995). “Biological nitrogen fixation: an efficient source of nitrogen for sustainable agricultural production?,” in Management of Biological Nitrogen Fixation for the Development of More Productive and Sustainable Agricultural Systems, eds. J. K. Ladha, and M. B. Peoples (Dordrecht: Springer Netherlands), 3–28.
Piikki, K., and Söderström, M. (2019). Digital soil mapping of arable land in Sweden - Validation of performance at multiple scales. Geoderma 352, 342–350. doi: 10.1016/j.geoderma.2017.10.049
Plaza-Bonilla, D., Nogué-Serra, I., Raffaillac, D., Cantero-Martínez, C., and Justes, É. (2018). Carbon footprint of cropping systems with grain legumes and cover crops: a case-study in SW France. Agric. Syst. 167, 92–102. doi: 10.1016/j.agsy.2018.09.004
Rao, N. H., and Rogers, P. P. (2006). Assessment of agricultural sustainability. Curr. Sci. 91, 439–448. Available online at: http://www.jstor.org/stable/24093944
Renwick, L. L. R., Bowles, T. M., Deen, W., and Gaudin, A. C. M. (2019). “Potential of increased temporal crop diversity to improve resource use efficiencies,” in Agroecosystem Diversity, eds. G. Lemaire, P. C. D. F. Carvalho, S. Kronberg, and S. Recous (Academic Press), 55–73. doi: 10.1016/B978-0-12-811050-8.00004-2
Riley, J. (2001). Indicator quality for assessment of impact of multidisciplinary systems. Agric. Ecosyst. Environ. 87, 121–128. doi: 10.1016/S0167-8809(01)00272-9
Rockstrom, J., Williams, J., Daily, G., Noble, A., Matthews, N., Gordon, L., et al. (2017). Sustainable intensification of agriculture for human prosperity and global sustainability. Ambio 46, 4–17. doi: 10.1007/s13280-016-0793-6
Roesch-McNally, G. E., Basche, A. D., Arbuckle, J. G., Tyndall, J. C., Miguez, F. E., Bowman, T., et al. (2017). The trouble with cover crops: farmers' experiences with overcoming barriers to adoption. Renew. Agric. Food Syst. 33, 322–333. doi: 10.1017/S1742170517000096
Röös, E., Fischer, K., Tidåker, P., and Nordström Källström, H. (2019). How well is farmers' social situation captured by sustainability assessment tools? A Swedish case study. Int. J. Sustain. Dev. World Ecol. 26, 268–281. doi: 10.1080/13504509.2018.1560371
Sala, S., Ciuffo, B., and Nijkamp, P. (2015). A systemic framework for sustainability assessment. Ecol. Econ. 119, 314–325. doi: 10.1016/j.ecolecon.2015.09.015
Schipanski, M. E., Barbercheck, M., Douglas, M. R., Finney, D. M., Haider, K., Kaye, J. P., et al. (2014). A framework for evaluating ecosystem services provided by cover crops in agroecosystems. Agric. Syst. 125, 12–22. doi: 10.1016/j.agsy.2013.11.004
Skinner, C., Gattinger, A., Krauss, M., Krause, H. M., Mayer, J., van der Heijden, M. G. A., et al. (2019). The impact of long-term organic farming on soil-derived greenhouse gas emissions. Sci. Rep. 9:1702. doi: 10.1038/s41598-018-38207-w
Soinne, H., Keskinen, R., Räty, M., Kanerva, S., Turtola, E., Kaseva, J., et al. (2020). Soil organic carbon and clay content as deciding factors for net nitrogen mineralization and cereal yields in boreal mineral soils. Eur. J. Soil Sci. 72, 1497–1512. doi: 10.1111/ejss.13003
Statistics Sweden (2020a). Ekologisk Växtodling 2019 Serie JO - Jordbruk, Skogsbruk och Fiske [Organic Crop Production 2019 Series JO - Agriculture, Forestry and Fish]. Statistiska Centralbyrån-Jordbruksverk. Available online at: https://djur.jordbruksverket.se/webdav/files/SJV/Amnesomraden/Statistik%20fakta/Arealer/JO13/JO13SM2001/JO13SM2001_omstatistiken.htm (accessed October 03, 2020).
Statistics Sweden (2020b). Jordbruksstatistisk Sammanstallning 2020 Med Data om Livsmedel - Tabeller [Agricultural Statistics 2020 Including Food Statistics - Tables]. Available online at: https://jordbruksverket.se/download/18.78dd5d7d173e2fbbcda98893/1597390150166/JS_2020.pdf (accessed December 15, 2020).
Statistics Sweden (2020c). Jordbruksverkets Statistikdatabas [The Swedish Board of Agriculture's Statistics Database]. Statistiska Centralbyrån-Jordbruksverk. Available online at: https://statistik.sjv.se/PXWeb/pxweb/sv/Jordbruksverkets%20statistikdatabas/ (accessed October 06, 2020).
St. Luce, M., Lemke, R., Gan, Y., McConkey, B., May, W., Campbell, C., et al. (2020). Diversifying cropping systems enhances productivity, stability, and nitrogen use efficiency. Agron. J. 112, 1517–1536. doi: 10.1002/agj2.20162
Swedish Board of Agriculture (2014). Landsbygdsprogrammet 2014-2020 [The Rural Development Program 2014-2020]. Available online at: https://jordbruksverket.se/stod/programmen-som-finansierar-stoden/landsbygdsprogrammet (accessed September 18, 2015).
Tamburini, G., Bommarco, R., Wanger, T. C., Kremen, C., van der Heijden, M. G. A., Liebman, M., et al. (2020). Agricultural diversification promotes multiple ecosystem services without compromising yield. Sci. Adv. 6:eaba1715. doi: 10.1126/sciadv.aba1715
Thorup-Kristensen, K., Magid, J., and Jensen, L. S. (2003). Catch crops and green manures as biological tools in nitrogen management in temperate zones. Adv. Agron. 79, 227–302. doi: 10.1016/S0065-2113(02)79005-6
Tonitto, C., David, M. B., and Drinkwater, L. E. (2006). Replacing bare fallows with cover crops in fertilizer-intensive cropping systems: a meta-analysis of crop yield and N dynamics. Agric. Ecosyst. Environ. 112, 58–72. doi: 10.1016/j.agee.2005.07.003
Tribouillois, H., Cohan, J. P., and Justes, E. (2016). Cover crop mixtures including legume produce ecosystem services of nitrate capture and green manuring: assessment combining experimentation and modelling. Plant Soil 401, 347–364. doi: 10.1007/s11104-015-2734-8
Trivino-Tarradas, P., Gomez-Ariza, M. R., Basch, G., and Gonzalez-Sanchez, E. J. (2019). Sustainability assessment of annual and permanent crops: the Inspia Model. Sustainability 11:738. doi: 10.3390/su11030738
Viguier, L., Cavan, N., Bockstaller, C., Cadoux, S., Corre-Hellou, G., Dubois, S., et al. (2021). Combining diversification practices to enhance the sustainability of conventional cropping systems. Eur. J. Agron. 127:126279. doi: 10.1016/j.eja.2021.126279
Voisin, A.-S., Guéguen, J., Huyghe, C., Jeuffroy, M.-H., Magrini, M.-B., Meynard, J.-M., et al. (2013). Legumes for feed, food, biomaterials and bioenergy in Europe: a review. Agron. Sustain. Dev. 34, 361–380. doi: 10.1007/s13593-013-0189-y
Watson, C. A., Reckling, M., Preissel, S., Bachinger, J., Bergkvist, G., Kuhlman, T., et al. (2017). “Chapter 4: Grain legume production and use in European Agricultural Systems,” in Advances in Agronomy, ed. D. L. Sparks (Academic Press), 235–303.
Willett, W., Rockstrom, J., Loken, B., Springmann, M., Lang, T., Vermeulen, S., et al. (2019). Food in the Anthropocene: the EAT-Lancet Commission on healthy diets from sustainable food systems. Lancet 393, 447–492. doi: 10.1016/S0140-6736(18)31788-4
Willey, R. W. (1979). Intercropping - its importance and research needs: part 1. Competition and Yield Advantages. Field Crop Abstr. 32, 1–10.
Yazdanpanah, N., Mahmoodabadi, M., and Cerdà, A. (2016). The impact of organic amendments on soil hydrology, structure and microbial respiration in semiarid lands. Geoderma 266, 58–65. doi: 10.1016/j.geoderma.2015.11.032
Keywords: sustainability assessments, crop diversification, crop rotation, farmers' perceptions, ex ante, cover crops, intercropping, mixed methods
Citation: Rodriguez C, Dimitrova Mårtensson L-M, Zachrison M and Carlsson G (2021) Sustainability of Diversified Organic Cropping Systems—Challenges Identified by Farmer Interviews and Multi-Criteria Assessments. Front. Agron. 3:698968. doi: 10.3389/fagro.2021.698968
Received: 22 April 2021; Accepted: 26 August 2021;
Published: 23 September 2021.
Edited by:
Daniel Plaza-Bonilla, Universitat de Lleida, SpainReviewed by:
Daniele Antichi, University of Pisa, ItalyCopyright © 2021 Rodriguez, Dimitrova Mårtensson, Zachrison and Carlsson. This is an open-access article distributed under the terms of the Creative Commons Attribution License (CC BY). The use, distribution or reproduction in other forums is permitted, provided the original author(s) and the copyright owner(s) are credited and that the original publication in this journal is cited, in accordance with accepted academic practice. No use, distribution or reproduction is permitted which does not comply with these terms.
*Correspondence: Carolina Rodriguez, carolina.rodriguez@slu.se
Disclaimer: All claims expressed in this article are solely those of the authors and do not necessarily represent those of their affiliated organizations, or those of the publisher, the editors and the reviewers. Any product that may be evaluated in this article or claim that may be made by its manufacturer is not guaranteed or endorsed by the publisher.
Research integrity at Frontiers
Learn more about the work of our research integrity team to safeguard the quality of each article we publish.