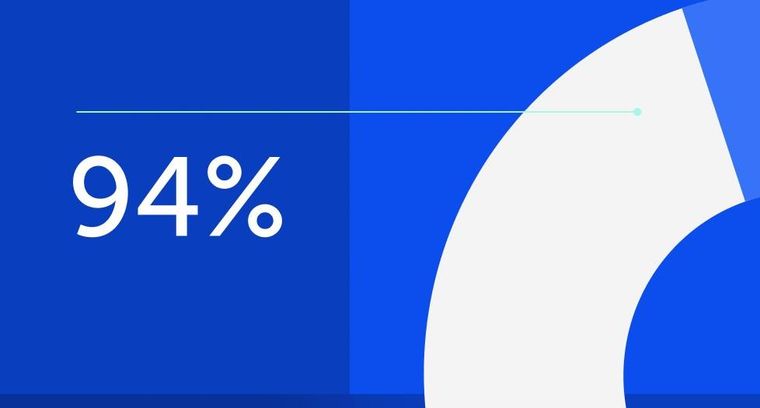
94% of researchers rate our articles as excellent or good
Learn more about the work of our research integrity team to safeguard the quality of each article we publish.
Find out more
ORIGINAL RESEARCH article
Front. Agron., 13 April 2021
Sec. Field Water Management
Volume 3 - 2021 | https://doi.org/10.3389/fagro.2021.659722
Skip furrow irrigation (SFI) can save water by irrigating every alternate furrow. Usually, irrigated furrows are alternated at each irrigation event under SFI. If irrigated furrows are fixed (permanent SFI, PSFI), more water may be saved by reducing the wetting area, and salts may accumulate on the dry side of the ridge. Salt removal sheet, a method of collecting salts on the sheet laid on the soil surface utilizing high evaporation demand in drylands, may be an efficient measure for removal of accumulated salt under PSFI. We evaluated salinity movement and water saving under PSFI through a field experiment in Uzbekistan. In addition, a salt removal sheet was applied to the shoulder of the ridge on the drier side under PSFI to evaluate its potential in removing salt from the soil with the practice. The results showed that salts tend to accumulate on the dry side of the ridge, and the amount of irrigation water was halved without a large crop yield reduction. The PSFI + Sheet was able to catch 6.4% of salts in the top 10 cm soil layer.
In drylands, which occupy ~40% of the world's land area (Niemeijer et al., 2005), irrigation is essential for agricultural production. However, saline irrigation water used in drylands often causes massive input of salts to farmlands. Improper irrigation management has often caused salinization, which negatively affects agricultural production. Salinization of farmlands has increased dramatically in recent decades (Martin et al., 2012), causing large economic losses. It is estimated that an economic loss of 27.3 billion dollars will occur if salt accumulation occurs in about 20% (about 60 million ha) of the ~310 million of irrigated farmland (Qadir et al., 2014).
The main causes of salinization in irrigated agriculture are over-irrigation, non-uniformity in water application, and poor drainage. Over-irrigation has often raised the groundwater table and led to subsequent capillary-driven salt accumulation. Therefore, to prevent salinization, irrigation water should be saved. However, there is a tendency to over-irrigate in developing countries due to field undulation and leakage from irrigation canal, which then leads to salinization. Farmers in developing countries are unable to conduct precise land leveling and renew or maintain irrigation facilities properly due to lack of funds. This type of salinization is remarkable in Central Asia. The soil in the plains of Central Asia is naturally rich in salt, and together with the dry climate, the risk of irrigation-induced salinization is high (Shirokova and Morozov, 2006). Uzbekistan suffers from extensive damages by salinization, and the salt-affected area is the largest among the Central Asian countries. In Uzbekistan, cotton production had increased dramatically by large-scale irrigation development during the Soviet era. It peaked around 1980 and then declined due to salinization associated with inappropriate water management (Ishii, 1994). The area of irrigated farmland in Uzbekistan was 4.31 million ha (2017), most of which was surface-irrigated (Frenken, 2013). Furrow irrigation is still widely used, but field undulation and leakage from irrigation canal due to lack of funds and labor often causes over-irrigation (Forkutsa et al., 2009).
Under saline conditions, frequent irrigation, uniform water distribution, and regimes that provide leaching requirements are important (Minhas et al., 2020). Water saving by introducing modern technologies such as sprinkler or drip irrigation systems and surface/sub-surface drainage is effective against salinization; however, installation of these technologies is still difficult for farmers in the countryside due to lack of funds. In Uzbekistan, leaching is widely conducted in winter after wheat cultivation as a traditional measure against salinization. However, since the flow rate of rivers is relatively high in winter, farmers tend to use large volumes of water, resulting in groundwater rise in some farmlands with poor drainage (Okuda and Onishi, 2012; Okuda et al., 2015). Poor drainage is partly due to hardened soil owing to compaction by the long-term use of agricultural machinery (Okuda et al., 2018).
Skip furrow irrigation (SFI) is a water-saving method based on furrow irrigation (Khan et al., 1999; Horst et al., 2007; Leininger et al., 2019). Irrigation water can be saved by irrigating every other furrow instead of all furrows, as illustrated in Figure 1.
Figure 1. The concept of skip furrow irrigation (SFI). (A) is conventional furrow irrigation, (B) is skip furrow irrigation.
Lateral compensative infiltration, driven by enhanced suction under non-irrigated furrows by SFI, may help to maintain yields (Leininger et al., 2019). Khan et al. (1999) reported ~40% reduction in irrigation water under SFI with a slight decrease in cotton yield (Khan et al., 1999). Horst et al. (2007) stated that ~29% of irrigation water was saved using SFI without a significant reduction in cotton yield (Horst et al., 2007). Usually, wet furrows are alternatively replaced at each irrigation event under the SFI (Brouwer et al., 1985). However, if water is delivered to both furrows, even if it is alternatively at each irrigation events, salts tend to accumulate at the center of ridges, negatively affecting germination and subsequent plant growth (Ayers and Westcot, 1985). Therefore, to prevent salt accumulation at the center of ridges, Zaman et al. (2018) recommended fixing the wetting furrows (permanent SFI, PSFI) (Zaman et al., 2018). Another advantage of PSFI over SFI is further water saving by reducing the wetting area of the soil surface. In conventional SFI, the soil surface of the watered furrow is not fully dry at the next irrigation event, and evaporation continues at a low rate even if the furrow is not watered after the next irrigation event. In contrast, in PSFI, the soil surface of the non-watered furrow is almost air dried, and evaporation is negligible. Onishi et al. (2018) reported that under PSFI, salts are pushed to the dry side of the ridge (Onishi et al., 2018). However, if these accumulated salts are not removed for several months, occasional rain will leach the salts back to the center of the ridge, salinize the main root zone.
Abe (1995) proposed a method to capture the accumulated salts on soil surfaces to a sheet utilizing high evaporative demand in drylands (Abe, 1995). In this method, a cotton sheet is laid on the soil surface with good connection, and salts in the soil move to the soil surface due to evaporation-driven capillary flow, resulting in salt accumulation in/on the sheet. Then, the salts are easily removed by peel off the sheet from the soil. We may remove the accumulated salts on the shoulder of ridges under PSFI by covering the soil surface with a cotton sheet and allowing salts to accumulate in/on the sheet. If salts pushed out to the side of the ridge under PSFI can be efficiently removed using a cotton sheet, this method will be an effective approach to remove salts from field surface. In this study, focusing on a shallow soil layer where salinity is severe (Devkota et al., 2015), we applied PSFI with half amount of irrigation water compared with that in conventional furrow irrigation and investigated salt movement in the ridges and cotton yield. In addition, a salt removal sheet was applied to the shoulder of the ridge under PSFI to evaluate its potential to remove salt under field conditions.
A field experiment was conducted during the cotton cultivation period of 2017. We selected a field (40°34′58" N, 68°39′01" E) in the Axmedov Water Consumers' Association area, Mirzabod District, Syrdarya Region, Uzbekistan (farm A) (Figure 2). Located at the midstream of the Syrdarya River, the Syrdarya Region is rich in water resources, but 98% of irrigated farmland is affected by salinization (Onishi et al., 2017). The average daily temperature reaches 30°C in summer and drops to 0°C in winter. The annual precipitation is ~320 mm, but precipitation from June to September is very low (~20 mm). The standard size of farmlands in the Syrdaria Region is ~40–60 ha. The size of the farmland used in the experiment was ~51 ha (820 m × 620 m). Cotton is usually cultivated from April to September, and irrigation is conducted two or three times during the season (Onishi et al., 2019).
In farm A, the irrigation canal is located on the east side of the farmland, while the drainage canal is located on the north and west sides. The electric conductivity (EC) of irrigation water was around 1.1 dS m−1. Groundwater level increased to a depth of ~0.5 m in January and then gradually decreased to around 3.5 m depth toward December. The EC of groundwater was ~20.0 dS m−1. Test plots were set up on the northern side of the farm. The width of the ridge and furrow was 0.5 and 0.4 m, respectively, and the test plot was 1.3 m wide (one ridge and two furrows) and 4.0 m long. Two treatments for conventional furrow irrigation, namely every furrow irrigated (Control) and PSFI + salt removal sheet (PSFI + Sheet), were established with three replicates. The layout of the irrigation and drainage canals at farm A and the settings of the test plots are illustrated in Figure 3.
The physical properties of the soil are listed in Table 1. Soil samples were taken using 100 mL stainless sampling cylinders. Bulk density was measured using the drying method. The falling head method was employed to measure saturated hydraulic conductivity. Particle size analysis was conducted using the pipette method. The bulk density was ~1.40 g cm−3, but a hard soil layer with a bulk density of ~1.60 g cm−3 was formed from 25 to 35 cm depth. Soil texture of farm A was classified as loam or clay loam according to the standard of the International Society of Soil Science.
The measured volumetric water contents at pF1.8, 2.6, and 3.0 are shown in Figure 4. The saturated hydraulic conductivity and readily available water (RAW) of these hard layers were relatively lower than those of the other layers.
In the PSFI + sheet, a black cotton sheet (Katsuragi, No.10) was applied. This sheet is a standard fabric for use in clothing and is available in general markets. The price was ~4 USD m−2. We selected a black color, which absorbs solar radiation, to promote evaporation and subsequent capillary flow. In order to efficiently remove salts pushed to the side of the ridge under PSFI, the sheet, 0.3 m in width and 4.0 m in length, was laid on the dry side of the ridge. Immediately after laying the sheet, ~2 mm of water was showered to enhance contact between the sheet and the soil. First and second irrigations were conducted on 11 and 24 July, respectively. Water was applied using a gasoline pump (LGP 20-A; Leo Group Co Ltd, Binhai, China; discharge rate 5 L s−1) to both sides of the ridge in the control and to the right (wet) side of the ridge in PSFI + Sheet treatment, as shown in Figure 5.
Figure 5. Application of PSFI and salt removal sheet. Before irrigation, the salt removal sheet was applied on the left (dry) side of the ridge, and the furrow located to the right was irrigated (A). The situation of the field is shown in (B).
The amount of water applied to the control and PSFI + Sheet at each irrigation was 0.52 and 0.26 m3, respectively. These amounts were determined to be 100 mm, as recommended by the Research Institute of Irrigation and Water Problem in Uzbekistan (RIIWP). The volumetric water content before the first and second irrigation was 0.04 and 0.07 m3 m−3, respectively. Before and after the irrigation, the sheet was removed and cut into a square of ~5 cm, and soil samples were collected at depths of 0, 1, 5, and 10 cm on the left, right and in the center of the ridge (Figure 6). The soil was collected manually using a thin shovel. The soil samples were packed in plastic bags and transported to the laboratory.
Soil salinity was measured in terms of the EC of the suspension with the 1:1 (EC1:1) method. The soil solution was prepared by drying the soil samples at 105°C for 24 h and adding the same weight of distilled water as that of the dried soil. The salinity of the sheet was measured in terms of EC by adding distilled water 100 times the mass of the sheet. The salt concentrations of the suspensions, C (g L−1), were estimated using the following equation, assuming that the major ions were calcium and chloride (Egamberdieva et al., 2010), which were obtained through laboratory calibration:
where σ is the EC of the solution (dS m−1).
The concentration of the suspension (g L−1) obtained by the above equation was converted to salt content per unit volume (mg cm−3). The soil volume of 10 cm length in each block (left, center, right and 0–1, 1–5, 5–10 cm depth) was calculated using cross-sectional area obtained with the piecewise quadrature method. The mass of salt in each position was obtained by multiplying the average unit salt content by the soil volume of each block. The salt removal ratio was defined as the ratio of salt content in the salt removal sheet to the total mass of salts on the left side of the ridge in the top 10 cm soil layer, including the salt removal sheet.
In order to evaluate the salinity movement under PFSI, contour map of unit salt content (mg cm−3) was made with unit salt contents at each position of sampled soil. The distribution of salt content before and after irrigation under each treatment is shown in Figure 7.
Figure 7. Distribution of mass of salt per unit volume before and after irrigation. In the control (A), salts were accumulated on the center of the ridge. In PSFI + Sheet (B), salts were moved to the left (dry) side of the ridge.
In the control, salts were transported to the center of the ridge, resulting in a remarkably increased salt content in the center of the ridge after the second irrigation. The mass of salt at the ridge center (10 cm wide and 10 cm thick at the center of the ridge, as shown in Figure 6) after the second irrigation accounted for ~57.9% of the salinity in the entire ridge (all ranges for which the mass was calculated, refer to Figure 6). Salts tend to accumulate in the center of the ridge due to the pressure gradient under conventional furrow irrigation, which may adversely affect plant growth. On the other hand, in the PSFI + Sheet, salts were pushed out to the left (dry) side of the ridge, and the mass of salt on the left (dry) side of the ridge (10 cm wide and 10 cm thick on the left side of the ridge) after the second irrigation accounted for about 51.2% of the salinity in the entire ridge (all ranges for which mass was calculated). These results indicate that soil salinity of the ridge was pushed to dry side due to the water flow of ridge was fixed on same direction under the application of PSFI, preventing salt accumulation in the center of the ridge. There is a possibility of mitigating the effects of salinization on the plants at the center of the ridges. The amount of salts per square centimeter (mg cm−2) collected on the salt removal sheet at each sampling day are shown in Figure 8.
The salinity of the sheet gradually increased with time, and greatly increased after the second irrigation. Although the deviation of the three plots was high, a remarkable increment of salts in the sheet to 0.24 mg cm−2 was measured in one plot. This result suggested that the longer laying period, the salinity of the sheet become higher, if good connection of soil and sheet could be kept long, it can be expected that the sheet catch a lot of salt. The time evolution of the salt removal ratio for each plot is shown in Figure 9.
The amount of removed salt fraction on July 14, 3 days after the first irrigation, were almost identical to those before irrigation; 1.1% was the highest value detected in the three plots. However, on July 27, 3 days after the second irrigation, the fraction of removed salt increased to 6.4% in plot No. 6. This result suggests that salt removal sheets may be applicable to the farmland as farming activity. And this method may be useful at farmlands which drainage are poor because soil salinity is removed from the soil surface. This value was lower than that reported by Abe et al. (2000); these authors confirmed that many salts could be removed using a cotton sheet from soil columns under laboratory conditions (Abe et al., 2000). The smaller fraction of removed salt in the present study may had been caused by the looser contact between the sheet and soil surface, which hindered the capillary flow of saline soil water to the sheet. The contact may have been lost because of the weak fastening of the sheet onto the soil surface. Therefore, pressing onto the soil surface by hand or roller may be required after each irrigation. The fresh cotton yield of each plot measured on 11 September is shown in Figure 10. Even though the amount of irrigation water was halved under the application of PSFI, the cotton yield did not decrease. This result indicates that PSFI will save water without incurring additional costs and causing a large reduction in yield for at least one season. In the case of applying PSFI in some years, water stress may be occurred, supplemental irrigation should be conducted according to crop growth.
Figure 10. Cotton yield in control and PFSI + sheet treatments. The cotton yield in control and PFSI + sheet were 279.3 and 283.1 g m−2, respectively. Significant reduction in yield with the application of PFSI + Sheet was not observed.
In this study, we focused on the top 10 cm soil layer, a critical zone of root water uptake and early growth, to evaluate the effect of permanent skip furrow irrigation (PSFI) on cotton yield and salt movement in the soil through a field experiment in Uzbekistan. We confirmed that PSFI can halve the amount of irrigation water without causing a significant decrease in crop yield, the salts tend to accumulate on the drier side of the ridge. PSFI is an effective method for water saving and to mitigate salinization in developing countries because of its low cost and simple application. However, in the case of application of PSFI in some years, since water stress may cause, crop growth should be observed carefully. On the other hand, we also confirmed that the combination of PSFI and salt removal sheet laid on the surface of dry side of the ridges has the potential to remove soil salinity; the maximum removal amount of salt was 0.24 mg cm−2, accounted for 6.4% of salts in the top 10 cm soil layer. This result suggests that salt removal sheets may be a method that can be implemented for salt removing of soil surface in farmlands. The effect may be higher in farmland which poor drainage because this method remove salinity from soil surface. Inefficient removal using the sheet may be due to poor contact between the sheet and the soil, which may have blocked the capillary flow of saline water into the sheet. In fields, the salt removal sheet that is simply laid on the soil surface will detach soon due to wind or weeds. In addition, the price of the sheet is may present some expense to farmers. Therefore, for practical application of the salt removal sheet, it is necessary to seek a realistic method for their use in crop fields in consideration of the actual situation in developing countries where funds and labor are limited. Reuse of the sheets after washing and their selected application to spots with severe salinization may bolster their use in practice. Further studies are needed for practical use, such as the drought and salinity stresses caused by applying PSFI under different combinations of soil and crop, and the method of laying sheets for enhancing adhesion to soil.
The original contributions presented in the study are included in the article/supplementary material, further inquiries can be directed to the corresponding author/s.
JO and HF conceptualized and designed the study. JO, HF, GP, and YS collected and analyzed the data. JO and HF wrote, reviewed, and edited the manuscript. All authors have read and agreed to the published version of the manuscript.
This study was conducted as a research project in the Resource Management in Asia and Pacific Islands by the Japan International Research Center for Agricultural Sciences (JIRCAS).
The authors declare that the research was conducted in the absence of any commercial or financial relationships that could be construed as a potential conflict of interest.
We gratefully acknowledge the Farmers' Council of Uzbekistan as a research counterpart, the Farmer of the Experimental Field, and the support staff of the Research Institute of Irrigation and Water Problems.
Abe, Y. (1995). Removal of salt and excess water from soils using evaporative force. J. Arid Land Stud. 5S, 163–166.
Abe, Y., Nakatani, T., Kuwahata, K., and Yokota, S. (2000). Comparative study on new desalination method (Salt removal sheet) by evaporative force and leaching method. J. Arid Land Stud. 10, 147–156.
Ayers, R. S., and Westcot, D. W. (1985). 2.4.4 Cultural Practices, Water Quality for Agriculture Irrigation and Drainage Paper No. 29. Rome: FAO. Available online at: http://www.fao.org/3/t0234e/T0234E03.htm (accessed August 6, 2020).
Brouwer, C., Prins, K., Kay, M., and Heibloem, M. (1985). 3.4.1 Wetting Patterns, Irrigation Water Management Irrigation Methods, Training Manual No. 5. Rome: FAO. Available online at: http://www.fao.org/3/S8684E/s8684e04.htm (accessed July 28, 2020).
Devkota, M., Gupta, R. K., Martius, C., Lamers, J. P. A., Devkota, K. P., Sayre, K. D., et al. (2015). Soil salinity management on raised beds with different furrow irrigation modes in salt-affected lands. Agric. Water Manage. 152, 243–250. doi: 10.1016/j.agwat.2015.01.013
Egamberdieva, D., Renella, G., Wirth, S., and Islam, R. (2010). Secondary salinity effects on soil microbial biomass. Biol. Fertil. Soils 46, 445–449. doi: 10.1007/s00374-010-0452-1
Forkutsa, I., Sommer, R., Shirokova, Y. I., Lamers, J. P. A., Kienzler, K., Tischbein, B., et al. (2009). Modeling irrigated cotton with shallow groundwater in the Aral Sea Basin of Uzbekistan II. Soil salinity dynamics. Irrig. Sci. 27, 319–330. doi: 10.1007/s00271-009-0149-0
Frenken, K. (2013). Irrigation in Central Asia in Figures. FAO Water Reports 39 AQUASTAT Survey-2012. Rome: FAO. 183–205.
Horst, M. G., Shamutalov, S. S., Goncalves, J. M., and Pereira, L. S. (2007). Assessing impacts of surge-flow irrigation on water saving and productivity of cotton. Agric. Water Manag. 87, 115–127. doi: 10.1016/j.agwat.2006.06.014
Ishii, S. (1994). Agricultural issues in Central Asia. Bulletin of the Institute of Middle Eastern Studies. Int. Univ. Japan 8, 1–17.
Khan, K. H., Rana, M. A., and Arshad, M. (1999). Alternate furrow irrigation for enhancing water use efficiency in cotton. Pak. J. Agric. Sci. 36, 175–177.
Leininger, S. D., Krutz, L. J., Sarver, J. M., Gore, J., Henn, A., Bryant, C. J., et al. (2019). Skip row furrow irrigation optimizes peanut pod yield net returns and irrigation water use efficiency. Crop Forage Turfgrass Manag. 5, 1–6. doi: 10.2134/cftm2018.08.0061
Martin, R. C., Glover-Cutter, K., Baldwin, J. C., and Dombrowski, J. E. (2012). Identification and characterization of a salt stress-inducible zinc finger protein from Festuca arundinacea. BMC Res. Notes 5:66. doi: 10.1186/1756-0500-5-66
Minhas, P. S., Ramos, B. T., Ben-Galc, A., and Pereirad, L. S. (2020). Coping with salinity in irrigated agriculture: crop evapotranspiration and water management issues. Agric. Water Manag. 227:105832. doi: 10.1016/j.agwat.2019.105832
Niemeijer, D., Puigdefabregas, J., White, R., Lal, R., Winslow, M., Ziedler, J., et al. (2005). Chapter 22 Dryland Systems, Ecosystems and Human Well-being: Current State and Trends, Vol. 1. Millennium Ecosystem Assessment Series. Washington, DC: Island Press. 623–662.
Okuda, Y., Omori, K., and Onishi, J. (2018). Shallow Sub-surface Drainage for Mitigating Salinization Technical Manual. Japan International Research Center for Agricultural Sciences (JIRCAS), Tsukuba, vii–viii.
Okuda, Y., and Onishi, J. (2012). Current status and problems of farmland damage from salinization in Uzbekistan. J. Japan Soc. Irrig. Drainage Rural Eng. 80, 3–6.
Okuda, Y., Onishi, J., Omori, K., Oya, T., Fukuo, A., Kurvantaev, R., et al. (2015). Current status and problems of the drainage system in Uzbekistan. J. Arid Land Stud. 25, 81–84. doi: 10.14976/jals.25.3_81
Onishi, J., Ikeura, H., Paluashova, G. K., Shirokova, Y. I., Kitamura, Y., and Fujimaki, H. (2019). Suitable inflow rate and furrow length for simplified surge flow irrigation. Paddy Water Environ. 17, 185–193. doi: 10.1007/s10333-019-00710-9
Onishi, J., Ikeura, H., Yamanaka, I., Kitamura, Y., and Fujimaki, H. (2017). Water-saving effect of simplified surge flow irrigation on irrigated farmlands in arid areas - case study in the Republic of Uzbekistan J. Arid Land Stud. 27, 91–101. doi: 10.14976/jals.27.3_91
Onishi, J., Okuda, Y., Paluashova, G. K., Shirokova, Y. I., Kitamura, Y., and Fujimaki, H. (2018). Salt pushing effect to the side of the ridge by fixed skip furrow irrigation -case study in the Republic of Uzbekistan-. J. Arid Land Stud. 28S, 155–158. doi: 10.14976/jals.28.S_155
Qadir, M., Quillérou, E., Nangia, V., Murtaza, G., Singh, M., Thomas, R. J., et al. (2014). Economics of salt-induced land degradation and restoration. Nat. Resour. Forum 38, 282–295. doi: 10.1111/1477-8947.12054
Shirokova, Y. I., and Morozov, A. N. (2006). Salinity of Irrigated Land of Uzbekistan Causes and Present statE. Tasks for Vegetation Science Book Series (TAVS, Volume 42). Sabkha Ecosystems. Dordrecht: Springer. 249–259.
Keywords: salinization, drylands, water-saving, skip furrow irrigation, salt removal sheet, Uzbekistan
Citation: Onishi J, Paluashova GK, Shirokova YI and Fujimaki H (2021) Salt Removal by Combining a Permanent Skip Furrow Irrigation and Salt Removal Sheet. Front. Agron. 3:659722. doi: 10.3389/fagro.2021.659722
Received: 28 January 2021; Accepted: 16 March 2021;
Published: 13 April 2021.
Edited by:
Junliang Fan, Northwest A and F University, ChinaReviewed by:
Tibin Zhang, Northwest A and F University, ChinaCopyright © 2021 Onishi, Paluashova, Shirokova and Fujimaki. This is an open-access article distributed under the terms of the Creative Commons Attribution License (CC BY). The use, distribution or reproduction in other forums is permitted, provided the original author(s) and the copyright owner(s) are credited and that the original publication in this journal is cited, in accordance with accepted academic practice. No use, distribution or reproduction is permitted which does not comply with these terms.
*Correspondence: Junya Onishi, anVueWE4OUBhZmZyYy5nby5qcA==
Disclaimer: All claims expressed in this article are solely those of the authors and do not necessarily represent those of their affiliated organizations, or those of the publisher, the editors and the reviewers. Any product that may be evaluated in this article or claim that may be made by its manufacturer is not guaranteed or endorsed by the publisher.
Research integrity at Frontiers
Learn more about the work of our research integrity team to safeguard the quality of each article we publish.