- 1Directorate of Research, Tamil Nadu Agricultural University, Coimbatore, India
- 2Remote Sensing and GIS, Tamil Nadu Agricultural University, Coimbatore, India
- 3Department of Agronomy, Tamil Nadu Agricultural University, Coimbatore, India
- 4Directorate of Natural Resource Management, Tamil Nadu Agricultural University Coimbatore, India
- 5Agricultural Entomology, Tamil Nadu Agricultural University, Coimbatore, India
One of the major components in precision agriculture is crop health monitoring, which includes irrigation, fertilization, pesticide sprays, and timely harvest of the crop. Further, the progressive change in growth and development is critical in crop monitoring and taking suitable decisions to maintain health status. In order to accomplish the task, drones are highly useful for on site detection of problems so as to undertake corrective measures instantly. Although it is expensive to build algorithms and establish relationships between ground truth and spectral signatures, it is a user-friendly technique once the basics studies are done. As labor availability and technical manpower are extremely limited, particularly in India, drones are gaining popularity in the context of smart farming. Insect pests are known to cause catastrophe and drastic reduction in food grain production across the globe. The losses that have been predicted by FAO is over 37% due to pests and diseases. Recently, crops cultivated in India have been threatened by invasive pests like fall army worm (Spodoptera frugiperda) in corn and Rugose spiraling whitefly in coconut (Aleurodicus rugiperculatous Martin); these pests caused extensive damage during the years 2018 and 2019. The plant protection measures are to be taken on a community basis so as to ensure effective management of pests. In India, more than 80% of farmlands are in the category of small and marginal (<1 ha), so it is very difficult to manage the invasive pests. If one field is sprayed, the pests simply shift their feeding to the neighboring fields. To address this, drones become essential. Drones are unmanned aerial vehicles exploited in a wide array of disciplines such as defense, monitoring systems, and disaster management but are only beginning to be utilized in agricultural sciences. There are three major types of drones, namely fixed wing, multi-rotor, and hybrid type, and the usage depends on specific applications. The other types depend on degree of automation, size, weight, and power source. The set operational parameters such as flight speed, height, and endurance need to be optimized to use drones appropriately in agriculture and allied sectors. In addition, parameters related to drone-based spraying such as droplet size, spread, density, uniformity, deposition, and penetrability should also be factored in when implementing drone-based mitigation strategies. Despite the fact that drone technology is highly relevant and appropriate for pest management, the adoption of the technology is restricted. Regulatory guidelines have been set across the globe to perform site-specific farm management with higher precision at a very high resolution. Overall, drones can be employed in almost all agricultural field operations and are considered excellent tools for rapid, reliable, and non-destructive detection of field problems. This review provides panoramic views of drone technology and its application in the management of pests in a digital agriculture era.
Introduction
The biotic stresses caused by pests and diseases are well-known to cause devastation that results in reductions in global food grain production. The FAO has predicted that the losses due to pests and diseases are over 37% (Cao, 2015). They are also severely affecting the crop growth, yield, and quality of produce (Gossen et al., 2008; Berger-Neto et al., 2017). Recently, crops cultivated in India are being threatened by invasive pests such as fall army worm (Spodoptera frugiperda JE Smith) in corn and Rugose spiraling whitefly in coconut (Aleurodicus rugiperculatous Martin), causing extensive damage during 2018 and 2019 (Lal and Bikram, 2019). The fall army worm has become a serious matter of concern to the farmers of India, first hitting the Indian subcontinent in May 2018 in the State of Karnataka. The Indian Agricultural Research Institute–Natural Bureau of Agricultural Insect Resources estimated the intensity of infestation to the tune of 9–62% with an economic yield loss of 34%. The incidence of FAW has spread to the neighboring state of Tamil Nadu where more than 20 districts out of 38 were badly affected in 2019. The effective and rapid interventions and implementation of strategic work plans included drone technology that helped to lessen the incidence of FAW and protect maize crop from infestation while ensuring crop productivity. These pests are to be meticulously monitored and proper technology capsules are to be adopted to save the crops from devastation. Several plant protection strategies are being followed in an integrated way to ensure that crops are protected during the entire crop growing season.
The plant protection measures are to be taken on a community basis so as to ensure effective management of pests and diseases. In India, more than 80% of farmlands are in the category of small and marginal (<1 ha), so it is very difficult to manage invasive pests. If one field is sprayed, the pests simply shift their feeding to the neighboring fields. The invasive pests have enormous potential to multiply in an alarming proportion and are almost impossible to manage with conventional methods of plant protection strategies. It is reported that the annual global use of plant protection chemicals against trans-boundary pests was more than 3 billion kg (Heidary et al., 2014). The utilization of pesticides that are sprayed on the crops exceeds 20–30% and the remaining 70–80% goes as run-off, leaching, evaporation, and drift that cause soil and aquatic pollution as well as deteriorating the quality of the crop produce (Markle et al., 2016; Torrent et al., 2017).
Under these circumstances, effective and timely spraying of plant protection measures are very important. For this, miniaturized unmanned aerial vehicles possess a wide array of benefits that include high efficiency, reduced labor requirement, saving of time and energy, quick response time, and vast area coverage, as well as environmental safety (Meng et al., 2018; Shamshiri et al., 2018). The agricultural research institution State Department of Agriculture have devoted attention to designing and fabricating drones that suit Indian conditions.
The design of UAVs should consider various parameters such as droplet size, wind speed, flight speed, and flight height (Zhang et al., 2012, 2015; Qin et al., 2014). Further, meteorological parameters like wind speed, temperature, and relative humidity can affect the efficacy of pesticide sprays under field conditions (Wang et al., 2018). Under natural conditions, it is very difficult to control the meteorological parameters and thus scientists have attempted to study the drones under protected conditions.
Lv et al. (2019) have examined the efficacy of drones under a protected environment in order to prevent the external interference and the impact of drone flying speed on droplet size, shape, distribution, and uniformity. The study is very useful in optimizing various parameters to determine the effective spray of pesticides using drones. One of the most important criteria for the successful delivery of pesticides or any other input is droplet size. Lv et al. (2019) have conducted a series of elegant experiments to set the parameters for drones to enable pesticide spray and its impact on crop productivity.
Delivery of Droplets
One of the critical factors to be considered for the effectiveness of drone-enabled spray is droplet deposition. The parameters used for measuring the effectiveness of droplet deposition include density, area coverage, and arithmetic mean of droplet size and variation coefficient (Zhu et al., 2011). The droplet deposition density is defined as the number of droplets deposited per unit area which is often measured using blotting paper. Droplet deposition coverage is yet another parameter usually recorded for assessing effectiveness, and is the area of all droplet particles deposited per unit area (Cunha et al., 2012). The arithmetic mean droplet size is the average value of all droplet diameters in one spray sample (Fan, 2011). The co-efficient of variation (CV) measures the uniform spread of droplet deposition in an aerial spraying operation. These four indices can be calculated using the formulae outlined below:
a. Droplet deposition density (D)
No. of droplets deposited (n)/area of droplet collection material (A)
b. Droplet deposition coverage (C)
Area of droplet deposition (S)/Area of the blotting paper (A)
c. Arithmetic mean of droplet size (D0) = Σ Di Ni/Σ Ni
Di is the droplet diameter over time interval
Ni is the number of droplets over time interval
d. Co-efficient of Variation (CV) = SD/X
Xi is the droplet deposition particle size per unit area of blotting paper.
μ is the average droplet size per unit area of blotting paper.
SD is the standard deviation.
n is the number of droplets per blotting paper.
When the CV is smaller, the distribution is considered as uniform, the spray covers the target area, and improves the effectiveness of the sprayed pesticides.
Infrared Thermal Imaging
Thermal imaging is a technique to improve the visibility of the reference objects in a dark environment by detecting it using infrared radiation and creating an image based on the information. Thermal imaging, near-infrared illumination, and low-light imaging are the three most commonly used night vision technologies. Infrared thermal imaging can be exploited for assessing the droplet size and distribution in drone-enabled pesticide sprays.
Lv et al. (2019) have used the infrared thermal imaging technique to accurately measure droplet deposition. After the drones spray, there will be changes in leaf temperature and the infrared thermal imaging technique detects infrared-specific band signal of object thermal radiation by optoelectronic technology, which was used as a supplementary means for droplet deposition measurement. In order to avoid external interference, closed environmental chambers can be employed along with the acquisition of thermal images after the spray test. With a view to prevent changes in temperature before and after spraying, sampling can be done in the middle of the fields using infrared thermal imaging (Lv et al., 2019). A classic method developed by Lv et al. (2019) can be employed to determine droplet deposition with the drones. They have optimized the droplet density, deposition coverage, and droplet size decreases with the drone flight speed. Their studies further inferred that the infrared thermal imager is highly useful for assessing the droplet deposition in the farm drone-enabled spraying. The Tamil Nadu Agricultural University, India, has initiated work on infrared imaging of rice fields using a quadcopter attached with a sensor in order to monitor the health status of the plant (Figure 1).
Rice
Aerial Spray for Brown Plant Hoppers in Rice
Rice is a staple food crop for more than 2.7 billion people in Asia; the loss of productivity of the crop has been estimated as more than 20% (Brookes and Barfoot, 2003). The brown plant hopper (BPH) Nilaparvata lugens causes considerable loss of crop yields globally and is a major pest in India in the late season rice crop planted during September–October (Zhang et al., 2011). The BPH causes damage at the late stage of rice growth. During the late stage of the crop, it is very difficult to undertake manual spraying as the leaves of the rice canopy overlap (Sheng et al., 2002). Further, BPH often colonize at the lower part of the plant which is inaccessible through a manual sprayer. In addition, the muddy fields and overlapping plants makes the conventional system of pesticide spray difficult. In order to overcome the bundle of practical difficulties and acute labor shortage, aerial spray of pesticide using UAVs becomes inevitable (Zhou et al., 2013).
Qin et al. (2016) have explored the possibility of using a miniaturized UAV for pesticide spray with high efficiency with no damage to the rice crop in hilly areas of China. They found that the deposition and distribution of droplets increased with the progression of rice growth which synchronizes with operational height and velocity of crop spraying as executed by the UAV. They have standardized flying height (1.5 m) and speed (5 m s−1) that provides effective delivery of pesticides at the lower leaf as well as uniform distribution (CV = 23%). This also registered an insecticidal efficacy of 92–74% from 3 to 10 days after spraying insecticide. Qin et al. (2016) have offered a strong data base for the optimized design, improved performance, and rational application of UAV in spraying insecticide in rice fields. The range of drone flying height (0.8 and 1.5 m) and flight speed (3 and 5 m s_1) have shown distinct performance in the deposition of droplets. Since the BPH stays in the bottom of the leaves, increased spraying height and speed can enable effective delivery of pesticides and control of pests. Overall, the study has clearly shown that UAV spraying exhibited a superior efficiency than the conventional stretcher sprayer, especially when operated at an altitude of 1.5 m and a velocity of 5 m s_1. Moreover, even 5 and 10 days after pesticide application, a high controlling efficiency was still observed, indicating that the spraying method of low volume and high concentration enhanced the duration of pesticide activity.
Recently, the Tamil Nadu Agricultural University, Coimbatore, India, made a maiden attempt to study the efficacy of pesticide spray (fungicide copper oxychloride 53.8% @ 35 g 16 L−1 against bacterial and fungal diseases) in rice fields using drones during the cropping season of September 2020. A hexacopter type drone (payload 16 L; fuel capacity 3.5 L) was employed to study the application of pesticides in rice fields (Figure 2). Preliminary studies have shown the optimal flying height (3 m), speed (5 m s−1), swath (4 m), and the area coverage (4 min acre−1). The literature review in combination with the preliminary data from Tamil Nadu Agricultural University, India, clearly demonstrate that drone-enabled pesticide spray is an emerging potential technology to overcome labor shortages and to carry out plant protection measures without loss of time.
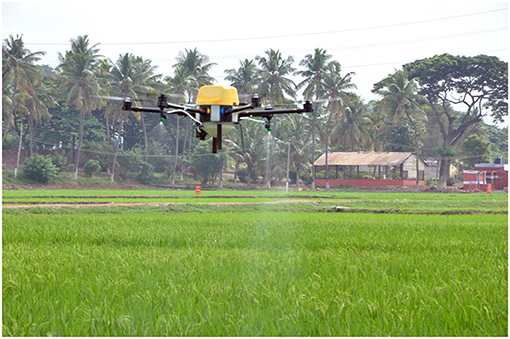
Figure 2. Hexacopter spraying pesticides in rice fields of Tamil Nadu Agricultural University Farm in Tamil Nadu, India during October 2019.
Cotton
Cotton is predominantly cultivated in Australia, Pakistan, India, China, Brazil, and the USA, constituting 80% of the global cotton produced (https://www.wto.org/english/news_e/news16_e/cdac_01jul16_e.pdf). The crop is badly affected by a wide array of defoliating, boll feeding, and sucking pests that devastate the crop to the tune of 10–80% (Sharma et al., 2017). In order to protect the crop from insect pests, huge quantities of pesticides have been used in the past several decades. Indeed, cotton crop alone accounts for 16% of pesticides used globally (https://ejfoundation.org/resources/downloads/the_deadly_chemicals_in_cotton.pdf). This led to the introduction of Bt cotton to reduce the pesticide use to some extent (Krishna and Qaim, 2012). As cotton is cultivated in contiguous blocks, there is every chance that the insect pests will shift their habitat if neighboring fields are sprayed. This situation warrants drone spray to enable a quick response and protect the crop from devastation. There are a few classic works that are summarized below.
Droplet Drift, Deposition, and Distribution Pattern
The unmanned aerial vehicles (UAVs) performance is often affected by environmental factors such as wind speed, direction of wind, temperature, and rain. When spray is undertaken with drones, a small portion of the dosage does not reach the target area, which is popularly referred to as droplet drift or spray drift (Kirk, 2004). Such drift in pesticides is closely associated with wind speed and direction. In order to reduce the drift and wastage of pesticide use, optimal meteorological parameters are set in a standard operational protocol for drone-enabled spray of agro-chemicals. The optimal flight height of 1.5–2.0 m, flight speed 5 m s−1, in combination with wind speed of below 5 km h−1 was found to be effective for spraying pesticides with drones.
Lou et al. (2018) have established a relationship between CV of droplet distribution and flight heights of UAV in cotton fields. Their studies have shown that the co-efficient of droplet densities in the upper, middle, and lower layers of the cotton canopy were at 1.5 m (117.1, 178.1, and 85.8%) and 2.0 m (79.4, 50.3, and 146.4%), respectively. The CV of upper and middle droplet densities at the flight height of 1.5 m was significantly higher than 2 m. It is observed that the reduced flight height, which generates a strong downward swirling airflow that causes the plants to sway substantially and affects the droplet density, causes a significant change of the cotton canopy (Qin et al., 2014). In the study, the droplets' distribution in the vertical direction was considered as density of the droplet. The coverage at the drone flying altitude of 1.5 m (2.5, 3.2, and 1.9%) and 2 m (4.9, 5.5, and 5.0%) were on the upper, middle, and lower layers of the cotton. The spread of the pesticide was more effective in 2 m than 1.5 m. As the flight height increases, the downward pressure gets weakened with wind field below the rotor. There may be a small portion of drift which may be associated with the lateral wind effects.
Aerial Spray of Pesticides on Aphids and Spider Mites in Cotton
In cotton, aphids and mites are serious sucking pests of great concern that cause extensive damage to the crop (Lou et al., 2018). In order to address these pests, aerial sprays using drones were attempted. Lou et al. (2018) studied the efficacy of unmanned aerial vehicles (UAVs) on cotton aphids and spider mites. Similar to other literature, they too recorded that droplet uniformity, spread of the pesticide, and deposition were higher at a flight height of 2 m. The control of aphids and spider mites in cotton were registered as 63.7 and 61.3%, respectively, and the efficacy was lower than boom spraying. They also observed that the UAV spray was slightly less effective in comparison to boom spraying due to the spiral arrangement of leaves in cotton. These data serve as the basis to determine the theoretical prediction of the pesticide effectiveness in cotton fields using drones.
Chillies
The chilly crop is badly affected by pests and diseases causing yield reduction to the tune of 30–40% (Zhang et al., 2013). One of the most dreadful diseases is the Phytophthora capsica, the causal organism for Phytophthora blight in pepper, which shows typical symptoms in the roots, stems, leaves, and fruits, and causes deadly diseases throughout the world (Hausbeck and Lamour, 2004). The spread of the disease is very quick and rapid action is required to contain the disease at the early stage of incidence (He et al., 2019). Yet another sucking pest, Aphids Aphis gossypii Glover, is equally as devastating and reduces crop yield drastically. Aphids suck the plant sap of pepper plants, causing the leaf to curl and its associated honeydew secretion attracts sooty mold infestation, leading to reduced photosynthesis and yield reduction (Chen et al., 2018). In order to address these devastating pests and diseases in chillies, recently, Xiao et al. (2020) have used drones to contain them effectively, rapidly, and with good time management.
Droplet Coverage and Density
Droplet coverage is crucial to measure the effectiveness of drone spray. Xiao et al. (2020) have compared the droplet coverage rate of the electric air-pressure knapsack (EAP) sprayer with drone spray. They found that the EAP coverage was twice as high than that of UAV-enabled spraying of pesticides (21.12 vs. 1.83% and 18.59 vs. 1.43%). Such reduction in coverage closely coincided with the spraying volume of the EAP sprayer (300 L/ha), which was 20 times that of the UAV sprayer (15 L/ha). The results clearly demonstrate that the coverage of pesticide is positively correlated with spray volume. With the progression of plant growth, the droplet coverage was lower in the middle and lower part of the plant in comparison to the first spray. As the leaf area index of the chillies increases with the advancement of growth, the droplet coverage decreased progressively. On the other hand, when double spraying was done, both the upper and middle parts of the plant had better droplet coverage in drones spray as well as EAP (Xiao et al., 2020). Scattered growth within the plants affects the spray fluid penetration in the plants (Zhu et al., 2004). In chillies, there are several pests and diseases that occur in the lower part of the plant and that can be effectively controlled only through droplet penetrability (Wang et al., 2019b). This study further suggests that the penetration of droplets from the UAV-enabled spray is still worse than an EAP sprayer. Spray deposits decreased from the top to bottom of the canopies and decreased linearly with the increase in leaf area index.
Droplet density is also equally important to determine the effectiveness of the drone-enabled spraying (Yuan and Wang, 2015). Xiao et al. (2020) established a relationship between the spray volume and droplet intensity. Spray volume has a remarkable impact on the droplet size and the increasing spray volume increased the droplet intensity. The droplet density of upper, middle, and lower part of the chillies plants and the ground were 34.91, 23.03, 15.06, and 9.50 cm−2, respectively, while EAP had twice as high droplet intensity regardless of the position of the plant. The uniformity of the deposition is also very important for controlling pests and diseases. The uniformity is better in upper layers of the plant canopy than that of the middle and lower layers. Further, such measurements decreased with the progression of crop growth due to expansion of the canopy spread area and leaf area index. In addition to the droplet density, distribution, and uniformity, penetrability of the pesticides is very significant in determining the efficacy of UAV-enabled spray (Xiao et al., 2020).
The pest and disease control efficiencies of both EAP sprayer and drone spray were critically evaluated. Xiao et al. (2020) have shown that the control efficiency of drone spray was more effective only when one-third of the concentration of pesticide was used in comparison to the conventional spray. Since EAP sprayer had better deposition and uniformity, the control of processing peppers with P. capsici was more effective. Similarly, control of aphids was also better in EAP than in UAV spraying. These data clearly demonstrate that drone spray has the potential to reduce the pesticide use, which is safer and reduces the cost to farmers.
Sugarcane
Sugarcane is widely cultivated in tropical countries and India is one of the leading producers and consumers of sugar. In the past decade, areas producing sugarcane have declined drastically, particularly in Tamil Nadu, where the area under sugarcane reduced from 5 lakh ha to <1 ha in the past 5 years. One of the prime reasons for such a phenomenon is the requirement of labor and mechanization which is very scarce (Huang et al., 2014) (Table 1). During the cropping period, any intercultural spray pesticides, nutrients, or bioinoculants are extremely difficult due to the morphology of the crop and sharp edges of the leaf blades which often injure workers (Gebregiorgis, 2012). Further, some of the pests (internode borer, top borer) diseases (smut, red rot, yellow leaf disease) are highly devastating and farmers find it difficult take up any plant protection measures. Under these circumstances, drones may be of help to deliver sprays in sugarcane fields (Lan et al., 2017).
Optimal UAV Parameters for Sugarcane
Sugarcane is a long duration crop, and the canopy arrangements are different from other crops. Aerial spray by drones may be advantageous as accessibility to the cropped field is very difficult in sugarcane (Zhang et al., 2011). Use of UAV in sugarcane is very limited. Zhang et al. (2020) conducted experiments to optimize various spray parameters (spray volume, flight height, and flight velocity) and three levels by quad-rotor drone.
The results revealed that with a comprehensive consideration of the density, uniformity, and penetration of droplet deposition, the optimal spraying parameters were 15 L/ha of spray volume, 3 m of flight height, and 4 m/s of flight velocity, which could be used as a reference parameter for drones when applied in sugarcane crop.
Drones to Manage Fall Army Worm in Sugarcane
The Fall Army Worm (Spodoptera furgiperda) is one of the invasive pests causing extensive damage in maize (Ganiger et al., 2018). The pest, originally from the USA, migrated to Africa in 2016 and entered southern India in 2018 (Padhee and Prasanna, 2019). It is a polyphagous pest that feeds on a wide range of cereals, millets, sugarcane, banana, and other crops (Khan et al., 2018). It causes extensive damage in a very short span of time. In order to control the pest, quick action has to be taken to ensure that pest population is kept at bay. Drones are highly useful technology to undertake spray quickly to contain the pest population. Song et al. (2020) have shown that the application of chlorfenapyr–chlorantraniliprole–lufenuron through drones had control efficacy of 94.94% and declined the pest population by about 94.86%.
Conclusion
An extensive review was undertaken to determine the feasibility and utilization of Unmanned Aerial Vehicles (UAVs) or drones for pesticide sprays in various crops; the output from the literature is summarized in Table 2. The table clearly suggests that there are several studies that have been undertaken and demonstrated on the use of drones successfully in pesticide spray in agricultural and horticultural crops. The following are the observations:
1. Drone application in agriculture is primarily focused on pesticide applications. Extensive research has been done on optimization of spray volume, droplet size, spread of droplets, and penetrability as well as efficacy of pesticides in insect pest control (Lou et al., 2018). Many of the optimization parameters indicated were done mainly for pesticides use in agriculture.
2. A majority of the research on UAV for pesticide spray in crops was carried out in rice (Qin et al., 2016), wheat (Wang et al., 2019a), corn (Zheng et al., 2017), cotton (Lou et al., 2018), pepper (Xiao et al., 2020), and sugarcane (Zhang et al., 2019) as these crops consume more pesticides than any others. Further, these crops are cultivated in larger areas in contiguous blocks in developed and developing countries where drone application is feasible.
3. In order to improve insecticidal use efficiencies in crops, drone operational parameters such as flight speed, flight height, nozzle type, payload, and drone type are be optimized for the given situation. Overall, flight height of 2–3 m, flight speed of 3–5 ms−1, two fan nozzle, four rotor UAV, and 15 L payload are found to be optimal to undertake pesticide sprays using drones in agricultural crops (Zhang et al., 2011).
4. Drone efficiencies are to be examined for certain application parameters such as droplet size distribution, droplet coverage, uniformity of droplets, droplet penetrability, droplet drift, and insecticidal efficiency prior to commercial use of drones in agriculture (Lv et al., 2019).
5. There are potential benefits to drone usage in agriculture that include large area coverage, less quantities of pesticides, labor saving, quick response time, and timely operation well before pest occurrence exceeds economic threshold levels (Huang et al., 2018).
6. Despite the fact that there are ample advantages attached to drone technology, every country has its own regulatory guidelines for the use of drones in agriculture (Ayamga et al., 2021). Prior approvals are required from local authorities to use drones in agriculture.
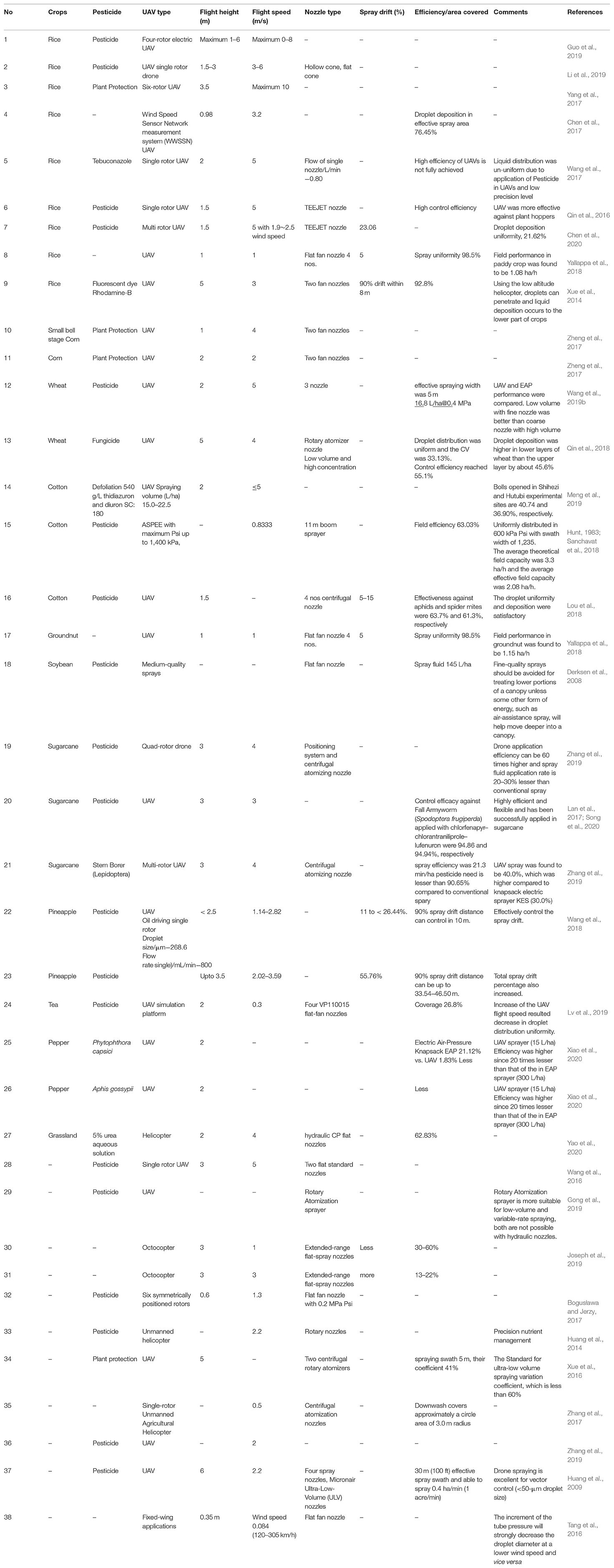
Table 2. Application of UAVs with the set of parameters (Flight height, Flight speed, Nozzle Type) for pesticides spray in various crops and their efficiencies in comparison to conventional sprayers.
Overall, the literature review clearly demonstrated that drone technology is very effective in delivering pesticides for a wide array of crops. The effectiveness has been validated with conventional hand-operated sprayers. The data suggest that, although the operational parameters and delivery parameters have been optimized for a specific crop or a particular pests/disease, some fine tuning is required to improve the efficacy for a given situation. This literature review has given an overview of drone technology employed for pesticide sprays. Since there are no ultralow volume pesticide formulations available in the market, the conventional pesticides were used in drone technology with the same optimized concentration. Thus, there is an urgent need to develop innovative new nano formulations to improve the efficacy of drone technology while minimizing the cost and improving environmental safety.
Author Contributions
KS: synthesis and orchestration of the review. SP: responsible for drones enabled application. GS: collection of literature and plagiarism check. RS: drone application in agriculture. NS: entomologist involved in drone enabled spray. All authors contributed to the article and approved the submitted version.
Conflict of Interest
The authors declare that the research was conducted in the absence of any commercial or financial relationships that could be construed as a potential conflict of interest.
Publisher's Note
All claims expressed in this article are solely those of the authors and do not necessarily represent those of their affiliated organizations, or those of the publisher, the editors and the reviewers. Any product that may be evaluated in this article, or claim that may be made by its manufacturer, is not guaranteed or endorsed by the publisher.
References
Ayamga, M., Tekinerdogan, B., and Kassahun, A. (2021). Exploring the challenges posed by regulations for the use of drones in agriculture in the African Context. Land 10:164. doi: 10.3390/land10020164
Berger-Neto, A., Jaccoud-Filho, D. D. S., Wutzki, C. R., Tullio, H. E., Pierre, M. L. C., Manfron, F., et al. (2017). Effect of spray droplet size, spray volume and fungicide on the control of white mold in soybeans. Crop Protect. 92, 190–197. doi: 10.1016/j.cropro.2016.10.016
Bogusława, B., and Jerzy, C. (2017). Farm machinery and processes management in sustainable agriculture. Use of drones in crop protection. Available online at: https://www.researchgate.net/publication/321708513_USE_OF_DRONES_IN_CROP_PROTECTION/citation/download (accessed July 29, 2020).
Brookes, G., and Barfoot, P. (2003). GM Rice: Will This Lead the Way for Global Acceptance of GM Crop Technology?. Los Banos: ISAAA.
Cao, L. P.. (2015). The research progress on machine recognition of plant diseases and insect pests. Chin. Agricult. Sci. Bull. 31, 244–249.
Chen, J., Fan, Y., Wang, T., Zhang, C., Qiu, Z., and He, Y. (2018). Automatic segmentation and counting of aphid nymphs on leaves using convolutional neural networks. Agronomy 8:129. doi: 10.3390/agronomy8080129
Chen, S., Lan, Y., Zhou, Z., Ouyang, F., Wang, G., Huang, X., et al. (2020). Effect of droplet size parameters on droplet deposition and drift of aerial spraying by using plant protection UAV. Agronomy 10:195. doi: 10.3390/agronomy10020195
Chen, S. D., Lan, Y. B., and Li, J. Y. (2017). Effect of wind field below rotor on distribution of aerial spraying droplet deposition by using multi-rotor UAV. Trans. Chin. Soc. Agricult. Mach. 48, 105–113. doi: 10.6041/j.issn.1000-1298.2017.08.011
Cunha, M., Carvalho, C., and Marcal, A. R. S. (2012). Assessing the ability of image processing software to analyse spray quality on water-sensitive papers used as artificial targets. Biosyst. Eng. 111, 11–23. doi: 10.1016/j.biosystemseng.2011.10.002
Derksen, R. C., Zhu, H., and Ozkan, H. E. (2008). Determining the influence of spray quality, nozzle type, spray volume, and air-assisted application strategies on deposition of pesticides in soybean canopy. Am. Soc. Agricult. Eng. 51, 1529–1537. doi: 10.13031/2013.25301
Fan, Q. N.. (2011). The Research on the Pesticide Spray System Using for the Mini Unmanned Helicopter. Nanjing Forestry University.
Ganiger, P., Yeshwanth, H. M., Muralimohan, K., Vinay, N., Ranjith Kumar, V., and Chandrashekara, K. (2018). Occurrence of the new invasive pest, fall armyworm, Spodoptera frugiperda (J.E. Smith)(Lepidoptera: Noctuidae), in the maize fields of Karnataka, India. Curr. Sci. 115, 621–623. doi: 10.18520/cs/v115/i4/621-623
Gebregiorgis, F.. (2012). Increasing the efficiency of knapsack sprayers by modifying a single nozzle sprayer into a low cost multi nozzle sprayer. Ethiopian J. Health Dev. 5, 28–42.
Gong, J. Q., Fan, W. Q., and Peng, J. (2019). Application analysis of hydraulic nozzle and rotary atomization sprayer on plant protection UAV. Int. J. Precis. Agric. Aviat. 2, 25–29. doi: 10.33440/j.ijpaa.20190201.0021
Gossen, B. D., Peng, G., Wolf, T. M., and McDonald, M. R. (2008). Improving spray retention to enhance the efficacy of foliar-applied disease- and pest-management products in field and row crops. Canad. J. Plant Pathol. 30, 505–516. doi: 10.1080/07060660809507550
Guo, S., Li, J., Yao, W., Zhan, Y., Li, Y., and Shi, Y. (2019). Distribution characteristics on droplet deposition of wind field vortex formed by multirotor UAV. PLoS ONE 14:e0220024. doi: 10.1371/journal.pone.0220024
Hausbeck, M. K., and Lamour, K. H. (2004). Phytophthora capsici on vegetable crops: Research progress and management challenges. Plant Dis. 88, 1292–1303. doi: 10.1094/PDIS.2004.88.12.1292
He, Y. M., Liu, K. K., Zhang, H. X., Cheng, G. X., Ali, M., Ul Haq, S., et al. (2019). Contribution of CaBPM4, a BTB domain–containing gene, to the response of pepper to Phytophthora capsici infection and abiotic stresses. Agronomy 9:417. doi: 10.3390/agronomy9080417
Heidary, M. A., Douzals, J. P., Sinfort, C., and Vallet, A. (2014). Influence of spray characteristics on potential spray drift of field crop sprayers: a literature review. Crop Protect. 63, 120–130. doi: 10.1016/j.cropro.2014.05.006
Huang, Y., Hoffman, W. C., Lan, Y., Bradley, K. F., and Steven, J. T. (2014). Development of a low-volume sprayer for an unmanned helicopter. J. Agricult. Sci. 7, 1916–9752. doi: 10.5539/jas.v7n1p148
Huang, Y., Hoffmann, W. C., Lan, Y., Wu, W., and Fritz, B. K. (2009). Development of a Spray System for an Unmanned Aerial Vehicle Platform. USDA-ARS/UNL Faculty. Available online at: https://digitalcommons.unl.edu/usdaarsfacpub/2089 (accessed December 10, 2020).
Huang, Y. K., Li, W. F., Zhang, R. Y., and Wang, X. Y. (2018). Integrated Control of Sugarcane Diseases and Pests. Color Illustration of Diagnosis and Control for Modern Sugarcane Diseases, Pests, and Weeds. Beijing: China Agriculture Press, 361–377. doi: 10.1007/978-981-13-1319-6_5
Hunt, D. R.. (1983). Farm Power and Machinery Management (8th Edition). Ames, IA: Iowa State University Press, 365.
Joseph, E., Hunter III., Gannon, T. W., Richardson, R. J., Yelverton, F. H., and Leon, R. G. (2019). Integration of remote–weed mapping and an autonomous spraying unmanned aerial vehicle for site-specific weed management. Pest Manag. Sci. 76, 1386–92.
Khan, Z. R., Pittchar, J. O., Midega, C. A. O., and Pickett, J. A. (2018). Push-pull farming system controls Fall Armyworm: lessons from Africa. Outlooks Pest Manag. 29, 220–224. doi: 10.1564/v29_oct_09
Kirk, I. W.. (2004). Aerial Applicators Spray Nozzle Handbook. AH-726. Washington, DC: USDA Agricultural Research Service.
Krishna, V. V., and Qaim, M. (2012). Bt cotton and sustainability of pesticide reductions in India. Agric. Syst. 107, 47–55.334. doi: 10.1016/j.agsy.2011.11.005
Lal, C. B., and Bikram, A. (2019). Fall armyworm (Spodoptera frugiperda): A threat to food security for south Asian country: Control and management options: A review. Farm manag. 4, 38–44.
Lan, Y. B., Chen, S. D., and Fritz, B. K. (2017). Current status and future trends of precision agricultural aviation technologies. Int. J. Agricult. Biol. Eng. 10, 1–17. doi: 10.3965/j.ijabe.20171003.3088
Li, X., Andaloro, J., Lang, E., and Pan, Y. (2019). “Best management practices for unmanned aerial vehicles (UAVs) application of insecticide products on rice,” in 2019 ASABE Annual International Meeting, Session of Precision Aerial Spray Applications: UAV/Drones and Manned Aircraft (Boston, MA). doi: 10.13031/aim.201901493
Lou, Z., Xin, F., Han, X., Lan, Y., Duan, T., and Fu, W. (2018). Effect of unmanned aerial vehicle flight height on droplet distribution, drift and control of cotton aphids and spider mites. Agronomy 8:187. doi: 10.3390/agronomy8090187
Lv, M. Q., Xiao, S. P., Tang, Y., and He, Y. (2019). Influence of UAV flight speed on droplet deposition characteristics with the application of infrared thermal imaging. Int. J. Agric. Biol. Eng. 12, 10–17. doi: 10.25165/j.ijabe.20191203.4868
Markle, J. C., Niederholzer, F. J., and Zalom, F. G. (2016). Evaluation of spray application methods for navel orange worm control in almonds. Pest Manag. Sci. 72, 2339–2346. doi: 10.1002/ps.4279
Meng, Y., Song, J., Lan, Y., Mei, G., Liang, Z., and Han, Y. (2019). Harvest aids efficacy applied by unmanned aerial vehicles on cotton crop. Ind. Crops Prod. 140:111645. doi: 10.1016/j.indcrop.2019.111645
Meng, Y. H., Lan, Y. B., Mei, G. Y., Guo, Y. W., Song, J. L., and Wang, Z. G. (2018). Effect of aerial spray adjuvant applying on the efficiency of small unmanned aerial vehicle on wheat aphids. Int. J. Agricult. Biol. Eng. 11, 46–53. doi: 10.25165/j.ijabe.20181105.4298
Padhee, A. K., and Prasanna, B. M. (2019). The emerging threat of Fall Armyworm in India. Ind. Farm. 69, 51–54.
Qin, W. C., Qiu, B. J., and Xue, X. Y. (2016). Droplet deposition and control effect of insecticides sprayed with an unmanned aerial vehicle against plant hoppers. Crop Protect. 85, 79–88. doi: 10.1016/j.cropro.2016.03.018
Qin, W. C., Xue, X. Y., Zhang, S. M., Gu, W., and Wang, B. K. (2018). Droplet deposition and efficiency of fungicides sprayed with small UAV against wheat powdery mildew. Int. J. Agricult. Biol. Eng. 11, 27–32. doi: 10.25165/j.ijabe.20181102.3157
Qin, W. C., Xue, X. Y., Zhou, L. X., Zhang, S. C., Sun, Z., Kong, W., et al. (2014). Effects of spraying parameters of unmanned aerial vehicle on droplets deposition distribution of maize canopies. Trans. Chin. Soc. Agricult. Eng. 30, 50–56. doi: 10.3969/j.issn.1002-6819.2014.05.007
Sanchavat, H., Chudhary, H., Bhautik, G., and Singh, S. N. (2018). Field evaluation of a tractor mounted boom sprayer. Agric. Eng. Today 41, 67–71.
Shamshiri, R. R., Hameed, I. A., Balasundram, S. K., Ahmad, D., Weltzien, C., and Yamin, M. (2018). “Fundamental research on unmanned aerial vehicles to support precision agriculture in oil palm plantations,” in Agricultural Robots: Fundamentals and Applications, eds J. Zhou and B. Zhang (Intech Open). doi: 10.5772/intechopen.80936
Sharma, S., Kooner, R., and Arora, R. (2017). “Insect pests and crop losses.” in Breeding Insect Resistant Crops for Sustainable Agriculture, eds R. Arora and S. Sandhu (Singapore: Springer), 45–66. doi: 10.1007/978-981-10-6056-4_2
Sheng, C. F., Xuan, W. J., Jiao, X. G., Su, J. W., Shao, Q. C., and Song, F. B. (2002). Causes, trend and control strategies of disaster of rice borers in China. J. Nat. Disasters 11:103–108.
Song, X. P., Liang, Y. J., Zhang, X. Q., Qin, Z. Q., Wei, J. J., Li, Y. R., et al. (2020). Intrusion of fall armyworm (Spodoptera frugiperda) in sugarcane and its control by drone in China. Sugar Tech. 22, 734–737. doi: 10.1007/s12355-020-00799-x
Tang, Q., Chen, L., Zhang, R., Xu, M., Xu, G., and Yi, T. (2016). “Airspeed and pressure affect spray droplet spectrum from an aerial nozzle for fixed-wing applications,” in Proceedings of the 13th International Conference on Precision Agriculture July 31 – August 3, 2016 (St. Louis, MI).
Torrent, X., Garcer,á, C, Molt,ó, E, Chueca, P., Abad, R., Grafulla, C., et al. (2017). Comparison between standard and drift reducing nozzles for pesticide application in citrus: Part I. Effects on wind tunnel and field spray drift. Crop Protect. 96, 130–143. doi: 10.1016/j.cropro.2017.02.001
Wang, C. L., He, X. K., and Wang, X. N. (2016). Testing method of spatial pesticide spraying deposition quality balance for unmanned aerial vehicle. Trans. Chin. Soc. Agricult. Eng. 32, 54–61. doi: 10.11975/j.issn.1002-6819.2016.11.008
Wang, G., Lan, Y., Qi, H., Chen, P., Hewittd, A., and Hana, Y. (2019a). Field evaluation of an unmanned aerial vehicle(UAV) sprayer: effect of spray volumeon deposition and the control of pests and disease in wheat. Pest Manag. Sci. 75, 1546–55. doi: 10.1002/ps.5321
Wang, G. B., Lan, Y. B., Yuan, H. Z., Qi, H. X., Chen, P. C., Ouyang, F., et al. (2019b). Comparison of spray deposition, control efficacy on wheat aphids and working efficiency in the wheat field of the unmanned aerial vehicle with boom sprayer and two conventional knapsack sprayers. Appl. Sci. 9:218. doi: 10.3390/app9020218
Wang, J., Lan, Y. B., Zhang, H. H., Zhang, Y. L., Wen, S., Yao, W. X., et al. (2018). Drift and deposition of pesticide applied by UAV on pineapple plants under different meteorological conditions. Int. J. Agricult. Biol. Eng. 11, 5–12. doi: 10.25165/j.ijabe.20181106.4038
Wang, S. L., Song, J. L., and He, X. K. (2017). Performances evaluation of four typical unmanned aerial vehicles used for pesticide application in China. Int. J. Agricult. Biol. Eng. 10, 22–31. doi: 10.25165/j.ijabe.20171004.3219
Xiao, Q., Du, R., Yang, L., Han, X., Zhao, S., Zhang, G., et al. (2020). Comparison of droplet deposition control efficacy on Phytophthora capsica and aphids in the processing pepper field of the unmanned aerial vehicle and knapsack sprayer. Agronomy 10:215. doi: 10.3390/agronomy10020215
Xue, X., Lan, Y., Sun, Z., Chang, C., and Hoffmann, W. C. (2016). Develop an unmanned aerial vehicle based automatic aerial spraying system. Comput. Electr. Agricult. 128, 58–66. doi: 10.1016/j.compag.2016.07.022
Xue, X. Y., Tu, K., Qin, W. C., Lan, Y. B., and Zhang, H. H. (2014). Drift and deposition of ultra-low altitude and low volume application in paddy field. Int. J. Agricult. Biol. Eng. 7, 23–28. doi: 10.3965/j.ijabe.20140704.003
Yallappa, D., Veerangouda, M., Maski, D., Palled, V., and Bheemanna, M. (2018). “Development and evaluation of drone mounted sprayer for pesticide applications to crops,” in 2017 IEEE Global Humanitarian Technology Conference, 1–7. doi: 10.1109/GHTC.2017.8239330
Yang, F. B., Xue, X. Y., Zhang, L., and Sun, Z. (2017). Numerical simulation and experimental verification on downwash air flow of six-rotor agricultural unmanned aerial vehicle in hover. Int. J. Agricult. Biol. Eng. 10, 41–53. doi: 10.25165/j.ijabe.20171004.3077
Yao, W., Lan, Y., Ho_mann, W. C., Li, J., Guo, S., Zhang, H., et al. (2020). Droplet size distribution characteristics of aerial nozzles by bell206l4 helicopter under medium and low airflow velocity wind tunnel conditions and field verification test. Appl. Sci. 10:2179. doi: 10.3390/app10062179
Yuan, H. Z., and Wang, G. B. (2015). Effects of droplet size and deposition density on field efficacy of pesticides. Plant Prot. 41, 9–16.
Zhang, J., He, X. K., Song, L. J., Zeng, A. J., Liu, Y. J., and Li, X. F. (2012). Influence of spraying parameters of unmanned aircraft on droplets deposition. Trans. Chin. Soc. Agric. Machinery 43, 94–96. doi: 10.6041/j.issn.1000-1298.2012.12.017
Zhang, S. C., Xue, X. Y., Qin, W. C., Sun, Z., Ding, SM, and L. X., Zhou. (2015). Simulation and experimental verification of aerial spraying drift on N-3 unmanned spraying helicopter. Trans. Chin. Soc. Agricult. Eng. 31, 87–93. doi: 10.3969/j.issn.1002-6819.2015.03.012
Zhang, S. C., Xue, X. Y., Sun, Z., Zhou, L. X., and Jin, Y. K. (2017). Downwash distribution of single-rotor unmanned agricultural helicopter on hovering state. Int. J. Agricult. Biol. Eng. 10, 14–24. doi: 10.25165/j.ijabe.20171005.3079
Zhang, W. J., Jiang, F. B., and Ou, J. F. (2011). Global pesticide consumption and pollution: With China as a focus. Proc. Int. Acad. Ecol. Environ. Sci. 1, 125–144.
Zhang, X. Q., Liang, Y. J., Qin, Z. Q., Li, D. W., Wei, C. Y., Wei, J. J., et al. (2019). Application of multi-rotor unmanned aerial vehicle application in management of stem borer (lepidoptera) in sugarcane. Sugar Tech 21, 847–852. doi: 10.1007/s12355-018-0695-y
Zhang, X. Q., Song, X. P., Liang, Y. J., Qin, Z. Q., Zhang, B. Q., Wei, J. J., et al. (2020). Effects of spray parameters of drone on the droplet deposition in sugarcane canopy. Sugar Tech. 22, 583–588. doi: 10.1007/s12355-019-00792-z
Zhang, Y. L., Jia, Q. L., Li, D. W., Wang, J. E., Yin, Y. X., and Gong, Z. H. (2013). Characteristic of the pepper CaRGA2 gene in defense responses against Phytophthora capsici Leonian. Int. J. Mol. Sci. 14, 8985–9004. doi: 10.3390/ijms14058985
Zheng, Y. J., Yang, S. H., and Zhao, C. J. (2017). Modelling operation parameters of UAV on spray effects at different growth stages of corns. Int. J. Agricult. Biol. Eng. 10, 57–66. doi: 10.3965/j.ijabe.20171003.2578
Zhou, Z. Y., Zang, Y., Luo, X. W., Lan, Y. B., and Xue, X. Y. (2013). Technology innovation development strategy on agricultural aviation industry for plant protection in China. Trans. CSAE 29, 1–10. doi: 10.3969/j.issn.1002-6819.2013.24.001
Zhu, H., Dorner, J. W., Rowland, D. L., Derksen, R. C., and Ozkan, H. E. (2004). Spray penetration into peanut canopies with hydraulic nozzle tips. Biosyst. Eng. 87, 275–283. doi: 10.1016/j.biosystemseng.2003.11.012
Keywords: unmanned aerial vehicle, drone technology, pest management, sensors, precision agriculture
Citation: Subramanian KS, Pazhanivelan S, Srinivasan G, Santhi R and Sathiah N (2021) Drones in Insect Pest Management. Front. Agron. 3:640885. doi: 10.3389/fagro.2021.640885
Received: 12 December 2020; Accepted: 02 November 2021;
Published: 09 December 2021.
Edited by:
Ujjwal Bhattacharya, Indian Statistical Institute, IndiaReviewed by:
Soumi Mitra, New Mexico State University, United StatesTania Prinsloo, University of Pretoria, South Africa
Copyright © 2021 Subramanian, Pazhanivelan, Srinivasan, Santhi and Sathiah. This is an open-access article distributed under the terms of the Creative Commons Attribution License (CC BY). The use, distribution or reproduction in other forums is permitted, provided the original author(s) and the copyright owner(s) are credited and that the original publication in this journal is cited, in accordance with accepted academic practice. No use, distribution or reproduction is permitted which does not comply with these terms.
*Correspondence: K. S. Subramanian, a3NzQHRuYXUuYWMuaW4=