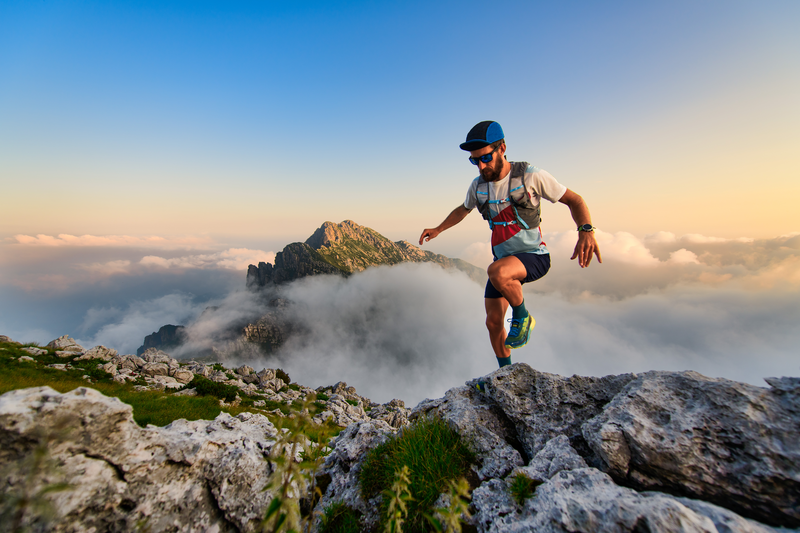
95% of researchers rate our articles as excellent or good
Learn more about the work of our research integrity team to safeguard the quality of each article we publish.
Find out more
ORIGINAL RESEARCH article
Front. Agron. , 12 November 2020
Sec. Disease Management
Volume 2 - 2020 | https://doi.org/10.3389/fagro.2020.582268
This article is part of the Research Topic New Trends in Integrated Plant Disease Management View all 5 articles
Phytochemicals with antimicrobial and antioxidant properties have tremendous potential in suppressing both plant and human diseases. Screening and identification of such compounds from diverse plant species is the first step toward realizing their medicinal and agricultural application. In agriculture, application of antimicrobial phytochemicals as part of an Integrated Disease Management strategy would reduce the detrimental residual effect of synthetic chemicals. In the present study, an invasive plant species, i.e., Lantana camara L. was screened for potential antimicrobial and antioxidant phytochemicals. Extracts of leaves (LE) and flowers (FE) from L. camara were tested for their polyphenol content (total phenol, total flavonoid, and total alkaloid) and antioxidant potential [total antioxidant activity, iron chelating activity and enzymatic activity (peroxidase and polyphenol oxidase)]. Both extracts exhibited high antioxidant and free radical scavenging activities with relatively stronger antioxidant activity in the case of whole flower extracts. FTIR and GC-MS also carried out for chemical analysis of crude extracts. FTIR spectral study of LE and FE revealed the presence of different functional groups such as N–H, C–H, OH, C = O indicates the existence of various metabolites in the extracts. GC-MS study revealed the presence of 66 bioactive compounds, of which 19 components were predicted to have various functions like anti-inflammatory, antiandrogenic, anti-tumor, antimicrobial, etc. Furthermore, in vitro antifungal and antibacterial studies confirmed the antimicrobial effect of both LE and FE against phytopathogens like Magnaporthe oryzae, Xanthomonas axonopodis pv. glycines (Xag) and Xanthomonas oryzae pv. oryzae (Xoo). Molecular study further revealed that phytochemicals (Loliolide, Eicosapentaenoic acid, Salicylic Acid Methyl Ester, and Phytol) of Lantana camara could targets MAPK1, PDF, and SUH like enzymes of phytopathogens, which could inhibit their growth. Therefore, L. camara extracts can be an important ingredient in the suppression of plant diseases.
Plants are a major source of medicine with a variety of biological deliberations, including antioxidant, antibacterial, and antifungal activities. Almost 25% of conventional drugs and primary health care of majority of world population relies essentially on plants (Ekor, 2014). Natural antioxidants are broad-spectrum, safe and effective in regulating destructive processes triggered by oxidative stress, induced by free radical's overproduction (Tan et al., 2018). Modern medicine mostly uses synthetic/semi-synthetic antibiotics for dealing with microbial diseases. However, many microbes have developed resistance to antibiotics, resulted as therapeutic failure. Additionally, modern antibiotics are very expensive. Hence, the situation has led to increase the use of plant extract and their derived compounds (Hassine et al., 2014). Earlier studies have shown the curative potential of several botanicals against chronic diseases like cancer, diabetes, inflammation, stroke, aging etc. (Kalita et al., 2012). These reports indicate that plants still represent an important pool for the discovery of novel drugs and therapeutic compounds.
Unlike plant cells, human cells sometimes do not produce adequate amounts of antioxidants (Pisoschi et al., 2009). The use of synthetic antioxidants like Butylated Hydroxy Toluene (BHT), ethoxquin, propyl gallate, Tert-butylhydroquinone (TBHQ), and Butylated Hydroxyanisole (BHA) etc. to mitigate oxidative stress were often associated with toxicity, carcinogenicity, and mutagenic effect (Bendiabdellah et al., 2012). Therefore, alternative natural and safer source of antioxidant is required, which can be given as a supplement. A plant-based antioxidant such as vitamins, phenolics, flavonoids, tannins, and carotenes are considered to play an imperative role through the process of oxidation by reacting with free radicals and protecting cells from free radical-induced damage without causing any side effect (Pisoschi et al., 2009; Prasad et al., 2010). Prior work has shown the positive response of taking high dietary natural antioxidants over chronic heart diseases and cancer (Prasad et al., 2010). Therefore, the demand for natural antioxidants has increased immensely in recent years in pharmaceuticals and nutraceuticals in addition to food additives.
Similar circumstances exist in agriculture with respect to crop diseases. Fungi and bacteria are mostly responsible for major crop diseases and yield loss (Kumar et al., 2020). Farmers usually apply synthetic pesticides to control the diseases and save yield as a quick pest management option. However, the residues of synthetic pesticides are highly toxic and have shown hazardous effects on non-targeted organisms, thus it impacts negatively on biodiversity. Additionally, indiscriminate and unmethodical use have led to the development of resistant pathogen strains and disease resurgence. Therefore, the better specific pesticide has to be developed capable of controlling plant diseases. The alternative to synthetic pesticides is plant-derived eco-friendly greener botanical pesticides. Importantly, active plant compounds are less toxic and biodegradable (Fayaz et al., 2017).
The current global trends are more toward organic foods. Consumer's awareness of food safety has increased tremendously, which has resulted to ban certain pesticides in agriculture. Consequently, botanical pesticides have got momentum because of their efficacy, biodegradability, less toxicity and humongous availability of source materials (Campos et al., 2019). There are many plant species which are less exploited and one such species is L. camara L. It belongs to Verbenaceae family, is a notorious invasive weed reported to be native of tropical, subtropical and temperate regions of more than 60 countries (Ghisalberti, 2000). Extract of L. camara has been used by traditional healers for centuries to cure various ailments such as gastrointestinal, inflammatory, dermatological, rheumatism, fever, and headache (Hernández et al., 2003; Mahdi-Pour et al., 2012). Interestingly, different species of Lantana have been reported to contain the diverse type and level of phytochemicals (Sharma et al., 1991). Therefore, L. camara L. of Amarkantak, MP, India (High altitude of ~3,438 ft) may contain different type and level of phytoconstituents.
Therefore, the present study aims to systematically investigate the methanolic crude extract of Leaf (LE) and flower (FE) for its phytochemical properties, free radical scavenging activity, and antimicrobial activities. FTIR and GC-MS studies were also performed to find out functional groups and active antimicrobial compounds in the extract of L. camara.
Fresh L. camara leaves and flower was collected from Podki village (geographical coordinates 22°47′ 3″ N and 81°43′ 44″ E) near Indira Gandhi National Tribal University (IGNTU) campus, Annuppur, MP, India (Supplementary Figure 1). The identification and authentication of plant taxonomy were performed at IGNTU, Department of Botany. The plant leaves and flowers were washed softly through tap water to eliminate undesirable dirt particles. Subsequently, samples were air-dried under shade. Slant culture of M. oryzae were purchased from National Research Center on Plant Biotechnology, New Delhi.
All chemicals such as gallic acid, sodium carbonate, ammonium molybdate, sulfuric acid, sodium phosphate, ascorbic acid, potassium ferricyanide, TCA (trichloroacetic acid), quercetin, 2,2-diphenyl-1-picrylhydrazyl (DPPH), ABTS reagent 2,2′-azinobis-(3-ethylbenzothiaziline-6-sulfonate), catechol, ferrozine, ferrous sulfate and guaiacol were purchased from Hi-Media, India. Hydrogen peroxide and Folin-Ciocalteu reagent were from Central Drug House (P) Ltd. India. All the chemicals were of analytical grade. Working standards and samples were made by diluting stock solution (1 mg/ml) in methanol and double distilled water based on an experiment to give final concentrations. The different solvent used in this study were of analytical grade.
Freshly harvested leaves and flowers were air-dried and powdered with a mechanical grinder, each of 10 grams. Subsequently, samples were applied for extraction in a Soxhlet apparatus using 150 ml of methanol. The extract was then filtered through a Whatman No. 1 paper filter (Whatman International Ltd., Maidstone, England) and concentrated on the dry mass at room temperature in a fume hood. The quantity of extracts yield was evaluated and residues were stored in dark to use further. The designation of samples was excuted as LE (for leaf extract) and FE (for flower extract). The dried extract was dissolved in methanol and distilled water based on an experiment to give final concentrations.
Phytochemical screening of L. camara LE and FE was performed as per the method described previously to unveil the presence of saponins, tannins, flavonoids, steroids, anthocyanin (Harborne, 1998), and alkaloids, terpenoids, glycosides, quinones, cardiac glycosides, caumarins, phlobatannins, anthraquinones, phenols (Roghini and Vijayalakshmi, 2018).
The phenolics in extracts was evaluated through spectrophotometric method (Singleton et al., 1999). The methanolic solution was prepared by mixing 0.5 ml (1 mg/ml) extract, water dissolved 10% Folin-Ciocalteu's reagent 2.5 ml, and 7.5% Na2CO3 2.5 ml and the whole solution was agitated to homogenize the dilution. Blank was concomitantly prepared in a similar manner using 0.5 ml methanol instead of extract. After incubating samples at 45°C for 45 min in the dark in a thermostat, absorbance was measured at λmax = 730 nm using a spectrophotometer. Triplicate was maintained to obtain mean values of sample. The calibration curve of gallic acid was constructed in the range of 20–100 μg/ml. Lastly, phenolics concentration were expressed in gallic acid equivalent terms (mg GA/g of dw).
The total flavonoid content (TFC) of FE and LE was measured through AlCl3 colorimetry method (Chang et al., 2002). Both FE and LE extracts were diluted with methanol till the concentration reached 1mg/ml and a calibration curve was drawn using methanol dissolved quercetin (20–100 μg/ml). 2.0 ml diluted extracts/quercetin was mixed with AlCl3 [0.1 ml of 10 % (w/v)], and CH3COOK (0.1 ml of 0.1 mM) and whole solution was prepared in methanol. Subsequently, absorbance was measured at λmax = 415 nm post 30 min of incubation at 25°C. Finally, total flavonoid content in the extracts was represented as mg quercetin equivalent per gram dry weight of extracts (mg Q/g of dw).
Total alkaloid content (TAC) in the extract was evaluated using standard method (Harborne, 1973). Both LE and FE (2.50 gm) were taken in a beaker in which 200 ml of 10% CH3COOH in methanol was added and were incubated for 4 h at RT. Then, concentrated NH4OH was added dropwise until complete precipitation. Subsequently, supernatant was removed and precipitates were washed with 20 ml of 0.1 M of NH4OH. Lastly, filtrate residue was dried in an oven and alkaloid content was measured in terms of percentage.
Percent alkaloid = Weight of alkaloid ×100/Weight of sample.
The total antioxidant activity (TAA) of the LE and FE was evaluated standard method (Govindarajan et al., 2003; Subhasree et al., 2009) with slight modifications. In brief, LE and FE stock concentration was made to 1 mg/ml with double distilled water. An aliquot of 0.2 ml of each extract was mixed with 1.8 ml of reagent (0.6 M H2SO4, 28 mM Na3PO4, and 4 mM (NH4)6Mo7O24). Then, the reaction mixture was incubated in water bath at 90°C for 90 min, followed by cooling at room temperature (RT). Absorbance of the sample was read at λmax = 695 nm through UV-VIS spectrophotometer. AA was taken as standard (20–100 μg/ml) and TAA result was represented as mg of ascorbic acid equivalent per gram of plant extract.
The TAA of the extract was determined through standard Fe3+ reducing power assay (Chu et al., 2000). Both extracts and standard (ascorbic acid) (0.5 ml) were thoroughly mixed with 2.5 ml of phosphate buffer (0.2 M, pH 6.6) and 1% 2.5 ml of K3[Fe (CN)6] (potassium ferricyanide). The incubation of reaction mixture was done at 50°C for 30 min. Then, 2.5 ml (10%) of C2HCl3O2 (trichloroacetic acid) was added and the whole blend was centrifuged at 13,000 rpm for 10 min. Finally, 2.5 ml supernatant was separated and mixed with 2.5 ml DDW and 0.5 ml (0.1%) of FeCl3. The absorbance was taken at λmax = 700 nm using UV-VIS spectrophotometer. The reducing power of the extract was expressed as optical density of tested extract.
The 2,2- diphenyl-1-picrylhydrazyl (DPPH) assay was performed to evaluate the free radical scavenging activity of the extract following previously described methods (Bursal and Gulcin, 2011; Kumar et al., 2011, 2013). DPPH solution (0.004%) was prepared in 100 ml of methanol to achieve 0.98 ± 0.02 absorbance at λmax = 517 nm. An aliquot of 3 ml was taken and 200 μl of the sample and the standard were mixed at different concentration (20–300 μg/ml) followed by 15 min dark incubation at RT. The control was prepared exactly in the same way using 200 μl methanol instead of a sample. The DPPH radical scavenging activity of the extract was calculated by below equation.
% scavenging effect = (Absorbance of control–Absorbance of sample) × 100/Absorbance of control.
The 2,2′-azino-bis-3-ethylbenzotiazolin-6-sulfonic acid (ABTS) scavenging assay is based on radical cation decolorization method. In this method, reduction of ABTS·+ radicals depend on plant extracts antioxidant capacity (electron donating capacity) which leads to decolorization of the radical (Ben et al., 2017). Two solutions, ABTS (7 mM) and K2S2O8 (2.45 mM) was prepared in deionized water and were mixed in 1:1 ratio followed by dark incubation for 24–48 h. Afterward, dilution of ABTS solution was prepared in 1:25 ratio and 300 μl of plant extract was added to 3.0 ml of ABTS·+ solution. The absorbance of the homogenous solution was measured at λmax = 745 nm. The percentage inhibition of ABTS·+ was estimated through following formula:
Scavenging effect % = (Absorbance of control–Absorbance of sample) ×100/Absorbance of control.
The extract binding ability to iron (II) was calculated based on Khled et al. (2014) method. The formation of a red ferrous ion-ferrozine complex at λmax = 562 nm was used to monitor the ferrous ion. 2.0 ml iron chelators (20 & 40 μg/ml) were mixed with 0.4 ml FeSO4 (2 mM) and 0.2 mM ferrozine was added to initiate the reaction. The absorbance of sample was measured at λmax = 562 nm after 10 min of incubation at RT. Following formula was used to calculate the % inhibition.
% inhibition = (Absorbance of control–Absorbance of sample) ×100/Absorbance of control.
Polyphenol oxidase (PPO) assay was performed according to Sikora et al. (2019) protocol with minor changes. Briefly, One-gram of fresh leaves and flowers of L. camara was harvested. The extract was prepared in a 3 ml tube containing extraction buffer (0.1 M Na3PO4 buffer; pH 6.5). The whole mixture was centrifuged at 13,000 rpm for 18 min at 4°C and the supernatant was used an enzyme source. Catechol (0.2 ml of 0.01 M) was added to initiate the reaction, and the activity was represented as variance in absorbance at λmax = 495 nm at the interval of 30-s upto 3 min. Enzyme activity was expressed as unit/ml.
Fresh leaves and flowers (1.0 g each) were homogenized in 3 ml of 0.1 M Na3PO4 buffer (pH 7.0) with mortar and pestle. The whole mixture was centrifuged at 18,000 rpm at 5°C for 15 min and the experiments were performed subsequently (within 2–4 h). The supernatant was separated carefully and was used as an enzyme source. Finally, buffer solution (3.0 ml), guaiacol solution (0.05 ml), enzyme extract (0.1 ml), and H2O2 solution (0.03 ml) were mixed gently in the cuvette and absorbance was measured at λmax = 470 nm. The oxidase activity of the extract was calculated using following equation:
Where, AFsample indicates: Final absorption of the sample; AIsample: Initial absorption of the sample, AFblank: Final absorption of the control, AIblank: Initial absorption.
The antifungal property of the L. camara methanol extract was evaluated on PDA solid medium (19 ml) containing 25 mg/ml, (1 ml) of methanol extract in 60 mm Petri plates. The methanol was evaporated subsequently. Next, a small fragment of fungal mycelium (7 days old pure culture) was kept in the center of each Petri plate and were incubated at 23°C for 10 days. Subsequently, the radial mycelial growth was measured at 3rd, 6th, and 10th days after inoculation (DAI) (Singh and Tripathi, 1999).
To determine the antibacterial activity against Xanthomonas axonopodis pv. glycines and Xanthomonas oryzae pv. oryzae, 60 mm Petri plates were filled with peptone sucrose agar solid medium altered with 2,000 ppm methanolic LE and FE. The methanol was evaporated. Next, the 8 mm bacterial disc (24 h old) was placed in the center of each Petri plate and were incubated at 28°C. The bacterial radial growth was measured on 3, 9, and 15 DAI. All experiments were performed in triplicates. Lastly, the antibacterial activity of each extract was calculated in terms of the radial growth. Media altered with methanol and without alteration were served as control methanol and control, respectively.
The ability of extracts to protect DNA (Plasmid DNA pGEM-T) from the damaging effect of hydroxyl radicals generated by Fenton's reagent was evaluated by DNA nicking test with some modification (Wu and Dhanasekaran, 2020). The reaction mixture was prepared by adding 4.0 μl of plasmid DNA (100 ng/μl), 10 μl of Fenton's reagent (30 mM H2O2, 100 μl ascorbic acid and 200 μM FeCl3), and 5.0 μl of extracts (5 mg/ml) and final volume was made to 20 μl with double distilled water. Subsequently, incubation of reaction mixture was carried out at 37°C for 45 min, followed by addition of 2.5 μl loading buffer. The DNA fragmentation and protection activity were examined on 1% agarose gel through electrophoresis.
Dried sample of Lantana camara was subjected to FTIR (Fourier-transform infrared spectroscopy) to find out the functional groups present in extract using Thermo Nicolet iS5 instrument (Thermo Scientific, Madison, WT, United States). All reading was performed at room conditions. This machine is comprised of a deuterated triglycine sulfate (DTGS) and KBr which are used as a detector and beam splitter, respectively. This machine is connected to a software named OMNIC where output of FTIR spectra is recorded and analyzed. All spectra were taken in the range of 4,000–500 cm−1 at 4 cm−1 resolution and background air spectra were subtracted to optimize the actual spectra. Cleaning of ATR (attenuated total reflectance) crystal (sample spotting spot) was done regularly after each reading through 70% ethanol.
GC-MS of LE and FE was performed for compound identification following the method of Semwal and Painuli (2019) with minor modifications. GC-MS system (GCMS-QP2010, Shimadzu, Kyoto, Japan) was used which comprises an auto-injector (AOC-20i), the headspace sampler (AOC-20s) and a silica capillary column (Rtx-5). Initially, the oven temperature was set at 50°C, which was slowly raised to 280°C. One microliter of extract (1 mg/ml) (an equal mixture of FE and LEt) was injected into the column. Helium (purity 99.99%) gas was used as carrier gas (1.2 ml/min). Unique peak obtained was detected by MS detector. On the basis of RT (retention time) compounds were identified, and mass was measured in similar GC-MS conditions. Peak area was used for the quantitative determinations of compounds. Finally, components obtained spectral data of the peaks were compared with library data containing mass spectra (WILEY8 and NIST14). Complete running time of GC-MS was 33 min. The individual constituents' relative percentage was compared through peak area normalization.
The major 19 compounds obtained from GC-MS results were selected for docking analysis. These compounds were targeted against Peptide deformylase (PDF) (PDB ID-6IL0) of Xoo, Mitogen-Activated Protein Kinase (MAPK1) (PDB ID-5Z33) of M. oryzae, and sucrose hydrolase (SUH)-glucose complex (PDB ID 3CZG) of Xag. The 3D structure of PDF, MAPK1, and SUH were downloaded from Protein Data Bank (https://www.rcsb.org). Heteroatom removal and binding site detection of the protein was executed using Swiss-PdbViewer (v4.1) (Guex and Peitsch, 1997) and PLIP software (https://projects.biotec.tu-dresden.de/plip-web/plip), respectively. The 3D structure of each ligand was downloaded from PubChem (https://pubchem.ncbi.nlm.nih.gov/) in SDF format and proceed with PyRx (Virtual Screening Tool for energy minimization), PDBqt (Conversion and active site selection of protein). Virtual screening was conducted by rigid molecular docking into the active site of PDF, MAPK1, and SUH through AutoDock Vina (Trott and Olson, 2010). In the last, the binding energy table was extracted from the PyRx software. Compounds, which had lower binding energy were chosen for further analysis. Molecular interactions between protein-ligand complexes including bond lengths, participated amino acid, 2D, and 3D structure were analyzed and depicted using BIOVIA Discovery Studio 2016.
The results were stated as the mean ± standard deviation (SD) of three replicates. Significant variations achieved were evaluated by one-way analysis of variance (ANOVA) using Student's t-test. The statistical analysis and graph were prepared using Sigma Plot. A p ≤ 0.05 was treated as significant.
Methanol extracts from L. camara flower and leaf retains a percentage yield of 29.11 and 24.82%, respectively.
Qualitative phytochemical analysis revealed the existence of tannins, steroids, flavonoids, Phlobatannin, quinones, phenols, caumarins, alkaloids in both FE and LE. Terpenoids, saponins, and anthocyanin were found only in FE. Glycosides, cardiac glycoside, anthraquinones were found only in LE. Alkaloids, flavonoids, tannin, and coumarin were heavily present in LE while phenol was heavily present in both FE and LE (Table 1). Alkaloids are secondary nitrogenous compounds with known antioxidant potential, have been used in folk medicine (Quezada et al., 2006; Tiong et al., 2013). Predominantly terpenoid, has been used as a protective agent against oxidative stress-induced diseases (Grassmann, 2005; González-Burgos and Gómez-Serranillos, 2012). Saponin is used as a natural antioxidant and also stimulates apoptosis in tumor cells (Podolak et al., 2010; Tapondjou et al., 2011; Bi et al., 2012). Tannin antioxidant properties have been used as an active component in medicine and beverages (Amarowicz and Troszynska, 2003; Amarowicz et al., 2004). It works in two ways by donating hydrogen (Amarowicz, 2007) and chelating metal ion like Fe (II), Zn (II) Cu (II), and interferes with the Fenton reaction (Karamać, 2009). Similarly, glycosides such as Quercetin monoglycosides, diglycosides, and flavonol glycosides have been reported as strong lipid peroxidation inhibitor (Plumb et al., 1999). Plant-derived quinones have shown better antioxidant activity compared to synthetic antioxidants like BHA and impart superoxide scavenging activity (Inanami et al., 2001; Carvalho et al., 2005). The anti-tumor, antiparasitic and cytotoxic activities of quinones emanate due to redox cycling of their free radical (Hargreaves et al., 2000; Wardman, 2001). Anthraquinones, Coumarins, and Anthocyanin are endowed with many biological activities like antimicrobial, antioxidant, anti-inflammatory and anticancer (Chen et al., 2007; Garazd et al., 2007; Ifesan et al., 2009; He J. et al., 2011; He K. et al., 2011; Al-Amiery et al., 2012; Choi et al., 2013). Steroids are electron donors, which act upon free radicals and alter them to a more stable compound, thus culmination the terminal chain reaction (Yen and Chen, 1995). Cardiac glycosides have been practiced in heart failure treatment and cardiac arrhythmia by inhibiting Na+/K+ pump and increasing calcium ion concentration, which helps in contraction of the heart muscle (Vladimir and Ludmila, 2001).
Total alkaloid content in FE (2.9%) was higher than LE (1.8%) in terms of percentage. The TPC was expressed as mg GAE per gram extract. Plenty of phenolics were observed in FE amounting to 614.79 ± 1.25 mg GAE/g dw, while in LE GAE was found to be 563.57 ± 2.03 mg/g dw (Table 2). FE demonstrated a higher amount of flavonoid content (254.69 ± 0.88 mg/g) than LE (243.89 ± 1.30 mg/g). Thus, TPC and TFC of L. camara were higher in FE. Previous study suggests that leaves contain more amount of phenolics compared to flower extracts (Davies and Schwinn, 2006; Saboonchian et al., 2014). However, in current study higher concentration of phenolics were present in FE compared to LE. This might be related to the fact that flower pigment is composed of flavonoids and anthocyanins, which is synthesized from the phenylpropanoid pathway. The white clover flower contains more proanthocyanidins than vegetative tissues (Abeynayake et al., 2012). Interestingly, phenolics role in ultraviolet (UV) screening effect and flavonoids role in functional pollen development have been also reported (van der Meer et al., 1992; Cheynier et al., 2013) which further validates the abundance of polyphenols in flower tissues. TPC in FE predominantly proanthocyanidins entirely reinforced the more antioxidant capacity of FE compared to LE. In addition, the presence of hydroxyl groups in phenolic compound serves as a key to their scavenging ability. Also, the percentage of alkaloids was found to be higher in FE than LE. Hence, higher content of these phytochemicals in FE of L. camara leads to its greater radical scavenging activity.
Table 2. Phytochemical composition, antioxidant activity, free radical scavenging capacity, and enzymatic activity of L. camara LE and FE.
FTIR spectroscopy is an advanced technique used to identify pharmacologically active molecule in the natural products based on peak values of the FTIR spectrum. It performs a unique qualitative analysis and represents a chemical fingerprint, as there will be no two bioactive compounds with the same FTIR spectra (Easmin et al., 2017). FTIR analysis of L. camara LE and FE was performed to identify the functional group of the extract. Both FE and LE has shown the existence of different functional group. Several peaks were observed, which indicate the presence of different functional metabolites groups in both the extracts (Figure 1). It was interesting to note that both LE and FE have shown similar infra-red spectrum (IR) profile, possibly owing to presence of the polar nature of molecules in extracts. Some intense bands were also noted at various frequencies, which specify the presence of N–H, C–H, OH, C = O skeletal vibrations, which designate the occurrence of various metabolites such as amines, phenolics, alkanes, ester, and aldehydes in the extracts (Supplementary Table 1). However, only FTIR spectroscopy analysis is not sufficient enough to demonstrate the occurrence of various classes of compounds present in the extract (Gomathi et al., 2014; Bhat et al., 2018).
Figure 1. FTIR spectrum analysis of methanolic FE and LE of L. camara and their probable functional groups with wavenumbers. Both LE and FE have shown similar infra-red spectrum (IR) profile possibly due to polar nature of molecules presents in extracts. N–H, C–H, OH, C = O functional groups were observed which designate the presence of various metabolites in plant extracts.
GC-MS analysis of LE and FE mixture, component identification, and function prediction were performed according to Semwal and Painuli (2019). There were 65 peaks identified based on a separation of individual peaks, retention time, and percent area under the individual peak (Supplementary Table 2). Mass spectra of all identified compounds were matched with WILEY8.LIB and NIST08.LIB enlisted compounds. The function of only 19 components was predicted (Supplementary Table 3). The other functions could not be identified due to a lack of library data corresponding to compounds. Most of the components identified and reported here belong to different biological activities like antioxidants, anticancer, antifungal, antibacterial, antiviral, anti-inflammatory, etc. The major components were 3,7,11,15-Tetramethylhexadec-2-en-1-ol (14.72%), Methylhexopyranoside (13.83%), Linolenic acid (12.70%), 3-Deoxy-d-mannonic acid (7.97%), Palmitic acid (6.99%), Tiglic acid (6.47%), 2,3- Dihydrobenzofuran (6.25%), Tert-Butyl phenyl carbonate (4.18%), 2,6-Dimethoxyphenol (3.55%), and 9,12-Octadecadienoic acid (2.23%). Few components reported in this study have already been reported as responsible for many biological activities in other plants. Additionally, various other usage of these components are known in fragrance and perfume industry, flavor, and pesticides (Raman et al., 2012; Swamy and Sinniah, 2015; Semwal and Painuli, 2019).
TAA of the extracts was assessed by phosphomolebdenum method. Different degrees of activity exhibited by both extracts are shown in Table 2. Results indicated that FE has a significantly higher antioxidant capacity (1,376.41 ± 3.02 mg ascorbic acid equivalent/g dw) than LE (1,154.95 ± 1.43 mg AAE/g dw). In this study, total polyphenols, total flavonoid, and total antioxidant activity correlates, which could be one reason for overall antioxidant activity. Secondly, botanicals polyphenols act as both reducing agents as well as antioxidants by donating a hydrogen atom from their hydroxyl group. Therefore, it can be assumed that the polyphenols could be accountable for the extract antioxidant activity. The current result is in relation to previous research conducted by Mahdi-Pour et al. (2012), wherein the L. camara antioxidant potential was reported.
The effect of antioxidants on DPPH is because of its electron-donating capacity (Chen et al., 2020). Radical scavenging is very important in order to inhibit the harmful effect of free radicals. DPPH assay also indicates the presence of phenolic and flavonoid compounds in plant extracts (Aryal et al., 2019) and is a popular mechanism to study the antioxidant property of plant extract. Our result revealed significant antioxidant activities in both extracts and positive control and the values were noted higher with mounting concentration. DPPH activity values for FE>LE (Figure 2A). The FE and LE were able to reduce DPPH· radicals with a percentage of 94.31 ± 0.1 and 91.73 ± 1.01, respectively, at 300 μg/ml (highest concentration) with the IC50 values of 144.94 ± 1.36 and 172.03 ± 01.73 μg/ml, respectively (Figure 2D). However, the percentage inhibition of positive control (ascorbic acid) was higher than both LE and FE, with percentage inhibition of 97.27 % at 300 μg/ml and IC50 values of 74.16 ± 0.23 μg/ml. The presence of phenol in plants is necessary for free radical scavenging. Plant extracts having antioxidants with DPPH free radical scavenging property could donate hydrogen to lipid peroxidase or hydroperoxidase free radicals that are key propagators of the autoxidation of lipid chain. It also produces non-radicals that disrupt lipid peroxidation chain reaction (Sowndhararajan and Kang, 2013).
Figure 2. Antioxidant activity of L. camara LE and FE. (A) DPPH radical scavenging activities (B) ABTS radical scavenging activity leaf and flower extract at different concentrations. (C) Ferric reducing capacity. (D) Radical scavenging activity of L. camara extract in term of IC50. Values represented as mean ± SD (n = 3, P < 0.05).
Alternatively, the antioxidant activity of polyphenols is also evaluated by its reducing power competence. Existence of reductants in extracts leads to terminates free radicals chain reaction by donating 1H and hence, extract reduce Fe3+ to Fe2+ (Bhandari and Kawabata, 2004). The formation of Perl's Prussian blue color from yellow color of extract solution indicates the reducing activity of extract which is monitored by measuring the activity at λmax = 700 nm (Oktay et al., 2003). Thus, absorbance is directly proportional to reducing capability of the extract. Previous study endorses that flavonoids and phenolic acids (polyphenols) antioxidant activity was measured through the its reducing capacity of Fe3+-Fe2+ (Zhao et al., 2006). In current study, both extracts (FE & LE), have exhibited electron-donating activity by reducing Fe3+ to Fe2+ in a concentration-dependent manner (Figure 2C). FRAP activity of LE > FE in increasing concentration order (60 to 220 μg/ml), which suggest that LE has more potential to react with free radicals and terminate them into non-reactive stable form. Here, we infer that the presence of polyphenols in the extract has played the role of free radical scavenger by donation electron/hydrogen and thus imparted the extract reducing power activity.
The reaction between ABTS and K2S2O8 generate ABTS cations scavenging activity, which indicate the peculiar 1H/e− donating or reducing competences of botanicals. FE showed an inhibition of 71.47 ± 0.80% at the highest concentration of 260 μg/ml, whereas, LE was found to be least effective radical scavenger with 17.81 ± 0.39 at 260 μg/ml (Figure 2B). Prominent difference in the IC50 value of flower (214.9 ± 2.28 μg/ml) and leaf (784.83 ± 8.85 μg/ml) again indicating the effectiveness of FE as a potent inhibitor of free radical. The higher scavenging power of FE correlate with the higher amounts of flavonoids, polyphenols, and proanthocyanin. This further proved the L. camara extract potential to scavenge the free radicals, therefore, FE could be a better choice in preventing chain reaction in lipid peroxidation and could also serve as potential nutraceuticals.
FE of L. camara had a high chelating activity of 28.12 ± 2.38 than LE (16.57 ± 2.05) at a concentration of 40 μg/ml. Although, standard Na2EDTA had chelating activity (49.64 ± 1.88) higher than both FE and LE at a concentration of 40 μg/ml as shown in Table 2. Iron chelators like caffeic acid, 2,3-dihydroxybenzoic acid, and desferroxamine have shown both antioxidant and ROS scavenging activities. Chelation therapy is used to neutralize iron overload (Thalassemia and Anemia) and in controlling oxidative damage in neurodegenerative ailments using synthetic chelators, which show adverse effects as well (Ebrahimzadeh et al., 2008). Therefore, the chelation of metal ion by plant-derived molecules could be a safer therapeutic agent. The iron binding ability of chelators correspond to the functional group used in iron chelators (Adjimani and Asare, 2015).
Like the natural antioxidants and vitamins, also enzymes could neutralize the excess ROS (Vertuani et al., 2004). Total activity value found for peroxidase (POD), and polyphenol oxidase (PPO) of FE was 60 and 53.33 (U/ml/min), respectively, which was much higher than LE (36.00 and 40.33 U/ml/min) (Table 2). Free radicals induced oxidative stress in human is countered by enzymatic defense mechanisms such as superoxide dismutase (SOD), catalase (CAT), and glutathione peroxidase (GPx). Similarly, in plants, both non-enzymatic, and enzymatic antioxidants like CAT, SOD, POD scavenge excessive ROS. POD and PPO also provide resistance to plants against biotic and abiotic stresses (Nabity et al., 2006; He J. et al., 2011; He K. et al., 2011). Thus, we can say that FE and LE both had good enzyme activity and can be used in antimicrobial activity and also as a source of natural enzymatic antioxidants.
Free radicals could mediate DNA damage, which ultimately reduces carcinogenesis, mutagenesis, and cytotoxicity of ROS (Gul et al., 2011; Poorna et al., 2013). In this study, both FE and LE have shown significant antioxidant activity. Hence, both the extracts were examined for their protective ability against oxidative damage to plasmid DNA (pGEM-T) caused by Fenton's reagent (Figure 3). Fenton's reagent gives rise to Hydroxyl radicals, is widely known to damage DNA by introducing nicks in the strands, which may result in the slower movement of nicked DNA in agarose gel electrophoresis. The addition of Fenton's reagent alone generated nicks in the plasmid DNA, resulted in the formation of relaxed/linear DNA from the supercoiled form of plasmid DNA. However, the addition of LE and FE along with Fenton's reagent showed the presence of supercoiled plasmid DNA similar to native pGEM-T plasmid suggesting the reduced effect of Fenton's reagent-mediated DNA damage. Our result convincingly showed that both LE and FE moderated the oxidative stress and shielded the DNA from the damaging effect of hydroxyl radicals produced by Fenton's reagent (Figures 3A,B). The protective effect of both LE and FE against hydroxyl radical mediated DNA damage could have been attributed by various phenolic compounds occurrence. Similar protective effect on DNA were observed in various plant extracts (Paramaguru et al., 2013; Salar et al., 2017).
Figure 3. DNA damage protecting activity of L. camara FE and LE against hydroxyl radical induced DNA damage of pGEM-T plasmid. Lane 1: Ladder 1kb; Lane 2: pGEM-T plasmid DNA; Lane 3: DNA + Fenton's reagent; Lane 4: DNA + Fenton's reagent + extract (5 mg/ml). (A) FE and (B) LE.
Results indicate that both LE and FE have positive effects against fungi (M. oryzae) and bacteria (Xag and Xoo). In-vitro results of M. oryzae growth on PDA amended with LE showed antifungal properties at 25mg/ml concentrations. Mycelial growth of 0.56 ± 0.05 cm and 1.16 ± 0.15 cm in LE and FE was recorded at 3rd DAI, which was lower than control (1.30 ± 00 cm) and control methanol (1.20 ± 0.05 cm). Similarly, 10th DAI lower fungus growth in LE and FE (3.70 ± 0.10 cm & 4.00 ± 0.10 cm) was observed than control (6.9 ± 0.11 cm) and control methanol (6.56 ± 0.05 cm) (Figure 4). In terms of % inhibition, 39.02 to 46.60 % was observed on 10th DAI in FE and LE, respectively. Such results indicate that these plant extracts may have fungicidal or fungistatic properties that can be used for controlling M. oryzae by seed treatment, the causal agent of rice blast. Similarly, the antifungal properties of methanolic LE of L. camara on Aspergillus fumigatus and Aspergillus flavus have been reported previously (Naz and Bano, 2013). The greater antimicrobial activity of extracts could also link to peroxidases and the presence of various phytochemicals. It has been proved that dimerization of phenols by peroxidase oxidative activity results in toxic product for the pathogen, which might be in the case of M. oryzae (Martinez et al., 2000).
Figure 4. Antimicrobial activities of L. camara FE and LE. (A) Antifungal activity of FE and LE against M. oryzae after 3, 6, and 10 days of inoculation. (B) Antibacterial activity of FE and LE against Xoo and Xag of Xanthomonas after 3, 9, and 15 days of inoculation. Values represented as mean ± SD (n = 3, P < 0.05).
Both LE and FE inhibited the growth of Xoo and Xag in comparison to the control and control methanol. FE was highly effective as no growth of bacteria was observed on the 3rd DAI, while bacterial growth on 15th day was higher in FE than LE for both strains (Figure 4). In terms of % inhibition, 30.06–45.45% was observed in the case of Xoo in FE and LE, whereas 11.42–34.28% was observed in FE and LE on 15th DAI in case of Xag. Variation in inhibition of the bacterial growth pattern was observed on 9th DAI, wherein higher bacterial growth was observed in LE than FE against both strains (Xoo and Xag). This could be due to the secretion of other constituent exerting an antagonistic effect on the antibacterial bioactive compound. The difference in the activity of LE and FE may be due to differences in phytochemical constituents of the extracts (Vivek et al., 2013). Phytochemical constituents such as alkaloids, flavonoids, tannin, and coumarin were heavily present in LE, which could have possibly attributed the higher antimicrobial activities. This result partly indicates that since both extracts have inhibitory activities against phytopathogens, therefore could be a promising source of new antimicrobial agents and reaffirms the ethnomedicinal properties of L. camara.
Out of 19 major compounds tested, only four compounds showed strong binding affinity with both Peptide Deformylase (PDF) and Mitogen-Activated Protein Kinase 1 (MAPK 1). The binding affinity of Eicosapentaenoic acid was −6.9 Kcal/mol with PDF and −6.3 Kcal/mol with MAPK1 (Supplementary Table 3). For sucrose hydrolase (SUH)-glucose complex, Loliolide showed highest binding affinity (−7.3 Kcal/mol). Similarly, the binding affinity of Phytol and Salicylic acid methyl ester was −6.3 and −6.2 Kcal/mol with PDF. To understand the affinity between ligands and docked protein, the structure was further visualized in Discovery Studio (Figure 5). Eicosapentaenoic acid was able to form two hydrogen bonds with Gly104 and Leu105 of peptide PDF, and Lys52 and Arg66 of MAPK1(dashed green line). Salicylic acid methyl ester formed two hydrogen bonds with Gly46 and Gly98 of PDF. While, Loliolide have found to form three hydrogen bonds (His180, Gln248, and Ser281) with sucrose hydrolase (SUH)-glucose complex. Structure was further supported by some alkyl (dashed pink arrow), pi-alkyl (dotted dark yellow arrow), pi-sigma (dotted blue line), pi-pi stacked (dashed yellow line), and pi-anion (dashed red line). Results suggested the strong binding affinity of ligand with target protein particularly Eicosapentaenoic acid and Loliolide, which could have potential to disrupt the activity of these target protein. PDF catalyze the removal of N-formyl group from N-terminal methionine during translation, which make it an good target to formulate antibiotics (Guay, 2007; Fieulaine et al., 2016). Previous studies have shown bacterial growth inhibition by targeting PDF (Chen et al., 2004; Goemaere et al., 2005). MAPK1 catalyzes phosphorylation of proteins substrates on serine or threonine residues, regulates cell divisions and signaling pathways (Liu and Winey, 2012; Xu et al., 2017). Similarly, SUH is essential for sucrose metabolism and growth (Kim et al., 2004). Hence, our study suggests that these enzymes (MAPK1, PDF, and SUH) could be targeted using phytochemicals (Loliolide, Eicosapentaenoic acid, Salicylic Acid Methyl Ester, and Phytol) of Lantana species to inhibit the selected phytopathogens.
Figure 5. Left panel showing 3D image of protein and ligand interaction while right panel is 2D interaction. (A) Molecular docking interaction of Eicosapentaenoic acid with PDF Xoo. (B) Molecular docking interaction of Phytol with peptide deformylase protein of Xoo. (C) Molecular docking interaction of Salicylic acid methyl ester with PDF of Xoo. (D) Molecular docking interaction of Eicosapentaenoic acid with MAPK1 of M. oryzae. (E) Molecular docking interaction of Loliolide with sucrose hydrolase (SUH)-glucose complex of Xag.
The present study demonstrated that methanolic extracts of both leaf and flower of L. camara have considerable antioxidant and antimicrobial activity. Many phytochemical components tested were present in varying concentrations in both the extracts corresponding to differences in their antioxidant and free radical scavenging activities. A positive correlation between the phenolic contents and free radical scavenging activities were noted. In vitro assays indicated that the FE could act as direct antioxidants through free radical scavenging, or as indirect antioxidants through the induction of enzymes system accountable for antioxidant activity. The FE of L. camara also exhibited DNA damage inhibitory effects further suggesting its free radical scavenging ability which could be used to prevent free radical mediated disease progression. In vitro antimicrobial assays showed the ability of both FE and LE of L. camara in suppressing the growth of phytopathogens like Magnaporthe oryzae, Xanthomonas axonopodis pv. glycines (Xag), and Xanthomonas oryzae pv. oryzae (Xoo). GC-MS analysis of extracts detected various bioactive compounds that could contribute to antimicrobial activity. Additionally, molecular docking study also revealed that phytochemicals like Loliolide, Eicosapentaenoic acid, Salicylic Acid Methyl Ester and Phytol of Lantana camara coud be used to target microbial enzymes like MAPK1, PDF and SUH to controls phytopathogens. However, more investigation is required to isolate and identify the reliable antioxidant and antimicrobial molecules present in the crude extract.
All datasets generated for this study are included in the article/Supplementary Material.
AK, NA, TT, and SND designed the article. AM, NS, and SKD performed the work and prepare the rough draft of the article. AK, NA, TT, and SND corrected the article and finally all authors read and approved the final article.
AK sincerely aknowledge financial supports from MPCST (Endt. No. 3879/CST/R&D/ BioSci/2018) and start-up grant from the University Grants Commission (No. F.30-392/2017) (BSR). AM thanks DST INSPIRE (IF 180542) for the fellowship. SND thanks University Grants Commission (UGC) for a start-up grant.
The authors declare that the research was conducted in the absence of any commercial or financial relationships that could be construed as a potential conflict of interest.
Authors thanks Mr. Rajesh Ranjan, BHEL Noida, for assisting with English language corrections.
The Supplementary Material for this article can be found online at: https://www.frontiersin.org/articles/10.3389/fagro.2020.582268/full#supplementary-material
Supplementary Figure 1. Representative picture of flowers (A) and leaves (B) of Lantana camara L. collected from Amarkantak regions of MP, India.
Supplementary Figure 2. Antimicrobial activities of L. camara FE and LE (A) Antibacterial activity of FE and LE against Xoo and Xag 3, 9, and 15 DAI (B) Antifungal activity of FE and LE against M. oryzae after 3, 6, and 10 DAI.
Supplementary Table 1. Phytochemical constituents of flower and leaf extracts of L. camara identified in FTIR.
Supplementary Table 2. Chemical composition of methanolic extract of L. camara leaf and flower crude extract mixture (1:1).
Supplementary Table 3. Protein and ligand interactions study of major bioactive compounds obtained from GC-MS study.
AAE, Ascorbic acid equivalent; ABTS, 2,2′-azino-bis-(3-ethylbenzothiazoline-6-sulfonate); BHT, Butylated Hydroxy Toluene; DW, Dry weight; DPPH, 2,2-diphenyl-1-picrylhydrazyl; DAI, Days after inoculation; GAE, Gallic acid equivalent; GC-MS, Gas chromatography-mass spectrometry; FTIR, Fourier-transform infrared spectroscopy; FRAP, Ferric ion reducing antioxidant potential; ROS, Reactive oxygen species.
Abeynayake, S. W., Panter, S., Chapman, R., Webster, T., Rochfort, S., Mouradov, A., et al. (2012). Biosynthesis of proanthocyanidins in white clover flowers: cross talk within the flavonoid pathway. Plant Physiol. 158, 666–678. doi: 10.1104/pp.111.189258
Adjimani, J. P., and Asare, P. (2015). Antioxidant and free radical scavenging activity of iron chelators. Toxicol. Rep. 2, 721–728. doi: 10.1016/j.toxrep.2015.04.005
Al-Amiery, A. A., Al-Bayati, R., Saour, K., and Radi, M. (2012). Cytotoxicity, Antioxidant and Antimicrobial activities of novel 2-quinolone derivatives derived from coumarins. Res. Chem. Intermediat. 38, 559–569. doi: 10.1007/s11164-011-0371-2
Amarowicz, A., and Troszynska, A. (2003). Antioxidant and antiradical activity of extract of pea and its fractions of low molecular phenolics and tannins. Pol. J. Food Nutr. Sci. 12, 10–15.
Amarowicz, R. (2007). Tannins: the new natural antioxidants? Eur. J. Lipid Sci. Technol. 109, 549–551. doi: 10.1002/ejlt.200700145
Amarowicz, R., Toszynska, A., and Shahidi, F. (2004). Antioxidant activity of extract of almond seeds and its fractions. J. Food Lipids 12, 344-358. doi: 10.1111/j.1745-4522.2005.00029.x
Aryal, S., Baniya, M. K., Danekhu, K., Kunwar, P., Gurung, R., and Koirala, N. (2019). Total phenolic content, flavonoid content and antioxidant potential of wild vegetables from Western Nepal. Plants 8, 96–107. doi: 10.3390/plants8040096
Ben, N. A., Znati, M., Nguir, A., Daich, A., Othman, M., Lawson, A. M., et al. (2017). Phytochemical and biological studies of Tripplex inflata f. Muell isolation of secondary bioactive metabolites. J. Pharm. Pharmacol. 69, 1064–1074. doi: 10.1111/jphp.12735
Bendiabdellah, A., El Amine Dib, M., Djabou, N., Allali, H., Tabti, B., Muselli, A., et al. (2012). Biological activities and volatile constituents of Daucus muricatus L. from Algeria. Chem. Cent. J. 6, 48–58. doi: 10.1186/1752-153X-6-48
Bhandari, M. R., and Kawabata, M. (2004). Organic acid, phenolic content and antioxidant activity of wild yam (Dioscorea spp.) tubers of Nepal. Food Chem. 88, 163–168. doi: 10.1016/j.foodchem.2003.12.027
Bhat, M. Y., Gul, M. Z., Lohamror, L. R., Qureshi, I. A., and Ghazi, I. A. (2018). An in vitro study of the antioxidant and antiproliferative properties of artemisia absinthium—a potent medicinal plant. Free Radic. Antioxid. 8, 18–25. doi: 10.5530/fra.2018.1.4
Bi, L., Tian, X., Dou, F., Hong, L., Tang, H., and Wang, S. (2012). New antioxidant and antiglycation active triterpenoid saponins from the root bark of aralia taibaiensis. Fitoterapia 83, 234–240. doi: 10.1016/j.fitote.2011.11.002
Bursal, E., and Gulcin, I. (2011). Polyphenol contents and in vitro antioxidant activities of lyophilized aqueous extract of kiwifruit (Actinidia deliciosa). Food Res. Int. 44,1482–1489. doi: 10.1016/j.foodres.2011.03.031
Campos, E. V., Proença, P. L., Oliveira, J. L., Bakshi, M., Abhilash, P. C., and Fraceto, L. F. (2019). Use of botanical insecticides for sustainable agriculture: future perspectives. Ecol. Indica 105, 483–495. doi: 10.1016/j.ecolind.2018.04.038
Carvalho, R. N., Moura, L. S., Rosa, P. T. V., and Meireles, M. A. A. (2005). Supercritical fluid extraction from rosemary (Rosmarinus officinalis): kinetic data, extract's global yield, composition, and antioxidant activity. J. Supercrit. Fluids 35, 197–204. doi: 10.1016/j.supflu.2005.01.009
Chang, C. C., Yang, M. H., Wen, H. M., and Chem, J. C. (2002). Estimation of total flavonoid content in propolis by two complementary colorimetric methods. J. Food Drug Anal. 10, 178–182.
Chen, D., Hackbarth, C., Ni, Z. J., Wu, C., Wang, W., and Jain, R. (2004). peptide deformylase inhibitors as antibacterial agents: identification of VRC3375, a proline-3-alkylsuccinyl hydroxamate derivative, by using an integrated combinatorial and medicinal chemistry approach. Antimicrob. Agents Chemother. 48, 250–261. doi: 10.1128/AAC.48.1.250-261.2004
Chen, J., Yang, J., Ma, L., Li, J., Shahzad, N., and Kim, C. K. (2020). Structure antioxidant activity relationship of methoxy, phenolic hydroxyl, and carboxylic acid groups of phenolic acids. Sci. Rep. 10:2611. doi: 10.1038/s41598-020-59451-z
Chen, S. H., Lin, K. Y., Chang, C. C., Fang, C. L., and Lin, C. P. (2007). Aloe-emodin-induced apoptosis in human gastric carcinoma cells. Food Chem. Toxicol. 45, 2296–2303. doi: 10.1016/j.fct.2007.06.005
Cheynier, V., Comte, G., Davies, K. M., Lattanzio, V., and Martens, S. (2013). Plant phenolics: recent advances on their biosynthesis, genetics, and ecophysiology. Plant Physiol. Biochem. 72, 1–20. doi: 10.1016/j.plaphy.2013.05.009
Choi, R. J., Ngoc, T. M., Bae, K., Cho, H. J., Kim, D. D., Chun, J., et al. (2013). Anti-inflammatory properties of anthraquinones and their relationship with the regulation of P-glycoprotein function and expression. Eur. J. Pharm. Sci. 48, 272–281. doi: 10.1016/j.ejps.2012.10.027
Chu, Y. H., Chang, C. L., and Hsu, H. F. (2000). Flavonoid content of several vegetables and their antioxidant activity. J. Sci. Food Agric. 80, 561–566. doi: 10.1002/(SICI)1097-0010(200004)80:5<561::AID-JSFA574>3.3.CO
Davies, K. M., and Schwinn, K. E. (2006). “Molecular biology and biotechnology of flavonoids,” in Flavonoids Chemistry, Biochemistry and Applications, eds O. M. Andersen and K. R. Markham (Boca Raton, FL: CRC Press, Tylor & Francis Group), 143–218.
Easmin, S., Sarker, M. Z. I., Ghafoor, K., Ferdosh, S., Jaffri, J., Ali, M. E., et al. (2017). Rapid investigation of α-glucosidase inhibitory activity of phaleriamacrocarpa extracts using ftir-atr based fingerprinting. J. Food Drug Anal. 25, 306–315. doi: 10.1016/j.jfda.2016.09.007
Ebrahimzadeh, M. A., Pourmorad, F., and Bekhradnia, A. R. (2008). Iron chelating activity, phenol and flavonoid content of some medicinal plants from Iran. Afr. J. Biotechnol. 7, 3188–3192.
Ekor, M. (2014). The growing use of herbal medicines: issues relating to adverse reactions and challenges in monitoring safety. Front Pharmacol. 4:177. doi: 10.3389/fphar.2013.00177
Fayaz, M., Bhat, M. H., Fayaz, M., Kumar, A., and Jain, A. K. (2017). Antifungal activity of lantana camara L. Leaf extracts in different solvents against some pathogenic fungal strains. Pharmacologia. 8, 105–112. doi: 10.5567/pharmacologia.2017.105.112
Fieulaine, S., Alves de Sousa, R., Maigre, L., Hamiche, K., Alimi, M., and Bolla, J. M. (2016). A unique peptide deformylase platform to rationally design and challenge novel active compounds. Sci. Rep. 6:35429. doi: 10.1038/srep35429
Garazd, M. M, Muzychka, O. V., Voyk, A. I., Nagorichna, I. V., and Ogorodniichuk, A. S. (2007). Modified coumarins: Synthesis and antioxidant activity of 3-substituted 5,7-dihydroxy4-methyl coumarins. Chem. Nat. Compd. 43:19–23. doi: 10.1007/s10600-007-0055-8
Ghisalberti, E. L. (2000). Lantana camara L. (Verbenaceae). Fitoterapia 71, 467–486. doi: 10.1016/s0367-326x(00)00202-1
Goemaere, E., Melet, A., Larue, V. R., Lieutaud, A. L., Alves de Sousa, R., Chevalier, J., et al. (2005). New peptide deformylase inhibitors and cooperative interaction: a combination to improve antibacterial activity. J. Antimicrob. Chemother. 67, 1392–1400. doi: 10.1093/jac/dks058
Gomathi, D., Ravikumar, G., Kalaiselvi, M., Devaki, K., and Uma, C. (2014). Antioxidant activity and functional group analysis of evolvulus alsinoides. Chin. J. Nat. Med. 12, 827–832. doi: 10.1016/S1875-5364(14)60124-2
González-Burgos, E., and Gómez-Serranillos, M. P. (2012). Terpene compounds in nature: a review of their potential antioxidant activity. Curr. Med. Chem. 19, 5319–5341. doi: 10.2174/092986712803833335
Govindarajan, R., Rastogi, S., Vijayakumar, M., Rawat, A. K. S., Shirwaikar, A., Mehrotra, S., et al. (2003). Studies on the antioxidant activities of Desmodium gangeticum. Biol. Pharm. Bull. 26, 1424–1427. doi: 10.1248/bpb.26.1424
Grassmann, J. (2005). Terpenoids as plant antioxidants. Vitam Horm. 72, 505–535. doi: 10.1016/S0083-6729(05)72015-X
Guay, D. R. (2007). Drug forecast–the peptide deformylase inhibitors as antibacterial agents. Ther. Clin. Risk Manag. 3, 513-525.
Guex, N., and Peitsch, M. C. (1997). SWISS-MODEL and the Swiss-PdbViewer: an environment for comparative protein modeling. Electrophoresis 18, 2714–2723. doi: 10.1002/elps.1150181505
Gul, M., Bhakshu, L., Ahmed, F., Anand, K., Qureshi, I., and Ghazi, I.A. (2011). Evaluation of Abelmoschus moschatus extracts for antioxidant, free radical scavenging, antimicrobial and antiproliferative activities using in vitro assays. BMC Complement. Altern. Med. 11:64. doi: 10.1186/1472-6882-11-64
Harborne, J. B. (1973). Phytochemical Methods: A Guide to Modern Techniques of Plant Analysis, 2nd Edn. London; New York, NY: Chapman and Hall Ltd.
Harborne, J. B. (1998). Textbook of Phytochemical Methods. A Guide to Modern Techniques of Plant Analysis, 5th Edn. London; New York, NY: Chapman and Hall Ltd.
Hargreaves, R. H., Hartley, J. A., and Butler, J. (2000). Mechanisms of action of quinone containing alkylating agents: DNA alkylation by aziridinyl quinones. Front. Biosci. 5, 172–180. doi: 10.2741/hargreav
Hassine, M., Zardi-Berguaoui, A., Znati, M., Flamini, G., Jannet, H. B., and Hamza, M. A. (2014). Chemical composition, antibacterial and cytotoxic activities of the essential oil from the flowers of Tunisian Convolvulus althaeoides L. Nat. Prod. Res. 28, 769–775. doi: 10.1080/14786419.2013.879476
He, J., Chen, F., Chen, S., Lv, G., Deng, Y., Fang, W., et al. (2011). Chrysanthemum leaf epidermal surface morphology and antioxidant and defense enzyme activity in response to aphid infestation. J. Plant Physiol. 168, 687–693. doi: 10.1016/j.jplph.2010.10.009
He, K., Li, X. G., Chen, X., Ye, X. L., Huang, J., Jin, Y. N., et al. (2011). Evaluation of antidiabetic potential of selected traditional Chinese medicines in STZ-induced diabetic mice. J. Ethnopharmacol. 137, 1135–1142. doi: 10.1016/j.jep.2011.07.033
Hernández, T., Canales, M., Avila, J. G., Duran, A., Caballero, J, Romo de Vivar, A., et al. (2003). Ethnobotany and antibacterial activity of some plants used in tradicional medicine of zapotitlán de las Salinas, Puebla (México). J. Ethnopharmacol. 88, 181–188. doi: 10.1016/S0378-8741(03)00213-7
Ifesan, B. O., Hamtasin, C., Mahabusarakam, W., and Voravuthikunchai, S. P. (2009). Assessment of antistaphylococcal activity of partially purified fractions and pure compounds from eleutherine Americana. J. Food Protect. 72, 354–359. doi: 10.4315/0362-028X-72.2.354
Inanami, O., Yamamori, T., Shionoya, H., and Kuwabara, M. (2001). “Antioxidant activity of quinone-derivatives from freeze-dried powder of the ascidians,” in The Biology of Ascidians, eds H. Sawada, H. Yokosawa, and C. C. Lambert (Tokyo: Springer).
Kalita, S., Kumar, G., Karthik, L., and Rao, K.V.B. (2012). A review on medicinal properties of lantana camara linn. Res. J. Pharm. Tech. 5, 711–715.
Karamać, M. (2009). Chelation of Cu(II), Zn(II), and Fe(II) by tannin constituents of selected edible nuts. Int. J. Mol. Sci. 10, 5485–5497. doi: 10.3390/ijms10125485
Khled, K. N., Boulekbache-Makhlouf, L., and Madani, K. (2014). Antioxidant capacity of crude extracts and their solvent fractions of selected Algerian Lamiaceae. Ind. Crops Prod. 52, 177–182. doi: 10.1016/j.indcrop.2013.10.004
Kim, H.-S., Park, H.-J., Heu, S., and Jung, J. (2004). Molecular and functional characterization of a unique sucrose hydrolase from xanthomonas axonopodis pv. glycines. J. Bacteriol. 186, 411–418. doi: 10.1128/JB.186.2.411-418.2004
Kumar, A., John, M. M., Gul, M. Z., Bimolata, W., and Ghazi, I. A. (2011). “Differential responses of non-enzymatic antioxidative system underwater deficit condition in rice (Oryza sativaL.),” in International Proceedings of Chemical Biological and Environmental Engineering (Singapore: IACSIT Press).
Kumar, A., Kumar, R., Sengupta, D., Das, S. N., Pandey, M. K., Bohra, A., et al. (2020). Deployment of genetic and genomic tools toward gaining a better understanding of rice-Xanthomonas oryzae pv. oryzae interactions for development of durable bacterial blight resistant rice. Front. Plant Sci. 11:1152. doi: 10.3389/fpls.2020.01152
Kumar, A., Gul, M. Z., Zeeshan, A., Bimolata, W, Qureshi, I. A., and Ghazi, I.A. (2013). Differential antioxidative responses of three different rice genotypes during bacterial blight infection. Aust. J. Crop. Sci. 7, 1893–1900.
Liu, X., and Winey, M. (2012). The MPS1 family of protein kinases. Annu. Rev. Biochem 81, 561–585. doi: 10.1146/annurev-biochem-061611-090435
Mahdi-Pour, B., Jothy, S. L., Latha, L. Y., Chen, Y., and Sasidharan, S. (2012). Antioxidant activity of methanol extracts of different parts of Lantana camara. Asian Pac. J. Trop Biomed. 2, 960–965. doi: 10.1016/S2221-1691(13)60007-6
Martinez, C., Baccou, J. C., Bresson, E., Baissac, Y., Daniel, F., Jalloul, A., et al. (2000). Salicylic acid mediated by the oxidative burst is a key molecule in local and systemic responses of cotton challenged by an avirulent race of Xanthomonas campestris pv. malvacearum. Plant Physiol. 122, 757–766. doi: 10.1104/pp.122.3.757
Nabity, P., Heng-Moss, T., and Higley, L. G. (2006). Effects of insect herbivory on physiological and biochemical (oxidative enzyme) responses of the halophyte Atriplex subspicata (Chenopodiaceae). Environ. Entomol. 35, 1677–1689. doi: 10.1093/ee/35.6.1677
Naz, R., and Bano, A. (2013). Phytochemical screening, antioxidants and antimicrobial potential of Lantana camara in different solvents. Asian Pac. J. Trop. Dis. 3, 480–486. doi: 10.1016/S2222-1808(13)60104-8
Oktay, M., Gülçin, I., and Küfrevioglu, O. I. (2003). Determination of in vitro antioxidant activity of fennel (Foeniculum vulgare) seed extracts. LWT Food Sci. Technol. 36, 263–271. doi: 10.1016/S0023-6438(02)00226-8
Paramaguru, R., Mazumder, P. M., Sasmal, D., Kumar, D., and Mukhopadhyay, K. (2013). Evaluation of antioxidant and DNA nicking potential along with HPTLC fingerprint analysis of different parts of Pterospermum acerifolium (L.) Wild. Free Radic. Antioxid. 3, 100–106. doi: 10.1016/j.fra.2013.05.002
Pisoschi, A. M., Cheregi, M. C., and Danet, A. F. (2009). Total antioxidant capacity of some commercial fruit juices: electrochemical and spectrophotometrical approaches. Molecules 14, 480–493. doi: 10.3390/molecules14010480
Plumb, G. W., Price, K. R., and Williamson, G. (1999). Antioxidant properties of flavonol glycosides from tea. Redox Rep. 4, 13–16. doi: 10.1179/135100099101534684
Podolak, I., Galanty, A., and Sobolewska, D. (2010). Saponins as cytotoxic agents: a review. Phytochem. Rev. 9, 425–474. doi: 10.1007/s11101-010-9183-z
Poorna, C. A., Resmi, M. S., and Soniya, E. V. (2013). In vitro antioxidant analysis and the DNA damage protective activity of Leaf extract of the Excoecariaagallocha linn Mangrove plant, eds M. Stoytcheva and R. Zlatev (New York, NY: Agricultural Chemistry, InTech), 155–166.
Prasad, K. N., Chew, L. Y., Khoo, H. E., Kong, K. W., Azlan, A., and Ismail, A. (2010). Antioxidant capacities of peel, pulp, and seed fractions of canariumodontophyllum miq fruit. J. Biomed. Biotech. 2010:871379. doi: 10.1155/2010/871379
Quezada, N. M., Asencio, J. M., Valle, D., Aguilera, J. M., and Gómez, B. (2006). Antioxidant activity of crude extract, alkaloid fraction and flavonoid fraction from boldo (Peumusboldus molina) leaves. J. Food Sci. 69, 371–376. doi: 10.1111/j.1365-2621.2004.tb10700.x
Raman, V. B., Samuel, L.A., PardhaSaradhi, M., and Narashimha, R. B. (2012). Antibacterial, antioxidant activity and GC-MS analysis of Eupatorium odoratum. Asian J. Pharm. Clin. Res. 5, 99–106.
Roghini, R., and Vijayalakshmi, K. (2018). Phytochemical screening, quantitative analysis of flavonoids and minerals in ethanolic extract of Citrus paradise. Int. J. Pharm. Sci. Res. 9, 4859–4864. doi: 10.19080/JDVS.2019.09.555768
Saboonchian, F., Jamedi, R., and Sarghein, S. H. (2014). Phenolic and flavonoid content of Elaeagnus angustifolia L. (leaf and flower). Avic. J. Phytomed. 4, 231–238. doi: 10.22038/AJP.2014.1975
Salar, R. K., Purewal, S. S., and Sandhu, K. S. (2017). Relationships between DNA damage protection activity, total phenolic content, condensed tannin content and antioxidant potential among Indian barley cultivars. Biocatal. Agric. Biotechnol. 11, 201–206. doi: 10.1016/j.bcab.2017.07.006
Semwal, P., and Painuli, S. (2019). Antioxidant, antimicrobial, and GC MS profiling of Saussureaobvallata (Brahma Kamal) from Uttarakhand Himalaya. Clin. Phytosci. 5:12. doi: 10.1186/s40816-019-0105-3
Sharma, O. P., Jagdish, V., and Sharma, P. D. (1991). Comparison of lantadenes content and toxicity of different variety of the lantana plant. J. Chem. Ecol. 17, 2283–2291. doi: 10.1007/BF00988008
Sikora, M., Swieca, M., Franczyk, M., Jakubczyk, A., Bochnak, J., and Zlotek, U. (2019). Biochemical properties of polyphenol oxidases from ready-to-eat lentil (Lens culinaris medik.) sprouts and factors affecting their activities: a search for potent tools limiting enzymatic browning. Foods 8:154. doi: 10.3390/foods8050154
Singh, J., and Tripathi, N. N. (1999). Inhibition of storage fungi of blackgram (Vigna mungo) by some essential oils. Flavour Fragr. J. 14, 1–4. doi: 10.1002/(SICI)1099-1026(199901/02)14:1<1::AID-FFJ735>3.0.CO;2-R
Singleton, V. L., Orthofer, R., and Lamuela-Raventos, R. M. (1999). Analysis of totalphenols and other oxidation substrates and antioxidants by means of folin-ciocalteu reagent. Methods Enzymol. 299, 152–178. doi: 10.1016/S0076-6879(99)99017-1
Sowndhararajan, K., and Kang, S. C. (2013). Evaluation of in vitro free radical scavenging potential of Streptomyces sp. AM-S1 culture filtrate. Saudi J. Biol. Sci. 20, 227–233. doi: 10.1016/j.sjbs.2012.12.003
Subhasree, B., Baskar, R., Keerthana, R. L., Susan, R. L., and Rajasekaran, P. (2009). Evaluation of antioxidant potential in selected green leafy vegetables. Food Chem. 115, 1213–1220. doi: 10.1016/j.foodchem.2009.01.029
Swamy, M. K., and Sinniah, U. R. (2015). A comprehensive review on the phytochemical constituents and pharmacological activities of Pogostemon cablinBenth. An aromatic medicinal plant of industrial importance. Molecules 20, 8521–8547. doi: 10.3390/molecules20058521
Tan, B. L., Norhaizan, M. E., Liew, W. P., and Sulaiman, R. H. (2018). Antioxidant and oxidative stress: a mutual interplay in age-related diseases. Front. Pharmacol. 9:1162. doi: 10.3389/fphar.2018.01162
Tapondjou, L. A., Nyaa, L. B., Tane, P., Ricciutelli, M., Quassinti, L., Bramucci, M., et al. (2011). Cytotoxic and antioxidant triterpene saponins from Butyrospermumparkii (Sapotaceae). Carbohydr. Res. 346, 2699–2704. doi: 10.1016/j.carres.2011.09.014
Tiong, S. H., Looi, C. Y., Hazni, H., Arya, A., Paydar, M., Wong, W. F., et al. (2013). Antidiabetic and antioxidant properties of alkaloids from Catharanthus roseus (L.) G. Don. Molecules 18, 9770–9784. doi: 10.3390/molecules18089770
Trott, O., and Olson, A. J. (2010). AutoDock vina: improving the speed and accuracy of docking with a new scoring function, efficient optimization, and multithreading. J Comput Chem. 31, 455–461. doi: 10.1002/jcc.21334
van der Meer, I. M., Stam, M. E., van Tunen, A. J., Mol, J. N., and Stuitje, A. R. (1992). Antisense inhibition of flavonoid biosynthesis in petunia anthers results in male sterility. Plant Cell 4, 253–262. doi: 10.1105/tpc.4.3.253
Vertuani, S., Angusti, A., and Manfredini, S. (2004). The antioxidants and pro-antioxidants network, an overview. Curr. Pharm. Des. 10, 1677–1694. doi: 10.2174/1381612043384655
Vivek, M. N., Swamy, S. H. C., Manasa, M., Pallavi, S., Kambar, Y., Asha, M. M., et al. (2013). Antimicrobial and antioxidant activity of leaf and flower extract of caesalpinia pulcherrima, delonix regia and peltaphorum ferrugineum. J. Appl. Pharma Sci. 3, 64–71. doi: 10.7324/JAPS.2013.3811
Vladimir, K., and Ludmila, M. (2001). Glycosides in medicine: the role of glycosidic residue in biological activity. Curr. Med. Chem. 8, 1303–1328. doi: 10.2174/0929867013372193
Wardman, P. (2001). Electron transfer and oxidative stress as key factors in the design of drugs selectively active in hypoxia. Curr. Med. Chem. 8, 739–761. doi: 10.2174/0929867013372959
Wu, X., and Dhanasekaran, S. (2020). Protective effect of leaf extract of Abutilon indicum on DNA damage and peripheral blood lymphocytes in combating the oxidative stress. Saudi Pharm. J. 28, 943–950. doi: 10.1016/j.jsps.2020.06.015
Xu, C., Liu, R., Zhang, Q., Chen, X., Qian, Y., and Fang, W. (2017). The diversification of evolutionarily conserved MAPK cascades correlates with the evolution of fungal species and development of lifestyles. Genome Biol. Evol. 9, 311–322. doi: 10.1093/gbe/evw051
Yen, G. C., and Chen, H. Y. (1995). Antioxidant activity of various tea extracts in relation to their antimutagenicity. J. Agric. Food Chem. 43, 27–32. doi: 10.1021/jf00049a007
Zhao, H., Dong, J., Lu, J., Chen, J., Li, Y., Shan, Y., et al. (2006). Effects of extraction solvent mixtures on antioxidant activity evaluation and their extraction capacity and selectivity for free phenolic compounds in barley (Hordeum vulgare L.). J Agric. Food Chem. 54, 7277–7286. doi: 10.1021/jf061087w
Keywords: antioxidant, antimicrobial activity, bioactive compounds, free radicals, Lantana camara, polyphenols, plant extract, reducing power
Citation: Mansoori A, Singh N, Dubey SK, Thakur TK, Alkan N, Das SN and Kumar A (2020) Phytochemical Characterization and Assessment of Crude Extracts From Lantana camara L. for Antioxidant and Antimicrobial Activity. Front. Agron. 2:582268. doi: 10.3389/fagro.2020.582268
Received: 11 July 2020; Accepted: 05 October 2020;
Published: 12 November 2020.
Edited by:
Geert Jozej Willem Haesaert, Ghent University, BelgiumCopyright © 2020 Mansoori, Singh, Dubey, Thakur, Alkan, Das and Kumar. This is an open-access article distributed under the terms of the Creative Commons Attribution License (CC BY). The use, distribution or reproduction in other forums is permitted, provided the original author(s) and the copyright owner(s) are credited and that the original publication in this journal is cited, in accordance with accepted academic practice. No use, distribution or reproduction is permitted which does not comply with these terms.
*Correspondence: Anirudh Kumar, YW5pcnVkaC5rdW1hckBpZ250dS5hYy5pbg==
Disclaimer: All claims expressed in this article are solely those of the authors and do not necessarily represent those of their affiliated organizations, or those of the publisher, the editors and the reviewers. Any product that may be evaluated in this article or claim that may be made by its manufacturer is not guaranteed or endorsed by the publisher.
Research integrity at Frontiers
Learn more about the work of our research integrity team to safeguard the quality of each article we publish.