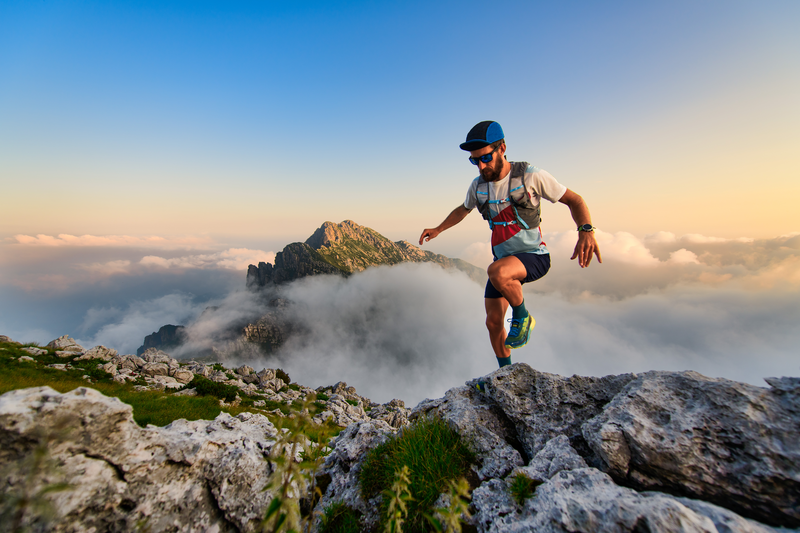
94% of researchers rate our articles as excellent or good
Learn more about the work of our research integrity team to safeguard the quality of each article we publish.
Find out more
REVIEW article
Front. Agron. , 22 October 2020
Sec. Disease Management
Volume 2 - 2020 | https://doi.org/10.3389/fagro.2020.590908
The introduction of high-yielding and hybrid cultivars and the opening of new markets in the food and feed sector have steadily increased rapeseed production since the 1980s in the main production regions, Canada, Europe, China, India, and Australia. Since the 1990s, however, the average growth rate of yields has declined in Europe and Australia, which has been associated with a less effective control of biotic stresses. A global survey including the knowledge of 22 experts from 10 countries revealed a total of 16 diseases, 37 insect pests, several species of nematodes, and slugs currently affecting rapeseed production globally. A ranking of the top 10 most important biotic stresses in the four global regions where Brassica napus is grown (Canada, China, Europe, Australia) indicated an increase in several important stresses and distinct regional differences in the priority of prevailing diseases and pests. A stronger overlap exists among diseases, with Sclerotinia stem rot, Phoma stem canker, and clubroot occurring in all the four global regions on the top 10 list, while the range of prevailing insect pests was more diverse among the regions, with no top 10 insect playing an equally important role worldwide. Management options are substantially broader in disease than in pest control, making the latter the larger challenge. Since common integrated pest management (IPM) tools such as crop rotation, soil management, resistant cultivars or biocontrol are ineffective or not available, insect control largely relies on insecticides. Increasing restrictions on insecticide use, particularly in Europe, and losses in insecticide efficacy threaten the profitability of oilseed rape production and its role as an important break crop in cereal dominated cropping systems. Since the survival time of insects in the absence of their main host is relatively short (<1 year), a regional synchronization of cropping schemes resulting in one or more years without the crop could lead to a substantial disruption of regional insect populations. If rotation schemes were implemented on the landscape instead the farm level, by coordination among growers in zones covering the range distances of insect pests, an efficient and chemical low management strategy could be established and enable a more sustainable rapeseed production in the future.
Oilseed rape (Brassica napus) is the second most important oilseed crop and belongs to the mustard family (Brassicaceae), which consists of 338 genera with more than 3,709 species cultivated worldwide, especially in temperate and mountainous regions (Al-Shehbaz et al., 2006; Warwick et al., 2010). B. napus is a relatively young crop species (AACC genome, 2n = 38) that derived from a spontaneous hybridization between B. rapa (AA genome, 2n = 20) and B. oleracea (CC genome, 2n = 18) (Koh et al., 2017). Europe, Canada, China, India, and Australia are the leading production zones with 25.5, 20.3, 13.3, 8.4, and 3.9 million metric tons of rapeseed production in 2018, respectively (FAO Database).
The average yield of oilseed rape has increased since 1981, mainly due to the introduction of high-yielding varieties with an increasing share of hybrids. The improved yield potential together with the introduction of double-low varieties (<2% erucic acid and <25 μmol/g glucosinolates in seeds), has opened new markets for rapeseed oil used for human consumption and the meal as a valuable animal feed (Dimov and Möllers, 2010). This has significantly enhanced the profitability of the crop and has expanded its cultivation area. However, since 1990, the annual growth rate of average yields in Europe (Germany, France, and the United Kingdom) and Australia has declined, e.g., in Germany from 2.1% in 1961–1990 to 0.5% in 1991–2018, while it continues to increase in China (2.0%) and Canada (1.5%) (Figure 1). The decline in average yields in Europe and Australia was associated with increasing stresses from pests and diseases, warm temperature, low precipitation (Kutcher et al., 2010; Assefa et al., 2018) and decreasing availability of registered active ingredients for chemical control, e.g., through the ban on neonicotinoids (Noleppa, 2017).
Figure 1. Development of average yields of oilseed rape in main producing countries from 1961 to 2017 (FAO Database).
In order to illustrate the status and trends in oilseed rape production and display actual major biotic constraints in the main growing regions, a global survey (see Supplementary Table 1) was conducted in 2019. To this end, a questionnaire was filled by 22 experts (see Supplementary Table 2) from 10 countries (Australia, Canada, China, Czech Republic, France, Germany, India, Sweden, Ukraine, and the United Kingdom), representing more than 95% of the global oilseed rape production area who are intensely involved in research and management of diseases and/or pests in oilseed rape (B. napus) or oilseed mustard (B. juncea). In addition, results of a long-term crop rotation study conducted in Göttingen, Germany, since 1986 were included to demonstrate the impact of crop rotation on the development of diseases and pests and on yield of oilseed rape.
Overall, the questionnaire return revealed 16 diseases and 37 insect pests, as well as nematodes, slugs and snails which were mentioned by the experts to occur in oilseed rape or mustard growing regions since 2016 until present (Table 1). The biotic stresses predominantly affect leaves (10 diseases and 22 insect pests) and stems (7 diseases and 12 insect pests), while only 2 diseases and 11 insect pests affect pods and seeds of oilseed rape.
Table 1. Diseases and insect pests on oilseed rape or mustard worldwide and their infection/feeding site on the plants (2016 until present), based on statements by 22 experts from 10 countries.
In contrast to oilseed rape, bihar hairy caterpillar (Diacrasia obliqua), painted bug (Bagrada hilaris), and southern blight (Sclerotium rolfsii) were only reported in mustard production from India. Due to the specific profile of diseases and pests in mustard and its regional scope, the further analysis and discussion in this paper will focus on global B. napus production.
As shown in Table 2, Sclerotinia stem rot and clubroot are the most common and significant diseases in oilseed rape cultivation globally, while flea beetles represent the key insect problems worldwide, except for Australia. In spring oilseed rape producing regions, such as Canada, Phyllotreta spp. are the most common flea beetles, while in winter and semi-winter oilseed rape regions, Psylliodes spp. are prevailing. In contrast to Australia, where redlegged earth mite is the only pest mentioned in the top 10 list of biotic threats, the importance of insect vs. disease threats in Europe, China, and Canada is more balanced.
Table 2. Top 10 most significant biotic threats to oilseed rape cultivation in Australia, Europe, China, and Canada at present (based on statements by 21 experts from 9 countries; n, number of statements).
Clubroot, Sclerotinia stem rot, and Phoma stem canker are the prevailing diseases commonly found in all oilseed rape producing regions, while light leaf spot and Mycosphaerella ring spot are only reported from Europe (Figure 2A). The Aster yellows phytoplasma disease, only mentioned by the Canadian expert and considered as 7th most important biotic threat to oilseed rape production, has caused a 20–30% yield loss in 2012, while common losses in Canada were <1% in most other years. Verticillium stem striping has recently become one of the most important diseases in Europe and Canada but has not yet been reported from China, although the causal fungal pathogen, Verticillium longisporum, has been found in Chinese cabbage. So far, Australia has not seen stem striping at all (Yu et al., 2015; Depotter et al., 2016; Canadian Food Inspection Agency, 2017). Compared to other regions, where winter oilseed rape and semi-winter oilseed rape are cultivated, Canada with its consistent cultivation of spring oilseed rape faces a lower abundance of fungal diseases. White rust, which has been only recorded in China and Australia, is one of the most destructive diseases of brassica in tropical and subtropical areas such as Pakistan (Asif et al., 2017). Although B. napus has been reported to be immune to white rust, low level average annual yield losses have been reported from China between 2007 and 2016, if no disease management was conducted (Subudhi and Raut, 1994; Yang et al., 2018).
Figure 2. Occurrence of important diseases on oilseed rape worldwide (A) and in Europe (B). Top 10 diseases worldwide are marked in bold letters. *In China only Leptosphaeria biglobosa but not L. maculans has been reported.
Within Europe, the occurrence of diseases in the four considered geographic regions is more homogenous, although slight differences can be still found. In contrast to the cultivation on the continent, oilseed rape production on the British Isles displays a lower diversity of diseases (n = 6, Figure 2B). Due to its geographic position, Ukraine, at a lower latitude with a warmer climate is more suitable for development of powdery mildew (Ciola and Cipollini, 2011). This disease mainly occurs in Southern Ukraine but also in the central and western parts during warm years, whereas none of the experts from other regions in Europe regarded this disease as significant on oilseed rape. So far only one species of powdery mildew, Erysiphe cruciferarum, (Table 1) is known on Brassica spp., which may lead to yield losses by reducing plant growth and the quantity and quality of the seeds (Braun and Cook, 2012; Alkooranee et al., 2015; Abasova, 2018). Besides, another leaf disease, white leaf spot, has occurred infrequently in the Czech Republic and Ukraine since 2006, which since 2016 has become a consistent problem requiring regular control, e.g., in the eastern half of the Czech Republic. The causal agent of this disease, Pseudocercosporella capsellae, can produce cercosporin, a mycotoxin, which enhances the virulence of the pathogen on oilseed rape (Gunasinghe et al., 2016).
Several major diseases on oilseed rape are caused by soil-borne pathogens (Plasmodiophora brassicae, Verticillium longisporum) or by pathogens that affect the stem (Sclerotinia sclerotiorum, Leptosphaeria maculans, L. biglobosa, Alternaria spp., Pseudocercosporella capsellae, Pyrenopeziza brassicae). Sclerotinia stem rot (or white mold) is present in all major oilseed rape growing regions and considered a key disease causing significant yield losses ranging from 0.18 to 1.3% per percent disease incidence (Kirkegaard et al., 2006; Del Río et al., 2007). The causal agent, Sclerotinia sclerotiorum, is a devastating pathogen that is able to infect more than 400 plant species (Mizubuti, 2019). Its sclerotia can survive in the soil for more than 4 years (Table 3), which narrows the selection of rotation crops and increases the risk of accumulation of sclerotia in the soil. During periods of cool and humid weather during flowering, ascospores can be produced and spread typically to lower parts of stems within the canopy but may also be also disseminated by wind, insects or rain splash to upper leaves, and pods or neighboring plants (Link and Johnson, 2007).
Table 3. Current management options for the control of important pests and diseases in oilseed rape.
Clubroot caused by Plasmodiophora brassicae has become more important in the last two decades as a major threat to oilseed rape production worldwide. In Europe, awareness of the disease has risen only in the last 10–15 years. Average yield loss of 0.03 t/ha per every 1% increase in clubroot severity has been recorded (McGrann et al., 2016), while the potential total yield loss may reach up to 100%, current estimations in field surveys are ranging from 5 to 60% (Wallenhammar, 1998; Ren et al., 2014; Strehlow et al., 2015). Resting spores of P. brassicae from the infected root galls remain in the soil for more than 4 years in the absence of host plants (Table 7). A previous study has demonstrated a half-life of resting spores of 3.6 years (Wallenhammar, 1996). Besides, a strong increase in spore density in the soil has been recorded after ploughing (Murakami et al., 2004; Donald and Porter, 2009). Clubroot is mainly disseminated by the movement of soil containing resting spores via farm equipment or through water erosion (Ríčarová et al., 2016).
Stem striping caused by V. longisporum is a soil-borne, root-invasive, vascular disease with a damage potential ranging from 10 to 50% due single plant yield reduction. Yield losses are relatively moderate as stem striping symptoms occur only at the beginning of ripening (Dunker et al., 2008; Gladders et al., 2013; Depotter et al., 2019; Zheng et al., 2019b). Early season cabbage root fly damage during autumn and mechanical injury in roots may significantly enhance disease severity and fungal colonization in susceptible cultivars (Keunecke, 2009). Besides, the vascular spread upwards in the stem can be accelerated at increased soil temperature (Siebold and von Tiedemann, 2013; Zheng et al., 2019b). After harvest, the fungal resting structures, microsclerotia, remain in the stubbles, or are released into the soil. Three years after embedding oilseed rape stubbles carrying microsclerotia in 10 and 20 cm soil depth, the survival rate of microsclerotia was 7 and 35%, respectively (unpublished data). In addition, recent research indicated that V. longisporum may be transmitted by seeds of spring oilseed rape, while this pathway of transmission is unlikely to happen on winter oilseed rape (Zheng et al., 2019a).
Phoma stem canker or blackleg is one of the most important diseases and has been associated with yield losses ranging from 5 to 50% in Europe, Canada, and Australia, where Leptosphaeria maculans and/or L. biglobosa have been recorded to be widespread (Hall et al., 1993; Barbetti and Khangura, 1999; Zhou et al., 1999; Aubertot et al., 2004; Fitt et al., 2006; Hwang et al., 2016). L. biglobosa, which is less aggressive than L. maculans, is the only Leptosphaeria species reported from Chinese oilseed rape fields and may cause substantial seed yield losses ranging from 10 to 37% as estimated for 4 counties in the Hubei province in 2012 and 2013 (Fitt et al., 2006; Cai et al., 2017). Similar to V. longisporum, mechanical injury and feeding damage by the cabbage root fly, cabbage stem flea beetle, and rape stem weevil may significantly increase disease incidence, volume of diseased tissue and disease severity of Phoma stem canker in susceptible cultivars (Alford et al., 2003; Keunecke, 2009). While inoculum survival time on residues is <2 years in Europe, a longer survival up to 4 years is expected in Western Australia (West et al., 2001; Fitt et al., 2006). Air-borne ascospores are the main source of inoculum to start epidemics (Gladders and Musa, 1980) and the release of ascospores does not largely differ in a temperature range from 5 to 20°C, but is significantly increased by rainfall (Huang et al., 2005). A limited distance of spread, mainly within 14 cm, has been estimated to be due to rain-splash (Travadon et al., 2007). However, spores may be transmitted by wind over distances up to 10 km (Piliponyte-Dzikiene et al., 2014).
Globally, insect pests play a superior role among biotic stresses in oilseed rape production compared to diseases (Table 1). Flea beetles, weevils, pollen beetle, aphids, brassica pod midge, diamondback moth, cabbage root fly, and redlegged earth mites are the most important insect pests in many regions according to the survey. A previous survey from 2002 to 2003 showed a similar result and indicated that pollen beetle, flea beetle, weevils, brassica pod midge, and cabbage root fly were also commonly recorded as important pests by the oilseed rape growers in 6 European countries (Menzler-Hokkanen et al., 2006). Similarly, more recent studies indicated that these pests can cause serious damage to oilseed rape production although their relative importance varies with country and year (Williams, 2010; Reddy, 2017). Similar to diseases, Europe has the highest diversity in insect pests (n = 17), while other main producing regions such as China, Australia, and Canada have less diversity and relatively specific insect pest problems (Figure 3A). In contrast to diseases, there is only a single insect species, diamondback moth, which has been reported to occur worldwide. Except for Canada, aphids are amongst the most important insect pests in all main producing regions. Canada, where mainly spring oilseed rape is cultivated, has the lowest number of insect pests (n = 7) with some individual species such as bertha armyworm, lygus bugs and swede midge, while Europe and China, where mostly winter oilseed rape and semi-winter oilseed rape is grown, respectively, share two important insect problems, brassica pod midge, and pollen beetle. In spite of this overlap, more leaf damaging insects are reported from China, while stem damaging insects prevail in Europe (Reddy, 2017). So far, the cabbage root fly and flea beetles have been regarded to be restricted to the Northern hemisphere and are not yet found in Australia. Interestingly, Australia, maybe due to its remote geographic position, displays a quite unique pattern of insect pests not existing elsewhere such as lucerne flea, cabbage webworm, budworm native, blue oat mite, and redlegged earth mite.
Figure 3. Distribution of important pests on oilseed rape worldwide (A) and in Europe (B). Top 10 pests in the worldwide are marked in bold letters.
Flea beetles (cabbage stem flea beetle), rape stem weevil, pollen beetle, aphids, and cabbage stem weevil are the most important insect pests that affect European oilseed rape crops (Figure 3B). Similar to diseases, Eastern Europe has the highest number of pest species (n = 15), followed by Western Europe (n = 13), the British Isles (n = 9), and Northern Europe (n = 8).
Damage by insect pests is a significant yield reducing factor in oilseed rape production, with an average annual yield loss of 13% on a global scale and 15% annual yield loss on a European scale (Cramer, 1967; Milovac et al., 2017). Due to their high mobility, most insect pests are not restricted to the infested field but can disperse and migrate over long distances, not only invading adjacent fields but more likely spreading on a landscape level (Table 7). Flea beetles (Psylliodes spp. on winter and semi-winter oilseed rape, Phyllotreta spp. on spring oilseed rape) are the most important pests worldwide. Under warm climatic conditions, leaf damage induced by flea beetles can advance from 25 to 50% within hours and treatment delayed by 1–2 days can result in loss of the entire harvest when a high feeding pressure occurs (Sekulic and Rempel, 2016).
The cabbage stem flea beetle (Psylliodes chrysocephala) can damage the plants by adult feeding on the cotyledons and leaves and reduces plant vigour due to larval mining within petioles and stems. Because of severe infestations by the cabbage stem flea beetle in recent years, many farmers in the UK had to re-sow fields with alternative crops resulting in a 21% reduction of the oilseed rape growing area since 2013 (Department for Environment Food and Rural Affairs, 2017; Wynn et al., 2017). In the complete absence of host plants, flea beetles are only able to survive for less than a year, but they can migrate distances of 3–4 km to search suitable host plants which is mainly guided by olfactory cues (Bonnemaison, 1965; Finch and Collier, 2000). In the Northern hemisphere and winter type oilseed rape, the immigration flight begins in late August/early September and reaches the maximum in September at daily temperatures above 20°C (Johnen et al., 2010).
The rape stem weevil (Ceutorhynchus napi) is a further detrimental pest in continental European winter oilseed rape production. The adults immigrate from previous year rapeseed fields and lay their eggs in the shooting stems in February and March (Alford et al., 2003). Oviposition can cause twisting and splitting of the stems resulting in distortion and considerable disruption of growth, low pod production, and plant lodging (Juran et al., 2011). In the recent 5–10 years, the cabbage stem weevil (C. pallidactylus) has been also reported as a consistent problem in the Czech Republic, Germany, France, Poland, and the UK. Similar to the rape stem weevil, it usually immigrates into oilseed rape crops in March and April (Johnen et al., 2010; Döring, 2012). However, instead of oviposition into stems, this species lays its eggs into petioles and the neonate larvae feed inside the petioles before they invade the stems (Alford et al., 2003).
The pollen beetle (Brassicogethes aeneus) is a major pest throughout Europe, with its importance increasing due to the development of insecticide resistance (Skellern and Cook, 2018). Feeding damage of adults induces bud abscission and may result in yield losses up to 70% (Nilsson, 1987; Williams, 2010). However, at low to moderate levels of infestation, bud losses can be compensated by newly produced racemes and buds or higher seed weight (Williams, 2010; Gagic et al., 2016).
Although there are several management tools in oilseed rape to control pests and diseases, the availability and diversity of these options strongly differs between the two types of biotic stresses (Table 3). While a relatively broad range of tools exist for disease control, including crop rotation, use of biological control agents, cultivation of resistant varieties, soil tillage and application of chemicals, insect control largely relies on insecticide use. By February 2020, four biocontrol agents have been registered for disease control in the EU, namely Coniothyrium minitans CON/M/91-08 against Sclerotinia sclerotiorum, Phythium oligandrum M1 against S. sclerotiorum and Leptosphaeria maculans, Bacillus amyloliquefaciens MBI 600 against L. maculans, and B. amyloliquefaciens QST 713 against Sclerotinia spp. Coniothyrium minitans CON/M/91-08 strongly reduced the carpogenic germination rate of sclerotia of S. sclerotiorum from 25 to 1.2% within 6 months (unpublished data). Still on research level, several candidate antagonists showed promising effects in controlling Verticillium longisporum and Plasmodiophora brassicae (Peng et al., 2011; Deketelaere et al., 2017; Zhu et al., 2019).
Crop rotation is a crucial factor affecting oilseed rape yields (Sieling et al., 1997; Sieling and Christen, 2015) and considered a tool to reduce risks of diseases mainly induced by soil-borne or soil-based pathogens, such as clubroot, Verticillium, and Sclerotinia which leave durable inoculum in the particular field. In addition, cultivars with enhanced resistance to stem canker and clubroot are available. Further progress is also made in breeding for resistance to Verticillium. Soil tillage represents a further tool which may reduce diseases through the management of plant debris carrying fungal inoculum. Taken together, a considerable range of measures for control of diseases in oilseed rape is available on the farm level.
In contrast to diseases, options for pest management are significantly scarcer. Since effective methods of biocontrol and cultivar resistance are lacking and the impact of crop rotation and soil tillage is rather limited, the main pillar of insect control in oilseed rape is insecticides (Table 3).
A long-term crop rotation study has been conducted near Göttingen, Germany (51°33′49″ N 9°56′49″ E) during 34 years (since 1986). Winter oilseed rape was grown every 2–4 years after winter wheat, winter wheat/winter barley, and winter wheat/oats/winter barley, respectively. Oilseed rape cultivars were chosen according to the most commonly grown in practice in the referred year (Figure 4). No fungicides were applied throughout the whole duration of the experiment. Herbicides were evenly applied on the whole area according to good agricultural practice, as well as insecticides, however, plots were split into two halves leaving one half without any insecticide treatment. Insecticide treatments were adjusted to actual pest situations in individual years (see Supplementary Table 3). The experiment was arranged in a split plot design with three replicates of each treatment and a plot size of 15 × 30 m.
Figure 4. Effect of crop rotation on yield of oilseed rape. Long-term crop rotation field experiment conducted since 1986 in Göttingen, Germany. No replication was conducted in the years 1990 and 1994. Experiment is arranged in a split-plot design with three replicates (plot size 15 × 30 m). Crops are grown according to good agricultural practice, however, without any fungicides. Bars indicate standard errors. Statistical analysis was conducted with t-test in each year. 2-year, oilseed rape cultivated every second year; 4-year, oilseed rape cultivated every 4 years; -, cultivar was not recorded; *P < 0.05; ***P < 0.001.
The results showed that compared to a 2-year rotation with winter wheat, a significant positive effect of the 4-year rotation on yield can be observed, however, only after 20 years of cultivation (Figure 4). The average yield difference between the 2 and 4-year rotation in 2006, 2010, and 2014 was 11.8% (4.9 dt/ha). This confirms previous studies which have shown significant yield losses in crop rotations with more than 40% cultivation of oilseed rape. In addition, these earlier studies which are based on a similarly designed long-term experiment, indicated a significant effect on grain yield of oilseed rape by the preceding crop and even the pre-preceding crop (Sieling et al., 1997; Sieling and Christen, 2015). However, in seasons with extreme weather conditions, such as drought, differences in yields due to crop rotation were strongly masked, as shown in the Göttingen experiment in 2018 (Figure 4). Moreover, in the experimental plots treated with insecticides following common agricultural practice, the negative yield effect caused by the 2-year rotation partly disappeared (Figure 5). These plots showed significant increases in yield compared to insecticide untreated plots, which further indicated that insect pests play an essential role in declining the potential yield of oilseed rape.
Figure 5. Effect of insecticide application on yield of oilseed rape in the long-term crop rotation experiment in Göttingen Germany. Three insecticide treatments were applied in 2014 on 11th March at growth stage (GS, Weber and Bleiholder, 1990) 33 with 0.2 l/ha of Trebon (ethofenprox) against pollen beetle and on 1st April and 23rd April at GS 61 with 0.3 l/ha of Biscaya (thiacloprid) against aphids. In the season 2017/2018, insecticides were applied three times on 28th September 2017 at GS 15 with 0.3 l/ha of Bulldock (beta-cyfluthrin), on 10th April 2018 at GS 38 with 0.2 l/ha of Trebon and on 17th April 2018 at GS 51 with 0.17 l/ha of Avaunt (indoxacarb) against pollen beetle, respectively. Bars indicate standard errors. Different letters denote significant differences among treatments in each year (LSD test, P < 0.05). 0, without insecticide application; +, with insecticide application, 2-year, oilseed rape cultivated every two years; 4-year, oilseed rape cultivated every 4 years.
A possible explanation for lower yields in narrow crop rotations is the accumulation of soil-borne or soil-associated host specific pathogens. This is implied by former studies relating the impact of shorter crop rotation on oilseed rape yield to the diversity of soil and rhizosphere microbes (Hilton et al., 2013, 2018; Floc'h et al., 2020). Two soil-borne plant pathogens Olpidium brassicae and Pyrenochaeta lycopersici were significantly accumulated in the field continuously grown with oilseed rape as compared to wider rotations (Hilton et al., 2013). Surprisingly, assessments of two major diseases occurring in the Göttingen experiment, Phoma stem canker, and Verticillium stem striping, did not indicate any significant increase in disease severity with narrowing the rotation, during 29 and 13 years of observation, respectively (Table 4). Sclerotinia stem rot did not establish in the plots at sufficiently high levels to be recordable. The potential explanation for these phenomena, that after such a long time period under monoculture a maximum level of inoculum has built up which cannot further increase, can be ruled out since the overall levels of the two diseases remained consistently low. Also, in a separate study, soil suppressive effects, which may have established after long-term mono-cropping, were not confirmed (data not shown).
Table 4. Long-term effects of crop rotation on severity of Phoma stem canker and Verticillium stem striping.
According to the experts' statements from the four global regions, the insecticide treatment frequency index in Europe and China has increased in the last 20 years, while the use of fungicides has remained constant or even decreased (Table 5). Besides, increased frequency of both insecticide and fungicide applications in recent years is reported from Canada and an increased use of fungicides has occurred in Australia. Although the demands for chemical treatments have been generally grown, the development of active ingredients with novel modes of action has not significantly changed or even decreased. The latter applies for insecticides, where new active ingredients have been rarely found in the last 25 years resulting in only four different modes of action available for oilseed rape in 2019 (voltage-dependent sodium channel blocker, sodium channel modulators, nicotinic acetylcholine receptor competitive modulators, and chordotonal organ transient receptor potential vanilloid family of ion channel modulators) (Table 6).
Table 6. Number of active ingredients (a.i.) and modes of action (MOA) registered for use in oilseed rape in Europe from 1995 to 2019.
In the last 5 years, no new insecticidal active ingredient has been approved in Europe (Bundesamt für Verbraucherschutz und Lebensmittelsicherheit, 2020a). Due to the limitations in use of registered active substances on account of securing bee health, the control of insect pests in oilseed rape currently heavily relies on pyrethroids. However, major pests like cabbage stem flea beetle, rape winter stem weevil, pollen beetle and green peach aphid have already developed high levels of resistance to pyrethroids in several countries, such as Germany, France, and the UK (Heimbach and Müller, 2013; Sekulic and Rempel, 2016; Insecticide Resistance Action Group, 2019b).
Due to the ban of neonicotinoid seed dressing in 2014 (including imidacloprid, clothianidin and thiamethoxam) and decreasing yields, the area grown to oilseed rape has significantly declined in Germany, from 1.465 Mio ha in 2013 to 856,800 ha in 2019 (Eurostat, 2020). Similarly, a strong reduction in oilseed rape cultivation and increased yield losses caused by the cabbage stem flea beetle, as reported from England, is considered a consequence of neonicotinoid and seed dressing ban (Scott and Bilsborrow, 2019). As there has been so far no evidence for strong resistance to neonicotinoids in the British green peach aphid population, the foliar application of thiacloprid has been an important tool in aphid management, until also banned in April 2020. However, in stone fruit orchards of Southern Europe (France, Spain, and Italy), and more recently in Greece, Morocco, Tunisia, and in vegetable greenhouses in Belgium, the green peach aphid has been found to carry the R81T target site mutation causing strong resistance to neonicotinoids (Slater et al., 2012; Insecticide Resistance Action Group, 2019a,b).
For fungicide applications in German oilseed rape fields, 17 active ingredients including nine candidates for substitution with six different modes of action are currently available for the management of the major diseases, except for Verticillium stem striping and clubroot, which represents a much more comfortable situation than with insecticides (Table 6).
In spite of the increased intensity of chemical crop protection applied in oilseed rape in recent decades, major biotic stresses have been generally on the rise, which indicates that the current management strategy is not sustainable. The experts' replies and recent reports from the agricultural practice (Menzler-Hokkanen et al., 2006; Arthey, 2020) indicate that insect pests currently are the major threat for oilseed rape in all global production regions, except for Australia. The exceptional role of Australia is both due to the regional differences in the range of prevalent insects (lack of flea beetles, pollen beetles, and stem weevils) and the larger number of available insecticides. In contrast to Australia, insect control has become particularly difficult in Europe due to two reasons, (i) the increase in resistance of several key insect pests to insecticides and (ii) a narrowed range of available modes of actions (Insecticide Resistance Action Group, 2019b; Bundesamt für Verbraucherschutz und Lebensmittelsicherheit, 2020a). This situation is further aggravated by the lack of alternative or preventive control measures available for insect pest management as outlined above. Among the missing tools of insect control, the general lack of effective cultivar resistance to insects appears to be the most severe constraint. This is in contrast to a diversity of options of disease control which are available to the farmers. Overall, the limited and decreasing competence in insect control, which threatens the profitability of the crop, has caused a substantial decrease in the production area in major oilseed rape producing countries such as France, Germany and UK (Arthey, 2020).
The current situation in hot spot regions of biotic stresses to oilseed rape like Europe requires novel strategies of pest management. Such innovative approaches should target the bottlenecks in the life cycle of pest populations. Continuous cultivation of oilseed rape for many years has led to the establishment of large populations of insect pests in the growing regions, which is indicated by the massive appearance of many species already in the early season enabling them to start an early attack on the crop at a high infestation level. A key factor is the high dispersal ability of major pest insects which allows them to roam on a landscape level (Table 7). While the five major fungal pathogens in oilseed rape are bound to the field, the insect pests are not, thus making the widening of crop rotation schemes on the field level a poorly efficient measure against insects. Insects such as the pollen beetle can disperse over several kilometers at a landscape scale by using upwind anemotaxis and following visual and olfactory cues (Skellern et al., 2017). Migrating insect pests which hibernate or aestivate in adjacent natural habitats or in previous oilseed rape fields can easily colonize new fields where their host plants are grown, thus compromising any crop alternation scheme on the farm level. In conclusion, effective management of insect pests in oilseed rape requires to operate on the landscape scale.
Table 7. Survival and range of dispersal of the top 5 important pests and diseases of oilseed rape in Europe.
A potential Achilles heel is the limited survival time of insects in the absence of host plants, which is significantly shorter than of fungal pathogens (Table 7). In fact, four out of five major insect pests in Europe have a survival potential of <1 year, which means that the new-generation adults rely on finding a field with their host plants in the following season and within their roaming distance. Organizing synchronous crop rotations on a landscape scale resulting in the total absence of host crops within larger areas for one or several years therefore appears to be a promising measure to disrupt the survival of regional pest populations. Such areas may be bordered and separated from neighboring growing areas by non-agricultural land such as forests, or natural landscape barriers such as mountains or water surfaces.
A landscape management approach has been tested in a large-scale experiment in the 1970s in North-West Germany, where oilseed rape cultivation was completely offset for one season on 29 farms in an area of 800 hectares (Schütte, 1979). Compared to an area with a regular, farm-level rotation scheme which was separated from the test area by a strip of 5–6 km without oilseed rape, in oilseed rape crops regrown in the following season pod infestation by the brassica pod midge (D. brassicae) was significantly decreased from 27.8 to 2.4%. Similar substantial effects were recorded for the cabbage seedpod weevil (C. obstrictus), while effects on the pollen beetle population were less pronounced. Although only conducted in one season, this experiment provides evidence that a regional synchronization of cropping schemes (“zoning”) may have a substantial effect in reducing populations of harmful insect pests.
We conclude that successful pest management has become crucial for the profitability of oilseed rape production in the hot spot zones in Europe and elsewhere. Since the current management measures such as field-bound and farm-level crop rotation fail to be effective and other preventive measures, including cultivar resistance, are lacking, an innovative approach is required. Synchronizing the regional crop frequency on the landscape level might be a promising innovation to sustainably reduce regionally established insect populations operating from habitats outside the fields. The effects of such strategy requires further research in order to explore the potential efficacy and applicability in the control of particular insect species. A broad cooperation and coordination among growers within larger growing regions will be required to establish such novel sustainable systems of insect control in oilseed rape production at the landscape level. Besides its expected higher effectiveness, such strategy would allow a substantial reduction in the amount of insecticide use. This strategy may be further supported by reduced or non-inversion soil tillage to increase the abundance and diversity of parasitoids and predators of important oilseed rape pests (Nilsson, 2010), further reducing the risks from insect pests. This strategy which may be suitable for European oilseed rape production, could be also useful in other global regions which encounter similar biotic constraints by insects. Overall, such novel strategies appear to be essential to safeguard the role of oilseed rape as a valuable crop in productive arable cropping schemes.
XZ and AT conceived of the presented idea and wrote the manuscript. BK and AT devised the questionnaire. BK, BU, and AT devised the long-term field experiment. XZ and BK analyzed the data. XZ, BK, BU, and AT discussed results and implications. All authors have read, reviewed, and approved the final manuscript.
The authors declare that the research was conducted in the absence of any commercial or financial relationships that could be construed as a potential conflict of interest.
We gratefully acknowledge the support of the following experts for participating in our survey and providing us with their valuable expertise: Ann-Charlotte Wallenhammar (Department of Crop Production Ecology, Swedish University of Agricultural Sciences, Sweden), Annette Penaud (Terres Inovia, France), Bruce Fitt (Department of Biological and Environmental Sciences, University of Hertfordshire, UK), Celine Robert (Terres Inovia, France), Clinton Jurke (Canola Council of Canada), Daohong Jiang (College of Plant Sciences and Technology, Huazhong Agricultural University), Detlev Dölger (Hanse Agro GmbH, Germany), Gunilla Berg (Swedish Board of Agriculture, Sweden), Holger Kreye (Landwirtschaftskammer Niedersachsen, Germany), Marek Seidenglanz (Department of Plant Protection, AGRITEC, Plant Research Ltd., Czech Republic), Martin Barbetti (School of Agriculture and Environment, The University of Western Australia, Australia), Olaf Gaue (Norddeutsche Pflanzenzucht Hans-Georg Lembke KG, Germany), Pankaj Sharma (Directorate of Rapeseed-Mustard Research, ICAR, India), Peter Höper (Norddeutsche Pflanzenzucht Hans-Georg Lembke KG, Germany), Robert Wilson (Australian Oilseeds Federation), Samantha Cook (Biointeractions and Crop Protection Department, Rothamsted Research, UK), Shengyi Liu (Oil Crop Research Institute, Chinese Academy of Agricultural Sciences, China), Shumin Hou (National Oil Crop Improvement Center, Anhui Academy of Agricultural Sciences, China), Steve Marcroft (Marcroft Grains Pathology, Australia), Xavier Pinochet (Terres Inovia, France), and Zhongping Hao (National Oil Crop Improvement Center, Anhui Academy of Agricultural Sciences, China). We are grateful to Hubertus Reintke, Evelin Vorbeck, and Jutta Schaper for their excellent work in the field experiments.
The Supplementary Material for this article can be found online at: https://www.frontiersin.org/articles/10.3389/fagro.2020.590908/full#supplementary-material
Abasova, L. V. (2018). Notes on powdery mildews of the genus Erysiphe from Azerbaijan. Cream 8, 30–53. doi: 10.5943/cream/8/1/3
Alford, D. V., Nilsson, C., and Ulber, B. (2003). “Insect pests of oilseed rape crops,” in Biocontrol of Oilseed Rape Pests. ed. D. V. Alford (Oxford, Malden, MA: Blackwell Science), 9–42. doi: 10.1002/9780470750988.ch2
Alkooranee, J. T., Yin, Y., Aledan, T. R., Jiang, Y., Lu, G., Wu, J., et al. (2015). Systemic resistance to powdery mildew in Brassica napus (AACC) and Raphanus alboglabra (RRCC) by Trichoderma harzianum TH12. PLoS ONE 10:e0142177. doi: 10.1371/journal.pone.0142177
Al-Shehbaz, I. A., Beilstein, M. A., and Kellogg, E. A. (2006). Systematics and phylogeny of the Brassicaceae (Cruciferae): an overview. Plant Syst. Evol. 259, 89–120. doi: 10.1007/s00606-006-0415-z
Arthey, T. (2020). Challenges and Perspectives in Global Rapeseed Production. Available on line at: http://www.agribenchmark.org/cash-crop/publications-and-projects0/reports/challenges-and-perspectives-in-global-rapeseed-production.html
Asif, M., SaqibMushtaq, M., Firdous, H., Zafar, M. M., Imran, A., Ahmad, T., et al. (2017). An overview of white rust disease in Brassica: taxonomical, biochemical aspects and management approaches. Discovery 53, 571–586.
Assefa, Y., Prasad, P. V. V., Foster, C., Wright, Y., Young, S., Bradley, P., et al. (2018). Major management factors determining spring and winter canola yield in North America. Crop Sci. 58, 1–16. doi: 10.2135/cropsci2017.02.0079
Aubertot, J.-N., Pinochet, X., and Dor,é, T. (2004). The effects of sowing date and nitrogen availability during vegetative stages on Leptosphaeria maculans development on winter oilseed rape. Crop Protect. 23, 635–645. doi: 10.1016/j.cropro.2003.11.015
Barbetti, M. J., and Khangura, R. K. (1999). Managing Blackleg in the Disease-Prone Environment of Western Australia. Available online at: http://www.regional.org.au/au/gcirc/3/8.htm (accessed September 15, 2020).
Bonnemaison, L. (1965). Insect pests of crucifers and their control. Annu. Rev. Entomol. 10, 233–256. doi: 10.1146/annurev.en.10.010165.001313
Braun, U., and Cook, R. T. A. (2012). Taxonomic Manual of the Erysiphales (powdery mildews). Utrecht: CBS-KNAW Fungal Biodiversity Centre.
Bundesamt für Verbraucherschutz und Lebensmittelsicherheit (2020a). List of Authorised Plant Protection Products in Germany: With Information On Terminated Authorisations. Available online at: https://www.bvl.bund.de/SharedDocs/Downloads/04_Pflanzenschutzmittel/psm_uebersichtsliste.pdf?__blob=publicationFileandv=9 (accessed September 15, 2020).
Bundesamt für Verbraucherschutz und Lebensmittelsicherheit (2020b). Zugelassene Pflanzenschutzmittel: Auswahl für Den Ökologischen Landbau nach der Verordnung (EG) Nr. 834/2007. Available online at: https://www.bvl.bund.de/SharedDocs/Downloads/04_Pflanzenschutzmittel/psm_oekoliste-DE.pdf?__blob=publicationFile (accessed September 15, 2020).
Cai, X., Huang, Y., Jiang, D., Fitt, B. D. L., Li, G., and Yang, L. (2017). Evaluation of oilseed rape seed yield losses caused by Leptosphaeria biglobosa in central China. Eur. J. Plant Pathol. 150, 179–190. doi: 10.1007/s10658-017-1266-x
Canadian Food Inspection Agency (2017). Pest Risk Management Document: Verticillium longisporum (Verticillium stripe). Available online at: http://www.inspection.gc.ca/plants/plant-pests-invasive-species/directives/risk-management/rmd-17-01/eng/1487004855251/1487004951480 (accessed November 13, 2018).
Ciola, V., and Cipollini, D. (2011). Distribution and host range of a powdery mildew fungus infecting garlic mustard, Alliaria petiolata, in Southwestern Ohio. Am. Midland Nat. 66, 40–52. doi: 10.1674/0003-0031-166.1.40
Cramer, H. H. (1967). Plant Protection and World Crop Production. Leverkusen: Farbenfabriken Bayer AG.
Deketelaere, S., Tyvaert, L., França, S. C., and Höfte, M. (2017). Desirable traits of a good biocontrol agent against verticillium wilt. Front. Microbiol. 8:1186. doi: 10.3389/fmicb.2017.01186
Del Río, L. E., Bradley, C. A., Henson, R. A., Endres, G. J., Hanson, B. K., McKay, K., et al. (2007). Impact of Sclerotinia stem rot on yield of canola. Plant Dis. 91, 191–194. doi: 10.1094/PDIS-91-2-0191
Department for Environment Food and Rural Affairs (2017). Farming Statistics, Provisional Crop Areas, Yields and Livestock Populations at June 2017. Available online at: https://assets.publishing.service.gov.uk/government/uploads/system/uploads/attachment_data/file/651173/structure-jun2017prov-UK-12oct17.pdf (accessed October 8, 2020).
Depotter, J. R. L., Deketelaere, S., Inderbitzin, P., von Tiedemann, A., Höfte, M., Subbarao, K. V., et al. (2016). Verticillium longisporum, the invisible threat to oilseed rape and other brassicaceous plant hosts. Mol. Plant Pathol. 17, 1004–1016. doi: 10.1111/mpp.12350
Depotter, J. R. L., Thomma, B. P. H. J., and Wood, T. A. (2019). Measuring the impact of Verticillium longisporum on oilseed rape (Brassica napus) yield in field trials in the United Kingdom. Eur. J. Plant Pathol. 153, 321–326. doi: 10.1007/s10658-018-1537-1
Dimov, Z., and Möllers, C. (2010). Genetic variation for saturated fatty acid content in a collection of European winter oilseed rape material (Brassica napus). Plant Breed. 129, 82–86. doi: 10.1111/j.1439-0523.2009.01652.x
Donald, C., and Porter, I. (2009). Integrated control of clubroot. J. Plant Growth Regul. 28, 289–303. doi: 10.1007/s00344-009-9094-7
Döring, A. (2012). Effect of Plant Characteristics on Host Plant Selection and Larval Performance of Specialist Insect Pests on Brassicaceae. Göttingen: Cuvillier Verlag.
Dunker, S., Keunecke, H., Steinbach, P., and von Tiedemann, A. (2008). Impact of Verticillium longisporum on yield and morphology of winter oilseed rape (Brassica napus) in relation to systemic spread in the plant. J. Phytopathol. 156, 698–707. doi: 10.1111/j.1439-0434.2008.01429.x
Eurostat (2020). Rape, Turnip Rape, Sunflower Seeds and Soya by Area. Available online at: https://ec.europa.eu/eurostat/databrowser/view/tag00100/default/table?lang=en (accessed October 8, 2020)
Eynck, C., Koopmann, B., and von Tiedemann, A. (2009). Identification of Brassica accessions with enhanced resistance to Verticillium longisporum under controlled and field conditions. J. Plant Dis. Protect. 116, 63–72. doi: 10.1007/BF03356288
Finch, S., and Collier, R. H. (2000). Host-plant selection by insects - a theory based on 'appropriate/inappropriate landings' by pest insects of cruciferous plants. Entomol. Exp. et Applicata 96, 91–102. doi: 10.1046/j.1570-7458.2000.00684.x
Fitt, B. D. L., Brun, H., Barbetti, M. J., and Rimmer, S. R. (2006). World-wide importance of phoma stem canker (Leptosphaeria maculans and L. biglobosa) on oilseed rape (Brassica napus). Eur. J. Plant Pathol. 114, 3–15. doi: 10.1007/s10658-005-2233-5
Floc'h, J.-B., Hamel, C., Harker, K. N., and St-Arnaud, M. (2020). Fungal communities of the canola rhizosphere: keystone species and substantial between-year variation of the rhizosphere microbiome. Microbial Ecol. 80, 762–777. doi: 10.1007/s00248-019-01475-8
Gagic, V., Riggi, L. G., Ekbom, B., Malsher, G., Rusch, A., and Bommarco, R. (2016). Interactive effects of pests increase seed yield. Ecol. Evol. 6, 2149–2157. doi: 10.1002/ece3.2003
Gladders, P., and Musa, T. M. (1980). Observations on the epidemiology of Leptosphaeria macutans stem canker in winter oilseed rape. Plant Pathol. 29, 28–37. doi: 10.1111/j.1365-3059.1980.tb01134.x
Gladders, P., Richie, F., Babara, D., Clarkson, J., Chantry, T., Taylor, M., et al. (2013). Importance and Management of Verticillium wilt in Winter Oilseed Rape. London: Home-Grown Cereals Authority, Agriculture and Horticulture Development Board.
Gunasinghe, N., You, M. P., Cawthray, G. R., and Barbetti, M. J. (2016). Cercosporin from Pseudocercosporella capsellae and its critical role in white leaf spot development. Plant Dis. 100, 1521–1531. doi: 10.1094/PDIS-10-15-1192-RE
Hall, R., Peters, R. D., and Assabgui, R. A. (1993). Occurrence and impact of blackleg on oilseed rape in Ontario. Can. J. Plant Pathol. 15, 305–313. doi: 10.1080/07060669309501928
Heimbach, U., and Müller, A. (2013). Incidence of pyrethroid-resistant oilseed rape pests in Germany. Pest Manag. Sci. 69, 209–216. doi: 10.1002/ps.3351
Hilton, S., Bennett, A. J., Chandler, D., Mills, P., and Bending, G. D. (2018). Preceding crop and seasonal effects influence fungal, bacterial and nematode diversity in wheat and oilseed rape rhizosphere and soil. Appl. Soil Ecol. 126, 34–46. doi: 10.1016/j.apsoil.2018.02.007
Hilton, S., Bennett, A. J., Keane, G., Bending, G. D., Chandler, D., Stobart, R., et al. (2013). Impact of shortened crop rotation of oilseed rape on soil and rhizosphere microbial diversity in relation to yield decline. PLoS ONE 8:e59859. doi: 10.1371/journal.pone.0059859
Huang, Y.-J., Fitt, B. D. L., Jedryczka, M., Dakowska, S., West, J. S., Gladders, P., et al. (2005). Patterns of ascospore release in relation to phoma stem canker epidemiology in England (Leptosphaeria maculans) and Poland (Leptosphaeria biglobosa). Eur. J. Plant Pathol. 111, 263–277. doi: 10.1007/s10658-004-4421-0
Hwang, S.-F., Strelkov, S. E., Peng, G., Ahmed, H., Zhou, Q., and Turnbull, G. (2016). Blackleg (Leptosphaeria maculans) severity and yield loss in canola in Alberta, Canada. Plants 5:31. doi: 10.3390/plants5030031
Insecticide Resistance Action Group (2019a). Resistance Management Guideline for Controlling R81T Target Site Resistant Myzus Persicae Populations, IRAC SPWG, 2019. Available online at: https://irac-online.org/documents/myzus-persicae-irm-english/?ext=pdf (accessed October 8, 2020).
Insecticide Resistance Action Group (2019b). Insecticide Resistance Status in UK Oilseed Rape Crop. Available online at: https://media.ahdb.org.uk/media/Default/Imported%20Publication%20Docs/AHDB%20Cereals%20&%20Oilseeds/Pests/IRAG3%20%20Insecticide%20resistance%20status%20in%20UK%20oilseed%20rape%20crops.pdf (accessed October 8, 2020).
Johnen, A., Williams, I. H., Nilsson, C., Klukowski, Z., Luik, A., and Ulber, B. (2010). “The proPlant decision support system: Phenological models for the major pests of oilseed rape and their key parasitoids in Europe,” in Biocontrol-based integrated management of oilseed rape pests. ed. I. H. Williams (Dordrecht: Springer), 381–403. doi: 10.1007/978-90-481-3983-5_15
Juran, I., Gothlin Culjak, T., and Grubišić, D. (2011). Rape stem weevil (Ceutorhynchus napi Gyll. 1837) and cabbage stem weevil (Ceutorhynchus pallidactylus Marsh. 1802) (Coleoptera: Curculionidae) – important oilseed rape pests. Agric. Consp. Sci. 76, 93–100.
Keunecke, H. (2009). Impact of cabbage root fly on infections and damage potential of Verticillium longisporum and Phoma lingam in oilseed rape. (PhD dissertation). Georg August University, Göttingen, Germany.
Kirkegaard, J. A., Robertson, M. J., Hamblin, P., and Sprague, S. J. (2006). Effect of blackleg and Sclerotinia stem rot on canola yield in the high rainfall zone of southern New South Wales, Australia. Aust. J. Agric. Res. 57, 201–212. doi: 10.1071/AR05179
Koh, J. C. O., Barbulescu, D. M., Norton, S., Redden, B., Salisbury, P. A., Kaur, S., et al. (2017). A multiplex PCR for rapid identification of Brassica species in the triangle of U. Plant Methods 13:49. doi: 10.1186/s13007-017-0200-8
Krüger, W. (1982). The root collar and stem rot of oil-seed rape caused by Phoma lingam (stat. gen. Leptosphaeria maculans), a disease difficult to control. Zeitschrift für Pflanzenkrankheiten und Pflanzenschutz / J. Plant Dis. Protect. 89, 498–507.
Kutcher, H. R., Warland, J. S., and Brandt, S. A. (2010). Temperature and precipitation effects on canola yields in Saskatchewan, Canada. Agr. Forest Meteorol. 150, 161–165. doi: 10.1016/j.agrformet.2009.09.011
Link, H. V., and Johnson, K. B. (2007). White Mold (Sclerotinia). Available online at: https://www.apsnet.org/edcenter/disandpath/fungalasco/pdlessons/Pages/WhiteMold.aspx (accessed September 15, 2020).
McGrann, G. R. D., Gladders, P., Smith, J. A., and Burnett, F. (2016). Control of clubroot (Plasmodiophora brassicae) in oilseed rape using varietal resistance and soil amendments. Field Crops Res. 186, 146–156. doi: 10.1016/j.fcr.2015.11.013
Menzler-Hokkanen, I., Hokkanen, H. M. T., Buchs, W., Klukowski, Z., Luik, A., Nilsson, C., et al. (2006). Insect problems in European oilseed rape cultivation, and how to deal with them: the OSR farmers' perspective. Bull. IOBC/WPRS/SROP 29, 91–94.
Milovac, Ž., Zorić, M., Franeta, F., Terzić, S., Petrović Obradović, O., and Marjanović Jeromela, A. (2017). Analysis of oilseed rape stem weevil chemical control using a damage rating scale. Pest Manag Sci. 73, 1962–1971. doi: 10.1002/ps.4568
Mizubuti, E. S. G. (2019). Special issue on white mold - Sclerotinia sclerotiorum. Trop. Plant Pathol. 44, 1–2. doi: 10.1007/s40858-019-00276-1
Murakami, H., Tsushima, S., Akimoto, T., Kuroyanagi, Y., and Shishido, Y. (2004). Quantitative studies on the relationship between plowing into soil of clubbed roots of preceding crops caused by Plasmodiophora brassicae and disease severity in succeeding crops. Soil Sci. Plant Nutr. 50, 1307–1311. doi: 10.1080/00380768.2004.10408609
Nilsson, C. (1987). Yield losses in summer rape caused by pollen beetles (Meligethes spp.). Swed. J. Agric. Res. 17, 105–112.
Nilsson, C. (2010). “Impact of soil tillage on parasitoids of oilseed rape pests,” in Biocontrol-Based Integrated Management Of Oilseed Rape Pests, ed. I. H. Williams (New York, NY: Springer), 305–311. doi: 10.1007/978-90-481-3983-5_11
Noleppa, S. (2017). Banning Neonicotinoids in the European Union: An Ex-post Assessment of Economic and Environmental Costs. Berlin, Germany.
Peng, G., McGregor, L., Lahlali, R., Gossen, B. D., Hwang, S. F., Adhikari, K. K., et al. (2011). Potential biological control of clubroot on canola and crucifer vegetable crops. Plant Pathol. 60, 566–574. doi: 10.1111/j.1365-3059.2010.02400.x
Piliponyte-Dzikiene, A., Kaczmarek, J., Petraitiene, E., Kasprzyk, I., Brazauskiene, I., Brazauskas, G., et al. (2014). Microscopic and molecular detection of Leptosphaeria maculans and L. biglobosa ascospore content in air samples. Zemdirbyste Agric. 101, 303–312. doi: 10.13080/z-a.2014.101.039
Reddy, G. V. P. (2017). Integrated Management of Insect Pests on Canola and OTHER BRASSICA OILSEED CROPs. Boston, MA: CABI. doi: 10.1079/9781780648200.0000
Ren, Y., Zhu, J., Hussain, N., Ma, S., Ye, G., Zhang, D., et al. (2014). Seedling age and quality upon transplanting affect seed yield of canola (Brassica napus L.). Can. J. Plant Sci. 94, 1461–1469. doi: 10.4141/cjps-2014-021
Ríčarová, V., Kazda, J., Singh, K., and Ryšánek, P. (2016). Clubroot caused by Plasmodiophora brassicae Wor. a review of emerging serious disease of oilseed rape in the Czech Republic. Plant Protect. Sci. 52, 71–86. doi: 10.17221/87/2015-PPS
Schütte, F. (1979). Integrierte Bekämpfung von Rapsschädlingen durch Vermeidung des Zusammentreffens mit ihren Wirtspflanzen. Hamburg: Verlag Paul Parey.
Scott, C., and Bilsborrow, P. E. (2019). The impact of the EU neonicotinoid seed-dressing ban on oilseed rape production in England. Pest Manag. Sci. 75, 125–133. doi: 10.1002/ps.5189
Sekulic, G., and Rempel, C. B. (2016). Evaluating the role of seed treatments in canola/oilseed rape production: integrated pest management, pollinator health, and biodiversity. Plants 5:32. doi: 10.3390/plants5030032
Siebold, M., and von Tiedemann, A. (2013). Effects of experimental warming on fungal disease progress in oilseed rape. Glob. Change Biol. 19, 1736–1747. doi: 10.1111/gcb.12180
Sieling, K., and Christen, O. (2015). Crop rotation effects on yield of oilseed rape, wheat and barley and residual effects on the subsequent wheat. Arch. Agron. Soil Sci. 22, 1–19. doi: 10.1080/03650340.2015.1017569
Sieling, K., Christen, O., Nemati, B., and Hanus, H. (1997). Effects of previous cropping on seed yield and yield components of oil-seed rape (Brassica napus L.). Eur. J. Agron. 6, 215–223. doi: 10.1016/S1161-0301(96)02049-7
Skellern, M. P., and Cook, S. M. (2018). The potential of crop management practices to reduce pollen beetle damage in oilseed rape. Arthropod Plant Interac. 12, 867–879. doi: 10.1007/s11829-017-9571-z
Skellern, M. P., Welham, S. J., Watts, N. P., and Cook, S. M. (2017). Meteorological and landscape influences on pollen beetle immigration into oilseed rape crops. Agric. Ecosyst. Environ. 241, 150–159. doi: 10.1016/j.agee.2017.03.008
Slater, R., Paul, V. L., Andrews, M., Garbay, M., and Camblin, P. (2012). Identifying the presence of neonicotinoidresistant peach-potato aphid (Myzus persicae) in the peach-growing regions of southern France and northern Spain. Pest Manage. Sci. 68, 634–638. doi: 10.1002/ps.2307
Strehlow, B., Mol, F., and andd de Struck, C. (2015). Risk potential of clubroot disease on winter oilseed rape. Plant Dis. 99, 667–675. doi: 10.1094/PDIS-05-14-0482-RE
Subudhi, P. K., and Raut, R. N. (1994). White rust resistance and its association with parental species type and leaf waxiness in Brassica juncea L. Czern and Coss Brassica napus L. crosses under the action of EDTA and gamma-ray. Euphytica 74, 1–7. doi: 10.1007/BF00033760
Travadon, R., Bousset, L., Saint-Jean, S., Brun, H., and Sache, I. (2007). Splash dispersal of Leptosphaeria maculans pycnidiospores and the spread of blackleg on oilseed rape. Plant Pathol. 56, 595–603. doi: 10.1111/j.1365-3059.2007.01572.x
Wallenhammar, A. C. (1996). Prevalence of Plasmodiophora brassicae in a spring oilseed rape growing area in central Sweden and factors influencing soil infestation levels. Plant Pathol. 45, 710–719. doi: 10.1046/j.1365-3059.1996.d01-173.x
Wallenhammar, A. C. (1998). Observations on yield loss from Plasmodiophora brassicae infections in spring oilseed rape. J. Plant Dis. Protect. 105, 1–7.
Warwick, S. I., Mummenhoff, K., Sauder, C. A., Koch, M. A., and Al-Shehbaz, I. A. (2010). Closing the gaps: phylogenetic relationships in the brassicaceae based on DNA sequence data of nuclear ribosomal ITS region. Plant Syst. Evol. 285, 209–232. doi: 10.1007/s00606-010-0271-8
Weber, E., and Bleiholder, H. (1990). Erläuterungen zu den BBCH-Dezimal-Codes für die Entwicklungsstadien von Mais, Raps, Faba-Bohne, Sonnenblume und Erbse - mit Abbildungen. Gesunde Pflanzen 42, 308–321.
West, J. S., Biddulph, J. E., Fitt, B. D. L., and Gladders, P. (1999). Epidemiology of Leptosphaeria maculans in relation to forecasting stem canker severity on winter oilseed rape in the UK. Ann. Appl. Biol. 135, 535–546. doi: 10.1111/j.1744-7348.1999.tb00885.x
West, J. S., Kharbanda, P. D., Barbetti, M. J., and Fitt, B. D. L. (2001). Epidemiology and management of Leptosphaeria maculans (phoma stem canker) on oilseed rape in Australia, Canada and Europe. Plant Pathol. 50, 10–27. doi: 10.1046/j.1365-3059.2001.00546.x
Williams, I. H. (2010). “The major insect pests of oilseed rape in Europe and their management: An overview,” in Biocontrol-based integrated management of oilseed rape pests, ed. I. H. Williams (Dordrecht: Springer), 1–43. doi: 10.1007/978-90-481-3983-5_1
Wynn, S., Ecclestone, E., and Carter, R. (2017). Cabbage Stem Flea Beetle Live Incidence and Severity Monitoring Autumn 2016 and Spring 2017. Project report No. 571. Agriculture and Horticulture Development Board. Available online at: https://projectblue.blob.core.windows.net/media/Default/Research%20Papers/Cereals%20and%20Oilseed/21120050-csfb-adult-damage-survey-final-project-report-final-corrected.pdf (accessed October 8, 2020).
Yang, Q., Liu, W., and Huang, C. (2018). Statistics and analysis of oilseed rape losses caused by main diseases and insect pests in recent 10 years. Plant Protect. 44, 24–30.
Yu, S., Su, T., Chen, J., Wang, W., Zhang, F., Yu, Y., et al. (2015). Real-time PCR as a diagnostic tool for evaluating the resistance of Chinese cabbage cultivars to Verticillium wilt. Eur. J. Plant Pathol. 143, 549–557. doi: 10.1007/s10658-015-0706-8
Zheng, X., Loppisso, D. T., Eseola, A. B., Koopmann, B., and von Tiedemann, A. (2019a). Potential for seed transmission of Verticillium longisporum in oilseed rape (Brassica napus L.). Plant Dis. 103, 1843–1849. doi: 10.1094/PDIS-11-18-2024-RE
Zheng, X., Pfordt, A., Khatri, L., Eseola, A. B., Wilch, A., Koopmann, B., et al. (2019b). Contrasting patterns of colonization with Verticillium longisporum in winter and spring type oilseed rape (Brassica napus L.) in the field and greenhouse and the role of soil temperature. Plant Dis. 103, 2090–2099. doi: 10.1094/PDIS-01-19-0236-RE
Zhou, Y., Fitt, B. D. L., Welham, S. J., Gladders, P., Sansford, C. E., and West, J. S. (1999). Effects of severity and timing of stem canker (Leptosphaeria maculans) symptoms on yield of winter oilseed rape (Brassica napus) in the UK. Eur. J. Plant Pathol. 105, 715–728. doi: 10.1023/A:1008761219493
Keywords: oilseed rape (B. napus L.), disease management, pest management, crop rotation, management strategies
Citation: Zheng X, Koopmann B, Ulber B and von Tiedemann A (2020) A Global Survey on Diseases and Pests in Oilseed Rape—Current Challenges and Innovative Strategies of Control. Front. Agron. 2:590908. doi: 10.3389/fagro.2020.590908
Received: 03 August 2020; Accepted: 28 September 2020;
Published: 22 October 2020.
Edited by:
Francesco Spinelli, University of Bologna, ItalyCopyright © 2020 Zheng, Koopmann, Ulber and von Tiedemann. This is an open-access article distributed under the terms of the Creative Commons Attribution License (CC BY). The use, distribution or reproduction in other forums is permitted, provided the original author(s) and the copyright owner(s) are credited and that the original publication in this journal is cited, in accordance with accepted academic practice. No use, distribution or reproduction is permitted which does not comply with these terms.
*Correspondence: Andreas von Tiedemann, YXRpZWRlbUBnd2RnLmRl
Disclaimer: All claims expressed in this article are solely those of the authors and do not necessarily represent those of their affiliated organizations, or those of the publisher, the editors and the reviewers. Any product that may be evaluated in this article or claim that may be made by its manufacturer is not guaranteed or endorsed by the publisher.
Research integrity at Frontiers
Learn more about the work of our research integrity team to safeguard the quality of each article we publish.