- 1Department of Crop and Soil Sciences, Faculty of Agriculture, Kwame Nkrumah University of Science and Technology, Kumasi, Ghana
- 2Forest and Horticultural Crops Research Center, College of Basic and Applied Science, University of Ghana, Accra, Ghana
- 3Department of Theoretical and Applied Biology, Kwame Nkrumah University of Science and Technology, Kumasi, Ghana
- 4International Institute of Tropical Agriculture, Ibadan, Nigeria
- 5Council for Scientific and Industrial Research (CSIR) – Soil Research Institute, Kumasi, Ghana
Rhizobia inoculation with effective strains is an environmentally friendly approach for enhancing nodulation and yield of legumes. To obtain an ideal strain for inoculant production, the strain's performance must be matched to the environmental conditions. A 2 × 9 cross-factorial experiment laid in a randomized complete block design with three replications on farmers' fields in the northern part of Ghana was set up to evaluate the rhizobia's performance. The factors were groundnut varieties (Chinese and Samnut 22) and nine strains consisting of five native rhizobia (KNUST 1001, 1002, 1003, 1032, and 1031), two commercial strains (Biofix and BR3267), and a positive (N+) and absolute control (N–). In addition, the population of the strains was assessed after a cropping season. The strains' performance on groundnut was location and variety dependent. At Cheshegu, KNUST 1031 and 1002 significantly increased seed yield of the Chinese variety compared to other strains; however, KNUST 1031 elicited a 24% yield increase while KNUST 1002 caused a 16% yield increase over the control. Strain KNUST 1031 increased the seed yield of the Chinese variety by 24% while KNUST 1002 increased the seed yield of Samnut by 16%. Only KNUST 1002 elicited a significant seed yield increase in Samnut 22 at Cheshegu. At Binduri, strain KNUST 1003 significantly increased the seed yield of the Chinese variety by 35% relative to the control. Samnut 22 did not show a clear preference for any of the strains. The Chinese variety did not show a clear response to the strains at Tanina. However, Samnut 22 responded to KNUST 1002 as it increased seed yield by 45% relative to the control. On average, the seed yield at Tanina (846.15 kg ha−1) was less than the seed yield recorded at Binduri (1,077.66 kg ha−1) and Cheshegu (1,502.78 kg ha−1). Inoculation with strains KNUST 1002, 1003, and 1031 was all profitable under the current experimental conditions as they recorded value cost ratios (VCRs) above the threshold of 3–4. The study has shown that strains KNUST 1002, 1003, and 1031 have the potential to be used in inoculant formulation to increase groundnut production and enhance the income of smallholder groundnut farmers in northern Ghana.
Introduction
Groundnut is an important source of protein and forms part of many delicacies in Ghana. The crop provides income to smallholder farmers through the sale of its seed and the oil produced from it. Groundnut biomass and haulms are used to improve the fertility of soils. The annual production of groundnut in Ghana is 521,030 Mt from an area of 319,680 ha (Statistics, Research and Information Directorate (SRID), 2019). The northern part of Ghana contributes about 75.6% of the annual production (Breisinger et al., 2008).
Grain legumes such as groundnut (Arachis hypogea L.) require high amounts of nitrogen for optimum growth (Peoples and Herridge, 1990). The nitrogen demand of such crops can be met through mineral nitrogen fertilization or inoculation with highly competitive and effective rhizobia strains. Nitrogen fertilization is very effective under optimum field conditions; however, the high cost and accessibility limit its use by most smallholder farmers in sub-Saharan Africa (SSA) (Zengeni et al., 2006). Mineral nitrogen fertilizers are one of the main sources of nitrous oxide, which contributes to greenhouse gas emissions and ultimately climate change and its attendant effects (Erbas and Solakoglu, 2017). Also, mineral nitrogen fertilizers have been identified as a major source of water pollution (Erbas and Solakoglu, 2017). These negative effects on the environment threaten the sustainable use of mineral nitrogen fertilizers.
Rhizobia inoculation with highly effective strains is a feasible environmentally friendly approach for enhancing the grain yield of legumes. Several reports in SSA show the positive effect of inoculating groundnut with appropriate rhizobia strain (Yakubu et al., 2010; Kabir et al., 2013; Biswas and Gresshoff, 2014).
However, smallholder farmers have difficulty in accessing quality inoculant(s) partly because there are few inoculant-producing centers in SSA. The quality of inoculants is sometimes compromised before and/or after the farmer(s) receives them due to poor handling and storage practices as well as a lack of strong quality control measures. There has been contrasting reports on field evaluation of imported inoculants due to the degree of effectiveness of the strains and their adaptability to local conditions in SSA (Mathu et al., 2012; Thuita et al., 2012; Masso et al., 2016; Ulzen et al., 2016), creating doubt about the contribution of rhizobia inoculants to legume grain yields. In recent times, there has been a renewed interest in inoculant production in SSA by N2AFRICA (www.n2africa.org) and COMPRO II (www.compro2.org) projects. This has led to the establishment of an inoculant production facility in the northern part of Ghana, necessitating the need to bioprospect, from the indigenous rhizobia population, highly saprophytic and effective strains to feed this production center and to boost groundnut production in northern Ghana.
Several types of research aimed at selecting effective rhizobia for use as inoculants revealed that effective strains capable of inducing high N2 fixation exist within the native rhizobia populations (Ampomah et al., 2008; Yakubu et al., 2010; Kawaka et al., 2014; Osei et al., 2018). Also, the native rhizobia are well-adapted to local conditions and are therefore presumed to have a highly competitive advantage. An equally important attribute is the high saprophytic competence relative to exotic strains, which allows researchers to make informed decisions about subsequent inoculation practices. Thus, bioprospecting for and characterizing native rhizobia to identify effective and persistent strains for use in prescribed doses as inoculants will increase the likelihood of successful inoculation responses. Although this is an entry point for successful inoculation, the success or otherwise of rhizobia inoculation is influenced by the specificity between rhizobia, legume genotype used, and environmental conditions as well as management practices (Woomer et al., 2014). Consequently, studies geared toward matching superior rhizobia strains with improved crop varieties under different environmental conditions are needed. The present study hypothesized that superior effective strains with saprophytic abilities that can meet the N requirement of groundnut exist in soils of Ghana and can effectively increase seed yields of groundnut. This study was, therefore, conducted to (i) determine the response of groundnut to inoculation with rhizobia originating from native populations in soils of northern Ghana and (ii) quantitively assess the population of the introduced strains after a cropping season. Identifying effective and saprophytic competent strains will not only be a major step toward increasing groundnut production and seed yield but will also reduce the frequency of reinoculation.
Materials and Methods
Site Selection and Characteristics
The field trials were conducted at three different locations in the northern part of Ghana during the 2015 cropping season. The experimental sites were located at Cheshegu (northern region) (latitude N09°27′18.2″ to longitude W000°57′22.4″), Binduri (upper east region) (latitude N10°56′57.6″ to longitude W000°18′52.0″), and Tanina (upper west region) (latitude N09°53′13.0″ to longitude W002°27′48.5″). The study sites have a unimodal rainfall distribution with an annual rainfall amount of 1,000–1,200 mm and mean temperature between 26 and 30°C with little variation within the year. The rainfall distribution during the cropping season is presented as Supplementary Figures 1A–C. All the fields had no known history of rhizobia inoculation. Before planting, five soil core samples were taken from each plot and thoroughly mixed, and composite samples were taken into transparent polythene bags and kept in the refrigerator at 4°C before laboratory analysis. The soil parameters analyzed were particle size (hydrometer method), soil pH (1:2.5 soil to distilled water ratio), organic carbon (OC) (modified Walkley and Black procedure) as described by Nelson and Sommers (1996), total nitrogen (Kjeldahl method) as described by Bremner and Mulvaney (1982), available soil phosphorus (Bray No. 1 solution) as described by Olsen (1982), and exchangeable potassium [ammonium acetate (NH4OAc) extract] as described by Helmke and Sparks (1996); details of the characteristics are given in Table 1. Calcium and magnesium were also determined in 1.0 M ammonium acetate (NH4OAc) extract (Black, 1965). The study was conducted under rainfed conditions; hence, no manual irrigation was carried out.
Estimation of Rhizobia Population
Enumeration of indigenous rhizobia population was done using the most probable number (MPN) of plant infection techniques (Vincent, 1970; Somasegaran and Hoben, 2012) before planting and after a cropping season. Soil samples were taken from a depth of 0–20 cm using the W design and bulked. A composite sample was taken and used for the MPN count. Seeds of groundnut varieties (Samnut 22 and Chinese) were used as a trap host for the estimation of the indigenous rhizobia population. The groundnut seeds were surface-sterilized with 95% ethanol and 3% (v/v) hydrogen peroxide (H2O2) solution. The seeds were rinsed in five successive changes of sterilized distilled water and pre-germinated on moistened filter paper in Petri dishes at a temperature of 30°C. After 2 days, well-grown healthy seedlings of similar radicle length were transferred aseptically into growth pouches containing 65 ml nitrogen-free mineral nutrient solution (Broughton and Dilworth, 1971). Twenty grams of the composite soil sample was serially diluted in distilled water using the fivefold, six-step serial dilution method (5−1) and was mixed thoroughly on a vortex mixer. One milliliter of each dilution was used to inoculate seedlings. Four seedlings were inoculated per dilution level. Plants were watered as and when required. The setup was monitored for 28 days, after which scoring was done for the presence or absence of nodules. Population estimates were assigned using the MPNES software (Woomer et al., 1990).
Inoculant Preparation
The peat-based inoculant was prepared at the soil microbiology laboratory of Kwame Nkrumah University of Science and Technology, Kumasi, as previously described by Ulzen et al. (2016) following the method of Somasegaran and Hoben (2012).
Field Preparation, Experimental Design, and Treatments
The field at Cheshegu was planted on July 13, that at Binduri on July 18, and that at Tanina on July 28, 2015. The experimental fields were disc plowed and harrowed using a harrow to a depth of 15 cm. Each plot measured 4 × 4 m with an alley of 1 m within plots and 2 m between blocks. Each experiment was 9 × 2 cross factorial, laid out in randomized complete block design (RCBD) with three replications. The treatment factors were rhizobia strains and varieties. There were nine strains consisting of five native rhizobia strains (KNUST 1001, 1002, 1032, 1003, and 1031) (Osei et al., 2018), two reference strains (BR3267 and Biofix, USDA 110), a positive control with 100 kg N ha−1, and an absolute control (uninoculated and without mineral N). The strain BR3267 is from Brazil and has originally been described by Leite et al. (2018). The two groundnut varieties were Chinese and Samnut 22. The seeds were inoculated under a shade with a peat-based inoculum of respective strains at a rate of 5 g peat kg−1 of seeds using 10% of gum Arabic solution as a sticker. Three seeds of the groundnut varieties, Samnut 22 and Chinese, were sown per hill at a spacing of 60 × 20 cm at each location. The seedlings were thinned to two, after 2 weeks to maintain optimum plant population. Other cultural practices such as weeding and earthing-up were carried out accordingly as and when needed. Samnut 22 is a dual-purpose groundnut cultivar with good oil content, high haulms, and high pod yield (Ajeigbe et al., 2015). It matures within 115–120 days with a potential yield of 2.5 t ha−1 (Ajeigbe et al., 2015). The Chinese variety is an early-maturing (100 days) cultivar with a potential yield of about 3.5 t ha−1. A basal dose of 20 kg P ha−1 in the form of triple superphosphate (46% P2O5) was applied through band placement 2 weeks after planting. Nitrogen (urea) was split-applied at 20 kg N ha−1 2 weeks after emergence and 80 kg N ha−1 at mid-flowering.
Measurement of N2 Fixation
The amount of nitrogen derived from the atmosphere through biological nitrogen fixation (BNF) was estimated using the total nitrogen difference (TND) method in both field and greenhouse experiments. Guinea grass (Panicum maximum) was used as a reference plant (Ashworth et al., 2015). The total amounts of nitrogen in the shoot at mid-flowering and in the reference plant were assessed, and the amount of N2 fixed was calculated using the modified equations of Viera-Vargas et al. (1995). To account for the nitrogen that remained in the roots and/or was lost in the soil, the calculated value was then multiplied by a factor of 1.4 (Unkovich and Baldock, 2008).
Harvesting for Nodulation and Grain Yield
At the R1 stage (beginning bloom) (Fehr et al., 1971) of Samnut 22 and Chinese varieties in the field, 10 plants were randomly selected from the row directly adjacent to the border rows for each treatment plot. With the help of a spade, the selected plants were carefully uprooted by digging 15 cm around each plant, after which the shoots were separated from roots. The adhered soil on the roots was carefully washed in 1-mm sieve mesh after, which the nodules were detached and counted. The nodules and shoots were oven-dried separately at 60°C for 72 h, after which the dry weights were recorded. At the R8 stage (Fehr et al., 1971), the plants were harvested for their pods and grains.
Economic Viability of Using Indigenous Rhizobia Isolates
The profitability of using indigenous rhizobia strains in inoculant formulation was determined through the value cost ratio (VCR) (Roy et al., 2006; Dittoh et al., 2012).
where A represents total benefits as a result of the applied treatment and B represents the total treatment cost. Rhizobia inoculant was estimated at a cost of US$6 ha−1. The cost of 1 kg of groundnut from the open market was US$0.7. An exchange rate of US$1 to GH 3.60 was used in the conversion of the cost to US dollars. A VCR value greater than a threshold of 3–4 was considered profitable (Dittoh et al., 2012).
Statistical Analysis
The data from each location were checked for normality using Shapiro–Wilk's test. Analysis of variance (ANOVA) was applied to the data through Sisvar version 5.6 (Ferreira, 2008). The Scott–Knot test at 5% probability was used to compare treatment means.
Results
Physical and Chemical Properties and the Most Probable Rhizobia Count
The physical and chemical soil properties of the study sites are shown in Table 1. The texture of the soils from Binduri and Tanina was loamy sand while that from Cheshegu was sandy loam. The soil pH levels (6.10–6.38) were considered to be in the medium range. The OC levels at all the study sites were very low: 0.42% at Cheshegu, 0.96% at Binduri, and 0.62% at Tanina. Total N at all the study sites was generally very low, ranging from 0.022 to 0.030%. Available phosphorus (P) ranged from low to medium (17.61–20.54 mg kg−1). In general, the fertility status of the soil at the study sites was very low. The estimated population sizes of the indigenous rhizobia at the study sites were 26.3, 11.2, and 32.8 cells g−1 soil for Cheshegu, Binduri, and Tanina, respectively.
Nodulation, N2 Fixation, Pod Formation, and Grain Yield
The results showed a significant interaction between the strains and groundnut variety for all the parameters measured at Cheshegu. The seed yield increases of the Chinese variety inoculated with strain KNUST 1031 was statistically higher than all the other treatments except strain KNUST 1002 and the mineral N-fertilized treatment. Except for strains KNUST 1002 and 1031, the seed yield of all other test strains was not significantly different from that of the control treatment. Strains KNUST 1002 and 1031 increased seed yield over the control by 16% and 24%, respectively. The Samnut 22 variety, however, responded better to the KNUST 1002 strain as it markedly increased seed yield over the control and the other strains. The seed yield increase by KNUST 1002 on Samnut 22 was comparable only to the yield of plants that received mineral N fertilizer (Table 2). Strain KNUST 1002 significantly increased seed yield over control by 30% and above 22% over the other strains. The results followed a definite pattern at Cheshegu and showed a clear response of the Chinese variety to KNUST 1031 and of Samnut 22 to KNUST 1002 in all the measured parameters. For instance, KNUST 1031 increased the seed yield of the Chinese variety by 24% compared to Samnut 22. Meanwhile, KNUST 1002 increased seed yield on Samnut 22 by 21% compared to the Chinese variety. Generally, seed yields observed for the control treatment on both varieties were at par with all the inoculated treatments (test strains and reference strains) except KNUST 1031 on the Chinese variety and KNUST 1002 on Samnut 22. The two strains KNUST 1031 and KNUST 1002 increased nodulation, shoot biomass, pod number, and BNF of Chinese and Samnut 22 varieties, respectively, relative to the control and the other strains (Table 2).
At Binduri, a significant interaction between the strains and groundnut variety was observed. Unlike Cheshegu, the Chinese variety showed a response to KNUST 1003. The isolate KNUST 1003 significantly increased shoot biomass, BNF, pod number, and seed yield of the Chinese variety relative to the other strains. KNUST 1003 increased the seed yield of the Chinese variety by 35% over the control treatment (Table 3). On the other hand, Samnut 22 did not show a clear response to one particular strain. Although KNUST 1003 recorded the highest grain yield, it was only significant compared to KNUST 1032, BR3267, and the control (Table 3). A seed yield increase of 21% over the control was recorded by strain KNUST 1003 on Samnut 22. The isolate KNUST 1003 recorded a significantly higher yield on Samnut 22 than on the Chinese variety.
Similar to the results at Cheshegu and Binduri, a significant interaction was observed between the strains and groundnut varieties at Tanina for all the parameters measured. On average, the seed yield recorded at Tanina was lower than that recorded at Cheshegu and Binduri (Table 4). The pattern of results was different from Cheshegu and Binduri. Between the varieties, the Chinese variety did not show a clear response to the strains; however, Samnut 22 responded to KNUST 1002 (Table 4). The strain BR3267 recorded the highest seed yield on the Chinese variety but was only significantly different from the yields recorded by the control, Biofix, KNUST 1001, and KNUST 1003 (Table 4). KNUST 1002 significantly increased the seed yield of Samnut 22 over all the other strains except the mineral N-fertilized treatment. KNUST 1002 increased the seed yield of Samnut 22 over the control by 45%. Strain KNUST 1002 performed better on Samnut 22 than on the Chinese variety as it significantly increased all parameter measures on Samnut 22 compared to the Chinese variety.
Economic Viability of Using Indigenous Rhizobia Isolates
About five of the strains (KNUST 1001, 1003, and 1032; Biofix; and BR3267) had VCRs below the threshold of 3–4 at Cheshegu (Figure 1A). Strains KNUST 1002 and 1031 had VCRs of 5.6 and 8.3 for the Chinese variety, which were above the threshold of 3–4. Only strain KNUST 1002 was profitable on Samnut 22 with a VCR of 11.
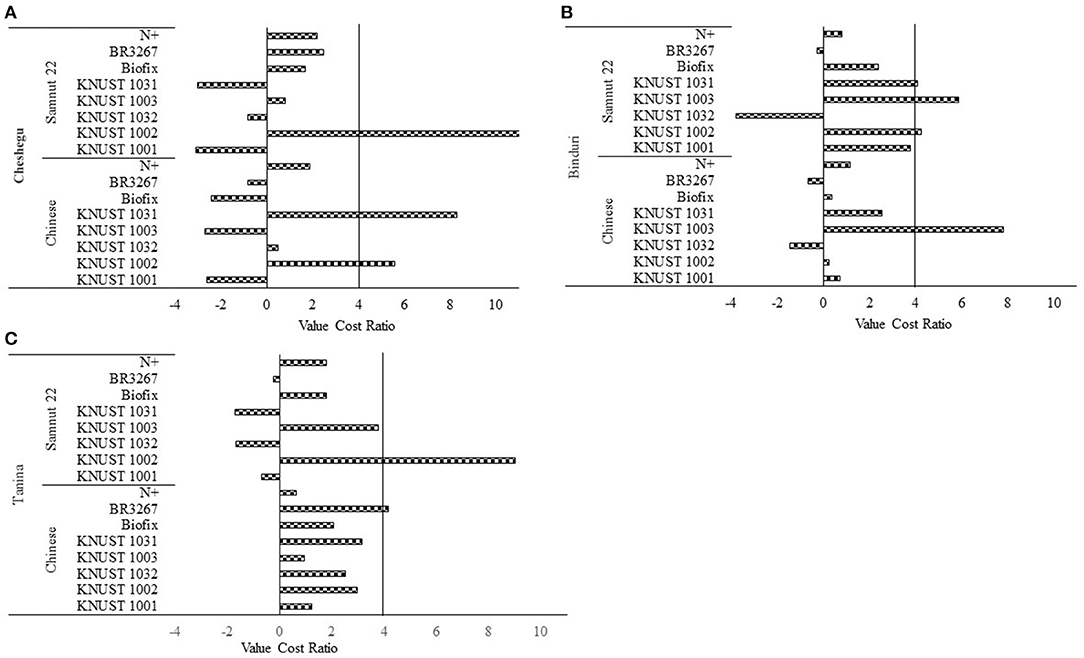
Figure 1. Value cost ratio of strains on Samnut 22 and Chinese varieties Cheshegu (A), Binduri (B) and Tanina (C). The vertical line indicates the threshold beyond which a treatment is considered profitable.
The VCR results at Binduri showed that strain KNUST 1003 recorded the highest VCR of 7.83 on the Chinese variety. The other strains had a VCR less than or within the range of 3–4. On the other hand, strains KNUST 1002, 1003, and 1031 recorded VCR values of 4.24, 5.87, and 4.09, respectively, on Samnut 22 (Figure 1B).
None of the tested strains were profitable on the Chinese variety at Tanina per the threshold of 3–4. Only the industrial strain BR3267 had a VCR of 4.17. However, strain KNUST 1002 had a VCR of 9.04 for Samnut 22 at Tanina. The other strains had VCR values that were less than or within the threshold of 3–4 (Figure 1C).
Rhizobia Population After a Cropping Season of Introduction Into the Soil
The rhizobia population count varied among the strains and across different locations; however, strain KNUST 1002 demonstrated consistency in persistence. The highest rhizobia population count (283.4 cells g−1 soil) after a cropping season was recorded at Binduri by strains KNUST 1002 and 1003. However, only strain KNUST 1002 persisted in high numbers at Cheshegu and Tanina (Figure 2). The population of KNUST 1002 in inoculated soils was more than double that of the uninoculated plots after a year of introduction at all three study locations.
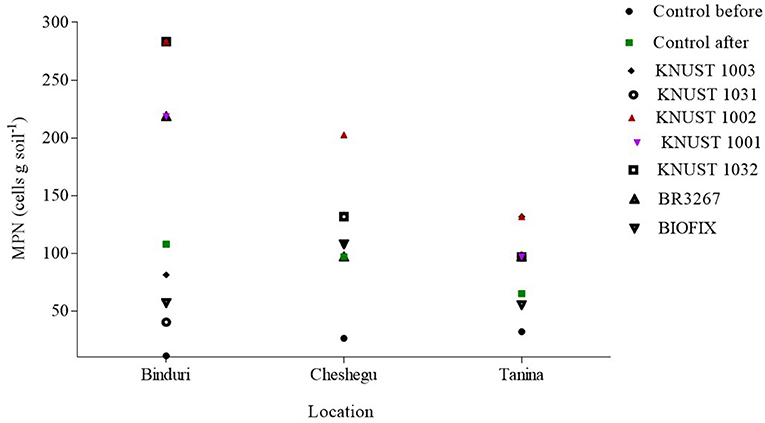
Figure 2. Rhizobia population after one cropping season of introduction. The legends describe the rhizobia population in the control plots before and after the experiment as well as the plots that received the various strains.
Discussion
Until recently, groundnut was regarded as a promiscuous grain legume that nodulated freely with indigenous rhizobia and therefore did not require inoculation during establishment. This skepticism has been challenged by the outcome of several inoculation studies (Ashraf et al., 2006; Sajid et al., 2010; Yakubu et al., 2010; Biswas and Gresshoff, 2014; Grönemeyer et al., 2016; Osei et al., 2020). Groundnut has been reported to respond positively to inoculation with effective rhizobia, leading to enhanced nodulation, BNF, and ultimately yields in Ghana and elsewhere (Ashraf et al., 2006; Sajid et al., 2010; Yakubu et al., 2010; Osei et al., 2020, Grönemeyer et al., 2016). The results of this study corroborate these reports as inoculation with effective native strains particularly KNUST 1002, 1031, and 1003 elicited seed yield increases of Samnut 22 and Chinese varieties at different locations, thus confirming the widely known assertion that most strains are site specific and variety specific (Date, 2000; Woomer et al., 2014). The significant interaction response indicates that the strain performance varied with location and variety. The positive response observed in this study may have been influenced by the superior symbiotic effectiveness of the introduced strains over the indigenous rhizobia population, the low population of native rhizobia before the establishment of the experiment, and the low fertility of soils at the study sites. Buri et al. (2010) indicated that soils in the Savanna zones of Ghana are predominantly low in organic matter, nitrogen, and available P. These low nutrient contents, particularly N (ranging between 0.022 and 0.03%), coupled with the rhizobia population, which was below the threshold reported by Thies et al. (1991) to obviate inoculation response, revealed a need for plant N that was impossible to obtain from the soil by groundnut plants. Hence, introducing effective native strains led to an efficient symbiosis, subsequently making N available for plant growth and increased yields. Mathenge (2017) reported that the potential of legumes to fix nitrogen is likely to be high when the mineral N of the soil is low compared to when soils have higher N levels. The response of groundnut varieties (Chinese and Samnut 22) to inoculated strains, which varied significantly, could be due to the specificity in the interaction between rhizobia and plant genotype (de Alcantara et al., 2014). For instance, inoculation with strain KNUST 1031 enhanced nodulation of the Chinese variety compared to Samnut 22 at Cheshegu. Furthermore, nodulation of the negative control was higher for Samnut 22 than for the Chinese variety. This shows that although the native rhizobia population was low, Samnut 22 was highly compatible with the native soil rhizobia, giving them a competitive advantage over some of the introduced strains. According to Sanginga et al. (2000), genotypes of the same legume may differ in terms of their nodulation and N2 fixation capacities, hence influencing the performance of strain(s). Abi-Ghanem et al. (2011) also reported that crop genotype and its interaction with rhizobia significantly influence nitrogen fixation. This is due to the inherent genetic capacity of a strain. Perret et al. (2000) reported that the specificity between symbiotic partners minimizes the formation of non-fixing nodules by the host plant to enhance N2 fixation.
The fact that introduced rhizobia strains increased nodulation over the native rhizobia population suggests that the introduced strains were probably competitive for nodulation sites. The performance of some of the introduced strains was comparable to the commercial strain, suggesting their potential for use in inoculant formulation. Even though nodulation was higher in this study, it was limited by moisture stress at Tanina as indicated in Supplementary Figure 1C. Moisture stress has also been reported to generally affect nodulation (Bordeleau and Prévost, 1994; Hungria and Vargas, 2000). Moisture stress compromises plant and rhizobia growth and is a major cause of nodulation failure due to its effect on rhizobia survival, growth, and population structure in soil; the formation and longevity of nodules; synthesis of leghemoglobin; and nodule function (O'Hara, 2001).
Our results suggest the potential saprophytic competence abilities of the introduced strain, especially KNUST 1002, as its population was higher compared to other strains after a cropping season of its introduction. Confirming the strain's ability to sustain effectiveness after a cropping season will be a major step toward reducing the number of successive inoculations. This has positive implications for groundnut farmers, as inoculants are rarely available in the remote areas and thus costs are cut down.
The VCR values showed that the strains varied in profitability between groundnut varieties and across locations. Using the threshold of 3–4 indicated by Dittoh et al. (2012), strains KNUST 1002 and 1031 were profitable on Samnut 22 at Cheshegu, while KNUST 1003 was profitable on the Chinese variety and KNUST 1002, 1003, and 1031 were profitable on Samnut 22 at Binduri. Per the threshold, only KNUST 1002 was profitable on Samnut 22 at Tanina. Ronner et al. (2016) and Ulzen et al. (2018) indicated that the use of rhizobia inoculant is financially rewarding for farmers in northern Ghana and northern Nigeria. Thus, this study has demonstrated that not only will the use of the test strains improve yields of groundnut for smallholder farmers under similar environmental conditions but that it will also increase their livelihoods.
Conclusion
The findings of this study support the hypothesis that superior effective strains with saprophytic abilities that can meet the N requirement of groundnut exist in soils of Ghana and can effectively increase seed yields of groundnut. The study has shown the potential of strains KNUST 1002, 1003, and 1031 as possible candidates for use in the commercial production of rhizobia inoculant for Samnut 22 and Chinese varieties in Cheshegu, Binduri, and Tanina as the grain yields produced by these strains were better or comparable to those produced by mineral N-fertilized treatment. Strain KNUST 1002 can persist in the soil for at least one cropping season irrespective of the location. The study also revealed that strains KNUST 1002, 1031, and 1003 were highly profitable under the current environmental conditions of the study. Our results showed that with the same rhizobia strain, genotypes, and management practices, environmental conditions are the most important factors that limit rhizobia efficiency. Long-term studies are required to unravel the mechanisms underlying rhizobia survival under field conditions and the interaction between environmental conditions and rhizobia strains.
Data Availability Statement
The original contributions presented in the study are included in the article/Supplementary Material, further inquiries can be directed to the Corresponding author.
Author Contributions
GW, JU, RA, AO, OO, and SA-N designed the experiment. GW, JU, RA, AO, and OO planned the research activities. GW, JU, and OO conducted the field work, collected and analyzed the data, and drafted the manuscript. RA, AO, and SA-N fine-tuned the manuscript. All authors contributed to the article and approved the submitted version.
Funding
The authors gratefully acknowledge the financial contribution made toward this work by the Bill and Melinda Gates Foundation through a grant (OPP1020032) to the Plant Production Systems Group of Wageningen University and research on the project N2Africa-Putting Nitrogen Fixation to Work for Smallholder Farmers in Africa.
Conflict of Interest
The authors declare that the research was conducted in the absence of any commercial or financial relationships that could be construed as a potential conflict of interest.
Acknowledgments
The authors express their appreciation to Kwame Nkrumah University of Science and Technology for technical and infrastructural assistance.
Supplementary Material
The Supplementary Material for this article can be found online at: https://www.frontiersin.org/articles/10.3389/fagro.2021.653044/full#supplementary-material
Supplementary Figure 1. Rainfall distribution during the cropping season [(A): Cheshegu, (B): Binduri, and (C): Tanina].
References
Abi-Ghanem, R., Carpenter-Boggs, L., and Smith, J. L. (2011). Cultivar effects on nitrogen fixation in peas and lentils. Biol. Fertil. Soils 47, 115–120. doi: 10.1007/s00374-010-0492-6
Ajeigbe, H. A., Waliyar, F., Echekwu, C. A., Kunihya, A., Motagi, B. N., Eniaiyeju, D., et al. (2015). A Farmer's Guide to Profitable Groundnut Production in Nigeria. Telangana: ICRISAT.
Ampomah, O. Y., Ofori-Ayeh, E., Solheim, B., and Svenning, M. M. (2008). Host range, symbiotic effectiveness and nodulation competitiveness of some indigenous cowpea bradyrhizobia isolates from the transitional savanna zone of Ghana. Afr. J. Biotechnol. 7:8.
Ashraf, M., Ahmad, M., and Bakush, H. M. (2006). Efficacy of rhizobia isolates for groundnut inoculation under rain fed conditions. Pak. J. Agri. Sci. 43, 3–4.
Ashworth, A. J., West, C. P., Allen, F. L., Keyser, P. D., Weiss, S. A., Tyler, D. D., et al. (2015). Biologically fixed nitrogen in legume intercropped systems: comparison of nitrogen-difference and nitrogen-15 enrichment techniques. Agron. J. 107, 2419–2430. doi: 10.2134/agronj14.0639
Biswas, B., and Gresshoff, P. M. (2014). The role of symbiotic nitrogen fixation in sustainable production of biofuels. Int. J. Mol. Sci. 15, 7380–7397. doi: 10.3390/ijms15057380
Black, C. A. (1965). Methods of soil analysis: physical and mineralogical properties, including statistics of measurement and sampling. Part 2. Chemical and microbiological properties. Agronomy 9, 1387–1388. doi: 10.2134/agronmonogr9.1
Bordeleau, L., and Prévost, D. (1994). Nodulation and nitrogen fixation in extreme environments. Plant Soil 161, 115–125. doi: 10.1007/BF02183092
Breisinger, C., Diao, X., Thurlow, J., and Al-Hassan, R. M. (2008). Agriculture for Development in Ghana. Available online at: https://www.ifpri.org/publication/agriculture-development-ghana (accessed March 12, 2021).
Bremner, J. M., and Mulvaney, C. (1982). Nitrogen—Total. Methods of soil analysis. Part 2. Chemical and microbiological properties. Agronomy 9, 595–624. doi: 10.2134/agronmonogr9.2.2ed.c31
Broughton, W., and Dilworth, M. (1971). Control of leghaemoglobin synthesis in snake beans. Biochem. J. 125:1075. doi: 10.1042/bj1251075
Buri, M. M., Issaka, R., Fujii, H., and Wakatsuki, T. (2010). Comparison of soil nutrient status of some rice growing environments in the major agro-ecological zones of Ghana. J. Food Agric. Environ. 8, 384–388.
Date, R. A. (2000). Inoculated legumes in cropping systems of the tropics. Field Crops Res. 65,123–136. doi: 10.1016/S0378-4290(99)00082-9
de Alcantara, M. C., Xavier, G. R., Rumjanek, N. G., de Moura Rocha, M., and dos Santos Carvalho, J. (2014). Eficiencia simbiotica de progenitores de cultivares brasileiras de feij? o-caupi. Rev. Ciên Agron 45, 1–9. doi: 10.1590/S1806-66902014000100001
Dittoh, S., Omotosho, O., Belemwire, A., Akuriba, M., and Haider, K. (2012). Improving the effectiveness, efficiency and sustainability of fertilizer use in sub-Saharan Africa. Briefing Paper Number 3. Washington, DC: GDNA Agriculture Policy Series.
Erbas, C. B., and Solakoglu, G. E. (2017). In the presence of climate change, the use of fertilizers and the effect of income on agricultural emissions. Sustainability 9:1989. doi: 10.3390/su9111989
Fehr, W. R., Caviness, C. E., Burmood, D. T., and Pennington, J. S. (1971). Stage of development descriptions for soybeans, Glycine Max (L.) merrill. Crop Sci. 11, 929–931. doi: 10.2135/cropsci1971.0011183X001100060051x
Grönemeyer, J. L., Hurek, T., Bünger, W., and Reinhold-Hurek, B. (2016). Bradyrhizobia vignae sp. nov., a nitrogen-fixing symbiont isolated from effective nodules of Vigna and Arachis. Int. J. Syst. Evol. Microbiol. 66, 62–69. doi: 10.1099/ijsem.0.000674
Helmke, P. A., and Sparks, D. (1996). “Lithium, sodium, potassium, rubidium, and cesium,” in Methods of Soil Analysis Part 3—Chemical Methods, eds D. L. Sparks, A. L. Page, P. A. Helmke, R. H. Loeppert, P. N. Soltanpour, and M. A. Tabatabai (Madison: American Society of Agronomy), 551–574. doi: 10.2136/sssabookser5.3.c19
Hungria, M., and Vargas, M. A. (2000). Environmental factors affecting N2 fixation in grain legumes in the tropics, with an emphasis on Brazil. Field Crops Res. 65, 151–164. doi: 10.1016/S0378-4290(99)00084-2
Kabir, R., Yeasmin, S., Islam, A., and Sarkar, M. R. (2013). Effect of phosphorus, calcium and boron on the growth and yield of groundnut (Arachis hypogea L.). IJBST 5, 51–60.
Kawaka, F., Dida, M. M., Opala, P. A., Ombori, O., Maingi, J., Osoro, N., et al. (2014). Symbiotic efficiency of native rhizobia nodulating common bean (Phaseolus vulgaris L.) in soils of Western Kenya. Int. Sch. Res. Notices 2014:258497. doi: 10.1155/2014/258497
Leite, J., Passos, S. R., Simoes-Araujo, J. L., Rumjanek, N. G., Xavier, G. R., and Zilli, J. E. (2018). Genomic identification and characterization of the elite strains Bradyrhizobium yuanmingense BR 3267 and Bradyrhizobium pachyrhizi BR 3262 recommended for cowpea inoculation in Brazil. Braz. J. Microbiol. 49, 703–713. doi: 10.1016/j.bjm.2017.01.007
Masso, C., Mukhongo, R., Thuita, M., Abaidoo, R., Ulzen, J., Kariuki, G., et al. (2016). “Biological inoculants for sustainable intensification of agriculture in sub-Saharan Africa smallholder farming systems,” in Climate Change and Multi-Dimensional Sustainability in African Agriculture, eds R. Lal, D. Kraybill, D. Hansen, B. Singh, T. Mosogoya, and L. Eik (Cham: Springer), 639–658. doi: 10.1007/978-3-319-41238-2_33
Mathenge, C. N. (2017). Soybean (Glycine Max) Response to Rhizobia Inoculation as Influenced by Soil Nitrogen Levels. Nairobi: Kenyatta University.
Mathu, S., Herrmann, L., Pypers, P., Matiru, V., Mwirichia, R., and Lesueur, D. (2012). Potential of indigenous bradyrhizobia versus commercial inoculants to improve cowpea (Vigna unguiculata L. walp.) and green gram (Vigna radiata L. wilczek.) yields in Kenya. Soil Sci. Plant Nutr. 58, 750–763. doi: 10.1080/00380768.2012.741041
Nelson, D. W., and Sommers, L. E. (1996). “Total carbon, organic carbon, and organic matter,” in Methods of Soil Analysis Part 3—Chemical Methods, eds D. L. Sparks, A. L. Page, P. A. Helmke, R. H. Loeppert, P. N. Soltanpour, M. A. Tabatabai, et al. (Madison: American Society of Agronomy), 961–1010. doi: 10.2136/sssabookser5.3.c34
O'Hara, G. (2001). Nutritional conisolatets on root nodule bacteria affecting symbiotic nitrogen fixation: a review. Aust. J Exp. Agric. 41:417–433. doi: 10.1071/EA00087
Olsen, S. (1982). “Phosphorus,” in Methods of Soil Analysis, Part 2, ed A. L. Page (Wymondham: American Society of Agronomy), 403–430. doi: 10.2134/agronmonogr9.2.2ed.c24
Osei, O., Abaidoo, R. C., Ahiabor, B. D., Boddey, R. M., and Rouws, L. F. (2018). Bacteria related to Bradyrhizobia yuanmingense from Ghana are effective groundnut micro-symbionts. Appl. Soil Ecol. 127, 41–50. doi: 10.1016/j.apsoil.2018.03.003
Osei, O., Abaidoo, R. C., Opoku, A., Rouws, J. R., Boddey, R. M., Ahiabor, B. D., et al. (2020). Native bradyrhizobium strains from Ghana can enhance grain yields of field-grown cowpea and groundnut. Front Agron. 2:2. doi: 10.3389/fagro.2020.00002
Peoples, M. B., and Herridge, D. F. (1990). Nitrogen fixation by legumes in tropical and subtropical agriculture. Adv Agron. 44, 155–223. doi: 10.1016/S0065-2113(08)60822-6
Perret, X., Viprey, V., and Broughton, W. J. (2000). Physical and Genetic Analysis of the Broad Host-Range Rhizobia sp. NGR234. Wymondham: Horizon Scientific Press.
Ronner, E., Franke, A. C., Vanlauwe, B., Dianda, M., Edeh, E., Ukem, B., et al. (2016). Understanding variability in soybean yield and response to P-fertilizer and rhizobia inoculants on farmers' fields in northern Nigeria. Field Crops Res. 186, 133–145. doi: 10.1016/j.fcr.2015.10.023
Roy, R. N., Finck, A., Blair, G. J., and Tandon, H. L. S. (2006). Plant nutrition for food security. A guide for integrated nutrient management. FAO Fertilizer Plant Nutr. Bull. 16:368. doi: 10.1017/S0014479706394537
Sajid, M., Rab, A., Wahid, F., Shah, S., Jan, I., Khan, M., et al. (2010). Influence of rhizobia inoculation on growth and yield of groundnut cultivars. SJA. 27, 573–576.
Sanginga, N., Thottappilly, G., and Dashiell, K. (2000). Effectiveness of rhizobia nodulating recent promiscuous soyabean selections in the moist savanna of Nigeria. Soil Biol. Biochem. 32, 127–133. doi: 10.1016/S0038-0717(99)00143-1
Somasegaran, P., and Hoben, H. J. (2012). Handbook for Rhizobia: Methods in Legume-Rhizobia Technology. Wymondham: Springer Science and Business Media.
Statistics Research and Information Directorate (SRID). (2019). Agriculture in Ghana; Facts and Figures, 2018. Accra: Ministry of Food and Agriculture (MoFA).
Thies, J. E., Singleton, P. W., and Bohlool, B. B. (1991). Influence of the size of indigenous rhizobial populations on establishment and symbiotic performance of introduced rhizobia on field-grown legumes. Appl. Environ. Microbial. 57, 19–28. doi: 10.1128/AEM.57.1.19-28.1991
Thuita, M., Pypers, P., Herrmann, L., Okalebo, R. J., Othieno, C., Muema, E., et al. (2012). Commercial rhizobial inoculants significantly enhance growth and nitrogen fixation of a promiscuous soybean variety in Kenyan soils. Biol. Fertil. Soils 48, 87–96. doi: 10.1007/s00374-011-0611-z
Ulzen, J., Abaidoo, R. C., Masso, C., Owusu-Ansah, E. D. J., and Ewusi-Mensah, N. (2018). Is there a need for Bradyrhizobia yuanmingense and B. japonicum reinoculation in subsequent cropping seasons under smallholder farmers' conditions? Appl. Soil Ecol. 128, 54–60. doi: 10.1016/j.apsoil.2018.04.003
Ulzen, J., Abaidoo, R. C., Mensah, N. E., Masso, C., and AbdelGadir, A. H. (2016). Bradyrhizobia inoculants enhance grain yields of soybean and cowpea in Northern Ghana. Front Plant Sci. 7:1770. doi: 10.3389/fpls.2016.01770
Unkovich, M., and Baldock, J. (2008). Measurement of asymbiotic N2 fixation in Australian agriculture. Soil Biol. Biochem. 40, 2915–2921. doi: 10.1016/j.soilbio.2008.08.021
Viera-Vargas, M. S., Souto, C. M., Urquiaga, S., and Boddey, R. M. (1995). Quantification of the contribution of N2 fixation to tropical forage legumes and transfer to associated grass. Soil Biol. Biochem. 27, 1193–1200. doi: 10.1016/0038-0717(95)00022-7
Vincent, J. M. (1970). A Manual for the Practical Study of the Root-Nodule Bacteria. Wymondham: Wiley.
Woomer, P., Bennett, J., and Yost, R. (1990). Overcoming the inflexibility of most-probable-number procedures. Agron J. 82, 349–353. doi: 10.2134/agronj1990.00021962008200020035x
Woomer, P., Huising, J., Giller, K., Baijukya, F., Kantengwa, S., Vanlauwe, B., et al. (2014). N2Africa Final Report of the First Phase: 2009–2013.
Yakubu, H., Kwari, J., and Ngala, A. (2010). N2 Fixation by grain legume varieties as affected by rhizobia inoculation in the sandy loam soil of Sudano-Sahelian zone of North Eastern Nigeria. NJBAS 18. doi: 10.4314/njbas.v18i2.64325
Keywords: native strains, value cost ratio, biological nitrogen fixation, grain yield, smallholder farmers
Citation: Wilson G, Ulzen J, Abaidoo RC, Opoku A, Adjei-Nsiah S and Osei O (2021) Native Rhizobia Strains Enhance Seed Yield of Groundnut Varieties in Northern Ghana. Front. Agron. 3:653044. doi: 10.3389/fagro.2021.653044
Received: 13 January 2021; Accepted: 06 April 2021;
Published: 14 May 2021.
Edited by:
Magdi T. Abdelhamid, National Research Centre, EgyptReviewed by:
John M. Maingi, Kenyatta University, KenyaRafaela Simão Abrahão Nóbrega, Federal University of the Recôncavo of Bahia, Brazil
El-Sayed Mohamed Desoky, Zagazig University, Egypt
Saad Farouk, Mansoura University, Egypt
Copyright © 2021 Wilson, Ulzen, Abaidoo, Opoku, Adjei-Nsiah and Osei. This is an open-access article distributed under the terms of the Creative Commons Attribution License (CC BY). The use, distribution or reproduction in other forums is permitted, provided the original author(s) and the copyright owner(s) are credited and that the original publication in this journal is cited, in accordance with accepted academic practice. No use, distribution or reproduction is permitted which does not comply with these terms.
*Correspondence: Jacob Ulzen, amFrZXVsemVuQHlhaG9vLmNvbQ==