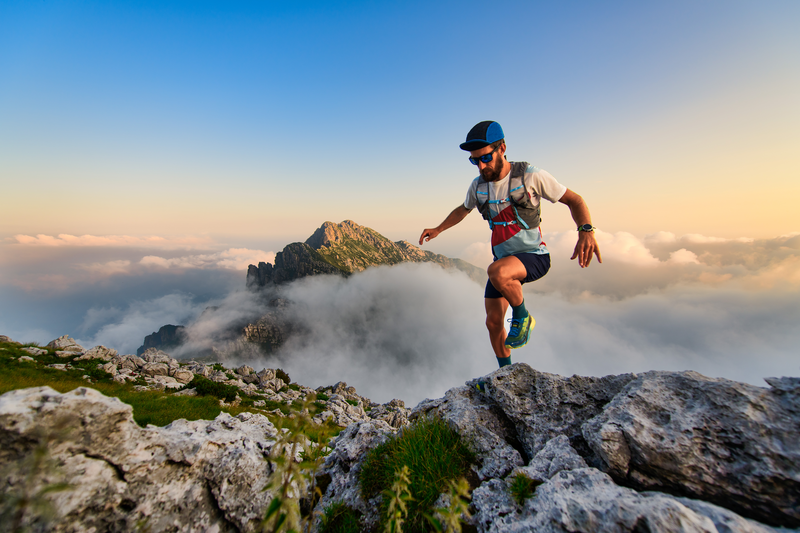
94% of researchers rate our articles as excellent or good
Learn more about the work of our research integrity team to safeguard the quality of each article we publish.
Find out more
ORIGINAL RESEARCH article
Front. Agron. , 28 January 2021
Sec. Weed Management
Volume 2 - 2020 | https://doi.org/10.3389/fagro.2020.598426
This article is part of the Research Topic Weed Biology and Ecology in Agroecosystems View all 13 articles
As resistance to herbicides limits growers' weed management options, integrated weed management (IWM) systems that combine non-chemical tactics with herbicides are becoming critical. A 2 year integrated weed management (IWM) study was conducted at three locations in VA, USA. The factorial study evaluated: (1) soybean planting date (early or late planted) (2) with or without winter cover (cereal rye/wheat or no cover), and (3) with or without harvest weed seed control (HWSC). Prior to soybean planting in the first year, winter cover resulted in a 22% reduction in common ragweed density compared to no cover. At soybean harvest in the first year, the lowest common ragweed densities were in the late planted plots following winter wheat, and common ragweed aboveground biomass was reduced by 46 and 22% at two locations in late planted compared to early planted soybean. To evaluate the impact of the first year's treatments and HWSC, full season soybeans were planted across the trial in the second year. Prior to soybean planting in the second year, late planting in the first year common ragweed density was reduced by 83% at one location, but significant reductions were not observed elsewhere. When comparing winter cover to no cover, common ragweed densities were reduced by 31 and 49% at two locations and densities were similar at the third location. Harvest weed seed control reduced common ragweed density by 43% at one location compared to the conventional harvest plots but no significant reductions were observed at the other locations or at other rating timings. However, there was a significant location by planting date by winter cover interaction and the overall lowest common ragweed densities (4.1 to 10.3 plants m−2) were in the late planted plots with winter cover. This research indicated that winter cover, late planting, and HWSC can reduce common ragweed populations with late planting being the most influential. Therefore, double-cropping soybean after wheat is likely the most viable means to better control common ragweed using IWM as it combines both winter cover and late planting date.
As herbicide resistance in weed species continues to develop (Heap, 2020) there is a need to develop new integrated weed management (IWM) strategies for weed control systems that rely a multi-tactic approach to control weeds (Swanton and Weise, 1991; Thill et al., 1991). A multi-tactic approach is crucial, as weeds can quickly adapt to high selection pressures from a single tactic approach (Thill et al., 1991; Norsworthy et al., 2012). To combat the growing problem of herbicide resistance in the USA, farmers need to rapidly adopt IWM strategies (Redlick et al., 2017). Weed control tactics that can be components of an IWM system include using cover crops, tillage, cultural practices, harvest weed seed control (HWSC), and herbicide programs, among others (Swanton and Weise, 1991).
Common ragweed is a major problem in the Mid-Atlantic soybean production region (Scruggs et al., 2019) due to resistance to four different sites of action (SOA) including groups 2, 5, 9, and 14 (Heap, 2020). There are also biotypes reported to be multiple resistant to groups 2 and 9, 2, and 14, and 2, 9, and 14 in several states around the USA (Heap, 2020). Coupled with the fact that common ragweed presents significant allergenic risk, common ragweed is listed as the ninth most common and troublesome weed in all broadleaf crops, and the number seventh most troublesome weed in soybeans by the Weed Science Society of America (WSSA) (Van Wychen, 2016). A common ragweed density as low as 4 plants 10 m−1 row reduced soybean yield up to 132 kg ha−1 and when left uncontrolled all-season, soybean yield can be reduced up to 62%, with densities of up to 160 plants m−2 (Coble et al., 1981). An IWM approach is needed to limit the potential impact common ragweed can have on soybean production.
Weed scientists in the USA are adapting HWSC strategies developed in Australia as part of an IWM approach (Norsworthy et al., 2016; Beam et al., 2019; Shergill et al., 2020). HWSC removes or kills seed that are retained on the mother plant with harvest operations (Walsh et al., 2013). There are several HWSC systems including narrow windrow burning, direct bale, chaff removal, chaff lining, and seed impact mills (i.e., the integrated Harrington Seed Destructor and Seed Terminator) (Walsh et al., 2013). All of these systems are being used commercially in Australia (Walsh et al., 2017a) where they are similarly effective on rigid ryegrass (Lolium rigidum Gaud.) (Walsh et al., 2017b), and some are being used on an experimental basis by early adopters in the USA (Schwartz-Lazaro et al., 2017; Tidemann et al., 2017; Walsh et al., 2018; Soni et al., 2019; Shergill et al., 2020). If weed seed has already shattered and is on the ground, HWSC is not effective (Walsh and Powles, 2014). Common ragweed seed retention was generally 80% or more at soybean harvest in the mid-Atlantic region (Schwartz-Lazaro et al., 2020). Beam et al. (2019) reported 22 to 26% reductions in common ragweed density in the growing season following HWSC. Both reports indicate potential for HWSC to manage common ragweed.
Agronomic cultural practices can also be deployed to further impact summer annual weeds like common ragweed including crop rotation, planting date, and row spacing. To give crops a competitive advantage over weeds, well-adapted genetics and agronomic practices need to be used. Delaying crop planting can result in lower weed densities due to asynchrony with weed emergence periodicity. Common ragweed emerges earlier than most summer annual weeds in the mid-Atlantic and has a shorter emergence periodicity. The majority of common ragweed emergence (>90%) occurs by mid-May in many regions of the USA (Myers et al., 2014; Werle et al., 2014; Barnes et al., 2017), prior to when double crop soybean is typically planted. Due to this early germination window, double-cropping soybean after wheat harvest is a tool that may be utilized to manage common ragweed in the mid-Atlantic region. Reducing soybean row width from 72 to 38 cm also increases crop competitiveness against weeds (Norsworthy et al., 2012) and can even lower weed fecundity (Chandler et al., 2001).
Cover crops are another tactic as part of an IWM program that can impact weeds. Cover crops suppress weeds physically (light, temperature, and impedance), nutritively (nitrogen immobilization) and chemically via allelochemicals (Moore et al., 1984; Barnes and Putnam, 1987; Teasdale and Mohler, 1993, 2000; Teasdale et al., 2012; Mirsky et al., 2013; Wells et al., 2017; Williams et al., 2018). Cereal rye has been shown to consistently produce the most biomass of fall-planted grass cover crops (Finney et al., 2009; Mirsky et al., 2013). While there is compelling evidence in the literature that cover crops can suppress weeds, suppression of summer annual weeds is variable driven by cover crop biomass levels, weed species, and management (Teasdale and Mirsky, 2015; Wallace et al., 2018).
There is also only limited research on the interaction between cover crops and other weed control tactics (Teasdale et al., 2005; Ryan et al., 2011a,b; Nord et al., 2012; Norsworthy et al., 2016; Hay et al., 2019), specifically cover crops with HWSC, despite numerous calls for such research (Swanton and Weise, 1991; Thill et al., 1991; Swanton et al., 2008; Harker and O'Donovan, 2013). It is likely that integration of management techniques that target various common ragweed life stages will provide better control than tactics used individually. The objective of this research was to evaluate integrated common ragweed management strategies in soybean including planting time, use of a cover crop, and HWSC.
A 2 year study to evaluate integrated approaches to common ragweed management in soybean was conducted at three locations in Virginia, USA. Locations included Kentland Farm in Blacksburg initiated in 2016, a grower's field in Lawrenceville initiated in 2016, and the Southern Piedmont Agricultural Research and Extension Center in Blackstone initiated in 2017. Soil types were a Ross loam (Fine-loamy, mixed, superactive, mesic Cumulic Hapludolls) with a pH of 6.6 and 3.4% organic matter. At Blacksburg (37°11'40.2 “N 80°34'16.6” W; 510 m), an Emporia sandy loam (Fine-loamy, siliceous, subactive, thermic Typic Haludults) with a pH of 5.48 and 0.9% organic matter at Lawrenceville (36°39'01.2 “N 77°49'34.2” W; 101 m), and an Appling sandy loam (Fine, kaolinitic, thermic, Typic Kanhapludults) with a pH of 6.42 and 3% organic matter at Blackstone (37°04'59.6 “N 77°58'19.5” W; 125 m). Blacksburg and Blackstone sites did not have a naturalized common ragweed population, so these sites were over seeded with common ragweed in late fall prior to the initiation of the experiment. These seeds were harvested from the Lawrenceville location in an area outside but adjacent to the study. This population of common ragweed was approximately 30% glyphosate resistant, based on glyphosate response in replicated research trials adjacent to this study. Common ragweed seed were spread using a rotary spreader at ~11.6 million seed ha−1 on November 11, 2016 and November 15, 2017 at Blacksburg and Blackstone, respectively. This rate of common ragweed seed was used to ensure a dense uniform stand and account for potential low germination of ripe dormant seed (Willemsen, 1975; Baskin and Baskin, 1977).
Experiments were a factorial design with 3 factors, each with 2 levels and 5 replications and arranged as a randomized complete block. Factors included (1) soybean planting date, (2) ± winter cover, and (3) ± HWSC. Soybean planting dates were in mid- to late May, to represent early planted soybean or early July to represent late planted soybean (Table 1). Winter cover was either cereal rye, planted in the fall prior to early planted soybean, or wheat planted in the fall and harvested prior to late planted soybean. Plots without winter cover were left fallow over the winter. HWSC was implemented at the end of the soybean growing season as described by Beam et al. (2019). All crop residues and weed seeds contained therein were removed from the plot. Using similar methods, Matthews et al. (1996) found that of the rigid ryegrass seeds that enter the combine between 75 and 85% were collected in the chaff cart and up to 94% of wild mustard (Sinapis arvensis L.) seeds were captured. Plots without HWSC had crop residues evenly distributed back across the plot as with a standard harvest operation. Fertility, herbicide programs, planting dates, row spacing, crop varieties, and other practices were selected to mimic standard production practices for the region and are described below and in Table 1. While these differences between treatments certainly impacted results, it makes the results directly applicable to farmers by putting results into the management context of production agriculture. All herbicide applications were made using a 6-nozzle boom with 45.7 cm nozzle spacing equipped with XR11002 nozzles calibrated to apply 140 L ha−1 of spray solution. All plots measured 4.57 by 7.62 m.
Table 1. Dates for cover crop and soybean planting, cover crop termination, POST herbicide application, and soybean harvest for all locations and years of the experiment.
Cereal rye, variety not stated (Southern States Cooperative, Richmond VA, USA), was drilled on 16.5 cm spacing at 134 kg ha−1. Cereal rye planting date, along with other termination, planting, and harvesting dates are located in Table 1. Cereal rye was terminated 2 wk before soybean planting using a roller crimper and glyphosate (Roundup Powermax, Monsanto Co, St. Louis, MO, USA) at 1,126 g ae ha−1 plus 2,4-D (Shredder Amine 4, WinField Solutions LLC, St. Paul, MN, USA) at 532 g ae ha−1 plus flumioxazin (Valor SX, Valent USA Corp., Walnut Creek, CA, USA) at 89.25 g ai ha−1. Early planted soybean plots that had no winter cover received the same herbicide application as the plots with cereal rye. Soybeans were planted into the early planted plots in rows on 76 cm centers, at 407,550 seed ha−1 (AG48X7 in 2017 and AG56X8 in 2018, Monsanto Co., St. Louis MO, USA) with 6 rows per plot. At planting, glufosinate (Liberty 280 SL, Bayer CropScience LP, Research Triangle Park, NC, USA) was applied at 59.38 g ai ha−1 plus ammonium sulfate at 1.68 kg ha−1 (Spray Grade Ammonium Sulfate, DSM Chemicals North America, Inc., Augusta, GA, USA) and crop oil concentrate (Crop Oil Concentrate, Southern States Cooperative, Richmond, VA, USA) at 1% v v−1. Early planted plots both with and without winter cover residue were fertilized at soybean planting with 56 kg ha−1 of P2O5 and 56 kg ha−1 of K2O. When common ragweed average height reached 30 cm tall in the no cover plots, a POST application of glyphosate plus fomesafen (Flexstar GT 3.5, Syngenta Crop Protection LLC, Greensboro, NC, USA) at 1,107 g ae plus 274 g ai ha−1 plus non-ionic surfactant (Scanner, Loveland Products, Greeley, CO, USA) at 0.25% v v−1 was made. The POST herbicide application timing was late by design, ensuring that not all common ragweed plants were controlled by the herbicide program and allowing all treatment effects to be measured, but still realistically similar to what often occurs in grower fields. Early planted soybean harvest occurred and HWSC treatments implemented in the fall of the year. Yield, however, was not measured due to poor soybean stand from drought and deer herbivory at all locations.
Winter wheat was drilled (SS8340 in 2017, Southern States Cooperative, Richmond, VA, USA and Hilliard in 2018, Featherstone Seed, Amelia, VA, USA) at 134 kg ha−1, on 16.5 cm spacing (Table 1). Plots with a wheat cover crop had 56 kg ha−1 of N and thifensulfuron (Harmony SG, Corteva, Indianapolis, IN, USA) applied at 26.25 g ai ha−1 plus non-ionic surfactant at 0.25% v v−1 in late winter. Wheat was harvested in June of each year (Table 1). Late planted plots that had a wheat cover or that had been left fallow had glufosinate applied at 65.52 g ai ha−1 plus flumioxazin at 89.25 g ai ha−1 plus crop oil concentrate at 1% v v−1 immediately after wheat harvest. Late planted soybean (AG48X7 in 2017 and AG56X8 in 2018) were drilled at 494,000 seed ha−1 in rows on 33 cm centers with 15 rows per plot. Glufosinate was applied again at 65.52 g ai ha−1 plus crop oil concentrate at 1% v v−1 following drilling soybean. Late planted plots, both with and without winter wheat, were fertilized at soybean planting with 56 kg ha−1 of P2O5 and 56 kg ha−1 of K2O. A postemergence application of glyphosate plus fomesafen plus non-ionic surfactant at 0.25% v v−1 was made when common ragweed average height was 30 cm tall in the no cover plots, for reasons previously described.
The second year of the study was used to evaluate the effect of different IWM tactics (soybean planting time, winter cover, and HWSC) on common ragweed populations in the following growing season. Following soybean harvest in the first year of the study, the site was left fallow over the winter. In year 2, the entire study at each site was planted full season soybean (early planted) using the same herbicide program, fertility, planting rate, and row spacing as previously described, with the exceptions of soybean variety (AG56X8 and AG41X8 in 2018 and 2019, respectively).
Blacksburg contained large crabgrass (Digitaria sanguinalis (L.) Scop), giant foxtail (Setaria faberi Herrm.), and johnsongrass (Sorghum halepense (L.) Pers.) that was controlled with sethoxydim (Poast, BASF Corp., Research Triangle Park, NC, USA) at 315 g ai ha−1 plus crop oil concentrate at 1% v v−1 on June 17, 2017 in the full season soybean plots and August 16, 2017 in the late planted soybean plots. The Lawrenceville site was previously in tobacco and the soil pH was low, slowing the growth of both the cereal rye and winter wheat. To help correct this problem and get sufficient biomass for weed control, the field was fertilized with 50.4 kg of N, 16.8 kg of P2O5, and 67.2 kg of K2O ha−1 plus 560 kg ha−1 of lime (as per soil test recommendation) in mid-February 2017. The Blackstone site contained large crabgrass that was controlled with sethoxydim at 315 g ai ha−1 plus crop oil concentrate at 1% v v−1 on June 12, 2018.
Common ragweed density measurements were conducted at preplant herbicide application, at POST herbicide application and harvest in two random 0.25 m2 quadrats per plot. Height data were collected by measuring 10 random common ragweed plants per plot at cereal rye termination or wheat harvest for both the winter cover and no cover plots for each planting timing and again just prior to the POST herbicide application. At soybean harvest, common ragweed density measurements were taken in two random 0.25 m2 quadrats per plot and four representative common ragweed plants were hand harvested and air dried. The samples were weighed and then threshed to determine total seed remaining on the plant at the time of soybean harvest. Using the density at soybean harvest and the average number of seeds per plant the total number of seeds that could be impacted by HWSC was calculated. Data collected in the second year of the experiment included common ragweed density and height, as described for year 1.
All data were analyzed in JMP Pro 14 (SAS Institute Inc., Cary, NC, USA) with a model that included main effects of location, planting date, winter cover, HWSC, block, and interactions with all main effects, excluding block. All model effects were considered to be fixed effects. The models were reduced using stepwise model selection to remove non-significant interactions. Main model terms were never removed. Means were separated using Fisher's Protected LSD (P = 0.05).
Cereal rye biomass, assessed just prior to termination, was 5,940, 2,205, and 1,508 kg ha−1 at Blacksburg, Lawrenceville, and Blackstone, respectively, due to difference in inherent soil fertility and growing conditions. These biomass levels are below the 8,000 kg ha−1 threshold that has been reported for summer annual weed suppression (Teasdale and Mohler, 1993). At wheat harvest, most of the wheat residue was removed from the plots with the harvest operation. The remaining wheat residue was ~15 to 20 cm in height.
For initial common ragweed density, there was a significant location by planting time interaction (Table 2). Common ragweed density at Blacksburg in the late planted timing was 0 plants m−2 compared to all other locations and planting timings, which had similar common ragweed densities of 92.4 to 116.8 plants m−2 (data not shown). Winter cover as a main effect alone was significant for common ragweed density prior to soybean planting. In the plots that had a winter cover, either cereal rye or winter wheat, common ragweed density was lower at 77.2 plants m−2 compared to the no cover plots, which had a density of 99 plants m−2 (data not shown).
Table 2. Effects table for common ragweed density across all locations for years 1 and 2 of field experiments at Blacksburg and Lawrenceville, VA, USA in 2017–2018 and Blackstone, VA, USA in 2018–2019.
At POST herbicide application (6 WAP), there was a significant location by planting time by winter cover interaction for common ragweed density (Table 2). Overall, the Lawrenceville location had greater common ragweed densities than either Blacksburg or Blackstone. Both the Blacksburg and Blackstone locations had similar common ragweed densities for each treatment. Across all three locations, the late planted plots with winter cover had the least common ragweed with 7.6, 5.4, and 5 plants m−2 at Blacksburg, Lawrenceville, and Blackstone, respectively (Figure 1A). The greatest common ragweed densities were both at the Lawrenceville location in both treatments without winter cover with 26 and 20.6 plants m−2 (Figure 1A) in the early planted without cover and the late planted without cover treatments, respectively. In most instances, plots with winter cover had lower common ragweed densities compared to the no cover plots for both soybean planting times.
Figure 1. Common ragweed density (A) at POST herbicide application (6 wk after planting) and (B) at soybean harvest by location, planting time, and ± winter cover in the first year of the field experiment. Means are considered statistically different when they do not share a letter according to Fisher's Protected LSD (P=0.05).
At harvest, a significant location by planting time by winter cover interaction was observed for common ragweed density (Table 2). Common ragweed densities at harvest were again overall greater at the Lawrenceville location and densities were similar at both the Blacksburg and Blackstone locations. The treatments with the greatest overall density were the late planted no cover treatments with 20.8 and 22.5 plants m−2 (Figure 1B) at Lawrenceville and Blackstone, respectively. Comparing the winter cover and no cover treatments within the late planting timing, there was a 98% reduction in common ragweed densities at Lawrenceville and 85% at Blackstone. At the Blacksburg location, regardless of winter cover, the common ragweed densities in the late planted treatments were the same at 1.5 plants m−2 (Figure 1B). Common ragweed density at soybean harvest is mostly the result of emergence after postemergence herbicide application. The Blacksburg site was the northernmost and highest elevation site, likely providing from better germination conditions for common ragweed compared to other sites.
It has been reported that common ragweed has a short germination window in the spring compared to many summer annual weeds. Barnes et al. (2017) reported that 90% of common ragweed emergence occurs around the first to middle of May in Nebraska. In Pennsylvania, Delaware, and New Jersey, it has been reported that 95% of common ragweed emergence for the growing season occurs around mid-April to the first of May (Myers et al., 2004). Werle et al. (2014) reported that 90% of cumulative common ragweed emergence occurs around mid-May in Iowa. Common ragweed emergence patterns are similar across a wide area of the United States. These dates of common ragweed emergence are similar to the current study, which saw little common ragweed emergence occurring after preplant herbicide application in the treatments that were late planted either behind wheat (what is known as a double-crop soybean) or had been left fallow until late soybean planting timing and planted in late June to early July. Amuri et al. (2010) reported that overall weed densities in double-crop soybean following winter wheat were lower when residues were left on the soil surface instead of burning. This trend of lower weed densities in late planted with soybean with winter cover is similar to what was observed in the current study.
Initial common ragweed height was measured prior to wheat harvest in the late planted soybean treatments. In the late planted treatments, a significant location by winter cover interaction was observed (Table 3). At all locations, common ragweed height was reduced in the late planted treatments with winter cover (60, 50, and 29% at Blacksburg, Lawrenceville, and Blackstone, respectively) compared to the no cover treatments (Figure 2A). The wheat growing in competition with common ragweed resulted in shorter plants at the time of wheat harvest than where left fallow.
Table 3. Effects table for initial common ragweed height and at POST herbicide application across all locations for year 1 of the field experiment at Blacksburg and Lawrenceville, VA, USA in 2017 and Blackstone, VA, USA in 2018.
Figure 2. Common ragweed height (A) at late planting across all locations in year 1 of the field experiment and (B) at POST herbicide application (6 wk after planting) by location, planting time, and ± winter cover (cereal rye for early planting and winter wheat for late planting) in year 1 of the field experiment. Means are considered statistically different when they do not share a letter according to Fisher's Protected LSD (P=0.05).
Common ragweed heights at POST herbicide applications showed a significant location by planting time by winter cover interaction (Table 3), similar to the density data. Common ragweed height in early planted treatments was similar regardless of whether there was winter cover or not at the Lawrenceville and Blackstone locations, with heights ranging from 31.7 to 38.0 cm (Figure 2B). At the Blacksburg location, the common ragweed plants in the early planted, winter cover treatments were shorter than the common ragweed plants in the early planted, no cover treatments with heights of 28.9 and 35.5 cm, respectively, a 19% reduction. The difference in location was likely the result of greater cereal rye biomass in Blacksburg location compared to the other locations. In most instances, common ragweed was shorter in the early planted treatments when compared to late planted treatments regardless of winter cover. At the Blacksburg location, there was no difference in common ragweed height in either late planted treatment. At Lawrenceville, common ragweed was 21% shorter in the late planted, winter cover treatment (56.3 cm) compared to the late planted, no cover treatment (71.6 cm). At Blackstone, a similar trend was seen with plants in the late planted, winter cover treatment (28.4 cm) being shorter than the late planted no cover treatment (45.9 cm), by 38% (Figure 2B). Competition from a cover crop has been shown to reduce the height of Palmer amaranth. Hay et al. (2019) reported a 26 to 40% reduction in Palmer amaranth height when grown in competition with winter wheat compared to no cover crop. This finding is similar to the current study where a 19 to 38% reduction in common ragweed height was observed with winter cover compared to no cover crop.
Common ragweed aboveground biomass and seed retention data were collected just prior to soybean harvest. A significant location by planting time interaction was observed with common ragweed aboveground biomass (Table 4). At the Blacksburg location, the common ragweed biomass was less in the late planted treatments (22.6 g plant−1) compared to the early planted treatments (42.3 g plant−1), a 46% reduction (Figure 3A). At the Lawrenceville and Blackstone locations, biomasses were similar across both soybean planting timings with biomasses ranging from 26.6 to 35.4 g plant−1.
Table 4. Effects table for common ragweed biomass and seed retention at soybean harvest across all locations for year 1 of the field experiment at Blacksburg and Lawrenceville, VA, USA in 2017 and Blackstone, VA, USA in 2018.
Figure 3. (A) common ragweed biomass at soybean harvest by location and planting time for the first year of the field experiment and (B) common ragweed seed retention at soybean harvest by location, planting time, and cover (cereal rye for early planting and winter wheat for late planting) for the first year of the field experiment. Means are considered statistically different when they do not share a letter according to Fisher's Protected LSD (P=0.05).
A significant location by planting time by winter cover interaction was observed for common ragweed seed retention at soybean harvest (Table 4). Common ragweed seed retention was variable across the three locations, ranging from 836 to 3,611 seed plant−1. Although not always significantly different from all treatments, the numerically greatest seed retention was in the Lawrenceville early planted no cover treatment and the Blackstone late planted no cover treatment with 3,609 and 3,611 seed plant−1, respectively (Figure 3B). Common ragweed that emerges later in the growing season and grown in competition with a soybean crop are smaller and produce less aboveground biomass and seed (Dickerson and Sweet, 1971; Simard and Benoit, 2012). Simard and Benoit (2012) reported that common ragweed produced 3,694 seed plant−1 when grown in competition with soybean. This finding is similar to what was observed in the current study, however, in the current study only seed retained at harvest were recorded.
Common ragweed density in the second year of the experiment was collected prior to preplant herbicide application in the spring and again at POST herbicide application when common ragweed reached 30 cm in height. There were multiple significant interactions observed for common ragweed density at the start of the second year of the experiment. These interactions include location by planting time, location by winter cover, and location by HWSC (Table 2). Since all these interactions included location, these interactions are likely influenced by differences among locations observed in the first year of the study.
At the Blacksburg location, common ragweed densities were 83% lower in the late planted treatments from year 1 compared to the early planted treatments (Figure 4A). At the Lawrenceville and Blackstone locations, densities were similar between the early and late planted treatments with densities of 83.0 and 81.2 and 52.8 and 46.8 plants m−2, respectively.
Figure 4. Common ragweed density at preplant herbicide application in year 2 of the experiment (A) by location and planting time (B) by location and ± winter cover and (C) by location and ± harvest weed seed control (HWSC) prior to preplant herbicide application. Means are considered statistically different when they do not share a letter according to Fisher's Protected LSD (P=0.05).
When comparing treatments across location based on winter cover, common ragweed densities at the Blacksburg location were similar between the winter cover and no cover treatments the prior year with densities of 41.2 and 44.6 plants m−2, respectively (Figure 4B). At the Lawrenceville location, common ragweed density in the winter cover treatments (55.1 plants m−2) were 50% lower than in the no cover treatments (109.1 plants m−2). At the Blackstone location, similar to the Lawrenceville location, the common ragweed density in the winter cover treatments (40.7 plants m−2) were 31% lower than in the no cover treatments (58.9 plants m−2).
The effect of HWSC on common ragweed density was only different at Lawrenceville where HWSC significantly reduced common ragweed density compared to the conventional harvest treatments with densities of 59.7 and 104.5 plants m−2, respectively, a 43% reduction (Figure 4C). Prior research on HWSC has demonstrated that it can be variable on a species such as common ragweed. Beam et al. (2019) demonstrated that a one-time implementation of HWSC can reduce common ragweed density by 22% in the spring of the following year prior to preplant herbicide application, similar to the Lawrenceville location in the current study. Norsworthy et al. (2016) reported variability in the effect of HWSC at reducing weed density when using field residue removal with Palmer amaranth (Amaranthus palmeri S. Wats.). The efficacy of HWSC can be influenced by weed seed retention and the size of soil seedbank in a given field (Walsh et al., 2017b). The Lawrenceville location had a naturalized common ragweed infestation where as other sites had introduced seed at the initiation of the experiment, potentially accounting for this difference.
At POST herbicide application in the second year of the study, a significant location by planting time by winter cover interaction was observed (Table 2). This interaction includes two of the three tactics evaluated (planting time and winter cover), indicating that these tactics work better together than separately, but this varied by location. Similar to in the first year of the study, the late planted, winter cover treatments had less common ragweed compared to the other treatments across all locations with 4.7, 6.7, and 10.3 plants m−2 at Blacksburg, Lawrenceville, and Blackstone, respectively (Figure 5A). While not significantly greater than some treatments, the greatest common ragweed densities were in the Blacksburg early planted, no cover treatment and the Blackstone late planted, no cover treatment with 29.2 and 30.1 plants m−2, respectively. There was no significant effect of HWSC on common ragweed density at POST herbicide application (Table 2). The effect of HWSC on weed populations can be variable depending on the quantity of seed shattered prior to HWSC implementation, efficacy of subsequent herbicide applications, and the size of the soil seedbank (Norsworthy et al., 2016; Tidemann et al., 2016; Walsh et al., 2017b; Beam et al., 2019).
Figure 5. Common ragweed density (A) at POST herbicide application by location, planting time, and ± winter cover (cereal rye for early planting and winter wheat for late planting) in year 2 of the experiment. POST herbicide applications were made when common ragweed reached 30 cm in height and (B) at soybean harvest by location and planting time in year 2 of the experiment. Means are considered statistically different when they do not share a letter according to Fisher's Protected LSD (P=0.05).
At soybean harvest, in the second year of the study a significant location by planting time interaction was observed (Table 2). Similar to the first year of the study the treatments that had been late planted had significantly lower common ragweed densities compared to the treatments that had been planted early in year 1 at Blacksburg and Lawrenceville locations. Common ragweed densities were 2.1 and 6.6 plants m−2 in the late planted treatments at Blacksburg and Lawrenceville, respectively, compared to 12.3 and 12.9 plants m−2 in the early planted treatments (Figure 5B). Conversely at the Blackstone location, common ragweed densities were lower in the early planted treatments compared to the late planted treatments with 0.18 and 5.2 plants m−2. The reason for this difference among locations not clear, but may be due to differences in weather in 2019 (the second year of the study at this site) compared to 2018 for the other locations.
Common ragweed height, at the beginning of the second year of the study a significant location by planting time by winter cover interaction was observed similar to the first year of the study (Table 3). Common ragweed height ranged from 3.1 to 7.8 cm across all locations and treatments (Figure 6A). The shortest common ragweed plants were observed in the early planted with winter cover plots in Blacksburg. The tallest common ragweed plants were observed in the early planted with no winter cover plots. Common ragweed height at POST applications, in the second year of the study a significant location by planting time by HWSC interaction was observed. The Lawrenceville location had common ragweed that was significantly taller (33.1 to 36.3 cm) compared to the other locations (21.2 to 23.5 cm) (Figure 6B).
Figure 6. Common ragweed heights (A) at preplant herbicide application in year 2 of the experiment by location, planting time, and location and (B) at POST application in the second year of the experiment by location, planting time, and HWSC. Means are considered statistically different when they do not share a letter according to Fisher's Protected LSD (P=0.05).
Common ragweed aboveground biomass in the second year of the study was only significant by location (Table 4). Common ragweed aboveground biomass was 47.81, 16.95, and 27.13 g plant−1 at Blacksburg, Lawrenceville, and Blackstone, respectively. Common ragweed seed retention data for the second year of the study only included the Blackstone and Lawrenceville locations (Table 4). At the Blackstone location, common ragweed failed to set seed by soybean harvest due to severe drought conditions. There was a significant location by planting time interaction observed for common ragweed seed retention. At Blacksburg, common ragweed seed retention was 886 and 681 seed plant−1 in the early and late planted treatments, respectively. At Lawrenceville, common ragweed seed retention was 375 and 525 seed plant−1 in the early and late planted treatments, respectively (data not shown).
Herbicide resistance is an increasing problem and diversifying weed control strategies into an IWM system is a necessity. The current study demonstrates that using multiple methods including planting timing, winter cover, and HWSC can help reduce common ragweed populations in 1 year, but effects varied by location. It appears that the planting timing (late planting or double-cropping soybean after winter wheat compared to early planted) reduces common ragweed populations more than other treatments tested in this study. Therefore, double-cropping soybeans after wheat is a recommended strategy for integrated common ragweed management, where feasible. This system should be used in conjunction with crop rotation and other weed management techniques to keep common ragweed densities at manageable levels. Including a winter cover (wheat or cereal rye) resulted in similar or reduced common ragweed density and reduced common ragweed heights across locations at soybean planting. The effectiveness of HWSC was variable but reduced common ragweed densities at one of three locations. Variability in HWSC has been demonstrated in other research (Norsworthy et al., 2016; Walsh et al., 2017b; Beam et al., 2019) and highlights the need for additional research. The impact of planting timing and HWSC and its effect on soil seedbanks should be evaluated further.
The raw data supporting the conclusions of this article will be made available by the authors, without undue reservation.
SB and MF performed the research and analyzed the data. SB, MF, and SM wrote the paper. CC, DCH, and DLH edited the paper. All authors designed the research.
The authors declare that the research was conducted in the absence of any commercial or financial relationships that could be construed as a potential conflict of interest.
The authors would like to thank Wiley Lynch for allowing this research to be conducted on his farm, Taylor Clarke for his assistance in field operations, Kara Pittman for her assistance with the statistical analysis, and fellow members of Dr. Flessner's lab Lucas Rector, Eric Scruggs, Wykle Greene, Kevin Bamber, and undergraduate workers for their assistance in data collection and processing samples. The authors also thank the Virginia Small Grains Board, the Virginia Agricultural Experiment Station, the Hatch Program of the National Institute of Food and Agriculture, US Department of Agriculture, and the US Department of Agriculture, Agricultural Research Service Areawide program (award 58-8042-5-054) for providing partial funding for this research.
Amuri, N., Brye, K. R., Gbur, E. E., Oliver, D., and Kelley, J. (2010). Weed populations as affected by residue management practices in a wheat-soybean double-crop production system. Weed Sci. 58, 234–243. doi: 10.1614/WS-09-088.1
Barnes, E. R., Werle, R., Sandell, L. D., Lindquist, J. L., Knezevic, S. Z., Sikkema, P. H., et al. (2017). Influence of tillage on common ragweed (Ambrosia artemisiifolia) emergence pattern in Nebraska. Weed Technol. 31, 623–631. doi: 10.1017/wet.2017.38
Barnes, J., and Putnam, A. (1987). Role of benzoxazinones in allelopathy by rye (Secale cereale L.). J. Chem. Ecol. 13, 889–896. doi: 10.1007/BF01020168
Baskin, J. M., and Baskin, C. C. (1977). Role of temperature in the germination ecology of three summer annual weeds. Oecologia 30, 377–382. doi: 10.1007/BF00399768
Beam, S. C., Mirsky, S., Cahoon, C., Haak, D., and Flessner, M. (2019). Harvest weed seed control of Italian ryegrass [Lolium perenne ssp multiflorum (Lam.) Husnot], common ragweed (Ambrosia artemisiifolia L.), and Palmer amaranth (Amaranthus palmeri S. Watson). Weed Technol. 33, 627–632. doi: 10.1017/wet.2019.46
Chandler, K., Shrestha, A., and Swanton, C. J. (2001). Weed seed return as influenced by the critical weed-free period and row spacing of no-till glyphosate-resistant soybean. Can. J. Plant Sci. 81, 977–880. doi: 10.4141/P01-049
Coble, H. D., Williams, F. M., and Ritter, R. L. (1981). Common ragweed (Ambrosia artemisiifolia) interference in soybeans (Glycine max). Weed Sci. 29, 339–342. doi: 10.1017/S0043174500062081
Dickerson, C. T., and Sweet, R. D. (1971). Common ragweed ecotypes. Weed Sci. 19, 64–66. doi: 10.1017/S0043174500048281
Finney, D. M., Creamer, N. G., Schultheis, J. R., Wagger, M. G., and Brownie, C. (2009). Sorghum sudangrass as a summer cover and hay crop for organic fall cabbage production. Renew. Agric. Food Syst. 24, 225–233. doi: 10.1017/S174217050999007X
Harker, K. N., and O'Donovan, J. T. (2013). Recent weed control, weed management, and integrated weed management. Weed Technol. 27, 1–11. doi: 10.1614/WT-D-12-00109.1
Hay, M. M., Dille, J. A., and Peterson, D. E. (2019). Integrated pigweed (Amaranthus spp.) management in glufosinate-resistant soybean with a cover crop, narrow row widths, row-crop cultivation, and herbicide program. Weed Technol. 33, 710–719. doi: 10.1017/wet.2019.62
Heap, I. (2020). The International Survey of Herbicide Resistant Weeds. Available online at: www.weedscience.org (accessed December 28, 2020).
Matthews, J. M., Llewellyn, P. S., Reeves, T., and Jaeschke, R. (1996). “Seed catching; a method to control annual weeds,” in 8th Australian Agronomy Conference, University of Southern Queensland. Toowoomba, QLD: Australian Society of Agronomy, 684–685.
Mirsky, S. B., Ryan, M. R., Teasdale, J. R., Curran, W. S., Reberg-Horton, C. S., Spargo, J. T., et al. (2013). Overcoming weed management challenges in cover crop-based organic rotational no-till soybean production in the eastern United States. Weed Technol. 27, 193–203. doi: 10.1614/WT-D-12-00078.1
Moore, R. E., Niemczura, W. P., Kwok, O. C. H., and Patil, S. S. (1984). Inhibitors of ornithine carbamoyltransferase from Pseudomonas syringae pv. Phaseaolicola. Tetrahedron Lett. 25, 3931–3934. doi: 10.1016/0040-4039(84)80033-7
Myers, M. W., Curran, W. S., VanGessel, M. J., Calvin, D. D., Mortensen, D. A., Majek, B. A., et al. (2004). Predicting weed emergence for eight annual weed species in the northeastern United States. Weed Sci. 52, 913–919. doi: 10.1614/WS-04-025R
Nord, E. A., Ryan, M. R., Curran, S., Mortensen, D. A., and Mirsky, S. B. (2012). Effects of management type and timing on weed suppression in soybean no-till planted into rolled-crimped cereal rye. Weed Sci. 60, 624–633. doi: 10.1614/WS-D-12-00024.1
Norsworthy, J. K., Korres, N. E., Walsh, M. J., and Powles, S. B. (2016). Integrating herbicide programs with harvest weed seed control and other fall management practices for the control of glyphosate-resistant Palmer amaranth (Amaranthus palmeri). Weed Sci. 64, 540–550. doi: 10.1614/WS-D-15-00210.1
Norsworthy, J. K., Ward, S. M., Shaw, D. R., Llewellyn, R. S., Nichols, R. L., Webster, T. M., et al. (2012). Reducing the risks of herbicide resistance: best management practices and recommendations. Weed Sci. 60, 31–62. doi: 10.1614/WS-D-11-00155.1
Redlick, C., Syrovy, L. D., Duddu, H. S. N., Benaragama, D., Johnson, E. N., Willenburg, C. J., et al. (2017). Developing an integrated weed management system for herbicide-resistant weeds using lentil (Lens culinaris) as a model crop. Weed Sci. 65, 778–786. doi: 10.1017/wsc.2017.47
Ryan, M. R., Curran, W. S., Grantham, A. M., Hunsberger, L. K., Mirsky, S. B., Mortensen, D. A., et al. (2011a). Effects of seeding rate and poultry litter on weed suppression from a rolled cereal rye cover crop. Weed Sci. 59, 438–444. doi: 10.1614/WS-D-10-00180.1
Ryan, M. R., Mirsky, S. B., Mortensen, D. A., Teasdale, J. R., and Curran, W. S. (2011b). Potential synergistic effects of cereal rye biomass and soybean planting density on weed suppression. Weed Sci. 59, 238–246. doi: 10.1614/WS-D-10-00110.1
Schwartz-Lazaro, L. M., Norsworthy, J. K., Walsh, M. J., and Bagavathiannan, M. V. (2017). Efficacy of the integrated Harrington seed destructor on weeds of soybean and rice production systems in the southern United States. Crop Sci. 57, 2812–2818. doi: 10.2135/cropsci2017.03.0210
Schwartz-Lazaro, L. M., Shergill, L. S., Evans, J. A., Bagavathiannan, M. V., Beam, S. C., Bish, M. D., et al. (2020). Seed shattering phenology at soybean harvest of economically important weeds in multiple regions of the United States. Part 1: broadleaf species. Weed Sci. 1–29. doi: 10.1017/wsc.2020.80
Scruggs, E., Beam, S., and Flessner, M. (2019). Common Ragweed (Ambrosia artemisiifolia) Control in Soybeans. Blacksburg, VA: Virginia Cooperative Extension SPES143NP.
Shergill, L. S., Bejleri, K., Davis, A., and Mirsky, S. B. (2020). Fate of weed seeds after impact mill processing in midwestersn and mid-Atlantic United States. Weed Sci. 68, 92–97. doi: 10.1017/wsc.2019.66
Simard, M. J., and Benoit, D. L. (2012). Potential pollen and seed production from early- and late-emerging common ragweed in corn and soybean. Weed Technol. 26, 510–516. doi: 10.1614/WT-D-11-00178.1
Soni, N., Nissen, S. J., Westra, P., and Norsworthy, J. K. (2019). Seed retention of winter annual grass weeds at winter wheat harvest maturity shows potential for harvest weed seed control. Weed Technol. 34, 266–271. doi: 10.1017/wet.2019.108
Swanton, C. J., Mahoney, K. J., Chandler, K., and Gulden, R. H. (2008). Integrated weed management knowledge-based weed management systems. Weed Sci. 56, 168–172. doi: 10.1614/WS-07-126.1
Swanton, C. J., and Weise, S. F. (1991). Integrated weed management: the rationale and approach. Weed Technol. 5, 657–663. doi: 10.1017/S0890037X00027512
Teasdale, J. R., and Mirsky, S. B. (2015). Tillage and planting date effects on weed dormancy, emergence, and early growth in organic cotton. Weed Sci. 63, 477–490. doi: 10.1614/WS-D-14-00112.1
Teasdale, J. R., and Mohler, C. L. (1993). Light transmittance, soil-temperature, and soil-moisture under residue of hairy vetch and rye. Agron. J. 85, 673–680. doi: 10.2134/agronj1993.00021962008500030029x
Teasdale, J. R., and Mohler, C. L. (2000). The quantitative relationship between weed emergence and the physical properties of mulches. Weed Sci. 48, 385–392. doi: 10.1614/0043-1745(2000)048[0385:TQRBWE]2.0.CO;2
Teasdale, J. R., Pillai, P., and Collins, R. T. (2005). Synergism between cover crop residue and herbicide activity on emergence and early growth of weeds. Weed Sci. 53, 521–527. doi: 10.1614/WS-04-212R
Teasdale, J. R., Rice, C., Cai, G., and Mangum, R. A. (2012). Expression of allelopathy in the soil environment: soil concentration and activity of benzoxazinoid compounds released by rye cover crop residue. Plant Ecol. 213, 1893–1905. doi: 10.1007/s11258-012-0057-x
Thill, D. C., Lish, J. M., Callihan, R. H., and Bechinski, E. J. (1991). Integrated weed management-a component of integrated pest management: a critical review. Weed Technol. 5, 648–656. doi: 10.1017/S0890037X00027500
Tidemann, B. D., Hall, L. M., Harker, K. N., and Alexander, B. C. S. (2016). Identifying critical control points in the wild oat (Avena fatua) life cycle and the potential effects of harvest weed-seed control. Weed Sci. 64, 463–473. doi: 10.1614/WS-D-15-00200.1
Tidemann, B. D., Hall, L. M., Harker, K. N., and Beckie, H. J. (2017). Factors affecting weed seed devitalization with the Harrington seed destructor. Weed Sci. 65, 650–658. doi: 10.1017/wsc.2017.23
Van Wychen, L. (2016). 2016 Survey of the Most Common and Troublesome Weeds in Broadleaf Crops, Fruits and Vegetables in the United States and Canada. Weed Science Society of America National Weed Survey Dataset. Available online at: http://wssa.net/wp-content/uploads/2016-Weed-Survey_Broadleaf-crops.xlsx (accessed August 19, 2019).
Wallace, J. M., Keene, C. L., Curran, W., Mirsky, S. S., Ryan, M. R., and VanGessel, M. J. (2018). Integrated weed management strategies in cover crop-based, organic rotational no-till corn and soybean in the mid-Atlantic region. Weed Sci. 66, 94–108. doi: 10.1017/wsc.2017.53
Walsh, M., Newman, P., and Powles, S. (2013). Targeting weed seeds in-crop: a new weed control paradigm for global agriculture. Weed Technol. 27, 431–436. doi: 10.1614/WT-D-12-00181.1
Walsh, M., Ouzman, M., Newman, P., Powles, S., and Llewellyn, R. (2017a). High levels of adoption indicate that harvest weed seed control is now an established weed control practice in Australian cropping. Weed Technol. 31, 341–347. doi: 10.1017/wet.2017.9
Walsh, M. J., Aves, C., and Powles, S. B. (2017b). Harvest weed seed control systems are similarly effective on rigid ryegrass. Weed Technol. 31, 178–183. doi: 10.1017/wet.2017.6
Walsh, M. J., Broster, J. C., Schwaartz-Lazaro, L. M., Norsworthy, J. K., Davis, A. S., Tidemann, B. D., et al. (2018). Opportunities and challenges for harvest weed seed control in global cropping systems. Pest Mgmt Sci. 74, 2235–2245. doi: 10.1002/ps.4802
Walsh, M. J., and Powles, S. B. (2014). High seed retention at maturity of annual weeds infesting crop fields highlights the potential for harvest weed seed control. Weed Technol. 28, 486–493. doi: 10.1614/WT-D-13-00183.1
Wells, M. S., Reberg-Horton, S. C., Mirsky, S. B., Maul, J. E., and Hu, S. (2017). In situ validation of fungal N translocation to cereal rye mulches under no-till soybean production. Plant Soil 410, 153–165. doi: 10.1007/s11104-016-2989-8
Werle, R., Sandell, L. D., Buhler, D. D., Hartzler, R. G., and Lindquist, J. L. (2014). Predicting emergence of 23 summer annual weed species. Weed Sci. 62, 267–279. doi: 10.1614/WS-D-13-00116.1
Willemsen, R. W. (1975). Effect of stratification temperature and germination temperature on germination and the induction of secondary dormancy in common ragweed seeds. Am. J. Bot. 62, 1–5. doi: 10.1002/j.1537-2197.1975.tb12333.x
Williams, A., Wells, M. S., Dickey, D. A., Hu, S., Maul, J., Raskin, D. T., et al. (2018). Establishing the relationship of soil nitrogen immobilization to cereal rye residues in a mulched system. Plant Soil 426, 95–107. doi: 10.1007/s11104-018-3566-0
Common ragweed, Ambrosia artemisiifolia L. AMBEL; cereal rye, Secale cereale L.; soybean Glycine max (L.) Merr.; winter wheat, Tritcum aestivum L.
Keywords: cover crops, harvest weed seed control, planting date, weed density, seed retention
Citation: Beam SC, Cahoon CW, Haak DC, Holshouser DL, Mirsky SB and Flessner ML (2021) Integrated Weed Management Systems to Control Common Ragweed (Ambrosia artemisiifolia L.) in Soybean. Front. Agron. 2:598426. doi: 10.3389/fagro.2020.598426
Received: 24 August 2020; Accepted: 18 December 2020;
Published: 28 January 2021.
Edited by:
Karla Leigh Gage, Southern Illinois University Carbondale, United StatesReviewed by:
Harminder Pal Singh, Panjab University, IndiaCopyright © 2021 Beam, Cahoon, Haak, Holshouser, Mirsky and Flessner. This is an open-access article distributed under the terms of the Creative Commons Attribution License (CC BY). The use, distribution or reproduction in other forums is permitted, provided the original author(s) and the copyright owner(s) are credited and that the original publication in this journal is cited, in accordance with accepted academic practice. No use, distribution or reproduction is permitted which does not comply with these terms.
*Correspondence: Michael L. Flessner, Zmxlc3NuZXJAdnQuZWR1
Disclaimer: All claims expressed in this article are solely those of the authors and do not necessarily represent those of their affiliated organizations, or those of the publisher, the editors and the reviewers. Any product that may be evaluated in this article or claim that may be made by its manufacturer is not guaranteed or endorsed by the publisher.
Research integrity at Frontiers
Learn more about the work of our research integrity team to safeguard the quality of each article we publish.