- 1Department of Plant and Soil Sciences, Mississippi State University, Mississippi State, MS, United States
- 2Department of Crop, Soil, and Environmental Sciences, University of Arkansas, Fayetteville, AR, United States
Rice is a staple food for more than 3.5 billion people worldwide, with Asia producing almost 90% of the global rice yield. In the US, rice is primarily produced in four regions: Arkansas Grand Prairie, Mississippi Delta, Gulf Coast, and Sacramento Valley of California. Arkansas currently accounts for more than 50% of the rice produced in the US. As global temperatures continue to rise and fluctuate, crop-breeding programs must continue to evolve. Unfortunately, sudden submergence due to climate change and unpredictable flash flooding can cause yield reduction up to 100% and affect 20 million ha of agricultural farmlands. Similarly, it has been demonstrated that temperatures higher than 34°C can cause spikelet infertility resulting in up to 60% reduction in yield. One major drawback to developing abiotic stress-tolerant rice is the loss of critical traits such as vegetative vigor, spikelet fertility, and grain quality, which are essential in increasing economic return for farmers. To replace traits lost in past breeding endeavors, weedy rice (WR) has been proposed as a source for novel trait discovery to improve rice breeding programs. Therefore, the goal of this study was to screen and identify heat- and submergence-tolerant WR accessions. A WR mini germplasm consisting of seedlings at the 3–4 leaf stage was exposed to heat (38°C) and complete submergence for 21 days. After each treatment, height was recorded every 7 days for 28 days, and biomass was collected 28 days after treatment. The average height reduction across all accessions was 19 and 21% at 14 and 28 days after treatment (DAT) for the heat-stress treatment. The average height reduction across all accessions was 25 and 33% for the complete submergence stress. The average biomass reduction across all accessions was 18 and 21% for heat and complete submergence stress, respectively. Morphologically, at 28 DAT, 28% of the surviving WR accessions in the heat-stress treatment with <20% height reduction were straw-colored hull types without awns. Under complete submergence stress, 33% of the surviving WR accessions were blackhull types without awns. These specific biotypes may play a role in the increased resilience of WR populations to heat or submergence stress. The results presented in this paper will highlight elite WR accessions that can survive the effects of climate change.
Introduction
Rice, Oryza sativa, is a major food staple and arguably one of the most essential crops feeding close to half of the world's population and providing more than 50% of the daily calories (Muthayya et al., 2014; Kim et al., 2015; Dong and Xiao, 2016). Rice is primarily grown in major river deltas of Asia and Vietnam, and it is a rapidly growing food source in Africa (Kuenzer and Knauer, 2013). Cultivated rice is separated into two species: O. sativa (subspecies japonica orindica) and O. glaberrima, with origins from North Asia, South Asia, and West Africa, respectively (Vaughan et al., 2008). In the US, japonica cultivars are primarily grown in the southern rice belt while indica cultivars are primarily grown California (USDA ERS, 2021). China and India consume more than 50% of the rice produced worldwide, while the US exports more than 4,000 tons of rice annually (USDA ERS, 2021).
In the US, rice yields continue to increase annually due to the adoption of optimum management practices and the introduction and adoption of hybrid rice varieties, but rice production systems still struggle against increased weed pressure and impacts of climate change (Espe et al., 2016; Fargione et al., 2018). Of the significant abiotic stresses, rice can be impacted by salinity, drought, flooding, and high/low temperatures (Hasanuzzaman et al., 2018). In crops such as maize, soybeans, and rice, climate extremes explain 18–43% of the yield variance from year to year (Vogel et al., 2019). In rice, temperatures above 33°C can negatively impact all stages of plant growth and development with temperatures above 37°C causing a complete loss of plants at the reproductive stage (Jagadish et al., 2007; Aghamolki et al., 2014; Kilasi et al., 2018). Temperatures above this threshold are typical in the summer months in the southern US, but US rice is able to withstand these temperatures notwithstanding some yield losses (Tenorio et al., 2013; Chiang et al., 2018).
Although many studies have been conducted to evaluate the effects of heat stress at the reproductive stage, rice's increased sensitivity to heat and the direct correlation to grain yield lead Kilasi et al. (2018) to screen rice seedlings for heat stress tolerance (37°C) as a comparative means to identify genes controlling heat tolerance (2018). By opting to screen at the seedling stage, Kilasi et al. (2018) used the root and shoot growth as a percentage of the control to identify tolerant lines that can be genotyped quickly. Bewley (1997) noted that stored reserves are mobilized during post-germination, radicle cells elongate, cells divide, and DNA is synthesized. These processes happen rapidly and can be considerably affected by heat stress (Kilasi et al., 2018). In the case of complete submergence, Adkins et al. (1990) reported that submergence stress tolerance in rice is associated with the seedling stage as older plants are more tolerant than younger ones. Additionally, most rice plants that are submerged for more than 3 days are severely injured or dead, and few cultivars can survive complete submergence for 10–14 days (Gao et al., 2007). Survival and tolerance of rice cultivars have been associated with semi-dwarf cultivars and suppressed leaf elongation and decreased carbohydrate consumption while submerged (Gao et al., 2007).
In an effort to mitigate the effects of climate change, there has been a shift in research to study genetically similar weed species to uncover stress-tolerant traits to improve crop systems (Barrett, 1983; Ghanizadeh et al., 2019; Hopper et al., 2019). In rice, studies have turned to weedy rice (WR), Oryza spp. (also commonly referred to as red rice or weedy red rice), to understand the evolutionary pathways that lead to increased competitiveness (Olsen et al., 2007). In the US, WR infestations are most prominent in the Arkansas and Mississippi delta leading to a reduction in rice yield by up to 80% (Estorninos et al., 2005). As one of the major weeds in rice, WR can reduce both the quality and quantity of cultivated rice as WR is adapted to rice production agroecology (Jia and Gealy, 2018).
WR has numerous characteristics similar to that of cultivated rice, but the contrasting differences such as increased height, seed shattering, and seed dormancy, coupled with its ability to mimic cultivated rice, make WR challenging to control. At the same time, WR can be a source of genetic variation previously lost through the domestication of cultivated rice (Bevilacqua et al., 2015; Nadir et al., 2017). WR also possesses competitive characteristics to produce more tillers and culms and be taller than rice while accumulating more nitrogen and sucrose, leading to increased biomass production (Kwon et al., 1992; Burgos et al., 2006).
WR grows taller and faster than cultivated rice even when planted deeper, suggesting that it may harbor some traits that could overcome cold stress (Gealy et al., 2000). Research has shown that weedy rice has increased tolerance to cold stress; however, those accessions identified as cold tolerant still exhibited a height reduction of 29–37% compared to non-stressed controls (Bevilacqua et al., 2015). Currently, the US primarily grows japonica rice cultivars, which are more tolerant to cold stress than indica type rice varieties. However, farmers have to adhere to an appropriate rice planting window to ensure good crop stand and crop development (Xu et al., 2008). While japonica rice cultivars are planted in the US, Bevilacqua et al. (2015) determined that more than 90% of WR found in the US is of the indica biotype. Challenges exist in the hybridization of japonica x indica rice; one being the predominance of sterile offspring when breeding for cold tolerance (Borjas et al., 2016). To combat these challenges, it may be useful to cross cold-sensitive indica rice cultivars with cold-tolerant WR biotypes.
WR had also shown tolerance to high salinity during germination, with levels only dipping below 50% germination when salinity was >16 dS m−1 (decisiemens per meter) (Hakim et al., 2011). In the same study by Hakim et al. (2011), WR species exhibited <20% shoot length reduction at 4 dS m−1, and increased shoot lengths were recorded up to 24 dS m−1. WR also boasts broad-spectrum resistance to rice blast disease, with ~18 of the 60 screened WR accessions were resistant to 3–10 blast races (Liu et al., 2015). Of the 18 resistant WR accessions, one accession demonstrated complete resistance to 10 blast races, although the 14 blast races present had a virulence rate of 98.3% (Liu et al., 2015).
Weedy rice exhibit differential tolerance to herbicides. For example, the Arkansas WR germplasm showed 30–100% injury when treated with a commercial rate of glyphosate at 900 g a.e. ha−1(Burgos et al., 2011). Moreover, studies have shown that weedy rice is highly variable, genetically and phenotypically (Gealy et al., 2002; Cao et al., 2006; Burgos et al., 2014; Chen et al., 2014). Burgos et al. (2014) reported seven hull colors in imazethapyr-resistant WR, indicating the phenotypic diversity of WR populations. The same study presented six genetic groups of WR in the surveyed fields. Cao et al. (2006) used AMOVA analysis to confirm that ~35% of total genetic variation existed among WR regions. Using microsatellite markers, an average genetic diversity of 0.63 across 89 weedy rice accessions in Arkansas was reported (Gealy et al., 2002). Studies also point to a significant amount of diversity amongst and within weedy rice populations and between weedy rice and cultivated rice (Ferrero, 2003; Londo and Schaal, 2007; Tseng et al., 2013).
This study aimed to identify heat- and submergence-tolerant WR accessions and characterize the competitive traits of stress-tolerant accessions.
Materials and Methods
Plant Materials
Seeds of 200 different WR accessions were collected from the major rice-growing counties in Arkansas in 2008-09 and grown in a commong garden at the Rice Research and Extension Center, Stuttgart and at the Main Agricultural Research Center, Fayetteville, AR. Various morphological characteristics were evaluated (Tseng et al., 2013) and using these morphological data, 54 weedy rice accessions (Supplementary Table 1) were selected based on desirable competitive traits including height and high tillering. Four rice cultivars specific to the southeastern United States (CRL-Thad, CRL-Rex, CRL-CL163, and CRL-PM), and four rice-breeding lines (RBL) with traits for heat and submergence, were obtained from the Delta Research and Extension Center, Mississippi State University, Stoneville, Mississippi, for inclusion in the study. Each experiment consisted of 54 weedy rice lines (Supplementary Table 1), two rice-breeding lines (heat-stress specific), and three cultivated rice lines. After completing one run with all 54 WR accessions, 10 WR accessions with <20% height reduction and biomass reduction, and five (four in the case of the heat-stress treatment) accessions with >80% height reduction and biomass reduction were selected for runs two and three.
Heat Tolerance Screening
These experiments were conducted in 2016, 2017, and 2019 in the weed physiology laboratory located on the Mississippi State University campus (MS State, MS). Each experiment consisted of three treated replicates and three non-treated controls. Four seeds from each WR accession, rice-breeding-line, and cultivated rice line (CRL) were sown in a 72-well planting tray (Greenhouse Mega Store) containing potting soil (SunGro Professional Growing Mix; 3.8 cu. ft.) in a completely randomized design. Plants were covered with clear domes (Propagation domes; Greenhouse Mega Store) and grown under optimum conditions (28°C /16 h light, 24°C /8 h dark) in a growth chamber (Percival Scientific, Perry, IA) until germination, after which the clear domes were removed. After 21 days, 3–4 leaf stage sized plants were thinned to two plants per well, and plant height was measured. The plants were then transferred to a similar growth chamber set at 38°C and incubated for 21 days with a 16/8 h light/dark cycle. After 21 days, plants were returned to the growth chamber set at optimum growing conditions, and plant height was measured at 14 and 28 days after treatment (DAT). Plant height was measured from the soil surface to the tip of the healthiest, fully emerged leaf. The plants were cut at soil level, oven-dried for 7 days at 50°C, and weighed.
Submergence Tolerance Screening
Submergence experiments were conducted in 2016, 2017, and 2019 in the weed physiology laboratory at the Mississippi State University campus (MS State, MS). The plants were prepared following the same procedure as the heat tolerance experiment. Once emerged, plants were thinned to two plants per well and covered with a modified 72-well tray. Wells were cut from the bottom of the 72-well tray to allow roots to grow through. Twenty-one days after planting, the plants were completely submerged for 21 days within 102 liter (78.4 × 51.5 × 36.9 cm) black totes (Command XL; Lowes). Water levels were maintained at 20–30 cm (submergence depth) above the plant canopy to ensure complete coverage of plants throughout the experiment. After 21 days of submergence, water was drained to maintain 5–10 cm depth in each plastic tote, simulating normal flood conditions. Plant heights were recorded at 0 DAT and every 7 days for 28 days. Plant height was measured from the soil surface to the end of the healthiest, fully emerged leaf blade. After the experiment (28 DAT), plants were cut at the soil level, oven-dried for 7 days at 50°C, and weighed.
Statistical Analysis
Data was collected from a completely randomized design with three replicates and two plants per replicate to evaluate the effects of heat and complete submergence on a WR population. The reduction in plant height was obtained by subtracting the individual heights of the treated plants (HTP) from the height of the control plants (HCP), then dividing by the HCP and multiplying by 100 to obtain a percent (Equation 1). For biomass reduction, Equation (1) was used where height was replaced with biomass of the corresponding plant. Data were analyzed using ANOVA to compare treated WR accessions and corresponding untreated controls, rice-breeding lines, and cultivated rice lines based on individual mean height and biomass reduction. Where factor effect was significant, treatment means were compared Tukey's multiple comparisons test in JMP 14. The LSD values were separated at a 0.05 level of significance. Additionally, the data were subjected to principal component analysis (PCA) with 16 agronomic traits alongside mean height and biomass reduction. K-means clustering with hierarchical clusters was used to separate the WR population into groups based on these traits.
Results and Discussion
Heat Tolerance Screening
Due to the high vigor of WR, seedlings were kept at 38°C for 21 days. Heat stress effect was manifested as reduced plant height and biomass at 14 and 28 DAT. The WR population showed substantial differences in response to sustained heat stress at 38°C during the seedling stage. Approximately 57 and 42% of the 14 WR populations showed 20% or less reduction in plant height at 14 and 28 DAT, respectively. This indicates that severe heat stress could kill rice seedlings. In an experiment with rice mutants, Bahuguna et al. (2015) found that mean temperatures ranging from 36 to 45°C resulted in a loss of 53% of the seedling population within 15 days after transplanting resulting in only eight seedlings surviving the stress (Bahuguna et al., 2015). In the current study, consistent temperatures of 38°C allowed for the survival of 14 WR accessions treated at the 3–4 leaf stage. Selecting a temperature within the range provided by Bahuguna et al. (2015) provided sufficient stress to plants while maintaining a consistent population size. In another study, Kilasi et al. (2018) found that aus variety N22 had ~30% longer root and shoot lengths compared to the heat-sensitive variety IR64 after only being exposed to heat stress (37°C) for 4 days during germination. These results are similar to findings in this study as rice seedlings were just 21 days old before the onset of heat stress.
In this study, plant height reduction ranged from about 1 to 33% at 14 DAT (Figure 1). At 14 DAT, RBL-61 (0.8%) showed the least reduction in height, followed by WR accession S108 (10%) and CRL-CL163 (10%). Rice-breeding line, RBL-61, is a commercial indica type rice from Pakistan often used as a reference for tolerance to heat stress (Pervaiz et al., 2010). RBL-61 is a semi-dwarf variety ( ≤ 110 cm tall) that matures in 116–130 days (Pervaiz et al., 2010). WR accession S108 is a high-yielding, straw-colored, awn less ecotype from Randolph County, Arkansas. The WR accessions were collected from random fields across the rice-producing counties in the Arkansas Delta and were characterized morphologically in a common garden (Tseng et al., 2013). WR accession S9 showed the most height reduction at 33% at 14 DAT. This WR accession was collected from Arkansas County, Arkansas, and is a straw hull, awn less ecotype. Poli et al. (2013) also reported stunting of heat-stressed rice “N22.” Findings showed that plant height increased by only 5% although the current study resulted in few plants with decreased height but the 3-week heat stress treatment (Poli et al., 2013).
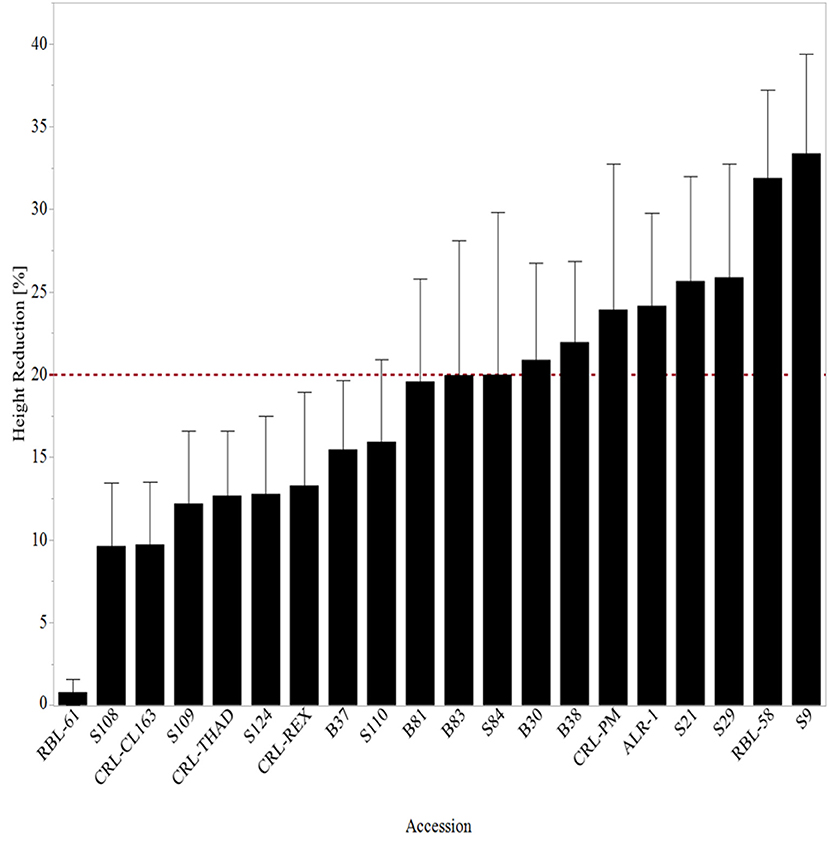
Figure 1. Mean height reduction 14 days after heat stress treatment arranged from lowest reduction to the highest reduction in height. The line at 20% represents all lines with ≥20% reduction in height. RBL, Rice breeding line; CRL, cultivated rice line.
At 28 DAT, mean plant height reduction across cultivars and WR accessions ranged from 6 to 41% (Figure 2). This increased stunting with time is a manifestation of long-term effects of heat stress, especially reduced photosynthetic processes, reduced cell growth, and even cell death. Injury and cell death due to extreme heat stress can occur within a few minutes of exposure to heat and is characterized by foliar senescence and inhibition of root and shoot growth (Nievola et al., 2017). In our study, WR accession S108 had the least reduction in height (6%), while CRL-PM was most stunted (41%). As previously mentioned, WR accession S108 showed significant recovery and continued to grow taller after being exposed to heat stress for 21 days. The height reduction for S108 was 10% at 14 DAT compared to the control, which decreased to 6% at 28 DAT. Severe heat stress can also reduce panicle length, contributing to yield loss. Research by Wu et al. (2016) showed that high-temperature stress reduced the length of exerted panicles by ~60%.
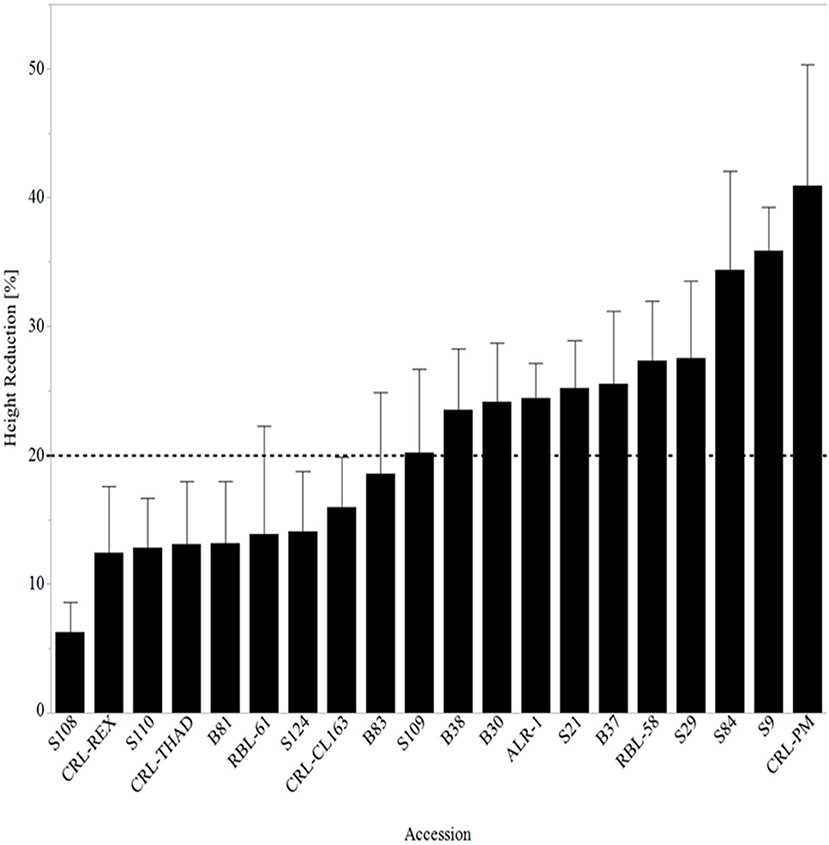
Figure 2. Mean height reduction 28 days after heat stress treatment arranged from lowest reduction to the highest reduction in height. The line at 20% represents all lines with ≥20% reduction in height. RBL, Rice breeding line; CRL, cultivated rice line.
After 21 days of exposure to 38°C temperatures, the mean biomass reduction ranged from 0 to 55%. Rice is a grain crop that has demonstrated the ability to continue to grow new leaves after the senescence of older leaves (Inada et al., 1999). Extended leaf exposure to high temperatures leads to the degradation of chloroplasts and the rapid decline of soluble proteins and chlorophyll (Inada et al., 1999). Considering this cycle of production and loss of proteins and chlorophyll under heat stress, some WR plants demonstrated the ability to continue producing biomass even after prolonged exposure to high temperatures. WR accessions B83 and S124 did not show biomass reduction whereas RBL-58 lost 55% of biomass after the 3-wk heat stress (Figure 3). Interestingly, CRL-PM had the highest height reduction (38%) at 28 DAT but had no biomass reduction at 28 DAT. CRL-PM is commonly used in breeding programs to visually distinguish cross changes in early generation breeding programs (Gravois et al., 2005). This line lodges readily and has high degree of seed dormancy, but has been used as the parental line in many breeding efforts from the early 90's to 2000's (Roche et al., 2005).
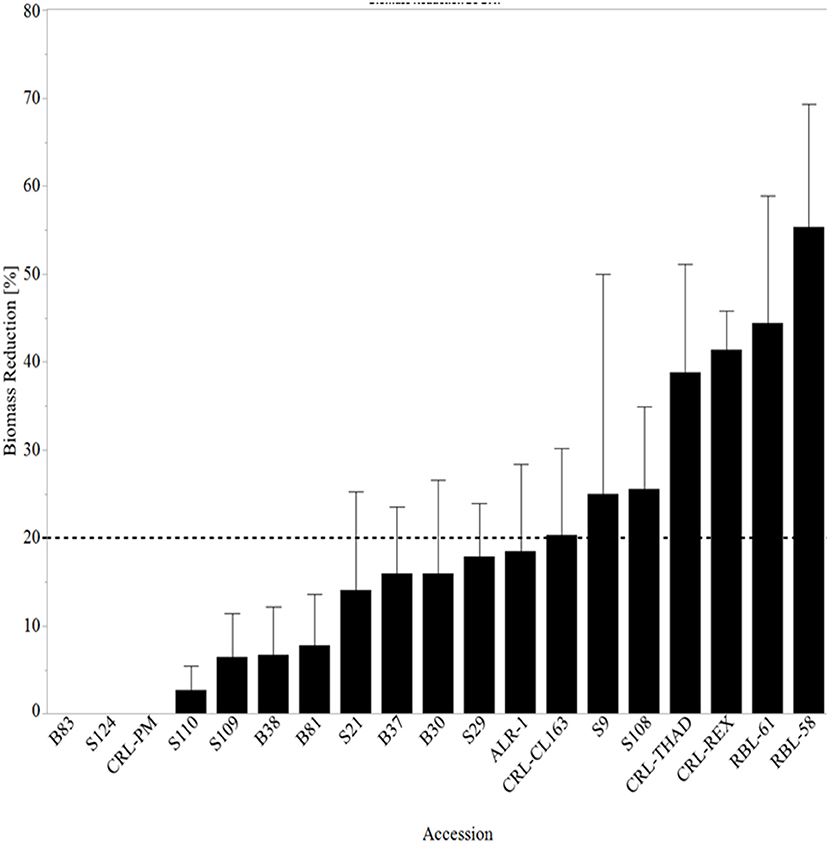
Figure 3. Mean biomass reduction 28 days after heat stress treatment arranged from lowest reduction to the highest biomass reduction. The line at 20% represents all lines with ≥20% reduction in height. RBL, Rice breeding line; CRL, cultivated rice line.
Yoshida (1981) identified the threshold temperature for rice seedlings to be 35°C. Heat stress is characterized by irreversible damage that impacts plant growth and activity (Xiao et al., 2011). Reduced growth due to severe heat stress had been reported before, causing up to 90% height reduction (Yoshida, 1981). In this study, three lines (BKN6624-46-2, IR7478-2-6, and N22) were stunted and showed 90–100% reduction in spikelet fertility at temperatures from 35 to 31°C (Yoshida, 1981). At temperatures just above 25°C, both spikelet number and tiller number declined with further increase in temperature (Yoshida, 1973). Heat stress (38°C) during flowering time can cause 50–80% reduction in spikelet fertility (Cheng et al., 2012). A study of rice mutants using crosses between heat-sensitive, high-yielding rice variety, IR64, and drought- and heat-tolerant mutant, aus variety, N22, found that mutants had a 13% increase in plant height at 38°C when compared to parents (Poli et al., 2013). Increases in plant height can increase the transpiration cooling effect seen in many plants under heat stress (Weerakoon et al., 2008; Hasanuzzaman et al., 2013). Similar results may be valid in heat-tolerant WR as two (2) WR lines had no height reduction at 28 DAT. In this study, height reduction was a significant focus as plant height is a critical characteristic for breeding rice cultivars with increased yield (Jeng et al., 2012).
To further assess the variability and performance of those lines with low and high height and biomass reduction, a principal component analysis (PCA) was employed using hierarchical clustering (Tseng, 2013). In the PCA, 16 agronomic traits were used to access the variability captured in components 1 and 2. The PCA revealed that 36% variation in heat-stress-tolerant WR accessions was attributed to component 1 compared to just 23% in component 2 (Figure 4). When PCA was paired with hierarchical cluster analysis, WR accessions S108 and S109 formed an independent group (Group 1) associated with leaf width (mean = 1.4 cm). These leaves are wider than the population average of 1.26 cm. While WR accession S108 did have a reduction in biomass 28 DAT, the accession consistently had <20% reduction in height at 14 and 28 DAT. Group 1 is also comprised of WR plants with straw-colored hulls without awns. A second grouping (Group 2) consisting of accessions B30, B37, and B38 had above average measurements in flowering time (mean = 105 days), grain length (mean = 6 cm), and culm length (mean = 129 cm), but the PCA reveals that these agronomic traits are not associated with heat-stress tolerance. These selected agronomic traits are associated with increased plant height while culm length is directly related to plant height (Jeng et al., 2012). Additionally, agronomic associations can be made related to increased ligule length, panicle length, and leaf length in similar PCA planes. Similar results are observed in Arkansas weedy rice as PCA attributes hull color as the primary trait separating the population (Kanapeckas et al., 2018).
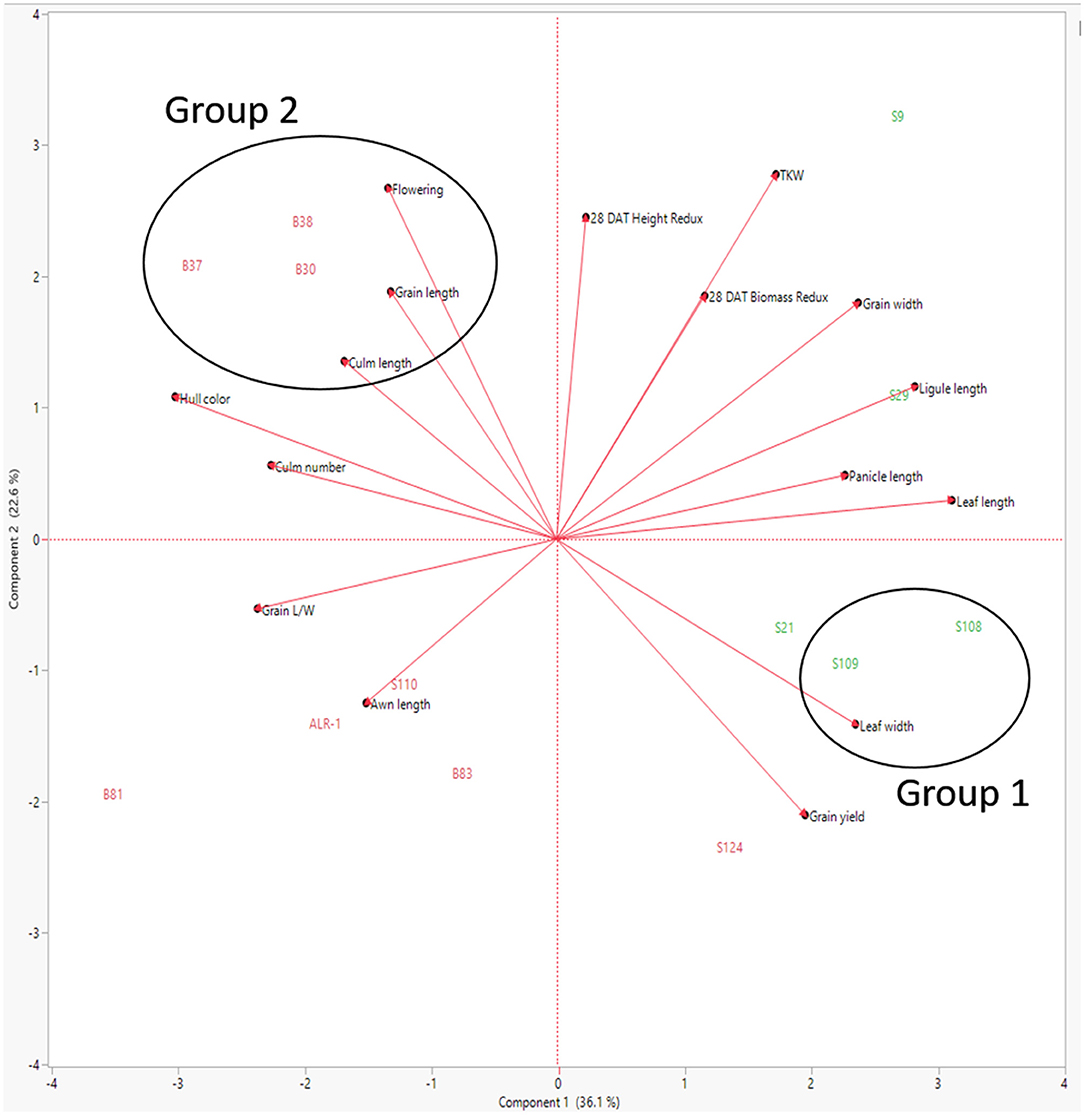
Figure 4. Principal component analysis (PCA) of 16 agronomic traits, including plant height reduction and plant biomass reduction 28 days after heat stress treatment.
Submergence Tolerance Screening
At 14 DAT, ~33% (five out of 15 WR accessions) of the WR accessions had no height reduction, while 53% (eight out of 15 WR accessions) of WR had <20% height reduction (Figure 5). The accessions with no height reduction, S21, B38, B45, B49, and B51, are predominantly black-pericarp, awned WR accessions. Although Setter and Laureles (1996) found a negative correlation between shoot elongation and plant survival, stating that increased elongation in flooded rice was characteristic of low surviving seedlings after exposure to complete submergence for 14 days, the effects have not been studied in WR. In this study, varying effects were observed where those accessions that had no reduction in height continued to survive the effects of submergence stress 28 DAT. This trend was observed at 7 and 14 DAT, but at 28 DAT, 40% of the WR had <20% reduction in height, but no WR accessions had no height reduction (Figure 6). Moreover, it is worth noting that those lines that had <20% height reduction at 14 DAT, surviving weedy rice accessions seemed to recover after de-submergence and maintain <20% height reduction at 28 DAT while CRL-Rex and RBL-60 remained stunted at 28 DAT.
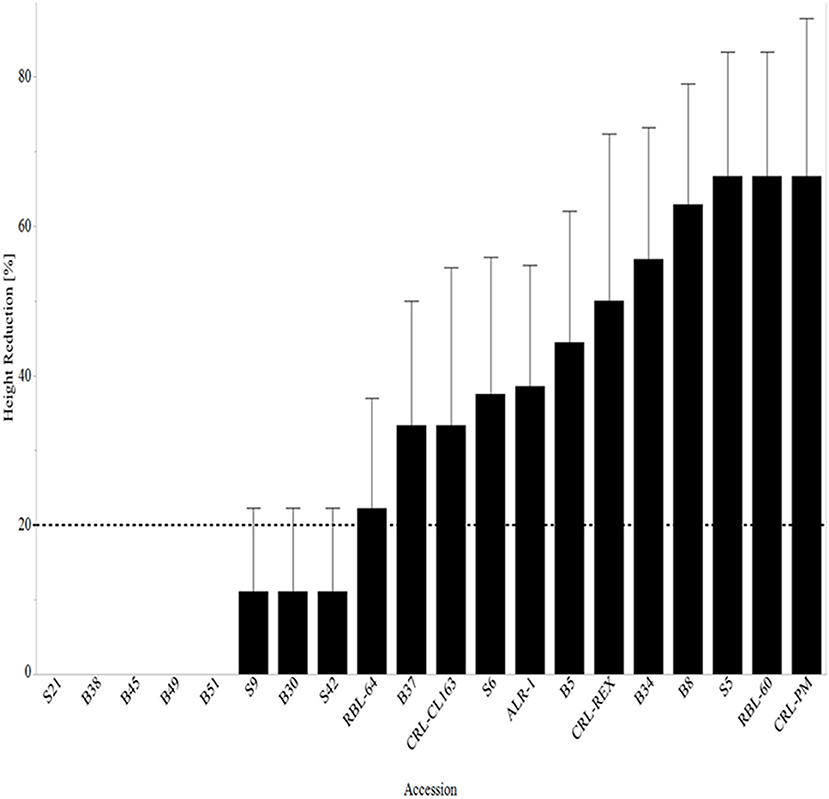
Figure 5. Mean height reduction 14 days after complete submergence stress treatment arranged from lowest reduction to the highest reduction in height. The line at 20% represents all lines with ≥20% reduction in height. RBL, Rice breeding line; CRL, cultivated rice line.
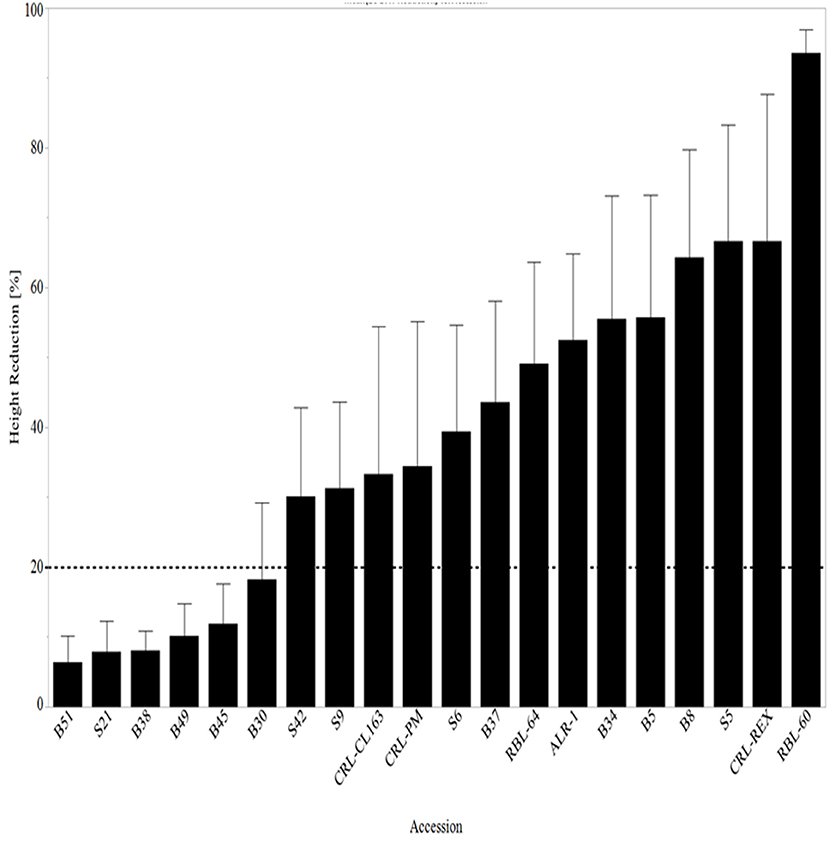
Figure 6. Mean height reduction 28 days after complete submergence stress treatment arranged from lowest reduction to the highest reduction in height. The line at 20% represents all lines with ≥20% reduction in height. RBL, Rice breeding line; CRL, cultivated rice line.
Unsurprisingly, when these WR accessions were compared to cultivated rice lines and rice breeding lines, CRL-REX and RBL-60 had more than 70% height reduction 28 DAT. It is worth mentioning that tolerant rice cultivars are commonly known to suppress leaf elongation and maintain higher carbohydrate levels under complete submergence, but it is unknown if this is true about its weedy counterpart (Gao et al., 2007). This ability may account for the increase in height reduction compared to untreated controls. CRL-REX is a Mississippi developed, conventional, semi-dwarf cultivar with excellent yield performance, good straw strength, milling, and stand ability (Solomon et al., 2012). CRL-REX is also known to be susceptible to rotten neck blast and sheath blight disease (Hardke et al., 2013). Disease susceptibility could also be why increased height reduction in this rice cultivar as flooded waters can contribute to an increase in these diseases. RBL-60 is a submergence-tolerant rice breeding line developed at the Delta Research and Extension Center in Stoneville, MS. RBL-60 is a derivative of IR49830 developed as a converted mega variety with the Sub1 gene to increase submergence stress tolerance (Septiningsih et al., 2009).
At 28 DAT, 27% of WR accessions (S21, B30, B38, and B45) did not show biomass reduction while 67% of accessions had <20% reduction in biomass (Figure 7). Three of the four accessions with no biomass reduction were awned blackhull types. In submergence-tolerant rice, biomass increased by 60% compared to sensitive rice after exposure to complete submergence for 10 days (Das et al., 2009). Das et al. (2009) also found that biomass reduction (23%) was only observed when silt was introduced into the flooding environment suggesting that silt has a negative impact on plant biomass.
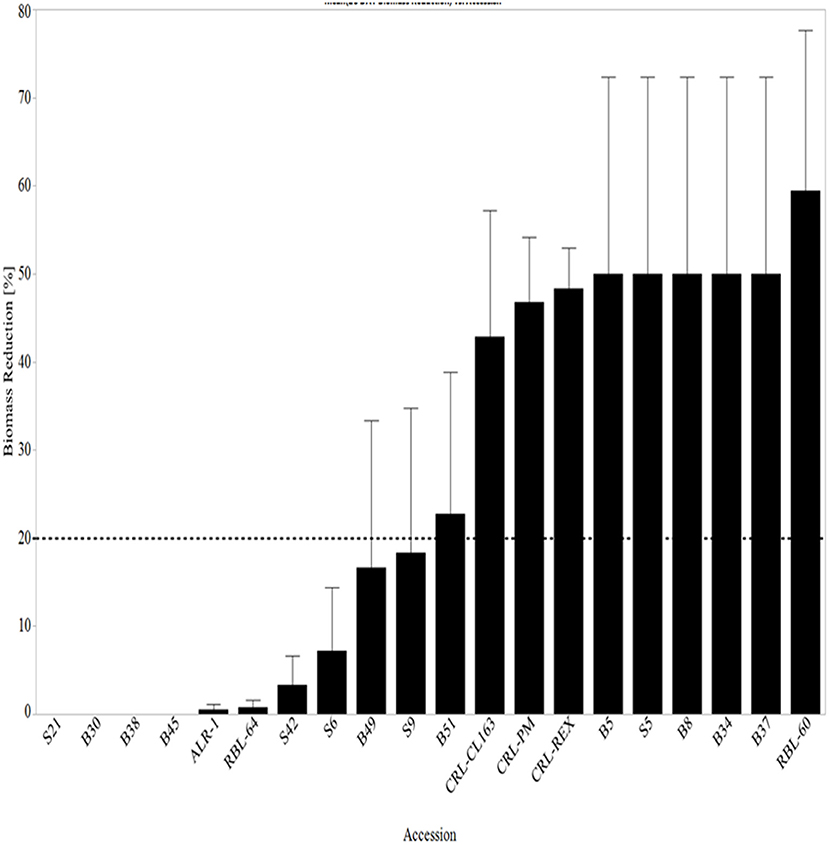
Figure 7. Mean biomass reduction 28 days after complete submergence stress treatment arranged from lowest reduction to the highest biomass reduction. The line at 20% represents all lines with ≥20% reduction in height. RBL, Rice breeding line; CRL, cultivated rice line.
Although rice is well-known for withstanding flooded conditions due to its ability to germinate without CO2 and aerobically escape slow rising waters, limitations still arise in rice's ability to escape and survive sudden and complete submergence due to flash flooding (Jackson and Ram, 2003). Severe injury has been reported when rice coleoptiles, leaves, or stems cannot escape flooding conditions, resulting in sustained complete submergence (Pearce and Jackson, 1991). In instances of sudden flooding, it has been reported that rice height can increase by 25 cm per day, but drastic increases in water levels can reduce plant survival (Vergara et al., 1976). Rice seedlings have demonstrated the ability to survive complete submergence for up to 20 days, but seedling survival rate strongly depends on the age of seedlings and the growth stage at the time of submergence (Richharia and Parasuram, 1963). Kotera and Nawata (2007) reported ≥60% yield loss when complete submergence occurred 17 days after transplanting. Although plants were completely submerged for only 2 days, yield loss was high (Kotera and Nawata, 2007).
While many studies have been performed to assess rice survival rate and performance when exposed to complete submergence stress at the seedling stage, few studies have evaluated the WR performance under submergence at the seedling stage. In our study, many WR seedlings died after 21 d of submergence. Those that did survive were significantly stunted and incurred severe loss of biomass at 14 and 28 DAT. The survivors showed clear signs of exposure to long duration of complete submergence stress such as chlorosis, reduced leaf extension and growth resulting in loss of biomass. In addition, submergence survivors produced more ethylene and had reduced respiration (Jackson et al., 1987).
The PCA and hierarchal clustering of WR accessions using 16 agronomic traits in response to complete submergence stress produced two distinct clusters (Figure 8). Cluster 1 was comprised of WR accessions with more than 20% height and biomass reduction 28 DAT, while cluster 2 consisted of accessions with <20% height and biomass reduction 28 DAT. Component 1 explained 28% of the variation in the data, while component 2 captured 22%. Because rice is known to escape complete submergence with decreased survival rates, agronomic traits such as awn length and grain length/weight may not be significant indicators of submergence stress tolerance. Submergence stress-tolerant WR accessions had increased culm length (mean = 134 cm) and grain yield (mean = 97 g), suggesting that these can be agronomic indicators of submergence stress tolerance.
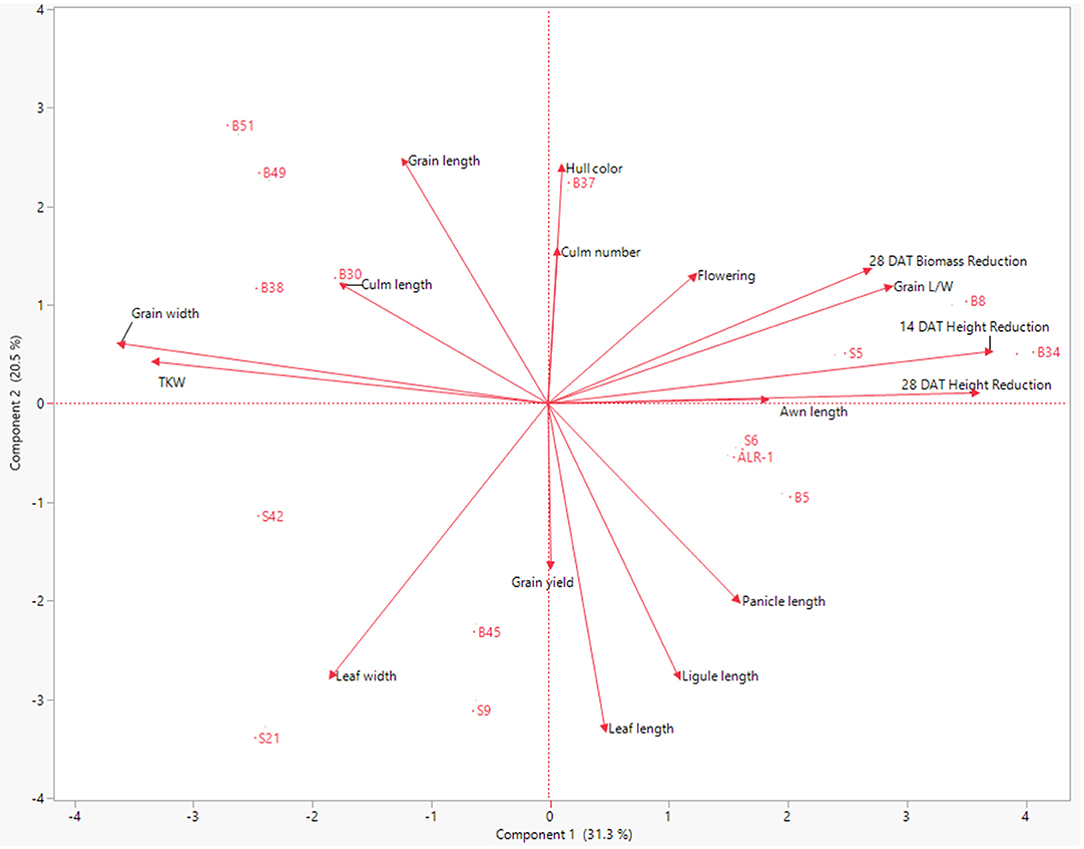
Figure 8. Principal component analysis (PCA) of 16 agronomic traits plus plant height reduction 28 days after complete submergence stress treatment and plant biomass reduction 28 days after complete submergence stress treatment.
Conclusion
This study aimed to highlight the increased competitiveness of a diverse WR population compared to cultivated rice lines and developmental rice breeding lines. By analyzing plant height and biomass reduction and associations of agronomic traits, we can see why weedy rice continues to be a challenge in rice production in the US and elsewhere. Some WR accessions, such as S21 and B28, were more tolerant to heat and submergence stress than the reference tolerant rice cultivar. Given their survival to both heat and submergence-stress, these lines may be useful in the discovery of abiotic stress tolerant traits.
Data Availability Statement
The original contributions presented in the study are included in the article/Supplementary Material, further inquiries can be directed to the corresponding author/s.
Author Contributions
All authors equally contributed to the article and approved the submitted version.
Funding
Funding for this project was provided by the Special Research Initiative Grant sponsored by the Mississippi Agricultural and Forestry Experiment Station. It is based upon work supported by the National Institute of Food and Agriculture, US Department of Agriculture, Hatch project under accession number 230060.
Conflict of Interest
The authors declare that the research was conducted in the absence of any commercial or financial relationships that could be construed as a potential conflict of interest.
Acknowledgments
We acknowledge the Delta Research and Extension Center for kindly providing the use of developmental rice breeding lines to be evaluated in this study. We also acknowledge the collaboration with the University of Arkansas for graciously providing supplemental research information for the selection of tested accessions.
Supplementary Material
The Supplementary Material for this article can be found online at: https://www.frontiersin.org/articles/10.3389/fagro.2021.642335/full#supplementary-material
References
Adkins, S. W., Shiraishi, T., and McComb, J. A. (1990). Submergence tolerance of rice–a new glasshouse method for the experimental submergence of plants. Physiol. Plantarum 80, 642–646. doi: 10.1111/j.1399-3054.1990.tb05691.x
Aghamolki, M. T. K., Yusop, M. K., Oad, F. C., Zakikhani, H., Jaafar, H. Z., Kharidah, S., et al. (2014). Heat stress effects on yield parameters of selected rice cultivars at reproductive growth stages. J. Food Agric. Environ 12, 741–746.
Bahuguna, R. N., Jha, J., Pal, M., Shah, D., Lawas, L. M., Khetarpal, S., et al. (2015). Physiological and biochemical characterization of NERICA-L-44: a novel source of heat tolerance at the vegetative and reproductive stages in rice. Physiol. Plantarum 154, 543–559. doi: 10.1111/ppl.12299
Bevilacqua, C. B., Basu, S., Pereira, A., Tseng, T. M., Zimmer, P. D., and Burgos, N. R. (2015). Analysis of stress-responsive gene expression in cultivated and weedy rice differing in cold stress tolerance. PLoS ONE 10:e0132100. doi: 10.1371/journal.pone.0132100
Bewley, J. D. (1997). Seed germination and dormancy. The Plant Cell 9:1055. doi: 10.1105/tpc.9.7.1055
Borjas, A. H., De Leon, T. B., and Subudhi, P. K. (2016). Genetic analysis of germinating ability and seedling vigor under cold stress in US weedy rice. Euphytica 208, 251–264. doi: 10.1007/s10681-015-1584-z
Burgos, N. R., Norman, R. J., Gealy, D. R., and Black, H. (2006). Competitive N uptake between rice and weedy rice. Field Crops Res. 99, 96–105. doi: 10.1016/j.fcr.2006.03.009
Burgos, N. R., Shivrain, V. K., Scott, R. C., Mauromoustakos, A., Kuk, Y. I., Sales, M. A., et al. (2011). Differential tolerance of weedy red rice (Oryza sativa L.) from Arkansas, USA to glyphosate. Crop Protection 30, 986–994. doi: 10.1016/j.cropro.2011.03.024
Burgos, N. R., Singh, V., Tseng, T. M., Black, H., Young, N. D., Huang, Z., et al. (2014). The impact of herbicide-resistant rice technology on phenotypic diversity and population structure of United States weedy rice. Plant Physiol. 166, 1208–1220. doi: 10.1104/pp.114.242719
Cao, Q., Lu, B. R., Xia, H. U. I., Rong, J., Sala, F., Spada, A., et al. (2006). Genetic diversity and origin of weedy rice (Oryza sativa f. spontanea) populations found in north-eastern China revealed by simple sequence repeat (SSR) markers. Ann. Bot. 98, 1241–1252. doi: 10.1093/aob/mcl210
Chen, H., Xie, W., He, H., Yu, H., Chen, W., Li, J., et al. (2014). A high-density SNP genotyping array for rice biology and molecular breeding. Mol. Plant 7, 541–553. doi: 10.1093/mp/sst135
Cheng, L. R., Wang, J. M., Uzokwe, V., Meng, L. J., Wang, Y., Sun, Y., et al. (2012). Genetic analysis of cold tolerance at seedling stage and heat tolerance at anthesis in rice (Oryza sativa L.). J. Integr. Agri. 11, 359–367. doi: 10.1016/S2095-3119(12)60020-3
Chiang, F., Mazdiyasni, O., and AghaKouchak, A. (2018). Amplified warming of droughts in southern United States in observations and model simulations. Sci. Adv. 4:eaat2380. doi: 10.1126/sciadv.aat2380
Das, K. K., Panda, D., Sarkar, R. K., Reddy, J. N., and Ismail, A. M. (2009). Submergence tolerance in relation to variable floodwater conditions in rice. Environ. Exp. Bot. 66, 425–434. doi: 10.1016/j.envexpbot.2009.02.015
Dong, J., and Xiao, X. (2016). Evolution of regional to global paddy rice mapping methods: a review. ISPRS J. Photogram. Remote Sens. 119, 214–227. doi: 10.1016/j.isprsjprs.2016.05.010
Espe, M. B., Cassman, K. G., Yang, H., Guilpart, N., Grassini, P., Van Wart, J., et al. (2016). Yield gap analysis of US rice production systems shows opportunities for improvement. Field Crops Res. 196, 276–283. doi: 10.1016/j.fcr.2016.07.011
Estorninos, L. E., Gealy, D. R., Gbur, E. E., Talbert, R. E., and McClelland, M. R. (2005). Rice and red rice interference. II. Rice response to population densities of three red rice (Oryza sativa) ecotypes. Weed Sci. 53, 683–689. doi: 10.1614/WS-04-040R1.1
Fargione, J. E., Bassett, S., Boucher, T., Bridgham, S. D., Conant, R. T., Cook-Patton, S. C., et al. (2018). Natural climate solutions for the United States. Sci. Adv. 4:eaat1869. doi: 10.1126/sciadv.aat1869
Ferrero, A. (2003). “Weedy rice, biological features and control,” in Weed Management for Developing Countries, Plant Production and Protection Paper, eds R. Labrada, and I. Addendum (Rome: FAO), 89–109.
Gao, J. P., Chao, D. Y., and Lin, H. X. (2007). Understanding abiotic stress tolerance mechanisms: recent studies on stress response in rice. J. Integr. Plant Biol. 49, 742–750. doi: 10.1111/j.1744-7909.2007.00495.x
Gealy, D. R., Saldain, N. E., and Talbert, R. E. (2000). Emergence of red rice (Oryza sativa) ecotypes under dry-seeded rice (Oryza sativa) culture. Weed Technol. 14, 406–412. doi: 10.1614/0890-037X(2000)0140406:EORROS2.0.CO
Gealy, D. R., Tai, T. H., and Sneller, C. H. (2002). Identification of red rice, rice, and hybrid populations using microsatellite markers. Weed Sci. 2002, 333–339. doi: 10.1614/0043-1745(2002)0500333:IORRRA2.0.CO
Ghanizadeh, H., Buddenhagen, C. E., Harrington, K. C., and James, T. K. (2019). The genetic inheritance of herbicide resistance in weeds. Crit. Rev. Plant Sci. 38, 295–312. doi: 10.1080/07352689.2019.1665769
Gravois, K. A., Moldenhauer, K. A. K., and Lee, F. N. (2005). Registration of “improved purple marker” rice germplasm. Crop Sci. 45, 1164–1165. doi: 10.2135/cropsci2004.0364CV
Hakim, M. A., Juraimi, A. S., Hanafi, M. M., Selamat, A., Ismail, M. R., and Karim, S. R. (2011). Studies on seed germination and growth in weed species of rice field under salinity stress. J. Environ. Biol. 32:529.
Hardke, J., Moldenhauer, K., and Sha, X. (2013). “Rice cultivars and seed production,” in Rice Production Handbook. Publication MP192, ed J. T. Hardke (Little Rock, AR: University of Arkansas Division of Agriculture), 21–28.
Hasanuzzaman, M., Fujita, M., Nahar, K., and Biswas, J. K. (2018). Advances in Rice Research for Abiotic Stress Tolerance. Cambridge, MA: Woodhead Publishing.
Hasanuzzaman, M., Nahar, K., Alam, M., Roychowdhury, R., and Fujita, M. (2013). Physiological, biochemical, and molecular mechanisms of heat stress tolerance in plants. Int. J. Mol. Sci. 14, 9643–9684. doi: 10.3390/ijms14059643
Hopper, J. V., McCue, K. F., Pratt, P. D., Duchesne, P., Grosholz, E. D., and Hufbauer, R. A. (2019). Into the weeds: matching importation history to genetic consequences and pathways in two widely used biological control agents. Evolution. Applicat. 12, 773–790. doi: 10.1111/eva.12755
Inada, N., Sakai, A., Kuroiwa, H., and Kuroiwa, T. (1999). Senescence program in rice (Oryza sautiva L.) leaves: analysis of the blade of the second leaf at the tissue and cellular levels. Protoplasma 207, 222–232. doi: 10.1007/BF01283003
Jackson, M. B., and Ram, P. C. (2003). Physiological and molecular basis of susceptibility and tolerance of rice plants to complete submergence. Ann. Bot. 91, 227–241. doi: 10.1093/aob/mcf242
Jackson, M. B., Waters, I., Setter, T., and Greenway, H. (1987). Injury to rice plants caused by complete submergence; a contribution by ethylerie (ethene). J. Exp. Bot. 38, 1826–1838. doi: 10.1093/jxb/38.11.1826
Jagadish, S. K., Craufurd, P. Q., and Wheeler, T. R. (2007). High temperature stress and spikelet fertility in rice (Oryza sativa L.). J. Exp. Bot. 58, 1627–1635. doi: 10.1093/jxb/erm003
Jeng, T. L., Lai, C. C., Ho, P. T., Shih, Y. J., and Sung, J. M. (2012). Agronomic, molecular and antioxidative characterization of red-and purple-pericarp rice (Oryza sativa L.) mutants in Taiwan. J. Cereal Sci. 56, 425–431. doi: 10.1016/j.jcs.2012.05.015
Jia, Y., and Gealy, D. (2018). Weedy red rice has novel sources of resistance to biotic stress. Crop J. 6, 443–450. doi: 10.1016/j.cj.2018.07.001
Kanapeckas, K. L., Tseng, T. M., Vigueira, C. C., Ortiz, A., Bridges, W. C., Burgos, et al. (2018). Contrasting patterns of variation in weedy traits and unique crop features in divergent populations of US weedy rice (Oryza sativa sp.) in Arkansas and California. Pest Manag. Sci. 74, 1404–1415. doi: 10.1002/ps.4820
Kilasi, N. L., Singh, J., Vallejos, C. E., Ye, C., Jagadish, S. V., Kusolwa, P., et al. (2018). Heat stress tolerance in rice (Oryza sativa L.): identification of quantitative trait loci and candidate genes for seedling growth under heat stress. Front. Plant Sci. 9:1578. doi: 10.3389/fpls.2018.01578
Kim, K., Lee, S. C., Lee, J., Yu, Y., Yang, K., Choi, B. S., et al. (2015). Complete chloroplast and ribosomal sequences for 30 accessions elucidate evolution of Oryza AA genome species. Sci. Rep. 5, 1–13. doi: 10.1038/srep15655
Kotera, A., and Nawata, E. (2007). Role of plant height in the submergence tolerance of rice: a simulation analysis using an empirical model. Agri. Water Manag. 89, 49–58. doi: 10.1016/j.agwat.2006.12.002
Kuenzer, C., and Knauer, K. (2013). Remote sensing of rice crop areas. Int. J. Remote Sens. 34, 2101–2139. doi: 10.1080/01431161.2012.738946
Kwon, S. L., Smith Jr, R. J., and Talbert, R. E. (1992). Comparative growth and development of red rice (Oryza sativa) and rice (O. sativa). Weed Sci. 1992, 57–62. doi: 10.1017/S0043174500056952
Liu, Y., Qi, X., Gealy, D. R., Olsen, K. M., Caicedo, A. L., and Jia, Y. (2015). QTL analysis for resistance to blast disease in US weedy rice. Mol. Plant Microbe Interact. 28, 834–844. doi: 10.1094/MPMI-12-14-0386-R
Londo, J. P., and Schaal, B. A. (2007). Origins and population genetics of weedy red rice in the USA. Mol. Ecol. 16, 4523–4535. doi: 10.1111/j.1365-294X.2007.03489.x
Muthayya, S., Sugimoto, J. D., Montgomery, S., and Maberly, G. F. (2014). An overview of global rice production, supply, trade, and consumption. Ann. N. Y. Acad. Sci. 1324, 7–14. doi: 10.1111/nyas.12540
Nadir, S., Xiong, H. B., Zhu, Q., Zhang, X. L., Xu, H. Y., Li, J., et al. (2017). Weedy rice in sustainable rice production. A review. Agron. Sustain. Dev. 37, 1–14. doi: 10.1007/s13593-017-0456-4
Nievola, C. C., Carvalho, C. P., Carvalho, V., and Rodrigues, E. (2017). Rapid responses of plants to temperature changes. Temperature 4, 371–405. doi: 10.1080/23328940.2017.1377812
Olsen, K. M., Caicedo, A. L., and Jia, Y. (2007). Evolutionary genomics of weedy rice in the USA. J. Integr. Plant Biol. 49, 811–816. doi: 10.1111/j.1744-7909.2007.00506.x
Pearce, D. M., and Jackson, M. B. (1991). Comparison of growth responses of barnyard grass (Echinochloa oryzoides) and rice (Oryza sativa) to submergence, ethylene, carbon dioxide and oxygen shortage. Ann. Bot. 68, 201–209. doi: 10.1093/oxfordjournals.aob.a088245
Pervaiz, Z. H., Rabbani, M. A., Khaliq, I., Pearce, S. R., and Malik, S. A. (2010). Genetic diversity associated with agronomic traits using microsatellite markers in Pakistani rice landraces. Electr. J. Biotechnol. 13, 4–5. doi: 10.2225/vol13-issue3-fulltext-5
Poli, Y., Basava, R. K., Panigrahy, M., Vinukonda, V. P., Dokula, N. R., Voleti, S. R., et al. (2013). Characterization of a Nagina22 rice mutant for heat tolerance and mapping of yield traits. Rice 6, 1–9. doi: 10.1186/1939-8433-6-36
Richharia, R. H., and Parasuram, N. A. (1963). Resistance to submergence in rice. Sci. Cult. 29, 149–150.
Roche, D., Hole, D. J., Albrechtsen, R. S., Clawson, S. M., and Young, S. A. (2005). Registrations of cultivars. Crop Sci. 45:236. doi: 10.2135/cropsci2004.0236CV
Septiningsih, E. M., Pamplona, A. M., Sanchez, D. L., Neeraja, C. N., Vergara, G. V., Heuer, S., et al. (2009). Development of submergence-tolerant rice cultivars: the Sub1 locus and beyond. Ann. Bot. 103, 151–160. doi: 10.1093/aob/mcn206
Setter, T. L., and Laureles, E. V. (1996). The beneficial effect of reduced elongation growth on submergence tolerance of rice. J. Exp. Bot. 47, 1551–1559. doi: 10.1093/jxb/47.10.1551
Solomon, W. L., Kanter, D. G., Walker, T. W., Baird, G. E. III., Scheffler, B. E., Lanford, L. S., et al. (2012). Registration of “rex” Southern long-grain rice. J. Plant Registr. 6, 27–30. doi: 10.3198/jpr2011.04.0230crc
Tenorio, F. A., Ye, C., Redoña, E., Sierra, S., Laza, M., and Argayoso, M. A. (2013). Screening rice genetic resources for heat tolerance. Sabrao J. Breed. Genet. 45, 371–381. doi: 10.1186/s12863-015-0199-7
Tseng, T. M. (2013). Genetic diversity of seed dormancy and molecular evolution of weedy red rice (Theses and Dissertations). Retrieved from: https://scholarworks.uark.edu/etd/595
Tseng, T. M., Burgos, N. R., Shivrain, V. K., Alcober, E. A., and Mauromoustakos, A. (2013). Inter-and intrapopulation variation in dormancy of Oryza sativa (weedy red rice) and allelic variation in dormancy-linked loci. Weed Res. 53, 440–451. doi: 10.1111/wre.12044
USDA ERS (2021). Rice Sector at a Glance. USDA ERS – Rice Sector at a Glance. Available online at: https://www.ers.usda.gov/topics/crops/rice/rice-sector-at-a-glance/ (accessed May 18, 2021).
Vaughan, D. A., Lu, B. R., and Tomooka, N. (2008). The evolving story of rice evolution. Plant Sci. 174, 394–408. doi: 10.1016/j.plantsci.2008.01.016
Vergara, B. S., Jackson, B., and De Datta, S. K. (1976). Deep Water Rice and Its Response to Deep Water Stress. Climate and Rice. Los Banos: International Rice Research Institute, 301–319.
Vogel, E., Donat, M. G., Alexander, L. V., Meinshausen, M., Ray, D. K., Karoly, D., et al. (2019). The effects of climate extremes on global agricultural yields. Environ. Res. Lett. 14:054010. doi: 10.1088/1748-9326/ab154b
Weerakoon, W. M. W., Maruyama, A., and Ohba, K. (2008). Impact of humidity on temperature-induced grain sterility in rice (Oryza sativa L). J. Agron. Crop Sci. 194, 135–140. doi: 10.1111/j.1439-037X.2008.00293.x
Wu, C., Cui, K., Wang, W., Li, Q., Fahad, S., Hu, Q., et al. (2016). Heat-induced phytohormone changes are associated with disrupted early reproductive development and reduced yield in rice. Sci. Rep. 6, 1–14. doi: 10.1038/srep34978
Xiao, Y. H., Yi, P. A. N., Luo, L. H., Deng, H. B., Zhang, G. L., Tang, W. B., et al. (2011). Quantitative trait loci associated with pollen fertility under high temperature stress at flowering stage in rice (Oryza sativa). Rice Sci. 18, 204–209. doi: 10.1016/S1672-6308(11)60028-0
Xu, L. M., Zhou, L., Zeng, Y. W., Wang, F. M., Zhang, H. L., Shen, S. Q., et al. (2008). Identification and mapping of quantitative trait loci for cold tolerance at the booting stage in a japonica rice near-isogenic line. Plant Sci. 174, 340–347. doi: 10.1016/j.plantsci.2007.12.003
Yoshida, S. (1973). Effects of temperature on growth of the rice plant (Oryza sativa L.) in a controlled environment. Soil Sci. Plant Nutr. 19, 299–310. doi: 10.1080/00380768.1973.10432599
Keywords: abiotic stress, weed physiology, weed control, rice breeding, crop improvement, climate change, weedy rice, Oryza sativa ssp.
Citation: Stallworth S, Shrestha S, Schumaker B, Roma-Burgos N and Tseng TM (2021) Screening Diverse Weedy Rice (Oryza sativa ssp.) Mini Germplasm for Tolerance to Heat and Complete Submergence Stress During Seedling Stage. Front. Agron. 3:642335. doi: 10.3389/fagro.2021.642335
Received: 15 December 2020; Accepted: 18 May 2021;
Published: 05 July 2021.
Edited by:
Mithila Jugulam, Kansas State University, United StatesReviewed by:
Simerjeet Kaur, Punjab Agricultural University, IndiaRaveendran Muthurajan, Tamil Nadu Agricultural University, India
Copyright © 2021 Stallworth, Shrestha, Schumaker, Roma-Burgos and Tseng. This is an open-access article distributed under the terms of the Creative Commons Attribution License (CC BY). The use, distribution or reproduction in other forums is permitted, provided the original author(s) and the copyright owner(s) are credited and that the original publication in this journal is cited, in accordance with accepted academic practice. No use, distribution or reproduction is permitted which does not comply with these terms.
*Correspondence: Te-Ming Tseng, t.tseng@msstate.edu