- 1Chair of Plant Health, Institute of Agricultural and Environmental Sciences, Estonian University of Life Sciences, Tartu, Estonia
- 2Department of Plants and Crops, Laboratory of Agrozoology, Faculty of Bioscience Engineering, Ghent University, Ghent, Belgium
- 3Department of Psychiatry and Neuropsychology, School for Mental Health and Neuroscience, Faculty of Health, Medicine and Life Sciences, Maastricht University, Maastricht, Netherlands
The biosafety aspect of applying double-stranded RNA (dsRNA) in crop pest management is rooted in dsRNA's mode of action, which displays nucleotide sequence-specificity to a particular region of a messenger RNA (mRNA), against which the insecticidal dsRNA is designed. This prominent and promising class of insecticides therefore has the potential to target a single pest species while conferring negligible effect on non-target organisms. Recent studies examining the effect of target-specific dsRNA in adults of the pollen beetle Brassicogethes aeneus, a major pest of oilseed rape (Brassica napus) crops in Europe, suggest the potential for developing a gene-silencing approach within integrated B. aeneus management. The present study examines the efficacy of target-specific dsRNA on target-mRNA silencing, and subsequent gene-silencing-induced mortality, in B. aeneus larvae, as this life stage represents a critical target for achieving optimal integrated B. aeneus control. Treatment applications occurred via feeding on dsRNA-treated anthers for 3 d. We observed variable gene-silencing efficacy, all target treatments having a significant or marginally significant effect after 3 d of dsRNA feeding, with greater variability at 6 d. These results further validated significant gene-silencing-induced mortality observed for one of the target treatments. Moreover, gene-silencing-induced mortality occurred at a quicker rate in B. aeneus larvae compared to what has been previously observed in B. aeneus adults. Finally, we consider refinements that must be made to B. aeneus larval bioassay setups to promote and strengthen future larval studies regarding this important crop pest species.
Introduction
Double-stranded RNA (dsRNA) is a prominent class of insecticides and can be applied to crops with potentially no impact on non-target taxa. Cagliari et al. (2018) and Taning et al. (2019) review the prospects of an approach in which dsRNA can be sprayed onto crops in order to manage target pests. Indeed, this approach has been demonstrated in both the greenhouse (Miguel and Scott, 2015) and field (Petek et al., 2020) for the control of Colorado potato beetle (Leptinotarsa decemlineata Say). The biosafety of dsRNA-based insecticides lies in their mode of action against the target pest species, that being nucleotide sequence-specificity. Indeed, the structure of a dsRNA for use in crop protection can be designed to target a specific region of a messenger RNA (mRNA), potentially resulting in RNA interference (RNAi)-induced gene silencing and subsequent mortality in the target species.
Recent studies suggest potential for developing an RNAi approach for use in integrated management of a major oilseed rape (Brassica napus L.) pest, the pollen beetle B. aeneus Fab. (syn. Meligethes aeneus), via field relevant routes of exposure, including consumption of dsRNA-treated oilseed rape buds (Willow et al., 2020a) and anthers (Willow et al., 2021) as well as dsRNA-treated honey water, representing dsRNA-contaminated nectar (Willow et al., 2020b). The above-mentioned studies were performed on B. aeneus adults, the adult stage being the typical focus of insecticide bioassays regarding this pest species (but see Melander et al., 2003).
Brassicogethes aeneus development starts inside the reproductive bud, where hatched larvae feed on the anthers within, followed by the emergence of late first- and early second instar larvae that proceed to feed on the pollen and nectar of open flowers. Therefore, it is plausible that dsRNA application during oilseed rape's flowering stage could additionally target larval B. aeneus and, in turn, reduce not only the abundances of pupating larvae and overwintering next-generation adults but oilseed rape yield losses as well. Thus, it is critical that B. aeneus larvae are examined as a potential RNAi target for oilseed rape protection. Indeed, if the potential for implementing RNAi-based management of B. aeneus is ever examined under natural outdoor conditions, larvae will undoubtedly represent the life stage monitored in such a study. This is because adults can fly from plot to plot, confounding monitoring; whereas larvae will remain on their natal plant until pupation. The present study represents the first attempt to examine RNAi in B. aeneus larvae. We expected consumption of B. aeneus-specific dsRNA, via treated anthers, to result in reduced target mRNA expression followed by gene silencing-induced mortality.
Methods
A 222 bp region of B. aeneus's coatomer subunit alpha (αCOP) sequence, and a 455 bp region of green fluorescent protein [gfp; Supplementary Table 1; (Willow et al., 2020a,b)], were the basis for in vitro synthesis of two corresponding dsRNAs (AgroRNA, Seoul, South Korea). Both dsRNAs were shipped in distilled water (dH2O) and kept at 5 ± 1°C upon reception. The absence of nucleic contaminants in these dsRNAs, hereafter respectively called dsαCOP and dsGFP (control since gfp is not present in insects), was verified via gel electrophoresis.
Pollen beetle larvae were collected via the collection of oilseed rape flowers from an untreated field (58.36377°N, 26.66145°E) in the village of Õssu, Tartu County, Estonia. Flowers were transported to the lab and examined for the presence of pollen beetle larvae. Only late first and early second instar larvae identified as B. aeneus via Osborne (1965) were used in the study. B. aeneus larvae were immediately transferred to polystyrene insect-breeding dishes (diameter 10 x height 4 cm; SPL Life Sciences, Gyeonggi-do, South Korea) that were ventilated and transparent, hereafter referred to as cages. Eight randomly chosen B. aeneus larvae were gently placed in each cage, using a fine paintbrush to avoid any mechanical damage to larvae, and were immediately provisioned with their respective dietary treatment.
There were four treatments, including dsαCOP at 0.5, 2.5 and 5 μg/μL, and dsGFP at 5 μg/μL. Previous studies on B. aeneus adults showed no difference in control dsGFP concentration used (Willow et al., 2020b), and therefore we continued to use only the highest corresponding concentration to the dsαCOP concentrations examined, as in, for example, Willow et al. (2020a). Each treatment was allocated ten cages (n = 10; 80 larvae per treatment). Treatments were provided as ad libitum access to dsRNA-treated anthers of oilseed rape flowers. Petals were removed from flowers, and anthers were soaked in treatment solution for 15 s and allowed to air dry. Treatment solutions consisted of dsRNA, dH2O, and the surfactant Triton X-100 (always at 180 ppm; Fisher Bioreagents, Leicestershire, UK), and were vortexed prior to treating anthers. After treatment provision, cages were placed in a climate chamber (Sanyo MLR-351H, Osaka, Japan) at 20°C, 70% relative humidity and 16:8 h light:dark cycle. Freshly-treated anthers were replaced every 24 ± 1 h for 3 d. After 3 d, B. aeneus larvae were allowed to feed ad libitum on untreated oilseed rape anthers, which were replaced every 24 ± 1 h for a subsequent 4 d. Survival monitoring occurred over a total of 7 d, and dead larvae were removed from cages daily. After the first 24 h of the experiment, any dead larvae were removed from the experiment, since at this time no mortality could be attributed to RNAi, but rather stress from manipulations and changing conditions. These mortalities after 1 d were few and were accounted for in the statistical analysis. One B. aeneus larva was also removed from the study (dsαCOP 0.5 μg/μL treatment, at 5 d) due to predation by a dipteran larva that was inadvertently introduced to a cage when providing untreated oilseed rape flowers B. aeneus larvae. This RNAi-unrelated loss was also accounted for in the statistical analysis.
At 3 and 6 d after the start of the experiment, 15 larvae per treatment were randomly removed from cages, and relative mRNA expression was analyzed via quantitative polymerase chain reaction (qPCR; n = 3 per time point, per treatment; 5 larvae pooled per sample). Removal of larvae for qPCR was accounted for in the statistical analysis of survival. Larvae used for qPCR were immediately placed in Eppendorf tubes. Samples were homogenized in 600 μL of RTL buffer (with 10 μL of β-mercaptoethanol added) using a sterile plastic pestle designed for Eppendorf tubes and stored at −80 °C until analysis. Total RNA was extracted using RNeasy Mini Kit (Qiagen, Venlo, Netherlands). RNA concentration and purity were assessed via NanoDrop spectrophotometer (Thermo Fisher Scientific, Waltham, USA); the absence of nucleic contaminants was further verified via gel electrophoresis. Genomic DNA was removed via Turbo DNA-Free Kit (Invitrogen, Carlsbad, USA). Reverse transcription of cDNA was performed via FIREScript RT cDNA Synthesis Kit (Solis BioDyne, Tartu, Estonia), using 1 μg of total RNA. The qPCR was performed in Quantistudio 5 Real-Time PCR System (Applied BioSciences, Foster City, USA); and the reaction mixture consisted of 4 μL of 5xHOT FIREPol EvaGreen qPCR Supermix (Solis BioDyne), 0.5 μL of 10 μM forward and reverse primers (Supplementary Table 2; Willow et al., 2020a,b; Microsynth, Balgach, Switzerland), 14 μL of nuclease-free water and 1 μg of cDNA, in a total volume 20 μL. Amplification conditions were 15 min at 95°C, 40 cycles of 15 s at 95°C, 1 min at 58°C, and ending with a melting curve analysis (range 60–95°C). Reactions were organized, in triplicate, in a 384-well PCR plate. The housekeeping genes actin (act) and ribosomal protein S3 (rps3) were used to normalize target gene levels. Primer amplification efficiencies were calculated via cDNA dilution series (Supplementary Table 2). Relative αCOP expression values were calculated using the 2−ΔΔCt method. A no-template- and no-reverse-transcriptase control were both included in the assay.
For both survival- and gene expression analysis, comparisons were made between the dsGFP control and dsαCOP at 0.5, 2.5, and 5 μg/μL. For survival analysis, homogeneity of variance and normality of data distributions were respectively determined via Levene- and Shapiro–Wilk tests. As the data were overall not normally distributed, we used the Kruskal–Wallis test as a non-parametric alternative to ANOVA, followed by the Wilcoxon rank-sums test with post-hoc Bonferroni correction for multiple comparisons. Comparisons regarding gene expression were made via Welch's t-test. All statistical analyses were performed in R v3.6.3 (R Foundation for Statistical Computing, Vienna, Austria).
Results
After 3 d of feeding on dsRNA-treated oilseed rape anthers, B. aeneus larvae showed 57% (t = 2.46, df = 2.94, p = 0.093), 77% (t = 3.25, df = 3.16, p = 0.044) and 83% (t = 3.93, df = 2.17, p = 0.052) mean reductions in αCOP expression, respectively for dsαCOP 0.5, 2.5 and 5 μg/μL treatments, compared to the dsGFP 5 μg/μL control treatment (Figure 1). At 6 d after the start of the experiment, B. aeneus larvae showed no reduction in αCOP expression, and more variability within treatments.
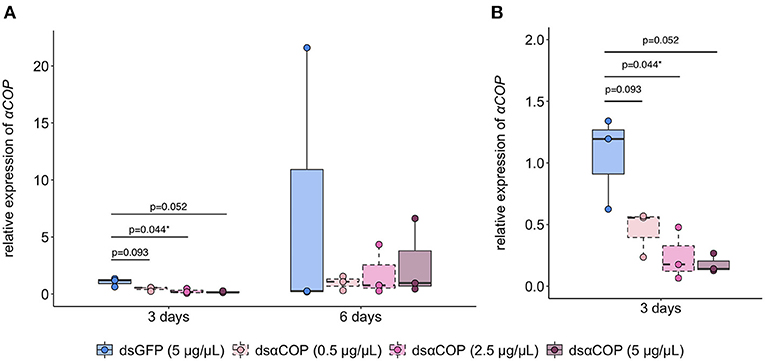
Figure 1. Relative expression of B. aeneus coatomer subunit alpha (αCOP) in B. aeneus larvae at (A) 3 and 6 d after the start of the experiment; (B) reduced y-axis value-limits, for better visualization of 3 d qPCR data. Data were normalized using the housekeeping genes actin (act) and ribosomal protein S3 (rps3). Relative gene expression values were calculated using the 2−ΔΔCt method. n = 3 (3 replicates of 5 larvae) for each time point of analysis within each treatment. Statistical comparisons were made via Welch's t-test. An asterisk indicates a significant difference between dsαCOP and dsGFP treatment. * = p < 0.05.
Survival monitoring showed significant reductions in survival, in larvae fed dsαCOP at 2.5 μg/μL, at 4 d (79% survival, df = 3, p = 0.041) and 5 d (63% survival, p = 0.02) after the start of the experiment, followed by marginal significance (47% survival, p = 0.07) at 6 d, compared to the dsGFP 5 μg/μL control treatment (Figure 2). At 7 d, survival of dsGFP control larvae dropped to 49% (dsαCOP 0.5 μg/μL = 39% survival; dsαCOP 2.5 μg/μL = 21% survival; dsαCOP 5 μg/μL = 62% survival). At no time point did mortality significantly differ between dsGFP control larvae and those fed with dsαCOP at either 0.5 or 5 μg/μL, even though marginally significant αCOP silencing was observed for both of the dsαCOP concentrations at 3 d.
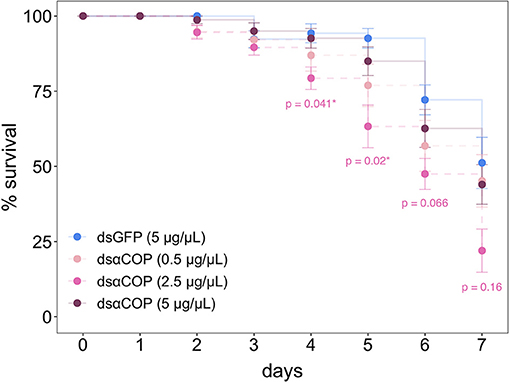
Figure 2. Survival curves, comparing the mortality effect of dsαCOP treatments to the dsGFP control treatment in B. aeneus larvae. Starting n = 10 (10 cages of 8 larvae) per treatment. Data were analyzed via Kruskal–Wallis test, followed by the Wilcoxon rank-sums test with post-hoc Bonferroni correction for multiple comparisons (error bars: ± SEM). An asterisk indicates a significant difference between dsαCOP and dsGFP treatment. * = p < 0.05.
Discussion
In a previous experiment comparing short-term- to chronic dsRNA feeding, B. aeneus adults that fed for 3 d on dsαCOP 2.5 μg/μL-treated anthers did not show significantly reduced survival (Willow et al., 2021). A significant reduction in B. aeneus adult survival was observed rather after 8 d of chronic feeding on dsαCOP 2.5 μg/μL-treated anthers, compared to the present study which showed significantly reduced survival at 4 d in larvae fed dsαCOP 2.5 μg/μL-treated anthers for 3 d. Thus, we here observed quicker RNAi-induced mortality in B. aeneus larvae, compared to what has been previously observed in B. aeneus adults that were fed comparable dsαCOP treatments. Quicker RNAi-induced mortality in earlier life stages has been observed in both the spotted wing drosophila (Drosophila suzukii; Taning et al., 2016) and emerald ash borer (Agrilus planipennis; Rodrigues et al., 2018). In the present study, significant αCOP silencing at 3 d, in B. aeneus larvae fed dsαCOP at 2.5 μg/μL, substantiates the interpretation that this significant mortality was indeed the result of αCOP silencing. Willow et al. (2021) also showed significantly greater mortality in B. aeneus adults chronically fed dsαCOP, compared to the mortality observed in short-term (3 d) treatments. Similar to the effect we observed in the present study regarding B. aeneus larvae fed dsαCOP 2.5 μg/μL-treated anthers, B. aeneus adults fed honey water at 1 and 3 μg dsαCOP/μL showed significant mortality at 6 d and 4 d, respectively (Willow et al., 2020b). Regarding B. aeneus adults, the quicker effect observed via honey water feeding, compared to anther feeding, may be attributed to the potentially lower total amount of dsRNA being consumed when coating edible plant parts with dsRNA, compared to feeding on a liquid solution consisting entirely of a near-equivalent concentration of dsRNA. The design of future RNAi studies with B. aeneus larvae should consider the potential difference in RNAi efficacy between short-term and chronic feeding on dsRNA-treated anthers. In addition, other field-relevant routes of oral exposure to B. aeneus-specific dsRNA should be examined in larvae, including oilseed rape buds sprayed with highly surface-active dsRNA formulations prior to larval emergence from buds; as well as bioengineered, B. aeneus-specific, RNAi cultivars of oilseed rape.
While larvae that fed on dsαCOP at both 0.5 and 5 μg/μL showed marginally significant αCOP silencing at 3 d, significant reductions in survival were never observed for these treatments. One reason for this could be the sudden increases in control mortality at 6 and 7 d. This potential confound coincides with 6 d qPCR results that suggest greater variability in αCOP expression at this time, compared to the somewhat expected results observed at 3 d. Nevertheless, a trend of increased αCOP silencing, with respect to dsαCOP treatments at 3 d, suggests the potential for developing an RNAi approach targeting B. aeneus larvae. We unexpectedly observed less mortality in larvae fed dsαCOP at 5 μg/μL compared to those fed dsαCOP at 2.5 μg/μL. As saturation of the RNAi machinery has yet to be shown to exist in insects, this cannot represent a possible explanation for our observation. In addition, previous studies on adult B. aeneus show clear negative relationships between survival and dsαCOP concentration (Willow et al., 2020a,b, 2021). Therefore, we cannot be certain as to what resulted in this observation in B. aeneus larvae; and further work must be done in order to address these uncertainties. Regarding the sudden increases in control mortality at 6 and 7 d, similar results were observed by Melander et al. (2003), which is to our knowledge the only other study performing insecticide bioassays with B. aeneus larvae. Previous experiments using the same gfp-specific control dsRNA showed no effect of this control dsRNA on B. aeneus adult mortality (Willow et al., 2020a,b, 2021). It is instead likely that B. aeneus larvae are very sensitive under unnatural conditions, and, consequently, high mortality can occur, as evidenced by both Melander et al. (2003) and the present study.
Refinements must be made to B. aeneus larval bioassay setups, in order to ensure optimal conditions for keeping B. aeneus larvae alive in a controlled environment. These refinements should strive to mimic conditions to which B. aeneus larvae are subjected under natural conditions. For example, in the field, B. aeneus larvae are able to seek refuge within flower petals, providing them a microhabitat that facilitates greater retention of moisture and less direct light. We removed this microhabitat from the feeding setup, for ease of dsRNA application and monitoring of larvae. If petals are removed in future studies, comparable microhabitats should be provided in the setup, or climate chamber conditions (e.g., relative humidity and light intensity) should be adjusted accordingly, in order to reduce potential stressors. Future studies aiming to examine, via anther feeding, the effect of dsRNA-based insecticides on B. aeneus larvae under more natural conditions, should consider spraying highly surface-active dsRNA formulations on oilseed rape raceme tips consisting of whole flower clusters and allowing cohorts of larvae to feed ad libitum in this type of semi-field-realistic bioassay setup.
Conclusion
In B. aeneus larvae, we observed significant αCOP silencing and corresponding αCOP silencing-induced mortality, via 3 d of feeding on dsαCOP 2.5 μg/μL-treated anthers of oilseed rape. These results suggest that, compared to what has previously been observed in B. aeneus adults, B. aeneus larvae may represent a more RNAi-sensitive life stage of this pest species. While larval mortality rates were variable between treatments, our observed trend of αCOP silencing via field-relevant dsRNA feeding suggests the potential for further research in RNAi targeting B. aeneus larvae. Further experiments examining the potential for RNAi-based oilseed rape protection against B. aeneus larvae should include semi-field-realistic bioassay setups not only through spraying dsRNA formulations on open flowers and closed buds containing B. aeneus larvae but also via the use of bioengineered RNAi cultivars for targeting both larval and adult B. aeneus. Finally, other target genes should be examined for potentially greater RNAi efficacy against B. aeneus.
Data Availability Statement
The raw data supporting the conclusions of this article will be made available by the authors, without undue reservation.
Author Contributions
JW and EV conceived the study. JW, LS, SS, RK, and EV designed the methods. JW, LS, SS, and RK performed the investigation. JW and AS analyzed and visualized the data. JW wrote the original draft. All authors contributed to revisions and approved the final manuscript.
Funding
The authors acknowledge financial support from the institutional Research Funding project no IUT36-2 of the Estonian Research Council, the ERA-NET C-IPM project IPM4Meligethes (project no. 3G0H0416 and 8T160091), the institutional Research Funding project no. PRG1056 of the Estonian Research Council, the Special Research Fund (BOF) of Ghent University, and the Research Foundation – Flanders (FWO-Vlaanderen).
Conflict of Interest
The authors declare that the research was conducted in the absence of any commercial or financial relationships that could be construed as a potential conflict of interest.
Acknowledgments
We thank the farm-owner whose land was used for collecting pollen beetle larvae and oilseed rape flowers. JW is the recipient of a PhD grant from the European Social Fund for Doctoral Students, as well as the Internationalisation Programme DoRa (carried out by the Archimedes Foundation). OC is a recipient of a postdoctoral fellowship from the Research Foundation – Flanders (FWO-Vlaanderen).
Supplementary Material
The Supplementary Material for this article can be found online at: https://www.frontiersin.org/articles/10.3389/fagro.2021.633120/full#supplementary-material
References
Cagliari, D., dos Santos, E. A., Dias, N., Smagghe, G., and Zotti, M. (2018). “Nontransformative strategies for RNAi in crop protection,” in Modulating Gene Expression - Abridging the RNAi and CRISPR-Cas9 Technologies, eds Khan MW and Singh A. doi: 10.5772/intechopen.80874
Melander, M., Åhman, I., Kamnert, I., and Strömdahl, A. C. (2003). Pea lectin expressed transgenically in oilseed rape reduces growth rate of pollen beetle larvae. Transgenic Res. 12, 555–567. doi: 10.1023/A:1025813526283
Miguel, K. S., and Scott, J. G. (2015). The next generation of insecticides: dsRNA is stable as a foliar-applied insecticide. Pest. Manag. Sci. 72, 801–809. doi: 10.1002/ps.4056
Osborne, P.. (1965). Morphology of the immature stages of Meligethes aeneus (F.) and M. viridescens (F.) (Coleoptera, Nitidulidae). Bull. Entomol. Res. 55, 747–759. doi: 10.1017/S0007485300049853
Petek, M., Coll, A., Ferenc, R., Razinger, J., and Gruden, K. (2020). Validating the potential of double-stranded RNA targeting Colorado potato beetle mesh gene in laboratory and field trials. Front. Plant Sci. 11:1250. doi: 10.3389/fpls.2020.01250
Rodrigues, T. B., Duan, J. J., Palli, S. R., and Rieske, L. K. (2018). Identification of highly effective target genes for RNAi-mediated control of emerald ash borer, Agrilus planipennis. Sci. Rep. 8:5020. doi: 10.1038/s41598-018-23216-6
Taning, C. N. T., Arpaia, S., Christiaens, O., Dietz-Pfeilstetter, A., Jones, H., Mezzetti, B., et al. (2019). RNA-based biocontrol compounds: current status and perspectives to reach the market. Pest. Manag. Sci. 76, 841–845. doi: 10.1002/ps.5686
Taning, C. N. T., Christiaens, O., Berkvens, N., Casteels, H., Maes, M., and Smagghe, G. (2016). Oral RNAi to control Drosophila suzukii: laboratory testing against larval and adult stages. J. Pest. Sci. 89:803–814. doi: 10.1007/s10340-016-0736-9
Willow, J., Soonvald, L., Sulg, S., Kaasik, R., Silva, A. I., Taning, C. N. T., et al. (2020a). First evidence of bud feeding-induced RNAi in a crop pest via exogenous application of dsRNA. Insects 11:769. doi: 10.3390/insects11110769
Willow, J., Soonvald, L., Sulg, S., Kaasik, R., Silva, A. I., Taning, C. N. T., et al. (2021). RNAi efficacy is enhanced by chronic dsRNA feeding in pollen beetle. Commun. Biol. 444. doi: 10.1038/s42003-021-01975-9
Keywords: RNA interference, RNAi, Brassicogethes aeneus, Meligethes aeneus, Brassica napus, biopesticide, insecticide, Coleoptera
Citation: Willow J, Soonvald L, Sulg S, Kaasik R, Silva AI, Taning CNT, Christiaens O, Smagghe G and Veromann E (2021) Anther-Feeding-Induced RNAi in Brassicogethes aeneus Larvae. Front. Agron. 3:633120. doi: 10.3389/fagro.2021.633120
Received: 24 November 2020; Accepted: 30 April 2021;
Published: 21 June 2021.
Edited by:
Ana M. Velez Arango, University of Nebraska-Lincoln, United StatesReviewed by:
Dimitrios N. Avtzis, Forest Research Institute and Hellenic Agricultural Organization, GreeceKe Wu, University of Florida, United States
Copyright © 2021 Willow, Soonvald, Sulg, Kaasik, Silva, Taning, Christiaens, Smagghe and Veromann. This is an open-access article distributed under the terms of the Creative Commons Attribution License (CC BY). The use, distribution or reproduction in other forums is permitted, provided the original author(s) and the copyright owner(s) are credited and that the original publication in this journal is cited, in accordance with accepted academic practice. No use, distribution or reproduction is permitted which does not comply with these terms.
*Correspondence: Jonathan Willow, jonathan@emu.ee; Eve Veromann, eve.veromann@emu.ee