- 1School of Natural Sciences, Bangor, United Kingdom
- 2Lancaster Environment Centre, Lancaster University, Lancaster, United Kingdom
- 3Faculty of Engineering and Environment, University of Southampton, Southampton, United Kingdom
- 4Crop & Soil Systems, SRUC Aberdeen Campus, Aberdeen, United Kingdom
- 5College of Agriculture Luiz de Queiroz-Escola Superior de Agricultura Luiz de Queiroz, University of São Paulo, Piracicaba, Brazil
- 6UWA School of Agriculture and Environment, The University of Western Australia, Perth, WA, Australia
Phosphorus (P) fertilizers have a dramatic effect on agricultural productivity, but conventional methods of application result in only limited recovery of the applied P. Given the increasing volatility in rock phosphate prices, more efficient strategies for P fertilizer use would be of economic and environmental benefit in the drive for sustainable intensification. This study used a combination of controlled-environment experiments and radioisotopic labeling to investigate the fertilizer use efficiency of a combination of seed (grain) dressing and foliar applications of P to spring wheat (Triticum aestivum L.). Radioisotopic labeling showed that the application of foliar P in the presence of photosynthetic light substantially increased both P-uptake into the leaf and P-mobilization within the plant, especially when an adjuvant was used. When compared with soil application of inorganic P buried into the rooting zone, a combination of a 3 μmol seed dressing and three successive 46.3 μmol plant−1 foliar applications were far more efficient at providing P fertilization benefits in P-limiting conditions. We conclude that a combination of seed dressing and foliar applications of P is potentially a better alternative to conventional soil-based application, offering greater efficiency in use of applied P both in terms of P-uptake rate and grain yield. Further work is required to evaluate whether these results can be obtained under a range of field conditions.
Introduction
Due to its low mobility in soil phosphorus (P) availability to plants is often limited, with corresponding constraints upon growth and yield. Low P availability can also negatively affect root growth (Drew, 1975; Li et al., 2020), with concurrent consequences for the acquisition of water and other nutrients (Takahashi and Anwar, 2007). Agriculture has compensated for this by applying high quantities of soluble inorganic P fertilizers, which has produced a significant positive effect on agricultural productivity, but often results in a negative effect on environmental quality (Zhang et al., 2015; Withers et al., 2020). This is particularly prevalent in wheat production systems where over fertilization of the soil leads to excessive P losses to surface and groundwater via surface run-off and leaching (Dodd and Sharpley, 2015). Strategies are therefore needed to use P fertilizer more efficiently in wheat cropping systems and to retain P in the soil after removal of the crop, for example, through the use of cover crops and residue management (Liu et al., 2019).
Conventional P-fertilizer strategies aim to maintain high levels of plant-available P in soil, ensuring that P does not become limiting during crop growth and development (Tunney et al., 1997; Moody, 2007; Valkama et al., 2011). This entails the application of the entire predicted plant P-offtake in a single dose before sowing to ensure that the soil P content is maintained above critical levels during all stages of crop growth (Syers et al., 2008). However, due in part to the ability of crop plants to acquire native and residual soil P, less than half of the applied fertilizer P is typically acquired by the current season's crop (Wang et al., 2016). The remainder is either immobilized in the soil (Stutter et al., 2012) or lost to the environment through leaching or run-off (Withers et al., 2001a, 2014). Whilst rock P prices remained low this inefficiency was not a significant issue for agriculture, however, with rock P prices both increasing and becoming far more volatile in recent years, this current approach to P fertilizer use appears to be unsustainable (Lyon et al., 2020). An alternative strategy of targeted P-fertilization has been proposed, whereby smaller quantities of P are applied with greater precision to meet crop demand (Simpson et al., 2011; Withers et al., 2014). Maximizing the recovery of residual or legacy soil P also helps to reduce the losses both to the soil and watercourses, thus improving the financial efficiency of the process, while reducing the risk of pollution.
One method of P fertilization that has exhibited potentially large gains in recovery of applied P within the crop over conventional soil fertilization is direct application to the leaf (Silberstein and Wittwer, 1951; Bindraban et al., 2020). The temporal flexibility of foliar P application relative to incorporating or placing P in the soil at the time of sowing offers the potential for P to be more accurately matched to the plant's increasing demand for P later in the growing season (Sutton et al., 1983; Allison et al., 2002). The benefits of foliar feeding, however, remain controversial, especially in terms of its impact on final grain yield (McBeath et al., 2020).
Foliar applied P is proposed to enter the leaf through direct stomatal penetration and through cuticular pores, although opinion differs as to their relative contributions (Noack et al., 2010). Stomatal entry requires that plant water status and atmospheric conditions maintain stomatal opening, but potentially promoted rapid P uptake. Movement though cuticular pores is a more complex process, with aqueous pores of <1 nm diameter formed around trichomes and cuticular ledges allowing the limited exchange of water and dissolved salts the environment (Schönherr, 2006). This process is far slower than stomatal penetration (Schönherr, 2006; Xie et al., 2020). The formulation of the foliar P product (especially pH, counter-ion, and adjuvant content) also has a major effect on its rate of uptake into the plant. Low solution pH increases the potential uptake rate achievable by reducing ionization of the orthophosphate molecule (pKa1 2.12; pKa2 7.21; pKa3 12.67), thus increasing its capacity to penetrate the leaf (Bouma, 1969). The identity of the cation used as the counter-ion to the P form has been suggested to affect the P uptake potential by altering the ability of the formulation to retain moisture (Koontz and Biddulph, 1957). Increasing the amount of time that the P is in solution on the leaf surface has been considered key to improving the rate of P uptake by changes in formulation of foliar P fertilizers (Koontz and Biddulph, 1957; Fernández and Brown, 2013). The major strategy for this has been the use of different types of adjuvants to increase the spread of droplets and increase retention of moisture; they may also have the benefit of increasing the permeability of the cuticle to P (Holloway, 1994). While these adjuvants have been shown to greatly increase foliar uptake of P, their phytotoxicity in high concentrations means care is required for their optimal use (Stein and Storey, 1986; Appah et al., 2020).
Having entered the leaf, foliar applied P is mobile throughout the plant, albeit to a lesser extent than that taken up by roots (Bastani and Hajiboland, 2017). Typically 30–40% translocated from the leaf to which it was applied (Barrier and Loomis, 1957; Koontz and Biddulph, 1957). Of particular importance is the translocation of foliar applied P to the meristematic tissues in both shoots and roots where high levels of nutrient consumption are essential for continued growth and development of the plant (Gordon-Weeks et al., 2003).
One difficulty in applying sufficient foliar P to satisfy plant demand is that all crop species are limited in the amount that can be applied in a single dose without scorching the leaf. This can reduce the plant's photosynthetic capacity and has particularly serious consequences in early growth stages (Gray, 1977; Barel and Black, 1979a,b; Noack et al., 2010). Winter wheat grown without any soil P-fertilizer has been shown to have optimum foliar dose rates of 2 kg ha−1 P at GS32 (stem elongation), and 4 kg ha−1 P at GS39 (flag leaf emerged), with foliar fertilization above these rates found to cause crop yield to decline to untreated control levels (Mosali et al., 2006). The benefit of repeated foliar applications of small amounts of P has also been demonstrated in other crops (Sohair et al., 2018).
Another major reason for past reluctance to use foliar P as a replacement for soil-applied P has been its failure, at the dosages used, to supply sufficient P at early growth stages to maximize a crop's yield potential. Such early supply of P to a crop has been shown to be essential to maximize both early growth and final yield (Grant et al., 2001). This negative effect can be compounded by low surface cover of the crop during early growth leading to a large proportion of applied foliar P falling straight onto the soil surface (Noack et al., 2010).
An approach to P that specifically addresses the need to supply P for early growth is the application of small quantities of P to the seed (grain) surface prior to sowing. This P is released very close to the developing root system, thus maximizing the capture of the applied P by the plant. However, the seed surface can only be coated with limited quantities of P, meaning that such treatments have not always been shown to be beneficial to final yields (Peske et al., 2009; Valluru et al., 2010). Greater success has been found by combining seed treatment with other, more substantial P application methods (e.g., conventional soil-based P fertilization). The application to the seed surface disproportionately reduces the plant's requirement for additional P-fertilizer and so significantly improves the overall efficiency of use (Sekiya and Yano, 2009).
The primary aim of this study was to evaluate whether P-fertilizer recovery and crop yield could be improved by replacing conventional soil-fertilizer use with a combined fertilization strategy that uses early and later growth stage P application, with minimum fertilizer contact with the soil: a combination of seed dressing and foliar application. Our second aim was to investigate the optimal timing and dose of seed dressing and foliar applications to maximize its contribution to plant P uptake.
Materials and Methods
Seed Dressing of P Fertilizer
For the seed dressing trial, wheat (Triticum aestivum L., cv. Paragon; bread wheat) was grown in 8 cm diameter opaque pots filled with 300 g of sandy loam textured soil (Eutric Cambisol; FAO, 2015). The pots had aeration holes in the base. The soil was collected from the Henfaes Experimental Station, Abergwyngregyn, UK (53°14′21.3″N, 4°0′50.3″W; 10 m above sea level), and had a 0.5 M NaHCO3 (pH 8.5) extractable P concentration (Olsen P) of 10 mg l−1 (Olsen et al., 1954). This is a potentially limiting level of plant available P in the soil according to the current UK soil assessment system for arable crops (Index 1 classification; AHDB, 2019). The same soil and wheat variety was used for all trials. Further details of the soil chemical, biological and physical properties can be found in Sánchez-Rodríguez et al. (2018). Seed dressings were applied to the seed coat using a micro-pipette and then air dried at 20°C for 2 h before planting. For this experiment, seeds were dressed with varying quantities (1, 2, 3, or 4 μmol P seed−1; 31–62 μg P seed−1) of a commercial potassium phosphate seed dressing solution (OMEX Agriculture Ltd., Lincoln, UK). In total 1.6 μl was added to the surface of each seed. The amount of K in each treatment was kept constant by adding the corresponding amount of KCl (for each K-PO4 dose) so that each seed received 4.6 μmol K in total. Based on sowing density of 350 seeds m−2 (HGCA, 2000), this equates to a P application dose of 0.43 kg P ha−1. For the positive controls, the readily soluble P-fertilizers diammonium phosphate (DAP) or triple super phosphate (TSP) were applied at 885 μmol P per pot based on 35 kg P ha−1 equivalent as per UK government recommendations for this soil (AHDB, 2019). The dose was scaled down to a 10 cm diameter circle to ensure standardization of fertilizer input per plant with the other experiments where the pot diameter was different (see below). This fertilizer was placed in the soil at 3 cm below the depth of the planted seeds, and offset from the seed laterally by 1 cm. Three seeds were planted in each pot at 1 cm depth, and at emergence two seedlings were removed to leave only the largest seedling per pot. The pots were kept in a climate-controlled glasshouse with artificial lighting (light intensity = 260 μmol m−2 s−1 PAR) set to 20°C and minimum 16 h day length. Soil water holding capacity was measured according to the saturation-drainage method of Verheijen et al. (2019). The pots were placed in a completely randomized design (n = 4) and maintained at 80% of their water holding capacity by weighing the pots three times a week (Mon, Wed, Fri), measuring the weight loss due to evaporation and transpiration and then replacing the water lost. To ensure that P was the only limiting macronutrient, the equivalent of 60 kg ha−1 N (as NH4NO3) and 60 kg ha−1 K2O (as KCl) were applied in solution form to each pot at seedling emergence. Other nutrients were controlled by the weekly application of 10 ml of a modified Hoagland's nutrient solution containing: 5 mM Ca; 3.87 mM Fe; 3.87 μM Na; 765 μM Zn; 2 mM SO4; 320 nM Cu; 46.3 nM B; 500 μM Mo; 9.1 nM Mn; 18 μM Cl; 38.7 μM EDTA (Talboys et al., 2014). At 25 d after planting, the whole plant was extracted from the pot, and the root systems washed free from soil using a stainless steel mesh and a stream of deionised-water (Paudel et al., 2018). The soil-free root systems were then floated in water-filled transparent plastic trays, and scanned using a flatbed scanner (Perfection 4990 Photo; Epson Electronics America Inc., San Jose, CA, USA). The resulting image was processed using WinRhizo® software (Regent Instruments Inc., Chemin Ste-Foy, Quebec, Canada) to determine the length of seminal and lateral root for each plant. The boundary conditions used by the software were that roots ≥0.350 mm in diameter were classified as seminal roots, and <0.350 mm were classed as lateral roots (Heppell et al., 2014). The plants were then dried at 85°C overnight, weighed, and dry-ashed (550°C, 16 h). The residue was dissolved in 0.5 M HCl and then their P content determined using the ascorbate/molybdate blue method of Murphy and Riley (1962).
Foliar Application of Radioisotopically Labeled P Fertilizer
Wheat seedlings were grown in the same growth conditions as described above, but with no seed dressing. The pots were placed in a completely randomized design (n = 5). Once they had three leaves (GS13) they were laid horizontally with one leaf lightly affixed to a clean bench surface using electrical tape. A 16 mm2 square was then marked out using petroleum jelly on either the underside or the top leaf surface. 3 μl of a commercial ammonium phosphate foliar fertilizer (6.62 M P; OMEX Agriculture Ltd.) labeled with 22.2 MBq ml−1 33P (American Radiolabeled Chemicals Inc., St Louis, MO, USA) was then applied to the leaf surface, either mixed with 0.1% (v/v) of the adjuvant IA-500 (Interagro Ltd., Braintree, UK) or without, and left for 16 h with artificial illumination (light intensity = 260 μmol m−2 s−1 PAR representative of a cloudy day in the UK summer). Experiments to assess the effect of daylight were conducted in the same manner, but all treatments had 0.1% (v/v) IA-500 and dark treatments were covered to eliminate the presence of light and the level of replication was n = 3. After 16 h, the seedlings were dried at 80°C and the 33P distribution in the plant imaged using a Cyclone Plus phosphor imaging system (Perkin-Elmer Inc., Waltham, MA, USA) with an exposure time of 15 min. The dried plants were then dry-ashed (550°C, 16 h), the residue dissolved in 0.5 M HCl and the 33P content of the resulting solution quantified using a Wallac 1404 scintillation counter (Perkin-Elmer Inc.).
Effects of Seed and Foliar P Fertilization of Plants Grown to Maturity
For an experiment in which wheat plants were grown to mature grain harvest, seeds were planted in either 11 cm diameter, 30 cm deep pots containing 3 kg of sandy loam textured soil (as described previously but with an Olsen P-value of 29 mg l−1; national Index 3; AHDB, 2019), or in 10 cm diameter pots, 8.5 cm deep pots containing 500 g of the original sandy loam soil (Olsen P 10 mg l−1; national Index 1; AHDB, 2019). Based on the Olsen P-values and national guidelines (AHDB, 2019), these two experiments provided both extreme P limiting (500 g pots) and P-sufficient (3 kg pots) environmental conditions. For the seed dressing treatment, potassium phosphate (3.0 μmol P seed−1; OMEX Agriculture Ltd.) was applied to the seed coat as described above. In all other treatments, the seeds received KCl so that the addition of K was the same (3.45 μmol K seed−1) for both the P-treated and P-untreated seeds. For the positive controls, diammonium phosphate (DAP) or triple super phosphate (TSP) was applied at 885 μmol P per pot at a rate equivalent to 35 kg P ha−1. The fertilizer addition and plant growth conditions are as described in section Seed Dressing of P Fertilizer. To ensure that P was the only limiting macronutrient, the equivalent of 60 kg ha−1 N (as NH4NO3) and 100 kg ha−1 K2O (as KCl) was applied in liquid form to each pot at seedling emergence, with another 60 kg ha−1 N applied at the stem extension growth stage (GS30). Micronutrients were controlled by the weekly application of 10 ml of Hoagland's solution as used in the seed dressing experiments detailed above. The pots were placed in a completely randomized design (n = 4) and maintained on a self-watering bench with capillary matting to ensure that soil moisture was never limiting.
Foliar applications were performed at Zadoks growth stages GS13 (three leaf), GS31 (stem extension) and GS39 (flag leaf) (Zadoks et al., 1974). These applications were pipetted onto the leaves of each plant using a micro-pipette, and manually spread such that they covered as much leaf as possible. A spray was not used so that we could be certain that all the applied P dose remained on the surface of the leaves. Equal quantities were applied to the top and bottom of the leaf, and applications were conducted in the morning so that there was a minimum of 8 h of photosynthetic light directly afterwards to maximize initial uptake rates. Treatments were either the ammonium phosphate solution described above (OMEX Agriculture Ltd.) for the P treatments or an NH4Cl solution control for all other plants to ensure equal application of foliar NH4+, both mixed with 0.1% (v/v) of the adjuvant IA-500 (Interagro Ltd.). Each dose consisted of 70 μmol N plant−1 and for the P treatments 46.3 μmol P plant−1 with the amount applied following commercial recommendations (Omex, 2020). Based on a final field establishment of 275 plants m−2 (HGCA, 2000), this equates to a P dose of 4 kg P ha−1. Once the grains had hardened, they were judged mature, and the entire aerial portion of each plant was harvested and the grain removed. The fresh weight of the grain yield and total biomass were then determined before drying at 85°C overnight. The dried tissue was then re-weighed, dry-ashed (550°C, 16 h), the residue dissolved in 0.5 M HCl and then their P content determined using the ascorbate/molybdate blue method of Murphy and Riley (1962). In the results section all masses are quoted as dry weight.
Statistical Analysis
P recovery (% of P applied) was calculated as plant P content of the treated plants minus the average P content of the control (zero P) plants, divided by the amount of fertilizer P applied. All the growth and fertilizer experiments had a completely randomized design and replicated at n = 3 or greater. Statistical testing was performed using Student's unpaired t-test in Microsoft Excel (Microsoft Corp., Redwood City, CA) or by one-way or two-way ANOVA with Tukey post-hoc pairwise comparisons using Minitab v17 (Minitab Inc., State College, PA). p < 0.05 was used as the cut-off value for statistical significance.
Results
Seed Dressing With P Is an Efficient Way to Promote Early Growth and Uptake
Seed dressing with 2, 3, or 4 μmol P per seed showed significant benefits in total P uptake by 25 d (of 59–108%) over control plants that had received no P addition (Figure 1A). For the 3 and 4 μmol P dosages there was no significant difference in P uptake compared with plants grown in soil to which 885 μmol P (35 kg P ha−1) had been added in the form of TSP or DAP. Seed dressing at rates of 1, 3, and 4 μmol P seed−1 also produced significantly greater total lateral root length (by 52–79%) over no-P controls, although data for 2 μmol P dosages were not significant (Figure 1B). All four seed treatment doses produced lateral root lengths that did not differ significantly from the soil DAP/TSP treatments (they ranged from 14 to 28% lower than the average of the TSP and DSP treatments). For seminal root lengths, only the soil DAP/TSP treatments produced significant increases over the controls. As a consequence of the much lower P doses per plant applied in the seed dressings than the soil application treatments, the rate of recovery of applied P was much greater in the seed coat treatments; it did not show a linear response with dose: 90% for 1 μmol, 207% for 2 μmol, 252% for 3 μmol, and 185% for 4 μmol (compared with 1.23% for TSP and 1.16% for DAP). These recovery rates suggest considerable soil immobilization of fertilizer P in the TSP and DAP treatments.
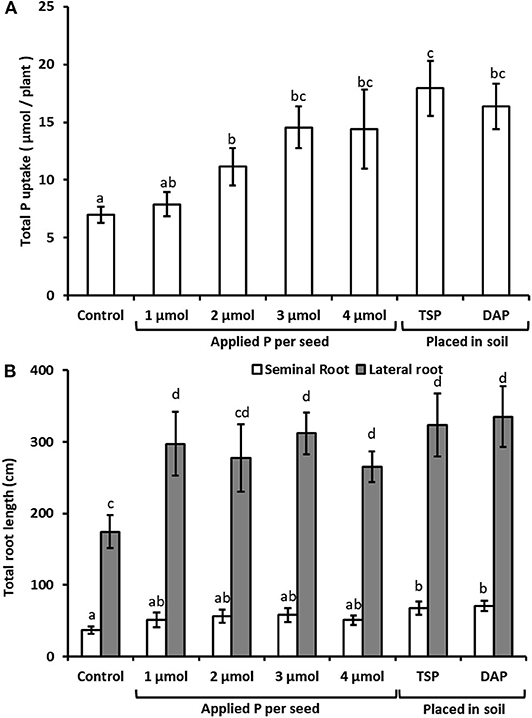
Figure 1. Micro-molar concentration P seed dressings enhance root production and P uptake in Triticum aestivum. Panel (A) shows total length of seminal and lateral roots per plant, after 25 d when using seed P dressings or placed soil P fertilizer compared with an untreated control. Placed fertilizer treatments equated to 885 μmol P plant−1. Panel (B) shows total plant P uptake of the plants reported in Panel (A), expressed as total P acquired per plant. n = 4 for each treatment except for the 4 μmol seed dressings where n = 3. Lower case letters indicate values that differ significantly at the p < 0.05 level (one-way ANOVA). Error bars are standard errors of the mean (SEM). The seed treatment was potassium phosphate, the soil treatments were granules of TSP (triple super phosphate) and DAP (diammonium phosphate).
Foliar P Entry Is Enhanced by Adjuvant and Photosynthetic Activity
The amendment of the foliar P solution with the adjuvant IA-500 resulted in a significant increase in the rate at which P was capable of entering the plant through the top surface of the leaf for plants grown for 16 h in the light (Figure 2). There was, however, no significant difference in the entry rate of P applied to the leaf underside when using IA-500 compared to when it was absent. Grain yield in t ha−1 was estimated by scaling up the pots to the field scale according to HGCA (2000).
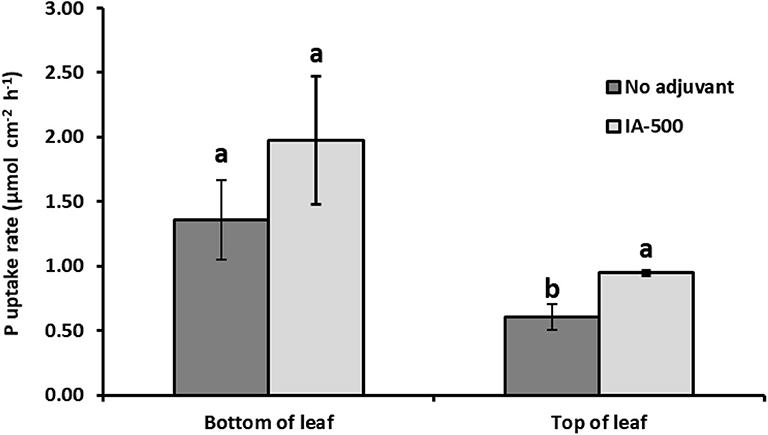
Figure 2. Effect of the adjuvant IA-500 upon the uptake rate of foliar P into Triticum aestivum leaves in photosynthetic light. Average uptake rates into the whole plant of 33P after application to a 16 mm2 area of either the bottom or top surface of one leaf over 16 h. Treatments incorporated either no adjuvant (dark bars) or 0.1% v/v IA-500 (light bars). n = 5 for each treatment, lower case letters mark values significantly different from each other at the p < 0.05 level (two-way ANOVA). Error bars are standard errors of the mean (SEM).
Illumination of seedlings with 260 μmol m−2 s−1 PAR for 16 h, following application of foliar P formulations incorporating the adjuvant IA-500 to the leaf underside, significantly increased both the uptake of P through the lower leaf surface into the whole plant (Figure 3A), and the subsequent translocation of the applied P through the plant (Figures 3B,C), compared with seedlings incubated in the dark.
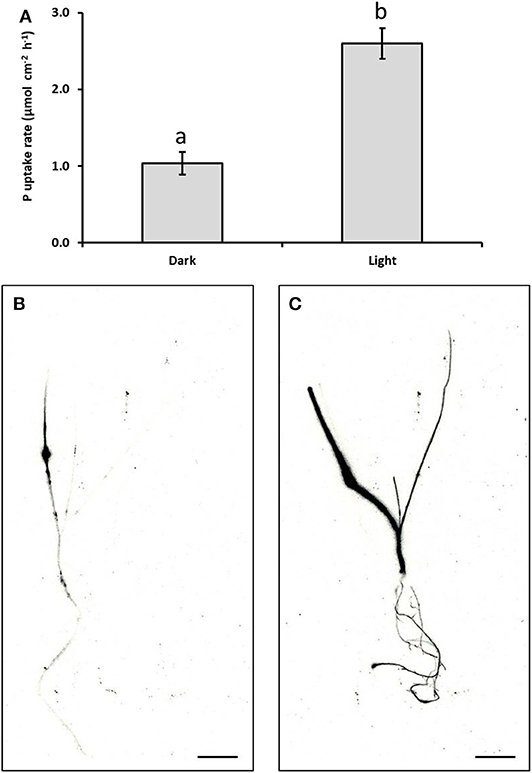
Figure 3. Effect of photosynthetic light upon the uptake rate of foliar P into Triticum aestivum leaves. Panel (A) shows the average uptakes rates of 33P into the whole plant (n = 3). Panels (B,C) show images of the distribution of 33P in whole wheat seedlings displaying the mobility of 33P following its application to a 16 mm2 area of the bottom surface of one leaf over 16 h when placed in either the light [Panel (B)] or dark [Panel (C)]. Scale bars are 2 cm. Lower case letters mark values significantly different from each other at the p < 0.05 level (unpaired t-test). Error bars are standard errors of the mean (SEM).
Combining Seed Dressing and Foliar Applications Can Replicate the P-Uptake and Grain Yield Achieved With Placed Soil Fertilization and Improve P Recovery
In the two pot experiments in which the wheat plants were grown to grain yield maturity, the use of the two forms of readily soluble, soil-applied P-fertilizers (TSP and DAP, respectively) at a rate equivalent to 35 kg P ha−1 produced significant increases (by ≥79%) in total plant P uptake over the no-P addition controls (Figures 4A, 5A). In comparison to the control, an increase in P-uptake (by ≥104%) was also observed in both experiments when P seed dressings of 3 μmol seed−1 were combined with three foliar applications of 46.3 μmol plant−1; this treatment also produced uptake rates not significantly different from the soil applied P treatment in both experiments (Figures 4A, 5A). In the experiment using a smaller soil volume (and less intrinsic soil P), there was also a significant increase (by 119%) in P uptake over the no-P controls for the treatment with the three foliar P applications without seed P dressing (Figure 4A), whereas this was not the case for the experiment using the larger soil volume with a greater soil P concentration (Figure 5A).
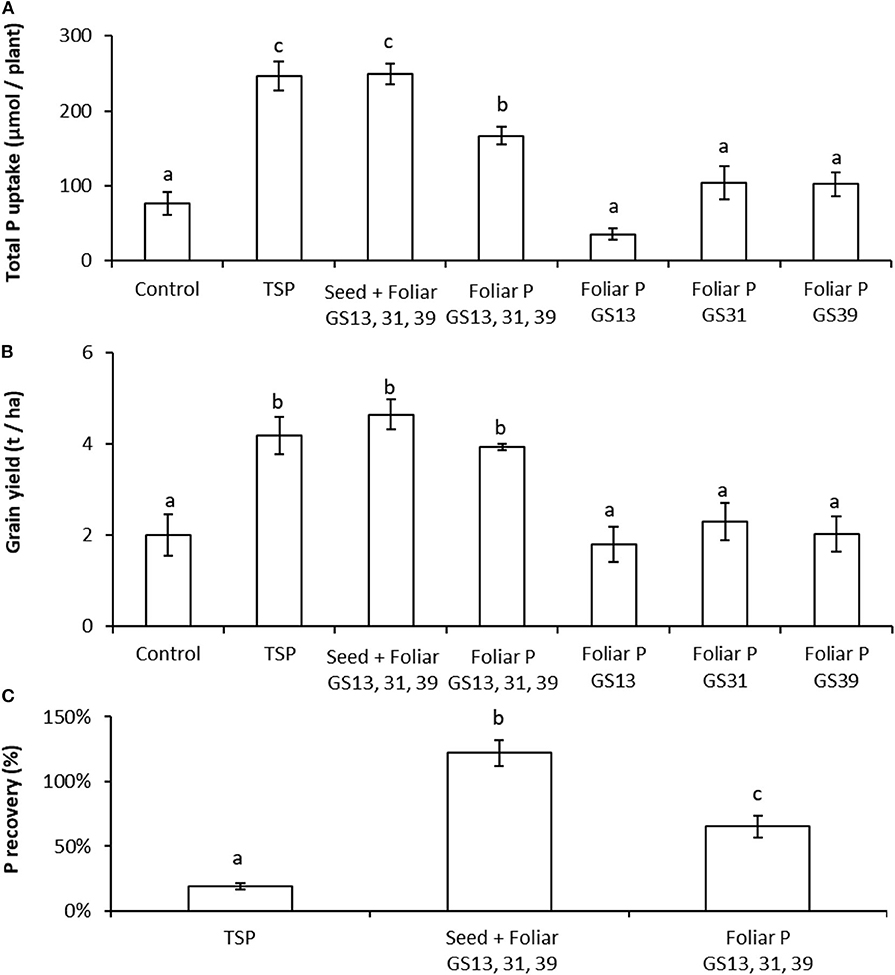
Figure 4. Combining foliar P application with seed P dressing can produce comparable yields of Triticum aestivum to soil P fertilization with greater P recovery rates over the untreated control in P-limiting conditions. Panel (A): Total P content of soil grown wheat plants grown to maturity with P-fertilization regimes of untreated control; triple super phosphate (TSP) granules placed in soil (885 μmol P plant−1); single or triple applications of foliar P (46.3 μmol P plant−1 application−1); and seed dressing (3 μmol P plant−1) combined with three foliar applications (46.3 μmol P plant−1 application−1). Panel (B): Grain yields scaled to t ha−1 for the treatments reported in (A). Panel (C): Recovery rates of all the applied P in the whole plant for the treatments that produced significant differences to the control in (A). Values represent means ± SEM (n = 4) with lowercase letters showing significant differences between treatments (p < 0.05; one-way ANOVA).
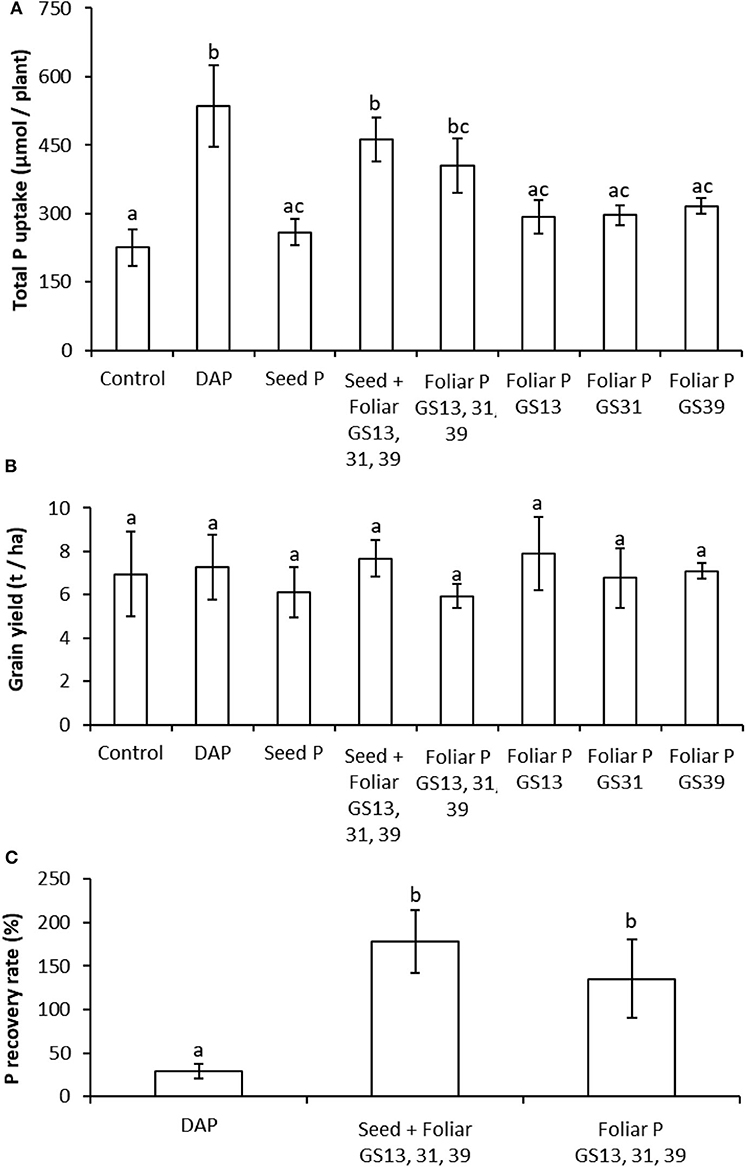
Figure 5. Combining foliar P application with seed P dressing can produce comparable yields of Triticum aestivum to soil P fertilization with greater P recovery rates over the untreated control in P-sufficient conditions. Panel (A): Total P content of wheat plants grown to maturity in 3 kg sandy loam soil. P-fertilization treatments were untreated controls, di-ammonium phosphate (DAP) granules placed in soil (885 μmol P plant−1); single or triple applications of foliar P (46.3 μmol P plant−1 application−1); seed dressings (3 μmol P plant−1) combined with three foliar applications (46.3 μmol P plant−1 application−1). Panel (B): Grain yields scaled to t ha−1 for the treatments reported in (A). Panel (C): Recovery rates of the applied P in the whole plant for the treatments that produced significant differences to the control in (A). Values represent means ± SEM (n = 4) with lowercase letters showing significant differences between treatments (p < 0.05; one-way ANOVA).
In the smaller soil volume, grain yield was significantly greater than the no-P controls for the treatments using soil applied P (TSP), seed dressing combined with three foliar applications and three foliar applications alone, respectively (Figure 4B). There were no significant differences in grain yield for any of the treatments in the larger soil volume. Applications of foliar P at a single growth stage produced no significant increases in P uptake or grain yield over the no-P controls in either experiment.
In both experiments, the recovery rate of P in the whole plant as a percentage of the amount of applied P was much greater (by ≥240%) in both the treatments with three foliar P applications alone and with seed dressing combined with the three foliar applications over the soil applied P treatments (Figures 4C, 5C). In the smaller soil volume the seed dressing combined with three foliar applications treatment produced a significantly greater P recovery rate (by 87%) than the three foliar applications alone treatment, whereas this difference was not significant in the larger soil volume.
Discussion
Evidence From 33P Labeling Allows Optimisation of Foliar P Application Strategy
Most previous studies on P foliar fertilizers have relied on increases in growth and leaf 31P content to measure the success of different foliar treatments. Using a combination of 31P and 33P isotopes, this study has provided direct evidence that the use of an adjuvant in foliar P formulations causes an increase in P uptake following application to the upper leaf surface, confirming its value for promoting P uptake rates into the leaf (Noack et al., 2010). We also showed that the applied P has the ability to penetrate the cuticle of the upper leaf surface, and especially the lower leaf surface, even without amendment with an adjuvant. Our result contrasts strongly with that of Peirce et al. (2014) who found that more P uptake occurred when fertilizer was applied to the leaf surface. In our case, we attribute foliar entry to the presence of stomata which are present on both sides of T. aestivum leaves at similar densities (Zhu et al., 2018). Trichomes are a significant barrier to leaf surface penetration, reducing the contact area of droplets with the leaf surface (Brewer et al., 1991). The increased uptake rates on the lower side of the leaf we observed can be attributed to T. aestivum leaves having a lower density of trichomes here compared to the upper surface (Fernández et al., 2014). In addition, our results are consistent with photosynthesis and transpiration rates being higher in stomata on the underside of cereal leaves (Driscoll et al., 2006). The important role of stomatal conductance in transfer of foliar applied P into the plant can also be inferred from the positive effect of growing plants in photosynthetic light (compared with the dark); stomata are known to open in response to light (Dietrich, 2001). Growing plants in photosynthetic light also increased the mobility of the foliar applied P within the plant, which is likely to be linked to the increased flux of organic compounds into the phloem observed during active photosynthesis (Giaquinta, 1978). Phosphorus could be carried into the phloem this way either via increased esterification of applied P or through simple mass flow delivering the P to the vicinity of vascular tissues. It is unclear whether the increased P mobilization from the leaf enhances the rate of P entry into the leaf tissue through maintaining a stronger concentration gradient, or vice versa. Nonetheless, given that foliar scorch has been attributed to high nutrient imbalance at the area of foliar fertilizer application (Marschner, 1995), high mobilization rates of foliar P from the leaf may enable higher dosage applications. Our results also indicate that foliar sprays may work better when applied in the early morning to maximize uptake, although clearly this needs further testing at the field scale. It should be noted that there is no overall consensus in the literature on the optimal timing for foliar applications with the optimal response window in the day being dependent on crop type, prevailing weather conditions and nutrient (Fageria et al., 2009). However, results with other nutrients (e.g., N, Se) have indicated maximal uptake rates in the morning or evening in comparison to the middle of the day (Kyllingsbaek, 1980; Liu et al., 2012).
Uptake Efficiency of a Combination of Seed Dressing and Foliar Applications Is Greater Than for Soil Applied P Fertilizer
Application of P as a seed dressing at any of the doses used in the experiment increased lateral root production to a similar extent as did its application in the soil at ≥221 times the dose rate. However, seed application tended not to have the same magnitude of benefit to soil application in seminal root growth. The enhancement of lateral root growth is likely to be particularly important in enabling the exploitation of reserves of P and other mineral nutrients in a larger soil volume (Zhu and Lynch, 2004). This benefit is likely to be especially important for uptake of P because of its low mobility in the soil, and given the increase in P uptake over controls is greater than the quantity applied as a seed dressing, a high proportion of P taken up by the plants in the experiment is likely to have come from soil reserves. These effects of enhanced early root production, and concurrent enhancements in P uptake are consistent with the findings of previous studies in other plant species (Valluru et al., 2010), and point to seed dressings being a valuable tool in increasing P-fertilizer use efficiency.
The combination of a P seed dressing and three subsequent foliar applications resulted in high rates of P uptake (equivalent to those achieved with much larger doses of soil applied fertilizer) in both pot experiments. The added advantage of combining seed with foliar application was, however, not consistent between the two experiments. The benefit over foliar application alone was clear when plants were grown in a smaller soil volume (500 g per plant) with low intrinsic soil P reserves. However, when plants were grown in a larger soil volume with a much higher available P reserves, the combined seed and foliar application was better than the seed-only application but did not lead to a significantly greater P uptake than the foliar-only treatment. When T. aestivum seedlings are grown under severe P stress, they have been shown to have a markedly reduced ability to acquire P from foliar applications (Fernández et al., 2014). This could possibly explain our results, where seed dressing P was required to maintain the plants' ability to uptake foliar P when grown in small soil volumes, but in larger soil volumes, P supply was maintained by the higher soil P status regardless of seed treatments.
The effectiveness of seed plus foliar or foliar applications alone was greatly enhanced in the P-limiting conditions of a small volume of low P soil (Figure 4) compared to the P-sufficient conditions of a large volume of high P soil (Figure 5). In P-sufficient conditions, foliar applications enhanced total plant uptake of P (Figure 5A) with greater efficiency than soil fertilization (Figure 5C), but consistent with other studies in high P soils (Bai et al., 2013), there was no effect of P application on grain yield (Figure 5B) (McBeath et al., 2020). We attribute this to the P in the high P soil becoming available progressively during the growing period due to the mineralization of organic P reserves in the soil and greater exploitation of native soil P reserves as the root system develops.
The pot experiments were all performed using foliar P loading rates of 46.3 μmol plant−1 for each application at GS13, GS31, and GS39. Previous work by Mosali et al. (2006) has shown that under field conditions, winter wheat had higher optimal dose rates at an equivalent to our experiments of 50.7 μmol plant−1 dosage−1 at GS32 and 101.3 μmol plant−1 dosage−1 at GS39. These applications did not include an adjuvant in the formulation. The total quantity of foliar P applied per plant combining all three applications in our experiments (138.9 μmol) is just 8.6% less than the optimal dose for two applications found by Mosali et al. (2006) in their field experiment (152.0 μmol). In reality, the quantity applied to the leaf surface is likely to have been much greater in our experiment as all of the foliar P was applied directly to the leaves together with an adjuvant, rather than being sprayed onto the crop where a proportion can fall onto the soil without contacting the crop. Our plants showed no negative effects of applying such quantities of P early in the growing season. This indicates that our combination of adjuvant supplementation and application of foliar P at the beginning of the day, to maximize initial uptake rates, appeared to prevent any negative effects related to foliar scorch. In addition, the addition of IA-500 into the formulation will have considerably reduced the ability of leaf trichomes to suspend droplets above the leaf surface. This presumably also reduced the already limited possibilities for optically produced foliar scorch, as this is caused by such droplets rather than the solution in contact with the leaf surface (Egri et al., 2010).
Limits to the level of P-fertilization possible though foliar application, due to the constraints of foliar scorch, have previously limited their usefulness to situations where other factors such as environmental conditions have depressed yields to sub-optimal levels, with crop P demand reduced concurrently to a level that foliar P can satisfy (Mosali et al., 2006; Noack et al., 2010). That we have demonstrated the capacity to apply larger quantities of P to leaves resulting in grain yields not significantly different from those obtained by conventional soil fertilization illustrates that foliar application of P may be a more versatile strategy than previously thought.
In our experiments, a much higher dose of soil applied P fertilizer produced no advantage over the other treatments in terms of grain yield. Important evidence to explain this result is that the rate of recovery in the plant of applied P was significantly much larger in the foliar, and especially the combined seed with foliar, applications than for the soil application. When correcting for the amount of P uptake by untreated control plants, the rate of fertilizer recovery for the seed dressings with foliar applications is consistently close to 100% in both experiments. It is also notable that the combination of seed and foliar application produced a higher mean P recovery rate than foliar application alone in both experiments, significantly so in the larger-soil volume experiment. The advantage of direct and precise application of much smaller doses of P to the seed and leaves over conventional soil application is a reduction in the quantity of P immobilized in the soil for long periods of time. This advantage of this precision is likely to be both spatial and temporal: the specific targeting of P application to times of greatest plant demand is expected to have enhanced the ability of the plant to it take up and utilize it (Withers et al., 2014).
The capacity of crops to take up larger quantities of P than that applied in fertilizer during the current growing season, which was demonstrated by this study, is important for strategies to increase the sustainability of food production. The study demonstrated that precise application of small quantities of P fertilizer to seed and leaves led to a much greater magnitude of P uptake from soil P reserves. While we have no evidence about the chemical form of soil P accessed by the plants, their enhanced rate of lateral root growth is likely to have been important for this outcome. Direct effects of organic acids exuded from these roots (Ström et al., 2002) or indirect effects via rhizosphere microbes (Rodriguez and Fraga, 1999) or mycorrhizal fungi (Smith et al., 2011) can play an important role in enabling plant uptake of insoluble forms of P that have been immobilized in the soil matrix (Withers et al., 2014). Through such mechanisms, these soil P reserves can maintain a gradually diminishing supply of plant-available P for over a century (Blake et al., 2003). The importance of such a low dose seed and foliar P fertilization strategy for more sustainable production over the medium-term is particularly important in countries such as the UK, where high levels of historical P fertilizer application to agricultural soils have built up large P reserves in excess of immediate crop nutritional requirements (Withers et al., 2001b). Even when this eventually reduces soil P reserves, or if novel crop varieties are produced that have a greater P demand, this strategy has the potential to be adapted to increase the rate of P supply. This could be achieved by increasing the number of P foliar applications per growing season, or by its combination with application of more sustainable sources of slow-release P fertilizer to the soil, such as struvite (Massey et al., 2009).
Conclusion
This study provides evidence that a combination of seed dressing and foliar applications could be an effective alternative P-fertilization strategy for spring wheat. The combination of a seed dressing dosage that promotes early vigor with foliar P applications which include an adjuvant produced a P nutrition response comparable to conventional soil P fertilization at much smaller (≤16%) rates of P application. This approach minimizes the contact of fertilizer P with the soil, and thus minimizes soil immobilization into less available forms of P, with potential benefit for increased fertilizer P-use efficiency and reduced risk of pollution. In addition, it promotes crop use of intrinsic soil P reserves. This strategy therefore has the potential to increase the efficiency of both future use of fertilizer P resources and the exploitation of the soil legacy of past P fertilization in agriculture, and so can play an important role in the sustainable intensification of food production. Further work is now required to evaluate whether these results can be obtained under a range of field conditions (e.g., soil, crop, and weather combinations).
Data Availability Statement
The raw data supporting the conclusions of this article will be made available by the authors, without undue reservation.
Author Contributions
PT, JH, PW, and DJ designed the research. PT conducted the research. PT and DJ collected and analyzed the data. PT wrote the first draft of the manuscript. All authors contributed to the final version of the manuscript.
Funding
The authors would like to thank the UK Department for Environment, Food and Rural Affairs, Biotechnology and Biological Sciences Research Council, and Scottish Government for funding this work as a component of the Sustainable Arable LINK project (LK09136).
Conflict of Interest
The authors declare that the research was conducted in the absence of any commercial or financial relationships that could be construed as a potential conflict of interest.
Acknowledgments
We would like to thank Roger Sylvester-Bradley (ADAS), Robin Walker (SRUC), and Alison Rollett (ADAS) for their scientific input to the work and Ian Elliot and David Booty at Omex Agriculture Ltd for the provision of the fertilizer products. This manuscript has been released as a report at https://ahdb.org.uk/improving-the-sustainability-of-phosphorus-use-in-arable-farming-targeted-p (AHDB, 2016).
References
AHDB (2016). Improving the Sustainability of Phosphorus Use in Arable Farming – ‘Targeted P’. Project Report PR569. Agriculture and Horticulture Development Board, Kenilworth, UK.
AHDB (2019). Nutrient Management Guide (RB209). Kenilworth, UK: Agriculture and Horticulture Development Board.
Allison, M. F., Fowler, J. H., and Allen, E. J. (2002). Effects of soil- and foliar-applied phosphorus fertilizers on the potato (Solanum tuberosum) crop. J. Agric. Sci. 137, 379–395. doi: 10.1017/S0021859601001526
Appah, S., Jia, W. D., Ou, M. X., Wang, P., and Asante, E. A. (2020). Analysis of potential impaction and phytotoxicity of surfactant-plant surface interaction in pesticide application. Crop Protect. 127:104961. doi: 10.1016/j.cropro.2019.104961
Bai, Z., Li, H., Yang, X., Zhou, B., Shi, X., Wang, B., et al. (2013). The critical soil P levels for crop yield, soil fertility and environmental safety in different soil types. Plant Soil 372, 27–37. doi: 10.1007/s11104-013-1696-y
Barel, D., and Black, C. A. (1979a). Foliar application of P. I. Screening of various inorganic and organic P compounds. Agron. J. 71, 15–21. doi: 10.2134/agronj1979.00021962007100010004x
Barel, D., and Black, C. A. (1979b). Foliar application of P. II. Yield responses of corn and soybeans sprayed with various condensed phosphates and P-N compounds in greenhouse and field experiments. Agron. J. 71, 21–24. doi: 10.2134/agronj1979.00021962007100010005x
Barrier, G. E., and Loomis, W. E. (1957). Absorption and translocation of 2,4-dichlorophenoxyacetic acid and P by leaves. Plant Physiol. 32, 225–231. doi: 10.1104/pp.32.3.225
Bastani, S., and Hajiboland, R. (2017). Uptake and utilization of applied phosphorus in oilseed rape (Brassica napus L. cv. Hayola) plants at vegetative and reproductive stages: comparison of root with foliar phosphorus application. Soil Sci. Plant Nutr. 63, 254–263. doi: 10.1080/00380768.2017.1321471
Bindraban, P. S., Dimkpa, C. O., and Pandey, R. (2020). Exploring phosphorus fertilizers and fertilization strategies for improved human and environmental health. Biol. Fertil. Soils 56, 299–317. doi: 10.1007/s00374-019-01430-2
Blake, L., Johnston, A. E., Poulton, P. R., and Goulding, K. W. T. (2003). Changes in soil phosphorus fractions following positive and negative phosphorus balances for long periods. Plant Soil 254, 245–261. doi: 10.1023/A:1025544817872
Bouma, D. (1969). The response of subterranean clover (Trifolium subterraneum L.) to foliar applications of phosphorus. Aust. J. Agric. Res. 20, 435–445. doi: 10.1071/AR9690435
Brewer, C. A., Smith, W. K., and Vogelmann, T. C. (1991). Functional interaction between leaf trichomes, leaf wettability and the optical properties of water droplets. Plant Cell Environ. 14, 955–962. doi: 10.1111/j.1365-3040.1991.tb00965.x
Dietrich, P. (2001). The role of ion channels in light-dependent stomatal opening. J. Exp. Bot. 52, 1959–1967. doi: 10.1093/jexbot/52.363.1959
Dodd, R. J., and Sharpley, A. N. (2015). Recognizing the role of soil organic phosphorus in soil fertility and water quality. Resour. Conserv. Recycl. 105, 282–293. doi: 10.1016/j.resconrec.2015.10.001
Drew, M. C. (1975). Comparison of the effects of a localised supply of phosphate, nitrate, ammonium and potassium on the growth of the seminal root system, and the shoot in Barley. New Phytologist 75, 479–490. doi: 10.1111/j.1469-8137.1975.tb01409.x
Driscoll, S. P., Prins, A., Olmos, E., Kunert, K. J., and Foyer, C. H. (2006). Specification of adaxial and abaxial stomata epidermal structure and photosynthesis to CO2 enrichment in maize leaves. J. Exp. Bot. 57, 381–390. doi: 10.1093/jxb/erj030
Egri, A., Horváth, A., Kriska, G., and Horváth, G. (2010). Optics of sunlit water drops on leaves, conditions under which sunburn is possible. New Phytologist 185, 979–987. doi: 10.1111/j.1469-8137.2009.03150.x
Fageria, N. K., Barbosa Filho, M. P., Moreira, A., and Guimarães, C. M. (2009). Foliar fertilization of crop plants. J. Plant Nutr. 32, 1044–1064. doi: 10.1080/01904160902872826
FAO (2015). World Reference Base for Soil Resources 2014, update 2015 International Soil Classification System for Naming Soils and Creating Legends for Soil Maps. IUSS Working Group WRB World Soil Resources Reports No. 106. FAO, Rome.
Fernández, V., and Brown, P. H. (2013). From plant surface to plant metabolism, the uncertain fate of foliar-applied nutrients. Front. Plant Sci. 4:289. doi: 10.3389/fpls.2013.00289
Fernández, V., Guzmán, P., Peirce, C. A. E., McBeath, T. M., Khayet, M., and McLaughlin, M. J. (2014). Effect of wheat phosphorus status on leaf surface properties and permeability to foliar-applied phosphorus. Plant Soil 384, 7–20. doi: 10.1007/s11104-014-2052-6
Giaquinta, R. (1978). Source and sink leaf metabolism in relation to phloem translocation. Plant Physiol 61, 380–385. doi: 10.1104/pp.61.3.380
Gordon-Weeks, R., Tong, Y. P., Davies, T. G. E., and Leggewie, G. (2003). Restricted spatial expression of a high-affinity phosphate transporter in potato roots. J. Cell Sci. 116, 3135–3144. doi: 10.1242/jcs.00615
Grant, C. A., Flaten, D. N., Tomasiewicz, D. J., and Sheppard, S. C. (2001). The importance of early season phosphorus nutrition. Can. J. Plant Sci. 81, 211–224. doi: 10.4141/P00-093
Gray, R. C. (1977). Foliar fertilization with primary nutrients during the reproductive stage of plant growth. Proc. Fertilizer Soc. 164, 1–23.
Heppell, J., Talboys, P., Payvandi, S., Zygalakis, K. C., Fliege, J., Jones, D. L., et al. (2014). How changing root system architecture can help tackle a reduction in soil phosphate (P) levels for better plant P acquisition. Plant Cell Environ. 38, 118–128. doi: 10.1111/pce.12376
HGCA (2000). Optimum Winter Wheat Plant Population. Topic Sheet 32. Home-Grown Cereals Authority, London, UK.
Holloway, P. J. (1994). “Physicochemical factors influencing the adjuvant-enhanced spray deposition and coverage of foliage-applied agrochemicals,” in Interactions Between Adjuvants, Agrochemicals and Target Organisms, Ernst Schering Research Foundation Workshop Volume 12, eds P. J. Holloway, R. T. Rees, and D. Stock (Berlin: Springer-Verlag), 83–106. doi: 10.1007/978-3-662-02988-6_4
Koontz, H., and Biddulph, O. (1957). Factors affecting absorption and translocation of foliar applied phosphorus. Plant Physiol. 32, 463–470. doi: 10.1104/pp.32.5.463
Kyllingsbaek, A. (1980). Absorption of urea by barley plants after foliar fertilization at different times during the day and night. Tidsskrift for Planteavl 84, 343–348.
Li, C. H., Wang, J. D., and Zhang, Y. C. (2020). Root growth and phosphorus efficiency among sweet potato genotypes under low phosphorus. J. Plant Nutr. 43, 1320–1330. doi: 10.1080/01904167.2020.1729803
Liu, C. J., Liu, F. G., Chen, W., Zhu, D. Y., Li, D. J., Liu, C. Q., et al. (2012). Effects of selenium foliar spraying on its accumulation in fresh corn. Jiangsu J. Agric. Sci. 28, 713–716.
Liu, J., Macrae, M. L., Elliott, J. A., Baulch, H. M., Wilson, H. F., and Kleinman, P. J. A. (2019). Impacts of cover crops and crop residues on phosphorus losses in cold climates: a review. J. Environ. Qual. 48, 850–868. doi: 10.2134/jeq2019.03.0119
Lyon, C., Cordell, D., Jacobs, B., Martin-Ortega, J., Marshall, R., Camargo-Valero, M. A., et al. (2020). Five pillars for stakeholder analyses in sustainability transformations: the global case of phosphorus. Environ. Sci. Pol. 107, 80–89. doi: 10.1016/j.envsci.2020.02.019
Massey, M. S., Davis, J. G., Ippolito, J., and Sheffield, R. E. (2009). Effectiveness of recovered magnesium phosphates as fertilizers in neutral and slightly alkaline soils. Agron. J. 101, 323–329. doi: 10.2134/agronj2008.0144
McBeath, T. M., Facelli, E., Peirce, C. A. E., Arachchige, V. K., and McLaughlin, M. J. (2020). Assessment of foliar-applied phosphorus fertiliser formulations to enhance phosphorus nutrition and grain production in wheat. Crop Pasture Sci. 71, 795–806. doi: 10.1071/CP20241
Moody, P. W. (2007). Interpretation of a single-point P buffering index for adjusting critical levels of the Colwell soil P test. Aust. J. Soil Res. 45, 55–62. doi: 10.1071/SR06056
Mosali, J., Desta, K., Teal, R. K., Freeman, K. W., Martin, K. L., Lawles, J. W., et al. (2006). Effect of foliar application of phosphorus on winter wheat grain yield, phosphorus uptake, and use efficiency. J. Plant Nutr. 29, 2147–2163. doi: 10.1080/01904160600972811
Murphy, J., and Riley, J. P. (1962). A modified single solution method for the determination of phosphate in natural waters. Anal. Chim. Acta 27, 31–36. doi: 10.1016/S0003-2670(00)88444-5
Noack, S. R., McBeath, T. M., and McLaughlin, M. J. (2010). Potential for foliar phosphorus fertilisation of dryland cereal crops: a review. Crop Pasture Sci. 61, 659–669. doi: 10.1071/CP10080
Olsen, S. R., Cole, C. V., Watanabe, F. S., and Dean, L. A. (1954). Estimation of Available Phosphorus in Soils by Extraction With Sodium Bicarbonate. Circular 939, United States Department of Agriculture, Washington DC, USA.
Paudel, I., Bar-Tal, A., Rotbart, N., Aphrath, A., and Cohen, S. (2018). Water quality changes seasonal variations in root respiration, xylem CO2, and sap pH in citrus orchards. Agric. Water Manage. 197, 147–157. doi: 10.1016/j.agwat.2017.11.007
Peirce, C. A. E., McBeath, T. M., and Fernandez, V. (2014). Wheat leaf properties affecting the absorption and subsequent translocation of foliar-applied phosphoric acid fertiliser. Plant Soil 384, 37–51. doi: 10.1007/s11104-014-2245-z
Peske, F. B., Baudet, L., and Peske, S. T. (2009). Yield of soybean plants derived from seeds coated with phosphorus. Revista Brasileira de Sementes 31, 95–101. doi: 10.1590/S0101-31222009000100011
Rodriguez, H., and Fraga, R. (1999). Phosphate solubilizing bacteria and their role in plant growth promotion. Biotechnol. Adv. 17, 319–339. doi: 10.1016/S0734-9750(99)00014-2
Sánchez-Rodríguez, A. R., Carswell, A. M., Shaw, R., Hunt, J., Saunders, K., Cotton, J., et al. (2018). Advanced processing of food waste based digestate for mitigating nitrogen losses in a winter wheat crop. Front. Sustain Food Syst. 2:35. doi: 10.3389/fsufs.2018.00035
Schönherr, J. (2006). Characterization of aqueous pores in plant cuticles and permeation of ionic solutes. J. Exp. Bot. 57, 2471–2491. doi: 10.1093/jxb/erj217
Sekiya, N., and Yano, K. (2009). Seed P-enrichment as an effective P supply to wheat. Plant Soil 327, 347–354. doi: 10.1007/s11104-009-0058-2
Silberstein, O., and Wittwer, S. H. (1951). Foliar applications of phosphatic fertilizers to vegetable crops. Proc. Am. Soc. Hortic. Sci. 58, 179–180.
Simpson, R. J., Oberson, A., Culvenor, R. A., Ryan, M. H., Veneklaas, E. J., Lambers, H., et al. (2011). Strategies and agronomic interventions to improve the phosphorus-use efficiency of farming systems. Plant Soil 349, 89–120. doi: 10.1007/s11104-011-0880-1
Smith, S. E., Jakobsen, I., Grønlund, M., and Smith, F. A. (2011). Roles of arbuscular mycorrhizas in plant phosphorus nutrition, interactions between pathways of phosphorus uptake in arbuscular mycorrhizal roots Have important implications for understanding and manipulating plant phosphorus acquisition. Plant Physiol. 156, 1050–1057. doi: 10.1104/pp.111.174581
Sohair, E. D. E., Abdall, A. A., Amany, M. A., and Houda, A. R. (2018). Effect of nitrogen, phosphorus and potassium nano fertilizers with different application times, methods and rates on some growth parameters of Egyptian cotton (Gossypium barbadense L.). Bioscience Res. 15, 549–564.
Stein, L. A., and Storey, J. B. (1986). Influence of adjuvants on foliar absorption of nitrogen and phosphorus by soybeans. Proc. Am. Soc. Hortic. Sci. 111, 829–832.
Ström, L., Owen, A. G., Godbold, D. L., and Jones, D. L. (2002). Organic acid mediated P mobilization in the rhizosphere and uptake by maize roots. Soil Biol. Biochem. 34, 703–710. doi: 10.1016/S0038-0717(01)00235-8
Stutter, M. I., Shand, C. A., George, T. S., Blackwell, M. S. A., Bol, R., Mackay, R. L., et al. (2012). Recovering phosphorus from soil, a root solution? Environ. Sci. Technol. 46, 1977–1978. doi: 10.1021/es2044745
Sutton, P. J., Peterson, G. A., and Sander, D. H. (1983). Dry matter production in tops and roots of winter wheat as affected by phosphorus availability during various growth stages. Agron. J. 75, 657–663. doi: 10.2134/agronj1983.00021962007500040019x
Syers, J. J. K., Johnston, A. E., and Curtin, D. (2008). Efficiency of Soil and Fertilizer Phosphorus Use. Rome: Food and Agriculture Organization of the United Nations.
Takahashi, S., and Anwar, M. R. (2007). Wheat grain yield, phosphorus uptake and soil phosphorus fraction after 23 years of annual fertilizer application to an Andosol. Field Crops Res. 101, 160–171. doi: 10.1016/j.fcr.2006.11.003
Talboys, P. J., Owen, D. W., Healey, J. R., MWithers, P. A. W., and Jones, D. L. (2014). Auxin secretion by Bacillus amyloliquefaciens FZB42 both stimulates root exudation and limits phosphorus uptake in Triticum aestivum. BMC Plant Biol. 14:51. doi: 10.1186/1471-2229-14-51
Tunney, H., Breeuwsma, A., Withers, P. J. A., and Ehlert, P. A. I. (1997). “Phosphorus fertilizer strategies, present and future,” in Phosphorus Loss From Soil to Water, eds H. Tunney, O. T. Carton, P. C. Brookes, and A. E. Johnston (Wallingford: CAB International), 177–203.
Valkama, E., Uusitalo, R., and Turtola, E. (2011). Yield response models to phosphorus application: a research synthesis of Finnish field trials to optimize fertilizer P use of cereals. Nutr. Cycl. Agroecosystems 91, 1–15. doi: 10.1007/s10705-011-9434-4
Valluru, R., Vadez, V., Hash, C. T., and Karanam, P. (2010). A minute P application contributes to a better establishment of pearl millet (Pennisetum glaucum (L.) R. Br.) seedling in P deficient soils. Soil Use Manage. 26, 36–43. doi: 10.1111/j.1475-2743.2009.00245.x
Verheijen, F. G. A., Zhuravel, A., Silva, F. C., Amaro, A., Ben-Hur, M., and Keizer, J. J. (2019). The influence of biochar particle size and concentration on bulk density and maximum water holding capacity of sandy vs sandy loam soil in a column experiment. Geoderma 347, 194–202. doi: 10.1016/j.geoderma.2019.03.044
Wang, F. M., Rose, T., Jeong, K., Kretzschmar, T., and Wissuwa, M. (2016). The knowns and unknowns of phosphorus loading into grains, and implications for phosphorus efficiency in cropping systems. J. Exp. Bot. 67, 1221–1229. doi: 10.1093/jxb/erv517
Withers, P. J. A., Clay, S., and Breeze, V. (2001a). Phosphorus transfer in runoff following application of fertilizer, manure, and sewage sludge. J. Environ. Qual. 30, 180–188. doi: 10.2134/jeq2001.301180x
Withers, P. J. A., Edwards, A. C., and Foy, R. H. (2001b). Phosphorus cycling in UK agriculture and implications for phosphorus loss from soil. Soil Use Manage. 17, 139–149. doi: 10.1079/SUM200181
Withers, P. J. A., Forber, K. G., Lyon, C., Rothwell, S., Doody, D. G., Jarvie, H. P., et al. (2020). Towards resolving the phosphorus chaos created by food systems. Ambio 49, 1076–1089. doi: 10.1007/s13280-019-01255-1
Withers, P. J. A., Sylvester-Bradley, R., Jones, D. L., Healey, J. R., and Talboys, P. J. (2014). Feed the crop not the soil: rethinking phosphorus management in the food chain. Environ. Sci. Technol. 48, 6523–6530. doi: 10.1021/es501670j
Xie, R. H., Zhao, J. Q., Lu, L. L., Brown, P., Guo, J. S., and Tian, S. K. (2020). Penetration of foliar-applied Zn and its impact on apple plant nutrition status: in vivo evaluation by synchrotron-based X-ray fluorescence microscopy. Horticult. Res. 7:147. doi: 10.1038/s41438-020-00369-y
Zadoks, J. C., Chang, T. T., and Konzak, C. F. (1974). A decimal code for the growth stages of cereals. Weed Res. 14, 415–421. doi: 10.1111/j.1365-3180.1974.tb01084.x
Zhang, X. N., Guo, Q. P., Shen, X. X., Yu, S. W., and Qiu, G. Y. (2015). Water quality, agriculture and food safety in China: current situation, trends, interdependencies, and management. J. Integr. Agric. 14, 2365–2379. doi: 10.1016/S2095-3119(15)61128-5
Zhu, J., and Lynch, J. P. (2004). The contribution of lateral rooting to phosphorus acquisition efficiency in maize (Zea mays) seedlings. Funct. Plant Biol. 31, 949–958. doi: 10.1071/FP04046
Keywords: crop nutrition, foliar feeding, food security, integrated nutrient management, precision agriculture, fertilizer management, phosphorus use efficiency (PUE)
Citation: Talboys PJ, Healey JR, Withers PJA, Roose T, Edwards AC, Pavinato PS and Jones DL (2020) Combining Seed Dressing and Foliar Applications of Phosphorus Fertilizer Can Give Similar Crop Growth and Yield Benefits to Soil Applications Together With Greater Recovery Rates. Front. Agron. 2:605655. doi: 10.3389/fagro.2020.605655
Received: 12 September 2020; Accepted: 18 November 2020;
Published: 04 December 2020.
Edited by:
Magdi T. Abdelhamid, National Research Centre, EgyptReviewed by:
Mohammed Mohammed El Hawary, Field Crop Research Institute, EgyptZakaria Fouad Abdalla, National Research Centre, Egypt
Saad Farouk, Mansoura Universiy, Egypt
Copyright © 2020 Talboys, Healey, Withers, Roose, Edwards, Pavinato and Jones. This is an open-access article distributed under the terms of the Creative Commons Attribution License (CC BY). The use, distribution or reproduction in other forums is permitted, provided the original author(s) and the copyright owner(s) are credited and that the original publication in this journal is cited, in accordance with accepted academic practice. No use, distribution or reproduction is permitted which does not comply with these terms.
*Correspondence: Davey L. Jones, d.jones@bangor.ac.uk